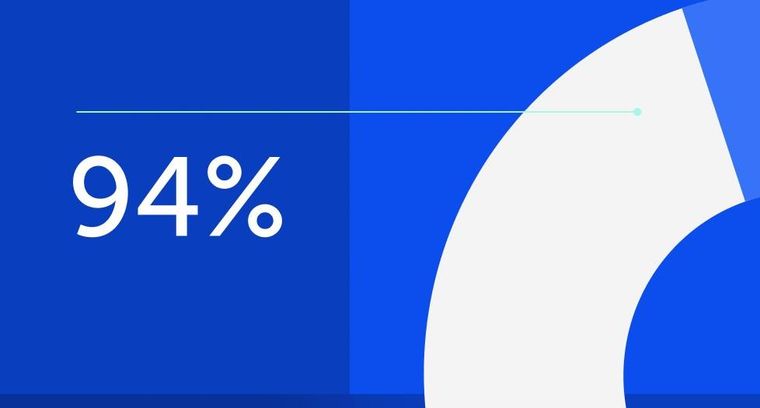
94% of researchers rate our articles as excellent or good
Learn more about the work of our research integrity team to safeguard the quality of each article we publish.
Find out more
EDITORIAL article
Front. Neurosci., 15 November 2023
Sec. Neurodegeneration
Volume 17 - 2023 | https://doi.org/10.3389/fnins.2023.1322091
This article is part of the Research TopicInsights into the use of Deep Brain Stimulation as a Treatment for Parkinson's Disease and Related Conditions.View all 5 articles
Editorial on the Research Topic
Insights into the use of deep brain stimulation as a treatment for Parkinson's disease and related conditions
Parkinson's Disease (PD), the second most prevalent progressive neurodegenerative disorder, manifests through motor symptoms, including resting tremors, rigidity, bradykinesia, and postural instability (Tolosa et al., 2021). Over the last 50 years, levodopa medication replacement therapy has reigned as the cornerstone of PD treatment (Romito et al., 2009). Nevertheless, as the disease advances, the medication's efficacy wanes, and extended use may precipitate complications such as dyskinesia and fluctuating responses. In the latter part of the 1990s, Benabid et al. uncovered that Deep Brain Stimulation (DBS) could alleviate PD's motor symptoms (Limousin et al., 1995). Owing to its minimally invasive, reversible, and adjustable nature, DBS has surfaced as an effective surgical intervention for PD. Recent years have ushered in notable advancements in both the fundamental research and clinical application of DBS. This editorial offers insights into employing DBS as a PD treatment.
The Subthalamic Nucleus (STN), recognized as the most prevalent DBS target in PD, enacts its therapeutic effects likely through widespread changes in neural activity across the brain. Animal research introduces nuances, allowing the activity of neuronal subpopulations to be selectively recorded and manipulated (Gittis and Yttri, 2018). With an optogenetic approach, researchers dissected out the excitatory afferents to STN alone, specifically fibers from the primary motor cortex, which is sufficient to induce therapeutic effects (Gradinaru et al., 2009). Nevertheless, electrophysiological observations during STN-DBS in monkeys revealed that the recruitment of cortex through antidromic signals diminished after 4 h of stimulation, without any accompanying reduction in therapeutic effectiveness (Johnson and Wang, 2020), implying that cortical activation is not a prerequisite for DBS's therapeutic efficacy. Conversely, focusing on local changes within the STN, the pathological firing patterns can also forecast the therapeutic efficacy of DBS (Dorval et al., 2010; Mcconnell et al., 2012, 2016). High-frequency local optogenetic stimulation (>75 Hz) that disrupts this firing pattern was adequate to enhance movement (Schor et al., 2022), while low-frequency or continuous stimulation, unable to alter the firing pattern, did not restore movement.
Historically, the significance of cell-type specificity in the pathological circuit has been marginally addressed in disease therapeutics. However, the cellular heterogeneity within the Globus Pallidus externa (GPe) has yielded intriguing findings. Interventions targeting distinct neuronal subgroups within the GPe manifest varied impacts on behavior (Cui et al., 2021; Isett et al., 2023). Optogenetic interventions that selectively elevated the activity of parvalbumin-expressing GPe neurons (PV-GPe) or suppressed the activity of lim homeobox 6-expressing GPe neurons (Lhx6-GPe) initiated a durable recovery of movement in bilaterally dopamine-depleted mice (Mastro and Zitelli, 2017). Spix et al. (2021) identified a frequency-dependent electrical stimulation paradigm to enact cell-type-specific neuromodulation in the GPe without resorting to optogenetics, with the therapeutic effect duration of this approach outpacing that achieved with traditional DBS protocols. While the frequency-dependent therapeutic effect has been clinically observed (Zapata Amaya et al.), further elucidation may arise from animal research.
Both STN and internal globus pallidus (GPi) stimulations have proven notably effective in ameliorating core motor symptoms such as tremors, rigidity, and bradykinesia in advanced-stage PD patients (Tsuboi et al., 2020; Park et al., 2022). Porta et al. found that administering DBS treatment prior to initiating L-Dopa treatment exerts beneficial effects on motor symptoms and quality of life in early-stage PD. Liu et al.'s research found that individual substantia nigra (SN) susceptibility features, derived from radiomics, can predict global motor and rigidity outcomes post-STN-DBS in PD patients. An ongoing debate persists regarding the efficacy of STN-DBS in ameliorating axial symptoms in PD, such as gait, swallowing, speech, and balance issues (Collomb-Clerc and Welter, 2015; Guimarães and Cury, 2022). A recent meta-analysis (Schlenstedt et al., 2017) revealed that STN-DBS indeed improved gait characteristics (such as stride length and speed) and alleviated the freezing of gait in patients within 48 months after electrode implantation during medication-off states. Furthermore, Navratilova et al.'s research has also found that, at late motor complications stages, DBS treatment in PD patients exhibits a positive effect on gait compared to dopaminergic treatment alone. Regarding non-motor symptoms, various studies (Witjas et al., 2007; Jost et al., 2023) have posited that STN-DBS could induce improvements in diverse non-motor symptom types.
Emerging research in Deep Brain Stimulation (DBS) for Parkinson's Disease (PD) predominantly focuses on precise electrode localization strategies, stimulation device development, and programming. Surgical robot intertwines image-guided navigation and robotic arm technology, offering a suite of advantages such as automated coordinate adjustments, minimizing manual operation deviations, reducing human error, and shortening the operation time (Jin et al., 2020). Owing to advancements in MRI visualization, scholars suggest that surgical plans might be automated through machine learning, utilizing purely anatomical information provided by MRI (Shamir et al., 2019). The deployment of intraoperative MRI (iMRI) facilitates real-time electrode adjustments during procedures, augmenting accuracy over traditional methodologies and bolstering mid-term therapeutic outcomes (Ostrem et al., 2013).
To attenuate adverse reactions spurred by the quasi-spherical activation domain of ring contact stimulation, directional leads have been devised, showcasing improved clinical outcomes (Fricke et al., 2021). Notably, 32-contact electrodes have not only demonstrated superior therapeutic effects relative to traditional electrodes but also the adeptness to record local field potentials (LFPs) from other contacts during stimulation (Contarino et al., 2014). When synchronized with adaptive DBS systems (aDBS) (An et al., 2023), β-oscillate as a biomarker was used for negative feedback regulation of stimulus parameters. aDBS showed broad therapeutic prospects, which was only used in STN-DBS.
Efforts in postoperative programming predominantly focus on STN-DBS, especially observing the therapeutic implications of varied parameters, such as low frequency, short pulse width, and frequency conversion. These parameters are designed to enhance therapeutic outcomes while averting adverse reactions through the meticulous adjustment of activation domains across diverse contacts (Dayal et al., 2020). Moreover, the onset of imaging-based programming, together with activated tissue volume estimations, might suggest optimal contacts and therapeutic thresholds, potentially making programming more efficient and time-economical (Neudorfer et al., 2023).
DBS maintains its pivotal role as a principal surgical method for effectively treating PD. Advancing research into PD neural networks continues to deepen our understanding of the neuroelectrophysiological mechanisms underpinning the disease, enhancing electrode implantation precision and, thus, amplifying DBS efficacy. However, there is still a pressing need to mitigate side effects, further amplify long-term therapeutic outcomes, and elevate programming efficiency. Hence, adaptive programming (An et al., 2023) based on electrophysiological biomarkers and automated computerized symptom assessment and programming (Shamir et al., 2015) are anticipated to gain momentum, dependent on sustained interdisciplinary collaboration and continual clinical trials.
ZL: Writing—original draft, Writing—review & editing. YZ: Writing—original draft, Writing—review & editing. YG: Writing—original draft, Writing—review & editing. XH: Writing—original draft, Writing—review & editing.
The author(s) declare financial support was received for the research, authorship, and/or publication of this article. This work was supported by the National Natural Science Foundation of Chongqing (cstc2021jcyj-msxm3744), Joint Medical Research Project of Chongqing Science and Technology Commission and Health Commission (2021MSXM262), and Beijing Postdoctoral Research Funding Project (2003-ZZ-002).
The authors declare that the research was conducted in the absence of any commercial or financial relationships that could be construed as a potential conflict of interest.
All claims expressed in this article are solely those of the authors and do not necessarily represent those of their affiliated organizations, or those of the publisher, the editors and the reviewers. Any product that may be evaluated in this article, or claim that may be made by its manufacturer, is not guaranteed or endorsed by the publisher.
An, Q., Yin, Z., Ma, R., Fan, H., Xu, Y., Gan, Y., et al. (2023). Adaptive deep brain stimulation for Parkinson's disease: looking back at the past decade on motor outcomes. J. Neurol. 270, 1371–1387. doi: 10.1007/s00415-022-11495-z
Collomb-Clerc, A., and Welter, M. L. (2015). Effects of deep brain stimulation on balance and gait in patients with Parkinson's disease: a systematic neurophysiological review. Neurophysiol. Clin. 45, 371–388. doi: 10.1016/j.neucli.2015.07.001
Contarino, M. F., Bour, L. J., Verhagen, R., Lourens, M. A., De Bie, R. M., Van Den Munckhof, P., et al. (2014). Directional steering: a novel approach to deep brain stimulation. Neurology 83, 1163–1169. doi: 10.1212/WNL.0000000000000823
Cui, Q., Du, X., Chang, I. Y. M., Pamukcu, A., Lilascharoen, V., and Berceau, B. L. (2021). Striatal direct pathway targets Npas1(+) pallidal neurons. J. Neurosci. 41, 3966–3987. doi: 10.1523/JNEUROSCI.2306-20.2021
Dayal, V., Grover, T., Tripoliti, E., Milabo, C., Salazar, M., Candelario-Mckeown, J., et al. (2020). Short versus conventional pulse-width deep brain stimulation in parkinson's disease: a randomized crossover comparison. Mov. Disorder 35, 101–108. doi: 10.1002/mds.27863
Dorval, A. D., Kuncel, A. M., Birdno, M. J., Turner, D. A., and Grill, W. M. (2010). Deep brain stimulation alleviates Parkinsonian bradykinesia by regularizing pallidal activity. J. Neurophysiol. 104, 911–921. doi: 10.1152/jn.00103.2010
Fricke, P., Nickl, R., Breun, M., Volkmann, J., Kirsch, D., Ernestus, R. I., et al. (2021). Directional leads for deep brain stimulation: technical notes and experiences. Stereot. Funct. Neuros 99, 305–312. doi: 10.1159/000512231
Gittis, A. H., and Yttri, E. A. (2018). Translating insights from optogenetics to therapies for Parkinson's disease. Curr. Opin. Biomed. Eng. 8, 14–19. doi: 10.1016/j.cobme.2018.08.008
Gradinaru, V., Mogri, M., Thompson, K. R., Henderson, J. M., and Deisseroth, K. (2009). Optical deconstruction of parkinsonian neural circuitry. Science 324, 354–359. doi: 10.1126/science.1167093
Guimarães, T. G., and Cury, R. G. (2022). Troubleshooting gait problems in Parkinson's disease patients with subthalamic nucleus deep brain stimulation. J. Parkinsons. Dis. 12, 737–741. doi: 10.3233/JPD-212771
Isett, B. R., Nguyen, K. P., Schwenk, J. C., Yurek, J. R., Snyder, C. N., Vounatsos, M. V., et al. (2023). The indirect pathway of the basal ganglia promotes transient punishment but not motor suppression. Neuron 111, 2218–2231.e2214. doi: 10.1016/j.neuron.2023.04.017
Jin, H., Gong, S., and Tao, Y. (2020). A comparative study of asleep and awake deep brain stimulation robot-assisted surgery for Parkinson's disease. npj Parkinson's Dis. 6, 27. doi: 10.1038/s41531-020-00130-1
Johnson, L. A., and Wang, J. (2020). Direct activation of primary motor cortex during subthalamic but not pallidal deep brain stimulation. J. Neurosci. 40, 2166–2177. doi: 10.1523/JNEUROSCI.2480-19.2020
Jost, S. T., Konitsioti, A., Loehrer, P. A., Ashkan, K., Rizos, A., Sauerbier, A., et al. (2023). Non-motor effects of deep brain stimulation in Parkinson's disease motor subtypes. Parkinson. Relat. Disord. 109, 105318. doi: 10.1016/j.parkreldis.2023.105318
Limousin, P., Pollak, P., Benazzouz, A., Hoffmann, D., Le Bas, J. F., Broussolle, E., et al. (1995). Effect of Parkinsonian signs and symptoms of bilateral subthalamic nucleus stimulation. Lancet 345, 91–95. doi: 10.1016/S0140-6736(95)90062-4
Mastro, K. J., and Zitelli, K. T. (2017). Cell-specific pallidal intervention induces long-lasting motor recovery in dopamine-depleted mice. Nat. Neurosci. 20, 815–823. doi: 10.1038/nn.4559
Mcconnell, G. C., So, R. Q., and Grill, W. M. (2016). Failure to suppress low-frequency neuronal oscillatory activity underlies the reduced effectiveness of random patterns of deep brain stimulation. J. Neurophysiol. 115, 2791–2802. doi: 10.1152/jn.00822.2015
Mcconnell, G. C., So, R. Q., Hilliard, J. D., Lopomo, P., and Grill, W. M. (2012). Effective deep brain stimulation suppresses low-frequency network oscillations in the basal ganglia by regularizing neural firing patterns. J. Neurosci. 32, 15657–15668. doi: 10.1523/JNEUROSCI.2824-12.2012
Neudorfer, C., Butenko, K., Oxenford, S., Rajamani, N., Achtzehn, J., Goede, L., et al. (2023). Lead-DBS v3.0: mapping deep brain stimulation effects to local anatomy and global networks. Neuroimage 268, 119862. doi: 10.1016/j.neuroimage.2023.119862
Ostrem, J. L., Galifianakis, N. B., Markun, L. C., Grace, J. K., Martin, A. J., Starr, P. A., et al. (2013). Clinical outcomes of PD patients having bilateral STN DBS using high-field interventional MR-imaging for lead placement. Clin. Neurol. Neurosurg. 115, 708–712. doi: 10.1016/j.clineuro.2012.08.019
Park, H. R., Im, H. J., Park, J., Yoon, B. W., Lim, Y. H., Song, E. J., et al. (2022). Long-term outcomes of bilateral subthalamic nucleus deep brain stimulation for patients with Parkinson's disease: 10 years and beyond. Neurosurgery 91, 726–733. doi: 10.1227/neu.0000000000002117
Romito, L. M., Contarino, M. F., Vanacore, N., Bentivoglio, A. R., Scerrati, M., and Albanese, A. (2009). Replacement of dopaminergic medication with subthalamic nucleus stimulation in Parkinson's disease: long-term observation. Mov. Disord. 24, 557–563. doi: 10.1002/mds.22390
Schlenstedt, C., Shalash, A., Muthuraman, M., Falk, D., Witt, K., and Deuschl, G. (2017). Effect of high-frequency subthalamic neurostimulation on gait and freezing of gait in Parkinson's disease: a systematic review and meta-analysis. Eur. J. Neurol. 24, 18–26. doi: 10.1111/ene.13167
Schor, J. S., Gonzalez Montalvo, I., Spratt, P. W. E., Brakaj, R. J., Stansil, J. A., Twedell, E. L., et al. (2022). Therapeutic deep brain stimulation disrupts movement-related subthalamic nucleus activity in parkinsonian mice. eLife 11, e75253. doi: 10.7554/eLife.75253.sa2
Shamir, R. R., Dolber, T., Noecker, A. M., Walter, B. L., and Mcintyre, C. C. (2015). Machine learning approach to optimizing combined stimulation and medication therapies for Parkinson's disease. Brain Stimul. 8, 1025–1032. doi: 10.1016/j.brs.2015.06.003
Shamir, R. R., Duchin, Y., Kim, J., Patriat, R., Marmor, O., Bergman, H., et al. (2019). Microelectrode recordings validate the clinical visualization of subthalamic-nucleus based on 7T magnetic resonance imaging and machine learning for deep brain stimulation surgery. Neurosurgery 84, 749–757. doi: 10.1093/neuros/nyy212
Spix, T. A., Nanivadekar, S., and Toong, N. (2021). Population-specific neuromodulation prolongs therapeutic benefits of deep brain stimulation. Science 374, 201–206. doi: 10.1126/science.abi7852
Tolosa, E., Garrido, A., Scholz, S. W., and Poewe, W. (2021). Challenges in the diagnosis of Parkinson's disease. Lancet Neurol. 20, 385–397. doi: 10.1016/S1474-4422(21)00030-2
Tsuboi, T., Lemos Melo Lobo Jofili Lopes, J., Moore, K., Patel, B., Legacy, J., Ratajska, A. M., et al. (2020). Long-term clinical outcomes of bilateral GPi deep brain stimulation in advanced Parkinson's disease: 5 years and beyond. J. Neurosurg. 9, 1–10. doi: 10.3171/2020.6.JNS20617
Keywords: deep brain stimulation, Parkinson's disease, gait, dyskinesia, akinesia
Citation: Liu Z, Zhou Y, Gao Y and Hu X (2023) Editorial: Insights into the use of deep brain stimulation as a treatment for Parkinson's disease and related conditions. Front. Neurosci. 17:1322091. doi: 10.3389/fnins.2023.1322091
Received: 15 October 2023; Accepted: 01 November 2023;
Published: 15 November 2023.
Edited and reviewed by: Wendy Noble, University of Exeter, United Kingdom
Copyright © 2023 Liu, Zhou, Gao and Hu. This is an open-access article distributed under the terms of the Creative Commons Attribution License (CC BY). The use, distribution or reproduction in other forums is permitted, provided the original author(s) and the copyright owner(s) are credited and that the original publication in this journal is cited, in accordance with accepted academic practice. No use, distribution or reproduction is permitted which does not comply with these terms.
*Correspondence: Xiaofei Hu, aGFycnl6b25ldG1tdUAxNjMuY29t
Disclaimer: All claims expressed in this article are solely those of the authors and do not necessarily represent those of their affiliated organizations, or those of the publisher, the editors and the reviewers. Any product that may be evaluated in this article or claim that may be made by its manufacturer is not guaranteed or endorsed by the publisher.
Research integrity at Frontiers
Learn more about the work of our research integrity team to safeguard the quality of each article we publish.