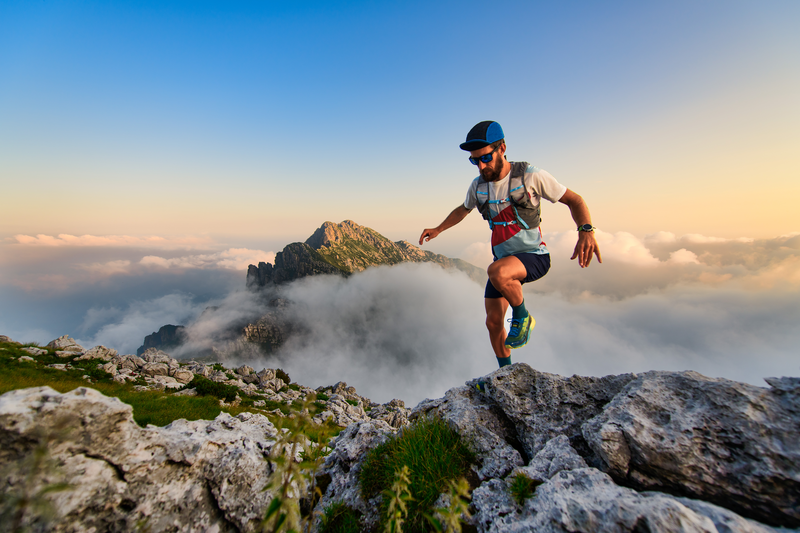
94% of researchers rate our articles as excellent or good
Learn more about the work of our research integrity team to safeguard the quality of each article we publish.
Find out more
REVIEW article
Front. Neurosci. , 23 June 2023
Sec. Neuropharmacology
Volume 17 - 2023 | https://doi.org/10.3389/fnins.2023.1188839
Neurological disorders affect the nervous system. Biochemical, structural, or electrical abnormalities in the spinal cord, brain, or other nerves lead to different symptoms, including muscle weakness, paralysis, poor coordination, seizures, loss of sensation, and pain. There are many recognized neurological diseases, like epilepsy, Alzheimer's disease (AD), Parkinson's disease (PD), multiple sclerosis (MS), stroke, autosomal recessive cerebellar ataxia 2 (ARCA2), Leber's hereditary optic neuropathy (LHON), and spinocerebellar ataxia autosomal recessive 9 (SCAR9). Different agents, such as coenzyme Q10 (CoQ10), exert neuroprotective effects against neuronal damage. Online databases, such as Scopus, Google Scholar, Web of Science, and PubMed/MEDLINE were systematically searched until December 2020 using keywords, including review, neurological disorders, and CoQ10. CoQ10 is endogenously produced in the body and also can be found in supplements or foods. CoQ10 has antioxidant and anti-inflammatory effects and plays a role in energy production and mitochondria stabilization, which are mechanisms, by which CoQ10 exerts its neuroprotective effects. Thus, in this review, we discussed the association between CoQ10 and neurological diseases, including AD, depression, MS, epilepsy, PD, LHON, ARCA2, SCAR9, and stroke. In addition, new therapeutic targets were introduced for the next drug discoveries.
Neurological diseases endanger human health and lifestyle. Neurological diseases affect a large number of people all over the world (World Health Organization, 2006). Stroke accounts for the death of over six million people annually, and more than 80% occur in low- and middle-income countries (Akinyemi et al., 2021). Over 50 million individuals in the world suffer from epilepsy (Scott et al., 2001). Also, there are 47.5 million cases of dementia, including 7.7 million new patients each year (Trandafir, 2016). Alzheimer's disease (AD) is the commonest cause of dementia accounting for 60–70% of dementia cases (Huang et al., 2020). Migraine affects over 10% of the world's population (Steiner et al., 2014).
Several causes and mechanisms have been suggested for neurological disorders (Urdinguio et al., 2009). Lifestyle, genetics, infections, diet, environmental factors, and physical damage have been revealed as the causes of neurological disorders (World Health Organization, 2006). Neurological disorders are associated with the following physical symptoms: partial or full paralysis, seizures, muscle weakness, partial or full loss of sensation, reading and writing disabilities, poor cognitive functions, unexplainable pain, and reduced alertness (Stone and Carson, 2011). Accordingly, oxidative stress and imperfective energy metabolism can be regarded as the pathogenesis of many neurodegenerative diseases, such as Parkinson's disease (PD), AD, multiple sclerosis (MS), epilepsy, depression, and stroke (Choonara et al., 2009). The prevalence of age-dependent disorders has recently been increasing (Bigal et al., 2006). Coenzyme Q10 (CoQ10) is a strong neuroprotective agent in neurodegenerative disorders. The mechanisms of CoQ10 on neurological disorders are shown in Table 1. The levels of CoQ10 diminish in the brain and different tissues in animals and humans with age; thus, CoQ10 has an effective therapeutic role in age-related neurodegenerative disorders (Spindler et al., 2009; Kadian et al., 2022). CoQ10 is also known as ubiquinone, ubidecarenone, CoQ10, CoQ, or Q10. It is a 1,4-benzoquinone and Q represents the quinone chemical group (Figure 1). In this review, the neuroprotective effects of CoQ10 on neurological diseases, including AD, depression, epilepsy, MS, PD, stroke, autosomal recessive cerebellar ataxia 2 (ARCA2), Leber's hereditary optic neuropathy (LHON), and spinocerebellar ataxia autosomal recessive 9 (SCAR9) were discussed (Table 2).
Figure 1. CoQ10 is a 1,4-benzoquinone and Q represents the quinone chemical group. Its tail contains 10 isoprenyl chemical subunits (image from the PubChem database).
CoQ should reduce itself following oxidization, which is done using various NAD(P)H oxidoreductases in the plasma membrane, like NAD(P)H: quinone oxidoreductase 1, NADH-cytochrome b5 reductase, or NADPH-CoQ reductase (Rashid et al., 2021). Accordingly, CoQ should be distributed among them, which is regulated by particular proteins (Hidalgo-Gutiérrez et al., 2021; Kemmerer et al., 2021). CoQ is a lipid-soluble compound in the inner mitochondrial membrane (IMM). IMM separates the mitochondrial matrix from the intermembrane space and is an environment for electron transport in the respiratory chain (Sharaf, 2017). In IMM, CoQ can accept electrons from FADH2 through succinate dehydrogenase complex II (CII) and/or NADH through NADH ubiquinone oxidoreductase complex I (CI). The electrons are transported to cytochrome c via CoQH2-cytochrome c reductase complex III (CIII), and cytochrome c can transfer the electrons to the oxygen via cytochrome c oxidase complex IV (CIV). Electron transportation among these complexes is associated with the pumping protons toward the intermembrane space, producing a proton motive force used by the ATP synthase complex V (CV) to generate ATP (Lodish et al., 2008). Electron transportation in the mitochondrial complexes of the respiratory chain (CI, CII, CIII, CIV, and CV) is done by the generation of super-complexes, a supramolecular organization joining the individual complexes in the mitochondria in a structure, where CoQ is an important component (Sharaf, 2017). The CoQH2/CoQ ratio as a sensor for the mitochondria metabolic status modulates electron flow direction in the mitochondrial respiration as well as the generation of mitochondrial complexes/super-complexes (Guaras et al., 2016). CoQH2/CoQ ratio plays a key role in using the alternative oxidase (AOX) to accept electrons from CoQ and cause a reduction in the CoQH2/CoQ ratio, leading to a decrease in rearranged during transfection (RET) and ROS generation (Szibor et al., 2020).
Mitochondria as dynamic organelles alter their shape, size, number, and location in response to environmental changes, in the health state. In the disease state, fission and fusion, as mitochondrial dynamics, exhibit some alterations. In mitochondrial fission, the mitochondria face division and two mitochondria are fused into one for mutual advantage (Chan, 2012). The absence of fission results in mitochondrial dysfunction, including mitochondria interconnection and elongation and motility loss toward the cell periphery. The absence of fusion causes mitochondrial fragmentation as well as ultrastructural impairments and consequently, dysfunction (Srivastava, 2017). Alterations in mitochondrial dynamics are controlled by some proteins. Dynamin-related protein 1 (Drp1) and fission protein 1 (Fis1) are two important fission protein markers. Drp1 is the main regulator of mitochondrial fission and Fis1 is a partner protein of Drp1 (Losón et al., 2013).
Orally administrated water-soluble CoQ10 enhanced bioavailability compared to lipid-soluble CoQ10 (Cui et al., 2021). Water-soluble CoQ10 is not natural and can be prepared artificially. The natural CoQ10 is lipid-soluble (Wear et al., 2021). CoQ can inhibit mitochondrial fission and improve mitochondrial dynamics by decreasing Drp1 and Fis1 proteins (Li et al., 2017). Moreover, treatment with CoQ10 inhibits mitochondrial fission in hydrogen peroxide-treated astrocytes of the optic nerve head (ONH) (Noh et al., 2013). Furthermore, CoQ10 prevents the trauma-induced phosphorylation of Drp1 and blocks the fission-induced activity of Drp1 (Zhang et al., 2021). CoQ10 partially inhibits the astrocyte mitochondrial structure against oxidative stress-related mitochondrial fission (Moreira et al., 2010). Moreover, Some CYP450 isoforms, such as CYP 2D6 or 2E1, may be involved in the development of neurodegenerative diseases. In an in vitro model, CYP induction causes neurorepair. The toxic effect of MPP+ on cell viability in undifferentiated neuroblastoma SH-SY5Y cells treated with the CYP inducers, β-naphthoflavone (βNF) and ethanol (EtOH), before and during exposure to the parkinsonian neurotoxin, was rescued by both βNF and EtOH treatments. Neuroprotective effect of CYP inducers was due to a decrease in ROS production, restoration of mitochondrial fusion kinetics, and mitochondrial membrane potential (Fernandez-Abascal et al., 2020). Furthermore, the hydroxy analog of CoQ10 can be produced by cytochrome P450 (CYP450) of mitochondria (Slowik, 2019).
Ultraviolet B irradiation causes the augmentation of ROS, which is highly toxic to many types of cells and leads to lipid peroxidation (LPO), protein oxidation, and mutagenesis (Pathak et al., 2019). ROS-induced damage can be prevented by CoQ10 in the neuronal cells and astrocytes. Therefore, CoQ10 stabilizes the mitochondrial membrane potential, protects the mitochondria from oxidative damage, improves mitochondrial respiration, inhibits the mitochondria-mediated cell death pathway, and activates mitochondrial biogenesis (Jing et al., 2015). Furthermore, CoQ10 by scavenging ROS protects neurons against oxidative stress in several neurodegenerative disorders and protects ONH structures and astrocyte components (Nakazawa et al., 2019). Prokaryotes and eukaryotes have similar CoQ10 biosynthesis: a long polyisoprenoid lipid tail attaches to a benzenoid precursor, followed by modifying the benzenoid ring through successive steps to obtain the ultimate product (Pierrel et al., 2022). In eukaryotes and some prokaryotes, the isoprene carbon units are obtained from the mevalonate pathway to make the CoQ side chain (Fernández-del-Río and Clarke, 2021), or the deoxyglucose-5-phosphate pathway in plants, prokaryotes, and some protozoa (Wang and Hekimi, 2016). CoQ using a long polyisoprenoid tail is anchored at the phospholipid bilayer midplane. Mutations in several CoQ and PDSS genes are linked to primary CoQ10 deficiency, while mitochondrial DNA (mtDNA) mutations result in secondary CoQ10 deficiency. CoQ5 and CoQ9 proteins are found in many mitochondrial protein complexes in human 143B cells and CoQ9 and CoQ5 knockdown inhibits CoQ10 levels (Yen et al., 2020). There are some antibodies and mitochondrial localizations of mature proteins for such proteins, except CoQ2 and PDSS1. There are also some PDSS2 and CoQ3 isoforms. PDSS1, CoQ3, and PDSS2 are involved in preserving the stability of the other proteins (Chen et al., 2013). In the mitochondria, some protein complexes contain CoQ3, CoQ4, CoQ6, CoQ7, or PDSS2 protein. There are two specific PDSS2-containing protein complexes. Their transient knockdown, except CoQ8 and CoQ6, reduced CoQ10 levels, but just CoQ7 knockdown could hamper mitochondrial respiration and elevated ubiquinol to ubiquinone ratios and also cause the accumulation of a putative biosynthetic intermediate characterized by reversible redox property, like CoQ10 (Yen et al., 2016). Also, PDSS2 suppressed the concentrations of different CoQ proteins (not CoQ3 and CoQ8A) that can be detected in cybrids consisting of the pathogenic mtDNA A8344G mutation or in 143B cells treated with carbonyl cyanide p-trifluoro-methoxyphenyl hydrazone (FCCP), which is consistent with our previous results for CoQ5 (Yen et al., 2011, 2016). These new findings may shed light on the possible centome of CoQ synthome in human cells as well as the understanding the role of PDSS and CoQ proteins in pathological and physiological conditions (Wang et al., 2022). The CoQ10 and CoQ levels showed a negative correlation with malignancy degree and a positive correlation with citrate synthase (CS) activity, while PDSS2 levels showed a positive correlation with malignancy. Also, lower mitochondrial DNA-encoded cytochrome c oxidase subunit 2 levels showed no association with a higher malignancy degree and lower CoQ protein levels. Mitochondrial abnormalities are linked to defected CoQ10 maintenance in the progression of human astrocytoma (Yen et al., 2020). Homozygous mutations in humans in both genes caused severe neuromuscular disease, with nephrotic syndrome observed in PDSS2 deficiency. Presumed autoimmune kidney disease with the missense Pdss2kd/kd genotype is possibly because of a mitochondrial CoQ biosynthetic defect in mice (Yen et al., 2022).
CoQ10 deficiencies are genetically and clinically heterogeneous. The syndrome has five main clinical phenotypes: (1) cerebellar ataxia, (2) severe infantile multisystemic disease, (3) encephalomyopathy, (4) isolated myopathy, and (5) nephrotic syndrome. In some cases, pathogenic mutations are observed in genes associated with the CoQ10 biosynthesis (primary CoQ10 deficiencies) or those not directly associated with CoQ10 biosynthesis (secondary CoQ10 deficiencies). The pathogenesis of primary CoQ10 deficiencies has been linked to respiratory chain defects, ROS generation, and apoptosis variably (Peng et al., 2008). Primary deficiency due to mutations in genes is associated with CoQ10 biosynthesis. Secondary deficiency is possibly associated with hydroxymethylglutaryl coenzyme A (HMG-CoA) reductase inhibitors (statins), which are used to treat hypercholesterolemia. CoQ10 dietary contributions are very small; however, supplementation can increase plasma CoQ10 levels (Quinzii and Hirano, 2011). CoQ10 is highly safe with limited adverse events. Several clinical trials have been done using some CoQ10 doses. Adverse gastrointestinal effects, such as nausea, are not due to the active ingredient because of no reported dose-response relationship. Daily intakes of 1,200 mg do not cause adverse effects than the dose of 60 mg (Potgieter et al., 2013). According to the “observed safe level” risk assessment method, CoQ10 is safe at up to 1,200 mg/day (Hathcock and Shao, 2006). CoQ10 plays a role in the reduced International Normalized Ratio (INR) in patients who use warfarin (Shalansky et al., 2007). Engelsen et al. (2003) reported alterations in prothrombin time, and INR levels are not important in patients on stable, long-term warfarin therapy receiving 100 mg of CoQ10 for 4 weeks. Mitochondrial cells have oxidized (ubiquinone) and reduced (ubiquinol) species of CoQ10. Ubiquinol is an antioxidant and is oxidized to ubiquinone in free radical reactions, limiting LPO. The ubiquinol reverse reduction activates endogenous regeneration systems (tocopherol and ascorbate) (Gille et al., 2008). The ratio between oxidized and reduced CoQ10 species is a biomarker for oxidative stress in vivo (Kalenikova et al., 2018). The ubiquinone therapeutic effectiveness has been reported in many diseases affecting this pathogenetic factor (Chan et al., 2015). Ubiquinol is the reduced form of CoQ10, associated with antioxidant function. Hence, the tissues and cells should have molecular mechanisms to recover their active form, including the dihydroorotate dehydrogenase action in the IMM, causing pyrimidine biosynthesis and reducing ubiquinone through the oxidation of dihydroorotate to rotate. In addition, the CoQ10 involvement in the flavoprotein/electron transfer of flavoprotein to ubiquinone ratio is needed in the oxidoreductase system, making the ubiquinol able to be recovered by involvement in the oxidation of the fatty acids (Littarru and Tiano, 2010). The stepwise one-electron oxidation of the two hydroxyl groups on the benzoquinone ring leads to three redox states of CoQ: the fully reduced ubiquinol (UQH2), the half-reduced ubisemiquinone radical (UQH• in the protonated form), and the fully oxidized ubiquinone (UQ) (Cedeno, 2010). Also, its tail contains ten isoprenyl chemical subunits (Matthews et al., 1998; Geromel et al., 2002). CoQ10 is a crucial cofactor to produce ATP in the electron transport chain (ETC) (Manzar et al., 2020). This coenzyme delivers electrons from complexes I and II and transfers them to complex III (Alcázar-Fabra et al., 2016). Moreover, an increase in the expression of mitochondrial uncoupling proteins (UCPs) demonstrates the antioxidant role of CoQ10 (Persson et al., 2012). CoQ10 as an important endogenous antioxidant is a crucial component of the mitochondrial respiratory chain (MRC). CoQ molecules are dynamically divided in a pool attached to and engulfed by the super-complexes I + III, and another pool related to complex II or other mitochondrial enzymes using CoQ as a cofactor. Such a CoQ-free pool can be applied by enzymes linking the MRC to other pathways, like the fatty acid β-oxidation and amino acid catabolism, pyrimidine de novo biosynthesis, proline, arginine, and glyoxylate metabolism, glycine metabolism, and sulfide oxidation metabolism that some of them are attached to metabolic pathways in other compartments (Pradhan et al., 2021). The antioxidant function of CoQ10 is because of its completely reduced ubiquinol form. Thus, a CoQ10 deficiency can cause some diseases due to a failure in energy metabolism and compromising cellular antioxidant capacity. Several diseases are caused by CoQ10 deficiency, such as heterogeneous MRC disorders. Defects in cellular CoQ10 status are due to its primary or secondary deficiency (Neergheen et al., 2019; Hargreaves, 2021).
Based on these findings, the antioxidant impact of the CoQ10 declines the function of inflammatory factors evidenced by gene expression analysis as well as cell culture assessments (Hargreaves, 2021). The robust neuroprotective effects of the CoQ10 on neurotoxicity have been shown in many in vitro investigations and also animal models of neurological diseases (Spindler et al., 2009).
Online databases, such as Scopus, Google Scholar, Web of Science, and PubMed/MEDLINE were systematically searched until December 2020 using keywords, such as review, neurological disorders, and CoQ10.
In 2017, an estimated 700,000 American people aged ≥ 65 years were found with AD when they died (Alzheimer's Association, 2017). AD is also known as the prevalent type of dementia (Wang et al., 2014). Deficits in memory and learning, defects in thinking, and behavioral signs are the symptoms of dementia (Alzheimer's Association, 2013; Shekarian et al., 2020). Recently, genetic (Picone et al., 2016a) and environmental (Chen et al., 2009; Picone et al., 2016b) risk factors were found effective in the pathogenesis of AD. The accumulation of Aβ senile plaques plays an important role in AD pathogenesis (Golde et al., 2000; Kowalska, 2004). It Presenilin 1 (PS-1) mutation (L235P: proline by leucine substitution at codon 235) causes the formation of Aβ42 and Aβ40 in the brains of transgenic mice and cultured cells (Borchelt et al., 1996; Duff et al., 1996) and leads to AD (Schellenberg et al., 2000; Xia, 2000; Yang et al., 2008). CoQ10 diminished plaque pathology in an amyloid precursor protein (APP)/PS1 mouse model of AD. Therefore, CoQ10 might be a therapeutic candidate for the treatment of AD (Yang et al., 2010).
According to in vitro investigations, Aβ induces oxidative stress. For instance, Aβ increases the hydrogen peroxide (H2O2) and lipid peroxide concentrations in cultured cells, and antioxidants, like vitamin E with a protective role in neurons against Aβ-related cytotoxicity (Chan and Shea, 2006). Oxidative stress can cause aging and results from the imbalance between oxidants and antioxidants (Wang et al., 2014; Jiang et al., 2016; Butterfield, 2018; Shekarian et al., 2020). Accordingly, oxidative stress causes AD (Markesbery, 1997; Santos et al., 2004; Swerdlow, 2012; Bonda et al., 2014). Both mitochondrial dysfunction and oxidative damage lead to Aβ deposition in AD (Beal, 2004). CoQ10 is capable of scavenging free radicals (Gazdík et al., 2003; Gvozdjáková et al., 2022). The synthesis of CoQ10 is diminished in older people (Borek, 2004). CoQ10 inhibited apoptotic death and damage caused by ROS (Li et al., 2014) and improved AD (Dumont et al., 2011). Oral administration of CoQ10 (10 mg/kg b.wt., i.p. in corn oil) in AD rats reduced thiobarbituric acid reactive substances (TBARS) and elevated the activity of antioxidant enzymes in the brain (Ishrat et al., 2006). Vinpocetine (VIN) and CoQ10 (200 mg/kg, suspended in saline) in combination with physical and mental activities caused a significant attenuation in the neurodegeneration due to AlCl3 administration by improving AD markers in brain tissue and inflammatory and oxidant markers (Ali et al., 2019). Treatment of hypercholesterolemia rats with Co-Q10 alone or in combination with omega-3 (1,000 mg) regulated cholinergic functioning, reduced brain inflammation and oxidative stress, and increased the functional outcome verified through the histopathological evaluation of brain tissues (Ibrahim Fouad, 2020).
The synaptic mechanisms of learning and memory in vertebrates are studied by long-term potentiation (LTP) of the hippocampal synaptic transmission (Bliss and Collingridge, 1993). The long-term synaptic plasticity in the hippocampus (HIP) is suppressed by Aβ peptides (Chen et al., 2000; Asadbegi et al., 2016; Ramezani et al., 2020a). Ubisol-Q10 in drinking water (at ~6 mg/kg/day) could decrease circulating Aβ peptide, improve long-term memory, preserve working spatial memory, and inhibit Aβ plaque generation in transgenic mice aged 18 months compared to untreated transgenic mice (Muthukumaran et al., 2018). The effects of oral administration of CoQ10 (50 mg/kg/oral gavage/daily) on the hippocampal synaptic plasticity in animals subjected to Aβ injection were examined by field potential recording methods and the results showed an improvement in memory and neuroplasticity of neurons following CoQ10 administration (Komaki et al., 2019) (Figure 2).
Figure 2. Oxidative stress and mitochondrial dysfunction lead to the formation of β-amyloid (Aβ) senile plaques. Deposition of Aβ increases the activity of acetylcholinesterase (AChE). Also, oxidative stress leads to apoptosis and memory impairment by activating glial cells. The antioxidant and anti-apoptotic effects of CoQ10 improve memory. AIF, apoptosis-inducing factor; AchE, acetylcholinesterase.
The administration of CoQ10 alone or in combination with other antioxidants improved learning and memory and prevented oxidative stress, inflammation, and cellular death in various models of AD and frontotemporal dementia, including aged rodents with aluminum-induced AD, rats with forebrain lesions, rats receiving ICV infusion of Aβ-42 or STZ, transgenic mice with different mutations inducing AD or frontotemporal dementia, and cell cultures using different human or rodent cells (Jiménez-Jiménez et al., 2023). Initial short-term randomized clinical trials have shown an improvement in several neuropsychological tests in AD patients treated with CoQ10 in comparison with those receiving the placebo due to the potential effectiveness of CoQ10 (Weyer et al., 1997; Gutzmann and Hadler, 1998). A systematic review was conducted to examine the possible therapeutic effects of CoQ10 in experimental models of AD and other dementias, as well as in humans with AD and mild cognitive impairment. The potential role of CoQ10 treatment in AD and improving memory in aged rodents in experimental models deserves future studies on patients with AD through the assessment of other causes of dementia and mild cognitive impairment (Jiménez-Jiménez et al., 2023).
Depression as a neurological disorder is caused by the lack of serotonin (Anderson and Maes, 2014). Lower serotonin levels can be a major outcome of tryptophan metabolism shift to kynurenine formation (Caspi et al., 2003; Oxenkrug, 2010). The kynurenine pathway degrades more than 95% of tryptophan (a precursor to serotonin). In tryptophan oxidation, indoleamine 2, 3-dioxygenase 1 (IDO 1) is a rate-limiting enzyme. The tryptophan-to-kynurenine conversion plays a role in the development of depression (Dantzer et al., 2008). Most people experience repeated episodes of mood disorders, psychosocial morbidity, and high use of healthcare services, which persist into later life (Bartels et al., 2000). Bipolar depression is the significant and least effectively treated stage of bipolar disorder (BD). BD is an aging-associated disease and longer periods of BD are spent depressed instead of having manic/hypomanic and cycling/mixed symptoms (Kalin, 1996; Judd et al., 2002, 2003). In BD, the proportion of the time spent in depressive episodes is more than the time spent in manic episodes (Forester et al., 2015). Treatment of BD has not been widely considered and also treating depressive symptoms is not easily achievable in the manic stage (Konradi et al., 2004).
Lower plasma CoQ10 concentrations in depressed patients were compared with healthy controls (Maes et al., 2009; Lesser et al., 2013). Treatment with CoQ10 (400 mg/d titrated up by 400 mg/d every 2 weeks up to 1,200 mg/d) diminished depression severity in patients with BD (Forester et al., 2012). Also, CoQ10 is effective in the pathophysiology of many disorders linked to depressive symptoms, like fibromyalgia (FM), major depression, and myalgic encephalomyelitis (Forester et al., 2012; Aboul-Fotouh, 2013). CoQ10 [25, 50, 100, and 150 mg/kg/day, i.p dissolved in 1% dimethyl sulfoxide (DMSO)] was injected into the HIP for 40 days, and its effect was assessed on serotonin levels in platelets of cases with FM. The results showed improved depressive symptoms compared to cases treated with the placebo (Alcocer-Gómez et al., 2014). CoQ10 (50, 100, and 200 mg/kg/day, dissolved in 1 % DMSO) received for 6 weeks exhibited significant antidepressant effects indicated by a significant decrease in stress-induced alterations in the forced swim test (FST) and open field test, and also a decrease in corticosterone levels and the weight of adrenal glands (Abuelezz et al., 2017). A high dose of CoQ10 (500 mg/day) caused an improvement in depression in older adults with BD (Forester et al., 2012).
Mitochondrial dysfunction, oxidative stress, and inflammation are involved in the BD pathophysiology. Inflammatory responses, such as elevated leucocyte and neutrophil counts and plasma concentrations of proinflammatory cytokines and their receptors are found in cases of severe depression. On the other hand, major depression is linked to reduced antioxidant levels as well as induced nitrosative and oxidative pathways (Maes et al., 2011). ROS, like hydroxyl radical, superoxide, H2O2, and peroxynitrite, are effective in the pathogenesis of major depression (Lucca et al., 2009a,b) (Figure 3). It CoQ10 plays a role in depression pathophysiology through the anti-inflammatory and neuroprotective properties and suppresses the generation of pro-inflammatory cytokines (Schmelzer et al., 2008; Mohamed and Said, 2021). Furthermore, the anti-inflammatory, antioxidant, and mitochondrial modulatory effects of CoQ10 (200 mg/d) administrated for 8 weeks are involved in its effectiveness in the treatment of BD patients (Mehrpooya et al., 2018). Reduced levels of CoQ10 are associated with an elevated level of tumor necrosis factor-alpha (TNF-α) and oxidants, such as ROS (Schmelzer et al., 2008; Leonard and Maes, 2012). In another study, treatment with CoQ10 (500 mg/kg/day, gavage) reduced LPO and elevated GSH levels and total antioxidant capacity (TAC) values, and SOD and glutathione peroxidase (GPx) activities in both brain areas of mice. The suppressed neuro-inflammatory response in the prefrontal cortex (PFC) and HIP was observed, evidenced by reduced NF-kB, p38, and JNK concentrations in the CoQ10 groups (Salehpour et al., 2019). Moreover, the CoQ10 antioxidant effect was exhibited by its capability to significantly decrease elevated hippocampal 4-hydroxynonenal and MDA levels and increase the decreased catalase and glutathione levels. Furthermore, CoQ10 caused a significant reduction in the levels of different pro-inflammatory cytokines, such as interleukin-1β (IL-1β), IL-2, IL-6, and TNF-α (Abuelezz et al., 2017). Therefore, CoQ10 due to its anti-inflammatory and anti-oxidative effects can be used to treat depression.
Figure 3. The effects of CoQ10 on depression by inhibiting inflammation and stress oxidative pathways.
Effect of CoQ10 (100 mg/kg/day) or/and fluoxetine (10 mg/kg/day) was assessed on mRNA expression, 5-HT1A and 5-HT2A receptors, GSK-3β, phosphorylated (p)GSK-3β, CREB, pCREB, and BDNF protein expression in rats receiving chronic unpredictable mild stress (CUMS) for 6 weeks (Abuelezz et al., 2018). Recently, we assessed the effects of CoQ10 (10 mg/kg, dissolved in corn oil) on behavioral dysfunction and CoQ10 levels in the rat brain. A significant difference was found between the depression induced by streptozotocin (STZ) and control groups tested by the splash test and FST 24 h after STZ treatment. In addition, according to the validated and accurate high-performance liquid chromatography (HPLC), reduced CoQ10 levels were found in the brain of the STZ group (Andalib et al., 2019). Supplementation with CoQ10 (500 mg/day) could improve depression in bipolar patients (Forester et al., 2012).
Epilepsy is the commonest neurological disease worldwide, in which spontaneous unusual electrical discharges of neurons are observed throughout the brain (Patel, 2002). Temporal lobe epilepsy (TLE) is the commonest form of epilepsy in adults that is commonly linked to hippocampal sclerosis, neurodegeneration, and hippocampal circuit reorganization (Jokeit and Schacher, 2004). An animal model of TLE was designed by unilateral intrahippocampal kainic acid injection in rodents. It was a “post-status” model where epilepsy can spread following the agent-induced status epilepticus (SE) (Löscher, 2002). These models are used to study the effects of possible antiepileptic agents because in the time interval between the status and the first spontaneous seizures, we can examine the effect of neuroprotective and prophylactic agents on epilepsy (Sharma et al., 2007). Epilepsy approximately affects 0.5–1% of the general population (Hauser et al., 1991). Contrary to the prevalence of recent effective antiepileptic agents in cases with epilepsy, novel antiepileptic agents are used with stronger anticonvulsant activity (Sattarinezhad et al., 2014). Therefore, new therapeutic strategies have been established to prevent or even reverse the molecular and cellular mechanisms of epileptogenesis (Löscher and Schmidt, 2006).
CoQ10 caused a reduction in the kainate-related model of epilepsy (Yalcin et al., 2004). CoQ10 has anti-apoptotic and antioxidant effects (Papucci et al., 2003). Moreover, CoQ10 (0.01, 0.1, and 1 mM) received for 3 weeks exerted a neuroprotective effect on the HIP against kainate neurotoxicity in vitro (Won et al., 2011; Kumar et al., 2022), and blunts cell death and cellular apoptosis in the hippocampal CA3 area after SE (Chuang et al., 2009). The neuroprotective properties of CoQ10 (i.p. at 10 mg/kg/day) dissolved in normal saline in the intrahippocampal kainate model of TLE have not yet been identified and are under investigation (Baluchnejadmojarad and Roghani, 2013).
Nitric oxide (NO) is a regulator of seizure activity because of its various anticonvulsant (Starr and Starr, 1993; Theard et al., 1995; Tsuda et al., 1997) and proconvulsant (Osonoe et al., 1994; Van Leeuwen et al., 1995; Nidhi et al., 1999) effects depending on the seizure type, the source of NO, and other neurotransmitter system contentions. Treatment with CoQ10 (10 mg/kg i.p) decreased inducible and endothelial NO generations in a rat with testicular ischemia/reperfusion injury (Erol et al., 2010). Also, CoQ10 (1.5 mg/kg, dissolved in serum physiologic administrated daily for 15 days by gavage) showed a decreasing effect on increasing induced- and endothelial nitric oxide synthase (iNOS and eNOS) expression levels in the hyperthyroid heart (Oztay et al., 2007). However, the oxidized low-density lipoprotein-associated down-regulation of eNOS is declined by CoQ10 (Tsai et al., 2012). In addition, this coenzyme, dissolved in corn oil, increases the aortal eNOS activity in acrylonitrile-related vascular endothelial abnormalities in rats (Guo et al., 2011).
In another study, CoQ10 (50 mg/kg) increased the total number of spike-wave discharges (SWDs) but did not change the mean duration of SWDs. CoQ10 (100 and 200 mg/kg) increased both the total number and the mean duration of SWDs abolished by coadministration of 7-nitroimidazole (7-NI, NOS inhibitor). Coadministration of l-arginine (l-Arg, an essential substrate for the synthesis of NO) (500 and 1,000 mg/kg) enhanced the CoQ10 effect on the total number of SWDs but not on its mean duration. Therefore, the effect of CoQ10 on seizure was attenuated by the NOS inhibitor. Furthermore, based on the electrophysiological evidence, CoQ10 administration increased the absence seizures through the stimulation of the neuronal NOS (Gunes et al., 2019). Therefore, the inhibition of NOS decreased the seizure activity of CoQ10.
In contrast, subchronic oral application of CoQ10 (100 mg/kg or more) enhanced time latencies to the onset of myoclonic jerks and clonic seizures induced by intraperitoneal pentylenetetrazole (PTZ) and at the doses of 25 mg/kg or more augmented the seizure threshold provoked by intravenous injection of PTZ. Subchronic doses of CoQ10 (50 mg/kg or more) reduced the tonic seizures induced by PTZ or electroshock. This antiseizure effect of subchronic CoQ10 was attenuated by the NOS inhibitor (Sattarinezhad et al., 2014). Therefore, the interaction between NO and subchronic CoQ10 in antiseizure activity is possibly accomplished by the induction of NOS.
Basically, non-inflammatory mechanisms, such as mitochondrial dysfunction cause MS (Kalman et al., 2007). Free radicals play a role in MS pathogenesis and enhance the transendothelial migration of leukocytes resulting in oligodendrocyte injury and axonal degeneration (Van Horssen et al., 2011). Macrophages produce free radicals, including NO, ROS, reactive nitrogen species, microglia, and astrocytes, which all damage the neurons, axons, myelin, and oligodendrocyte (Lee et al., 2012b) (Figure 4).
Figure 4. Inflammation, neuronal demyelination, mitochondrial dysfunction, destruction of axons and oligodendrocytes, and oxidative stress are the main pathological causes of multiple sclerosis (MS). CoQ10 improves the disease by reducing the activity of microglia and macrophages and the production of reactive oxygen species (ROS).
Also, inflammation, multifocal demyelination, loss of oligodendrocytes, breakdown of the blood-brain barrier (BBB), neural and axonal injury, and oxidative stress are the causes of MS (Van der Walt et al., 2010; Riccio, 2011). Inflammatory markers, ROS, and matrix metalloproteinases (MMPs), as the factors to enhance BBB permeability, can be released by the infiltrated activated leukocytes in MS cases (Larochelle et al., 2011). Pro-inflammatory factors, like TNF-α, IL-1, IL-6, and interferon (IFN)-γ, increase the cerebrospinal fluid, serum, and brain lesions in MS cases. They have relatively low concentrations of transforming growth factor-β and IL-4 (Miller et al., 1998; Spooren et al., 2011).
Relapsing-remitting MS (RRMS), progressive-relapsing MS, primary progressive MS, and secondary progressive MS are different MS types (Adamczyk-Sowa et al., 2012). RRMS is linked to immune-mediated reactions, like white matter inflammation, microglial activation, and cell infiltration in the CNS (Van Horssen et al., 2011; da Silva Fernandes et al., 2012). T cells of the T-helper type 1 (Th1) macrophages play a role in the immunopathogenesis of the CD4+ demyelination, whereas remission of the disease is induced by T cells of the Th2 and Th3 phenotypes (Miller et al., 1998). The immune system plays a role in the development of depression associated with MS.
Pro-inflammatory cytokines, like TNF-α, induce weight loss, anorexia, anxiety, locomotor retardation, and reduced social exploration (Kidd, 2003). CoQ10 supplementation (60–150 mg/d) reduced inflammatory cytokines in the serum of human models (Lee et al., 2012a). Schmelzer et al. (2009) reported that the lipopolysaccharide (LPS)-related pro-inflammatory markers and chemokines decreased following preincubation of human THP-1 cells using ubiquinol-10 (QH2). Fouad and Jresat prepared CoQ10 (i.p. injections, 10 mg/kg) supplementation in a 1% aqueous solution of Tween 80. This antioxidant could reduce NF-κB and iNOS expression levels in the rats' livers (Fouad and Jresat, 2012). Treatment with CoQ10 (150 mg/kg/day) via gavage once a day for 12 weeks, could alleviate stress oxidative status caused by Cuprizone (CPZ) and significantly inhibit inflammatory biomarkers. CoQ10 enhances remyelination in the CPZ model (Khalilian et al., 2021). CoQ10 treatment (500 mg/day) improved depression and fatigue in MS patients (Sanoobar et al., 2016). Clinical symptoms in animals with experimental autoimmune encephalomyelitis (EAE) were markedly reduced (P < 0.05) by CoQ10 compared to controls. Also, the TNF-α level showed a significant decrease following CoQ10 administration (10 mg/kg/three weeks) vs. IL-10. The TH1/TH2 ratio in CoQ10-treated animals showed a significant decrease than in non-treated animals (P < 0.01) (Soleimani et al., 2014). In a randomized, double-blinded, placebo-controlled trial, patients treated with CoQ10 showed a significant elevation in SOD activity (p = 0.013) and a reduction in MDA concentrations (P = 0.003) than controls. Although CoQ10 supplementation significantly affected plasma TAC values (p = 0.010), no significant difference was found between both groups. CoQ10 supplementation had no effect on GPx activity (Sanoobar et al., 2013).
PD is caused by the degradation of dopamine (DA) neurons in the SNpc. Over 95% of PD patients are sporadic and PD is developed in cases older than 60 years (Steece-Collier et al., 2002; Corti et al., 2005). The symptoms of this disorder include resting tremors, postural instability, rigidity, and bradykinesia (Colnat-Coulbois et al., 2005). Although the causes of sporadic have not yet been discovered, different environmental risk factors, like neurotoxins can be involved (Di Monte, 2003). PD-like symptoms in experimental animals are induced by environmental toxins, including paraquat (N, N′-dimethyl-4,4′-bipyridinium dichloride), rotenone, and maneb (Betarbet et al., 2002). Such neurotoxins inhibit complex I in the mitochondrial ETC. In normal circumstances, DA neurons also face a high level of oxidative stress because of ROS generation during DA metabolism (Dexter et al., 1994).
ATP depletion and oxidative stress production cause neuronal death. Oxidative stress, activation of the microglia, neuroinflammation, mitochondrial damage, protein aggregation due to defective clearance, and autophagic stress are the major events in the pathophysiology of PD (Kones, 2010). The disparity in mitochondrial dynamics causes augmentation of neuronal loss observed in PD patients (Srivastava, 2017). One of the main non-motor symptoms of the disease is PD with mild cognitive impairment (PDMCI). The early identification of PDMCI and treatment of this disease are of critical importance to improve the quality of life and prognosis in PD patients (Kwon et al., 2022).
The levodopa-3,4-dihydroxyphenylalanine (L-DOPA) administration is the initial treatment for PD (Nutt, 1990). Diminution of movement owing to delayed movement initiation (akinesia) is an important reason for disability in PD (Lundblad et al., 2002). L-DOPA pharmacotherapy can alleviate such symptoms. Moreover, prolonged treatments in the majority of patients lead to drug-induced abnormal involuntary movements (dyskinesia) (Cenci, 2007).
Currently, PD cannot be cured; however, neuroprotectants reduce the rate of neurodegeneration and improve the quality of life (Koller and Cersosimo, 2004). CoQ10 has shown neuroprotective activity in some neurodegenerative diseases, like PD (Mancuso et al., 2010; Wear et al., 2021). Screening for oxidative stress markers in patients with neurodegenerative disease, such as PD showed lower CoQ10 concentrations and higher lipoprotein oxidation levels in the cerebrospinal fluid, plasma, and brain cortex than in non-affected cases. Affected patients showed an increase in the levels of mitochondrial oxidative stress due to low CoQ10 levels because CoQ10 administration could improve the clinical symptoms of some patients (Jing et al., 2015). Thus, antioxidants, like CoQ10 and vitamin E, are used in both preclinical investigations and clinical trials using animal models (Shults et al., 2002; Beal, 2003; Mcdonald et al., 2005; Cleren et al., 2008; Kadian et al., 2022). In a model of PQ-related neurodegeneration in male Long-Evans rats, the water-soluble CoQ10 [WS-CoQ10; 50 mg in PBS (phosphate-buffered saline)] in drinking water was used to counteract the toxic effect of PQ. PQ induction resulted in oxidative stress and the lack of DA neurons in SNpc, which can affect the motor skill of the animals during the rotarod test. Such PD-like behavioral symptoms showed an improvement in rats treated with WS-CoQ10 added to the drinking water (Somayajulu-Nitu et al., 2009). WS-CoQ10 is not natural but can be artificially prepared. The natural CoQ10 is lipid-soluble (Parmar et al., 2015).
In another study, the neuroprotective effect of Ubisol Q10 (including 50 g/ml of CoQ10 and 150 g of PTS/ml) was assessed in the DJ-1/1-methyl-4-phenyl-1,2,3,6-tetrahydropyridine (MPTP) model of PD through histochemical and behavioral approaches. Application of Ubisol-Q10 could remarkably offset the neurotoxicity and ameliorate motor impairment by MPTP (Muthukumaran et al., 2014). The combination of creatine and CoQ10 treated the MPTP-associated PD model in mice, suppressed the loss of neurons containing tyrosine hydroxylase in the SNpc, and also significantly decreased LPO damage and α-synuclein accumulation in the neurons of this area but did not improve the loss of the dopaminergic neurons (Yang et al., 2009). CoQ10 (200 mg/kg/day) provided through the addition of peanut oil (glycerol could not be well-tolerated by mice during preliminary assessments) showed more effectiveness in a mouse model of PD caused by MPTP compared to oxidized CoQ10 at a similar dose. Accordingly, the elevated plasma level of CoQ10 following ingestion of the reduced form can be absorbed more effectively (Cleren et al., 2008) in comparison to the oxidized form. Treatment with CoQ10 (200 mg) for 26 weeks showed a considerable improvement in oligoasthenoteratozoospermia outcomes, which was associated with mitochondrial dysfunction in male subjects (Safarinejad et al., 2012) (Figure 5). In a clinical study, patients with PD consumed CoQ10 at three doses of 300, 600, and 1,200 mg/d for 60 days. The results showed that the first two doses were ineffective and the dose of 1,200 mg/d had healing effects (Shults et al., 2002). Furthermore, in a double-blind study, about 609 patients used CoQ10 at 400 mg daily for 6 months. CoQ10 had few toxic effects on HD, but its long-term treatment and high dose did not reduce the symptoms of the disease (McGarry et al., 2017). To gain neuroprotection, the prophylactic treatment was designed using CoQ10 (200 mg/kg (intraperitoneal) dissolved in saline) two times a week for three consecutive weeks and 30 min prior to paraquat (PQ) exposure. Furthermore, therapeutic interventions using CoQ10 in mice subjected to PQ (24 h following exposure), two times per week through 3 weeks, halted behavioral deterioration and ongoing neurodegeneration. The outcomes of the sustained treatment with CoQ10 for 3 weeks were compared to L-DOPA as the standard drug of choice. CoQ10 caused a notable improvement in most of the behavioral tests and reduced protein carbonyl content in the brain, principally when it was started before rather than after PQ induction of PD. In addition, water-soluble CoQ10 restored mitochondrial morphology and decreased fragmentation and consequently, mitochondrial fusion and improved mitochondrial dynamics, confirming the protective effect of CoQ10 against rotenone (PD-mimicking toxin) toxicity. Modulation of the fission/fusion index is therapeutically useful for the treatment of PD. Thus, water-soluble CoQ10 can be used to treat PD and is effective in other diseases due to mitochondrial dysfunction (Li et al., 2017). Therefore, CoQ10, which defends against mitochondrial damage, makes the progression of PD slow, mostly when started as prophylactic treatment (Attia and Maklad, 2018).
Figure 5. CoQ10 has a neuroprotective effect against Parkinson's disease by inhibiting inflammation, oxidative stress, activation of microglia, protein accumulation, and mitochondrial damage.
Intrastriatal delivery of CoQ10 at a mean rate of 1.8 and 2.6 μg daily, particularly in combination with implantable devices for deep brain stimulation or convection-enhanced delivery, can be effective to prevent neurodegeneration in PD (Park et al., 2020). Dietary CoQ10 supplementation (at 60 and 120 mg/kg of feed; i.p. along with oral saline) showed significant effectiveness in chlorpromazine -induced Parkinsonism-like alterations in mice (Onaolapo et al., 2021). A systematic review study showed decreased CoQ10 levels in the cerebellar cortex, platelets, and lymphocytes, increased total and oxidized CoQ10 levels in the cerebrospinal fluid, and a non-significant trend toward decreased serum/plasma CoQ10 levels in PD patients. Patients with multiple system atrophy (MSA) showed decreased CoQ10 levels in the cerebellar cortex, serum/plasma, cerebrospinal fluid, and skin fibroblasts. Patients with Lewy body dementia (LBD) showed decreased cerebellar cortex CoQ10 and those with progressive supranuclear palsy (PSP) had decreased CoQ10 levels in the cerebrospinal fluid (Jiménez-Jiménez et al., 2022).
Neurodegenerative disorders, including PD (Shults et al., 2002), amyotrophic lateral sclerosis (Ferrante et al., 2005), and HD can be treated with CoQ10 (Flint Beal and Shults, 2003). According to Yang et al. (2009), coadministration of 1% CoQ10 and the control diet, including Purina rodent chow and creatine, reduced MDA concentration (an indicator of LPO and oxidative damage) in substantia nigra pars compacta (SNpc) and showed a therapeutic effect on HD and PD (Prajapati et al., 2017).
Stroke is the third leading cause of mortality after heart disease and cancer. Three-quarters of stroke patients report an ischemic stroke, which can be due to blood vessel obstruction caused by a clot. Considerable advances have been made in neuropharmacology, but the only clinically effective treatments are acetylsalicylic acid and tissue plasminogen activator (Longa et al., 1989). Nonetheless, stroke-related mortality and morbidity rates are still high, and there is a need for the development of new treatments. Inflammation, excitotoxicity, oxidative stress, and apoptosis necrosis, are the main factors associated with lesion progression after ischemia (Ord et al., 2013). The efficacy of recanalization 3 h after limiting the onset of stroke symptoms has been approved in many patients.
Oxidative stress is the pathological mechanism of cerebral ischemia (Rodrigo et al., 2013) (Figure 6). In the ischemic/reperfusion injury, excessive reactive oxygen formation is unique. Inhibition of antioxidants damages the structure and function of cells (Simani et al., 2018). Therefore, nerve cells should be protected against oxidative stress (Flint Beal and Shults, 2003; McCarthy et al., 2004). MDA is the latest product of LPO that is increased in ischemic stroke patients depending on the infarct size, the stroke severity, and the patient's outcome (Allen and Bayraktutan, 2009). Therefore, elevated concentrations of MDA in ischemic stroke patients have been reported in many studies (Simani et al., 2018). The decreased superoxide dismutase (SOD) activity in acute ischemic stroke has been observed in previous studies (Cherubini et al., 2000; Demirkaya et al., 2001; Milanlioglu et al., 2016). This antioxidant enzyme, SOD, can reduce ROS levels (Gupta et al., 2009). CoQ10 scavenges superoxide radicals for the production of oxygen and H2O2 (Lee et al., 2012a).
Different cerebral ischemia models have shown promising therapeutic effectiveness for the constant administration of CoQ10 (Obolenskaia et al., 2020). However, the treatment of such urgent conditions, such as ischemic stroke should be performed with drugs through intravenous injections. The neuroprotective effect of the solubilized CoQ10 (30 mg/kg) dissolved in saline and injected intravenously into animals with cerebral ischemia has been assessed in several studies (Belousova et al., 2016; Obolenskaia et al., 2020). The expression of genes associated with metabolism and intracellular signaling, embryogenesis, cell differentiation, and production of cholesterol and proinflammatory factors, including TNFα is affected by CoQ10 (Groneberg et al., 2005; Schmelzer et al., 2008). UbiA prenyltransferase domain-containing protein 1 (UbiAd1) is involved in CoQ10 generation and can catalyze the conversion of vitamin K1 into vitamin K2 (Mugoni et al., 2013; Povarova et al., 2018). Recently, the neuroprotective impact of vitamin K2 (menaquinone isoform) has been considered (Shearer and Newman, 2014).
Some treatments, including statin use, have been suggested for transient ischemic attack (TIA) (Gargano et al., 2011; Kernan et al., 2014). Several experimental (García-Bonilla et al., 2012) and clinical (Ní Chróinín et al., 2013) examinations have supported the effectiveness of statins in the secondary prevention of stroke outbreaks (Nasoohi et al., 2019). In other studies, the levels of blood CoQ10 in patients were reduced after the administration of atorvastatin (Rundek et al., 2004; Mabuchi et al., 2007), whereas CoQ10 levels showed no reduction in tissues (Rundek et al., 2004). However, CoQ10 in the blood may disturb BBB following an ischemic insult. Atorvastatin exerts its degenerative effects through a decrease in CoQ10 and these effects are associated with the antioxidant-oxidant defense mechanism. Furthermore, co-administration of CoQ10 (200 mg/kg/day; PO/30 days dissolved in almond oil) and atorvastatin could improve stroke outcomes (Nasoohi et al., 2019). In an interventional study, serum CoQ10 levels significantly increased in the supplement-treated acute ischemic stroke (AIS) patients compared to the placebo group. Moreover, CoQ10 (300 mg/day) supplementation significantly improved Mini-Mental State Examination (MMSE) and National Institute of Health Stroke Scale (NIHSS) scores. Nonetheless, no significant difference was found in the Modified Ranking Scale score and MDA, SOD, and glial fibrillary acidic protein (GFAP) levels between the two groups (Ramezani et al., 2020b).
LHON is an acute/subacute painless loss of central vision (Nikoskelainen, 1984; Novotny et al., 1986; Riordan-Eva et al., 1995). Molecular analysis has shown that primary mutations for this disease are point mutations in mitochondrial DNA (mtDNA) at positions 3,460, 11,778, and 14,484 (Wallace et al., 1988; Harding et al., 1995; Puomila et al., 2007). LHON can be associated with movement disorders, spastic paraparesis, cardiac arrhythmia, peripheral neuropathy, and skeletal abnormalities (Shoffner et al., 1995). Progressive visual loss with permanent centrocecal scotoma has been reported in most affected patients (Lessell et al., 1983; Stone et al., 1992; Tanaka et al., 1998). Some patients have symptoms, including ataxia, tremor, posterior column dysfunction, corticospinal tract dysfunction, dystonia, and extrapyramidal rigidity. LHON is associated with numerous neurologic disorders (Chariot et al., 1999).
CoQ10 is effective in the treatment of patients with mitochondrial diseases, such as chronic progressive external ophthalmoplegia (CPEO), Kearns-Sayre syndrome (KSS), and other mitochondrial encephalomyopathies (Ogasahara et al., 1986; Zierz et al., 1989; Chen et al., 1997; Sobreira et al., 1997). In a study, patients received CoQ10 orally for 4 months, and its dose increased from 90 and 160 to 200 mg/day. CoQ10 caused a rapid improvement in visual acuity in these patients (Kuo et al., 2001). In another study, treatment was initiated with 250 mg CoQ10 per day and multiple vitamins, including tocopherol (500 mg), vitamin C (150 mg), vitamin K3 (10 mg), thiamine (10 mg), and riboflavin (10 mg). Gradual improvement in movement disorders occurred within a year. The lactate/pyruvate ratio was normalized at nine, and there were no changes in visual function. Moreover, lesions of the subthalamic nuclei almost entirely disappeared (Chariot et al., 1999).
ARCA2 and SCAR9 is a kind of hereditary CoQ deficiency. This rare ataxia is due to mutations in the aarF-domain-containing kinase 3 (ADCK3) gene as an ortholog of yeast coq8 (Lagier-Tourenne et al., 2008; Mollet et al., 2008). ARCA2 is known for slow progressive gait impairment, exercise intolerance, cerebellar atrophy, epilepsy, and intellectual disability (Mignot et al., 2013). The deficiency of CoQ10 causes MRC disorder (Hargreaves, 2021). A decrease in CoQ10 concentrations in tissues or cultured cells due to biallelic mutations in each of COQ2, COQ4, COQ6, COQ7, COQ8A, COQ8B, COQ9, PDSS1, and PDSS2 genes (COQ genes) involved in the CoQ10 biosynthesis can be observed in this kind of ataxia (Emmanuele et al., 2012; Laredj et al., 2014; Quinzii et al., 2014). Patients with ADCK3 mutations experience a marked improvement following 3 weeks of oral supplementation with CoQ10 (500 mg two times a day) (Shalata et al., 2019).
The purpose of this article was to review the studies using experimental and clinical treatments (Figure 7). Experimental treatments have often been studied in animal models with remarkable results. In addition, clinical studies using a specific dose and duration of treatment have been effective. However, CoQ10 has no effect on some of the symptoms of the disease. The antidepressant effect of this neuroprotective agent has not yet been studied in patients with MS (Sanoobar et al., 2016). Also, in an animal study, contrary to the antioxidant effects of CoQ10, it led to an increase in the aortal eNOS activity in vascular endothelial abnormalities caused by acrylonitrile in rats (Guo et al., 2011). The neuroprotective properties of CoQ10 in the intrahippocampal kainate model of TLE have not yet been identified and more research is needed (Baluchnejadmojarad and Roghani, 2013). In a clinical study, patients with PD were treated with CoQ10 at three doses of 300, 600, and 1,200 mg/d, and only the dose of 1,200 showed a healing effect (Shults et al., 2002). In addition, long-term, high-dose CoQ10 therapy did improve the symptoms of HD (McGarry et al., 2017). In an intervention study on AIS patients, there were no statistically significant differences in the Modified Ranking Scale score, and MDA, SOD, and GFAP levels between the two placebo and supplement-treated groups (Ramezani et al., 2020b). A recent study on patients with LHON showed no changes in visual function after the administration of CoQ10 (250 mg) and other vitamins in the bilateral pallor of the optic disks (Chariot et al., 1999). According to the mentioned studies and their results, more relevant studies are needed in the future.
CoQ10 as an antioxidant and neuroprotective agent can play a role in the treatment of neurological disorders. Although neurological diseases cannot be treated effectively, CoQ10 deficiency is involved in the pathogenesis of epilepsy, stroke, MS, depression, PD, AD, LHON, ARCA2, and SCAR9. More clinical and experimental studies are needed using electrophysiological and behavioral evaluation, genetic targeting, and molecular imaging.
SB, RH, SS, MK-A, AM, MR, and AK literature review and drafting the manuscript. SB and AK critically revision of the manuscript. All authors read and approved of the final manuscript.
This study was supported by Hamadan University of Medical Sciences, Hamadan, Iran (Grant No. 9412257459; IR.UMSHA.1394.582).
The authors declare that the research was conducted in the absence of any commercial or financial relationships that could be construed as a potential conflict of interest.
All claims expressed in this article are solely those of the authors and do not necessarily represent those of their affiliated organizations, or those of the publisher, the editors and the reviewers. Any product that may be evaluated in this article, or claim that may be made by its manufacturer, is not guaranteed or endorsed by the publisher.
Aboul-Fotouh, S. (2013). Coenzyme Q10 displays antidepressant-like activity with reduction of hippocampal oxidative/nitrosative DNA damage in chronically stressed rats. Pharmacol. Biochem. Behav. 104, 105–112. doi: 10.1016/j.pbb.2012.12.027
Abuelezz, S. A., Hendawy, N., and Magdy, Y. (2017). Targeting oxidative stress, cytokines and serotonin interactions via indoleamine 2, 3 dioxygenase by coenzyme Q10: role in suppressing depressive like behavior in rats. J. Neuroimmune Pharmacol. 12, 277–291. doi: 10.1007/s11481-016-9712-7
Abuelezz, S. A., Hendawy, N., and Magdy, Y. (2018). The potential benefit of combined versus monotherapy of coenzyme Q10 and fluoxetine on depressive-like behaviors and intermediates coupled to Gsk-3β in rats. Toxicol. Appl. Pharmacol. 340, 39–48. doi: 10.1016/j.taap.2017.12.018
Adamczyk-Sowa, M., Sowa, P., Pierzchala, K., Polaniak, R., and Labuz-Roszak, B. (2012). Antioxidative enzymes activity and malondialdehyde concentration during mitoxantrone therapy in multiple sclerosis patients. J. Physiol. Pharmacol. 63, 683–690.
Akinyemi, R. O., Ovbiagele, B., Adeniji, O. A., Sarfo, F. S., Abd-Allah, F., Adoukonou, T., et al. (2021). Stroke in Africa: profile, progress, prospects and priorities. Nat. Rev. Neurol. 17, 634–656. doi: 10.1038/s41582-021-00542-4
Alcázar-Fabra, M., Navas, P., and Brea-Calvo, G. (2016). Coenzyme Q biosynthesis and its role in the respiratory chain structure. Biochim. Biophys. Acta Bioenerget. 1857, 1073–1078. doi: 10.1016/j.bbabio.2016.03.010
Alcocer-Gómez, E., Sánchez-Alcázar, J. A., and Cordero, M. D. (2014). Coenzyme q10 regulates serotonin levels and depressive symptoms in fibromyalgia patients: results of a small clinical trial. J. Clin. Psychopharmacol. 34, 277–278. doi: 10.1097/JCP.0000000000000097
Ali, A. A., El-Ella, D. M. A., El-Emam, S. Z., Shahat, A. S., and El-Sayed, R. M. (2019). Physical & mental activities enhance the neuroprotective effect of vinpocetine & coenzyme Q10 combination against Alzheimer & bone remodeling in rats. Life Sci. 229, 21–35. doi: 10.1016/j.lfs.2019.05.006
Allen, C. L., and Bayraktutan, U. (2009). Oxidative stress and its role in the pathogenesis of ischaemic stroke. Int. J. Stroke 4, 461–470. doi: 10.1111/j.1747-4949.2009.00387.x
Alzheimer's Association (2013). Alzheimer's disease facts and figures. Alzheimers Dementia 9, 208–245. doi: 10.1016/j.jalz.2013.02.003
Alzheimer's Association (2017). Alzheimer's disease facts and figures. Alzheimers Dementia 13, 325–373. doi: 10.1016/j.jalz.2017.02.001
Andalib, S., Mashhadi-Mousapour, M., Bijani, S., and Hosseini, M.-J.. (2019). Coenzyme Q10 alleviated behavioral dysfunction and bioenergetic function in an animal model of depression. Neurochem. Res. 44, 1182–1191. doi: 10.1007/s11064-019-02761-0
Anderson, G., and Maes, M. (2014). Oxidative/nitrosative stress and immuno-inflammatory pathways in depression: treatment implications. Curr. Pharm. Des. 20, 3812–3847. doi: 10.2174/13816128113196660738
Asadbegi, M., Yaghmaei, P., Salehi, I., Ebrahim-Habibi, A., and Komaki, A. (2016). Neuroprotective effects of metformin against Aβ-mediated inhibition of long-term potentiation in rats fed a high-fat diet. Brain Res. Bull. 121, 178–185. doi: 10.1016/j.brainresbull.2016.02.005
Attia, H. N., and Maklad, Y. A. (2018). Neuroprotective effects of coenzyme Q10 on paraquat-induced Parkinson's disease in experimental animals. Behav. Pharmacol. 29, 79–86. doi: 10.1097/FBP.0000000000000342
Baluchnejadmojarad, T., and Roghani, M. (2013). Coenzyme q10 ameliorates neurodegeneration, mossy fiber sprouting, and oxidative stress in intrahippocampal kainate model of temporal lobe epilepsy in rat. J. Mol. Neurosci. 49, 194–201. doi: 10.1007/s12031-012-9886-2
Bartels, S. J., Forester, B., Miles, K. M., and Joyce, T. (2000). Mental health service use by elderly patients with bipolar disorder and unipolar major depression. Am. J. Geriatr. Psychiatry 8, 160–166. doi: 10.1097/00019442-200005000-00011
Beal, M. F. (2003). Bioenergetic approaches for neuroprotection in Parkinson's disease. Ann. Neurol. 53, S39–S48. doi: 10.1002/ana.10479
Beal, M. F. (2004). Mitochondrial dysfunction and oxidative damage in Alzheimer's and Parkinson's diseases and coenzyme Q 10 as a potential treatment. J. Bioenerg. Biomembr. 36, 381–386. doi: 10.1023/B:JOBB.0000041772.74810.92
Belousova, M., Tokareva, O., Gorodetskaya, E., Kalenikova, E., and Medvedev, O. (2016). Neuroprotective effectiveness of intravenous ubiquinone in rat model of irreversible cerebral ischemia. Bull. Exp. Biol. Med. 161, 245–247. doi: 10.1007/s10517-016-3387-1
Betarbet, R., Sherer, T. B., and Greenamyre, J. T. (2002). Animal models of Parkinson's disease. Bioessays 24, 308–318. doi: 10.1002/bies.10067
Bigal, M. E., Liberman, J. N., and Lipton, R. B. (2006). Age-dependent prevalence and clinical features of migraine. Neurology 67, 246–251. doi: 10.1212/01.wnl.0000225186.76323.69
Bliss, T. V., and Collingridge, G. L. A. (1993). synaptic model of memory: long-term potentiation in the hippocampus. Nature 361, 31–39. doi: 10.1038/361031a0
Bonda, D. J., Wang, X., Lee, H.-G., Smith, M. A., Perry, G., and Zhu, X. (2014). euronal failure in Alzheimer's disease: a view through the oxidative stress looking-glass. Neurosci. Bull. 30, 243–252. doi: 10.1007/s12264-013-1424-x
Borchelt, D. R., Thinakaran, G., Eckman, C. B., Lee, M. K., Davenport, F., Ratovitsky, T., et al. (1996). Familial Alzheimer's disease–linked presenilin 1 variants elevate Aβ1–42/1–40 ratio in vitro and in vivo. Neuron 17, 1005–1013. doi: 10.1016/S0896-6273(00)80230-5
Butterfield, D. A. (2018). Perspectives on oxidative stress in Alzheimer's disease and predictions of future research emphases. J. Alzheimers Dis. 64, S469–S79. doi: 10.3233/JAD-179912
Caspi, A., Sugden, K., Moffitt, T. E., Taylor, A., Craig, I. W., Harrington, H., et al. (2003). Influence of life stress on depression: moderation by a polymorphism in the 5-HTT gene. Science 301, 386–389. doi: 10.1126/science.1083968
Cedeno, D. (2010). Synthesis of UQ10analogs, Measurement of Their Midpoint Potentials and Their Effects on the Activity of WT and T61V bc1 Complexes From Rhodobacter sphaeroides (Dissertation). Department of Chemistry and Biochemistry, The University of Arizona, Tuscon, AZ, United States.
Cenci, M. A. (2007). Dopamine dysregulation of movement control in L-DOPA-induced dyskinesia. Trends Neurosci. 30, 236–243. doi: 10.1016/j.tins.2007.03.005
Chan, A., and Shea, T. B. (2006). Supplementation with apple juice attenuates presenilin-1 overexpression during dietary and genetically-induced oxidative stress. J. Alzheimers Dis. 10, 353–358. doi: 10.3233/JAD-2006-10401
Chan, D. C. (2012). Fusion and fission: interlinked processes critical for mitochondrial health. Annu. Rev. Genet. 46, 265–287. doi: 10.1146/annurev-genet-110410-132529
Chan, D. C., Pang, J., and Watts, G. F. (2015). Pathogenesis and management of the diabetogenic effect of statins: a role for adiponectin and coenzyme Q 10? Curr. Atheroscler. Rep. 17, 1–10. doi: 10.1007/s11883-014-0472-7
Chariot, P., Brugières, P., Eliezer-Vanerot, M. C., Gény, C., Binaghi, M., and Césaro, P. (1999). Choreic movements and MRI abnormalities in the subthalamic nuclei reversible after administration of coenzyme Q10 and multiple vitamins in a patient with bilateral optic neuropathy. Mov. Disord. 14, 855–9. doi: 10.1002/1531-8257(199909)14:5<855::AID-MDS1023>3.0.CO;2-9
Chen, Q. S., Kagan, B. L., Hirakura, Y., and Xie, C. W. (2000). Impairment of hippocampal long-term potentiation by Alzheimer amyloid β-peptides. J. Neurosci. Res. 60, 65–72. doi: 10.1002/(SICI)1097-4547(20000401)60:1<65::AID-JNR7>3.0.CO;2-Q
Chen, R.-S., Chin-Chang, H., and Chu, N.-S. (1997). Coenzyme Q10 treatment in mitochondrial encephalomyopathies. Eur. Neurol. 37, 212. doi: 10.1159/000117445
Chen, S.-W., Liu, C.-C., and Yen, H.-C. (2013). Detection of suppressed maturation of the human COQ5 protein in the mitochondria following mitochondrial uncoupling by an antibody recognizing both precursor and mature forms of COQ5. Mitochondrion 13, 143–152. doi: 10.1016/j.mito.2013.01.007
Chen, Y., Zhou, K., Wang, R., Liu, Y., Kwak, Y.-D., Ma, T., et al. (2009). Antidiabetic drug metformin (GlucophageR) increases biogenesis of Alzheimer's amyloid peptides via up-regulating BACE1 transcription. Proc. Nat. Acad. Sci. U. S. A. 106, 3907–3912. doi: 10.1073/pnas.0807991106
Cherubini, A., Polidori, M. C., Bregnocchi, M., Pezzuto, S., Cecchetti, R., Ingegni, T., et al. (2000). Antioxidant profile and early outcome in stroke patients. Stroke 31, 2295–2300. doi: 10.1161/01.STR.31.10.2295
Choonara, Y. E., Pillay, V., Du Toit, L. C., Modi, G., Naidoo, D., Ndesendo, V. M., et al. (2009). Trends in the molecular pathogenesis and clinical therapeutics of common neurodegenerative disorders. Int. J. Mol. Sci. 10, 2510–2557. doi: 10.3390/ijms10062510
Chuang, Y. C., Chen, S. D., Liou, C. W., Lin, T. K., Chang, W. N., Chan, S. H., et al. (2009). Contribution of nitric oxide, superoxide anion, and peroxynitrite to activation of mitochondrial apoptotic signaling in hippocampal CA3 subfield following experimental temporal lobe status epilepticus. Epilepsia 50, 731–746. doi: 10.1111/j.1528-1167.2008.01778.x
Cleren, C., Yang, L., Lorenzo, B., Calingasan, N. Y., Schomer, A., Sireci, A., et al. (2008). Therapeutic effects of coenzyme Q10 (CoQ10) and reduced CoQ10 in the MPTP model of Parkinsonism. J. Neurochem. 104, 1613–1621. doi: 10.1111/j.1471-4159.2007.05097.x
Colnat-Coulbois, S., Gauchard, G., Maillard, L., Barroche, G., Vespignani, H., Auque, J., et al. (2005). Bilateral subthalamic nucleus stimulation improves balance control in Parkinson's disease. J. Neurol. Neurosurg. Psychiatry 76, 780–787. doi: 10.1136/jnnp.2004.047829
Corti, O., Hampe, C., Darios, F., Ibanez, P., Ruberg, M., Brice, A., et al. (2005). Parkinson's disease: from causes to mechanisms. C. R. Biol. 328, 131–142. doi: 10.1016/j.crvi.2004.10.009
Cui, S., Luo, K., Quan, Y., Lim, S. W., Shin, Y. J., Lee, K. E., et al. (2021). Water-soluble coenzyme Q10 provides better protection than lipid-soluble coenzyme Q10 in a rat model of chronic tacrolimus nephropathy. Korean J. Intern. Med. 36, 949. doi: 10.3904/kjim.2020.211
da Silva Fernandes, K. S., Brum, D. G., Palei, A. C., Sandrim, V. C., Guerreiro, C. T., Tanus-Santos, J. E., et al. (2012). Functional MMP-9 polymorphisms modulate plasma MMP-9 levels in multiple sclerosis patients. J. Neuroimmunol. 249, 56–59. doi: 10.1016/j.jneuroim.2012.04.001
Dantzer, R., O'Connor, J. C., Freund, G. G., Johnson, R. W., and Kelley, K. W. (2008). From inflammation to sickness and depression: when the immune system subjugates the brain. Nat. Rev. Neurosci. 9, 46. doi: 10.1038/nrn2297
Demirkaya, S., Topcuoglu, M., Aydin, A., Ulas, U., Isimer, A., Vural, O., et al. (2001). Malondialdehyde, glutathione peroxidase and superoxide dismutase in peripheral blood erythrocytes of patients with acute cerebral ischemia. Eur. J. Neurol. 8, 43–51. doi: 10.1046/j.1468-1331.2001.00166.x
Dexter, D., Sian, J., Rose, S., Hindmarsh, J., Mann, V., Cooper, J., et al. (1994). Indices of oxidative stress and mitochondrial function in individuals with incidental Lewy body disease. Ann. Neurol. 35, 38–44. doi: 10.1002/ana.410350107
Di Monte, D. A. (2003). The Environment and Parkinson's disease: is the nigrostriatal system preferentially targeted by neurotoxins? Lancet Neurol. 2, 531–538. doi: 10.1016/S1474-4422(03)00501-5
Duff, K., Eckman, C., Zehr, C., Yu, X., Prada, C.-M., Perez-Tur, J., et al. (1996). Increased amyloid-β42 (43) in brains of mice expressing mutant presenilin 1. Nature 383, 710. doi: 10.1038/383710a0
Dumont, M., Kipiani, K., Yu, F., Wille, E., Katz, M., Calingasan, N. Y., et al. (2011). Coenzyme Q10 decreases amyloid pathology and improves behavior in a transgenic mouse model of Alzheimer's disease. J. Alzheimers Dis. 27, 211–223. doi: 10.3233/JAD-2011-110209
Emmanuele, V., López, L. C., Berardo, A., Naini, A., Tadesse, S., Wen, B., et al. (2012). Heterogeneity of coenzyme Q10 deficiency: patient study and literature review. Arch. Neurol. 69, 978–983. doi: 10.1001/archneurol.2012.206
Engelsen, J., Nielsen, J. D., and Hansen, K. (2003). Effect of coenzyme Q10 and ginkgo biloba on warfarin dosage in patients on long-term warfarin treatment. a randomized, double-blind, placebo-controlled cross-over trial. Ugeskr. Laeg. 165, 1868–1871. doi: 10.1055/s-0037-1613135
Erol, B., Bozlu, M., Hanci, V., Tokgoz, H., Bektas, S., Mungan, G., et al. (2010). Coenzyme Q10 treatment reduces lipid peroxidation, inducible and endothelial nitric oxide synthases, and germ cell–specific apoptosis in a rat model of testicular ischemia/reperfusion injury. Fertil. Steril. 93, 280–282. doi: 10.1016/j.fertnstert.2009.07.981
Fernandez-Abascal, J., Chiaino, E., Frosini, M., Davey, G. P., and Valoti, M. (2020). β-Naphthoflavone and ethanol reverse mitochondrial dysfunction in a parkinsonian model of neurodegeneration. Int. J. Mol. Sci. 21, 3955. doi: 10.3390/ijms21113955
Fernández-del-Río, L., and Clarke, C. F. (2021). Coenzyme Q biosynthesis: An update on the origins of the benzenoid ring and discovery of new ring precursors. Metabolites 11, 385. doi: 10.3390/metabo11060385
Ferrante, K., Shefner, J., Zhang, H., Betensky, R., O'brien, M., Yu, H., et al. (2005). Tolerance of high-dose (3,000 mg/day) coenzyme Q10 in ALS. Neurology 65, 1834–1836. doi: 10.1212/01.wnl.0000187070.35365.d7
Flint Beal, M., and Shults, C. W. (2003). Effects of coenzyme Q10 in Huntington's disease and early Parkinson's disease. Biofactors 18, 153–161. doi: 10.1002/biof.5520180218
Forester, B. P., Harper, D. G., Georgakas, J., Ravichandran, C., Madurai, N., Cohen, B. M., et al. (2015). Antidepressant effects of open label treatment with coenzyme Q10 in geriatric bipolar depression. J. Clin. Psychopharmacol. 35, 338. doi: 10.1097/JCP.0000000000000326
Forester, B. P., Zuo, C. S., Ravichandran, C., Harper, D. G., Du, F., Kim, S., et al. (2012). Coenzyme Q10 effects on creatine kinase activity and mood in geriatric bipolar depression. J. Geriatr. Psychiatry Neurol. 25, 43–50. doi: 10.1177/0891988712436688
Fouad, A. A., and Jresat, I. (2012). Hepatoprotective effect of coenzyme Q10 in rats with acetaminophen toxicity. Environ. Toxicol. Pharmacol. 33, 158–167. doi: 10.1016/j.etap.2011.12.011
García-Bonilla, L., Campos, M., Giralt, D., Salat, D., Chacón, P., Hernández-Guillamon, M., et al. (2012). Evidence for the efficacy of statins in animal stroke models: a meta-analysis. J Neurochem. 122, 233–243. doi: 10.1111/j.1471-4159.2012.07773.x
Gargano, J. W., Wehner, S., and Reeves, M. J. (2011). Presenting symptoms and onset-to-arrival time in patients with acute stroke and transient ischemic attack. J. Stroke Cerebrovasc. Dis. 20, 494–502. doi: 10.1016/j.jstrokecerebrovasdis.2010.02.022
Gazdík, F., Piják, M., Borová, A., and Gazdíková, K. (2003). Biological properties of coenzyme Q10 and its effects on immunity. Cas. Lek. Cesk. 142, 390–393.
Geromel, V., Rötig, A., Munnich, A., and Rustin, P. (2002). Coenzyme Q 10 depletion is comparatively less detrimental to human cultured skin fibroblasts than respiratory chain complex deficiencies. Free Radic. Res. 36, 375–379. doi: 10.1080/10715760290021216
Gille, L., Rosenau, T., Kozlov, A., and Gregor, W. (2008). Ubiquinone and tocopherol: dissimilar siblings. Biochem. Pharmacol. 76, 289–302. doi: 10.1016/j.bcp.2008.04.003
Golde, T. E., Eckman, C. B., and Younkin, S. G. (2000). Biochemical detection of Aβ isoforms: implications for pathogenesis, diagnosis, and treatment of Alzheimer's disease. Biochim. Biophys. Acta Mol. Basis Dis. 1502, 172–187. doi: 10.1016/S0925-4439(00)00043-0
Groneberg, D. A., Kindermann, B., Althammer, M., Klapper, M., Vormann, J., Littarru, G. P., et al. (2005). Coenzyme Q10 affects expression of genes involved in cell signalling, metabolism and transport in human CaCo-2 cells. Int. J. Biochem. Cell Biol. 37, 1208–1218. doi: 10.1016/j.biocel.2004.11.017
Guaras, A., Perales-Clemente, E., Calvo, E., Acín-Pérez, R., Loureiro-Lopez, M., Pujol, C., et al. (2016). The CoQH2/CoQ ratio serves as a sensor of respiratory chain efficiency. Cell Rep. 15, 197–209. doi: 10.1016/j.celrep.2016.03.009
Gunes, H., Ozdemir, E., and Arslan, G. (2019). Coenzyme Q10 increases absence seizures in WAG/Rij rats: the role of the nitric oxide pathway. Epilepsy Res. 154, 69–73. doi: 10.1016/j.eplepsyres.2019.05.002
Guo, J., Wang, W., and Gong, H. (2011). Effects of milk and coenzyme Q10 on the interference of acrylonitrile on vascular endothelial functions. Zhonghua Yi Xue Za Zhi 91, 1136–1138.
Gupta, S., Sodhi, S., and Mahajan, V. (2009). Correlation of antioxidants with lipid peroxidation and lipid profile in patients suffering from coronary artery disease. Exp. Opin. Ther. Targets 13, 889–894. doi: 10.1517/14728220903099668
Gutzmann, H., and Hadler, D. (1998). Sustained Efficacy and Safety of Idebenone in the Treatment of Alzheimer's Disease: Update on a 2-year Double-Blind Multicentre Study. J. Neural Transm. Suppl. (Vienna: Springer), 54, 301–310. doi: 10.1007/978-3-7091-7508-8_30
Gvozdjáková, A., Kucharská, J., Sumbalová, Z., Cornélissen, G., Mojto, V., Rausová, Z., et al. (2022). “Coenzyme Q10 in the Pathogenesis and Prevention of Metabolic and Mitochondrial Non-communicable Diseases,” in Functional Foods and Nutraceuticals in Metabolic and Non-Communicable Diseases (Elsevier), 727–740. doi: 10.1016/B978-0-12-819815-5.00049-5
Harding, A., Sweeney, M., Govan, G., and Riordan-Eva, P. (1995). Pedigree analysis in Leber hereditary optic neuropathy families with a pathogenic mtDNA mutation. Am. J. Hum. Genet. 57, 77.
Hargreaves, I. P. (2021). Coenzyme Q10 in mitochondrial and lysosomal disorders. MDPI. 10, 1970. doi: 10.3390/jcm10091970
Hathcock, J. N., and Shao, A. (2006). Risk assessment for coenzyme Q10 (Ubiquinone). Regul. Toxicol. Pharmacol. 45, 282–288. doi: 10.1016/j.yrtph.2006.05.006
Hauser, W. A., Annegers, J. F., and Kurland, L. T. (1991). Prevalence of epilepsy in Rochester, Minnesota: 1940–1980. Epilepsia 32, 429–445. doi: 10.1111/j.1528-1157.1991.tb04675.x
Hidalgo-Gutiérrez, A., González-García, P., Díaz-Casado, M. E., Barriocanal-Casado, E., López-Herrador, S., Quinzii, C. M., et al. (2021). Metabolic targets of coenzyme Q10 in mitochondria. Antioxidants 10, 520. doi: 10.3390/antiox10040520
Huang, L.-K., Chao, S.-P., and Hu, C.-J. (2020). Clinical trials of new drugs for Alzheimer disease. J. Biomed. Sci. 27, 1–13. doi: 10.1186/s12929-019-0609-7
Ibrahim Fouad, G. (2020). Combination of omega 3 and coenzyme Q10 exerts neuroprotective potential against hypercholesterolemia-induced Alzheimer's-Like disease in rats. Neurochem. Res. 45, 1142–1155. doi: 10.1007/s11064-020-02996-2
Ishrat, T., Khan, M. B., Hoda, M. N., Yousuf, S., Ahmad, M., Ansari, M. A., et al. (2006). Coenzyme Q10 modulates cognitive impairment against intracerebroventricular injection of streptozotocin in rats. Behav. Brain Res. 171, 9–16. doi: 10.1016/j.bbr.2006.03.009
Jiang, T., Sun, Q., and Chen, S. (2016). Oxidative stress: a major pathogenesis and potential therapeutic target of antioxidative agents in Parkinson's disease and Alzheimer's disease. Prog. Neurobiol. 147, 1–19. doi: 10.1016/j.pneurobio.2016.07.005
Jiménez-Jiménez, F. J., Alonso-Navarro, H., García-Martín, E., and Agúndez, J. A. (2022). Coenzyme Q10 and Parkinsonian syndromes: a systematic review. J. Pers. Med. 12, 975. doi: 10.3390/jpm12060975
Jiménez-Jiménez, F. J., Alonso-Navarro, H., García-Martín, E., and Agúndez, J. A. (2023). Coenzyme Q10 and dementia: a systematic review. Antioxidants 12, 533. doi: 10.3390/antiox12020533
Jing, L., He, M.-T., Chang, Y., Mehta, S. L., He, Q.-P., Zhang, J.-Z., et al. (2015). Coenzyme Q10 protects astrocytes from ROS-induced damage through inhibition of mitochondria-mediated cell death pathway. Int. J. Biol. Sci. 11, 59. doi: 10.7150/ijbs.10174
Jokeit, H., and Schacher, M. (2004). Neuropsychological aspects of type of epilepsy and etiological factors in adults. Epilepsy Behav. 5, 14–20. doi: 10.1016/j.yebeh.2003.11.003
Judd, L. L., Akiskal, H. S., Schettler, P. J., Coryell, W., Endicott, J., Maser, J. D., et al. (2003). A prospective investigation of the natural history of the long-term weekly symptomatic status of bipolar II disorder. Arch. Gen. Psychiatry 60, 261–269. doi: 10.1001/archpsyc.60.3.261
Judd, L. L., Akiskal, H. S., Schettler, P. J., Endicott, J., Maser, J., Solomon, D. A., et al. (2002). The long-term natural history of the weekly symptomatic status of bipolar I disorder. Arch. Gen. Psychiatry 59, 530–537. doi: 10.1001/archpsyc.59.6.530
Kadian, M., Sharma, G., Pandita, S., Sharma, K., Shrivasatava, K., Saini, N., et al. (2022). The impact of coenzyme Q10 on neurodegeneration: a comprehensive review. Curr. Pharmacol. Rep. 8, 1–19. doi: 10.1007/s40495-021-00273-6
Kalenikova, E., Gorodetskaya, E., Obolenskaya, O., Shapoval, N., Makarov, V., Medvedev, O., et al. (2018). Tissue-level dynamics and redox state of Coenzyme Q10 in rats after intravenous injection of Ubiquinol. Pharmaceut. Chem. J. 52, 690–693. doi: 10.1007/s11094-018-1882-6
Kalin, N. H. (1996). Management of the depressive component of bipolar disorder. Depress. Anxiety 4, 190–198. doi: 10.1002/(SICI)1520-6394(1996)4:4<190::AID-DA5>3.0.CO;2-J
Kalman, B., Laitinen, K., and Komoly, S. (2007). The involvement of mitochondria in the pathogenesis of multiple sclerosis. J. Neuroimmunol. 188, 1–12. doi: 10.1016/j.jneuroim.2007.03.020
Kemmerer, Z. A., Robinson, K. P., Schmitz, J. M., Manicki, M., Paulson, B. R., Jochem, A., et al. (2021). UbiB proteins regulate cellular CoQ distribution in Saccharomyces cerevisiae. Nat. Commun. 12, 4769. doi: 10.1038/s41467-021-25084-7
Kernan, W. N., Ovbiagele, B., Black, H. R., Bravata, D. M., Chimowitz, M. I., Ezekowitz, M. D., et al. (2014). Guidelines for the prevention of stroke in patients with stroke and transient ischemic attack: a guideline for healthcare professionals from the American Heart Association/American Stroke Association. Stroke 45, 2160–2236. doi: 10.1161/STR.0000000000000024
Khalilian, B., Madadi, S., Fattahi, N., and Abouhamzeh, B. (2021). Coenzyme Q10 enhances remyelination and regulate inflammation effects of cuprizone in corpus callosum of chronic model of multiple sclerosis. J. Mol. Histol. 52, 125–134. doi: 10.1007/s10735-020-09929-x
Kidd, P. (2003). Th1/Th2 balance: the hypothesis, its limitations, and implications for health and disease. Altern. Med. Rev. 8, 223–246.
Koller, W. C., and Cersosimo, M. G. (2004). Neuroprotection in Parkinson's disease: an elusive goal. Curr. Neurol. Neurosci. Rep. 4, 277–283. doi: 10.1007/s11910-004-0052-2
Komaki, H., Faraji, N., Komaki, A., Shahidi, S., Etaee, F., Raoufi, S., et al. (2019). Investigation of protective effects of coenzyme Q10 on impaired synaptic plasticity in a male rat model of Alzheimer's disease. Brain Res. Bull. 147, 14–21. doi: 10.1016/j.brainresbull.2019.01.025
Kones, R. (2010). Mitochondrial therapy for Parkinson's disease: neuroprotective pharmaconutrition may be disease-modifying. Clin. Pharmacol. Adv. Appli. 2, 185. doi: 10.2147/CPAA.S12082
Konradi, C., Eaton, M., MacDonald, M. L., Walsh, J., Benes, F. M., Heckers, S., et al. (2004). Molecular evidence for mitochondrial dysfunction in bipolar disorder. Arch. Gen. Psychiatry 61, 300–308. doi: 10.1001/archpsyc.61.3.300
Kowalska, A. (2004). The beta-amyloid cascade hypothesis: a sequence of events leading to neurodegeneration in Alzheimer's disease. Neurol. Neurochir. Pol. 38, 405–411.
Kumar, N., Yadav, M., Kumar, A., Kadian, M., and Kumar, S. (2022). Neuroprotective effect of hesperidin and its combination with coenzyme Q10 on an animal model of ketamine-induced psychosis: behavioral changes, mitochondrial dysfunctions, and oxidative stress. Fut. J. Pharm. Sci. 8, 1–15. doi: 10.1186/s43094-022-00402-y
Kuo, H.-C., Huang, C.-C., Chu, C.-C., Kao, L.-Y., Lee, H.-C., Pang, C.-Y., et al. (2001). Coenzyme Q10 treatment in Leber's hereditary optic neuropathy. Neuroophthalmology 25, 199–207. doi: 10.1076/noph.25.4.199.8059
Kwon, K.-Y., Park, S., Kim, R. O., Lee, E. J., and Lee, M. (2022). Associations of cognitive dysfunction with motor and non-motor symptoms in patients with de novo Parkinson's disease. Sci. Rep. 12, 11461. doi: 10.1038/s41598-022-15630-8
Lagier-Tourenne, C., Tazir, M., López, L. C., Quinzii, C. M., Assoum, M., Drouot, N., et al. (2008). ADCK3, an ancestral kinase, is mutated in a form of recessive ataxia associated with coenzyme Q10 deficiency. Am. J. Hum. Genet. 82, 661–672. doi: 10.1016/j.ajhg.2007.12.024
Laredj, L. N., Licitra, F., and Puccio, H. M. (2014). The molecular genetics of coenzyme Q biosynthesis in health and disease. Biochimie. 100, 78–87. doi: 10.1016/j.biochi.2013.12.006
Larochelle, C., Alvarez, J. I., and Prat, A. (2011). How do immune cells overcome the blood–brain barrier in multiple sclerosis? FEBS Lett. 585, 3770–3780. doi: 10.1016/j.febslet.2011.04.066
Lee, B.-J., Huang, Y.-C., Chen, S.-J., and Lin, P.-T. (2012a). Effects of coenzyme Q10 supplementation on inflammatory markers (high-sensitivity C-reactive protein, interleukin-6, and homocysteine) in patients with coronary artery disease. Nutrition 28, 767–772. doi: 10.1016/j.nut.2011.11.008
Lee, B.-J., Lin, Y.-C., Huang, Y.-C., Ko, Y.-W., Hsia, S., Lin, P.-T., et al. (2012b). The relationship between coenzyme Q10, oxidative stress, and antioxidant enzymes activities and coronary artery disease. Scie. World J. doi: 10.1100/2012/792756
Lee, D.-H., Gold, R., and Linker, R. A. (2012). Mechanisms of oxidative damage in multiple sclerosis and neurodegenerative diseases: therapeutic modulation via fumaric acid esters. Int. J. Mol. Sci. 13, 11783–11803. doi: 10.3390/ijms130911783
Leonard, B., and Maes, M. (2012). Mechanistic explanations how cell-mediated immune activation, inflammation and oxidative and nitrosative stress pathways and their sequels and concomitants play a role in the pathophysiology of unipolar depression. Neurosci. Biobehav. Rev. 36, 764–785. doi: 10.1016/j.neubiorev.2011.12.005
Lessell, S., Gise, R. L., and Krohel, G. B. (1983). Bilateral optic neuropathy with remission in young men: variation on a theme by Leber? Arch. Neurol. 40, 2–6. doi: 10.1001/archneur.1983.04050010022005
Lesser, G. J., Case, D., Stark, N., Williford, S., Giguere, J., Garino, L. A., et al. (2013). A randomized double-blind, placebo-controlled study of oral coenzyme Q10 to relieve self-reported treatment related fatigue in newly diagnosed patients with breast cancer. J. Support. Oncol. 11, 31. doi: 10.1016/j.suponc.2012.03.003
Li, H., Chen, G., Ma, W., and Li, P.-A. A. (2014). Water-soluble coenzyme q10 inhibits nuclear translocation of apoptosis inducing factor and cell death caused by mitochondrial complex I inhibition. Int. J. Mol. Sci. 15, 13388–13400. doi: 10.3390/ijms150813388
Li, H.-N., Zimmerman, M., Milledge, G. Z., Hou, X.-L., Cheng, J., Wang, Z.-H., et al. (2017). Water-soluble coenzyme Q10 reduces rotenone-induced mitochondrial fission. Neurochem. Res. 42, 1096–1103. doi: 10.1007/s11064-016-2143-2
Littarru, G. P., and Tiano, L. (2010). Clinical aspects of coenzyme Q10: an update. Nutrition 26, 250–254. doi: 10.1016/j.nut.2009.08.008
Lodish, H., Berk, A., Kaiser, C. A., Kaiser, C., Krieger, M., Scott, M. P., et al. (2008). “Integrating cells into tissues,” in Molecular Cell Biology, 5th Edn (W. H. Freeman & Company).
Longa, E. Z., Weinstein, P. R., Carlson, S., and Cummins, R. (1989). Reversible middle cerebral artery occlusion without craniectomy in rats. Strok. 20, 84–91. doi: 10.1161/01.STR.20.1.84
Löscher, W. (2002). Animal models of epilepsy for the development of antiepileptogenic and disease-modifying drugs. A comparison of the pharmacology of kindling and post-status epilepticus models of temporal lobe epilepsy. Epilepsy Res. 50, 105–123. doi: 10.1016/S0920-1211(02)00073-6
Löscher, W., and Schmidt, D. (2006). New horizons in the development of antiepileptic drugs: Innovative strategies. Epilepsy Res. 69, 183–272. doi: 10.1016/j.eplepsyres.2006.03.014
Losón, O. C., Song, Z., Chen, H., and Chan, D. C. (2013). Fis1, Mff, MiD49, and MiD51 mediate Drp1 recruitment in mitochondrial fission. Mol. Biol. Cell. 24, 659–667. doi: 10.1091/mbc.e12-10-0721
Lucca, G., Comim, C. M., Valvassori, S. S., Réus, G. Z., Vuolo, F., Petronilho, F., et al. (2009a). Increased oxidative stress in submitochondrial particles into the brain of rats submitted to the chronic mild stress paradigm. J. Psychiatr. Res. 43, 864–869. doi: 10.1016/j.jpsychires.2008.11.002
Lucca, G., Comim, C. M., Valvassori, S. S., Réus, G. Z., Vuolo, F., Petronilho, F., et al. (2009b). Effects of chronic mild stress on the oxidative parameters in the rat brain. Neurochem. Int. 54, 358–362. doi: 10.1016/j.neuint.2009.01.001
Lundblad, M., Andersson, M., Winkler, C., Kirik, D., Wierup, N., Cenci, M. A., et al. (2002). Pharmacological validation of behavioural measures of akinesia and dyskinesia in a rat model of Parkinson's disease. Eur. J. Neurosci. 15, 120–132. doi: 10.1046/j.0953-816x.2001.01843.x
Mabuchi, H., Nohara, A., Kobayashi, J., Kawashiri, M.-A., Katsuda, S., Inazu, A., et al. (2007). Effects of CoQ10 supplementation on plasma lipoprotein lipid, CoQ10 and liver and muscle enzyme levels in hypercholesterolemic patients treated with atorvastatin: a randomized double-blind study. Atherosclerosis 195, e182–e9. doi: 10.1016/j.atherosclerosis.2007.06.010
Maes, M., Galecki, P., Chang, Y. S., and Berk, M. A. (2011). review on the oxidative and nitrosative stress (O&NS) pathways in major depression and their possible contribution to the (neuro) degenerative processes in that illness. Progr. Neuropsychopharmacol. Biol. Psychiatry 35, 676–692. doi: 10.1016/j.pnpbp.2010.05.004
Maes, M., Mihaylova, I., Kubera, M., Uytterhoeven, M., Vrydags, N., Bosmans, E., et al. (2009). Lower plasma Coenzyme Q 10 in depression: a marker for treatment resistance and chronic fatigue in depression and a risk factor to cardiovascular disorder in that illness. Neuroendocrinol. Lett. 30, 462–469.
Mancuso, M., Orsucci, D., Volpi, L., Calsolaro, V., and Siciliano, G. (2010). Coenzyme Q10 in neuromuscular and neurodegenerative disorders. Curr. Drug Targets 11, 111–121. doi: 10.2174/138945010790031018
Manzar, H., Abdulhussein, D., Yap, T. E., and Cordeiro, M. F. (2020). Cellular consequences of coenzyme Q10 deficiency in neurodegeneration of the retina and brain. Int. J. Mol. Sci. 21, 9299. doi: 10.3390/ijms21239299
Markesbery, W. R. (1997). Oxidative stress hypothesis in Alzheimer's disease. Free Radic. Biol. Med. 23, 134–147. doi: 10.1016/S0891-5849(96)00629-6
Matthews, R. T., Yang, L., Browne, S., Baik, M., and Beal, M. F. (1998). Coenzyme Q10 administration increases brain mitochondrial concentrations and exerts neuroprotective effects. Proc. Nat. Acad. Sci. U. S. A. 95, 8892–8897. doi: 10.1073/pnas.95.15.8892
McCarthy, S., Somayajulu, M., Sikorska, M., Borowy-Borowski, H., and Pandey, S. (2004). Paraquat induces oxidative stress and neuronal cell death; neuroprotection by water-soluble Coenzyme Q10. Toxicol. Appl. Pharmacol. 201, 21–31. doi: 10.1016/j.taap.2004.04.019
Mcdonald, S. R., Sohal, R. S., and Forster, M. J. (2005). Concurrent administration of coenzyme Q10 and α-tocopherol improves learning in aged mice. Free Radic. Biol. Med. 38, 729–736. doi: 10.1016/j.freeradbiomed.2004.11.014
McGarry, A., McDermott, M., Kieburtz, K., de Blieck, E. A., Beal, F., Marder, K., et al. (2017). A randomized, double-blind, placebo-controlled trial of coenzyme Q10 in Huntington disease. Neurology 88, 152–159. doi: 10.1212/WNL.0000000000003478
Mehrpooya, M., Yasrebifar, F., Haghighi, M., Mohammadi, Y., and Jahangard, L. (2018). Evaluating the effect of coenzyme Q10 augmentation on treatment of bipolar depression: a double-blind controlled clinical trial. J. Clin. Psychopharmacol. 38, 460–466. doi: 10.1097/JCP.0000000000000938
Mignot, C., Apartis, E., Durr, A., Marques Lourenço, C., Charles, P., Devos, D., et al. (2013). Phenotypic variability in ARCA2 and identification of a core ataxic phenotype with slow progression. Orphanet. J. Rare Dis. 8, 1–10. doi: 10.1186/1750-1172-8-173
Milanlioglu, A., Aslan, M., Ozkol, H., Çilingir, V., Aydin, M. N., and Karadas, S. (2016). Serum antioxidant enzymes activities and oxidative stress levels in patients with acute ischemic stroke: influence on neurological status and outcome. Wien. Klin. Wochenschr. 128, 169–174. doi: 10.1007/s00508-015-0742-6
Miller, A., Shapiro, S., Gershtein, R., Kinarty, A., Rawashdeh, H., Honigman, S., et al. (1998). Treatment of multiple sclerosis with copolymer-1 (Copaxone®): implicating mechanisms of Th1 to Th2/Th3 immune-deviation. J. Neuroimmunol. 92, 113–121. doi: 10.1016/S0165-5728(98)00191-X
Mohamed, H. A., and Said, R. S. (2021). Coenzyme Q10 attenuates inflammation and fibrosis implicated in radiation enteropathy through suppression of NF-kB/TGF-β/MMP-9 pathways. Int. Immunopharmacol. 92, 107347. doi: 10.1016/j.intimp.2020.107347
Mollet, J., Delahodde, A., Serre, V., Chretien, D., Schlemmer, D., Lombes, A., et al. (2008). CABC1 gene mutations cause ubiquinone deficiency with cerebellar ataxia and seizures. Am. J. Hum. Genet. 82, 623–630. doi: 10.1016/j.ajhg.2007.12.022
Moreira, P. I., Zhu, X., Wang, X., Lee, H.-,g, Nunomura, A., Petersen, R. B., et al. (2010). Mitochondria: a therapeutic target in neurodegeneration. Biochim. Biophys. Acta Mol. Basis Dis. 1802, 212–220. doi: 10.1016/j.bbadis.2009.10.007
Morris, G., Anderson, G., Berk, M., and Maes, M. (2013). Coenzyme Q10 depletion in medical and neuropsychiatric disorders: potential repercussions and therapeutic implications. Mol. Neurobiol. 48, 883–903. doi: 10.1007/s12035-013-8477-8
Mugoni, V., Postel, R., Catanzaro, V., De Luca, E., Turco, E., Digilio, G., et al. (2013). Ubiad1 is an antioxidant enzyme that regulates eNOS activity by CoQ10 synthesis. Cell 152, 504–518. doi: 10.1016/j.cell.2013.01.013
Muthukumaran, K., Kanwar, A., Vegh, C., Marginean, A., Elliott, A., Guilbeault, N., et al. (2018). Ubisol-Q 10 (a nanomicellar water-soluble formulation of CoQ 10) treatment inhibits Alzheimer-type behavioral and pathological symptoms in a double transgenic mouse (TgAPEswe, PSEN1dE9) model of Alzheimer's disease. J. Alzheimers Dis. 61, 221–236. doi: 10.3233/JAD-170275
Muthukumaran, K., Smith, J., Jasra, H., Sikorska, M., Sandhu, J. K., Cohen, J., et al. (2014). Genetic susceptibility model of parkinson's disease resulting from exposure of DJ-1 deficient mice to MPTP: evaluation of neuroprotection by Ubisol-Q 10. J. Parkinsons Dis. 4, 523–530. doi: 10.3233/JPD-140368
Nakazawa, H., Ikeda, K., Shinozaki, S., Yasuhara, S., Yu, Y. M., Martyn, J. J., et al. (2019). Coenzyme Q10 protects against burn-induced mitochondrial dysfunction and impaired insulin signaling in mouse skeletal muscle. FEBS Open Bio. 9, 348–363. doi: 10.1002/2211-5463.12580
Nasoohi, S., Simani, L., Khodagholi, F., Nikseresht, S., Faizi, M., Naderi, N., et al. (2019). Coenzyme Q10 supplementation improves acute outcomes of stroke in rats pretreated with atorvastatin. Nutr. Neurosci. 22, 264–272. doi: 10.1080/1028415X.2017.1376928
Ní Chróinín, D., Asplund, K., Åsberg, S., Callaly, E., Cuadrado-Godia, E., Díez-Tejedor, E., et al. (2013). Statin therapy and outcome after ischemic stroke: systematic review and meta-analysis of observational studies and randomized trials. Stroke 44, 448–456. doi: 10.1161/STROKEAHA.112.668277
Neergheen, V., Chalasani, A., Wainwright, L., Yubero, D., Montero, R., Artuch, R., et al. (2019). Coenzyme Q 10 in the treatment of mitochondrial disease. J. Inborn Errors Metab. Screen. 5, 1–8. doi: 10.1177/2326409817707771
Nidhi, G., Balakrishnan, S., and Pandhi, P. (1999). Role of nitric oxide in electroshock and pentylenetetrazole. Methods Find. Exp. Clin. Pharmacol. 21, 609–612. doi: 10.1358/mf.1999.21.9.795611
Nikoskelainen, E. (1984). New aspects of the genetic, etiologic, and clinical puzzle of Leber's disease. Neurology 34, 1482. doi: 10.1212/WNL.34.11.1482
Noh, Y., Kim, K., Shim, M., Choi, S., Choi, S., Ellisman, M., et al. (2013). Inhibition of oxidative stress by coenzyme Q10 increases mitochondrial mass and improves bioenergetic function in optic nerve head astrocytes. Cell Death Dis. 4, e820. doi: 10.1038/cddis.2013.341
Novotny, E. J., Singh, G., Wallace, D. C., Dorfman, L. J., Louis, A., Sogg, R. L., et al. (1986). Leber's disease and dystonia: a mitochondrial disease. Neurology 36, 1053. doi: 10.1212/WNL.36.8.1053
Nutt, J. G. (1990). Levodopa-induced dyskinesia: review, observations, and speculations. Neurology 40, 340. doi: 10.1212/WNL.40.2.340
Obolenskaia, O. N., Gorodetskaya, E. A., Kalenikova, E. I., Belousova, M. A., Gulyaev, M. V., Makarov, V. G., et al. (2020). Intravenous administration of coenzyme Q10 in acute period of cerebral ischemia decreases mortality by reducing brain necrosis and limiting its increase within 4 days in rat stroke model. Antioxidants 9, 1240. doi: 10.3390/antiox9121240
Ogasahara, S., Nishikawa, Y., Yorifuji, S., Soga, F., Nakamura, Y., Takahashi, M., et al. (1986). Treatment of Kearns-Sayre syndrome with coenzyme Q10. Neurology 36, 45. doi: 10.1212/WNL.36.1.45
Onaolapo, O. J., Odeniyi, A. O., Jonathan, S. O., Samuel, M. O., Amadiegwu, D., Olawale, A., et al. (2021). An investigation of the anti-Parkinsonism potential of co-enzyme Q10 and co-enzyme Q10/levodopa-carbidopa combination in mice. Curr. Aging Sci. 14, 62–75. doi: 10.2174/1874609812666191023153724
Ord, E. N., Shirley, R., McClure, J. D., McCabe, C., Kremer, E. J., Macrae, I. M., et al. (2013). Combined antiapoptotic and antioxidant approach to acute neuroprotection for stroke in hypertensive rats. J. Cerebr. Blood Flow Metab. 33, 1215–1224. doi: 10.1038/jcbfm.2013.70
Osonoe, K., Mori, N., Suzuki, K., and Osonoe, M. (1994). Antiepileptic effects of inhibitors of nitric oxide synthase examined in pentylenetetrazol-induced seizures in rats. Brain Res. 663, 338–340. doi: 10.1016/0006-8993(94)91283-1
Oxenkrug, G. F. (2010). Metabolic syndrome, age-associated neuroendocrine disorders, and dysregulation of tryptophan—kynurenine metabolism. Ann. N. Y. Acad. Sci. 1199, 1–14. doi: 10.1111/j.1749-6632.2009.05356.x
Oztay, F., Ergin, B., Ustunova, S., Balci, H., Kapucu, A., Caner, M., et al. (2007). Effects of coenzyme Q10 on the heart ultrastructure and nitric oxide synthase during hyperthyroidism. Chin. J. Physiol. 50, 217.
Papucci, L., Schiavone, N., Witort, E., Donnini, M., Lapucci, A., Tempestini, A., et al. (2003). Coenzyme q10 prevents apoptosis by inhibiting mitochondrial depolarization independently of its free radical scavenging property. J. Biol. Chem. 278, 28220–28228. doi: 10.1074/jbc.M302297200
Park, H. W., Park, C. G., Park, M., Lee, S. H., Park, H. R., Lim, J., et al. (2020). Intrastriatal administration of coenzyme Q10 enhances neuroprotection in a Parkinson's disease rat model. Sci. Rep. 10, 9572. doi: 10.1038/s41598-020-66493-w
Parmar, S. S., Jaiwal, A., Dhankher, O. P., and Jaiwal, P. K. (2015). Coenzyme Q10 production in plants: current status and future prospects. Crit. Rev. Biotechnol. 35, 152–164. doi: 10.3109/07388551.2013.823594
Patel, M. N. (2002). Oxidative stress, mitochondrial dysfunction, and epilepsy. Free Radic. Res. 36, 1139–1146. doi: 10.1080/1071576021000016391
Pathak, J., Rajneesh, Ahmed, H., Singh, D. K., Singh, P. R., Kumar, D., et al. (2019). “Oxidative stress and antioxidant defense in plants exposed to ultraviolet radiation,” in Reactive Oxygen, Nitrogen and Sulfur Species in Plants: Production, Metabolism, Signaling and Defense Mechanisms, eds M. Hasanuzzaman, V. Fotopoulos, K. Nahar, and M. Fujita (Hoboken, NJ: Wiley Blackwell), 371–420.
Peng, M., Falk, M. J., Haase, V. H., King, R., Polyak, E., Selak, M., et al. (2008). Primary coenzyme Q deficiency in Pdss2 mutant mice causes isolated renal disease. PLoS Genet. 4, e1000061. doi: 10.1371/journal.pgen.1000061
Persson, M. F., Franzén, S., Catrina, S-B., Dallner, G., Hansell, P., et al. (2012). Coenzyme Q10 prevents GDP-sensitive mitochondrial uncoupling, glomerular hyperfiltration and proteinuria in kidneys from db/db mice as a model of type 2 diabetes. Diabetologia 55, 1535–1543. doi: 10.1007/s00125-012-2469-5
Picone, P., Ditta, L. A., Sabatino, M. A., Militello, V., San Biagio, P. L., Di Giacinto, M. L., et al. (2016a). Ionizing radiation-engineered nanogels as insulin nanocarriers for the development of a new strategy for the treatment of Alzheimer's disease. Biomaterials 80, 179–194. doi: 10.1016/j.biomaterials.2015.11.057
Picone, P., Vilasi, S., Librizzi, F., Contardi, M., Nuzzo, D., Caruana, L., et al. (2016b). Biological and biophysics aspects of metformin-induced effects: cortex mitochondrial dysfunction and promotion of toxic amyloid pre-fibrillar aggregates. Aging 8, 1718. doi: 10.18632/aging.101004
Pierrel, F., Burgardt, A., Lee, J.-H., Pelosi, L., and Wendisch, V. F. (2022). Recent advances in the metabolic pathways and microbial production of coenzyme Q. World J. Microbiol. Biotechnol. 38, 58. doi: 10.1007/s11274-022-03242-3
Potgieter, M., Pretorius, E., and Pepper, M. S. (2013). Primary and secondary coenzyme Q10 deficiency: the role of therapeutic supplementation. Nutr. Rev. 71, 180–188. doi: 10.1111/nure.12011
Povarova, O., Balatsky, A., Gusakov, V., and Medvedev, O. (2018). Effect of coenzyme Q10 on expression of UbiAd1 gene in rat model of local cerebral ischemia. Bull. Exp. Biol. Med. 165, 69–71. doi: 10.1007/s10517-018-4101-2
Pradhan, N., Singh, C., and Singh, A. (2021). Coenzyme Q10 a mitochondrial restorer for various brain disorders. Naunyn-Schmiedebergs Arch. Pharmacol. 394, 1–26. doi: 10.1007/s00210-021-02161-8
Prajapati, S. K., Garabadu, D., and Krishnamurthy, S. (2017). Coenzyme Q10 prevents mitochondrial dysfunction and facilitates pharmacological activity of atorvastatin in 6-OHDA induced dopaminergic toxicity in rats. Neurotox. Res. 31, 478–492. doi: 10.1007/s12640-016-9693-6
Puomila, A., Hämäläinen, P., Kivioja, S., Savontaus, M.-L., Koivumäki, S., Huoponen, K., et al. (2007). Epidemiology and penetrance of Leber hereditary optic neuropathy in Finland. Eur. J. Hum. Genet. 15, 1079–1089. doi: 10.1038/sj.ejhg.5201828
Quinzii, C. M., Emmanuele, V., and Hirano, M. (2014). Clinical presentations of coenzyme q10 deficiency syndrome. Mol. Syndromol. 5, 141–146. doi: 10.1159/000360490
Quinzii, C. M., and Hirano, M. (2011). Primary and secondary CoQ10 deficiencies in humans. Biofactors 37, 361–365. doi: 10.1002/biof.155
Ramezani, M., Komaki, A., Hashemi-Firouzi, N., Mortezaee, K., Faraji, N., Golipoor, Z., et al. (2020a). Therapeutic effects of melatonin-treated bone marrow mesenchymal stem cells (BMSC) in a rat Model of Alzheimer's Disease. J. Chem. Neuroanat. 108, 101804. doi: 10.1016/j.jchemneu.2020.101804
Ramezani, M., Sahraei, Z., Simani, L., Heydari, K., and Shahidi, F. (2020b). Coenzyme Q10 supplementation in acute ischemic stroke: Is it beneficial in short-term administration? Nutr. Neurosci. 23, 640–645. doi: 10.1080/1028415X.2018.1541269
Rashid, M. H., Babu, D., and Siraki, A. G. (2021). Interactions of the antioxidant enzymes NAD (P) H: quinone oxidoreductase 1 (NQO1) and NRH: quinone oxidoreductase 2 (NQO2) with pharmacological agents, endogenous biochemicals and environmental contaminants. Chem. Biol. Interact. 345, 109574. doi: 10.1016/j.cbi.2021.109574
Riccio, P. (2011). The molecular basis of nutritional intervention in multiple sclerosis: a narrative review. Comp. Ther. Med. 19, 228–237. doi: 10.1016/j.ctim.2011.06.006
Riordan-Eva, P., Sanders, M., Govan, G., Sweeney, M., Costa, J. D., Harding, A., et al. (1995). The clinical features of Leber's hereditary optic neuropathy defined by the presence of a pathogenic mitochondrial DNA mutation. Brain 118, 319–337. doi: 10.1093/brain/118.2.319
Rodrigo, R., Fernández-Gajardo, R., Gutiérrez, R., Manuel Matamala, J., Carrasco, R., Miranda-Merchak, A., et al. (2013). Oxidative stress and pathophysiology of ischemic stroke: novel therapeutic opportunities. CNS Neurol. Disord. 12, 698–714. doi: 10.2174/1871527311312050015
Rundek, T., Naini, A., Sacco, R., Coates, K., and DiMauro, S. (2004). Atorvastatin decreases the coenzyme Q10 level in the blood of patients at risk for cardiovascular disease and stroke. Arch. Neurol. 61, 889–892. doi: 10.1001/archneur.61.6.889
Safarinejad, M. R., Safarinejad, S., Shafiei, N., and Safarinejad, S. (2012). Effects of the reduced form of coenzyme Q10 (ubiquinol) on semen parameters in men with idiopathic infertility: a double-blind, placebo controlled, randomized study. J. Urol. 188, 526–531. doi: 10.1016/j.juro.2012.03.131
Salehpour, F., Farajdokht, F., Cassano, P., Sadigh-Eteghad, S., Erfani, M., Hamblin, M. R., et al. (2019). Near-infrared photobiomodulation combined with coenzyme Q10 for depression in a mouse model of restraint stress: reduction in oxidative stress, neuroinflammation, and apoptosis. Brain Res. Bull. 144, 213–222. doi: 10.1016/j.brainresbull.2018.10.010
Sanoobar, M., Dehghan, P., Khalili, M., Azimi, A., and Seifar, F. (2016). Coenzyme Q10 as a treatment for fatigue and depression in multiple sclerosis patients: a double blind randomized clinical trial. Nutr. Neurosci. 19, 138–143. doi: 10.1179/1476830515Y.0000000002
Sanoobar, M., Eghtesadi, S., Azimi, A., Khalili, M., Jazayeri, S., Reza Gohari, M., et al. (2013). Coenzyme Q10 supplementation reduces oxidative stress and increases antioxidant enzyme activity in patients with relapsing–remitting multiple sclerosis. Int. J. Neurosci. 123, 776–782. doi: 10.3109/00207454.2013.801844
Santos, J., Soares, P., and Fontoura, L. M. (2004). “Análise de conteúdo: a pesquisa qualitativa no âmbito da geografia agrária,” in Anais do Encontro Estadual de Geografia (Santa Cruz do Sul), 24.
Sattarinezhad, E., Shafaroodi, H., Sheikhnouri, K., Mousavi, Z., and Moezi, L. (2014). The effects of coenzyme Q10 on seizures in mice: the involvement of nitric oxide. Epilepsy Behav. 37, 36–42. doi: 10.1016/j.yebeh.2014.05.024
Schellenberg, G. D., D'Souza, I., and Poorkaj, P. (2000). The genetics of Alzheimer's disease. Curr. Psychiatry Rep. 2, 158–164. doi: 10.1007/s11920-000-0061-z
Schmelzer, C., Lindner, I., Rimbach, G., Niklowitz, P., Menke, T., Döring, F., et al. (2008). Functions of coenzyme Q10 in inflammation and gene expression. Biofactors 32, 179–183. doi: 10.1002/biof.5520320121
Schmelzer, C., Lorenz, G., Rimbach, G., and Döring, F. (2009). In vitro effects of the reduced form of coenzyme Q10 on secretion levels of TNF-α and chemokines in response to LPS in the human monocytic cell line THP-1. J. Clin. Biochem. Nutr. 44, 62–66. doi: 10.3164/jcbn.08-182
Scott, R. A., Lhatoo, S. D., and Sander, J. W. (2001). The treatment of epilepsy in developing countries: where do we go from here? Bull. World Health Organ. 79, 344–351.
Shalansky, S., Lynd, L., Richardson, K., Ingaszewski, A., and Kerr, C. (2007). Risk of warfarin-related bleeding events and supratherapeutic international normalized ratios associated with complementary and alternative medicine: a longitudinal analysis. Pharmacotherapy 27, 1237–1247. doi: 10.1592/phco.27.9.1237
Shalata, A., Edery, M., Habib, C., Genizi, J., Mahroum, M., Khalaily, L., et al. (2019). Primary coenzyme Q deficiency due to novel ADCK3 variants, studies in fibroblasts and review of literature. Neurochem. Res. 44, 2372–2384. doi: 10.1007/s11064-019-02786-5
Sharaf, M. S. (2017). Modulation of mitochondrial bioenergetics in rainbow trout (Oncorhynchus mykiss) by zinc and calcium (Ph. D. Thesis). Department of Biomedical Sciences, Faculty of Veterinary Medicine, University of Prince Edward Island, Charlottetown, United States.
Sharma, A. K., Reams, R. Y., Jordan, W. H., Miller, M. A., Thacker, H. L., Snyder, P. W., et al. (2007). Mesial temporal lobe epilepsy: pathogenesis, induced rodent models and lesions. Toxicol. Pathol. 35, 984–999. doi: 10.1080/01926230701748305
Shearer, M. J., and Newman, P. (2014). Recent trends in the metabolism and cell biology of vitamin K with special reference to vitamin K cycling and MK-4 biosynthesis. J. Lipid Res. 55, 345–362. doi: 10.1194/jlr.R045559
Shekarian, M., Komaki, A., Shahidi, S., Sarihi, A., Salehi, I., Raoufi, S., et al. (2020). The protective and therapeutic effects of vinpocetine, a PDE1 inhibitor, on oxidative stress and learning and memory impairment induced by an intracerebroventricular (ICV) injection of amyloid beta (aβ) peptide. Behav. Brain Res. 383, 112512. doi: 10.1016/j.bbr.2020.112512
Shoffner, J. M., Brown, M. D., Stugard, C., June, A. S., Pollock, S., Haas, R. H., et al. (1995). Leber's hereditary optic neuropathy plus dystonia is caused by a mitochondrial DNA point mutation. Ann. Neurol. 38, 163–169. doi: 10.1002/ana.410380207
Shults, C. W., Oakes, D., Kieburtz, K., Beal, M. F., Haas, R., Plumb, S., et al. (2002). Effects of coenzyme Q10 in early Parkinson disease: evidence of slowing of the functional decline. Arch. Neurol. 59, 1541–1550. doi: 10.1001/archneur.59.10.1541
Simani, L., Ryan, F., Hashemifard, S., Hooshmandi, E., Madahi, M., Sahraei, Z., et al. (2018). Serum Coenzyme Q10 is associated with clinical neurological outcomes in acute stroke patients. J. Mol. Neurosci. 66, 53–58. doi: 10.1007/s12031-018-1115-1
Slowik, E. (2019). Synthesis, endogenous detection, and mitochondrial function of the hydroxy substituted Coenzyme Q 10 derivative HO-CoQ10 (Dissertation). Universität des Saarlandes, Saarbrucke, Germany
Sobreira, C., Hirano, M., Shanske, S., Keller, R., Haller, R., Davidson, E., et al. (1997). Mitochondrial encephalomyopathy with coenzyme Q10 deficiency. Neurology 48, 1238–1243. doi: 10.1212/WNL.48.5.1238
Soleimani, M., Jameie, S. B., Barati, M., Mehdizadeh, M., and Kerdari, M. (2014). Effects of coenzyme Q10 on the ratio of TH1/TH2 in experimental autoimmune encephalomyelitis model of multiple sclerosis in C57BL/6. Iran. Biomed. J. 18, 203. doi: 10.6091/ibj.13362.2014
Somayajulu-Nitu, M., Sandhu, J. K., Cohen, J., Sikorska, M., Sridhar, T., Matei, A., et al. (2009). Paraquat induces oxidative stress, neuronal loss in substantia nigra region and Parkinsonism in adult rats: neuroprotection and amelioration of symptoms by water-soluble formulation of coenzyme Q 10. BMC neurosci. 10, 88. doi: 10.1186/1471-2202-10-88
Spindler, M., Beal, M. F., and Henchcliffe, C. (2009). Coenzyme Q10 effects in neurodegenerative disease. Neuropsychiatr. Dis. Treat. 597–610. doi: 10.2147/NDT.S5212
Spooren, A., Kolmus, K., Laureys, G., Clinckers, R., De Keyser, J., Haegeman, G., et al. (2011). Interleukin-6, a mental cytokine. Brain Res. Rev. 67, 157–183. doi: 10.1016/j.brainresrev.2011.01.002
Srivastava, S. (2017). The mitochondrial basis of aging and age-related disorders. Genes 8, 398. doi: 10.3390/genes8120398
Starr, M. S., and Starr, B. S. (1993). Paradoxical facilitation of pilocarpine-induced seizures in the mouse by MK-801 and the nitric oxide synthesis inhibitor L-NAME. Pharmacol. Biochem. Behav. 45, 321–325. doi: 10.1016/0091-3057(93)90246-P
Steece-Collier, K., Maries, E., and Kordower, J. H. (2002). Etiology of Parkinson's disease: genetics and environment revisited. Proc. Nat. Acad. Sci. U. S. A. 99, 13972–13974. doi: 10.1073/pnas.242594999
Steiner, T. J., Stovner, L. J., Katsarava, Z., Lainez, J. M., Lampl, C., Lantéri-Minet, M., et al. (2014). The impact of headache in Europe: principal results of the Eurolight project. J. Headache Pain 15, 1–11. doi: 10.1186/1129-2377-15-31
Stone, E. M., Newman, N. J., Miller, N. R., Johns, D. R., Lott, M. T., Wallace, D. C., et al. (1992). Visual recovery in patients with Leber's hereditary optic neuropathy and the 11778 mutation. J. Clin. Neuroophthalmol. 12, 10–14.
Stone, J., and Carson, A. (2011). Functional neurologic symptoms: assessment and management. Neurol. Clin. 29, 1–18. doi: 10.1016/j.ncl.2010.10.011
Swerdlow, R. H. (2012). Mitochondria and cell bioenergetics: increasingly recognized components and a possible etiologic cause of Alzheimer's disease. Antioxid. Redox Signal. 16, 1434–1455. doi: 10.1089/ars.2011.4149
Szibor, M., Gainutdinov, T., Fernandez-Vizarra, E., Dufour, E., Gizatullina, Z., Debska-Vielhaber, G., et al. (2020). Bioenergetic consequences from xenotopic expression of a tunicate AOX in mouse mitochondria: Switch from RET and ROS to FET. Biochim. Biophys. Acta Bioenerget. 1861, 148137. doi: 10.1016/j.bbabio.2019.148137
Tanaka, A., Kiyosawa, M., Mashima, Y., and Tokoro, T. A. (1998). family with Leber's hereditary optic neuropathy with mitochondrial DNA heteroplasmy related to disease expression. J. Neuroophthalmol. 18, 81–83. doi: 10.1097/00041327-199806000-00001
Theard, M. A., Baughman, V. L., Wang, Q., Pelligrino, D. A., and Albrecht, R. F. (1995). The role of nitric oxide in modulating brain activity and blood flow during seizure. Neuroreport 6, 921–924. doi: 10.1097/00001756-199504190-00024
Tsai, K.-L., Huang, Y.-H., Kao, C.-L., Yang, D.-M., Lee, H.-C., Chou, H.-Y., et al. (2012). A novel mechanism of coenzyme Q10 protects against human endothelial cells from oxidative stress-induced injury by modulating NO-related pathways. J. Nutr. Biochem. 23, 458–468. doi: 10.1016/j.jnutbio.2011.01.011
Tsuda, M., Suzuki, T., and Misawa, M. (1997). Aggravation of DMCM-induced seizure by nitric oxide synthase inhibitors in mice. Life Sci. 60, L339–PL43. doi: 10.1016/S0024-3205(97)00226-9
Urdinguio, R. G., Sanchez-Mut, J. V., and Esteller, M. (2009). Epigenetic mechanisms in neurological diseases: genes, syndromes, and therapies. Lancet Neurol. 8, 1056–1072. doi: 10.1016/S1474-4422(09)70262-5
Van der Walt, A., Butzkueven, H., Kolbe, S., Marriott, M., Alexandrou, E., Gresle, M., et al. (2010). Neuroprotection in multiple sclerosis: a therapeutic challenge for the next decade. Pharmacol. Ther. 126, 82–93. doi: 10.1016/j.pharmthera.2010.01.006
Van Horssen, J., Witte, M. E., Schreibelt, G., Vries, d. e., and Radical, H. E. (2011). changes in multiple sclerosis pathogenesis. Biochim. Biophys. Acta Mol. Basis Dis. 1812, 141–150. doi: 10.1016/j.bbadis.2010.06.011
Van Leeuwen, R., De Vries, R., and Dzoljic, M. R. (1995). 7-Nitro indazole, an inhibitor of neuronal nitric oxide synthase, attenuates pilocarpine-induced seizures. Eur. J. Pharmacol. 287, 211–213. doi: 10.1016/0014-2999(95)00678-8
Wallace, D. C., Singh, G., Lott, M. T., Hodge, J. A., Schurr, T. G., Lezza, A., et al. (1988). Mitochondrial DNA mutation associated with Leber's hereditary optic neuropathy. Science 242, 1427–1430. doi: 10.1126/science.3201231
Wang, S., Jain, A., Novales, N. A., Nashner, A. N., Tran, F., Clarke, C. F., et al. (2022). Predicting and understanding the pathology of single nucleotide variants in human COQ genes. Antioxidants 11, 2308. doi: 10.3390/antiox11122308
Wang, X., Wang, W., Li, L., Perry, G., Lee, H.-,g., and Zhu, X. (2014). Oxidative stress and mitochondrial dysfunction in Alzheimer's disease. Biochim. Biophys. Acta Mol. Basis Dis. 1842, 1240–1247. doi: 10.1016/j.bbadis.2013.10.015
Wang, Y., and Hekimi, S. (2016). Understanding ubiquinone. Trends Cell Biol. 26, 367–378. doi: 10.1016/j.tcb.2015.12.007
Wear, D., Vegh, C., Sandhu, J. K., Sikorska, M., Cohen, J., Pandey, S., et al. (2021). Ubisol-Q10, a nanomicellar and water-dispersible formulation of coenzyme-Q10 as a potential treatment for Alzheimer's and Parkinson's disease. Antioxidants 10, 764. doi: 10.3390/antiox10050764
Weyer, G., Babej-Dölle, R., Hadler, D., Hofmann, S., and Herrmann, W. A. (1997). controlled study of 2 doses of idebenone in the treatment of Alzheimer's disease. Neuropsychobiology 36, 73–82. doi: 10.1159/000119366
Won, R., Lee, K. H., and Lee, B. H. (2011). Coenzyme Q10 protects neurons against neurotoxicity in hippocampal slice culture. Neuroreport 22, 721–726. doi: 10.1097/WNR.0b013e32834acb8d
World Health Organization (2006). Neurological Disorders: Public Health Challenges. Geneva: World Health Organization.
Xia, W. (2000). Role of presenilin in γ-secretase cleavage of amyloid precursor protein. Exp. Gerontol. 35, 453–460. doi: 10.1016/S0531-5565(00)00111-X
Yalcin, A., Kilinc, E., Kocturk, S., Resmi, H., and Sozmen, E. Y. (2004). Effect of melatonin cotreatment against kainic acid on coenzyme Q10, lipid peroxidation and Trx mRNA in rat hippocampus. Int. J. Neurosci. 114, 1085–97. doi: 10.1080/00207450490475535
Yang, L., Calingasan, N. Y., Wille, E. J., Cormier, K., Smith, K., Ferrante, R. J., et al. (2009). Combination therapy with coenzyme Q10 and creatine produces additive neuroprotective effects in models of Parkinson's and Huntington's diseases. J. Neurochem. 109, 1427–1439. doi: 10.1111/j.1471-4159.2009.06074.x
Yang, X., Dai, G., Li, G., and Yang, E. S. (2010). Coenzyme Q10 reduces β-amyloid plaque in an APP/PS1 transgenic mouse model of Alzheimer's disease. J. Mol. Neurosci. 41, 110–113. doi: 10.1007/s12031-009-9297-1
Yang, X., Yang, Y., Li, G., Wang, J., and Yang, E. S. (2008). Coenzyme Q10 attenuates β-amyloid pathology in the aged transgenic mice with Alzheimer presenilin 1 mutation. J. Mol. Neurosci. 34, 165–171. doi: 10.1007/s12031-007-9033-7
Yen, H.-C., Chen, B.-S., Yang, S.-L., Wu, S.-Y., Chang, C.-W., Wei, K.-C., et al. (2022). Levels of coenzyme Q10 and several COQ proteins in human astrocytoma tissues are inversely correlated with malignancy. Biomolecules 12, 336. doi: 10.3390/biom12020336
Yen, H.-C., Liu, Y.-C., Kan, C.-C., Wei, H.-J., Lee, S.-H., Wei, Y.-H., et al. (2016). Disruption of the human COQ5-containing protein complex is associated with diminished coenzyme Q10 levels under two different conditions of mitochondrial energy deficiency. Biochim. Biophys. Acta Gen. Sub. 1860, 1864–1876. doi: 10.1016/j.bbagen.2016.05.005
Yen, H.-C., Yeh, W.-Y., Lee, S.-H., Feng, Y.-H., and Yang, S.-L. (2020). Characterization of human mitochondrial PDSS and COQ proteins and their roles in maintaining coenzyme Q10 levels and each other's stability. Biochim. Biophys. Acta Bioenerget. 1861, 148192. doi: 10.1016/j.bbabio.2020.148192
Yen, H. C., Chen, F. Y., Chen, S. W., Huang, Y. H., Chen, Y. R., Chen, C. W., et al. (2011). Effect of mitochondrial dysfunction and oxidative stress on endogenous levels of coenzyme Q10 in human cells. J. Biochem. Mol. Toxicol. 25, 280–289. doi: 10.1002/jbt.20387
Zhang, X., Agborbesong, E., and Li, X. (2021). The role of mitochondria in acute kidney injury and chronic kidney disease and its therapeutic potential. Int. J. Mol. Sci. 22, 11253. doi: 10.3390/ijms222011253
Zierz, S., Jahns, G., and Jerusalem, F. (1989). Coenzyme Q in serum and muscle of 5 patients with Kearns-Sayre syndrome and 12 patients with ophthalmoplegia plus. J. Neurol. 236, 97–101. doi: 10.1007/BF00314404
AD, Alzheimer's Diseases; ARCA2, Autosomal Recessive Cerebellar Ataxia 2; ATP, Adenosine triphosphate; Aβ, β-amyloid; AChE, Acetylcholinesterase; ADCK3, AarF-domain-containing kinase 3; BD, Bipolar disorder; BBB, Blood-brain barrie; βNF, β-naphthoflavone; CYP, Cytochrome P450; CoQ10, Coenzyme Q10; CPEO, Ophthalmoplegia; CUMS, Chronic unpredictable mid stress; CPZ, Cuprizone; DA, Dopamine; DMSO, Dimethyl sulfoxide; Drp1, Dynamin-related protein 1; ETC, Electron transport chain; EtOH, Ethanol; EAE, Experimental autoimmune encephalomyelitis; eNOS, Endothelial nitric oxide synthase; FCCP, Carbonyl cyanide p-trifluoro-methoxyphenyl; Fis1, Fission protein 1; FM, Fibromyalgia; FST, Forced swim test; GFAP, Glial fibrillary acidic protein; GPx, Glutathione peroxidase; H2O2, Hydrogen peroxide; HD, Huntington disease; HIP, Hippocampus; HMG-CoA, Hydroxymethylglutaryl coenzyme A; HPLC, high-performance liquid chromatography; IDO-1, Indoleamine 2, 3-dioxygenase 1; IL-1β, Interleukin-1β; (IFN)-γ, Interferon; IMM, Inner mitochondrial membrane; iNOS, Induced nitric oxide synthase; ICV, Intracerebroventricular; KSS, Kearns-Sayre syndrome; LHON, Leber's hereditary optic neuropathy; LPO, Lipid peroxidation; LTP, Long-term potentiation; LPS, Lipopolysaccharide; L-DOPA, Levodopa-3,4-dihydroxyphenylalanine; LBD, Lewy body dementia; MDA, Malondialdehyde; MMPs, Matrix metalloproteinases; MPTP, 1-methyl-4-phenyl-1,2,3,6-tetrahydropyridine; MSA, Multiple system atrophy; MRC, Mitochondrial respiratory chain; MtDNA, Mitochondrial DNA; MS, Multiple sclerosis; NO, Nitric oxide; ONH, Optic nerve head; PD, Parkinson's Diseases; PSP, Progressive supranuclear palsy; PS-1, Presenilin 1; PTZ, Pentylenetetrazole; PDMCI, PD mild cognitive impairment; PQ, Paraquat; PH&M, Physical and mental activities (PH&M); PFC, Prefrontal cortex; QH2, Ubiquinol-10; ROS, Reactive oxygen species; RET, Rearranged during transfection; RRMS, Relapsing-remitting MS; SCAR9, Spinocerebellar ataxia autosomal recessive 9; SE, Status epilepticus; SNpc, Substantia nigra pars compacta; SOD, Superoxide dismutase; STZ, Streptozotocin; SWDs, Spike-wave discharges; TBARS, Thiobarbituric acid reactive substances; TNF-α, Tumor necrosis factor-alpha; TLE, Temporal lobe epilepsy; Th1, T-helper type 1; TIA, Transient ischemic attack; TAC, Total antioxidant capacity; UCPs, Uncoupling proteins; UQ, Oxidized ubiquinone; UbiAd1, UbiA prenyltransferase domain-containing protein 1; UQH2, Reduced ubiquinol; VIN, Vinpocetin; WS-CoQ10, Water-Soluble CoQ10; l-Arg, l-arginine; 7-NI, 7- nitroimidazole.
Keywords: Alzheimer's disease, depression, epilepsy, Parkinson's disease, neurological disorder
Citation: Bagheri S, Haddadi R, Saki S, Kourosh-Arami M, Rashno M, Mojaver A and Komaki A (2023) Neuroprotective effects of coenzyme Q10 on neurological diseases: a review article. Front. Neurosci. 17:1188839. doi: 10.3389/fnins.2023.1188839
Received: 17 March 2023; Accepted: 22 May 2023;
Published: 23 June 2023.
Edited by:
Hisashi Shirakawa, Kyoto University, JapanReviewed by:
Jesús Fernández Abascal, Universidad Pablo de Olavide, SpainCopyright © 2023 Bagheri, Haddadi, Saki, Kourosh-Arami, Rashno, Mojaver and Komaki. This is an open-access article distributed under the terms of the Creative Commons Attribution License (CC BY). The use, distribution or reproduction in other forums is permitted, provided the original author(s) and the copyright owner(s) are credited and that the original publication in this journal is cited, in accordance with accepted academic practice. No use, distribution or reproduction is permitted which does not comply with these terms.
*Correspondence: Alireza Komaki, YWxpcmV6YWtvbWFraUBnbWFpbC5jb20=; S29tYWtpQHVtc2hhLmFjLmly; TmV1cm9waHlzaW9sb2d5QHVtc2hhLmFjLmly
†ORCID: Alireza Komaki orcid.org/0000-0003-3865-9583
Disclaimer: All claims expressed in this article are solely those of the authors and do not necessarily represent those of their affiliated organizations, or those of the publisher, the editors and the reviewers. Any product that may be evaluated in this article or claim that may be made by its manufacturer is not guaranteed or endorsed by the publisher.
Research integrity at Frontiers
Learn more about the work of our research integrity team to safeguard the quality of each article we publish.