- 1School of Health and Rehabilitation, Chengdu University of Traditional Chinese Medicine, Chengdu, China
- 2Department of Rehabilitation, Mianyang Hospital of Traditional Chinese Medicine, Mianyang, China
- 3School of Pharmacy, Chengdu University of Traditional Chinese Medicine, Chengdu, China
Introduction: Cerebral ischaemic stroke is a common disease that poses a serious threat to human health. Butyrate is an important metabolite of intestinal microorganisms. Recent studies have shown that butyrate has a significant protective effect in animal models of cerebral ischaemic injury.
Objective: The aim of this study was to evaluate the protective effect of butyrate on cerebral ischaemic stroke by meta-analysis, aiming to provide a scientific basis for the clinical application of butyrate in patients with cerebral ischaemia.
Materials and methods: A systematic search was conducted for all relevant studies published before 23 January 2024, in PubMed, Web of Science, Cochrane Library, and Embase. Methodological quality was assessed using Syrcle’s risk of bias tool for animal studies. Data were analysed using Rev Man 5.3 software.
Results: A total of nine studies were included, and compared with controls, butyrate significantly increased BDNF levels in the brain (SMD = 2.33, 95%CI = [1.20, 3.47], p < 0.005) and P-Akt expression (SMD = 3.53, 95% CI = [0.97, 6.10], p < 0.05). Butyrate also decreased IL-β levels in the brain (SMD = −2.02, 95% CI = [−3.22, −0.81], p < 0.005), TNF-α levels (SMD = −0.86, 95% CI = [−1.60, −0.12], p < 0.05), and peripheral vascular IL-1β levels (SMD = −2.10, 95%CI = [−3.59, −0.61], p < 0.05). In addition, butyrate reduced cerebral infarct volume (MD = −11.29, 95%CI = [−17.03, −5.54], p < 0.05), mNSS score (MD = −2.86, 95%CI = [−4.12, −1.60], p < 0.005), foot fault score (MD = −7.59, 95%CI = [−9.83, −5, 35], p < 0.005), and Morris water maze time (SMD = −2.49, 95%CI = [−4.42, −0.55], p < 0.05).
Conclusion: The results of this study indicate that butyrate has a protective effect on cerebral ischaemic stroke in animal models, and the mechanism is related to reducing inflammation and inhibiting apoptosis. It provides an evidence-based basis for the future clinical development of butyrate in the treatment of ischaemic stroke.
Systematic Review Registration: https://www.crd.york.ac.uk/PROSPERO/, CRD42023482844.
1 Introduction
Stroke is a disease with high incidence and disability. Of these, ischaemic stroke (IS), which involves central nervous system infarction and a systemic inflammatory response, accounts for 71% of all strokes globally (Sacco et al., 2013; Lindsay et al., 2019). The disability-adjusted life-years (DALYs) attributable to IS are 51.9 million per year (Campbell et al., 2019), underscoring the enormous economic and social impact of the disease. A tissue plasminogen activator (tPA) is commonly used in the clinical treatment of IS to break down the clot, thereby chemically achieving rapid reperfusion (Fukuta et al., 2017). However, tPA therapy is effective in less than one-third of patients due to the strict time window requirements (Saver and Levine, 2010). Moreover, tPA only restores cerebrovascular blood flow and does not target neuronal cell damage after IS. Therefore, exploring a safe and effective treatment for IS is urgently needed.
The complex interactions between the gut microbiota and the brain have received widespread attention recently. It has been established that dysregulation of the central nervous system affects the gastrointestinal tract through the nervous, endocrine, and immune systems (O'Mahony et al., 2015). IS can lead to abnormalities in the composition and function of the gut microbiota (Li et al., 2019). For example, recent studies have found that IS affects gut microbial composition and abundance in mice by stimulating the release of norepinephrine from the autonomic nervous system and altering the number of intestinal mucin-producing cuprocytes in mouse models (Huang et al., 2021). In addition, in a porcine model, a significant decrease in the diversity of gut microbes occurred after IS, with the extent of the reduction correlating with the severity of IS (Jeon et al., 2020). Among the changes in phyla, the gut microbiota mainly showed an increase in the phylum Anabaena and a decrease in the phylum Ascomycota (Benakis et al., 2016). At the same time, disordered gut microbes and their metabolites have an essential impact on the pathogenesis of IS (Xia et al., 2019). The study suggests gut microbes can ameliorate post-IS inflammation via T cells (Malkki, 2016; Ridler, 2016). Short-chain fatty acids (SCFAs), a metabolite of gut microbes, also play an essential role in IS. One study found that acetic acid concentrations were significantly lower and valeric acid concentrations were significantly higher in IS patients than in controls. However, total organic acid concentrations were lower in stroke patients (Yamashiro et al., 2017). Butyrate is a particularly crucial signalling molecule among the SCFAs. Chen et al. found that SCFAs were low in IS mice, especially butyrate (Chen et al., 2019). In humans, butyrate is produced from dietary fibre by bacterial fermentation. It is rapidly absorbed by colonic cells by passive non-ionic diffusion or active carrier transport (Nedjadi et al., 2014). It is metabolised mainly in intestinal epithelial cells, hepatocytes, and other tissue cells (Wong et al., 2006). It is of widespread interest because of its regulation of maintaining epithelial integrity. Many animal experiments have reported the neuroprotective effects of butyrate on IS. However, no relevant clinical investigations have been reported. Therefore, this study aimed to systematically analyse these animal experiments to evaluate therapeutic effects of butyrate and provide adequate evidence-based medical support for the clinical application of butyrate in treating IS.
2 Materials and methods
A systematic review and meta-analysis of animal studies was performed according to PRISMA guidelines (Tam et al., 2019). The programme is registered in the International Prospective Systematic Evaluation Register (PROSPERO) (registration number CRD42023482844).
2.1 Search strategy
Two investigators independently searched PubMed, Web of Science, Cochrane Library, and Embase to collect experimental data on the therapeutic effects of butyrate in an animal model of cerebral ischaemic injury published on 23 January 2024 and disputed by a third investigator. The subject terms “butyrate” or “butyric acid” and “brain infarction,” “brain ischemia,” “cerebral thrombosis,” “cerebral embolism,” or “middle cerebral artery.” or “middle cerebral artery occlusion,” or “Stoke.”
2.2 Inclusion and exclusion criteria
The inclusion criteria were pre-specified as follows: (1) the type of study was an animal RCT experiment; (2) animals were used as the study subjects, and a cerebral ischaemia model was established; (3) the experimental group was given butyrate intervention; (4) the main regression indexes were inflammatory factors, BDNF, P-Akt, cerebral infarct volume, and the Neurological Scale Score.
The exclusion criteria were predetermined as follows: (1) non-RCT experiments; (2) non-animal models of cerebral ischaemia; (3) non-butyrate treatments or combinations of butyrate and other treatments; (4) reviews, conferences, and clinical trials; (5) studies with incomplete data, including unpublished data; (6) repetitively published studies; and (7) non-English literature.
2.3 Data extraction
Two researchers independently screened the literature using Endnote software. In case of disagreement, a third researcher resolved the dispute. The main contents of data extraction were as follows: (1) General information, including the title of the study, the name of the first author, the year of publication, the type of animal, weight, and model. (2) Interventions, including drug name, dose, mode, and administration time. (3) Outcome-related indicators and data. (4) For the literature that reported data only in images, we extracted the data from the images by using GetData Graph Digitizer software.
2.4 Quality assessment
The risk of bias tool from SYRCLE was used to judge each entry as low risk, high risk, and unclear based on appropriate criteria and to create a risk of bias map (Hooijmans et al., 2014).
2.5 Data analysis
Data were analysed using Rev Man 5.3 software. Continuous variables were used to calculate their 95% confidence intervals (95% CI) using weighted mean difference (WMD) or standardised mean difference (SMD). Depending on the heterogeneity test, it was treated with a fixed-effects model or a random-effects model. A fixed-effects model was used when the heterogeneity test results of the included studies were p > 0.05 and I2 < 50%; a random-effects model was used when p < 0.05 and I2 ≥ 50%. Sensitivity analysis was used to explore the causes of heterogeneity. Funnel plots were used to assess potential publication bias.
3 Results
3.1 Research data
A total of 116 relevant studies were searched. According to the inclusion criteria, 23 duplicates were excluded, and 74 studies that did not meet the requirements of experimental subjects, interventions, and experimental methods were deleted by reviewing study titles and abstracts. In total, 19 articles were reassessed through full-text reading. Of these, valid data were not available for 10 articles. Finally, nine studies were included for meta-analysis (Figure 1).
3.2 Basic research information
A total of nine studies were included in this study. The five (Kim et al., 2007; Park and Sohrabji, 2016; Tu et al., 2017; Chen et al., 2019; Zhou et al., 2021) studies were SD rats with a body weight range of 220–360 g. In total, four (Kim et al., 2007; Tu et al., 2017; Chen et al., 2019; Zhou et al., 2021) studies used animals that were males and one (Park and Sohrabji, 2016) study used animal that was a female; the animals used in two (Chen et al., 2023; Li et al., 2023) studies were male C57BL/6 mice with a range of body weights that were not reported; the one (Sun et al., 2015) study used animals that were male ICR mice with a weight range of 22–24 g; the animals used in 1 (Wang et al., 2021) study were male Dd mice, and the body weights of the mice were not reported; nine animal models of occluded middle cerebral arteries were used, including seven MCAO models, one photothrombotic stroke model, and one cerebral I/R injury model. Of the nine studies regarding the control modality, saline control intervention was used in 6 studies, Nacl control intervention was used in 1 study, and 2 studies were not reported. Regarding the mode of butyrate administration, drinking water was used in 2 studies, force-feeding was used in 2 studies, intraperitoneal injection was used in 3 studies, nasal delivery was used in 1 study, and 1 study was unspecified. The specific essential characteristics of the included studies are shown in Table 1.
3.3 Analysis of quality evaluation methods
One study explicitly used the random number table grouping method, and the remaining studies mentioned only the term randomised grouping. All analyses were adjusted for baseline characteristics. None of the studies mentioned allocation concealment; two studies mentioned placement randomisation, and 1 study indicated blinding. The study-specific risk of bias plots for the included studies are shown in Figure 2. Some of the data from seven studies were extracted from the images by GetData Graph Digitizer software and may be biased.
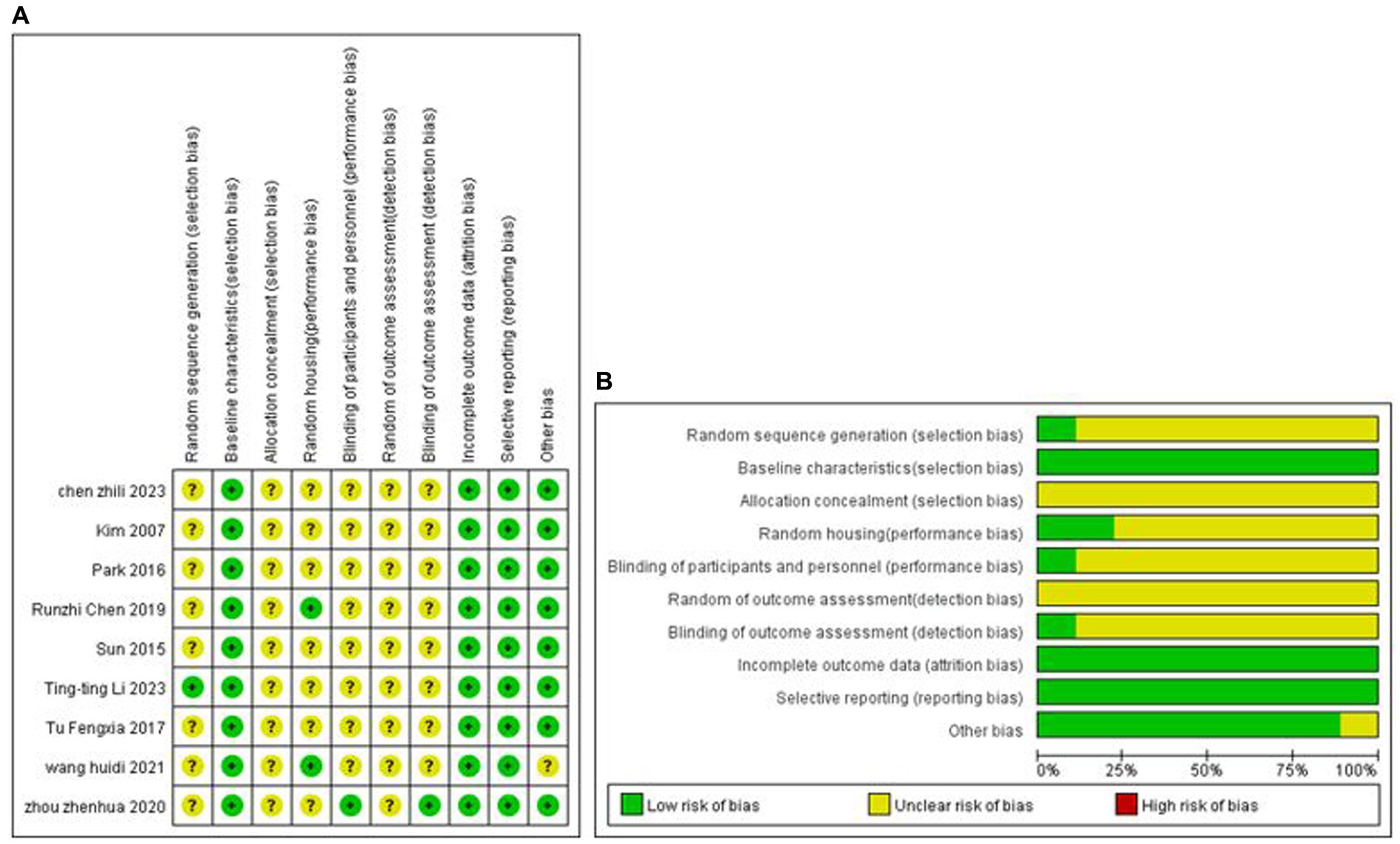
Figure 2. Methodological quality assessment of the risk of bias. (A) Risk of bias summary: review authors’ judgment of each risk of bias item for each included study. (B) Risk of bias plot: Review authors’ judgment for each risk of bias item, expressed as a percentage across all included studies.
3.4 Volume analysis of cerebral infarction
A total of 5 (Chen et al., 2019; Wang et al., 2021; Zhou et al., 2021;Chen et al., 2023; Li et al., 2023) studies reported the percentage of cerebral infarction volume. Heterogeneity was considerable across studies (p < 0.00001, I2 = 88%), and a random-effects model was used. The results showed that butyrate reduced cerebral infarct volume (MD = −11.29, 95% CI = [−17.03, −5.54], p < 0.05). The forest plot is shown in Figure 3.
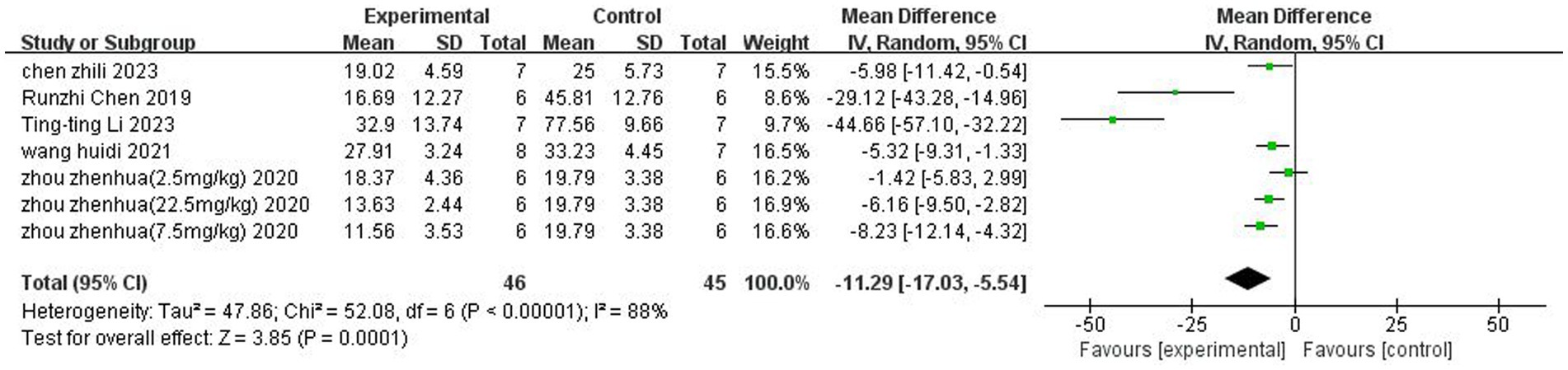
Figure 3. Forest plot: Reduction of cerebral infarct volume by butyrate compared with the control group.
3.5 Neurological function score analysis
3.5.1 The mNSS neurological function rating scale
A total of two studies (Chen et al., 2023; Li et al., 2023) reported mNSS neurologic function ratings. There was no heterogeneity across studies (p = 0.37, I2 = 0%), and a fixed-effects model was used. The results showed that butyrate reduced mNSS scores after IS (MD = −2.86, 95%CI = [−4.12, −1.60], p < 0.005). The forest plot is shown in Figure 4.

Figure 4. Forest plot: Effect of butyrate on reduction of mNSS score compared with the control group.
3.5.2 Foot fault test
A total of three (Zhou et al., 2021; Chen et al., 2023; Li et al., 2023) studies reported the foot fault test. There was no heterogeneity across studies (p = 0.43, I2 = 0%), and a fixed-effects model was used. The results showed that butyrate reduced foot fault scores after IS (MD = −7.59, 95%CI = [−9.83, −5, 35], p < 0.005). The forest plot is shown in Figure 5.

Figure 5. Forest plot: Effect of butyrate on reduction of foot fault score as compared to the control group.
3.6 Morris water maze analysis
A total of three (Tu et al., 2017; Zhou et al., 2021; Li et al., 2023) studies reported Morris water maze. Heterogeneity was considerable across studies (p < 0.05, I2 = 83%), and a random-effects model was used. The results showed that butyrate reduced the time to the Morris water maze after IS (SMD = −2.49, 95%CI = [−4.42, −0.55], p < 0.05). The forest plot is shown in Figure 6.

Figure 6. Forest plot: Effect of butyrate on reducing water maze time compared to the control group.
3.7 Analysis of inflammatory factors in the brain
3.7.1 IL-1β
A total of three (Sun et al., 2015; Park and Sohrabji, 2016; Li et al., 2023) studies reported IL-1β levels in the brain. Heterogeneity across studies was large (p = 0.04, I2 = 64%), and a random-effects model was used. The results showed that butyrate reduced IL-β content in the brain (SMD = −2.02, 95% CI = [−3.22, −0.81], p < 0.005). The forest plot is shown in Figure 7.

Figure 7. Forest plot: Effect of butyrate on lowering IL-1β in the brain compared to the control group.
3.7.2 TNF-α
A total of two (Sun et al., 2015; Li et al., 2023) studies reported TNF-α levels in the brain. Heterogeneity across studies was small (p = 0.22, I2 = 34%), and a fixed-effects model was used. The results showed that butyrate reduced TNF-α content in the brain (SMD = −0.86, 95% CI = [−1.60, −0.12], p < 0.05). The forest plot is shown in Figure 8.

Figure 8. Forest plot: Effect of butyrate on reducing TNF-α in the brain compared to the control group.
3.8 Analysis of inflammatory factor IL-1β in peripheral blood
A total of three (Park and Sohrabji, 2016; Wang et al., 2021; Li et al., 2023) studies reported IL-1β levels in the peripheral vasculature. There was significant heterogeneity among the studies (p = 0.09, I2 = 59%), and a random-effects model was used. The results showed that butyrate reduced IL-1β levels in the peripheral vasculature (SMD = −2.10, 95% CI = [−3.59, −0.61], p < 0.05). The forest plot is shown in Figure 9.

Figure 9. Forest plot: Effect of butyrate on lowering IL-1β in peripheral blood compared to the control group.
3.9 BDNF analysis
A total of two (Sun et al., 2015; Li et al., 2023) studies reported BDNF content. There was no heterogeneity across studies (p = 0.99, I2 = 0%), and a fixed-effects model was used. The results showed that butyrate increased BDNF content in the brain after IS (SMD = 2.33, 95% CI = [1.20, 3.47], p < 0.005). The forest plot is shown in Figure 10.
3.10 P-Akt analysis
A total of three (Kim et al., 2007; Sun et al., 2015; Zhou et al., 2021) studies reported P-Akt expression. There was heterogeneity across studies (p = 0.001, I2 = 86%), and a random-effects model was used. The results showed that butyrate increased P-Akt expression after IS (SMD = 3.53 95%CI = [0.97, 6.10], p < 0.05). The forest plot is shown in Figure 11.
3.11 Sensitivity analysis
3.11.1 Cerebral infarction volume
Due to the significant heterogeneity of this study, sensitivity analysis was used. After excluding (Li et al., 2023) and (Chen et al., 2019), I2 decreased from 92 to 25%. After careful analysis of each study, it was found that the butyrate treatment cycles of 14 and 28 days were much more significant in the two studies than in the other studies, which shows that different butyrate treatment cycles may produce heterogeneity. However, the results also support that butyrate effectively reduces cerebral infarction volume after IS.
3.11.2 Morris water maze
Sensitivity analysis was used because of the significant heterogeneity of this study. Excluding any of the studies that did not affect heterogeneity, the results were more stable. The results support that butyrate reduces Morris water maze time after IS.
3.11.3 IL-1β in the brain
Due to the significant heterogeneity of this study, sensitivity analysis was used. After excluding the risky Park (Park and Sohrabji, 2016), I2 decreased from 64 to 26%. After careful analysis of each study, the animals in this study were female rats, while all other studies were male rats. It can be seen that choosing different sexes of animals may produce heterogeneity. However, the results also support that butyrate effectively reduces IL-1β in the brain after IS.
3.11.4 IL-1β in peripheral blood
Due to the significant heterogeneity of this study, sensitivity analysis was used. I2 decreased from 59 to 0% after excluding the riskier Park (Park and Sohrabji, 2016). After careful analysis of each study, the detection time in this study was the 7th day after modelling, whereas, in the other two studies, the detection was performed within 2 days. It can be seen that different testing times may produce heterogeneity. However, the results also support that butyrate effectively reduces IL-1β in peripheral blood after IS.
3.11.5 P-Akt
Sensitivity analysis was used because of the significant heterogeneity of this study. Excluding any of the studies did not affect the heterogeneity, and the results were more stable. The results support that butyrate can increase P-Akt expression after IS.
3.12 Subgroup analysis
The improvement of IS was analysed separately for different concentrations of the strong-feeding method and nasal injection according to the butyrate administration method. The subgroup analysis of the strong-feeding method is shown in Figure 12, and the concentration of 10 mg/kg was better than 5 mg/kg in reducing IL-1β in the brain after IS. The subgroup analysis of the nasal injection is shown in Figure 13, and the concentration of 7.5 mg/kg was better than 22.5 mg/kg in reducing the area of cerebral infarcts after IS, which was superior to that of 2.5 mg/kg.
3.13 Publication bias
The analysis of bias for the outcome indicator of cerebral infarction volume included five studies (seven data sets). As shown in Figure 14, the asymmetry on both sides of the funnel plot indicates the presence of publication bias. Egger’s test results indicated that t = 0.02 < 0.05, indicating the presence of publication bias.
4 Discussion
This study is the first systematic review of the neuroprotective effects of butyrate in an animal model of IS. We comprehensively evaluated the neuroprotective effects of butyrate in terms of inflammatory factors, P-Akt, BDNF, cerebral infarct volume, and neurological function scores. We found that butyrate could exert neuroprotective effects in IS animal models by suppressing intracerebral and peripheral inflammatory responses, inhibiting apoptosis, and increasing neurotrophic factors in the brain.
IS is caused by an interruption in the blood supply to an area of the brain. This produces an area of hypoxia and glucose deprivation. Dead cells in this region release various danger-associated molecular patterns, DAMPs, which stimulate the production of inflammatory cytokines and chemokines. Microglia in the brain are encouraged to rapidly activate and polarise into the M1 subtype, which elicits an inflammatory cascade response and secretes various inflammatory factors and chemokines, such as TNFα and IL-1β. It has been shown that IL-1β is rapidly upregulated in the brain in response to IS (Nguyen et al., 2016). IL-1β exerts neurotoxic effects in IS, and blocking its products has been shown to reduce IS neurological damage (Weber et al., 2010). TNF-α is expressed at low levels in the healthy CNS. However, after the occurrence of IS, it is increased with its two known receptors, TNFR1 and TNFR (Nguyen et al., 2016). Lowering their levels is an effective treatment for IS (Liguz-Lecznar et al., 2015). However, in peripheral blood, changes in inflammatory factors are currently controversial. Some studies have indicated increased levels of IL-1β in the blood of IS patients compared to controls (Sotgiu et al., 2006; Tuttolomondo et al., 2009; Wytrykowska et al., 2016), but others have reported no change in IL-1β levels in the blood of IS patients (Emsley et al., 2007; Ormstad et al., 2011). As most of these studies showing increased IL-1β were tested within 72 h, the difference in results may be due to differences in the timing of blood draws. In recent years, it has been found that IS also causes damage to the intestinal mucosa (Zhang et al., 2021). Damaged intestinal mucosa leads to the entry of the bacterial product of the gut, lipopolysaccharide (LPS), into the circulatory system (Wang et al., 2022), which activates the LPS/TLR4/NF-κB pathway leading to increased levels of IL-1β in the blood. In conclusion, both cytokines have a role in ischaemia-induced neuronal injury preceded by an early inflammatory response that exacerbates nerve damage (Basu et al., 2005). Thus, IL-1β and TNF-α play critical roles in the pathogenesis of IS and are potential targets for IS therapy.
In the present study, we showed by analysis that butyrate can effectively attenuate the levels of TNFα and IL-1β in the brain and the level of IL-1β in the blood of IS animals. Within the brain, butyrate inhibited microglia activation and reduced the secretion of inflammatory factors in rats (Park and Sohrabji, 2016). Li et al. (2023) found that butyrate regulated microglia phenotype through Treg cells, thereby reducing TNFαIL-1β levels. In addition, in SCFA, butyrate was the most effective in inhibiting the activity of HDAC (Turner and Lupton, 2011; Flint et al., 2012). Butyrate inhibits HDAC recruitment to the promoter via transcription factor-specific protein 1/specific protein 3 (Sp1/Sp3), leading to histone hyperacetylation. Many of the anticancer activities of butyrate are mediated by the inhibition of HDAC, including cell proliferation, cell differentiation or apoptosis, and induction or inhibition of gene expression (Donohoe et al., 2012). In addition to its role as an antitumor agent, butyrate partially achieves its anti-inflammatory effects by inhibiting HDAC (Park et al., 2015). For example, butyrate reduced neuroinflammation caused by microglia in IS mice by inhibiting HDAC, thereby altering the gene initiation of histone-3-lysine 9-acetylation (H3K9ac) in microglia (Patnala et al., 2017). In conclusion, butyrate modulates microglia in the brain to attenuate neuroinflammation in IS through multiple mechanisms. However, the anti-inflammatory effects of butyrate in the blood are more complex. On the one hand, peripheral immune changes in the blood are mainly alterations in acquired immunity, manifested as changes in T-cell subsets. Butyrate can regulate peripheral immunity through Tregs/TGF-β/T cells, which significantly increases the ratio of CD4+/CD8+ T cells, thus improving peripheral inflammation (Li et al., 2023). On the other hand, butyrate could repair and enhance the function of the intestinal epithelial barrier, thereby reducing the inflammatory response induced by LPS entering the circulation of the body (Huang et al., 2015). MUC2 protein produced by cuprocytes in the intestine is the most prominent protein in the intestinal mucosal layer and can strengthen it (Canani et al., 2011). Zhang et al. (2021) found that the intestinal MUC2 mRNA transcript level was significantly reduced after brain injury. Butyrate can increase the expression of MUC2 protein and enhance the protection of the intestinal barrier of Caco-2 monolayer cells (Elamin et al., 2013). Butyrate also increases the expression of trefoil factor (TFF) (a mucin-related peptide), which maintains and repairs the intestinal mucosa (Hamer et al., 2008; Leonel and Alvarez-Leite, 2012). In addition, butyrate regulates the expression of compact proteins and reduces paracellular permeability (Matter et al., 2005; Meijer et al., 2010).
In the acute phase of IS, neuronal apoptosis is reversible in the ischaemic hemi-dark band. Therefore, salvaging the ischaemic semi-dark bar is the most effective treatment for IS. The phosphatidylinositol 3-kinase/protein kinase B (PI3K/Akt) pathway is critical for neuronal cell survival after IS (Kilic et al., 2017). In this study, we demonstrated by analysis that butyrate can effectively increase P-Akt expression, thereby reducing nerve damage. In addition, the present study showed that butyrate reduced cerebral infarct volume and mNSS, Foot fault score, decreased Morris water maze time, and increased BDNF content. Therefore, the present study reveals to some extent that butyrate can protect the animal brain from IS damage, as in Figure 15, and improve the neurological function of animals.
It has been shown that butyrate can also ameliorate neurological injury in IS through other mechanisms. IL-22 can positively regulate angiogenesis by binding to the IL-22R receptor on the surface of endothelial cells (Hu et al., 2018). Butyrate can reduce neuronal apoptosis by inducing vascular remodelling through IL-22 in IS-aged mice to attenuate nerve injury (Chen et al., 2023). In addition, after the onset of IS, apoptosis, neuroinflammation, and altered expression of TJ-related proteins cause damage to the blood–brain barrier (BBB) (Khatri et al., 2012), exacerbating the impairment of neurological function. A recent study found that the endothelial glycocalyx layer (the first line of defence to protect the BBB) was relatively thicker in the brains of butyrate-treated IS mice than distilled water (Wang et al., 2021). Wang et al. (2021) also found that the level of matrix metalloproteinase-9 (MMP-9) was significantly reduced in the brains of butyrate-treated stroke mice. In brain tissue, MMP-9 plays a crucial role in the BBB. In response to inflammatory factors, MMP-9 can be activated and secreted, leading to proteolytic damage of extracellular matrix components and degradation of basement membrane and TJ proteins (Zhu et al., 2018; Qin et al., 2019). Both in vivo and in vitro data suggest that low expression or downregulation of MMP-9 activity restores the BBB and increases TJ protein expression (Yang et al., 2007; Zhu et al., 2018; Qin et al., 2019). Thus, butyrate could alleviate the nerve damage in IS by repairing and improving the BBB. However, the pathophysiologic mechanisms of IS are complex, with overlapping and interacting links. The protective effect of butyrate on IS needs further study.
Subgroup analyses in the present study revealed differences in the neuroimproving effects of different butyrate concentrations on IS. Previous in vitro studies have shown that low concentrations (≤2 mm) of butyrate promote intestinal barrier function, but high concentrations (5 or 8 mm) of butyrate may be disruptive (Peng et al., 2009). Currently, the commonly used dose for rectal administration in clinical practice is 100 mm butyrate which is comparable to the physiological concentration in the human colon after consumption of a high-fibre diet (Wang et al., 2012). Wang et al. (2021) found that 100 mm of butyrate increased the concentration of butyrate in the faeces of IS mice and was influential in improving neurological function after IS. However, the most effective butyrate concentration for treating IS in clinical trials is still worth exploring.
Limitations of this systematic evaluation are listed as follows: (1) The included studies measured a wide range of neuroimmune responses. However, the few studies measuring these outcomes precluded a meta-analysis of most neuroimmune responses. (2) There was significant heterogeneity among the included studies. Different modelling methods, as well as animal breeds, were used in the studies, and in addition. However, butyrate was used in all intervention groups; there were differences in butyrate concentration, dose, and mode of administration. (3) Only one of the nine studies involved female animals, and the small number of studies did not allow for reliable subgroup analyses of sex. (4) The bias risk assessment showed that most of the bias risk criteria in the included studies were rated as unclear. This made it difficult to determine differences in results due to source bias. Future animal studies should follow the ARRIVE Animal Analysis Reporting Guidelines to ensure greater clarity in all methods (Kilkenny et al., 2011).
5 Conclusion
In conclusion, this review reveals that butyrate has a wide range of positive modulatory effects on neuroinflammation in animal models of IS and provides a relevant evidence-based basis for the future development of the clinical application of butyrate in treating IS. However, given that the effects of butyrate are affected by concentration, there is no literature on the optimal concentration range for IS, and it is suggested that future studies should fill this gap. In addition, the inclusion of literature in this review was slight and subject to publication bias, so further studies are needed.
Data availability statement
The original contributions presented in the study are included in the article/supplementary material, further inquiries can be directed to the corresponding author.
Author contributions
SY: Data curation, Software, Writing – original draft, Writing – review & editing. QJ: Writing – original draft, Writing – review & editing. JD: Writing – original draft, Writing – review & editing. ZL: Writing – review & editing. WW: Writing – review & editing. HL: Writing – review & editing. LL: Writing – review & editing, Data curation, Supervision. CM: Data curation, Supervision, Writing – review & editing. DL: Data curation, Supervision, Writing – review & editing. ZH: Data curation, Supervision, Writing – review & editing. SA: Conceptualization, Writing – review & editing.
Funding
The author(s) declare financial support was received for the research, authorship, and/or publication of this article. This work was supported by the Sichuan Provincial Department of Science and Technology (2023YFS0337 and 2023NSFSCI827).
Conflict of interest
The authors declare that the research was conducted in the absence of any commercial or financial relationships that could be construed as a potential conflict of interest.
Publisher’s note
All claims expressed in this article are solely those of the authors and do not necessarily represent those of their affiliated organizations, or those of the publisher, the editors and the reviewers. Any product that may be evaluated in this article, or claim that may be made by its manufacturer, is not guaranteed or endorsed by the publisher.
References
Basu, A., Lazovic, J., Krady, J. K., Mauger, D. T., Rothstein, R. P., Smith, M. B., et al. (2005). Interleukin-1 and the interleukin-1 type 1 receptor are essential for the progressive neurodegeneration that ensues subsequent to a mild hypoxic/ischemic injury. J. Cereb. Blood Flow Metab. 25, 17–29. doi: 10.1038/sj.jcbfm.9600002
Benakis, C., Brea, D., Caballero, S., Faraco, G., Moore, J., Murphy, M., et al. (2016). Commensal microbiota affects ischemic stroke outcome by regulating intestinal gammadelta T cells. Nat. Med. 22, 516–523. doi: 10.1038/nm.4068
Campbell, B. C. V., De Silva, D. A., Macleod, M. R., Coutts, S. B., Schwamm, L. H., Davis, S. M., et al. (2019). Ischaemic stroke. Nat. Rev. Dis. Primers 5:70. doi: 10.1038/s41572-019-0118-8
Canani, R. B., Costanzo, M. D., Leone, L., Pedata, M., Meli, R., and Calignano, A. (2011). Potential beneficial effects of butyrate in intestinal and extraintestinal diseases. World J. Gastroenterol. 17, 1519–1528. doi: 10.3748/wjg.v17.i12.1519
Chen, Z., Xin, L., Yang, L., Xu, M., Li, F., Zhou, M., et al. (2023). Butyrate promotes post-stroke outcomes in aged mice via interleukin-22. Exp. Neurol. 363:114351. doi: 10.1016/j.expneurol.2023.114351
Chen, R., Xu, Y., Wu, P., Zhou, H., Lasanajak, Y., Fang, Y., et al. (2019). Transplantation of fecal microbiota rich in short chain fatty acids and butyric acid treat cerebral ischemic stroke by regulating gut microbiota. Pharmacol. Res. 148:104403. doi: 10.1016/j.phrs.2019.104403
Donohoe, D. R., Collins, L. B., Wali, A., Bigler, R., Sun, W., and Bultman, S. J. (2012). The Warburg effect dictates the mechanism of butyrate-mediated histone acetylation and cell proliferation. Mol. Cell 48, 612–626. doi: 10.1016/j.molcel.2012.08.033
Elamin, E. E., Masclee, A. A., Dekker, J., Pieters, H. J., and Jonkers, D. M. (2013). Short-chain fatty acids activate AMP-activated protein kinase and ameliorate ethanol-induced intestinal barrier dysfunction in Caco-2 cell monolayers. J. Nutr. 143, 1872–1881. doi: 10.3945/jn.113.179549
Emsley, H. C., Smith, C. J., Gavin, C. M., Georgiou, R. F., Vail, A., Barberan, E. M., et al. (2007). Clinical outcome following acute ischaemic stroke relates to both activation and autoregulatory inhibition of cytokine production. BMC Neurol. 7:5. doi: 10.1186/1471-2377-7-5
Flint, H. J., Scott, K. P., Louis, P., and Duncan, S. H. (2012). The role of the gut microbiota in nutrition and health. Nat. Rev. Gastroenterol. Hepatol. 9, 577–589. doi: 10.1038/nrgastro.2012.156
Fukuta, T., Asai, T., Yanagida, Y., Namba, M., Koide, H., Shimizu, K., et al. (2017). Combination therapy with liposomal neuroprotectants and tissue plasminogen activator for treatment of ischemic stroke. FASEB J. 31, 1879–1890. doi: 10.1096/fj.201601209R
Hamer, H. M., Jonkers, D., Venema, K., Vanhoutvin, S., Troost, F. J., and Brummer, R. J. (2008). Review article: the role of butyrate on colonic function. Aliment. Pharmacol. Ther. 27, 104–119. doi: 10.1111/j.1365-2036.2007.03562.x
Hooijmans, C. R., Rovers, M. M., de Vries, R. B., Leenaars, M., Ritskes-Hoitinga, M., and Langendam, M. W. (2014). SYRCLE's risk of bias tool for animal studies. BMC Med. Res. Methodol. 14:43. doi: 10.1186/1471-2288-14-43
Hu, H., Li, L., Yu, T., Li, Y., and Tang, Y. (2018). Interleukin-22 receptor 1 upregulation and activation in hypoxic endothelial cells improves perfusion recovery in experimental peripheral arterial disease. Biochem. Biophys. Res. Commun. 505, 60–66. doi: 10.1016/j.bbrc.2018.08.163
Huang, J. T., Mao, Y. Q., Han, B., Zhang, Z. Y., Chen, H. L., Li, Z. M., et al. (2021). Calorie restriction conferred improvement effect on long-term rehabilitation of ischemic stroke via gut microbiota. Pharmacol. Res. 170:105726. doi: 10.1016/j.phrs.2021.105726
Huang, C., Song, P., Fan, P., Hou, C., Thacker, P., and Ma, X. (2015). Dietary sodium butyrate decreases postweaning diarrhea by modulating intestinal permeability and changing the bacterial communities in weaned piglets. J. Nutr. 145, 2774–2780. doi: 10.3945/jn.115.217406
Jeon, J., Lourenco, J., Kaiser, E. E., Waters, E. S., Scheulin, K. M., Fang, X., et al. (2020). Dynamic changes in the gut microbiome at the acute stage of ischemic stroke in a pig model. Front. Neurosci. 14:587986. doi: 10.3389/fnins.2020.587986
Khatri, R., McKinney, A. M., Swenson, B., and Janardhan, V. (2012). Blood-brain barrier, reperfusion injury, and hemorrhagic transformation in acute ischemic stroke. Neurology 79, S52–S57. doi: 10.1212/WNL.0b013e3182697e70
Kilic, U., Caglayan, A. B., Beker, M. C., Gunal, M. Y., Caglayan, B., Yalcin, E., et al. (2017). Particular phosphorylation of PI3K/Akt on Thr308 via PDK-1 and PTEN mediates melatonin's neuroprotective activity after focal cerebral ischemia in mice. Redox Biol. 12, 657–665. doi: 10.1016/j.redox.2017.04.006
Kilkenny, C., Browne, W., Cuthill, I. C., Emerson, M., and Altman, D. G.National Centre for the Replacement, Refinement and Reduction of Amimals in Research, et al. (2011). Animal research: reporting in vivo experiments--the ARRIVE guidelines. J. Cereb. Blood Flow Metab. 31, 991–993. doi: 10.1038/jcbfm.2010.220
Kim, H. J., Rowe, M., Ren, M., Hong, J. S., Chen, P. S., and Chuang, D. M. (2007). Histone deacetylase inhibitors exhibit anti-inflammatory and neuroprotective effects in a rat permanent ischemic model of stroke: multiple mechanisms of action. J. Pharmacol. Exp. Ther. 321, 892–901. doi: 10.1124/jpet.107.120188
Leonel, A. J., and Alvarez-Leite, J. I. (2012). Butyrate: implications for intestinal function. Curr. Opin. Clin. Nutr. Metab. Care 15, 474–479. doi: 10.1097/MCO.0b013e32835665fa
Li, N., Wang, X., Sun, C., Wu, X., Lu, M., Si, Y., et al. (2019). Change of intestinal microbiota in cerebral ischemic stroke patients. BMC Microbiol. 19:191. doi: 10.1186/s12866-019-1552-1
Li, T. T., Zhao, D. M., Wei, Y. T., Li, J. B., Li, X. F., Wan, Q., et al. (2023). Effect and mechanism of sodium butyrate on neuronal recovery and prognosis in diabetic stroke. J. Neuroimmune Pharmacol. 18, 366–382. doi: 10.1007/s11481-023-10071-0
Liguz-Lecznar, M., Zakrzewska, R., and Kossut, M. (2015). Inhibition of Tnf-alpha R1 signaling can rescue functional cortical plasticity impaired in early post-stroke period. Neurobiol. Aging 36, 2877–2884. doi: 10.1016/j.neurobiolaging.2015.06.015
Lindsay, M. P., Norrving, B., Sacco, R. L., Brainin, M., Hacke, W., Martins, S., et al. (2019). World stroke organization (WSO): global stroke fact sheet 2019. Int. J. Stroke 14, 806–817. doi: 10.1177/1747493019881353
Malkki, H. (2016). Stroke: gut microbiota influence stroke recovery in mice. Nat. Rev. Neurol. 12:252. doi: 10.1038/nrneurol.2016.52
Matter, K., Aijaz, S., Tsapara, A., and Balda, M. S. (2005). Mammalian tight junctions in the regulation of epithelial differentiation and proliferation. Curr. Opin. Cell Biol. 17, 453–458. doi: 10.1016/j.ceb.2005.08.003
Meijer, K., de Vos, P., and Priebe, M. G. (2010). Butyrate and other short-chain fatty acids as modulators of immunity: what relevance for health? Curr. Opin. Clin. Nutr. Metab. Care 13, 715–721. doi: 10.1097/MCO.0b013e32833eebe5
Nedjadi, T., Moran, A. W., Al-Rammahi, M. A., and Shirazi-Beechey, S. P. (2014). Characterization of butyrate transport across the luminal membranes of equine large intestine. Exp. Physiol. 99, 1335–1347. doi: 10.1113/expphysiol.2014.077982
Nguyen, T. V., Frye, J. B., Zbesko, J. C., Stepanovic, K., Hayes, M., Urzua, A., et al. (2016). Multiplex immunoassay characterization and species comparison of inflammation in acute and non-acute ischemic infarcts in human and mouse brain tissue. Acta Neuropathol. Commun. 4:100. doi: 10.1186/s40478-016-0371-y
O'Mahony, S. M., Clarke, G., Borre, Y. E., Dinan, T. G., and Cryan, J. F. (2015). Serotonin, tryptophan metabolism and the brain-gut-microbiome axis. Behav. Brain Res. 277, 32–48. doi: 10.1016/j.bbr.2014.07.027
Ormstad, H., Aass, H. C., Lund-Sorensen, N., Amthor, K. F., and Sandvik, L. (2011). Serum levels of cytokines and C-reactive protein in acute ischemic stroke patients, and their relationship to stroke lateralization, type, and infarct volume. J. Neurol. 258, 677–685. doi: 10.1007/s00415-011-6006-0
Park, J., Kim, M., Kang, S. G., Jannasch, A. H., Cooper, B., Patterson, J., et al. (2015). Short-chain fatty acids induce both effector and regulatory T cells by suppression of histone deacetylases and regulation of the mTOR-S6K pathway. Mucosal Immunol. 8, 80–93. doi: 10.1038/mi.2014.44
Park, M. J., and Sohrabji, F. (2016). The histone deacetylase inhibitor, sodium butyrate, exhibits neuroprotective effects for ischemic stroke in middle-aged female rats. J. Neuroinflammation 13:300. doi: 10.1186/s12974-016-0765-6
Patnala, R., Arumugam, T. V., Gupta, N., and Dheen, S. T. (2017). HDAC inhibitor sodium butyrate-mediated epigenetic regulation enhances neuroprotective function of microglia during ischemic stroke. Mol. Neurobiol. 54, 6391–6411. doi: 10.1007/s12035-016-0149-z
Peng, L., Li, Z. R., Green, R. S., Holzman, I. R., and Lin, J. (2009). Butyrate enhances the intestinal barrier by facilitating tight junction assembly via activation of AMP-activated protein kinase in Caco-2 cell monolayers. J. Nutr. 139, 1619–1625. doi: 10.3945/jn.109.104638
Qin, W., Li, J., Zhu, R., Gao, S., Fan, J., Xia, M., et al. (2019). Melatonin protects blood-brain barrier integrity and permeability by inhibiting matrix metalloproteinase-9 via the NOTCH3/NF-kappaB pathway. Aging 11, 11391–11415. doi: 10.18632/aging.102537
Ridler, C. (2016). Gut microbiota: gut bacteria affect post-ischaemic inflammation in stroke by modulating intestinal T cells. Nat. Rev. Gastroenterol. Hepatol. 13:250. doi: 10.1038/nrgastro.2016.64
Sacco, R. L., Kasner, S. E., Broderick, J. P., Caplan, L. R., Connors, J. J., Culebras, A., et al. (2013). An updated definition of stroke for the 21st century: a statement for healthcare professionals from the American Heart Association/American Stroke Association. Stroke 44, 2064–2089. doi: 10.1161/STR.0b013e318296aeca
Saver, J. L., and Levine, S. R. (2010). Alteplase for ischaemic stroke--much sooner is much better. Lancet 375, 1667–1668. doi: 10.1016/S0140-6736(10)60634-4
Sotgiu, S., Zanda, B., Marchetti, B., Fois, M. L., Arru, G., Pes, G. M., et al. (2006). Inflammatory biomarkers in blood of patients with acute brain ischemia. Eur. J. Neurol. 13, 505–513. doi: 10.1111/j.1468-1331.2006.01280.x
Sun, J., Wang, F., Li, H., Zhang, H., Jin, J., Chen, W., et al. (2015). Neuroprotective effect of sodium butyrate against cerebral ischemia/reperfusion injury in mice. Biomed. Res. Int. 2015:395895, 1–8. doi: 10.1155/2015/395895
Tam, W. W. S., Tang, A., Woo, B., and Goh, S. Y. S. (2019). Perception of the preferred reporting items for systematic reviews and meta-analyses (PRISMA) statement of authors publishing reviews in nursing journals: a cross-sectional online survey. BMJ Open 9:e026271. doi: 10.1136/bmjopen-2018-026271
Tu, F., Pang, Q., Huang, T., Zhao, Y., Liu, M., and Chen, X. (2017). Apigenin ameliorates post-stroke cognitive deficits in rats through histone acetylation-mediated neurochemical alterations. Med. Sci. Monit. 23, 4004–4013. doi: 10.12659/msm.902770
Turner, N. D., and Lupton, J. R. (2011). Dietary fiber. Adv. Nutr. 2, 151–152. doi: 10.3945/an.110.000281
Tuttolomondo, A., Di Sciacca, R., Di Raimondo, D., Serio, A., D'Aguanno, G., La Placa, S., et al. (2009). Plasma levels of inflammatory and thrombotic/fibrinolytic markers in acute ischemic strokes: relationship with TOAST subtype, outcome and infarct site. J. Neuroimmunol. 215, 84–89. doi: 10.1016/j.jneuroim.2009.06.019
Wang, H., Song, W., Wu, Q., Gao, X., Li, J., Tan, C., et al. (2021). Fecal transplantation from db/db mice treated with sodium butyrate attenuates ischemic stroke injury. Microbiol. Spectr. 9:e0004221. doi: 10.1128/Spectrum.00042-21
Wang, H. B., Wang, P. Y., Wang, X., Wan, Y. L., and Liu, Y. C. (2012). Butyrate enhances intestinal epithelial barrier function via up-regulation of tight junction protein Claudin-1 transcription. Dig. Dis. Sci. 57, 3126–3135. doi: 10.1007/s10620-012-2259-4
Wang, H., Zhang, M., Li, J., Liang, J., Yang, M., Xia, G., et al. (2022). Gut microbiota is causally associated with poststroke cognitive impairment through lipopolysaccharide and butyrate. J. Neuroinflammation 19:76. doi: 10.1186/s12974-022-02435-9
Weber, A., Wasiliew, P., and Kracht, M. (2010). Interleukin-1 (IL-1) pathway. Sci. Signal. 3:cm1. doi: 10.1126/scisignal.3105cm1
Wong, J. M., de Souza, R., Kendall, C. W., Emam, A., and Jenkins, D. J. (2006). Colonic health: fermentation and short chain fatty acids. J. Clin. Gastroenterol. 40, 235–243. doi: 10.1097/00004836-200603000-00015
Wytrykowska, A., Prosba-Mackiewicz, M., and Nyka, W. M. (2016). IL-1beta, TNF-alpha, and IL-6 levels in gingival fluid and serum of patients with ischemic stroke. J. Oral Sci. 58, 509–513. doi: 10.2334/josnusd.16-0278
Xia, G. H., You, C., Gao, X. X., Zeng, X. L., Zhu, J. J., Xu, K. Y., et al. (2019). Stroke Dysbiosis index (SDI) in gut microbiome are associated with brain injury and prognosis of stroke. Front. Neurol. 10:397. doi: 10.3389/fneur.2019.00397
Yamashiro, K., Tanaka, R., Urabe, T., Ueno, Y., Yamashiro, Y., Nomoto, K., et al. (2017). Gut dysbiosis is associated with metabolism and systemic inflammation in patients with ischemic stroke. PLoS One 12:e0171521. doi: 10.1371/journal.pone.0171521
Yang, Y., Estrada, E. Y., Thompson, J. F., Liu, W., and Rosenberg, G. A. (2007). Matrix metalloproteinase-mediated disruption of tight junction proteins in cerebral vessels is reversed by synthetic matrix metalloproteinase inhibitor in focal ischemia in rat. J. Cereb. Blood Flow Metab. 27, 697–709. doi: 10.1038/sj.jcbfm.9600375
Zhang, H., Huang, Y., Li, X., Han, X., Hu, J., Wang, B., et al. (2021). Dynamic process of secondary pulmonary infection in mice with intracerebral hemorrhage. Front. Immunol. 12:767155. doi: 10.3389/fimmu.2021.767155
Zhou, Z., Xu, N., Matei, N., McBride, D. W., Ding, Y., Liang, H., et al. (2021). Sodium butyrate attenuated neuronal apoptosis via GPR41/Gbetagamma/PI3K/Akt pathway after MCAO in rats. J. Cereb. Blood Flow Metab. 41, 267–281. doi: 10.1177/0271678X20910533
Keywords: ischaemic stroke, butyrates, inflammation, apoptosis, meta-analysis, microbial metabolites
Citation: Yan S, Ji Q, Ding J, Liu Z, Wei W, Li H, Li L, Ma C, Liao D, He Z and Ai S (2024) Protective effects of butyrate on cerebral ischaemic injury in animal models: a systematic review and meta-analysis. Front. Neurosci. 18:1304906. doi: 10.3389/fnins.2024.1304906
Edited by:
Gabriel Broocks, University of Hamburg, GermanyReviewed by:
Susan Klapproth, University Medical Center Hamburg-Eppendorf, GermanyJustyna Agier, Medical University of Lodz, Poland
Copyright © 2024 Yan, Ji, Ding, Liu, Wei, Li, Li, Ma, Liao, He and Ai. This is an open-access article distributed under the terms of the Creative Commons Attribution License (CC BY). The use, distribution or reproduction in other forums is permitted, provided the original author(s) and the copyright owner(s) are credited and that the original publication in this journal is cited, in accordance with accepted academic practice. No use, distribution or reproduction is permitted which does not comply with these terms.
*Correspondence: Shuangchun Ai, aishuangchun@163.com
†These authors have contributed equally to this work