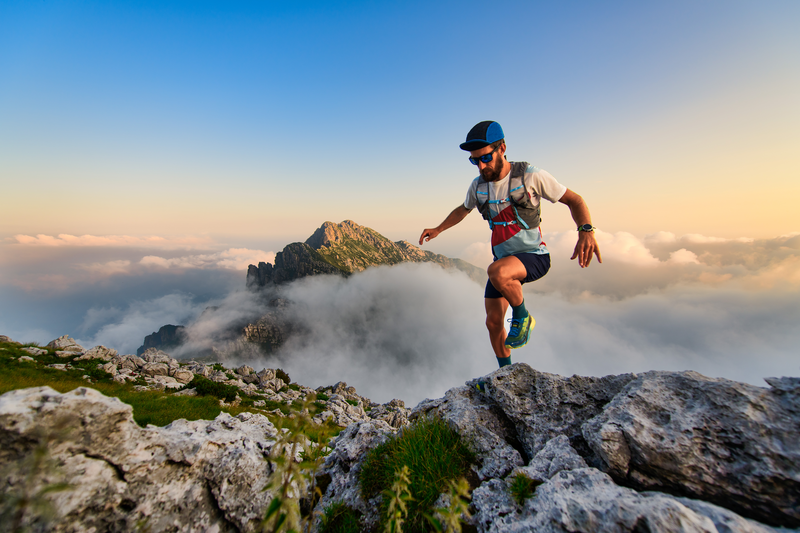
94% of researchers rate our articles as excellent or good
Learn more about the work of our research integrity team to safeguard the quality of each article we publish.
Find out more
BRIEF RESEARCH REPORT article
Front. Neurosci. , 02 June 2023
Sec. Neuroprosthetics
Volume 17 - 2023 | https://doi.org/10.3389/fnins.2023.1158438
This article is part of the Research Topic Women in Neuroengineering and Neurotechnologies View all 9 articles
We developed the TechArm system as a novel technological tool intended for visual rehabilitation settings. The system is designed to provide a quantitative assessment of the stage of development of perceptual and functional skills that are normally vision-dependent, and to be integrated in customized training protocols. Indeed, the system can provide uni- and multisensory stimulation, allowing visually impaired people to train their capability of correctly interpreting non-visual cues from the environment. Importantly, the TechArm is suitable to be used by very young children, when the rehabilitative potential is maximal. In the present work, we validated the TechArm system on a pediatric population of low-vision, blind, and sighted children. In particular, four TechArm units were used to deliver uni- (audio or tactile) or multi-sensory stimulation (audio-tactile) on the participant's arm, and subject was asked to evaluate the number of active units. Results showed no significant difference among groups (normal or impaired vision). Overall, we observed the best performance in tactile condition, while auditory accuracy was around chance level. Also, we found that the audio-tactile condition is better than the audio condition alone, suggesting that multisensory stimulation is beneficial when perceptual accuracy and precision are low. Interestingly, we observed that for low-vision children the accuracy in audio condition improved proportionally to the severity of the visual impairment. Our findings confirmed the TechArm system's effectiveness in assessing perceptual competencies in sighted and visually impaired children, and its potential to be used to develop personalized rehabilitation programs for people with visual and sensory impairments.
Since birth, humans rely on different sensory modalities to perceive the surrounding environment and they need to develop adaptive competencies to correctly interpret sensory information and act appropriately. Vision plays a crucial role in perceiving external information and developing a wide range of functional skills. For example, visual experience shapes the capability of orientation and localization in space and has an impact on the overall perceptual and cognitive development (Thinus-Blanc and Gaunet, 1997; Vasilyeva and Lourenco, 2010). Indeed, Bremner et al. (2011) demonstrated that visual information is predominant over vestibular information to guide orientation in both infants and toddlers. Also, vision guides the development of spatial skills, which are in turn crucial for social competencies (Cappagli and Gori, 2016). The central role of vision during the development is explained by its capability to simultaneously provide information about relationships between objects, individuals, and with the surrounding environment (Tinti et al., 2006; Bremner et al., 2011; Pasqualotto and Proulx, 2012). In support to this view, Cappagli et al. (2017a) found that also the perception of spatial information occurring through auditory and tactile modalities is affected by the presence of simultaneous visual information, suggesting that spatial information tends to be organized according to a visual frame of reference.
Investigating how spatial competencies develop when early visual experience is corrupted might further support this view. Over the last few decades, numerous studies have been conducted on visually impaired (VI) people to test the effect of visual deprivation on the overall development, especially in case of congenital visual impairments (e.g., in case of inherited retinal disorders). The classical assumption is that visual impairments promote a refinement of the residual senses, allowing for instance VI adults to perform equally or even better than sighted people in spatial tasks (compensatory hypothesis, see Collignon et al., 2009; Merabet and Pascual-Leone, 2010). Other evidences suggest that vision cannot be fully replaced by other sensory modalities in spatial tasks, causing for instance delays or deficits in the visually impaired population (general-loss hypothesis, see Eimer, 2004; Pasqualotto and Proulx, 2012). Gori et al. (2014) suggested that hearing and haptic spatial impairments in the blind might be explained in terms of missing cross-sensory calibration during the development, because vision (the optimal sensory modality for spatial tasks) cannot be used to calibrate the other senses during early stages of development. To date, only few studies investigated the relative impact of sensory modalities during early stages of development. For instance, Gori (2015) demonstrated that sighted children and adults rely more on visual than haptic information to solve size discrimination tasks, while younger children rely more on the haptic channel. Berto et al. (2021) recently hypothesized that interactions between audio and visual perception start only at a late stage of development, while in the very early stages of life auditory processing is independent of vision. At the same time, other studies support the hypothesis that early visual experience is essential to guide perception. For instance, Cappagli et al. (2017a) found that congenitally blind children are significantly impaired both in auditory distance discrimination and proprioceptive reproduction tasks. Moreover, extensive clinical research on VI children has shown the detrimental effects of early visual deprivation on navigation and spatial competencies (Giudice, 2018; Bathelt et al., 2019).
Overall, there is evidence that multisensory stimulation may be useful to prevent the negative impact of visual impairment on perceptual development. Indeed, results from experimental and clinical studies suggested that multisensory rehabilitation may provide long-term positive effects on the development of spatial cognition in VI children (Cappagli et al., 2017b, 2019; Cuturi et al., 2017; Morelli et al., 2020). This could be due to the positive effect of sensory redundancy on perception in ecological settings (Gori, 2015). For instance, it has been shown that multisensory stimulation improves the precision of perception and encoding of environmental events, with beneficial effects on the behavioral response to such events (Cao et al., 2019). These findings support the need for a deeper understanding about how the development and functioning of multisensory integration is affected by the absence of vision. Indeed, such a knowledge would provide a neuroscientific basis for the development of science-driven interventions in the context of rehabilitation, informing how to train residual perceptual competencies and elicit accurate responses. In this context, there is a strong need of developing novel technological tools, able to assess and train perceptual skills of VI people. This is especially valuable at an early age, when the brain plasticity is maximal and a proper training facilitates the emergence of adaptive skills. Indeed, the majority of technologies for VI people are developed for assistive purposes (e.g., Chebat et al., 2018), and they usually substitute vision by delivering information through the residual sensory modalities, rather than training such modalities and increase the subject's autonomy in retrieving useful information from the environment. However, recent works introduced the use of technological aids for rehabilitation purposes, and encouraging results were obtained both in adults (Cappagli et al., 2017b) and children (Cappagli et al., 2019).
Within this perspective, we developed the TechArm system, a technological tool designed to provide a quantitative assessment of different perceptual skills (residual vision, hearing, and touch) and to exploit unisensory and multisensory mechanisms in a rehabilitation setting. The system was designed to be easily operated by visually impaired patients from the very first years of life, and to be integrated by therapists and clinicians within their training protocols. The system proved its effectiveness in investigating perception on typical adults (Schiatti et al., 2020; Martolini et al., 2021). Here, the TechArm system was validated for the first time on visually impaired children, during uni- and multisensory perception tasks. Our purpose was to investigate the system's effectiveness in: (i) providing a quantitative measure of perceptive capabilities of visually impaired children (i.e., as an assessment tool); and (ii) providing sensory redundancy, which can potentially be used to train and improve the detection and discrimination of specific stimuli in the absence of vision, therefore yielding a rehabilitative function.
Overall, 8 blind children (B, mean age 10.50 ± 4.21 y.o.), 23 children with low vision (LV, mean age 9.87 ± 3.09 y.o.), and 23 age-matched sighted children (S, mean age 9.83 ± 3.01 y.o.) participated in the study. Sighted children were recruited from primary and secondary schools in Genoa, Italy, while low-vision and blind children were recruited from the Neuro-Ophthalmology Unit at IRCCS Mondino, Pavia, Italy. The visual functions were assessed using a multiple optotype based on the age and level of instruction (Lea Vision tests or Snellen optotype, Hyvärinen et al., 1980). As inclusion criteria for VI children, in accordance with the International Statistical Classification of Diseases and Related Health Problems (World Health Organization, 2009) and the Italian Law 134/2001 defining visual impairments, we considered: (a) visual acuity between 1/10 and 3/10 (evaluated at 40 cm distance) for LV group, and residual vision from light/sporadic light to no light perception for B group (see Supplementary Table 1 for VI participants' clinical details); (b) a typical level of cognitive development (cutoff = 70), as assessed with the verbal scale of the Wechsler Intelligence Scale for Children (Grizzle, 2011); (c) visual deficit related to a peripheral (and not central) impairment (i.e., involving pre-geniculate structures). We did not included participants with: (a) a low-limit intellectual functioning (i.e., intellectual or development quotient < 70); (b) a diagnosis of auditory or neuromotor disorder besides the visual deficit.
The local ethical committees (Comitato Etico della Regione Liguria and Comitato Etico Policlinico San Matteo Referente Area Pavia, for sighted and visually impaired groups, respectively) approved the study, and the participants' parents or their legal representatives were asked to sign written informed consent forms compliant to the Declaration of Helsinki, before participating in the experiment.
The experimental setup consisted of the TechArm System (Figure 1), developed by the Electronic Design Laboratory and the Unit for Visually Impaired People at the Istituto Italiano di Tecnologia (Genoa, Italy) in collaboration with the Developmental Neuro-ophthalmology Unit (Pavia, Italy). It is a wearable, wireless system, composed by single cubic units (dimensions of a single unit: 2.5 × 2.5 × 2.5cm) able to provide spatially- and temporally-coherent multisensory stimulation, and to collect a real-time feedback from the user. In particular, each unit includes embedded actuators to enable visual (RGB light-emitting diode), auditory (digital amplifier and speaker), and tactile (haptic moto-driver) stimuli, and a capacitive sensor placed on the top surface of each unit to collect and record touch inputs from the user (dimension of the upper sensitized area: 6.25 cm2). An extensive technical description of the system is provided in Schiatti et al. (2020).
Figure 1. Experimental setup: four TechArm's units were placed on the subject's forearm and used to provide uni- and multi-sensory stimulation, i.e., audio, tactile, and audio-tactile stimuli, thanks to the embedded speaker and haptic motor. Visual stimulation (LED) was not used for this experiment. A fifth unit was used to stop the trial and collect the subject's response, by tapping on the touch sensor on the top of the unit.
The experimental protocol was inspired by the one presented by Martolini et al. (2021). Four TechArm units were placed on the participant's left/right arm (right-handed/left-handed), with a 2 × 2 configuration, and used to deliver a uni- or multi-sensory stimulation to the subject during each trial. The dimension of the stimulation area was in the range of 6.25 cm2 (single unit) to 25 cm2 (four units). A fifth unit was placed on the table, next to the subject's right/left index finger, and it was used to collect the subject's response (Figure 1). Three stimuli conditions were implemented: audio (A), tactile (T), and audio-tactile (AT). Auditory stimuli were provided as a 79 dB white noise burst at 300 Hz, while tactile stimuli were conveyed by a vibro-motor (vibration frequency: 10 Hz). All stimuli lasted 100 ms, and 1 to 4 units were activated simultaneously during each trial, leading to 15 possible stimuli spatial configurations (4 for 1 active unit, 6 for 2 active units, 4 for 3 active unites, and 1 for 4 active units), and 45 trials in total (15 configurations × 3 sensory modalities), presented in randomized order. During each trial, the subject was instructed to tap the unit placed on the table as soon as possible after receiving the stimulation, regardless of the kind of stimulation conveyed, in order to stop the trial. Subsequently, the subject was asked to verbally report how many devices were perceived to be active (1, 2, 3, or 4). Five practice trials were allowed to make sure that subjects fully understood the task. The experiment lasted about half an hour, and short breaks were allowed at any time during the session.
We compared the performance of the three groups, by selecting a subset of 8 subjects from LV and S matching the average age of B (aged 10.63 ± 4.37 y.o. and 10.50 ± 4.21, respectively). Performance was assessed in terms of classification accuracy (ratio of correct answers over the total number of trials), considering as classes the number of active devices (1 to 4) for each sensory modality (A, T, and AT). Accuracy was computed from trials for each subject in each stimuli condition. Considering the fact that the classes are unbalanced, we also investigated the effect of two types of errors: (i) the detection of a certain class when the actual number of activated units belongs to one of the other classes (false positives, FPs), and (ii) the missed detection of a certain class when the actual number of active units belongs to it (false negatives, FNs). Specifically, we computed Precision (ratio of correctly classified trials, i.e., true positives, TP, over the sum of TP and FP), and Sensitivity (ratio of TP, over the sum of TP and FN) for each class and subject. The overall Precision and Sensitivity for each subject were computed by performing a weighted average of classes values, according to the total number of trials within each class. After verifying that the data did not follow a normal distribution (Shapiro-Wilk test), we performed a statistical analysis using non-parametric statistics. We ran three separate two-way permuted ANOVAs with Accuracy, Precision and Sensitivity as dependent variables respectively. We considered the group as between-factor (three levels: Blind—B, Low Vision—LV, and Sighted—S), and stimulation condition as within-factor (three levels: Auditory—A, Tactile—T, Audio-Tactile—AT). We performed post-hoc tests to assess the significant within- and between-group's differences. For both levels of analysis, the permuted Bonferroni correction for non-parametric data was applied in case of significant effects to adjust the p-value for multiple comparisons (significant value: α = 0.05). Considering the whole sample of LV, for each sensory condition, we computed the correlation (Pearson coefficient) among the individual performance (accuracy) and the degree of visual impairment, expressed in terms of Logarithm of the Minimum Angle of Resolution (LogMAR) at 40 cm (see Moussa et al., 2021 for the conversion between the near visual acuity expressed in decimal system and the logMAR chart).
Considering the index of correct responses among the total of trials, results show that for all groups the best accuracy is achieved when using the T modality, followed by the AT (Figure 2A). While for T and AT, Sighted perform better than Low Vision and Blind children, the accuracy for A condition is around chance level for all groups. From the two-way ANOVA analysis considering Accuracy as dependent variable, the group (B, LV, S) and the stimuli condition (A, T and AT) as between- and within- factors respectively, we found a main effect only for the stimuli condition (Residual Sum of Squares RSS = 0.193, iter = 5, 000, p = 0.002). Subsequent post-hoc tests were therefore performed on data from different conditions, regardless of the group (as shown on the box plot in Figure 3A). They revealed no significant difference between T-AT, while accuracy in A condition is always worse than both in T (p < 0.01**) and AT (p < 0.05*) conditions. Figures 2B, C show results in terms of Precision and Sensitivity for each group and condition. Also the effect of false negative errors (correct number of active units not detected) is stronger for A than T and AT conditions, as evidenced by the lower Sensitivity values on Figure 2C. Interestingly, while both sighted and low vision children tend to be more sensitive than precise for A stimulation, Blind children show a similar level of Precision and Sensitivity when using the auditory modality. Again, from the ANOVA analysis we found a significant main effect of the stimuli condition for both Precision (RSS = 0.451, iter = 5, 000, p = 0.013) and Sensitivity (RSS = 0.194, iter = 5, 000, p = 0.012), and a null group's effect. By performing post-hoc tests on data from all groups, we found that both Precision and Sensitivity are significantly lower in A compared to T (p < 0.01**) and AT (p < 0.05*), as shown in Figures 3B, C.
Figure 2. Bar plots of performance metrics (Mean ± Standard Error) for each group (Blind, Low Vision and Sighted), and different stimulation conditions (A, T, and AT, respectively, in blue, red and yellow color). From top to the bottom: (A) Classification Accuracy, with chance level = 0.25 indicated by a dotted line, (B) Precision and (C) Sensitivity.
Figure 3. Box plots of performance metrics regardless of the group, with scatter plots of individual data, in different stimulation conditions (A, T, and AT, respectively, in blue, red and yellow color). From top to the bottom: (A) Classification Accuracy, with chance level = 0.25 indicated by a dotted line, (B) Precision and (C) Sensitivity. Horizontal bars on the top of the boxes report the statistical significance according to the non-parametric post-hoc tests (p < 0.01** and p < 0.05*).
Figure 4 shows the results of the correlation analysis performed on the full-size (n = 23) group of LV children. Specifically, the Pearson correlation coefficient was computed considering the distributions of Accuracy and the degree of visual impairment (LogMAR at 40 cm). Results show no significant correlation between the performance and the severity of the visual impairment for tactile and audio-tactile conditions, while a significant correlation (p = 0.002) was found for audio condition (Figure 4A). Children with a lower visual acuity (higher LogMAR values) show a higher capability of detecting differences in audio signals (higher Accuracy) than children retaining a higher level of residual vision (lower LogMAR values). Qualitatively, individual values on Figures 4B, C suggest that the performance in AT increases compared to A for LogMAR lower than 1, while it decreases for higher LogMAR values.
Figure 4. Correlation plots for LV group. Blue dots represent the scatter plot of individual performance (Accuracy) compared to residual vision (logMAR value for near vision, i.e., 40 cm). A higher LogMAR value corresponds to a lower visual acuity. Least-squared fit lines are shown in red. From top to the bottom, correlation plots refer to subjects' performance during: (A) Audio, (B) Tactile and (C) Audio-Tactile stimulation condition. Pearson correlation coefficient (ρ) and associated p-value are indicated within each plot. Bold text indicates significant correlation (p < 0.05).
It is well-known that congenital or early acquired visual impairments might interfere with developmental processes (Dale and Sonksen, 2002). Therefore, there is the need to design and clinically validate rehabilitation devices for VI children both to assess and train perceptual skills toward the interpretation of non-visual cues. The present study aimed at assessing the effectiveness of a novel technological tool (TechArm system) in a pediatric population of VI children.
First, our results suggest that the effect of sensory redundancy is the same across different visual conditions (visual impairment vs. normal vision). This result is in line with literature about multisensory development, e.g., audio-visual integration (Neil et al., 2006; Gori et al., 2014). Indeed, audio and visual information are typically integrated in the brain when originating from the same location, and this typically improves localization capabilities. However, such cross-modal interaction is not balanced among sensory modalities. Indeed, vision is predominant and it can bias auditory information (to an extent depending on conditions, such as the distance between the two different stimuli, see Körding et al., 2007 and Cao et al., 2019). Barutchu et al. (2010) observed that facilitation of motor responses to multimodal (audio-visual) stimuli is visible from the age of 6–7 years. At the same time, such response is inconsistent in older children, who show greater variability in responses compared to adults (Barutchu et al., 2010). It has been hypothesized that audio-visual integration may be task-dependent, therefore requiring the integrity and maturation of superior cognitive competencies, such as attention. For instance, a recent study suggested that multisensory gain in simple detection tasks may be related to a child's cognitive level (Barutchu et al., 2010; Denervaud et al., 2020). Also, it has been shown that audio-visual integration emerges later for spatial localization on a vertical plane compared to the horizontal plane, demonstrating visual dominance over audio for vertical multisensory perception (Gori et al., 2021). The majority of studies about perceptual abilities on multisensory integration involve vision, variously combined with hearing or touch, due to the vision's unique characteristic of conveying simultaneously a big amount of information about surrounding objects and about objects-events relationships. By contrast, sensory redundancy for non-visual stimuli (e.g., audio-tactile) has been less investigated, especially in children. Some researchers have hypothesized that the effectiveness of audio-tactile interactions in improving perceptual skills may depend on the spatial coincidence of the stimuli. Moreover, the impact of audio-tactile interactions seems to be affected by the type of the experimental task, e.g., detection vs. discrimination (Guest et al., 2002; Murray et al., 2005; for a review, see Kitagawa and Spence, 2006).
The present study demonstrated a predominance of touch over both only-auditory and multimodal audio-tactile stimuli during a perceptual task. The lower precision in A condition compared to T and AT suggests that subjects wrongly predict the number of active devices, by committing a false positive error, more frequently in A than in T and AT condition (Figure 2B). Such an effect of the sensory modality is significant regardless of the visual condition (i.e., it is evident both in VI and sighted children). In accordance with previous works, this suggests that the multisensory gain may be not only age-dependent but also task-dependent. Indeed, touch is the first sense to mature in typically developing infants (Smith, 1972) and it has a task-specific prevalence over the other sensory modalities. For example, touch is the most effective sense for size discrimination tasks in younger children, while vision provides more accurate responses in orientation tasks (Gori et al., 2008; for a review see Burr and Gori, 2012). Studies on VI people provided conflicting results about the performance of VI individuals (e.g., better performance in haptic recognition tasks, worse performance during tasks involving the recognition of spatial attributes of objects such as orientation, see Morrongiello et al., 1994; Alary et al., 2009). Our study suggests that in some cases multisensory integration may develop later and follow a different trajectory, both for VI and sighted children. In the absence of vision, touch proves to be the most effective sense in size discrimination tasks, enhancing perceptual precision over auditory-only, as evident from the better performance obtained for tactile stimuli than auditory stimuli. The dominance of touch over audition has been previously reported both for infants and adults in tasks of information processing in the peri-personal space, in connection with head and hand position (Sanabria et al., 2005; Thomas et al., 2018; Martolini et al., 2021). Furthermore, previous studies have shown that in audio-tactile interaction tasks, tactile stimuli are more important than auditory ones at the level of information processing (Hötting and Röder, 2004; Soto-Faraco et al., 2004). From these findings, we might speculate that hearing could be trained or calibrated by the haptic sense for this specific kind of task, in the lack of vision.
To assess whether different types of stimulation can affect performance in the group of LV children, we analyzed the correlation between the severity of visual impairment and performance during different stimuli conditions. Interestingly, we found an inverse correlation between the accuracy of auditory processing and the visual acuity, resulting in a better performance for children with severe visual impairments. The impact of visual deprivation on the development of other sensory modalities, such as hearing, has been previously demonstrated. Nevertheless, most of the existing studies were performed on blind people (see Gori et al., 2014 on a spatial localization task), and little is known about the extent to which the severity of the visual impairment may affect the development of hearing. In a recent study, Kolarik et al. (2020) showed that the auditory interpretation of distance and room size is related to the severity of a visual impairment, e.g., the higher the severity the larger both perceived distances and size of a room. Moreover, Senna et al. (2022) found that children with treated congenital cataract perform better than children with untreated cataract in an auditory bisection task, but worse compared to sighted controls, with a significant correlation between the performance and the post-surgical visual acuity. Such results suggest that an early sensory deprivation may alter the intact sensory modalities as well. Both behavioral, neuro-physiological and neuroimaging methods were used to investigate the differences in spatial hearing skills on adults with congenital, early-onset and late-onset visual impairments (Voss et al., 2004; Collignon et al., 2011, 2013; Tao et al., 2015). Results showed that in late-onset VI people the auditory spatial competencies seem to be affected by the duration of blindness, and the behavioral and cortical responses approach those of congenitally or early-blind people as blindness progresses over time. This highlights the important role of visual calibration during childhood, which decreases with prolonged blindness (Amadeo et al., 2019; Gori et al., 2020). To explain the better performance of blind children compared to low vision in audio modality, we hypothesize that children with mild visual impairments are more likely to rely on their residual vision in everyday life and during rehabilitation activities (Morelli et al., 2020), and therefore they perform similarly to sighted children. At the same time, they might not effectively use hearing when audio is the only modality provided. Anyhow, further data are needed to draw stronger conclusions. Indeed, the LV group we considered mainly presents mild low vision (see Figure 4). We could find a significant difference between LV and S by narrowing the inclusion criteria for the LV group, or by considering LV sub-groups with different degrees of impairment. Blind or severely impaired children, on the other hand, perform better on A condition because they likely rely on hearing as their most trained sense to compensate the lack of vision. Individual values on the plots also suggest that the performance in AT increases compared to A for LogMAR lower than 1, while it decreases for higher LogMAR values (see Figures 4B, C). Such observation discloses the TechArm system's effectiveness in shedding light on individual differences, and its potential as a tool to assess how perceptive mechanisms develop, while taking into account the subject-specific condition as well as the degree of visual impairment, and therefore allowing to plan customized rehabilitation protocols.
Here, we validated for the first time the TechArm system on a clinical population of blind and low-vision children, comparing results with a control group of sighted children. Our findings revealed the dominance of touch over hearing in sensory discrimination within the peripersonal space and a positive effect of sensory redundancy for stimuli localization. Furthermore, the inverse correlation between residual vision and auditory accuracy highlighted the importance of developing personalized training interventions. Indeed, a subject-specific assessment and rehabilitation program is essential to identify the most impaired functional competencies, such as orientation and mobility, and effectively improve the person's autonomy during everyday-life activities. Our system proved to be effective at different levels, e.g., by allowing to compare perception-related skills using different sensory modalities and to disentangle the contribution of each sensory channel during a multisensory perceptual task. Beyond the protocol implemented for this study, the system can facilitate the design of different task conditions aimed at investigating perceptual capabilities in uni- and multi-sensory tasks, by varying both the overall stimulation area and the number of simultaneously activated sensory modalities. For instance, the system would allow to perform both reaching-to-the-body and reaching-to-the-sound tasks in which children are respectively required to localize sensory stimulation on their own body (e.g., to increase body awareness) and localize stimulation in the near and far space (e.g., to increase mobility and orientation skills). Overall, these results support the TechArm's potential as a tool with a bi-fold function as: (i) an assessment device, to provide quantitative measures about the role played by different sensory modalities in perception, when a sensory deprivation occurs, and (ii) a rehabilitative device, which could be integrated within personalized training programs, according to each patient's sensory and cognitive profile, for the rehabilitation of impaired perceptual functions.
The raw data supporting the conclusions of this article are available at the doi 10.5281/zenodo.7651325, without undue reservation.
The studies involving human participants were reviewed and approved by Comitato Etico della Regione Liguria (Genova, Italy, prot. IIT_UVIP_COMP_2019 N. 02/2020) and Comitato Etico Policlinico San Matteo Referente Area Pavia (Pavia, Italy, prot. p-20190078848). Written informed consent to participate in this study was provided by the participants' legal guardian.
FM and LS contributed to the study design and implementation, data collection, data analysis, and article writing and reviewing. GC contributed to the study design, data collection support, data analysis, and article writing and reviewing. CM contributed to the study design and data collection. SS and MG contributed to the study conception, supervision, funding, and article reviewing. All authors contributed to the article and approved the submitted version.
This work was supported by the joint lab between the Unit for Visually Impaired People at the Istituto Italiano di Tecnologia (IIT) and the Developmental Neuro-ophthalmology Unit at the IRCCS Mondino Foundation.
We thank Nicolò Balzarotti for his support in data analysis. We thank Marco Crepaldi, Antonio Maviglia, and the Electronic Design Laboratory at the Istituto Italiano di Tecnologia, for the technical support in the development of the TechArm system. Special thanks go to every child and family that we encountered for their willing participation in our clinical and research activity.
The authors declare that the research was conducted in the absence of any commercial or financial relationships that could be construed as a potential conflict of interest.
All claims expressed in this article are solely those of the authors and do not necessarily represent those of their affiliated organizations, or those of the publisher, the editors and the reviewers. Any product that may be evaluated in this article, or claim that may be made by its manufacturer, is not guaranteed or endorsed by the publisher.
The Supplementary Material for this article can be found online at: https://www.frontiersin.org/articles/10.3389/fnins.2023.1158438/full#supplementary-material
Alary, F., Duquette, M., Goldstein, R., Chapman, C. E., Voss, P., La Buissonnière-Ariza, V., et al. (2009). Tactile acuity in the blind: a closer look reveals superiority over the sighted in some but not all cutaneous tasks. Neuropsychologia 47, 2037–2043. doi: 10.1016/j.neuropsychologia.2009.03.014
Amadeo, M. B., Campus, C., and Gori, M. (2019). Impact of years of blindness on neural circuits underlying auditory spatial representation. Neuroimage 191, 140–149. doi: 10.1016/j.neuroimage.2019.01.073
Barutchu, A., Danaher, J., Crewther, S. G., Innes-Brown, H., Shivdasani, M. N., and Paolini, A. G. (2010). Audiovisual integration in noise by children and adults. J. Exp. Child Psychol. 105, 38–50. doi: 10.1016/j.jecp.2009.08.005
Bathelt, J., de Haan, M., and Dale, N. J. (2019). Adaptive behaviour and quality of life in school-age children with congenital visual disorders and different levels of visual impairment. Res. Dev. Disabil. 85, 154–162. doi: 10.1016/j.ridd.2018.12.003
Berto, M., Ricciardi, E., Pietrini, P., and Bottari, D. (2021). Interactions between auditory statistics processing and visual experience emerge only in late development. Iscience 24, 103383. doi: 10.1016/j.isci.2021.103383
Bremner, J. G., Hatton, F., Foster, K. A., and Mason, U. (2011). The contribution of visual and vestibular information to spatial orientation by 6-to 14-month-old infants and adults. Dev. Sci. 14, 1033–1045. doi: 10.1111/j.1467-7687.2011.01051.x
Burr, D., and Gori, M. (2012). “Multisensory integration develops late in humans,” in The Neural Bases of Multisensory Processes, eds M. M. Murray and M. T. Wallace MT (Boca Raton, FL: CRC Press/Taylor & Francis).
Cao, Y., Summerfield, C., Park, H., Giordano, B. L., and Kayser, C. (2019). Causal inference in the multisensory brain. Neuron 102, 1076–1087. doi: 10.1016/j.neuron.2019.03.043
Cappagli, G., Cocchi, E., and Gori, M. (2017a). Auditory and proprioceptive spatial impairments in blind children and adults. Dev. Sci. 20, e12374. doi: 10.1111/desc.12374
Cappagli, G., Finocchietti, S., Baud-Bovy, G., Cocchi, E., and Gori, M. (2017b). Multisensory rehabilitation training improves spatial perception in totally but not partially visually deprived children. Front. Integr. Neurosci. 11, 29. doi: 10.3389/fnint.2017.00029
Cappagli, G., Finocchietti, S., Cocchi, E., Giammari, G., Zumiani, R., Cuppone, A. V., et al. (2019). Audio motor training improves mobility and spatial cognition in visually impaired children. Sci. Rep. 9, 3303. doi: 10.1038/s41598-019-39981-x
Cappagli, G., and Gori, M. (2016). Auditory spatial localization: developmental delay in children with visual impairments. Res. Dev. Disabil. 53, 391–398. doi: 10.1016/j.ridd.2016.02.019
Chebat, D.-R., Harrar, V., Kupers, R., Maidenbaum, S., Amedi, A., and Ptito, M. (2018). “Sensory substitution and the neural correlates of navigation in blindness,” in Mobility of Visually Impaired People: Fundamentals and ICT Assistive Technologies, eds E. Pissaloux and R. Velazquez (Springer), 167–200.
Collignon, O., Dormal, G., Albouy, G., Vandewalle, G., Voss, P., Phillips, C., et al. (2013). Impact of blindness onset on the functional organization and the connectivity of the occipital cortex. Brain 136, 2769–2783. doi: 10.1093/brain/awt176
Collignon, O., Vandewalle, G., Voss, P., Albouy, G., Charbonneau, G., Lassonde, M., et al. (2011). Functional specialization for auditory–spatial processing in the occipital cortex of congenitally blind humans. Proc. Natl. Acad. Sci. U.S.A. 108, 4435–4440. doi: 10.1073/pnas.1013928108
Collignon, O., Voss, P., Lassonde, M., and Lepore, F. (2009). Cross-modal plasticity for the spatial processing of sounds in visually deprived subjects. Exp. Brain Res. 192, 343–358. doi: 10.1007/s00221-008-1553-z
Cuturi, L., Cappagli, G., Finocchietti, S., Cocchi, E., and Gori, M. (2017). New rehabilitation technology for visually impaired children and adults based on multisensory integration. J. Vis. 17, 592–592. doi: 10.1167/17.10.592
Dale, N., and Sonksen, P. (2002). Developmental outcome, including setback, in young children with severe visual impairment. Dev. Med. Child Neurol. 44, 613–622. doi: 10.1111/j.1469-8749.2002.tb00846.x
Denervaud, S., Gentaz, E., Matusz, P. J., and Murray, M. M. (2020). Multisensory gains in simple detection predict global cognition in schoolchildren. Sci. Rep. 10, 1394. doi: 10.1038/s41598-020-58329-4
Eimer, M. (2004). Multisensory integration: how visual experience shapes spatial perception. Curr. Biol. 14, R115–R117. doi: 10.1016/j.cub.2004.01.018
Giudice, N. A. (2018). “Navigating without vision: principles of blind spatial cognition,” in Handbook of Behavioral and Cognitive Geography, ed D. R. Montello (Edward Elgar Publishing), 260–288. doi: 10.4337/9781784717544.00024.
Gori, M. (2015). Multisensory integration and calibration in children and adults with and without sensory and motor disabilities. Multisens. Res. 28, 71–99. doi: 10.1163/22134808-00002478
Gori, M., Amadeo, M. B., and Campus, C. (2020). Spatial metric in blindness: behavioural and cortical processing. Neurosci. Biobehav. Rev. 109, 54–62. doi: 10.1016/j.neubiorev.2019.12.031
Gori, M., Campus, C., and Cappagli, G. (2021). Late development of audio-visual integration in the vertical plane. Curr. Res. Behav. Sci. 2, 100043. doi: 10.1016/j.crbeha.2021.100043
Gori, M., Del Viva, M., Sandini, G., and Burr, D. C. (2008). Young children do not integrate visual and haptic form information. Curr. Biol. 18, 694–698. doi: 10.1016/j.cub.2008.04.036
Gori, M., Sandini, G., Martinoli, C., and Burr, D. C. (2014). Impairment of auditory spatial localization in congenitally blind human subjects. Brain 137, 288–293. doi: 10.1093/brain/awt311
Grizzle, R. (2011). Wechsler Intelligence Scale for Children. Encyclopedia of child behavior and development, 1553–1555.
Guest, S., Catmur, C., Lloyd, D., and Spence, C. (2002). Audiotactile interactions in roughness perception. Exp. Brain Res. 146, 161–171. doi: 10.1007/s00221-002-1164-z
Hötting, K., and Röder, B. (2004). Hearing cheats touch, but less in congenitally blind than in sighted individuals. Psychol. Sci. 15, 60–64. doi: 10.1111/j.0963-7214.2004.01501010.x
Hyvärinen, L., Näsänen, R., and Laurinen, P. (1980). New visual acuity test for pre-school children. Acta Ophthalmol. 58, 507–511. doi: 10.1111/j.1755-3768.1980.tb08291.x
Kitagawa, N., and Spence, C. (2006). Audiotactile multisensory interactions in human information processing. Jpn. Psychol. Res. 48, 158–173. doi: 10.1111/j.1468-5884.2006.00317.x
Kolarik, A. J., Raman, R., Moore, B. C., Cirstea, S., Gopalakrishnan, S., and Pardhan, S. (2020). The accuracy of auditory spatial judgments in the visually impaired is dependent on sound source distance. Sci. Rep. 10, 7169. doi: 10.1038/s41598-020-64306-8
Körding, K. P., Beierholm, U., Ma, W. J., Quartz, S., Tenenbaum, J. B., and Shams, L. (2007). Causal inference in multisensory perception. PLoS ONE 2, e943. doi: 10.1371/journal.pone.0000943
Martolini, C., Cappagli, G., Signorini, S., and Gori, M. (2021). Effects of increasing stimulated area in spatiotemporally congruent unisensory and multisensory conditions. Brain Sci. 11, 343. doi: 10.3390/brainsci11030343
Merabet, L. B., and Pascual-Leone, A. (2010). Neural reorganization following sensory loss: the opportunity of change. Nat. Rev. Neurosci. 11, 44–52. doi: 10.1038/nrn2758
Morelli, F., Aprile, G., Cappagli, G., Luparia, A., Decortes, F., Gori, M., et al. (2020). A multidimensional, multisensory and comprehensive rehabilitation intervention to improve spatial functioning in the visually impaired child: a community case study. Front. Neurosci. 14, 768. doi: 10.3389/fnins.2020.00768
Morrongiello, B. A., Humphrey, G. K., Timney, B., Choi, J., and Rocca, P. T. (1994). Tactual object exploration and recognition in blind and sighted children. Perception 23, 833–848. doi: 10.1068/p230833
Moussa, G., Bassilious, K., and Mathews, N. (2021). A novel excel sheet conversion tool from Snellen fraction to LogMAR including ‘counting fingers', ‘hand movement', ‘light perception' and ‘no light perception' and focused review of literature of low visual acuity reference values. Acta Ophthalmol. 99, e963–e965. doi: 10.1111/aos.14659
Murray, M. M., Molholm, S., Michel, C. M., Heslenfeld, D. J., Ritter, W., Javitt, D. C., et al. (2005). Grabbing your ear: rapid auditory–somatosensory multisensory interactions in low-level sensory cortices are not constrained by stimulus alignment. Cereb. Cortex 15, 963–974. doi: 10.1093/cercor/bhh197
Neil, P. A., Chee-Ruiter, C., Scheier, C., Lewkowicz, D. J., and Shimojo, S. (2006). Development of multisensory spatial integration and perception in humans. Dev. Sci. 9, 454–464. doi: 10.1111/j.1467-7687.2006.00512.x
Pasqualotto, A., and Proulx, M. J. (2012). The role of visual experience for the neural basis of spatial cognition. Neurosci. Biobehav. Rev. 36, 1179–1187. doi: 10.1016/j.neubiorev.2012.01.008
Sanabria, D., Soto-Faraco, S., and Spence, C. (2005). Spatiotemporal interactions between audition and touch depend on hand posture. Exp. Brain Res. 165, 505–514. doi: 10.1007/s00221-005-2327-5
Schiatti, L., Cappagli, G., Martolini, C., Maviglia, A., Signorini, S., Gori, M., et al. (2020). “A novel wearable and wireless device to investigate perception in interactive scenarios,” in 2020 42nd Annual International Conference of the IEEE Engineering in Medicine & Biology Society (EMBC) (IEEE), 3252–3255.
Senna, I., Piller, S., Gori, M., and Ernst, M. (2022). The power of vision: calibration of auditory space after sight restoration from congenital cataracts. Proc. R. Soc. B 289, 20220768. doi: 10.1098/rspb.2022.0768
Smith, J. N. (1972). Development of Species Identification in Birds (Subtitled: An Inquiry into the Prenatal Determinants of Perception). Chicago, IL: University of Chicago Press.
Soto-Faraco, S., Spence, C., and Kingstone, A. (2004). Cross-modal dynamic capture: congruency effects in the perception of motion across sensory modalities. J. Exp. Psychol. Hum. Percept. Perform. 30, 330. doi: 10.1037/0096-1523.30.2.330
Tao, Q., Chan, C. C., Luo, Y.-J., Li, J.-J., Ting, K.-H., Wang, J., et al. (2015). How does experience modulate auditory spatial processing in individuals with blindness? Brain Topogr. 28, 506–519. doi: 10.1007/s10548-013-0339-1
Thinus-Blanc, C., and Gaunet, F. (1997). Representation of space in blind persons: vision as a spatial sense? Psychol. Bull. 121, 20. doi: 10.1037/0033-2909.121.1.20
Thomas, R. L., Misra, R., Akkunt, E., Ho, C., Spence, C., and Bremner, A. J. (2018). Sensitivity to auditory-tactile colocation in early infancy. Dev. Sci. 21, e12597. doi: 10.1111/desc.12597
Tinti, C., Adenzato, M., Tamietto, M., and Cornoldi, C. (2006). Visual experience is not necessary for efficient survey spatial cognition: evidence from blindness. Q. J. Exp. Psychol. 59, 1306–1328. doi: 10.1080/17470210500214275
Vasilyeva, M., and Lourenco, S. F. (2010). “Spatial development,” in The Handbook of Life-Span Development, Vol. 1. Cognition, Biology, and Method, eds W. F. Overton and R. M. Lerner (John Wiley & Sons, Inc), 720–753. doi: 10.1002/9780470880166.hlsd001020
Voss, P., Lassonde, M., Gougoux, F., Fortin, M., Guillemot, J.-P., and Lepore, F. (2004). Early-and late-onset blind individuals show supra-normal auditory abilities in far-space. Curr. Biol. 14, 1734–1738. doi: 10.1016/j.cub.2004.09.051
Keywords: assistive technologies, visual impairment, multisensory, rehabilitation, development
Citation: Morelli F, Schiatti L, Cappagli G, Martolini C, Gori M and Signorini S (2023) Clinical assessment of the TechArm system on visually impaired and blind children during uni- and multi-sensory perception tasks. Front. Neurosci. 17:1158438. doi: 10.3389/fnins.2023.1158438
Received: 03 February 2023; Accepted: 12 April 2023;
Published: 02 June 2023.
Edited by:
Alessandra Pedrocchi, Polytechnic University of Milan, ItalyReviewed by:
Filippo Amore, Fondazione Policlinico Universitario Agostino Gemelli-IRCCS, ItalyCopyright © 2023 Morelli, Schiatti, Cappagli, Martolini, Gori and Signorini. This is an open-access article distributed under the terms of the Creative Commons Attribution License (CC BY). The use, distribution or reproduction in other forums is permitted, provided the original author(s) and the copyright owner(s) are credited and that the original publication in this journal is cited, in accordance with accepted academic practice. No use, distribution or reproduction is permitted which does not comply with these terms.
*Correspondence: Giulia Cappagli, Z2l1bGlhLmNhcHBhZ2xpQGlpdC5pdA==
†These authors have contributed equally to this work and share first authorship
Disclaimer: All claims expressed in this article are solely those of the authors and do not necessarily represent those of their affiliated organizations, or those of the publisher, the editors and the reviewers. Any product that may be evaluated in this article or claim that may be made by its manufacturer is not guaranteed or endorsed by the publisher.
Research integrity at Frontiers
Learn more about the work of our research integrity team to safeguard the quality of each article we publish.