- 1Department of Otolaryngology-Head and Neck Surgery, the Second Affiliated Hospital of Anhui Medical University, Hefei, Anhui, China
- 2School of Life Sciences and Technology, Southeast University, Nanjing, China
- 3Department of Otolaryngology-Head and Neck Surgery, The First Affiliated Hospital of Soochow University, Suzhou, Jiangsu, China
- 4Department of Otolaryngology-Head and Neck Surgery, The First Affiliated Hospital of USTC, Division of Life Sciences and Medicine, University of Science and Technology of China, Hefei, Anhui, China
Hearing impairment is a global health problem. Stem cell therapy has become a cutting-edge approach to tissue regeneration. In this review, the recent advances in stem cell therapy for hearing loss have been discussed. Nanomaterials can modulate the stem cell microenvironment to augment the therapeutic effects further. The potential of combining nanomaterials with stem cells for repairing and regenerating damaged inner ear hair cells (HCs) and spiral ganglion neurons (SGNs) has also been discussed. Stem cell-derived exosomes can contribute to the repair and regeneration of damaged tissue, and the research progress on exosome-based hearing loss treatment has been summarized as well. Despite stem cell therapy’s technical and practical limitations, the findings reported so far are promising and warrant further investigation for eventual clinical translation.
Introduction
Hearing impairment is one of the most prevalent sensory disorders worldwide, affecting millions. Hearing aids and cochlear implants cannot restore normal hearing, warranting new therapeutic approaches (Lieu et al., 2020). Stem cell therapy has gained considerable attention over the years due to its substantial regenerative potential.
Depending on the location of the damage in the auditory system, deafness is divided into conductive and sensorineural types (Seddon et al., 2012). Conductive deafness occurs due to lesions in the tympanic membrane and the auditory tuberosity, which impede sound transmission to the inner ear (Lauer et al., 2019). On the other hand, sensorineural deafness is mainly the result of lesions in the auditory center, including the inner ear and the auditory nerve. HCs and SGNs are crucial in transmitting peripheral acoustic signals (Nayagam et al., 2011; Moser and Starr, 2016). However, mammalian cochlear HCs do not regenerate spontaneously after injury (Swan et al., 2008; Omichi et al., 2019). Causes of sensorineural deafness include noise, aging, drug cause hearing loss, genetics, bacterial and viral infections, immunological diseases, and endolymph fluid (Meniere’s disease) (Wang and Puel, 2018; Plontke et al., 2022). Currently, induction of stem cell differentiation and replacement of damaged HCs and SGNs are increasingly considered feasible treatment options for auditory regeneration.
Historical overview of stem cell research
The origin of stem cell therapy dates back to 1888. when German zoologists Theodor Heinrich Boveri and Valentin Haecker introduced the concept of stem cells, they identified various cell populations in the embryo that could differentiate into specific cell types (Ramalho-Santos and Willenbring, 2007). In 1961, Till and Mc (1961) discovered that stem cells obtained from mouse bone marrow cells, which could differentiate into various cell types, and termed pluripotent stem cells (PSCs). Reynolds and Weiss (1992) isolated pluripotent neural stem cells (NSCs) from the forebrain of adult mammals in 1992. Thomson et al. (1998) first isolated human embryonic stem cells (hESCs) from embryos in 1998. In 1999, Pittenger et al. showed that bone marrow-derived human adult mesenchymal stem cells (BM-MSCs) can differentiate into multiple cell types, thus demonstrating the pluripotency of adult stem cells (ASCs) in vitro. BM-MSCs exist in almost all tissues and are crucial for maintaining tissue homeostasis through their self-renewal capacity (Pittenger et al., 1999; Tuan et al., 2003). Huawei et al. identified PSCs in the inner ear of adult mice and found that these cells could self-renew and differentiate into HC-like cells (HCLs) when cultured in vitro 16 (Li et al., 2003a). Takahashi and Yamanaka used the four transcription factors Oct3/4, Sox2, c-Myc, and KLF4 to transform mouse fibroblasts into induced pluripotent stem cells (iPSCs) for the first time (Takahashi and Yamanaka, 2006; Takahashi et al., 2007). This groundbreaking 2006 study paved the way for reprogramming mature somatic cells into a pluripotent state and opened new avenues for stem cell research. For this discovery, Shinya Yamanaka and John Gurdon received the Nobel Prize in Physiology or Medicine in 2012 (Figure 1) (Johnson and Cohen, 2012). Over the past decade, stem cell-based therapies have garnered considerable attention in hearing loss treatment.
The diversity of stem cells in regenerative medicine
Stem cells are a group of undifferentiated cells that can self-renew and differentiate into one or more cell types at different times of life (Ho et al., 2012; Hao et al., 2020). Based on their origin, stem cells can be categorized into various types, such as embryonic stem cells (ESCs), iPSCs, adult or somatic stem cells, and NSCs (Bongso and Richards, 2004; Ilic and Polak, 2011; Bond et al., 2015).
ESCs are pluripotent stem cells derived from the inner cell mass of blastocysts formed 5–6 days after fertilization (Evans and Kaufman, 1981). All three ectoderm, mesoderm, and endoderm germ layers can be differentiated from ESCs (Yao et al., 2006). ESCs can be obtained by culturing inner cell masses isolated from trophoblasts under specific conditions (Bongso, 2006).
IPSCs are produced by reprogramming mature somatic cells into ESC-like cells through genetic or chemical intervention (Yamanaka, 2012; Hockemeyer and Jaenisch, 2016). IPSCs are suitable models for investigating disease treatment, drug discovery, and regenerative medicine because they can self-renew and differentiate into various cell types (Ohnuki and Takahashi, 2015). Somatic cells can be reprogrammed to iPSCs by transducing them with the Oct4, Sox2, Klf4, and c-Myc transcription factors (Takahashi et al., 2007). In addition, certain chemicals or microenvironmental factors have also been used to stimulate the generation of iPSCs.
Adult or somatic stem cells are undifferentiated cells derived from various adult tissues with pluripotency, self-renewal, and limited differentiation potential (Zakrzewski et al., 2019). MSCs are the most common adult or somatic stem cells. Among them, BM-MSCs have limited differentiation capacity for osteocytes, chondrocytes, and adipocytes (Caplan, 2010). Although their differentiation capacity is limited, they exhibit anti-inflammatory properties and augment tissue regeneration (Ilancheran et al., 2009; Moodley et al., 2010).
NSCs have remarkable self-renewal and differentiation capabilities and continuously generate new neurons and glial cells (Xing et al., 2021). They play crucial roles in embryonic development and post-natal growth, particularly in the brain and spinal cord, wherein they help maintain neural tissue homeostasis and regenerative capacity (Shao et al., 2019). NSCs are also the seed cells for neural stem cell therapy and can promote nerve regeneration and restore function when implanted into damaged nerve tissue (Wang et al., 2019). The clinical applicability of NSCs is constantly being explored for treating neurological diseases.
Stem cell therapy for hearing impairment
Due to their capacity to differentiate into numerous cell types and repair tissues that have been damaged, stem cells may offer a promising treatment option for hearing loss (Bacakova et al., 2018; Camp et al., 2018). The ESCs, iPSCs, and ASCs have been tested for treating hearing impairment (Boer et al., 2009; Stojkovic et al., 2021; Zine et al., 2021). Nevertheless, each variety has advantages and disadvantages concerning differentiated future potential applicability and immunogenicity (Figure 2) (He et al., 2021). In hearing loss research, stem cells have successfully generated HCLs in vitro (Li et al., 2003b; Takeda et al., 2018).
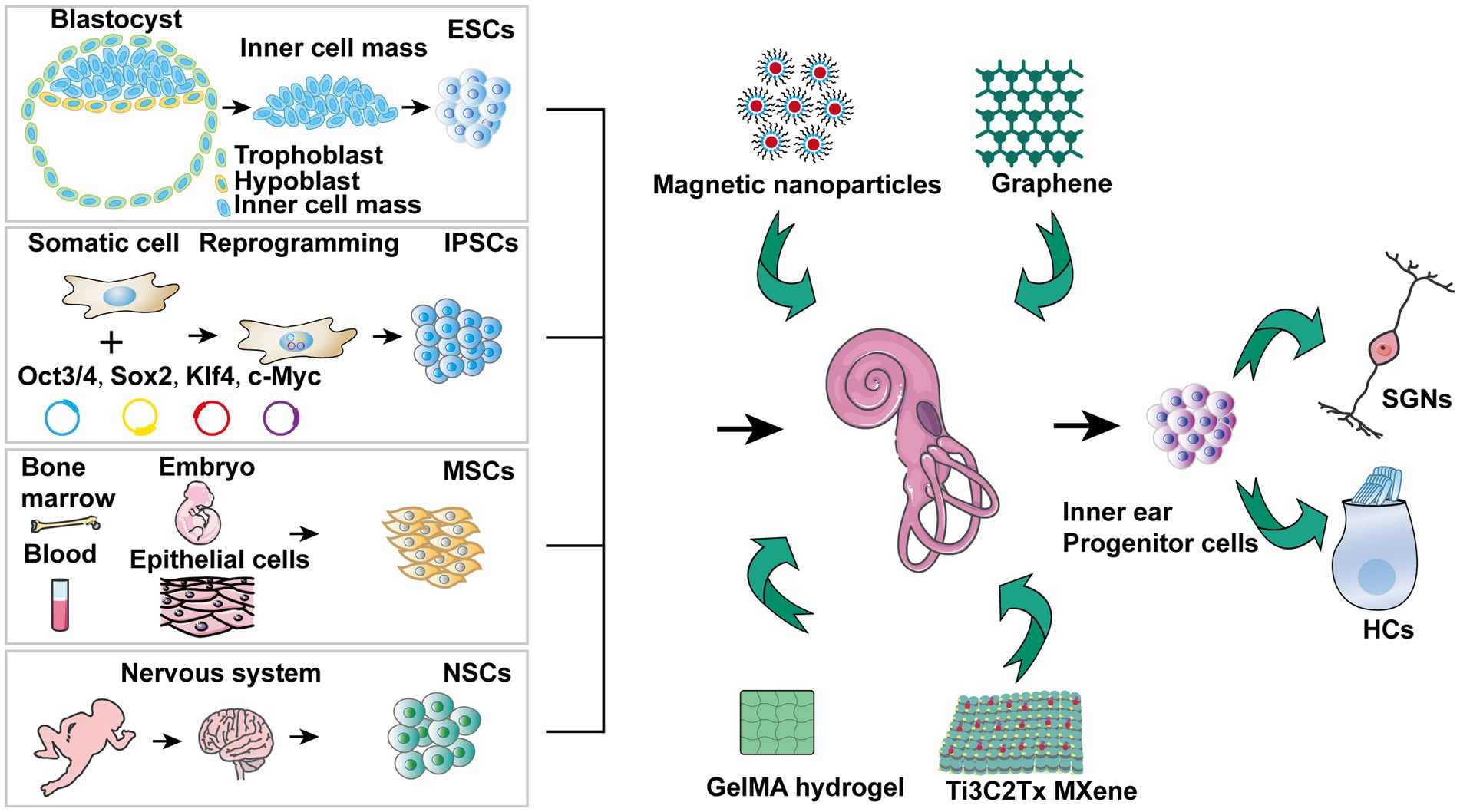
Figure 2. Stem cell treatment for hearing impairment mechanisms. 1) ESCs are able to differentiate into that resemble SGNs, SCs, and HCs, offering potential replacement strategies. 2) A particular strategy can be realized by stimulating iPSCs from patients who have hearing loss for developing into HCLs. 3) A range of growth factors and cytokines are secreted by MSCs, which may help prevent hearing loss. 4) NSCs together with nanomaterials hold promise for protecting against hearing loss. 5) The inner ear precursor cells are capable of being stimulated to develop into HCs and SGNs, offering another avenue for regeneration. 6) Exosomes derived from stem cells demonstrate potential in preventing sensorineural hearing loss.
ESCs for treating hearing loss
Recent studies have shown that hESCs can be differentiated in vitro into cochlear sensory epithelial cells containing HCs using a three-dimensional culture system (Koehler et al., 2013). In addition, hESCs have also been differentiated into purified ear nerve precursor cells and spiral ganglion-like cells, which can survive for extended periods in vitro (Matsuoka et al., 2017). hESC-derived precursor cells transplanted into the cochlear region of Pou4f3DTR/+ mice with selective diphtheria toxin-induced HC ablation were viable and differentiated into HC-like and SC-like cells (Takeda et al., 2021). These findings suggest hESCs may be a potential treatment for hearing impairment and warrant further investigation.
IPSCs for the treatment of deafness
Recently, iPSCs have become known as a potential biological treatment for deafness. IPSCs derived from human urinary cells obtained from donors in good health were differentiated into HCLs with the morphological and electrophysiological characteristics of inner ear HCs. These HCLs established synaptic connections with the SGNs that were co-cultured. In addition, the transplanted iPSCs migrated to the organ of Corti site of resident HCs, differentiated into HCLs, and established synaptic links with the native SGNs (Chen J. et al., 2018). Somatic cells from patients with myosin7a and myosin15a mutations were also reprogrammed into iPSCs, and the mutations were corrected using gene editing techniques. Restoring gene function in iPSCs enabled differentiated HCLs to regain morphology and functioning (Chen et al., 2016; Tang et al., 2016). Similarly, iPSCs derived from patients with A8344G and trmu mutations in mitochondrial DNA were differentiated into inner ear HCLs. These cells exhibited normal electrophysiological properties after gene restoration (Chen Y. C. et al., 2018; Chen and Guan, 2022). These cellular models can elucidate the functional connection between inner ear HCs development and mitochondrial DNA. Additionally, human iPSCs derived from skin cells of patients with connexin 26 mutations, encoded by GJB2, were differentiated into auditory neural progenitor and hair cell precursor cells (Fukunaga et al., 2021). Connexin 26 mutations are a common cause of hereditary deafness. Overall, these findings provide novel insights and highlight potential therapeutic uses of iPSCs for treating hearing loss.
The therapeutic potential of MSCs for hearing loss
Although ESCs and iPSCs can differentiate into inner ear HCs, their application in medicine is limited due to the risk of tumorigenicity. Direct reprogramming of fibroblasts into HCLs of the inner ear could be a viable alternative. Mouse Embryonic Fibroblasts can be differentiated into HCLs via mesenchymal-to-epithelial transition, followed by increased the expression of three important transcription factors, Sox2, Eya1 and Six1, to induce ear-sensory epithelial cell characteristics (Yang et al., 2021). Conductive hearing loss is commonly caused by cerumen embolism and chronic otitis media, leading to perforation of the tympanic membrane and erosion of the auditory ossicles due to recurrent infections. While the tympanic membrane can be regenerated using fascia or perichondrium, stem cells are essential for effectively enhancing hearing (Goncalves et al., 2017; Maharajan et al., 2020). In the rat model of subacute tympanic membrane perforation, bioprinted polycaprolactone/collagen/alginate-mesenchymal stem cell scaffolds have demonstrated efficacy and feasibility for subacute tympanic membrane regeneration (Jang et al., 2017). BM-MSCs have also been shown to promote healing in a chronic tympanic membrane perforation rat model (Shahal et al., 2022). Other ossicles or cartilage may be utilized to surgery restore hearing in cases of bone erosion. Additionally, MSCs have demonstrated promise in the therapy of conductive hearing loss (Maharajan et al., 2020). The resident MSCs protect the cochlear epithelium and prevent noise-induced hearing damage by secreting various growth factors and cytokines (Warnecke et al., 2021a). Moreover, pre-treatment of MSCs with deferoxamine can enhance their homing ability, which refers to the migration ability to damaged sites, through activation of the PI3K/AKT signaling pathway (Peyvandi et al., 2018).
NSCs for hearing loss
There have been considerable efforts in recent years to treat sensorineural hearing loss by inducing the regeneration of damaged auditory HCs and SGNs (Wang and Puel, 2018; Shu et al., 2019). The combination of nanomaterials and stem cells is a promising new therapeutic approach against hearing loss that combines the proliferation capacity of the stem cells with the tissue-targeting ability of the nanocarriers (Chang et al., 2020; Zhang et al., 2022). Several studies have demonstrated that stem cells and nanomaterials can support auditory regeneration by accelerating the repairing of damaged tissues (Zhong et al., 2016; Hu et al., 2021).
Graphene, a single layer of carbon atoms arranged in a hexagonal lattice, has been shown to play a critical role in tissue reconstruction (Kim et al., 2013; Akhavan, 2016; Guo et al., 2016). Autologous tissue grafts of perforated tympanic membranes can restore low-frequency hearing but often impair high-frequency hearing. In a rat deafness model, thin multilayer graphene membranes restored broadband hearing by inducing tympanic membrane repair (Li C. et al., 2022). In addition, the electrical stimulation device was developed by the combination of a cochlear implant and NSCs cultured on a graphene substrate. The machine was biocompatible and induced regeneration of NSCs in response to high-frequency, high-amplitude electroacoustic stimulation (Guo et al., 2021).
Magnetic nanoparticles are widely used in biomedical applications such as magnetic labeling, magnetic imaging, tumor treatment, and drug delivery due to their good biocompatibility (Lin et al., 2021; de Vincentiis et al., 2023). Superparamagnetic iron oxide nanoparticles can promote the proliferation of NSCs in a static magnetic field by enhancing cell cycle progression (Li et al., 2021). Furthermore, the directed growth of cochlear spiral neurons can be regulated by magnetic field-induced self-assembly of magnetic nanoparticles into multi-directional nanowires (Xia et al., 2022).
GelMA hydrogel is synthesized from methacrylic anhydride (MA) and gelatin. It is an ideal scaffold for 3D cell culture, tissue engineering, and biological 3D printing due to its excellent biocompatibility and visible light-curing properties (Fan et al., 2018; Cai C. et al., 2022). Composite scaffolds of super-aligned carbon nanotubes and GelMA promote the SGNs growth and orientation (Hu et al., 2022). Grooved GelMA-MXene enhanced the adhesion, differentiation, and directed proliferation of NSCs in vitro (Cai J. et al., 2022). Ti3C2Tx MXene, composed of transition metals, carbides, nitrides, or carbonitrides, exhibits a large surface area, adjustable surface functional groups, and good electrical conductivity (Rasool et al., 2016; Wu et al., 2021; Serles et al., 2022). It can enhance the proliferation and neural differentiation of NSCs, and promote the development of SGN growth cones and neurite growth by delivering electrical stimuli (Guo et al., 2022; Liao et al., 2022; Li Y. et al., 2022).
Inner ear progenitors for auditory regeneration
HCs and supporting cells (SCs) are critical inner ear components that arise from a common sensory progenitor. Inner ear progenitor cells are pluripotent cells with self-renewal ability that can differentiate into HCs under suitable induction conditions. During embryonic development, signaling pathway regulation plays vital roles in the formation of the organ of Corti. Activation of the Wnt pathway and inhibition of the Notch pathway promote partial regeneration of HCs (Mizutari et al., 2013; Shi et al., 2014; Li et al., 2015). Lgr5, a receptor of the Wnt pathway, is also a marker of cochlear stem cells (Chai et al., 2012; Shi et al., 2013; Bramhall et al., 2014). Under specific conditions, Lgr5-expressing Sertoli cells can transdifferentiate into HCs postnatally (McLean et al., 2017). Additionally, Sox2 is crucial for cell division and differentiation during development. Inner ear epithelial cells of Sox2 haploinsufficient mice showed increased differentiation and proliferation, resulting in expanded HCs and SCs and eventual regeneration of cochlear function. Sox2 haploinsufficiency also activates the cochlear Wnt pathway, further enhancing regeneration (Atkinson et al., 2018; Steevens et al., 2019).
The let-7 microRNA is a conserved activator that promotes proliferative quiescence and terminal differentiation by repressing CHD7, which controls progenitor cell behavior during cochlear development. Inhibition of let-7 in chicken auditory organ slices prolonged pre-sensory cell differentiation and proliferation (Evsen et al., 2020; Nie et al., 2022). In mice, the RNA-binding protein LIN28B promotes HC generation from auditory SCs via the mTOR pathway during embryonic development (Li and Doetzlhofer, 2020). The Yap-Lin28a axis can also activate Wnt signaling and promote inner ear cell regeneration by inhibiting let-7 expression (Kempfle et al., 2020; Ye et al., 2020). Knockdown of Foxg1 in neonatal mouse SCs promoted their transdifferentiation into HCs (Zhang et al., 2020). Furthermore, the Yap/Tead complex regulates a proliferation gene network in cochlear progenitors. Tead transcription factors directly bind regulatory elements of stem cell and cell cycle genes. In Sox2-positive cells, Yap as a Tead activator is rapidly degraded (Gnedeva et al., 2020; Currey et al., 2021). The transcriptional repressors TBX2 and TBX3 play essential roles in cochlear morphogenesis (García-Añoveros et al., 2022; Kaiser et al., 2022). Loss of Tbx2 causes cochlear hypoplasia, while Tbx3 mutants exhibit inner ear morphogenesis defects (Vitelli et al., 2003; Kaiser et al., 2021; Bi et al., 2022; Kaiser et al., 2022). The transcription factor ATOH1 promotes HC differentiation by upregulating Pou4f3, which facilitates ATOH1 binding and activation of other target genes (Yu et al., 2021; Costa et al., 2022). As a transcriptional activator of Sonic hedgehog (Shh), Gli2 is negatively regulated by Suppressor of Fused Homolog (Sufu). Controlling Gli2 is critical for regulating cochlear HC differentiation, as Sufu inhibition can disrupt Atoh1 expression and delay differentiation (Yin et al., 2019; Qin et al., 2022). Overexpression of Rps14 in the mouse cochlea promotes SC proliferation by activating Wnt signaling and inducing HC regeneration (Xu et al., 2023). These studies show that co-regulation of the Wnt, Notch and Shh pathways promotes HCs regeneration, and provides a new insight for the potential application of HC regeneration.
Stem cell-derived exosomes have broad therapeutic prospects in hearing impairment
A class of small extracellular vesicles called exosomes that diameters ranging between 30 and 150 nm (Kalluri and LeBleu, 2020). Many cells, such as immune cells, cancer cells, and stem cells, can secrete exosomes (Yang et al., 2019; Cully, 2021). Exosomes derived from various cell types are highly heterogeneous. However, stem cell-derived exosomes have multiple mechanisms for repairing tissue damage, including promotion of cell proliferation and survival, enhancement of angiogenesis, and inhibition of inflammation and oxidation. For example, exosomes secreted by adipose-derived mesenchymal stem cells that are enriched in miR-25-3p induced neuroprotection through activation of autophagic flux (Kuang et al., 2020). The formation of exosomes through the endocytic pathway includes the following process: cytoplasmic membrane invagination, encapsulating some extracellular components and cell membrane proteins to form early endosomes (ESEs), followed by fusion between different ESEs to form late endosomes (LSEs), and further formation of multivesicular bodies (MVBs) (Chang et al., 2021). MVBs contain many intraluminal vesicles (ILVs) that may be released into exosomes (Han et al., 2022). MVBs are degraded by fusion with lysosomes or by fusing with the plasma membrane, releasing their substances, including ILVs, which are the final exosomes (Figure 3) (Kumar et al., 2020).
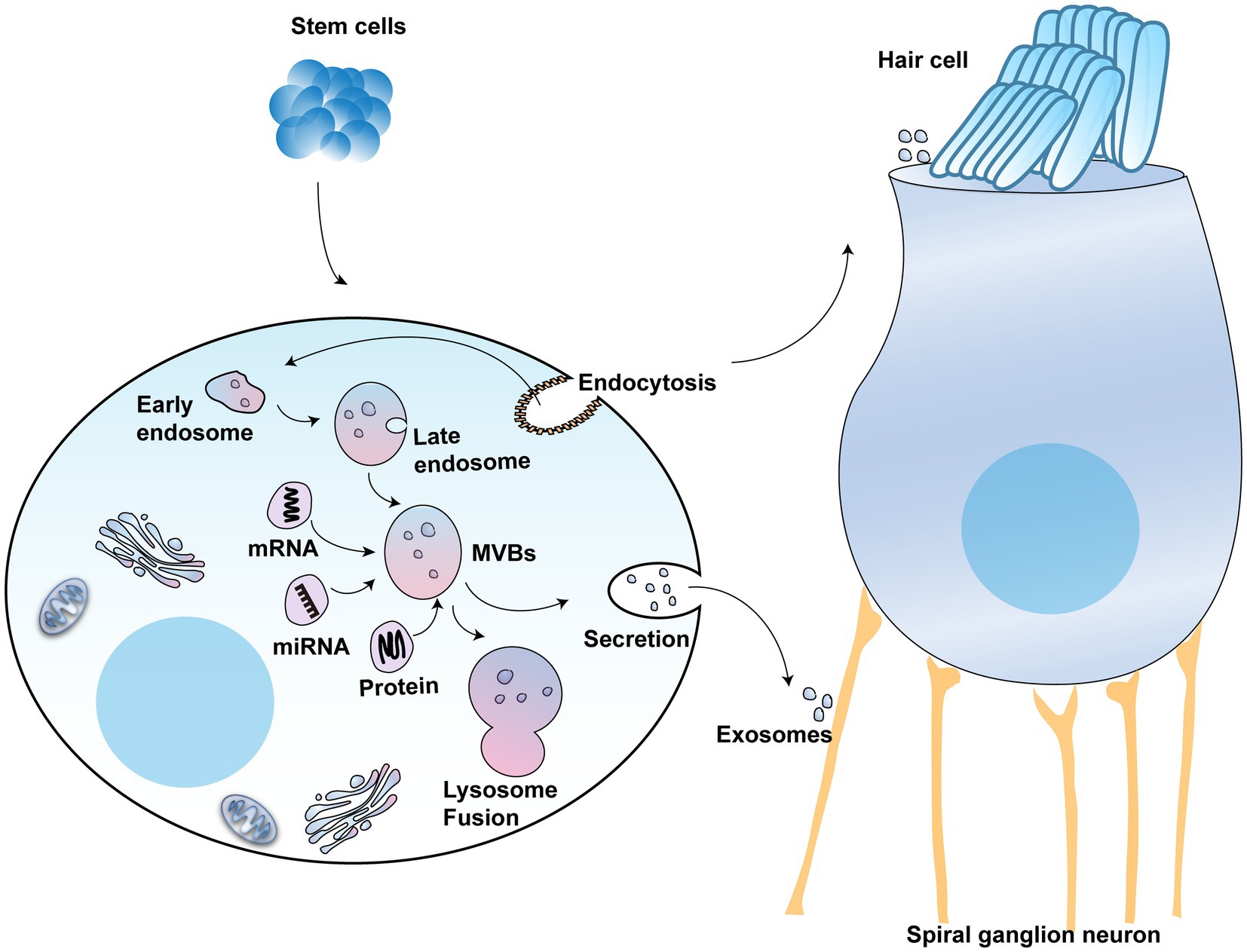
Figure 3. The potential use of exosomes in hearing protection: The schematic model shows the molecular composition of exosomes formed from stem cells, which include a wide range of cargo molecules, such as DNAs, RNAs, and proteins. Exosomes develop when the lipid bilayer membrane of MVBs undergoes an inward budding process. These MVBs can either be degraded by lysosomes or combined with the plasma membrane, releasing exosomes.
Exosomes deliver the vesicle’s load, such as lipids, proteins, and other molecules, to the destination cells (Sun et al., 2020). Studies show that these exosomes can promote the regeneration of damaged tissues, modulate cellular immune responses, and reduce cellular inflammatory responses by activating specific signaling pathways (Dai et al., 2020; Ocansey et al., 2020; Xu et al., 2020; Cao et al., 2021; Isaac et al., 2021). Exosomes derived from stem cells can help regenerate neurons and synapses, alleviating the symptoms of neurodegenerative disorders (Vogel et al., 2018; Riazifar et al., 2019; Guo et al., 2020; Fayazi et al., 2021). Moreover, exosomes play an important role in cochlear sensory HCs protection. After stress stimulation, the cochlear SCs can release exosomes containing heat shock protein 70 (HSP70). To prevent the death of HCs, HSP70 takes a paracrine method to act on toll-like receptor 4 (TLR4) (Breglio et al., 2020; Muller, 2020). Another study showed that extracellular vesicles from human vestibular schwannomas are able to damage cochlear HCs and SGNs, leading to hearing loss (Soares et al., 2016).
Exosomes have also been demonstrated to protect against drug-induced hearing loss. For example, in response to cisplatin and other drugs via the HSP70 pathway, exosomes secreted by BM-MSCs reduced the apoptosis of mouse cochlear HCs (Park et al., 2021). Furthermore, human MSCs were able to regenerate SGNs and restore hearing in mice with autoimmune sensorineural deafness induced by β-tubulin through paracrine activity (Tsai et al., 2021). Furthermore, human MSCs were able to regenerate SGNs and restore hearing in mice with autoimmune sensorineural deafness induced by β-tubulin through paracrine activity (Yoo et al., 2015). Human MSC-derived extracellular vesicles also protect against noise-induced deafness in mice (Warnecke et al., 2020; Huang et al., 2023).
Clinical trials of stem cell therapy for deafness
Although multiple cellular and animal studies have demonstrated the security and feasibility of stem cell treatment for deafness, stem cell-based clinical trials for deafness treatment are still scarce. Alpha mannosidase deficiency is a rare genetic disorder that can lead to multi-organ dysfunction and cognitive deficits. One clinical study showed that five patients with α-mannosidase deficiency significantly improved their symptoms after transplantation of the allogeneic hematopoietic stem cells (Grewal et al., 2004). Blood cells, nerve cells, and cardiomyocytes can differentiate from umbilical cord stem cells. Studies have shown that after transplanting stem cells from autologous cord blood, auditory function is restored in children with acquired sensorineural hearing loss (Baumgartner et al., 2018; Sun and Yang, 2020). miR-22-3p, a microRNA relatively highly expressed in mesenchymal stem cell-derived exosomes, reduces inflammation by inhibiting expression of NLRP3. Additionally, mesenchymal stem cell-derived exosomes significantly inhibit expression of the pro-inflammatory factors TNF-α, IL-1β, and iNOS while promoting expression of the anti-inflammatory factor IL-10, thereby suppressing inflammation (Liu et al., 2020; Wang et al., 2023). In a clinical trial concluded in 2021, human umbilical cord MSCs-derived extracellular capsules were transplanted into the inner ear, reducing the inflammatory side effects caused by cochlear implantation (Warnecke et al., 2021b).
Conclusion
This review discusses the present status of the use of MSCs, ESCs, iPSCs, inner ear progenitor cells, and NSCs in the repair and regeneration of auditory impairment. MSCs are easily accessible and expandable and are, therefore, the most commonly used stem cell type. ESCs and iPSCs have strong differentiation potential, but their clinical application is limited due to ethical and safety concerns. Cells that can differentiate into cochlear HC and spiral neurons are inner ear progenitor cells, a type of ASCs. Although several preclinical and clinical studies have proved the therapeutic potential of stem cells in auditory impairment, Stem cell therapy also has some significant limitations, such as safety and feasibility. Specifically speaking, stem cell transplantation carries risk of tumourigenesis and immune rejection after transplantation, and existing delivery methods for stem cells can affect their therapeutic efficiency. In addition, the ethical issues also need to be addressed. In the future, the source of stem cells and the time and cell dosage for treatment will be optimized, More and more superior biomaterials and targeted delivery modalities will be developed. Overall, stem cell therapy is a brilliant way to restore hearing loss.
Author contributions
QF: Conceptualization, Writing – original draft. YW: Data curation, Writing – original draft. YuZ: Formal analysis, Writing – original draft, Data curation. WC: Resources, Writing – original draft, Formal analysis. LY: Writing – original draft, Funding acquisition. MK: Writing – original draft, Investigation. YoZ: Formal analysis, Writing – original draft, Methodology. YX: Writing – original draft, Project administration. LG: Writing – original draft, Resources. LZ: Project administration, Resources, Writing – original draft, Software. WW: Writing – original draft, Supervision. YY: Funding acquisition, Supervision, Writing – review & editing. JS: Funding acquisition, Validation, Writing – review & editing, Supervision. JY: Funding acquisition, Supervision, Writing – review & editing, Writing – original draft, Validation.
Funding
The author(s) declare financial support was received for the research, authorship, and/or publication of this article. The writing of this manuscript was supported by the National Natural Science Foundation of China (82071055, 82271178, and 82271162), the Anhui Province Scientific Research Preparation Plan Project (2022AH050685, 2022AH050655), the Anhui Natural Science Foundation of China (2208085MH223), the Second Affiliated Hospital of Anhui Medical University National Natural Science Foundation Incubation Program (2022GMFY04), Anhui Province Key Specialties Construction Project in Medical and Health Care, the Project of State Key Laboratory of Radiation Medicine and Protection, Soochow University (GZK 1202209).
Acknowledgments
The authors thank TopEdit (www.topeditsci.com) for the language editing assistance provided during the preparation of this manuscript.
Conflict of interest
The authors declare that the research was conducted in the absence of any commercial or financial relationships that could be construed as a potential conflict of interest.
Publisher’s note
All claims expressed in this article are solely those of the authors and do not necessarily represent those of their affiliated organizations, or those of the publisher, the editors and the reviewers. Any product that may be evaluated in this article, or claim that may be made by its manufacturer, is not guaranteed or endorsed by the publisher.
References
Akhavan, O. (2016). Graphene scaffolds in progressive nanotechnology/stem cell-based tissue engineering of the nervous system. J. Mater. Chem. B 4, 3169–3190. doi: 10.1039/C6TB00152A
Atkinson, P. J., Dong, Y., Gu, S., Liu, W., Najarro, E. H., Udagawa, T., et al. (2018). Sox2 haploinsufficiency primes regeneration and Wnt responsiveness in the mouse cochlea. J. Clin. Invest. 128, 1641–1656. doi: 10.1172/JCI97248
Bacakova, L., Zarubova, J., Travnickova, M., Musilkova, J., Pajorova, J., Slepicka, P., et al. (2018). Stem cells: their source, potency and use in regenerative therapies with focus on adipose-derived stem cells - a review. Biotechnol. Adv. 36, 1111–1126. doi: 10.1016/j.biotechadv.2018.03.011
Baumgartner, L. S., Moore, E., Shook, D., Messina, S., Day, M. C., Green, J., et al. (2018). Safety of autologous umbilical cord blood therapy for acquired sensorineural hearing loss in children. J. Audiol. Otol. 22, 209–222. doi: 10.7874/jao.2018.00115
Bi, Z., Li, X., Ren, M., Gu, Y., Zhu, T., Li, S., et al. (2022). Development and transdifferentiation into inner hair cells require Tbx2. Natl. Sci. Rev. 9:nwac156. doi: 10.1093/nsr/nwac156
Boer, J. C., Carney, K. E., and van der Zee, S. (2009). Differentiation of mouse embryonic stem cells into spiral ganglion neurons: a therapeutic approach to deafness. J. Neurosci. 29, 5711–5712. doi: 10.1523/JNEUROSCI.0433-09.2009
Bond, A. M., Ming, G. L., and Song, H. (2015). Adult mammalian neural stem cells and neurogenesis: five decades later. Cell Stem Cell 17, 385–395. doi: 10.1016/j.stem.2015.09.003
Bongso, A. (2006). Blastocyst culture for deriving human embryonic stem cells. Methods Mol. Biol. 331, 13–22. doi: 10.1385/1-59745-046-4:13
Bongso, A., and Richards, M. (2004). History and perspective of stem cell research. Best Pract. Res. Clin. Obstet. Gynaecol. 18, 827–842. doi: 10.1016/j.bpobgyn.2004.09.002
Bramhall, N. F., Shi, F., Arnold, K., Hochedlinger, K., and Edge, A. S. B. (2014). Lgr5-positive supporting cells generate new hair cells in the postnatal cochlea. Stem Cell Rep. 2, 311–322. doi: 10.1016/j.stemcr.2014.01.008
Breglio, A. M., May, L. A., Barzik, M., Welsh, N. C., Francis, S. P., Costain, T. Q., et al. (2020). Exosomes mediate sensory hair cell protection in the inner ear. J. Clin. Invest. 130, 2657–2672. doi: 10.1172/JCI128867
Cai, J., Zhang, H., Hu, Y., Huang, Z., Wang, Y., Xia, Y., et al. (2022). GelMA-MXene hydrogel nerve conduits with microgrooves for spinal cord injury repair. J. Nanobiotechnol. 20:460. doi: 10.1186/s12951-022-01669-2
Cai, C., Zhang, X., Li, Y., Liu, X., Wang, S., Lu, M., et al. (2022). Self-healing hydrogel embodied with macrophage-regulation and responsive-gene-silencing properties for synergistic prevention of Peritendinous adhesion. Adv. Mater. 34:e2106564. doi: 10.1002/adma.202106564
Camp, J. G., Wollny, D., and Treutlein, B. (2018). Single-cell genomics to guide human stem cell and tissue engineering. Nat. Methods 15, 661–667. doi: 10.1038/s41592-018-0113-0
Cao, Y., Wang, Z., Yan, Y., Ji, L., He, J., Xuan, B., et al. (2021). Enterotoxigenic Bacteroidesfragilis promotes intestinal inflammation and malignancy by inhibiting exosome-packaged miR-149-3p. Gastroenterology 161, 1552–1566.e12. doi: 10.1053/j.gastro.2021.08.003
Caplan, A. I. (2010). Mesenchymal stem cells: the past, the present, the future. Cartilage 1, 6–9. doi: 10.1177/1947603509354992
Chai, R., Kuo, B., Wang, T., Liaw, E. J., Xia, A., Jan, T. A., et al. (2012). Wnt signaling induces proliferation of sensory precursors in the postnatal mouse cochlea. Proc. Natl. Acad. Sci. U. S. A. 109, 8167–8172. doi: 10.1073/pnas.1202774109
Chang, W. H., Cerione, R. A., and Antonyak, M. A. (2021). Extracellular vesicles and their roles in Cancer progression. Methods Mol. Biol. 2174, 143–170. doi: 10.1007/978-1-0716-0759-6_10
Chang, H. T., Heuer, R. A., Oleksijew, A. M., Coots, K. S., Roque, C. B., Nella, K. T., et al. (2020). An engineered three-dimensional stem cell niche in the inner ear by applying a nanofibrillar cellulose hydrogel with a sustained-release neurotrophic factor delivery system. Acta Biomater. 108, 111–127. doi: 10.1016/j.actbio.2020.03.007
Chen, C., and Guan, M. X. (2022). Genetic correction of TRMU allele restored the mitochondrial dysfunction-induced deficiencies in iPSCs-derived hair cells of hearing-impaired patients. Hum. Mol. Genet. 31, 3068–3082. doi: 10.1093/hmg/ddac096
Chen, J., Hong, F., Zhang, C., Li, L., Wang, C., Shi, H., et al. (2018). Differentiation and transplantation of human induced pluripotent stem cell-derived otic epithelial progenitors in mouse cochlea. Stem Cell Res Ther 9:230. doi: 10.1186/s13287-018-0967-1
Chen, J. R., Tang, Z. H., Zheng, J., Shi, H. S., Ding, J., Qian, X. D., et al. (2016). Effects of genetic correction on the differentiation of hair cell-like cells from iPSCs with MYO15A mutation. Cell Death Differ. 23, 1347–1357. doi: 10.1038/cdd.2016.16
Chen, Y. C., Tsai, C. L., Wei, Y. H., Wu, Y. T., Hsu, W. T., Lin, H. C., et al. (2018). ATOH1/RFX1/RFX3 transcription factors facilitate the differentiation and characterisation of inner ear hair cell-like cells from patient-specific induced pluripotent stem cells harbouring A8344G mutation of mitochondrial DNA. Cell Death Dis. 9:437. doi: 10.1038/s41419-018-0488-y
Costa, A., Powell, L. M., Malaguti, M., Soufi, A., Lowell, S., and Jarman, A. P. (2022). Repurposing the lineage-determining transcription factor Atoh1 without redistributing its genomic binding sites. Front. Cell Dev. Biol. 10:1016367. doi: 10.3389/fcell.2022.1016367
Cully, M. (2021). Exosome-based candidates move into the clinic. Nat. Rev. Drug Discov. 20, 6–7. doi: 10.1038/d41573-020-00220-y
Currey, L., Thor, S., and Piper, M. (2021). TEAD family transcription factors in development and disease. Development 148:dev196675. doi: 10.1242/dev.196675
Dai, J., Su, Y., Zhong, S., Cong, L., Liu, B., Yang, J., et al. (2020). Exosomes: key players in cancer and potential therapeutic strategy. Signal Transduct. Target. Ther. 5:145. doi: 10.1038/s41392-020-00261-0
de Vincentiis, S., Baggiani, M., Merighi, F., Cappello, V., Lopane, J., di Caprio, M., et al. (2023). Low forces push the maturation of neural precursors into neurons. Small 19:e2205871. doi: 10.1002/smll.202205871
Evans, M. J., and Kaufman, M. H. (1981). Establishment in culture of pluripotential cells from mouse embryos. Nature 292, 154–156. doi: 10.1038/292154a0
Evsen, L., Li, X., Zhang, S., Razin, S., and Doetzlhofer, A. (2020). Let-7 miRNAs inhibit CHD7 expression and control auditory-sensory progenitor cell behavior in the developing inner ear. Development 147:dev183384. doi: 10.1242/dev.183384
Fan, L., Liu, C., Chen, X., Zou, Y., Zhou, Z., Lin, C., et al. (2018). Directing induced pluripotent stem cell derived neural stem cell fate with a three-dimensional biomimetic hydrogel for spinal cord injury repair. ACS Appl. Mater. Interfaces 10, 17742–17755. doi: 10.1021/acsami.8b05293
Fayazi, N., Sheykhhasan, M., Soleimani Asl, S., and Najafi, R. (2021). Stem cell-derived exosomes: a new strategy of neurodegenerative disease treatment. Mol. Neurobiol. 58, 3494–3514. doi: 10.1007/s12035-021-02324-x
Fukunaga, I., Oe, Y., Danzaki, K., Ohta, S., Chen, C., Shirai, K., et al. (2021). Modeling gap junction beta 2 gene-related deafness with human iPSC. Hum. Mol. Genet. 30, 1429–1442. doi: 10.1093/hmg/ddab097
García-Añoveros, J., Clancy, J. C., Foo, C. Z., García-Gómez, I., Zhou, Y., Homma, K., et al. (2022). Tbx2 is a master regulator of inner versus outer hair cell differentiation. Nature 605, 298–303. doi: 10.1038/s41586-022-04668-3
Gnedeva, K., Wang, X., McGovern, M. M., Barton, M., Tao, L., Trecek, T., et al. (2020). Organ of Corti size is governed by yap/Tead-mediated progenitor self-renewal. Proc. Natl. Acad. Sci. U. S. A. 117, 13552–13561. doi: 10.1073/pnas.2000175117
Goncalves, S., Bas, E., Langston, M., Grobman, A., Goldstein, B. J., and Angeli, S. (2017). Histologic changes of mesenchymal stem cell repair of tympanic membrane perforation. Acta Otolaryngol. 137, 411–416. doi: 10.1080/00016489.2016.1261411
Grewal, S. S., Shapiro, E. G., Krivit, W., Charnas, L., Lockman, L. A., Delaney, K. A., et al. (2004). Effective treatment of alpha-mannosidosis by allogeneic hematopoietic stem cell transplantation. J. Pediatr. 144, 569–573. doi: 10.1016/j.jpeds.2004.01.025
Guo, R., Liao, M., Ma, X., Hu, Y., Qian, X., Xiao, M., et al. (2021). Cochlear implant-based electric-acoustic stimulation modulates neural stem cell-derived neural regeneration. J. Mater. Chem. B 9, 7793–7804. doi: 10.1039/D1TB01029H
Guo, R., Xiao, M., Zhao, W., Zhou, S., Hu, Y., Liao, M., et al. (2022). 2D Ti(3)C(2)T(x)MXene couples electrical stimulation to promote proliferation and neural differentiation of neural stem cells. Acta Biomater. 139, 105–117. doi: 10.1016/j.actbio.2020.12.035
Guo, M., Yin, Z., Chen, F., and Lei, P. (2020). Mesenchymal stem cell-derived exosome: a promising alternative in the therapy of Alzheimer's disease. Alzheimers Res. Ther. 12:109. doi: 10.1186/s13195-020-00670-x
Guo, R., Zhang, S., Xiao, M., Qian, F., He, Z., Li, D., et al. (2016). Accelerating bioelectric functional development of neural stem cells by graphene coupling: implications for neural interfacing with conductive materials. Biomaterials 106, 193–204. doi: 10.1016/j.biomaterials.2016.08.019
Han, Q. F., Li, W. J., Hu, K. S., Gao, J., Zhai, W. L., Yang, J. H., et al. (2022). Exosome biogenesis: machinery, regulation, and therapeutic implications in cancer. Mol. Cancer 21:207. doi: 10.1186/s12943-022-01671-0
Hao, J., Ma, A., Wang, L., Cao, J., Chen, S., Wang, L., et al. (2020). General requirements for stem cells. Cell Prolif. 53:e12926. doi: 10.1111/cpr.12926
He, Z., Ding, Y., Mu, Y., Xu, X., Kong, W., Chai, R., et al. (2021). Stem cell-based therapies in hearing loss. Front. Cell Dev. Biol. 9:730042. doi: 10.3389/fcell.2021.730042
Ho, P. J., Yen, M. L., Yet, S. F., and Yen, B. L. (2012). Current applications of human pluripotent stem cells: possibilities and challenges. Cell Transplant. 21, 801–814. doi: 10.3727/096368911X627507
Hockemeyer, D., and Jaenisch, R. (2016). Induced pluripotent stem cells meet genome editing. Cell Stem Cell 18, 573–586. doi: 10.1016/j.stem.2016.04.013
Hu, Y., Chen, W., Yin, H., Chen, X., Cai, J., Guo, J., et al. (2022). Super-aligned carbon nanotubes and GelMA hydrogel composite scaffolds promote spiral ganglion neuron growth and orientation. Materials Today Nano 18:100181. doi: 10.1016/j.mtnano.2022.100181
Hu, Y., Li, D., Wei, H., Zhou, S., Chen, W., Yan, X., et al. (2021). Neurite extension and orientation of spiral ganglion neurons can be directed by superparamagnetic Iron oxide nanoparticles in a magnetic field. Int. J. Nanomedicine 16, 4515–4526. doi: 10.2147/IJN.S313673
Huang, X., Kou, X., Zhan, T., Wei, G., He, F., Mao, X., et al. (2023). Apoptotic vesicles resist oxidative damage in noise-induced hearing loss through activation of FOXO3a-SOD2 pathway. Stem Cell Res Ther 14:88. doi: 10.1186/s13287-023-03314-7
Ilancheran, S., Moodley, Y., and Manuelpillai, U. (2009). Human fetal membranes: a source of stem cells for tissue regeneration and repair? Placenta 30, 2–10. doi: 10.1016/j.placenta.2008.09.009
Ilic, D., and Polak, J. M. (2011). Stem cells in regenerative medicine: introduction. Br. Med. Bull. 98, 117–126. doi: 10.1093/bmb/ldr012
Isaac, R., Reis, F. C. G., Ying, W., and Olefsky, J. M. (2021). Exosomes as mediators of intercellular crosstalk in metabolism. Cell Metab. 33, 1744–1762. doi: 10.1016/j.cmet.2021.08.006
Jang, C. H., Ahn, S. H., Lee, J. W., Lee, B. H., Lee, H., and Kim, G. H. (2017). Mesenchymal stem cell-laden hybrid scaffold for regenerating subacute tympanic membrane perforation. Mater. Sci. Eng. C Mater. Biol. Appl. 72, 456–463. doi: 10.1016/j.msec.2016.11.094
Johnson, M. H., and Cohen, J. (2012). Reprogramming rewarded: the 2012 Nobel prize for physiology or medicine awarded to John Gurdon and Shinya Yamanaka. Reprod. Biomed. Online 25, 549–550. doi: 10.1016/j.rbmo.2012.10.009
Kaiser, M., Lüdtke, T. H., Deuper, L., Rudat, C., Christoffels, V. M., Kispert, A., et al. (2022). TBX2 specifies and maintains inner hair and supporting cell fate in the organ of Corti. Nat. Commun. 13:7628. doi: 10.1038/s41467-022-35214-4
Kaiser, M., Wojahn, I., Rudat, C., Lüdtke, T. H., Christoffels, V. M., Moon, A., et al. (2021). Regulation of otocyst patterning by Tbx2 and Tbx3 is required for inner ear morphogenesis in the mouse. Development 148:dev195651. doi: 10.1242/dev.195651
Kalluri, R., and LeBleu, V. S. (2020). The biology, function, and biomedical applications of exosomes. Science 367:eaau6977. doi: 10.1126/science.aau6977
Kempfle, J. S., Luu, N. N. C., Petrillo, M., al-Asad, R., Zhang, A., and Edge, A. S. B. (2020). Lin28 reprograms inner ear glia to a neuronal fate. Stem Cells 38, 890–903. doi: 10.1002/stem.3181
Kim, T. H., Lee, K. B., and Choi, J. W. (2013). 3D graphene oxide-encapsulated gold nanoparticles to detect neural stem cell differentiation. Biomaterials 34, 8660–8670. doi: 10.1016/j.biomaterials.2013.07.101
Koehler, K. R., Mikosz, A. M., Molosh, A. I., Patel, D., and Hashino, E. (2013). Generation of inner ear sensory epithelia from pluripotent stem cells in 3D culture. Nature 500, 217–221. doi: 10.1038/nature12298
Kuang, Y., Zheng, X., Zhang, L., Ai, X., Venkataramani, V., Kilic, E., et al. (2020). Adipose-derived mesenchymal stem cells reduce autophagy in stroke mice by extracellular vesicle transfer of miR-25. J. Extracell. Vesicles 10:e12024. doi: 10.1002/jev2.12024
Kumar, R., Tang, Q., Müller, S. A., Gao, P., Mahlstedt, D., Zampagni, S., et al. (2020). Fibroblast growth factor 2-mediated regulation of neuronal exosome release depends on VAMP3/Cellubrevin in hippocampal neurons. Adv. Sci. (Weinh) 7:1902372. doi: 10.1002/advs.201902372
Lauer, A. M., Dent, M. L., Sun, W., and Xu-Friedman, M. A. (2019). Effects of non-traumatic noise and conductive hearing loss on auditory system function. Neuroscience 407, 182–191. doi: 10.1016/j.neuroscience.2019.01.020
Li, X. J., and Doetzlhofer, A. (2020). LIN28B/let-7 control the ability of neonatal murine auditory supporting cells to generate hair cells through mTOR signaling. Proc. Natl. Acad. Sci. U. S. A. 117, 22225–22236. doi: 10.1073/pnas.2000417117
Li, Y., Hu, Y., Wei, H., Cao, W., Qi, Y., Zhou, S., et al. (2022). Two-dimensional Ti(3)C(2)T(x) MXene promotes electrophysiological maturation of neural circuits. J. Nanobiotechnol. 20:398. doi: 10.1186/s12951-022-01590-8
Li, D., Hu, Y., Wei, H., Chen, W., Liu, Y., Yan, X., et al. (2021). Superparamagnetic Iron oxide nanoparticles and static magnetic field regulate neural stem cell proliferation. Front. Cell. Neurosci. 15:815280. doi: 10.3389/fncel.2021.815280
Li, H., Liu, H., and Heller, S. (2003a). Pluripotent stem cells from the adult mouse inner ear. Nat. Med. 9, 1293–1299. doi: 10.1038/nm925
Li, H., Roblin, G., Liu, H., and Heller, S. (2003b). Generation of hair cells by stepwise differentiation of embryonic stem cells. Proc. Natl. Acad. Sci. U. S. A. 100, 13495–13500. doi: 10.1073/pnas.2334503100
Li, W., Wu, J., Yang, J., Sun, S., Chai, R., Chen, Z. Y., et al. (2015). Notch inhibition induces mitotically generated hair cells in mammalian cochleae via activating the Wnt pathway. Proc. Natl. Acad. Sci. U. S. A. 112, 166–171. doi: 10.1073/pnas.1415901112
Li, C., Xiong, Z., Zhou, L., Huang, W., He, Y., Li, L., et al. (2022). Interfacing perforated eardrums with graphene-based membranes for broadband hearing recovery. Adv. Healthc. Mater. 11:e2201471. doi: 10.1002/adhm.202201471
Liao, M., Hu, Y., Zhang, Y., Wang, K., Fang, Q., Qi, Y., et al. (2022). 3D Ti(3)C(2)T(x) MXene-Matrigel with electroacoustic stimulation to promote the growth of spiral ganglion neurons. ACS Nano 16, 16744–16756. doi: 10.1021/acsnano.2c06306
Lieu, J. E. C., Kenna, M., Anne, S., and Davidson, L. (2020). Hearing loss in children: a review. JAMA 324, 2195–2205. doi: 10.1001/jama.2020.17647
Lin, B., Lu, L., Wang, Y., Zhang, Q., Wang, Z., Cheng, G., et al. (2021). Nanomedicine directs neuronal differentiation of neural stem cells via silencing long noncoding RNA for stroke therapy. Nano Lett. 21, 806–815. doi: 10.1021/acs.nanolett.0c04560
Liu, W., Yu, M., Xie, D., Wang, L., Ye, C., Zhu, Q., et al. (2020). Melatonin-stimulated MSC-derived exosomes improve diabetic wound healing through regulating macrophage M1 and M2 polarization by targeting the PTEN/AKT pathway. Stem Cell Res Ther 11:259. doi: 10.1186/s13287-020-01756-x
Maharajan, N., Cho, G. W., and Jang, C. H. (2020). Application of mesenchymal stem cell for tympanic membrane regeneration by tissue engineering approach. Int. J. Pediatr. Otorhinolaryngol. 133:109969. doi: 10.1016/j.ijporl.2020.109969
Matsuoka, A. J., Morrissey, Z. D., Zhang, C., Homma, K., Belmadani, A., Miller, C. A., et al. (2017). Directed differentiation of human embryonic stem cells toward placode-derived spiral ganglion-like sensory neurons. Stem Cells Transl. Med. 6, 923–936. doi: 10.1002/sctm.16-0032
McLean, W. J., Yin, X., Lu, L., Lenz, D. R., McLean, D., Langer, R., et al. (2017). Clonal expansion of Lgr5-positive cells from mammalian cochlea and high-purity generation of sensory hair cells. Cell Rep. 18, 1917–1929. doi: 10.1016/j.celrep.2017.01.066
Mizutari, K., Fujioka, M., Hosoya, M., Bramhall, N., Okano, H. J., Okano, H., et al. (2013). Notch inhibition induces cochlear hair cell regeneration and recovery of hearing after acoustic trauma. Neuron 77, 58–69. doi: 10.1016/j.neuron.2012.10.032
Moodley, Y., Ilancheran, S., Samuel, C., Vaghjiani, V., Atienza, D., Williams, E. D., et al. (2010). Human amnion epithelial cell transplantation abrogates lung fibrosis and augments repair. Am. J. Respir. Crit. Care Med. 182, 643–651. doi: 10.1164/rccm.201001-0014OC
Moser, T., and Starr, A. (2016). Auditory neuropathy--neural and synaptic mechanisms. Nat. Rev. Neurol. 12, 135–149. doi: 10.1038/nrneurol.2016.10
Muller, U. (2020). Exosome-mediated protection of auditory hair cells from ototoxic insults. J. Clin. Invest. 130, 2206–2208. doi: 10.1172/JCI135710
Nayagam, B. A., Muniak, M. A., and Ryugo, D. K. (2011). The spiral ganglion: connecting the peripheral and central auditory systems. Hear. Res. 278, 2–20. doi: 10.1016/j.heares.2011.04.003
Nie, J., Ueda, Y., Solivais, A. J., and Hashino, E. (2022). CHD7 regulates otic lineage specification and hair cell differentiation in human inner ear organoids. Nat. Commun. 13:7053. doi: 10.1038/s41467-022-34759-8
Ocansey, D. K. W., Zhang, L., Wang, Y., Yan, Y., Qian, H., Zhang, X., et al. (2020). Exosome-mediated effects and applications in inflammatory bowel disease. Biol. Rev. Camb. Philos. Soc. 95, 1287–1307. doi: 10.1111/brv.12608
Ohnuki, M., and Takahashi, K. (2015). Present and future challenges of induced pluripotent stem cells. Philos. Trans. R. Soc. Lond. Ser. B Biol. Sci. 370:20140367. doi: 10.1098/rstb.2014.0367
Omichi, R., Shibata, S. B., Morton, C. C., and Smith, R. J. H. (2019). Gene therapy for hearing loss. Hum. Mol. Genet. 28, R65–R79. doi: 10.1093/hmg/ddz129
Park, D. J., Park, J. E., Lee, S. H., Eliceiri, B. P., Choi, J. S., and Seo, Y. J. (2021). Protective effect of MSC-derived exosomes against cisplatin-induced apoptosis via heat shock protein 70 in auditory explant model. Nanomedicine 38:102447. doi: 10.1016/j.nano.2021.102447
Peyvandi, A. A., Abbaszadeh, H. A., Roozbahany, N. A., Pourbakht, A., Khoshsirat, S., Niri, H. H., et al. (2018). Deferoxamine promotes mesenchymal stem cell homing in noise-induced injured cochlea through PI3K/AKT pathway. Cell Prolif. 51:e12434. doi: 10.1111/cpr.12434
Pittenger, M. F., Mackay, A. M., Beck, S. C., Jaiswal, R. K., Douglas, R., Mosca, J. D., et al. (1999). Multilineage potential of adult human mesenchymal stem cells. Science 284, 143–147. doi: 10.1126/science.284.5411.143
Plontke, S. K., Meisner, C., Agrawal, S., Cayé-Thomasen, P., Galbraith, K., Mikulec, A. A., et al. (2022). Intratympanic corticosteroids for sudden sensorineural hearing loss. Cochrane Database Syst. Rev. 2022:CD008080. doi: 10.1002/14651858.CD008080.pub2
Qin, T., Ho, C. C., Wang, B., Hui, C. C., and Sham, M. H. (2022). Sufu- and Spop-mediated regulation of Gli2 is essential for the control of mammalian cochlear hair cell differentiation. Proc. Natl. Acad. Sci. U. S. A. 119:e2206571119. doi: 10.1073/pnas.2206571119
Ramalho-Santos, M., and Willenbring, H. (2007). On the origin of the term "stem cell". Cell Stem Cell 1, 35–38. doi: 10.1016/j.stem.2007.05.013
Rasool, K., Helal, M., Ali, A., Ren, C. E., Gogotsi, Y., and Mahmoud, K. A. (2016). Antibacterial activity of Ti(3)C(2)Tx MXene. ACS Nano 10, 3674–3684. doi: 10.1021/acsnano.6b00181
Reynolds, B. A., and Weiss, S. (1992). Generation of neurons and astrocytes from isolated cells of the adult mammalian central nervous system. Science 255, 1707–1710. doi: 10.1126/science.1553558
Riazifar, M., Mohammadi, M. R., Pone, E. J., Yeri, A., Lässer, C., Segaliny, A. I., et al. (2019). Stem cell-derived exosomes as Nanotherapeutics for autoimmune and neurodegenerative disorders. ACS Nano 13, 6670–6688. doi: 10.1021/acsnano.9b01004
Seddon, J. A., Godfrey-Faussett, P., Jacobs, K., Ebrahim, A., Hesseling, A. C., and Schaaf, H. S. (2012). Hearing loss in patients on treatment for drug-resistant tuberculosis. Eur. Respir. J. 40, 1277–1286. doi: 10.1183/09031936.00044812
Serles, P., Hamidinejad, M., Demingos, P. G., Ma, L., Barri, N., Taylor, H., et al. (2022). Friction of Ti(3)C(2)T(x) MXenes. Nano Lett. 22, 3356–3363. doi: 10.1021/acs.nanolett.2c00614
Shahal, D., Goncalves, S., and Angeli, S. I. (2022). Mesenchymal stem cells for treatment of delayed-healing tympanic membrane perforations using hyaluronate-based laminas as a delivery system: an animal model with histopathologic study. Otol. Neurotol. 43, e497–e506. doi: 10.1097/MAO.0000000000003468
Shao, A., Tu, S., Lu, J., and Zhang, J. (2019). Crosstalk between stem cell and spinal cord injury: pathophysiology and treatment strategies. Stem Cell Res Ther 10:238. doi: 10.1186/s13287-019-1357-z
Shi, F., Hu, L., and Edge, A. S. (2013). Generation of hair cells in neonatal mice by beta-catenin overexpression in Lgr5-positive cochlear progenitors. Proc. Natl. Acad. Sci. U. S. A. 110, 13851–13856. doi: 10.1073/pnas.1219952110
Shi, F., Hu, L., Jacques, B. E., Mulvaney, J. F., Dabdoub, A., and Edge, A. S. B. (2014). beta-catenin is required for hair-cell differentiation in the cochlea. J. Neurosci. 34, 6470–6479. doi: 10.1523/JNEUROSCI.4305-13.2014
Shu, Y., Li, W., Huang, M., Quan, Y. Z., Scheffer, D., Tian, C., et al. (2019). Renewed proliferation in adult mouse cochlea and regeneration of hair cells. Nat. Commun. 10:5530. doi: 10.1038/s41467-019-13157-7
Soares, V. Y., Atai, N. A., Fujita, T., Dilwali, S., Sivaraman, S., Landegger, L. D., et al. (2016). Extracellular vesicles derived from human vestibular schwannomas associated with poor hearing damage cochlear cells. Neuro-Oncology 18, now099–now1507. doi: 10.1093/neuonc/now099
Steevens, A. R., Glatzer, J. C., Kellogg, C. C., Low, W. C., Santi, P. A., and Kiernan, A. E. (2019). SOX2 is required for inner ear growth and cochlear nonsensory formation before sensory development. Development 146:dev170522. doi: 10.1242/dev.170522
Stojkovic, M., Han, D., Jeong, M., Stojkovic, P., and Stankovic, K. M. (2021). Human induced pluripotent stem cells and CRISPR/Cas-mediated targeted genome editing: platforms to tackle sensorineural hearing loss. Stem Cells 39, 673–696. doi: 10.1002/stem.3353
Sun, W., Ren, Y., Lu, Z., and Zhao, X. (2020). The potential roles of exosomes in pancreatic cancer initiation and metastasis. Mol. Cancer 19:135. doi: 10.1186/s12943-020-01255-w
Sun, X., and Yang, J. (2020). Research advances on umbilical cord blood derived mesenchymal stem cell in therapy of sensorineural hearing loss. Lin Chung Er Bi Yan Hou Tou Jing Wai Ke Za Zhi 34, 1149–1152. doi: 10.13201/j.issn.2096-7993.2020.12.023
Swan, E. E., Mescher, M. J., Sewell, W. F., Tao, S. L., and Borenstein, J. T. (2008). Inner ear drug delivery for auditory applications. Adv. Drug Deliv. Rev. 60, 1583–1599. doi: 10.1016/j.addr.2008.08.001
Takahashi, K., Tanabe, K., Ohnuki, M., Narita, M., Ichisaka, T., Tomoda, K., et al. (2007). Induction of pluripotent stem cells from adult human fibroblasts by defined factors. Cells 131, 861–872. doi: 10.1016/j.cell.2007.11.019
Takahashi, K., and Yamanaka, S. (2006). Induction of pluripotent stem cells from mouse embryonic and adult fibroblast cultures by defined factors. Cells 126, 663–676. doi: 10.1016/j.cell.2006.07.024
Takeda, H., Dondzillo, A., Randall, J. A., and Gubbels, S. P. (2018). Challenges in cell-based therapies for the treatment of hearing loss. Trends Neurosci. 41, 823–837. doi: 10.1016/j.tins.2018.06.008
Takeda, H., Dondzillo, A., Randall, J. A., and Gubbels, S. P. (2021). Selective ablation of cochlear hair cells promotes engraftment of human embryonic stem cell-derived progenitors in the mouse organ of Corti. Stem Cell Res Ther 12:352. doi: 10.1186/s13287-021-02403-9
Tang, Z. H., Chen, J. R., Zheng, J., Shi, H. S., Ding, J., Qian, X. D., et al. (2016). Genetic correction of induced pluripotent stem cells from a deaf patient with MYO7A mutation results in morphologic and functional recovery of the derived hair cell-like cells. Stem Cells Transl. Med. 5, 561–571. doi: 10.5966/sctm.2015-0252
Thomson, J. A., Itskovitz-Eldor, J., Shapiro, S. S., Waknitz, M. A., Swiergiel, J. J., Marshall, V. S., et al. (1998). Embryonic stem cell lines derived from human blastocysts. Science 282, 1145–1147. doi: 10.1126/science.282.5391.1145
Till, J. E., and Mc, C. E. (1961). A direct measurement of the radiation sensitivity of normal mouse bone marrow cells. Radiat. Res. 14, 213–222. doi: 10.2307/3570892
Tsai, S. C., Yang, K., Chang, K. H., Lin, F., Chou, R. H., Li, M. C., et al. (2021). Umbilical cord mesenchymal stromal cell-derived exosomes rescue the loss of outer hair cells and repair Cochlear damage in cisplatin-injected mice. Int. J. Mol. Sci. 22:6664. doi: 10.3390/ijms22136664
Tuan, R. S., Boland, G., and Tuli, R. (2003). Adult mesenchymal stem cells and cell-based tissue engineering. Arthritis Res. Ther. 5, 32–45. doi: 10.1186/ar614
Vitelli, F., Viola, A., Morishima, M., Pramparo, T., Baldini, A., and Lindsay, E. (2003). TBX1 is required for inner ear morphogenesis. Hum. Mol. Genet. 12, 2041–2048. doi: 10.1093/hmg/ddg216
Vogel, A. D., Upadhya, R., and Shetty, A. K. (2018). Neural stem cell derived extracellular vesicles: attributes and prospects for treating neurodegenerative disorders. EBioMedicine 38, 273–282. doi: 10.1016/j.ebiom.2018.11.026
Wang, Y., Liu, J., Wang, H., Lv, S., Liu, Q., Li, S., et al. (2023). Mesenchymal stem cell-derived exosomes ameliorate diabetic kidney disease through the NLRP3 signaling pathway. Stem Cells 41, 368–383. doi: 10.1093/stmcls/sxad010
Wang, J., and Puel, J. L. (2018). Toward Cochlear therapies. Physiol. Rev. 98, 2477–2522. doi: 10.1152/physrev.00053.2017
Wang, D., Wang, Y., Tian, W., and Pan, J. (2019). Advances of tooth-derived stem cells in neural diseases treatments and nerve tissue regeneration. Cell Prolif. 52:e12572. doi: 10.1111/cpr.12572
Warnecke, A., Harre, J., Shew, M., Mellott, A. J., Majewski, I., Durisin, M., et al. (2021a). Successful treatment of noise-induced hearing loss by mesenchymal stromal cells: an RNAseq analysis of protective/repair pathways. Front. Cell. Neurosci. 15:656930. doi: 10.3389/fncel.2021.656930
Warnecke, A., Harre, J., Staecker, H., Prenzler, N., Strunk, D., Couillard-Despres, S., et al. (2020). Extracellular vesicles from human multipotent stromal cells protect against hearing loss after noise trauma in vivo. Clin. Transl. Med. 10:e262. doi: 10.1002/ctm2.262
Warnecke, A., Prenzler, N., Harre, J., Köhl, U., Gärtner, L., Lenarz, T., et al. (2021b). First-in-human intracochlear application of human stromal cell-derived extracellular vesicles. J. Extracell. Vesicles 10:e12094. doi: 10.1002/jev2.12094
Wu, Z., Wei, L., Tang, S., Xiong, Y., Qin, X., Luo, J., et al. (2021). Recent Progress in Ti(3)C(2)T(x) MXene-based flexible pressure sensors. ACS Nano 15, 18880–18894. doi: 10.1021/acsnano.1c08239
Xia, L., Zhao, X., Ma, X., Hu, Y., Zhang, Y., Li, S., et al. (2022). Controllable growth of spiral ganglion neurons by magnetic colloidal nanochains. Nano Today 44:101507. doi: 10.1016/j.nantod.2022.101507
Xing, L., Wilsch-Brauninger, M., and Huttner, W. B. (2021). How neural stem cells contribute to neocortex development. Biochem. Soc. Trans. 49, 1997–2006. doi: 10.1042/BST20200923
Xu, C., Qi, J., Hu, X., Zhang, L., Sun, Q., Li, N., et al. (2023). Rps14 upregulation promotes inner ear progenitor proliferation and hair cell regeneration in the neonatal mouse cochlea. Cell Prolif. 56:e13458. doi: 10.1111/cpr.13458
Xu, Z., Zeng, S., Gong, Z., and Yan, Y. (2020). Exosome-based immunotherapy: a promising approach for cancer treatment. Mol. Cancer 19:160. doi: 10.1186/s12943-020-01278-3
Yamanaka, S. (2012). Induced pluripotent stem cells: past, present, and future. Cell Stem Cell 10, 678–684. doi: 10.1016/j.stem.2012.05.005
Yang, B., Chen, Y., and Shi, J. (2019). Exosome biochemistry and advanced nanotechnology for next-generation Theranostic platforms. Adv. Mater. 31:e1802896. doi: 10.1002/adma.201802896
Yang, Q., Shi, H., Quan, Y., Chen, Q., Li, W., Wang, L., et al. (2021). Stepwise induction of inner ear hair cells from mouse embryonic fibroblasts via mesenchymal- to-epithelial transition and formation of Otic epithelial cells. Front. Cell Dev. Biol. 9:672406. doi: 10.3389/fcell.2021.672406
Yao, S., Chen, S., Clark, J., Hao, E., Beattie, G. M., Hayek, A., et al. (2006). Long-term self-renewal and directed differentiation of human embryonic stem cells in chemically defined conditions. Proc. Natl. Acad. Sci. U. S. A. 103, 6907–6912. doi: 10.1073/pnas.0602280103
Ye, Z., Su, Z., Xie, S., Liu, Y., Wang, Y., Xu, X., et al. (2020). Yap-lin28a axis targets let7-Wnt pathway to restore progenitors for initiating regeneration. elife 9:9. doi: 10.7554/eLife.55771
Yin, W. C., Satkunendran, T., Mo, R., Morrissy, S., Zhang, X., Huang, E. S., et al. (2019). Dual regulatory functions of SUFU and Targetome of GLI2 in SHH subgroup Medulloblastoma. Dev. Cell 48, 167–183.e5. doi: 10.1016/j.devcel.2018.11.015
Yoo, T. J., Du, X., and Zhou, B. (2015). The paracrine effect of mesenchymal human stem cells restored hearing in beta-tubulin induced autoimmune sensorineural hearing loss. Hear. Res. 330, 57–61. doi: 10.1016/j.heares.2015.07.021
Yu, H. V., Tao, L., Llamas, J., Wang, X., Nguyen, J. D., Trecek, T., et al. (2021). POU4F3 pioneer activity enables ATOH1 to drive diverse mechanoreceptor differentiation through a feed-forward epigenetic mechanism. Proc. Natl. Acad. Sci. U. S. A. 118:e2105137118. doi: 10.1073/pnas.2105137118
Zakrzewski, W., Dobrzyński, M., Szymonowicz, M., and Rybak, Z. (2019). Stem cells: past, present, and future. Stem Cell Res Ther 10:68. doi: 10.1186/s13287-019-1165-5
Zhang, Z., Shin, J., Tague, N., Lin, H., Zhang, M., Ge, X., et al. (2022). Ti(3) C(2) T(x) MXene composite 3D hydrogel potentiates mTOR signaling to promote the generation of functional hair cells in cochlea organoids. Adv. Sci. (Weinh) 9:e2203557. doi: 10.1002/advs.202203887
Zhang, S., Zhang, Y., Dong, Y., Guo, L., Zhang, Z., Shao, B., et al. (2020). Knockdown of Foxg1 in supporting cells increases the trans-differentiation of supporting cells into hair cells in the neonatal mouse cochlea. Cell. Mol. Life Sci. 77, 1401–1419. doi: 10.1007/s00018-019-03291-2
Zhong, C., Shen, H., Han, Y., Wang, S., Wang, Y., Xu, X., et al. (2016). Alginate microcapsules co-embedded with MSCs and anti-EGF mAb for the induction of hair cell-like cells in guinea pigs by taking advantage of host EGF. J. Mater. Chem. B 4, 7387–7397. doi: 10.1039/C6TB02132H
Keywords: hearing loss, stem cells, exosomes, nanomaterials, clinical trial
Citation: Fang Q, Wei Y, Zhang Y, Cao W, Yan L, Kong M, Zhu Y, Xu Y, Guo L, Zhang L, Wang W, Yu Y, Sun J and Yang J (2023) Stem cells as potential therapeutics for hearing loss. Front. Neurosci. 17:1259889. doi: 10.3389/fnins.2023.1259889
Edited by:
Wenjie Zhou, Shanghai Jiao Tong University, ChinaReviewed by:
Yanyan Ding, Huazhong University of Science and Technology, ChinaChunjiang Wei, Hannover Medical School, Germany
Copyright © 2023 Fang, Wei, Zhang, Cao, Yan, Kong, Zhu, Xu, Guo, Zhang, Wang, Yu, Sun and Yang. This is an open-access article distributed under the terms of the Creative Commons Attribution License (CC BY). The use, distribution or reproduction in other forums is permitted, provided the original author(s) and the copyright owner(s) are credited and that the original publication in this journal is cited, in accordance with accepted academic practice. No use, distribution or reproduction is permitted which does not comply with these terms.
*Correspondence: Yafeng Yu, eWZ5dTEwMjRAMTYzLmNvbQ==; Jingwu Sun, MTM5NTY5MzYyMThAMTYzLmNvbQ==; Jianming Yang, am15YW5nODhAMTYzLmNvbQ==
†These authors have contributed equally to this work