- 1College of Acupuncture and Moxibustion, Shaanxi University of Traditional Chinese Medicine, Xianyang, China
- 2Department of Acupuncture and Moxibustion, Shaanxi Hospital of Traditional Chinese Medicine, Xi'an, China
- 3MOE Key Laboratory of Biomedical Information Engineering, Xi'an Jiaotong University, Xi'an, China
- 4Department of Acupuncture and Moxibustion, Xi'an Hospital of Traditional Chinese Medicine, Xi'an, China
- 5Department of Acupuncture and Moxibustion, Shaanxi Provincial Institute of Traditional Chinese Medicine, Xi'an, China
Irritable bowel syndrome (IBS) is a common digestive disorder observed in clinics. Current studies suggest that the pathogenesis of the disease is closely related to abnormal brain–gut interactions, hypokinesia, visceral sensory hypersensitivity in the gastrointestinal tract, and alterations in the intestinal microenvironment. However, it is difficult for a single factor to explain the heterogeneity of symptoms. The Rome IV criteria emphasized the holistic biologic-psycho-social model of IBS, suggesting that symptoms of the disease are closely related to neurogastroenterology and various abnormalities in brain–gut interaction. This study comprehensively reviewed the relationship between the brain–gut axis and IBS, the structure of the brain–gut axis, and the relationship between the brain–gut axis and intestinal microenvironment, and discussed the relationship between the abnormal regulation of the nervous system, endocrine system, and immune system and the incidence of IBS on the basis of brain–gut axis. In terms of treatment, acupuncture therapy can regulate the neuroendocrine-immune system of the body and improve the intestinal microenvironment, and it has the advantages of safety, economy, and effectiveness. We study the pathogenesis of IBS from local to global and micro to macro, and review the use of acupuncture to treat the disease as a whole so as to provide new ideas for the treatment of the disease.
1. Introduction
Irritable bowel syndrome (IBS) is a complex functional gastrointestinal disorder characterized by abdominal pain, abdominal distension, and intestinal disorders that do not involve structural and biochemical abnormalities of the gastrointestinal tract (Ford et al., 2020). The Rome IV criteria classify patients with IBS into four subtypes based on their abnormal bowel movement traits: diarrheal IBS (IBS with diarrhea, IBS-D), constipated IBS (IBS with constipation, IBS-C), mixed IBS (IBS-M), and unclassified IBS (IBS-U) (Drossman, 2016). The global prevalence of IBS is approximately 11.2 % (Lovell and Ford, 2012). IBM seriously affects the quality of life of patients due to its high prevalence, recurrent symptoms, and susceptibility to complications, such as peptic ulcers, chronic liver disease, depression, and anxiety (Lai et al., 2021). In addition, it has led to a significant increase in the global healthcare burden (Black and Ford, 2020; Sperber et al., 2021).
The pathogenesis of IBS is multifactorial, which also has an important role in gene regulation, but the specific pathogenesis has not been fully elucidated. The disease is thought to be closely associated with abnormal brain–gut interactions, gastrointestinal hypokinesis, visceral sensory hypersensitivity, and intestinal microenvironmental disorders, indicating that a single factor cannot adequately explain the complexity of IBS symptoms. Therefore, treatment is mostly focused on improving gastrointestinal symptoms for different subtypes of IBS, currently involving analgesics, prokinetics, antidiarrheal agents, and psychotherapy (Ford et al., 2018; Arokiadoss and Weber, 2021). This can temporarily alleviate symptoms, but recurrence and varying degrees of side effects can occur (Aziz and Simrén, 2021), which are of great concern for clinical practitioners. Therefore, a fuller understanding of the pathogenesis of IBS is necessary to seek targeted therapies.
As IBS research progressed, it was defined by Rome IV as a disorder of gut–brain interactions (Schmulson and Drossman, 2017). For the understanding of the etiology and clinical features of IBS, Rome IV emphasizes a holistic biopsychosocial model, suggesting that the development of IBS symptoms is closely related to neurogastroenterology and multifaceted abnormalities in brain–gut interaction (Drossman and Hasler, 2016). The brain–gut axis maintains central and local homeostasis in the gut by integrating the neural, endocrine, and immune systems to form a bidirectional regulatory pathway and by connecting closely with the gut microbiota. Abnormalities in any of the brain–gut pathways may lead to the disruption of homeostatic balance and eventually induce IBS (Person and Keefer, 2021), in which neurological, endocrinological, and immunological factors intersect to produce symptoms that are the characteristic manifestation of the disease (Buckley et al., 2016). Therefore, understanding IBS from the perspective of abnormal regulation of the neuroendocrine-immune system in the brain–gut axis can facilitate the understanding of its pathogenesis.
2. Brain–gut axis
The brain–gut axis is a bidirectional information exchange system that integrates brain and intestinal functions, thereby effectively maintaining homeostasis in these organs (Carabotti et al., 2015). Different studies have confirmed that abnormal brain–gut interactions are closely related to IBS. Therefore, a deep understanding of the brain–gut axis would facilitate the elucidation of the pathogenesis of IBS. In the following sections, we highlight the basic architecture of the brain–gut axis and subsequently explore the relationship between the brain–gut axis and the intestinal microenvironment.
2.1. Basic architecture of the brain–intestine axis
Brain–gut interactions occur not only directly through neural pathways but also indirectly through humoral pathways (Bercik et al., 2012). First, the neural pathway of brain–gut interaction is a bidirectional interaction pathway linking the central nervous system (CNS) and the enteric nervous system (ENS). It is generally accepted that the regulation of gastrointestinal motility by the nervous system is achieved through three-level coordinated actions. Level 1 is the local regulation of the ENS, which consists of two plexuses: the interosseous plexus, which innervates the motility of the intestinal smooth muscle, and the submucosal plexus, which regulates intestinal mucosal sensation, secretion, and absorption. The sensory neurons, interneurons, and motor neurons of the ENS are interconnected to form separate, independent functions that integrate and process information similar to those of the brain and the spinal cord. Level 2 occurs in the anterior vertebral ganglion, which receives and regulates information from both the ENS and the CNS. Level 3 occurs in the CNS, which is composed of various centers at all levels of the brain and the spinal cord. They receive various incoming information during changes in the internal and external environment, integrate, and then transmit the regulatory information from the vegetative nervous and the neuroendocrine systems to the ENS, and act directly on gastrointestinal effector cells to regulate smooth muscle, glands, and blood vessels. This neuro-endocrine network linking the gastrointestinal tract to the CNS at different levels is the structural basis for the realization of brain–gut axis function (Bonaz and Bernstein, 2013).
Second, the humoral pathway mainly involves two bidirectional regulatory pathways and brain–gut peptides. Among them, the bidirectional regulatory pathways, namely, the hypothalamic-pituitary-adrenal axis (HPA) and the hypothalamic-autonomic nervous system axis (HANS), are the main pathways involved in physiological and psychological stress responses (Stasi et al., 2012).
In addition, brain and intestinal peptides are hormones that regulate the movement of the gallbladder and bile ducts, such as gastrokinetic hormone, cholecystokinin, and glucagon. These peptides are highly distributed in the gastrointestinal and nervous systems, and hence, they are called brain and intestinal peptides. Brain and intestinal peptides of the gastrointestinal and nervous systems regulate the complex functions of the gastrointestinal tract, such as movement, sensation, secretion, and absorption through endocrine, neurosecretory, and paracrine secretory peptides (Wu I. X. Y. et al., 2019). Different types of intestinal peptides, such as 5-hydroxytryptamine (5-HT), substance P (SP), calcitonin gene-related peptide, vasoactive intestinal peptide, somatostatin, neuropeptide Y, and cholecystokinin, can act on the central nervous and the enteric nervous systems, such that both systems can jointly regulate the functions of the gastrointestinal tract. In addition, different brain and intestinal peptides have different mechanisms of action, and most brain and intestinal peptides do not act on IBS in isolation. There are complex interconnections between various brain and intestinal peptides, which are jointly involved in the regulation of the physiological activities of the gastrointestinal tract.
2.2. Relationship between the brain–gut axis and the intestinal microenvironment
In the brain–gut interaction, neurological dysfunction can lead to alterations in the intestinal microenvironment (Li et al., 2020). This is composed of the microbiota, local immune system, and epithelium of the single-cell layer, which can effectively regulate homeostasis in the body and consequently maintain normal physiological functions of the gastrointestinal tract (Fay et al., 2017). Therefore, the relationship between the brain–gut axis and the intestinal microenvironment was explored from three perspectives: the intestinal microbiota, intestinal immune system, and intestinal mucosal permeability (the relationship between the brain–gut axis and the intestinal flora is shown in Figure 1).
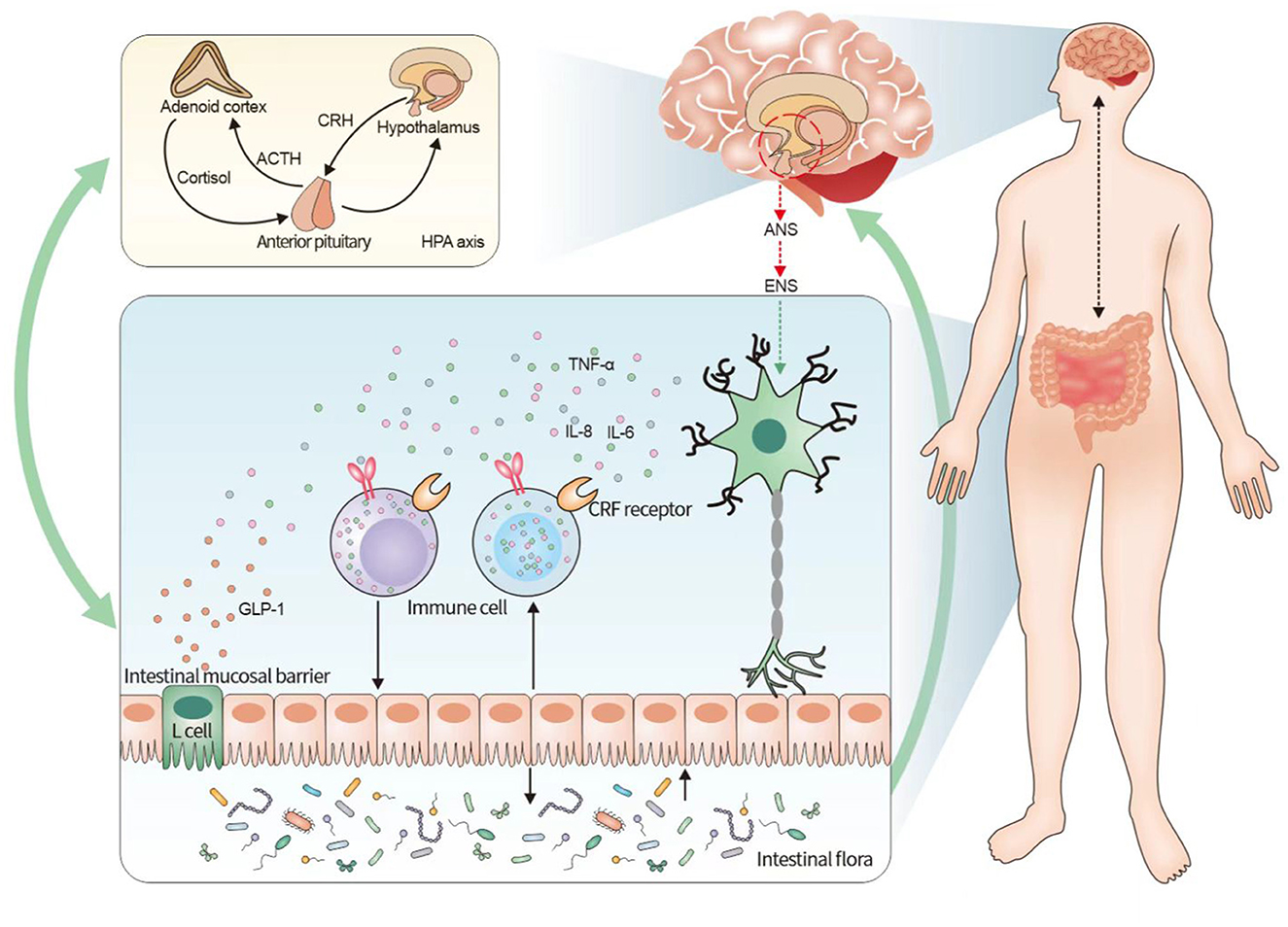
Figure 1. Relationship between irritable bowel syndrome and the neuroendocrine-immune system based on the brain–gut axis. The figure illustrates that the pathogenesis of irritable bowel syndrome can be understood in terms of the neuroendocrine-immune network system of the brain–gut axis. The brain–gut axis connects the ENS and the CNS and activates the endocrine system mainly by initiating the HPA axis in the CNS, while the endocrine system has a bidirectional regulation with the intestinal immune system, and the intestinal mucosal barrier, immune cells, and intestinal flora are involved in the intestinal immune system, among which the intestinal flora forms another bidirectional regulation with the brain–gut axis. It indicates that the neuroendocrine-immune system of the brain–gut axis influences the pathogenesis of IBS, and the three systems form a multidimensional and bidirectional regulation among themselves. CRF, adrenocorticotropin-releasing factor; HPA, hypothalamus-pituitary-adrenal; IBS, irritable bowel syndrome.
In recent years, studies on the pathogenesis of IBS have focused on the brain–gut axis, intestinal microecological relationships (Hillestad et al., 2022), and intestinal microbiota disorders (Aziz et al., 2017). The brain–gut axis integrates the neural, endocrine, and immune systems, forming a bidirectional regulatory pathway that maintains the homeostasis of brain and gut functions. The gut microbiota is an important player in the brain–gut bidirectional information exchange system. On the one hand, its interaction with the ENS and neurological and endocrine signaling pathways profoundly affects the brain–gut communication function (Martin et al., 2018); and on the other hand, it can influence the brain–gut axis function through direct and body circulation pathways. This indicates that the gut microbiota and the brain–gut axis are closely linked and interact with each other, thereby forming a complex brain–gut microbiota network to maintain normal gut function.
The brain–gut axis can influence the host gut flora, and similarly, the gut microbiota can effectively regulate some of the functions of the brain–gut axis (Khlevner et al., 2018). First, the brain–gut axis can influence the intestinal microbiota. On the one hand, the response signals from the CNS are transmitted to the smooth muscle layer of the intestine through the relevant fibers, thereby influencing gastrointestinal motility and secretion and regulating the intestinal flora environment (Mayer et al., 2014). On the other hand, stress and emergency stimulation of the host CNS will have an impact on the intestinal flora. It has been reported that neuroinflammation induced by traumatic brain injury through the brain–gut pathway results in impaired intestinal motility and increased intestinal mucosal permeability (Hanscom et al., 2021). Second, the intestinal microbiota can maintain the functional homeostasis of the host gut and participate in the regulation of neurological development as well as mood, appetite, cognition, and behavior in the host (Mu et al., 2016). The decrease in the number and diversity of gut flora is closely associated with neurological dysfunctions in the host, such as abnormalities in mood and behavior. Through the blood circulatory pathway, gut microbiota and its metabolites, such as short-chain fatty acids, neurotransmitters, and their precursors can influence the expression levels of neurotransmitters, precursors, and their receptors in the CNS, thus regulating brain function, cognition, and behavior (Cryan et al., 2020). Moreover, improving the disturbance of the intestinal microbiota and reconfiguring intestinal homeostasis may facilitate the recovery of the neurological function of an organism (O'Hagan et al., 2017). Bidirectional communication takes place between the brain and the gut, and the involvement of the gut microbiota has recently been discovered. An abundance of symbiotic microorganisms inhabit the lumen of the gastrointestinal tract and signal the immune and endocrine systems of the host through the secretion of metabolites such as short-chain fatty acids (SCFAs), amino acids, and polyamines. The aim of our study was to investigate specific types of microbiota-derived metabolites, specifically bile acids, short-chain fatty acids, vitamins, amino acids, serotonin, and hypoxanthines, which are all implicated in the pathogenesis of IBS.
Dysregulation of the microbial-gut-brain axis can exacerbate various CNS disorders through the expression of abnormal metabolites, such as SCFAs. Microbiota-derived metabolites play a central role in the communication between microbes and their hosts, with SCFAs probably being the most studied.
Short-chain fatty acids (SCFAs), mainly derived from the fermentation of dietary fiber, play a key role in host gut metabolism and immune function. Stress increases fecal acetate and total SCFA levels as well as colonic FFAR2 and FFAR3 expressions. Moreover, SCFA supplementation ameliorated acute stress-induced elevated body temperature and corticosterone levels in chronically stressed mice. Similar changes were found in colonic MR gene expression, although stress-induced increases in hippocampal MR gene expression were not affected. In addition, no differences in GR gene expression were found in any of the tissues investigated, and these differences may have disappeared over time. Sustained decreases in CRFR1, CRFR2, and MR expressions were observed in the group that received SCFA injections alone, and SCFA also had antidepressant and anxiolytic effects (Van De Wouw et al., 2018). Overall, these results suggest that SCFAs can down-regulate stress signaling and HPA axis responses, a critical pathway for microbe-gut-brain axis communication (Wiley et al., 2016). Studies using germ-free (GF) mice lacking all microbes provide compelling and consistent evidence that the microbiota is essential for brain development. GF mice exhibit altered expression of neurotransmitters and their receptors and neurotrophic factors in the brain, exhibit region-specific gene expression, and have an impaired blood–brain barrier. Even hippocampal neurogenesis and neuroplasticity in mice were affected by the absence of the gut microbiota. The maturation of oligodendrocytes is inhibited by specific gut microbial metabolites that alter myelin formation patterns in the limbic system. Therefore, a healthy, stable, and diverse intestinal microbiota is essential for normal brain–gut interactive communication.
In addition, the CNS directly affects the intestinal microbiota through stress-mediated virulence gene expression and indirectly through ANS-mediated intestinal motility, intestinal immune regulation, and secretion (Osadchiy et al., 2019). The top-down effect of the brain on the gut microbiota was also confirmed in a recent clinical randomized controlled trial (Jacobs et al., 2021).
The CNS damage increases intestinal mucosal permeability. For example, a large release of norepinephrine from cecum sympathetic nerves, altered cecal mucin production, and cupped cell numbers were observed in rats with brain injury, and this affected the intestinal microbiota and permeability (Houlden et al., 2016). We reviewed the literature and found that ANS dysfunction is seen after brain injury, and this exaggerates sympathetic activation (Purkayastha et al., 2019). In addition, under stressful stimuli, large amounts of neurotransmitters and receptors, such as norepinephrine and catecholamines, are released in the peripheral circulation (Zhang et al., 2020), and these regulate intestinal mucosal permeability.
Furthermore, the close relationship between HPA axis activation, a key humoral pathway in the brain–gut axis, and intestinal permeability has been demonstrated in clinical trials (Arciniega-maptinez et al., 2022). First, adrenocorticotropin-releasing factor (CRF) released by the HPA axis can increase intestinal permeability (Lyte et al., 2011). Second, the gut microbiome involves the blood–brain barrier (BBB) in its interactions with the peripheral and neuroimmune systems, and on the BBB, the gut microbiome can interact with the CNS and regulate body function. Bacteria can release the microbiome and its factors directly into the body's circulation and bloodstream. Once in the blood, the microbiome and its factors can alter peripheral immune cells to facilitate interaction with the BBB and eventually with other elements of the neurovascular unit. Bacteria and their factors or cytokines and other immunologically active substances released from peripheral sites under the influence of the microbiome can cross the BBB, alter the integrity of the BBB, change the rate of BBB transport, or induce the release of neuroimmune substances from barrier cells (Logsdon et al., 2018). In addition, metabolites produced by the microbiome, such as short-chain fatty acids, can cross the BBB to affect brain function. Through these and other mechanisms, microbiome–BBB interactions can influence the disease process, as reported for multiple sclerosis (Dopkins et al., 2018). Preclinical studies provide evidence that the BBB is altered when animals are subjected to experimental intestinal infections or when animals lack a normal gut microbiome.
In addition, the ENS has an important role in gastrointestinal peristalsis, digestion, secretion, and regulation of intestinal mucosal permeability (Furness, 2012). The ENS plays a key role in regulating pH in the gastrointestinal lumen and consequently intestinal inflammation, which is strongly associated with increased mucosal permeability (Tanaka et al., 2016).
With the exploration of the gut–brain axis, it was found that alpha synucleinopathy can be transmitted in both directions in the brain and the intestine. The pathology of PD [Parkinson's disease (PD)] is characterized by alpha synucleinopathy, which proves that the brain–gut axis also plays an important role in the pathogenesis of PD. In addition, the presence of a large microbiota in the gut, which is involved in the formation and transmission of alpha synucleinopathy, suggests that dysbiosis of the gut microbiota is closely related to the progression of PD.
3. Abnormal neural signal regulation
The cerebro-intestinal axis is a neuroendocrine network that connects the gastrointestinal system with the CNS, and it involves three main nervous systems: the CNS, ANS, and ENS. The gastrointestinal tract is the only organ in the body that is innervated by the CNS, ANS, and ENS, and its functional activities are neurologically regulated by these three systems. The impaired functions of any of the systems can cause gastrointestinal tract dysfunction and mediate the development of IBS.
3.1. ANS dysfunction
The ANS is part of the peripheral efferent nervous system that regulates the activity of the visceral and vascular smooth muscle, the cardiac muscle, and the glands. The sympathetic and parasympathetic nervous systems, under the control of the cerebral cortex and the hypothalamus, both antagonize and coordinate the physiological activities of the organs. The structure of the autonomic nervous system (ANS) can be divided into central and peripheral parts. The ANS is mainly distributed in the visceral, cardiovascular, and glandular bodies, whose central part is also located in the brain and the spinal cord. The peripheral part includes visceral motor (efferent) and visceral sensory (afferent) fibers, which constitute the visceral motor and visceral sensory nerves, respectively.
The ANS plays an important role in the brain–gut axis as a bridge between the central and the enteric nervous systems; hence, it is able to regulate the movement and sensation of the intestine. There are changes in autonomic function in patients with IBS that may be associated with autonomic dysfunction (Salvioli et al., 2015). The ANS comprises two nervous systems, sympathetic and parasympathetic, whose structures and functions are integrated into the brain–gut axis and work together to maintain functional homeostasis in the intestine. On the one hand, the sympathetic nervous system inhibits gastrointestinal motility and secretion, while the parasympathetic nervous system plays a stimulatory and pro-secretory role in smooth muscle. Sympathetic–parasympathetic imbalance causes altered bowel habits in patients with IBS. On the other hand, the modulation of visceral sensitivity by both is closely related to abdominal pain in IBS (De Winter et al., 2016). The parasympathetic afferent pathway begins in the vagus nerve and ends in the nucleus tractus solitarius, which transmits pain signals to the corticolimbic layer. The sympathetic afferent pathway mediates pain signals primarily through the spinal cord, first to the thalamus and then to the sensory cortex and pain stroma. The signal also travels to specific areas of the brain, such as the hippocampus, the amygdala, the prefrontal cortex, and finally to the hypothalamus (Fukudo, 2013). In addition, important tissues and organs in the CNS can interact with the HPA axis and the ANS. These central regions, which regulate intestinal function, are involved in both emotions (e.g., mood, anxiety, and pain) and cognitive behaviors (e.g., memory and decision-making), such that the dysfunction of the ANS directly or indirectly controls the development and progression of IBS disease.
3.2. CNS dysfunction
The CNS is composed of the brain and the spinal cord (the brain and the spinal cord are the central parts of various reflex arcs) and is the most dominant part of the human nervous system. It receives afferent information from all parts of the body, which is integrated and processed into coordinated motor efferents or stored in the CNS to become the neural basis for learning and memory.
Brain dysfunction has an important relationship with the development of IBS. Some key sites in the CNS associated with emotion-pain regulation are structurally altered in patients with IBS (Aziz and Simrén, 2021). For example, Sheehan et al. (2011) found a correlation between the activity of the cingulate gyrus and the pain-related cerebral cortex in patients with IBS and the clinical symptoms of IBS. Increased neuronal activity has been reported in the medial prefrontal cortex, the insula, and the anterior cingulate gyrus, which are brain regions associated with visceral pain in patients with IBS (Grinsvall et al., 2021), and a larger area of excitation was observed in brain regions associated with nociceptive processing (e.g., the prefrontal cortex, the anterior cingulate gyrus, the thalamus, etc.,) (Seminowicz et al., 2010). Similarly, in a cohort study, the IBS group exhibited extensive structural changes in the brain compared with that in the controls, and these changes in the brain included a reduction or increase in gray matter volume in multiple regions of the sensorimotor, central executive, and default mode networks, and these changes were strongly associated with chronic visceral pain (Öhlmann et al., 2021). Another study found that spontaneous activity in the amygdala and the anterior insula was significantly increased in patients with IBS, while spontaneous activity in sensorimotor areas tended to reduce (Hong et al., 2013). Moreover, cognitive-behavioral treatment reduced activity in the anterior cingulate gyrus, the amygdala, and the hypothalamus, suggesting that spontaneous activity of the brain may be associated with some symptoms of IBS. In addition, patients with IBS have smaller volumes in the bilateral superior frontal gyrus, the bilateral insular gyrus, the bilateral amygdala, the left cingulate gyrus, the left rectus gyrus, the left shell nucleus, and the brainstem, and larger volumes in the left postcentral gyrus (Labus et al., 2014), which are mostly part of the limbic system and are crucial for the body to perceive visceral injurious stimuli, pain production, emotional processing, and emotion regulation. Thus, CNS abnormalities can lead to visceral pain and emotional abnormalities in patients with IBS.
3.3. ENS dysfunction
The ENS consists of the ganglia contained in the gastrointestinal tract, the biliopancreatic system, and the network between them, which can connect the gastrointestinal tract with the CNS and ANS. On the one hand, it transmits sensory information to the brain through neurons, nerve fibers, and neurotransmitters connected to the central nerve, such that the activities of the intestine are regulated by the center. On the other hand, it has considerable autonomic functions to locally regulate the motility, secretion, and blood flow, as well as water and electrolyte transport of the intestine. The ENS consists of the ganglia and the biliopancreatic system contained in the gastrointestinal tract as well as the network between the ganglia and biliopancreatic system that connects the gastrointestinal tract to the CNS and ANS.
On the one hand, it transmits sensory information to the brain through neurons, nerve fibers, and neurotransmitters connected to the central nerve, such that the activities of the intestine are regulated by the center. On the other hand, it has considerable autonomic functions to locally regulate the motility, secretion, blood flow, as well as water and electrolyte transport of the intestine.
When the intestine is stimulated by mechanical dilatation, stress, inflammation, and allergens, chromophores release 5-HT as a paracrine signal acting on intestinal mast cells, spinal afferent cells, and neurons in the ENS. Intestinal mast cells release multiple mediators of paracrine signaling, including histamine and serine proteases, chymotrypsin and trypsin, and serotonin. Some of these mediators diffuse to receptors at the afferent injury-sensitive and mechanosensitive end of the spectrum and are known to cause abdominal pain and distention that may lead to IBS (Wood, 2020). In addition, 5-HT acts on spinal afferent neurons to mediate central responses to intestinal stimuli, as well as on 5-HT receptors on enteric neurons to influence intestinal sensory and motor functions. In IBS-D model rats, the total number of the ganglia and neurons in the submucosal plexus of the small intestine increased, the proportion of excitatory neurons (cholinergic neurons and vasoactive intestinal peptide neurons) increased, and the proportion of inhibitory neurons (nitrogenergic neurons) in the intermuscular plexus decreased (Li et al., 2016). Such morphological changes in the ENS are consistent with accelerated intestinal transmission and increased secretion in IBS-D.
The enteric nervous system (ENS) contains many neurotransmitters or brain intestinal peptides and can regulate intestinal function through a variety of neurotransmitters. When the internal environment of the gastrointestinal tract is altered, the intrinsic primary sensory neurons can be modified to produce strong sensory signals and transmitted upward to cause discomfort in the body. Therefore, the activation of primary neurons in the enteric plexus is one of the mechanisms associated with visceral hypersensitivity in IBS. Studies on enteric neurotransmitters and their complex signaling pathways as well as the interaction between enteric neurons and peripheral glial cells and Cajal mesenchymal cells have led to a better understanding of the functional regulation of the ENS in the pathogenesis of IBS (Zhang et al., 2019; Matheis et al., 2020). Therefore, ENS abnormalities are associated with the development of IBS.
4. Endocrine system abnormalities
The brain–gut axis has an important role in the development of IBS, and patients with IBS have hyperfunction of the HPA axis and impaired function of the endocrine system, which leads to abnormalities in an organism. Although there are many factors that affect the endocrine system, those with important roles include adrenocorticotropin-releasing factor (CRF), corticosteroids, and glucagon-like peptide 1 (GLP-1).
4.1. CRF
Adrenocorticotropin-releasing factor (CRF) is an important hormone associated with the body's response to stress. It is a major mediator of stress responses in the brain–gut axis, while the HPA axis is key to maintaining homeostasis and is responsible for various responses in the endocrine system. CRF plays a key role in the activation of the HPA axis under basal and stressful conditions (Chen et al., 2012). It may also explain the higher levels of adrenocorticotropic hormone and cortisol in patients with IBS, compared with that in healthy subjects (Distrutti et al., 2016). CRF exerts its biological effects by activating CRF1 and CRF2 receptors (CRFR1 and CRFR2), stimulating colonic motility, and inducing visceral hypersensitivity responses (Nozu and Okumura, 2015). CRFR1 is commonly found in the regions of the brain associated with pressure and nociceptive circuits, including the paraventricular nucleus, the ventricles, and the amygdala (Reyes et al., 2008). Toll-like receptor 4 (TLR4) or IL-1 receptor antagonists block abnormal visceral pain and increase intestinal permeability caused by CRF (Nozu et al., 2018). CRF receptor (CRF-R) signaling has critical roles in stress-related alterations in gastrointestinal function, stimulation of the colonic enteric nervous system, secretory motor function, intestinal permeability, and visceral hypersensitivity (Tache et al., 2018). In addition, Labus et al. found that peripheral administration of CRF1 receptor antagonists in patients with IBS reduced abdominal pain and anxiety (Labus et al., 2014). In addition, CRF receptors were found in interosseous nerves, sensory nerves, sympathetic nerves, intestinal chromophores, and immune cells in the guts of animals and humans. This suggests that the central and peripheral CRF system regulates the body's response to stress and modulates the syndromes that occur in IBS (Chen et al., 2012; Nozu and Okumura, 2015). In the immune context, CRF and CRF receptors are present in immune cells in the intestine. Stress, through the local CRF system, activates intestinal immune cells, leading to intestinal pro-inflammatory cytokine release, increased permeability, mucin secretion, and visceral allergy (Chatoo et al., 2018).
4.2. Corticosteroids
Corticosteroids are steroids produced by the adrenal cortex, and they mostly include hormones, such as glucocorticoids, mineralocorticoids, and sex hormones. They are readily absorbed by the gastrointestinal tract and are highly bound to proteins and play an important role in the treatment of intestinal disorders (Kapugi and Cunningham, 2019). Salt corticosteroids (MR) and glucocorticoids (GR) are steroid hormones that act in the stress system model through salt corticosteroid and glucocorticoid receptors (MRs and GRs, respectively), mediating the roles of adrenal hormones, such as cortisol, in the initiation and termination of the stress response, respectively. Corticosterone, which is the stress hormone in the amygdala, induces visceral hypersensitivity through the actions of GR and MR (Myers and Greenwood-Van Meerveld, 2012). It has been demonstrated that higher cortisol levels in patients with IBS positively correlated with psychological stress levels (Videlock et al., 2016). In addition, glucocorticoids, including endogenous cortisol, inhibit the production and activity of many inflammatory cells, as well as redistribute them to other parts of the body, resulting in fewer circulating immune cells (Ericson-Neilsen and Kaye, 2014). Chronic stress induces alterations in the expression of GR and CRH, leading to increased visceral pain and colonic permeability, while exposure to environmental enrichment suppresses stress-induced changes within the brain–gut axis to prevent visceral and somatic hypersensitivity and colonic hyperpermeability (Orock et al., 2021). All these indicate that the central signaling of corticosteroids is a potential target for the treatment of intestinal dysfunction in IBS.
4.3. GLP-1
Ingestion of certain foods is a predisposing factor for certain IBS symptoms (Simren et al., 2001). It has been shown that reducing the consumption of fermentable oligosaccharides, disaccharides, monosaccharides, and polyols (FODMAPs) may alleviate the symptoms of bloating, abdominal pain, and osmotic diarrhea in IBS (Barrett et al., 2010; Zahedi et al., 2018). Intestinal secretion of glucagon is an important physiological response that occurs in the intestine after eating. The secretion of glucagon-like peptide 1 (GLP-1) by L cells, expression of the surface receptor TGR5 by intestinal L cells, and induction of GLP-1 release from enteroendocrine cells by the TGR5 agonist 6α-ethyl-23(S)-methylcholic acid (EMCA, INT-777) inhibits glucagon secretion and gastric emptying and suppresses food uptake (Thomas et al., 2009). One study demonstrated that the glucagon-like peptide-1 receptor agonist ROSE-010 reduced pain during IBS episodes (Touny et al., 2022). However, GLP-1 acts on the enteric nervous system by reducing excitatory cholinergic neurotransmitters and regulating nitric oxide release through presynaptic GLP-1Rs (Amato et al., 2010). In addition, GLP-1 can initiate colonic enteric neurons and the vagus nerve (Amato et al., 2010); the vagus nerve is involved in the pathophysiological processes of IBS; hence, GLP-1 may influence the central regulation of visceral pain sensitivity. Interestingly, GLP-1 also modulates cytokines secreted by the GI tract and alters the central CRF pathway that regulates stress-induced alterations in colonic transit (Nakade et al., 2007).
5. Abnormal immune system
Current studies have confirmed the existence of an immune-activated state and the involvement of brain–gut interaction in the development of IBS. The immune system is an essential link in the neuroendocrine-immune network system, and IBS acts through immune cells, immune factors, and intestinal mucosal immune aspects and thus affects the whole system.
5.1. Immunocytes
Mast cells (MC), an intrinsic component of the neuroimmune axis, are present at elevated levels in IBS mucosal samples (Buhner and Schemann, 2012; Lee and Lee, 2016; Krammer et al., 2019). Although other immune cells may be involved in the pathophysiological process of IBS (Uranga et al., 2020), mast cells are important cellular mediators of local nerve fiber sensitization (Casado-Bedmar and Keita, 2020). The chronic inflammation of the intestinal mucosa in patients with IBS increases intestinal epithelial permeability. This leads to increased exposure of intestinal mucosa to intestinal contents, causes mast cell activation and degranulation to release large amounts of inflammatory mediators (Nogueira et al., 2017), stimulates visceral sensory neurons, causes the release of neuropeptides, and participates in pathophysiological processes, such as pain perception and cognition, through HANS upregulation mode (Skaper et al., 2014; Mackey et al., 2016). Similarly, mast cells can be activated by neuropeptides, such as SP, leading to the release of protein hydrolases, as a stress response to pain. Therefore, IBS is considered a brain–gut axis disease (Casado-Bedmar and Keita, 2020; Labanski et al., 2020). IBS biopsies showed an increased number of mast cells in the lamina propria, compared with that observed in the healthy controls (Krammer et al., 2019), and increased concentrations of products secreted by mast cells, such as histamine, proteases, cytokines, and prostaglandins, in patients with IBS activate intestinal neurons (Balestra et al., 2012). In addition, MC activation is associated with CRF, and it leads to increased visceral hypersensitivity and intestinal permeability by acting on mast cells and causing degranulation and release of trypsin-like enzymes, tumor necrosis factor-α, and histamine (Zhang et al., 2016; Tache et al., 2018). This effect may be due to the involvement of neuroendocrine-immune interaction mechanisms in the pathogenesis of IBS (Jarret et al., 2020).
In addition, the feedback response of the immune system induced by the HPA downregulation pattern can cause low-grade inflammation after which the colon is more susceptible to the effects of stress on intestinal nerve function (Wouters et al., 2016). The activated immune system in patients with IBS, as evidenced by increased expression of colonic mucosal cytokines and increased release of pro-inflammatory cytokines from monocytes isolated from peripheral blood, especially in patients with IBS-D, is specific to the gastrointestinal tract (Vicario et al., 2015; Van De Wouw et al., 2018). Increased humoral immunoreactivity in the jejunal mucosa of patients with IBS-D was identified by microarray analysis, which correlated with the activation of B lymphocytes and the production of immunoglobulins.
5.2. Immune factors
Evidence of immune activation in IBS includes elevated levels of pro-inflammatory cytokines, such as interleukin-6 (IL-6), IL-8, and tumor necrosis factor-α (Brzozowski et al., 2016; Seyedmirzaee et al., 2016; Ivashkin et al., 2021). On the one hand, the cytokines have neuromodulatory effects and can stimulate the excitation of intestinal mucosal pro-secretory neurons, which can also lead to changes in intestinal functions (e.g., contraction, absorption, and secretion). In addition, IL-6 releases the sympathetic brake by suppressing the presynaptic inhibition of norepinephrine release. On the other hand, visceral pain can be evoked after cytokine excitation of enteric neurons (Buckley et al., 2016), making cytokines an important cause of visceral pain-mediated IBS (Atmaramani et al., 2020).
It has been reported that the levels of anti-inflammatory cytokines (e.g., IL-10 and transforming growth factor beta) decreased in IBS colon and rectal biopsies (Coëffier et al., 2010; Ivashkin et al., 2021). Probiotics, as living microorganisms, can modulate the immune system, leading to the upregulation of anti-inflammatory cytokines and growth factors, and can interact with the brain–gut axis by regulating endocrine and neurological functions (Atmaramani et al., 2020). Thus, immune factors play an important role in the pathogenesis of IBS as intermediate transmitters or different “links” in the regulation of the neuroendocrine-immune network.
5.3. Intestinal mucosal immunity
The intestinal mucosal immune system is composed of intestinal epithelial cells, intestinal interepithelial lymphocytes, lymphocytes of the lamina propria, intestinal submucosal-collecting lymph nodes, and various monocytes, which directly participate in intestinal immune regulation. Some studies have confirmed the presence of abnormal mucosal immunity in patients with IBS (Aerssens et al., 2008), and stress factors are thought to be strongly associated with the development of IBS (Elsenbruch and Enck, 2016). Stress regulates the intestinal immune system by triggering the brain–gut axis to produce neurotransmitters and hormones. Secretory immunoglobulin A (SIgA) is the most important immunoglobulin on the mucosal surface of the intestine, and stress can cause the upregulation or downregulation of SIgA levels, thus affecting intestinal immunity (Campos-Rodríguez et al., 2013). SIgA production is regulated by stress (Brawner et al., 2020) and pIgR expression (Jarillo-Luna et al., 2015), and the immunoglobulin-pIgR complex (Diga-pIgR and PIGA-pIgR) is transported cellularly through the intestinal epithelium. Upon reaching the tip of these cells, pIgR is cleaved, releasing sIgA, and a pIgR-derived polypeptide into the intestinal lumen (Brandtzaeg, 2013). Therefore, SIgA and pIgR protect the intestinal epithelium from pathogens and regulate the intestinal inflammatory response to maintain homeostasis in vivo. In addition, visceral nerve fibers are distributed in the submucosa (Meissner's plexus) and mucosal plexus, which contain nerve endings that can contact antigen-presenting cells to control intestinal immune responses (De Jonge, 2013; Jacobson et al., 2021). These indicate that the IBS mucosal immune system also plays a significant role in the CNS.
6. Discussion
Irritable bowel syndrome (IBS) is a common functional gastrointestinal disorder that has a significant impact on the quality of life of patients due to its high prevalence, severity, and recurrence; hence, effective interventions are urgently needed. The efficacy of current interventions does not meet the clinical requirements of patients because the pathophysiology of the disease is not fully understood in terms of etiology and heterogeneity of symptoms. Therefore, it is necessary to have a comprehensive understanding of the disease toward the development of targeted interventions for improved clinical treatment.
Under the social–psychological–biological model advocated by Rome IV, this study highlights the pathogenesis of IBS from the perspective of abnormal regulation of the neuroendocrine-immune system of the brain–gut axis. In addition, it finds that a complex system consisting of several systems, such as the nervous, immune, and endocrine systems, participates in the regulation of the brain–gut axis. IBS may occur if there is a problem in a certain link, which may also trigger the dysfunction of other systems, resulting in the diversity and severity of IBS symptoms.
Traditional Chinese medicine is a medical theory system gradually formed and developed in long-term medical practice. In the long-term clinical use of TCM against digestive system diseases, we have discovered a close relationship between the brain and the intestine. Interestingly, this relationship is validated by the brain–gut axis theory from current microscopic studies, which suggest that there is a theoretical basis for the efficacy of TCM acupuncture therapy against IBS. Modern studies have shown that TCM acupuncture therapy can effectively regulate the functional levels of the brain–gut axis in rats with IBS (Sun et al., 2015), suggesting that TCM acupuncture therapy is a potential complementary alternative therapy for IBS.
In recent years, acupuncture therapy has attracted the attention of medical researchers in China and abroad because of its safety, efficiency, and green features. In particular, its efficacy in regulating gastrointestinal dysfunction and extraintestinal conditions has been favored by clinical medical practitioners worldwide. A large number of clinical studies have confirmed that acupuncture therapy contributes to the management of IBS, such as systematic evaluation studies demonstrating the effectiveness of acupuncture therapy (Wu Y. et al., 2019) and randomized controlled clinical trials investigating its feasibility and efficacy (Pei et al., 2020; Qi et al., 2021; Shen et al., 2022).
In addition, different studies have confirmed that acupuncture can modulate IBS from the intestinal and dorsal root ganglion perspectives (Jin et al., 2021), brain functional interconnections and effects (Ma et al., 2020), and at the level of the brain–gut axis (Zhu et al., 2017), and expert treatment consensus opinions have been formed (Zhu et al., 2017). In conclusion, acupuncture therapy can effectively treat IBS and modulate gastrointestinal symptoms and concomitant mood disorders in patients.
Irritable bowel syndrome (IBS) is a complex functional gastrointestinal disease, and it is difficult to dissect the complexity of the disease from the perspectives of abnormal brain–gut interactions, hypokinetic and visceral sensory hypersensitivity, and altered intestinal microenvironment alone. Rather, clinical workers can understand IBS from a holistic bio-psycho-social model, which has the following five guiding implications for patients and researchers: (1) the combination of anti-anxiety and depression drugs can be used to treat irritable bowel syndrome in clinical work, providing a new way of thinking for the clinical treatment of the disease; (2) the treatment can be done from the perspective of treating the bowel from the brain; (3) acupuncture can be used to treat irritable bowel syndrome from the aspect of anxiety and depression; (4) keeping patients away from stressors to avoid long-term stimulation can be a treatment method; and (5) patients can be provided with stress training, psychological training, and positive meditation to prevent later relapse or frequent relapse.
Author contributions
CJ and JZ: study concept and design. ZS, XW, and CJ: search for information. ZS and XW: draft of the manuscript. All authors contributed to the article and approved the submitted version.
Funding
This study was supported by grants from the National Natural Science Foundation of China (82074560), the Shaanxi Province Natural Science Basic Research Program Project (2020JM-697), the Shaanxi Province Innovation Capacity Support Program (2020KJXX-075), and the Shaanxi Provincial Traditional Chinese Medicine Administration Scientific Research Project (JCMS064).
Acknowledgments
The authors would like to thank Shanbo Ma, Kun Zhang, and Xiaocun Yang for their help during the discussion and revision.
Conflict of interest
The authors declare that the research was conducted in the absence of any commercial or financial relationships that could be construed as a potential conflict of interest.
Publisher's note
All claims expressed in this article are solely those of the authors and do not necessarily represent those of their affiliated organizations, or those of the publisher, the editors and the reviewers. Any product that may be evaluated in this article, or claim that may be made by its manufacturer, is not guaranteed or endorsed by the publisher.
References
Aerssens, J., Camilleri, M., Talloen, W., Thielemans, L., Göhlmann, H. W., Van Den Wyngaert, I., et al. (2008). Alterations in mucosal immunity identified in the colon of patients with irritable bowel syndrome. Clin. Gastroenterol. Hepatol. 6, 194–205. doi: 10.1016/j.cgh.2007.11.012
Amato, A., Cinci, L., Rotondo, A., Serio, R. Faussone-aussone-pellegrini, M. S., Vannucchi, M. G., and Mulè, F. (2010). Peripheral motor action of glucagon-like peptide-1 through enteric neuronal receptors. Neurogastroenterol. Motil. 22, 664–e203. doi: 10.1111/j.1365-2982.2010.01476.x
Arciniega-maptinez, I. M., Resendiz albor, A. A., Cárdenas jaramillo, L. M., Gutlerrez-meza, J. M., Falfan-valencia, R., Arroyo, B. M., et al. (2022). CD4.(+)/IL-4(+) lymphocytes of the lamina propria and substance P promote colonic protection during acute stress. Mol. Med. Rep. 25, 1–10. doi: 10.3892/mmr.2021.12579
Arokiadoss, A., and Weber, H. C. (2021). Targeted pharmacotherapy of irritable bowel syndrome. Curr. Opin. Endocrinol. Diabetes Obes. 28, 214–221. doi: 10.1097/MED.0000000000000618
Atmaramani, R. R., Black, B. J., de la Peña, J. B., Campbell, Z. T., and Pancrazio, J. J. B. J. (2020) Conserved expression of Nav1 7. Nav1.8 contribute to the spontaneous thermally evoked excitability in IL-6 NGF-sensitized adult dorsal root ganglion neurons in vitro. Bioengineering. 7, 44. doi: 10.3390/bioengineering7020044
Aziz, I., and Simrén, M. (2021). The overlap between irritable bowel syndrome and organic gastrointestinal diseases. Lancet Gastroenterol. Hepatol. 6, 139–148. doi: 10.1016/S2468-1253(20)30212-0
Aziz, I., Törnblom, H., and Simrén, M. (2017). Small intestinal bacterial overgrowth as a cause for irritable bowel syndrome: guilty or not guilty? Curr. Opin. Gastroenterol. 33, 196–202. doi: 10.1097/MOG.0000000000000348
Balestra, B., Vicini, R., Cremon, C., Zecchi, L., Dothel, G., Vasina, V., et al. (2012). Colonic mucosal mediators from patients with irritable bowel syndrome excite enteric cholinergic motor neurons. Neurogastroenterol. Motil 24, 1118–e570. doi: 10.1111/nmo.12000
Barrett, J. S., Gearry, R. B., Muir, J. G., Irving, P. M., Rose, R., Rosella, O., et al. (2010). Dietary poorly absorbed, short-chain carbohydrates increase delivery of water and fermentable substrates to the proximal colon. Aliment. Pharmacol. Ther. 31, 874–882. doi: 10.1111/j.1365-2036.2010.04237.x
Bercik, P., Collins, S. M., and Verdu, E. F. (2012). Microbes and the gut-brain axis. Neurogastroenterol. Motil. 24, 405–413. doi: 10.1111/j.1365-2982.2012.01906.x
Black, C. J., and Ford, A. C. (2020). Global burden of irritable bowel syndrome: trends, predictions and risk factors. Nat. Rev. Gastroenterol. Hepatol. 17, 473–486. doi: 10.1038/s41575-020-0286-8
Bonaz, B. L., and Bernstein, C. N. (2013). Brain-gut interactions in inflammatory bowel disease. Gastroenterology. 144, 36–49. doi: 10.1053/j.gastro.2012.10.003
Brandtzaeg, P. (2013). Secretory IgA: designed for anti-microbial defense. Front. Immunol. 4, 222. doi: 10.3389/fimmu.2013.00222
Brawner, K. M., Yeramilli, V. A., Kennedy, B. A., Patel, R. K., and Martin, C. A. (2020). Prenatal stress increases IgA coating of offspring microbiota and exacerbates necrotizing enterocolitis-like injury in a sex-dependent manner. Brain Behav. Immun. 89, 291–299. doi: 10.1016/j.bbi.2020.07.008
Brzozowski, B., Mazur-Bialy, A., Pajdo, R., Kwiecien, S., Bilski, J., Zwolinska-Wcislo, M., et al. (2016). Mechanisms by which stress affects the experimental and clinical inflammatory bowel disease (IBD): role of brain-gut axis. Curr. Neuropharmacol. 14, 892–900. doi: 10.2174/1570159X14666160404124127
Buckley, M. M., O'Brien, R., Devlin, M., Creed, A. A., Rae, M. G., Hyland, N. P., et al. (2016). Leptin modifies the prosecretory and prokinetic effects of the inflammatory cytokine interleukin-6 on colonic function in Sprague-Dawley rats. Exp. Physiol. 101, 1477–1491. doi: 10.1113/EP085917
Buhner, S., and Schemann, M. (2012). Mast cell-nerve axis with a focus on the human gut. Biochim. Biophys. Acta. 1822, 85–92. doi: 10.1016/j.bbadis.2011.06.004
Campos-Rodríguez, R. Godinez-victoria, M., Abarca-rojano, E., Pacheco-yépez, J., Reyna-garfias, H., Barbosa-cabrera, R. E., and Drago-serrano, M. E. (2013). Stress modulates intestinal secretory immunoglobulin A. Front. Integr. Neurosci. 7, 86. doi: 10.3389/fnint.2013.00086
Carabotti, M., Scirocco, A., Maselli, M. A., and Severi, C. (2015). The gut-brain axis: Interactions between enteric microbiota, central and enteric nervous systems. Ann. Gastroenterol. 28, 203–209.
Casado-Bedmar, M., and Keita, Å. V. (2020). Potential neuro-immune therapeutic targets in irritable bowel syndrome. Therap. Adv. Gastroenterol. 13, 1756284820910630. doi: 10.1177/1756284820910630
Chatoo, M., Li, Y., Ma, M. A., Coote, Z., Du, J. J., and Chen, X. (2018). Involvement of corticotropin-releasing factor and receptors in immune cells in irritable bowel syndrome. Front. Endocrinol. 9, 21. doi: 10.3389/fendo.2018.00021
Chen, X. Q., Kong, F. P., Zhao, Y., and Du, J. Z. (2012). High-altitude hypoxia induces disorders of the brain-endocrine-immune network through activation of corticotropin-releasing factor and its type-1 receptors. Zhongguo Ying Yong Sheng Li Xue Za Zhi. 28, 481–487.
Coëffier, M., Gloro, R., Boukhettala, N., Aziz, M., Lecleire, S., Vandaele, N., et al. (2010). Increased proteasome-mediated degradation of occludin in irritable bowel syndrome. Am. J. Gastroenterol. 105, 1181–8. doi: 10.1038/ajg.2009.700
Cryan, J. F., O'Riordan, K. J., Sandhu, K., Peterson, V., and Dinan, T. G. (2020). The gut microbiome in neurological disorders. Lancet Neurol. 19, 179–194. doi: 10.1016/S1474-4422(19)30356-4
De Jonge, W. J. (2013). The Gut's little brain in control of intestinal immunity. Gastroenterol. 2013, 630159. doi: 10.1155/2013/630159
De Winter, B. Y., Deiteren, A., and De Man, J. G. (2016). Novel nervous system mechanisms in visceral pain. Neurogastroenterol. Motil. 28, 309–315. doi: 10.1111/nmo.12785
Distrutti, E., Monaldi, L., Ricci, P., and Fiorucci, S. (2016). Gut microbiota role in irritable bowel syndrome: new therapeutic strategies. World J. Gastroenterol. 22, 2219–2241. doi: 10.3748/wjg.v22.i7.2219
Dopkins, N., Nagarkatti, P. S., and Nagarkatti, M. (2018). The role of gut microbiome and associated metabolome in the regulation of neuroinflammation in multiple sclerosis and its implications in attenuating chronic inflammation in other inflammatory and autoimmune disorders. Immunology. 154, 178–185. doi: 10.1111/imm.12903
Drossman, D. A. (2016). Functional gastrointestinal disorders: history, pathophysiology, clinical features and Rome IV. Gastroenterology 150:1262–79. doi: 10.24890/pc
Drossman, D. A., and Hasler, W. L. (2016). Rome IV-functional GI disorders: disorders of gut-brain interaction. Gastroenterology 150, 1257–1261. doi: 10.1053/j.gastro.2016.03.035
Elsenbruch, S., and Enck, P. (2016). Psychobiological mechanisms in the pathophysiology of chronicvisceral pain. Schmerz. 30, 407–411. doi: 10.1007/s00482-016-0130-9
Ericson-Neilsen, W., and Kaye, A. D. (2014). Steroids: pharmacology, complications, and practice delivery issues. Ochsner J. 14, 203–207. doi: 10.1142/9781848168305_0013
Fay, K. T., Ford, M. L., and Coopersmith, C. M. (2017). The intestinal microenvironment in sepsis. Biochim. Biophys. Acta Mol. Basis Dis. 1863, 2574–2583. doi: 10.1016/j.bbadis.2017.03.005
Ford, A. C., Moayyedi, P., Chey, W. D., Harris, L. A., Lacy, B. E., Saito, Y. A., et al. (2018). American college of gastroenterology monograph on management of irritable bowel syndrome. Am. J. Gastroenterol. 113, 1–18. doi: 10.1038/s41395-018-0084-x
Ford, A. C., Sperber, A. D., Corsetti, M., and Camilleri, M. (2020). Irritable bowel syndrome. Lancet. 396, 1675–1688. doi: 10.1016/S0140-6736(20)31548-8
Fukudo, S. (2013). Stress and visceral pain: focusing on irritable bowel syndrome. Pain. 154, S63–S70. doi: 10.1016/j.pain.2013.09.008
Furness, J. B. (2012). The enteric nervous system and neurogastroenterology. Nat. Rev. Gastroenterol. Hepatol. 9, 286–294. doi: 10.1038/nrgastro.2012.32
Grinsvall, C., Van Oudenhove, L., Dupont, P., Ryu, H. J., Ljungberg, M., Labus, J. S., et al. (2021). Altered structural covariance of insula, cerebellum and prefrontal cortex is associated with somatic symptom levels in irritable bowel syndrome (IBS). Brain Sci. 11, 1580. doi: 10.3390/brainsci11121580
Hanscom, M., Loane, D. J., and Shea-Donohue, T. (2021). Brain-gut axis dysfunction in the pathogenesis of traumatic brain injury. J. Clin. Invest. 131, 12. doi: 10.1172/JCI143777
Hillestad, E. M. R., Van Der Meeren, A., Nagaraja, B. H., Bjørsvik, B. R., Haleem, N., Benitez-Paez, A., et al. (2022). Gut bless you: the microbiota-gut-brain axis in irritable bowel syndrome. World J. Gastroenterol. 28, 412–431. doi: 10.3748/wjg.v28.i4.412
Hong, J. Y., Kilpatrick, L. A., Labus, J., Gupta, A., Jiang, Z., Ashe-Mcnalley, C., et al. (2013). Patients with chronic visceral pain show sex-related alterations in intrinsic oscillations of the resting brain. J. Neurosci. 33, 11994–12002. doi: 10.1523/JNEUROSCI.5733-12.2013
Houlden, A., Goldrick, M., Brough, D., Vizi, E. S. LéNáRT, N., Martinecz, B., et al. (2016). Brain injury induces specific changes in the caecal microbiota of mice via altered autonomic activity and mucoprotein production. Brain Behav. Immun. 57, 10–20. doi: 10.1016/j.bbi.2016.04.003
Ivashkin, V., Poluektov, Y., Kogan, E., Shifrin, O., Sheptulin, A., Kovaleva, A., et al. (2021). Disruption of the pro-inflammatory, anti-inflammatory cytokines and tight junction proteins expression, associated with changes of the composition of the gut microbiota in patients with irritable bowel syndrome. PLOS ONE. 16, e0252930. doi: 10.1371/journal.pone.0252930
Jacobs, J. P., Gupta, A., Bhatt, R. R., Brawer, J., Gao, K., Tillisch, K., et al. (2021). Cognitive behavioral therapy for irritable bowel syndrome induces bidirectional alterations in the brain-gut-microbiome axis associated with gastrointestinal symptom improvement. Microbiome. 9, 236. doi: 10.1186/s40168-021-01188-6
Jacobson, A., Yang, D., Vella, M., and Chiu, I. M. (2021). The intestinal neuro-immune axis: crosstalk between neurons, immune cells, and microbes. Mucosal Immunol. 14, 555–565. doi: 10.1038/s41385-020-00368-1
Jarillo-Luna, R. A., Rivera-Aguilar, V., Pacheco-Yépez, J. Godínez-victoria, M., Oros- pantoja, R., Miliar-garcía, A., and Campos-rodríguez, R. (2015). Nasal IgA secretion in a murine model of acute stress. The possible role of catecholamines. J. Neuroimmunol. 278, 223–31. doi: 10.1016/j.jneuroim.2014.11.009
Jarret, A., Jackson, R., Duizer, C., Healy, M. E., Zhao, J., Rone, J. M., et al. (2020). Enteric nervous system-derived IL-18 orchestrates mucosal barrier immunity. Cell 180, 50–63.e12. doi: 10.1016/j.cell.2019.12.016
Jin, D., Liu, Y., Lv, S., Qi, Q., Li, M., Wang, Y., et al. (2021). Electroacupuncture and moxibustion modulate the BDNF and TrkB expression in the colon and dorsal root ganglia of IBS rats with visceral hypersensitivity. Evid. Based Complement Alternat Med. 2021, 8137244. doi: 10.1155/2021/8137244
Kapugi, M., and Cunningham, K. (2019). Corticosteroids. Orthop. Nurs. 38, 336–339. doi: 10.1097/NOR.0000000000000595
Khlevner, J., Park, Y., and Margolis, K. G. (2018). Brain-gut axis: clinical implications. Gastroenterol. Clin. North Am. 47, 727–739. doi: 10.1016/j.gtc.2018.07.002
Krammer, L., Sowa, A. S., and Lorentz, A. (2019). Mast cells in irritable bowel syndrome: a systematic review. J. Gastrointestin. Liver Dis. 28, 463–472. doi: 10.15403/jgld-229
Labanski, A., Langhorst, J., Engler, H., and Elsenbruch, S. (2020). Stress and the brain-gut axis in functional and chronic-inflammatory gastrointestinal diseases: a transdisciplinary challenge. Psychoneuroendocrinology. 111, 104501. doi: 10.1016/j.psyneuen.2019.104501
Labus, J. S., Dinov, I. D., Jiang, Z., Ashe-Mcnalley, C., Zamanyan, A., Shi, Y., et al. (2014). Irritable bowel syndrome in female patients is associated with alterations in structural brain networks. Pain. 155, 137–149. doi: 10.1016/j.pain.2013.09.020
Lai, Y. T., Chen, C. Y., and Bair, M. J. (2021). Epidemiology, clinical features, and prescribing patterns of irritable bowel syndrome in Taiwan. Front. Pharmacol. 12, 788795. doi: 10.3389/fphar.2021.788795
Lee, K. N., and Lee, O. Y. (2016). The role of mast cells in irritable bowel syndrome. Gastroenterol. Res. Pract. 2016, 2031480. doi: 10.1155/2016/2031480
Li, S., Fei, G., Fang, X., Yang, X., Sun, X., Qian, J., et al. (2016). Changes in enteric neurons of small intestine in a rat model of irritable bowel syndrome with diarrhea. J. Neurogastroenterol. Motil. 22, 310–320. doi: 10.5056/jnm15082
Li, X. J., You, X. Y., Wang, C. Y., Li, X. L., Sheng, Y. Y., Zhuang, P. W., et al. (2020). Bidirectional Brain-gut-microbiota Axis in increased intestinal permeability induced by central nervous system injury. CNS Neurosci. Ther. 26, 783–790. doi: 10.1111/cns.13401
Logsdon, A. F., Erickson, M. A., Rhea, E. M., Salameh, T. S., and Banks, W. A. (2018). Gut reactions: how the blood-brain barrier connects the microbiome and the brain. Exp. Biol. Med. 243, 159–165. doi: 10.1177/1535370217743766
Lovell, R. M., and Ford, A. C. (2012). Global prevalence of and risk factors for irritable bowel syndrome: a meta-analysis. Clin. Gastroenterol. Hepatol. 10, 712–721.e4. doi: 10.1016/j.cgh.2012.02.029
Lyte, M., Vulchanova, L., and Brown, D. R. (2011). Stress at the intestinal surface: catecholamines and mucosa-bacteria interactions. Cell Tissue Res. 343, 23–32. doi: 10.1007/s00441-010-1050-0
Ma, K., Liu, Y., Shao, W., Sun, J., Li, J., Fang, X., et al. (2020). Brain functional interaction of acupuncture effects in diarrhea-dominant irritable bowel syndrome. Front. Neurosci. 14, 608688. doi: 10.3389/fnins.2020.608688
Mackey, E., Ayyadurai, S., Pohl, C. S. D' Costa, S., Li, Y., and Moeser, A. J. (2016). Sexual dimorphism in the mast cell transcriptome and the pathophysiological responses to immunological and psychological stress. Biol. Sex Differ. 7, 60. doi: 10.1186/s13293-016-0113-7
Martin, C. R., Osadchiy, V., Kalani, A., and Mayer, E. A. (2018). The brain-gut-microbiome axis. Cell. Mol. Gastroenterol. Hepatol. 6, 133–148. doi: 10.1016/j.jcmgh.2018.04.003
Matheis, F., Muller, P. A., Graves, C. L., Gabanyi, I., Kerner, Z. J., Costa-Borges, D., et al. (2020). Adrenergic signaling in muscularis macrophages limits infection-induced neuronal loss. Cell. 180, 64–78.e16. doi: 10.1016/j.cell.2019.12.002
Mayer, E. A., Knight, R., Mazmanian, S. K., Cryan, J. F., and Tillisch, K. (2014). Gut microbes and the brain: Paradigm shift in neuroscience. J. Neurosci. 34, 15490–15496. doi: 10.1523/JNEUROSCI.3299-14.2014
Mu, C., Yang, Y., and Zhu, W. (2016). Gut microbiota: the brain peacekeeper. Front. Microbiol. 7, 345. doi: 10.3389/fmicb.2016.00345
Myers, B., and Greenwood-Van Meerveld, B. (2012). Differential involvement of amygdala corticosteroid receptors in visceral hyperalgesia following acute or repeated stress. Am. J. Physiol. Gastrointest. Liver Physiol. 302, G260–G266. doi: 10.1152/ajpgi.00353.2011
Nakade, Y., Tsukamoto, K., Iwa, M., Pappas, T. N., and Takahashi, T. (2007). Glucagon like peptide-1 accelerates colonic transit via central CRF and peripheral vagal pathways in conscious rats. Auton. Neurosci. 131, 50–56. doi: 10.1016/j.autneu.2006.06.007
Nogueira, L. T., Costa, D. V., Gomes, A. S., Martins, C. S., Silva, A. M., Coelho-Aguiar, J. M., et al. (2017). The involvement of mast cells in the irinotecan-induced enteric neurons loss and reactive gliosis. J. Neuroinflammation. 14, 79. doi: 10.1186/s12974-017-0854-1
Nozu, T., Miyagishi, S., Nozu, R., Takakusaki, K., and Okumura, T. (2018). Altered colonic sensory and barrier functions by CRF: roles of TLR4 and IL-1. J. Endocrinol. 239, 241–252. doi: 10.1530/JOE-18-0441
Nozu, T., and Okumura, T. (2015). Corticotropin-releasing factor receptor type 1 and type 2 interaction in irritable bowel syndrome. J. Gastroenterol. 50, 819–830. doi: 10.1007/s00535-015-1086-8
O'Hagan, C., Li, J. V., Marchesi, J. R., Plummer, S., Garaiova, I., Good, M. A., et al. (2017). Long-term multi-species Lactobacillus and Bifidobacterium dietary supplement enhances memory and changes regional brain metabolites in middle-aged rats. Neurobiol. Learn. Mem. 144, 36–47. doi: 10.1016/j.nlm.2017.05.015
Öhlmann, H., Koenen, L. R., Labrenz, F., Engler, H., Theysohn, N., Langhorst, J., et al. (2021). Altered brain structure in chronic visceral pain: specific differences in gray matter volume and associations with visceral symptoms and chronic stress. Front. Neurol. 12, 733035. doi: 10.3389/fneur.2021.733035
Orock, A., Louwies, T., Ligon, C. O., Mohammadi, E., and Greenwood-Van Meerveld, B. (2021). Environmental enrichment prevents stress-induced epigenetic changes in the expression of glucocorticoid receptor and corticotrophin releasing hormone in the central nucleus of the amygdala to inhibit visceral hypersensitivity. Exp. Neurol. 345, 113841. doi: 10.1016/j.expneurol.2021.113841
Osadchiy, V., Martin, C. R., and Mayer, E. A. (2019). Gut microbiome and modulation of CNS function. Compr. Physiol. 10, 57–72. doi: 10.1002/cphy.c180031
Pei, L., Geng, H., Guo, J., Yang, G., Wang, L., Shen, R., et al. (2020). Effect of acupuncture in patients with irritable bowel syndrome: a randomized controlled trial. Mayo Clin. Proc. 95, 1671–1683. doi: 10.1016/j.mayocp.2020.01.042
Person, H., and Keefer, L. (2021). Psychological comorbidity in gastrointestinal diseases: update on the brain-gut-microbiome axis. Prog. Neuropsychopharmacol. Biol. Psychiatry. 107, 110209. doi: 10.1016/j.pnpbp.2020.110209
Purkayastha, S., Stokes, M., and Bell, K. R. (2019). Autonomic nervous system dysfunction in mild traumatic brain injury: a review of related pathophysiology and symptoms. Brain Inj. 33, 1129–1136. doi: 10.1080/02699052.2019.1631488
Qi, L. Y., Wang, Y., Wang, L. Q., She, Y. F., Shi, G. X., Li, Y., et al. (2021). Acupuncture for the treatment of diarrheal-predominant irritable bowel syndrome: study protocol for a pilot randomized controlled trial. Trials. 22, 253. doi: 10.1186/s13063-021-05211-x
Reyes, B. A., Valentino, R. J., and Van Bockstaele, E. J. (2008). Stress-induced intracellular trafficking of corticotropin-releasing factor receptors in rat locus coeruleus neurons. Endocrinology 149, 122–130. doi: 10.1210/en.2007-0705
Salvioli, B., Pellegatta, G., Malacarne, M., Pace, F., Malesci, A., Pagani, M., et al. (2015). Autonomic nervous system dysregulation in irritable bowel syndrome. Neurogastroenterol. Motil. 27, 423–430. doi: 10.1111/nmo.12512
Schmulson, M. J., and Drossman, D. A. (2017). What is new in Rome IV. J. Neurogastroenterol. Motil. 23, 151–163. doi: 10.5056/jnm16214
Seminowicz, D. A., Labus, J. S., Bueller, J. A., Tillisch, K., Naliboff, B. D., Bushnell, M. C., et al. (2010). Regional gray matter density changes in brains of patients with irritable bowel syndrome. Gastroenterology. 139, 48–57.e2. doi: 10.1053/j.gastro.2010.03.049
Seyedmirzaee, S., Hayatbakhsh, M. M., Ahmadi, B., Baniasadi, N., Bagheri Rafsanjani, A. M., Nikpoor, A. R., et al. (2016). Serum immune biomarkers in irritable bowel syndrome. Clin. Res. Hepatol. Gastroenterol. 40, 631–637. doi: 10.1016/j.clinre.2015.12.013
Sheehan, J., Gaman, A., Vangel, M., and Kuo, B. (2011). Pooled analysis of brain activity in irritable bowel syndrome and controls during rectal balloon distension. Neurogastroenterol. Motil. 23, 336–346. doi: 10.1111/j.1365-2982.2010.01635.x
Shen, J. H., Ye, Y. M., and Li, S. S. (2022). Acupuncture for diarrhea-predominant irritable bowel syndrome: a randomized control study. World J. Acupuncture-Moxibustion 32, 123–130. doi: 10.1016/j.wjam.2022.02.003
Simren, M., Mansson, A., Langkilde, A. M., Svedlund, J., Abrahamsson, H., Bengtsson, U., et al. (2001). Food-related gastrointestinal symptoms in the irritable bowel syndrome. Digestion. 63, 108–115. doi: 10.1159/000051878
Skaper, S. D., Facci, L., and Giusti, P. (2014). Mast cells, glia and neuroinflammation: partners in crime? Immunology. 141, 314–327. doi: 10.1111/imm.12170
Sperber, A. D., Bangdiwala, S. I., Drossman, D. A., Ghoshal, U. C., Simren, M., Tack, J., et al. (2021). Worldwide prevalence and burden of functional gastrointestinal disorders, results of Rome foundation global study. Gastroenterology. 160, 99–114.e3. doi: 10.1053/j.gastro.2020.04.014
Stasi, C., Rosselli, M., Bellini, M., Laffi, G., and Milani, S. (2012). Altered neuro-endocrine-immune pathways in the irritable bowel syndrome: the top-down and the bottom-up model. J. Gastroenterol. 47, 1177–1185. doi: 10.1007/s00535-012-0627-7
Sun, J., Wu, X., Meng, Y., Cheng, J., Ning, H., Peng, Y., et al. (2015). Electro-acupuncture decreases 5-HT, CGRP and increases NPY in the brain-gut axis in two rat models of diarrhea-predominant irritable bowel syndrome (D-IBS). BMC Complement. Altern. Med. 15, 340. doi: 10.1186/s12906-015-0863-5
Tache, Y., Larauche, M., Yuan, P. Q., and Million, M. (2018). Brain and gut CRF signaling: Biological actions and role in the gastrointestinal tract. Curr. Mol. Pharmacol. 11, 51–71. doi: 10.2174/1874467210666170224095741
Tanaka, F., Tominaga, K., Fujikawa, Y., Nagami, Y., Kamata, N., Yamagami, H., et al. (2016). Concentration of glial cell line-derived neurotrophic factor positively correlates with symptoms in functional dyspepsia. Dig. Dis. Sci. 61, 3478–3485. doi: 10.1007/s10620-016-4329-5
Thomas, C., Gioiello, A., Noriega, L., Strehle, A., Oury, J., Rizzo, G., et al. (2009). TGR5-mediated bile acid sensing controls glucose homeostasis. Cell Metab. 10, 167–177. doi: 10.1016/j.cmet.2009.08.001
Touny, A. A., Kenny, E., Månsson, M., Webb, D. L., and Hellström, P. M. (2022). Pain relief and pain intensity response to GLP-1 receptor agonist ROSE-010 in irritable bowel syndrome; clinical study cross-analysis with respect to patient characteristics. Scand. J. Gastroenterol. 57, 783–791. doi: 10.1080/00365521.2022.2041084
Uranga, J. A., Martínez, V., and Abalo, R. (2020). Mast cell regulation and irritable bowel syndrome: effects of food components with potential nutraceutical use. Molecules. 25, 4314. doi: 10.3390/molecules25184314
Van De Wouw, M., Boehme, M., Lyte, J. M., Wiley, N., Strain, C. O'sullivan, O., et al. (2018). Short-chain fatty acids: microbial metabolites that alleviate stress-induced brain-gut axis alterations. J. Physiol. 596, 4923–4944. doi: 10.1113/JP276431
Vicario, M., González-Castro, A. M., Martínez, C., Lobo, B., Pigrau, M., Guilarte, M., et al. (2015). Increased humoral immunity in the jejunum of diarrhoea-predominant irritable bowel syndrome associated with clinical manifestations. Gut. 64, 1379–1388. doi: 10.1136/gutjnl-2013-306236
Videlock, E. J., Shih, W., Adeyemo, M., Mahurkar-Joshi, S., Presson, A. P., Polytarchou, C., et al. (2016). The effect of sex and irritable bowel syndrome on HPA axis response and peripheral glucocorticoid receptor expression. Psychoneuroendocrinology 69, 67–76. doi: 10.1016/j.psyneuen.2016.03.016
Wiley, J. W., Higgins, G. A., and Athey, B. D. (2016). Stress and glucocorticoid receptor transcriptional programming in time and space: Implications for the brain-gut axis. Neurogastroenterol. Motil. 28, 12–25. doi: 10.1111/nmo.12706
Wood, J. D. (2020). Serotonergic integration in the intestinal mucosa. Curr. Pharm. Des. 26, 3010–3014. doi: 10.2174/1381612826666200612161542
Wouters, M. M., Vicario, M., and Santos, J. (2016). The role of mast cells in functional GI disorders. Gut. 65, 155–168. doi: 10.1136/gutjnl-2015-309151
Wu, I. X. Y., Wong, C. H. L., Ho, R. S. T., Cheung, W. K. W., Ford, A. C., Wu, J. C. Y., et al. (2019). Acupuncture and related therapies for treating irritable bowel syndrome: overview of systematic reviews and network meta-analysis. Therap. Adv. Gastroenterol. 12, 1756284818820438. doi: 10.1177/1756284818820438
Wu, Y., He, H., Cheng, Z., Bai, Y., and Ma, X. (2019). The role of neuropeptide Y and peptide YY in the development of obesity via gut-brain axis. Curr. Protein Pept. Sci. 20, 750–758. doi: 10.2174/1389203720666190125105401
Zahedi, M. J., Behrouz, V., and Azimi, M. (2018). Low fermentable oligo-di-mono-saccharides and polyols diet vs. general dietary advice in patients with diarrhea-predominant irritable bowel syndrome: a randomized controlled trial. J. Gastroenterol. Hepatol. 33, 1192–1199. doi: 10.1111/jgh.14051
Zhang, B., Ma, M. A., Rachmin, S., He, I., Baral, M., Choi, P. S., et al. (2020). Hyperactivation of sympathetic nerves drives depletion of melanocyte stem cells. Nature. 577, 676–681. doi: 10.1038/s41586-020-1935-3
Zhang, L., Song, J., and Hou, X. (2016). Mast cells and irritable bowel syndrome: from the bench to the bedside. J. Neurogastroenterol. Motil. 22, 181–192. doi: 10.5056/jnm15137
Zhang, L., Wang, R., Bai, T., Xiang, X., Qian, W., Song, J., et al. (2019). EphrinB2/ephB2-mediated myenteric synaptic plasticity: mechanisms underlying the persistent muscle hypercontractility and pain in post-infectious IBS. FASEB J. 33, 13644–13659. doi: 10.1096/fj.201901192R
Keywords: irritable bowel syndrome, brain-gut axis, neural signal regulation, endocrine system, immune system, acupuncture
Citation: Sun Z, Wang X, Feng S, Xie C, Xing Y, Guo L, Zhao J and Ji C (2023) A review of neuroendocrine immune system abnormalities in IBS based on the brain–gut axis and research progress of acupuncture intervention. Front. Neurosci. 17:934341. doi: 10.3389/fnins.2023.934341
Received: 02 May 2022; Accepted: 07 February 2023;
Published: 09 March 2023.
Edited by:
Chunhui Bao, Shanghai University of Traditional Chinese Medicine, ChinaReviewed by:
Na Liu, The Second Affiliated Hospital of Xi'an Jiaotong University, ChinaJirada Sringean, Chulalongkorn Centre of Excellence for Parkinson's Disease and Related Disorders, Thailand
Copyright © 2023 Sun, Wang, Feng, Xie, Xing, Guo, Zhao and Ji. This is an open-access article distributed under the terms of the Creative Commons Attribution License (CC BY). The use, distribution or reproduction in other forums is permitted, provided the original author(s) and the copyright owner(s) are credited and that the original publication in this journal is cited, in accordance with accepted academic practice. No use, distribution or reproduction is permitted which does not comply with these terms.
*Correspondence: Jingyu Zhao, amluZ3l1emhhbzIwMTBAMTYzLmNvbQ==; Changchun Ji, amljaGFuZ2NodW4xOTg0QDE2My5jb20=
†These authors have contributed equally to this work