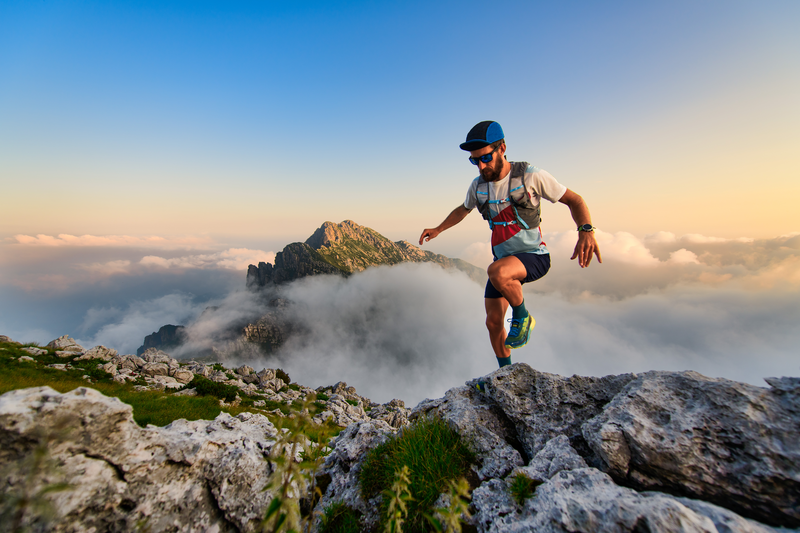
94% of researchers rate our articles as excellent or good
Learn more about the work of our research integrity team to safeguard the quality of each article we publish.
Find out more
ORIGINAL RESEARCH article
Front. Neurosci. , 12 April 2023
Sec. Translational Neuroscience
Volume 17 - 2023 | https://doi.org/10.3389/fnins.2023.1158111
This article is part of the Research Topic Prevention, Diagnosis, and Treatment for Brain-Heart Comorbid Disease View all 5 articles
Objective: Methionine sulfoxide (MetO) has been identified as a risk factor for vascular diseases and was considered as an important indicator of oxidative stress. However, the effects of MetO and its association with moyamoya disease (MMD) remained unclear. Therefore, we performed this study to evaluate the association between serum MetO levels and the risk of MMD and its subtypes.
Methods: We eventually included consecutive 353 MMD patients and 88 healthy controls (HCs) with complete data from September 2020 to December 2021 in our analyzes. Serum levels of MetO were quantified using liquid chromatography-mass spectrometry (LC–MS) analysis. We evaluated the role of MetO in MMD using logistic regression models and confirmed by receiver-operating characteristic (ROC) curves and area under curve (AUC) values.
Results: We found that the levels of MetO were significantly higher in MMD and its subtypes than in HCs (p < 0.001 for all). After adjusting for traditional risk factors, serum MetO levels were significantly associated with the risk of MMD and its subtypes (p < 0.001 for all). We further divided the MetO levels into low and high groups, and the high MetO level was significantly associated with the risk of MMD and its subtypes (p < 0.05 for all). When MetO levels were assessed as quartiles, we found that the third (Q3) and fourth (Q4) MetO quartiles had a significantly increased risk of MMD compared with the lowest quartile (Q3, OR: 2.323, 95%CI: 1.088–4.959, p = 0.029; Q4, OR: 5.559, 95%CI: 2.088–14.805, p = 0.001).
Conclusion: In this study, we found that a high level of serum MetO was associated with an increased risk of MMD and its subtypes. Our study raised a novel perspective on the pathogenesis of MMD and suggested potential therapeutic targets.
Moyamoya disease (MMD), as a chronic intracranial vasculopathy, was unknown for its etiology. MMD was characterized by the stenosis or occlusion at the terminal portion and main branches of internal carotid artery (ICA) associated with the formation of anomalous collateral circulation on angiography (Kuroda and Houkin, 2008). MMD was more prevalent in East Asian countries than in Europe and North America (Kim, 2016). Moreover, familial aggregation and RNF213 variants suggested the genetic etiology in MMD (Miyatake et al., 2012; Kim et al., 2016; Zhang et al., 2017). Our previous study has revealed the correlation between several traditional modifiable risk factors and MMD (Ge et al., 2020). These findings indicated that genetic, inflammation, and environmental factors were involved in MMD. Currently, direct and indirect revascularizations have been the most routine treatment for MMD patients (Acker et al., 2018; Deng et al., 2018). However, the outcome after surgery varied widely, and the MMD process seemed irreversible. Therefore, it has been necessary to investigate novel risk factors in the pathogenesis and progression of MMD.
In recent years, with the further study of multi-omics technologies, researchers have found that the ultimate effect of gene or protein regulatory action was to cause changes in metabolites in body (Holmes et al., 2018; Yang et al., 2018; Zhang et al., 2022). Metabolomics studied endogenous small molecule compounds at the end of metabolic pathways, which could skip the complex regulatory processes of body and directly reflect the final physiological and pathological changes (Ke et al., 2018; Guo et al., 2020; Chumachenko et al., 2022). Through the analysis of differential metabolites, researchers could reveal pathogenic mechanisms, confirm diagnostic biomarkers, estimate disease risk, and monitor treatment processes (Wishart, 2019; Shin et al., 2020; Wang et al., 2020). Homocysteine (Hcy), one of the methionine (Met) related metabolites, has been identified associated with MMD and its prognosis (Ge et al., 2020; He et al., 2022). It indicated the important role of Met metabolism in MMD. Methionine sulfoxide (MetO), another Met-related metabolite, has been studied to be associated with neurodegenerative diseases, mental health diseases, obesity, cancers, and cardiovascular diseases (Lei et al., 2007; De Luca et al., 2010; Ma et al., 2011; Rose and Hoffmann, 2015; Jiang and Moskovitz, 2018). Furthermore, MetO could reflect the level of oxidative stress. However, the metabolomics studies on MMD were still limited, and the role of MetO in MMD has hardly been studied.
Therefore, this study aimed to demonstrate the correlation between MetO and the risk of MMD, contributing to the exploration of novel risk factors and the identification of potential therapeutic targets in MMD.
In this prospective study, we consecutively included MMD patients from September 2020 to December 2021 and collected the clinical data. All patients were diagnosed as MMD with digital subtraction angiography (DSA) according to the 2012 Japanese diagnostic criteria (Guidelines for diagnosis and treatment of moyamoya disease, 2012). Minors and patients without complete liquid chromatography-mass spectrometry (LC–MS) data of Met-related metabolites were excluded. We recruited 89 age-matched healthy individuals as the control group. All healthy controls (HCs) underwent routine examinations and were excluded cerebrovascular diseases. One HC with inadequate Met-related metabolite data was excluded. A total of 353 adult MMD patients (253 ischemic-type, 100 hemorrhagic-type) and 88 HCs were eventually included (Figure 1). All participants have provided written informed consent to participate in this research. This study has been approved by the Ethics Committee of Beijing Tiantan Hospital.
All baseline characteristics, including demographic data (age and gender), medical history (hypertension, diabetes, hyperlipidemia, smoking, drinking), clinical features (heart rate, systolic blood pressure [SBP], diastolic blood pressure [DBP], body mass index [BMI]) were collected by independent chart reviews. Fasting peripheral blood samples from all participants were used for routine and biochemical blood tests, as shown in Table 1. We also calculated peripheral inflammation indicators based on the laboratory examination data, which were also presented in Table 1 (Zeng et al., 2022). The peripheral blood samples were obtained on the day of admission, then centrifuged, separated, and aliquoted into separation tubes in 1 h. All blood samples were stored at −80°C until testing. LC–MS analysis has been used for MetO quantification, and the laboratory technicians were blinded to all patients’ data. We detected RNF213 p.R4810K variant with primer designation of RNF213-4810F 5’-GCCCTCCATTTCTAGCACAC-3′ and RNF213-4810R 5’-AGCTGTGGCGAAAGCTTCTA-3′. Neurological status was assessed by the modified Rankin Scale (mRS) at admission and divided into two groups (0–2, 3–5). The Suzuki stage of MMD patients was defined as the more severe side.
The SPSS software (version 26.0) and R project (version 3.6.3) were used for all statistical analyzes. Continuous data were compared using t-tests or Mann–Whitney U tests between two groups. Kruskal-Wallis tests or one-way ANOVA were used for comparisons among multiple groups. Continuous data were presented as mean with standard deviation (SD) or median with interquartile range (IQR). Categorical variables were compared with Pearson chi-square tests, Fisher exact tests, and Kruskal-Wallis tests, and categorical variables were presented as frequencies. Spearman correlation tests were used to assess the association between clinical characteristics and MetO quartiles. We have performed three logistic regression models to analyze the role of MetO in MMD and its subtypes, including Crude model, Model 1, and Model 2, which have been described in previous studies (Ge et al., 2022; Zeng et al., 2022). Crude model was the unadjusted regression model of MetO. Model 1 was adjusted for age, gender, heart rate, SBP, DBP, and BMI. Model 2 was further adjusted for WBC count, LY count, NEUT count, MONO count, PLT count, GLU, ALB, Cr, UA, TG, TC, HDL-C, LDL-C, ApoA, ApoB, Hcy, NLR, MLR, PLR, SII, and MHR. Receiver-operating characteristic (ROC) curves and area under curve (AUC) values were used to evaluate the predictive ability of the models for the risk of MMD and its subtypes. The area under curve (AUC) has been calculated. For all analyzes, a two-sided p value<0.05 was considered statistically significant.
In this study, a total of 353 MMD patients (253 ischemic-type, 100 hemorrhagic-type) and 88 age-matched HCs were eventually included for analysis. Baseline variables were compared between HCs and MMD patients (Table 1). There were no significant differences in age and gender (p > 0.05). However, rates of hypertension, diabetes, hyperlipidemia, smoking, and drinking were significantly higher in the MMD group than in the HC group (p < 0.05 for all). Regarding clinical features, the MMD group had significantly higher levels of SBP (p < 0.001), DBP (p = 0.001), and BMI (p = 0.005). Additionally, levels of inflammatory biomarkers were significantly higher in the MMD group, including WBC count, NEUT count, NLR, SII, and MHR (p < 0.05 for all). There were also more risk factors in the MMD group, including significantly higher levels of TG, Hcy, and lower levels of HDL-C (p < 0.05 for all). In addition, the MMD group had significantly higher levels of MetO compared to the HCs (p < 0.001; Figure 2A).
Figure 2. Levels of MetO between HCs and MMD and its subtypes. ns, not significant; ***, p < 0.001. (A) Comparison of MetO levels between HCs and MMD patients. (B) Comparison of MetO levels between HCs and MMD subtypes.
We also compared these variables between HCs and MMD subtypes (Table 2), and the results were similar. Moreover, the level of glucose in the ischemic-type MMD group was significantly higher than in the HC group (p = 0.015), indicating a higher risk for ischemia. Levels of MetO were significantly higher in both ischemic-type and hemorrhagic-type MMD groups than in the HC group (p < 0.001 for both; Figure 2B).
The MMD patients were categorized into two groups based on the median level of MetO, including low-MetO group and high-MetO group. The clinical characteristics of MMD patients in both groups have been shown (Table 3). Although the difference was not statistically significant, patients in the high-MetO group tended to be elder. Furthermore, MMD patients in the high-MetO group had a higher proportion of males, higher levels of BMI, WBC count, NEUT count, MONO count, UA, and MHR. Conversely, these patients had lower levels of HDL-C and ApoA (p < 0.05 for all).
We also compared the clinical variables of MMD patients based on the quartile levels of MetO (Table 4). The results showed a correlation between higher levels of MetO and an increased proportion of females (p = 0.006). Moreover, patients with higher levels of MetO were associated with higher levels of BMI, UA, MHR, and lower levels of HDL-C and ApoA (p < 0.05 for all).
In this study, we observed a positive association between serum MetO and the risk of MMD in Crude model (OR: 28.695, 95%CI: 8.033–102.500, p < 0.001; Table 5). In Model 1, the risk of MMD increased with each increment in MetO level (OR: 30.332, 95%CI: 7.812–117.770, p < 0.001). In Model 2, MetO was also found to increase the risk of MMD (OR: 20.215, 95%CI: 4.842–84.397, p < 0.001). The ROC curves revealed that the predictive accuracy of Model 2 (AUC = 0.841; Figure 3A) significantly improved compared to Crude model (AUC = 0.692) and Model 1 (AUC = 0.776). Similar results were found in ROC curves of three models for MMD subtypes (Table 5; Figures 3B,C).
Figure 3. ROC curves of MetO in different models for the risk of MMD and its subtypes. (A) MMD overall; (B) Ischemic-type; (C) Hemorrhagic-type.
We also compared the risk of MMD between low and high MetO levels. We found cases with high MetO level had a significantly higher risk of MMD in Crude model (OR: 3.832, 95%CI: 2.264–6.487, p < 0.001), Model 1 (OR: 3.699, 95%CI: 2.142–6.389, p < 0.001), and Model 2 (OR: 2.888, 95%CI: 1.613–5.170, p < 0.001; Table 5). The AUC values showed an improvement in Crude model (AUC = 0.655; Figure 4A), Model 1 (AUC = 0.760), and Model 2 (AUC = 0.829). Similar results were observed in Crude model, Model 1, and Model 2 of ischemic and hemorrhagic MMD (Figures 4B,C).
Figure 4. ROC curves of low and high levels of MetO in different models for the risk of MMD and its subtypes. (A) MMD overall; (B) Ischemic-type; (C) Hemorrhagic-type.
Furthermore, we assessed MetO as quartiles and analyzed its risk in MMD. We found that the proportion of MMD events increased with the rise of MetO quartiles (Figures 5A–C). In contrast to Q1 of MetO, cases in Q3 and Q4 of MetO had a significantly higher risk of MMD in Crude model (Q3, OR: 2.742, 95%CI: 1.410–5.330, p = 0.003; Q4, OR: 8.167, 95%CI: 3.270–20.397, p < 0.001), Model 1 (Q3, OR: 3.064, 95%CI: 1.528–6.146, p = 0.002; Q4, OR: 7.855, 95%CI: 3.062–20.149, p < 0.001), and Model 2 (Q3, OR: 2.323, 95%CI: 1.088–4.959, p = 0.029; Q4, OR: 5.559, 95%CI: 2.088–14.805, p = 0.001). The AUC value of ROC curves increased with the development of the models (Crude model, AUC = 0.682; Model 1, AUC = 0.762; Model2, AUC = 0.834 Figure 6A). Consistent with MMD overall, the risk of ischemic and hemorrhagic MMD increased with increasing MetO quartiles. In comparison to the lowest quartile (Q1), cases in the third (Q3) and fourth (Q4) MetO quartiles showed a significantly correlation with ischemic MMD in Crude model (Q3, OR: 2.578, 95%CI: 1.293–5.142, p = 0.042; Q4, OR: 7.396, 95%CI: 2.906–18.823, p < 0.001), Model 1 (Q3, OR: 2.518, 95%CI: 1.187–5.341, p = 0.016; Q4, OR: 5.927, 95%CI: 2.215–15.858, p < 0.001), and Model 2 (Q3, OR: 2.806, 95%CI: 1.148–6.861, p = 0.024; Q4, OR: 5.620, 95%CI: 1.917–16.478, p = 0.002). The AUC of ROC curves in Crude model, Model 1, and Model 2 were 0.671, 0.792, and 0.889, respectively (Figure 6B). Similarly, the third (Q3) and fourth (Q4) MetO quartiles were significantly correlated with the risk of hemorrhagic MMD in Crude model (Q3, OR: 3.224, 95%CI: 1.405–7.394, p = 0.006; Q4, OR: 10.439, 95%CI: 3.719–29.300, p < 0.001), Model 1 (Q3, OR: 3.333, 95%CI: 1.429–7.772, p = 0.005; Q4, OR: 9.178, 95%CI: 3.217–26.185, p < 0.001), and Model 2 (Q3, OR: 3.400, 95%CI: 1.255–9.208, p = 0.016; Q4, OR: 12.496, 95%CI: 3.740–41.745, p < 0.001). The AUC of ROC curves in Crude model, Model 1, and Model 2 were 0.711, 0.754, and 0.831, respectively (Figure 6C).
Figure 5. Association between different quartiles of MetO and the risk of MMD and its subtypes. (A) MMD overall; (B) Ischemic-type; (C) Hemorrhagic-type.
Figure 6. ROC curves of MetO quartiles in different models for the risk of MMD and its subtypes. (A) MMD overall; (B) Ischemic-type; (C) Hemorrhagic-type.
In this prospective study, we compared the difference in clinical characteristics between MMD patients and HCs. We also investigated the differences between MMD subtypes and HCs. Our findings revealed that serum MetO levels were significantly higher in the MMD group and its subtypes than those in the HC group. Subsequently, we analyzed the relationship between MetO and the risk of MMD and found that MetO was an independent risk factor. The risk of MMD increased with the elevation of MetO levels. This study indicated that MetO might play a crucial role in the pathogenesis and progression of MMD.
Met, as an essential amino acid, was highly susceptible to oxidation. Under physiological and pathological oxidative stress conditions, reactive oxygen species (ROS) result in the oxidation of Met to MetO, including two stereoisomers, S-MetO and R-MetO (Brot et al., 1981; Moskovitz et al., 1996; Makukhin et al., 2016). The increase in MetO levels result in the accumulation of toxic proteins and alterations in cellular functions (Moskovitz and Smith, 2021). MetO is an attractive biomarker for oxidative stress and an important indicator for its assessment (Gu et al., 2015). The MetO reductase system could specifically reverse MetO to Met (Moskovitz et al., 2000; Grimaud et al., 2001; Lourenço Dos Santos et al., 2018). The MetO reductase system played an important role in regulating protein functions, signaling pathways, and repairing oxidative damage proteins. Several studies have confirmed that the enhanced function of the MetO reductase system could increase cellular resistance to oxidative stress and related diseases (Moskovitz et al., 1998, 2001; Lee et al., 2005). Elevated levels of MetO were associated with neurodegenerative diseases, cancers, cardiovascular disease, stroke, and other diseases (Gu et al., 2015; Reiterer et al., 2019). However, the metabolomics of patients with MMD has not been extensively studied. In this study, we found that serum MetO levels in MMD patients were significantly higher than those in HCs, and MetO was positively associated with the risk of MMD.
The link between MetO and cerebrovascular disease has been shown. A recent large prospective study on metabolomic characteristics of ischemic stroke found a significant association between MetO and incident stroke, with higher levels being associated with increased risk (Balasubramanian et al., 2022). Another study demonstrated that betaine could decrease proinflammatory cytokine production and reduce oxidative stress after brain ischemia and reperfusion injury by upragulating MetO reductase (Li et al., 2022). Moreover, the formation of MetO in von Willebrand factor was found to inhibit the ADAMTS-13 cleavage, promoting thrombosis in oxidative stress-related diseases (Lancellotti et al., 2010).
MMD was considered to result from a combination of inflammation, genetic, and other factors. Previous evidence showed that levels of oxidative stress in endothelial colony-forming cells were significantly increased in MMD patients (Choi et al., 2018). By eliminating ROS, the endothelial cells showed an improvement in angiogenesis capacity. It indicated that MMD was an oxidative stress-related disease with chronic inflammatory response. A previous study found that overexpression of MetO reductase A could reduce MetO in calcium/calmodulin-dependent protein kinase II, attenuating ROS-augmented NF-κB activation in endothelial cells (Gu et al., 2016). Another study showed that MetO reductase played an antioxidant role in vascular smooth muscle cells and prevented oxidative damage in cytoplasm and mitochondria (Haenold et al., 2008). In addition, consistent with the increase of MetO levels, vasodilatative activity decreased and vasoconstrictive mediators significantly increased (Pichler Hefti et al., 2013). Although the precise mechanism by which MetO contributed to MMD remained unclear, it was hypothesized that high levels of MetO induced the dysfunction in vessel-associated cells and the dysregulation in vasoactive substances, which might be involved in MMD pathogenesis.
Oxidative stress was generally accompanied by inflammation responses characterized by the production of ROS and the release of inflammatory cytokines. MetO has been shown to induce the activation of M1/classical macrophage activation, alter the extracellular nucleotide metabolism, and promote the increase of macrophage ATPase/ADPase activity (Dos Santos et al., 2017). The MetO reductase system might play a positive role in antioxidant defense and inflammation-related damage signal transduction pathways. Overexpression of MetO reductase A in microglia cells led to the reduction in lipopolysaccharide (LPS)-induced activation of ROS/MAPKs/NF-κB signaling pathways (Fan et al., 2015). Silencing MetO reductase A led to the activation of microglia activation and the production of Iba1, TNF-α, IL-1β, ROS, and NOX2 (Fan et al., 2020). Additionally, MetO reductase B1 activated the transcription-6 (STAT6) pathway after immunization and promoted the differentiation of T-helper cells type 1 and follicular helper T cells (Lee et al., 2017). Furthermore, MetO reductase deficiency could cause vascular smooth muscle cell proliferation and neointimal hyperplasia after vascular injury (Klutho et al., 2015). Therefore, we speculated that the combination of immune response disorder and abnormal intimal hyperplasia caused by MetO reductase deficiency might be the underlying cause of MMD.
Our previous study has shown that several factors, such as ALB, BMI and HDL-C, were associated with the risk of MMD (Ge et al., 2020). In this study, we found that some of these factors had the same trend with MetO levels, including BMI, uric acid, HDL-C, and ApoA. These results indicated that MetO was associated with the dysregulation of lipid metabolism in MMD. Moreover, our study revealed that inflammatory factors were mostly elevated in high MetO groups, which was consistent with the increasing levels of inflammatory biomarkers in MMD and its subtypes compared to HCs. In conclusion, MetO might have an impact on vascular damage through dyslipidemia and chronic inflammatory responses. After adjusting for potential confounders in multivariate regression models, we found that MetO was still significantly associated with the risk of MMD overall, ischemic-types, and hemorrhagic-types. These results highlighted the importance of MetO downregulation, and suggested that MetO reductases might serve as potential therapeutic targets for MMD. However, there were still several limitations in our study. Firstly, Due to the nature of single-center, the number of participants included in our study was relatively limited. Large multi-center prospective studies were needed for further validation. Secondly, the participants in our study were all adults. It was still unclear whether the findings were consistent in pediatric patients. Thirdly, the dietary intake information of participants was not collected in the study, which might affect the outcome. Fourthly, the regulatory mechanism and signaling pathways of MetO in MMD needed further studies in vitro and in vivo.
In summary, our study revealed that the levels of MetO was increased in MMD patients, and we identified MetO as an independent risk factor for MMD and its subtypes. These findings suggested that MetO reductases might serve as potential therapeutic targets. Our study provided a novel perspective on the risk of MMD.
The raw data supporting the conclusions of this article will be made available by the authors, without undue reservation.
The studies involving human participants were reviewed and approved by Ethics Committee of Beijing Tiantan Hospital, Capital Medical University. The patients/participants provided their written informed consent to participate in this study.
JL illustrated all the results and drafted the manuscript. PG and CZ performed all the statistical analyzes. QH, CL, CT, and YuZ collected the data. JW, QZ, RW, and YaZ revised the manuscript. DZ and JZ conceived and designed this research. All authors have read and approved the final version of the manuscript.
This study was supported by the National Natural Science Foundation of China (81701137), the National Key Research and Development Program of China (2021YFC2500502), Beijing Municipal Organization Department Talents Project (2015000021469G219), and Beijing Municipal Administration of Hospitals’ Mission Plan (SML20150501).
We acknowledged all the participants involved in this study.
The authors declare that the research was conducted in the absence of any commercial or financial relationships that could be construed as a potential conflict of interest.
All claims expressed in this article are solely those of the authors and do not necessarily represent those of their affiliated organizations, or those of the publisher, the editors and the reviewers. Any product that may be evaluated in this article, or claim that may be made by its manufacturer, is not guaranteed or endorsed by the publisher.
Acker, G., Fekonja, L., and Vajkoczy, P. (2018). Surgical Management of Moyamoya Disease. Stroke 49, 476–482. doi: 10.1161/strokeaha.117.018563
Balasubramanian, R., Hu, J., Guasch-Ferre, M., Li, J., Sorond, F., Zhao, Y., et al. (2022). Metabolomic profiles associated with incident ischemic stroke. Neurology 98, e483–e492. doi: 10.1212/wnl.0000000000013129
Brot, N., Weissbach, L., Werth, J., and Weissbach, H. (1981). Enzymatic reduction of protein-bound methionine sulfoxide. Proc. Natl. Acad. Sci. U. S. A. 78, 2155–2158. doi: 10.1073/pnas.78.4.2155
Choi, J. W., Son, S. M., Mook-Jung, I., Moon, Y. J., Lee, J. Y., Wang, K. C., et al. (2018). Mitochondrial abnormalities related to the dysfunction of circulating endothelial colony-forming cells in moyamoya disease. J. Neurosurg. 129, 1151–1159. doi: 10.3171/2017.5.jns17147
Chumachenko, M. S., Waseem, T. V., and Fedorovich, S. V. (2022). Metabolomics and metabolites in ischemic stroke. Rev. Neurosci. 33, 181–205. doi: 10.1515/revneuro-2021-0048
de Luca, A., Sanna, F., Sallese, M., Ruggiero, C., Grossi, M., Sacchetta, P., et al. (2010). Methionine sulfoxide reductase a down-regulation in human breast cancer cells results in a more aggressive phenotype. Proc. Natl. Acad. Sci. U. S. A. 107, 18628–18633. doi: 10.1073/pnas.1010171107
Deng, X., Ge, P., Wang, S., Zhang, D., Zhang, Y., Wang, R., et al. (2018). Treatment of Moyamoya disease. Neurosurgery 65, 62–65. doi: 10.1093/neuros/nyy114
dos Santos, L. M., da Silva, T. M., Azambuja, J. H., Ramos, P. T., Oliveira, P. S., da Silveira, E. F., et al. (2017). Methionine and methionine sulfoxide treatment induces M1/classical macrophage polarization and modulates oxidative stress and purinergic signaling parameters. Mol. Cell. Biochem. 424, 69–78. doi: 10.1007/s11010-016-2843-6
Fan, H., Li, D., Guan, X., Yang, Y., Yan, J., Shi, J., et al. (2020). <p>MsrA suppresses inflammatory activation of microglia and oxidative stress to prevent demyelination via inhibition of the NOX2-MAPKs/NF-κB signaling pathway</p>. Drug Des. Devel. Ther. 14, 1377–1389. doi: 10.2147/dddt.s223218
Fan, H., Wu, P. F., Zhang, L., Hu, Z. L., Wang, W., Guan, X. L., et al. (2015). Methionine sulfoxide reductase a negatively controls microglia-mediated NeuroinflammationviaInhibiting ROS/MAPKs/NF-κB signaling pathways through a catalytic antioxidant function. Antioxid. Redox Signal. 22, 832–847. doi: 10.1089/ars.2014.6022
Ge, P., Zhang, Q., Ye, X., Liu, X., Deng, X., Wang, J., et al. (2020). Modifiable risk factors associated with Moyamoya disease: a case-control study. Stroke 51, 2472–2479. doi: 10.1161/strokeaha.120.030027
Ge, P., Zhao, Y., Zhai, Y., Zhang, Q., Ye, X., Wang, J., et al. (2022). Circulating choline pathway nutrients and risk of moyamoya disease. Front. Nutr. 9:953426. doi: 10.3389/fnut.2022.953426
Grimaud, R., Ezraty, B., Mitchell, J. K., Lafitte, D., Briand, C., Derrick, P. J., et al. (2001). Repair of oxidized proteins. Identification of a new methionine sulfoxide reductase. J. Biol. Chem. 276, 48915–48920. doi: 10.1074/jbc.M105509200
Gu, S. X., Blokhin, I. O., Wilson, K. M., Dhanesha, N., Doddapattar, P., Grumbach, I. M., et al. (2016). Protein methionine oxidation augments reperfusion injury in acute ischemic stroke. JCI Insight 1. doi: 10.1172/jci.insight.86460
Gu, S. X., Stevens, J. W., and Lentz, S. R. (2015). Regulation of thrombosis and vascular function by protein methionine oxidation. Blood 125, 3851–3859. doi: 10.1182/blood-2015-01-544676
Guidelines for diagnosis and treatment of moyamoya disease (2012). Spontaneous occlusion of the circle of Willis. Neurol. Med. Chir. 52, 245–266. doi: 10.2176/nmc.52.245
Guo, S., Kong, J., Zhou, D., Lai, M., Chen, Y., Xie, D., et al. (2020). Serum metabolic characteristics and biomarkers of early-stage heart failure. Biomark. Med 14, 119–130. doi: 10.2217/bmm-2019-0176
Haenold, R., Wassef, R., Brot, N., Neugebauer, S., Leipold, E., Heinemann, S. H., et al. (2008). Protection of vascular smooth muscle cells by over-expressed methionine sulphoxide reductase a: role of intracellular localization and substrate availability. Free Radic. Res. 42, 978–988. doi: 10.1080/10715760802566541
He, Q., Ge, P., Ye, X., Liu, X., Wang, J., Wang, R., et al. (2022). Hyperhomocysteinemia is a predictor for poor postoperative angiogenesis in adult patients with Moyamoya disease. Front. Neurol. 13:902474. doi: 10.3389/fneur.2022.902474
Holmes, M. V., Millwood, I. Y., Kartsonaki, C., Hill, M. R., Bennett, D. A., Boxall, R., et al. (2018). Lipids, lipoproteins, and metabolites and risk of myocardial infarction and stroke. J. Am. Coll. Cardiol. 71, 620–632. doi: 10.1016/j.jacc.2017.12.006
Jiang, B., and Moskovitz, J. (2018). The functions of the mammalian methionine sulfoxide reductase system and related diseases. Antioxidants 7:122. doi: 10.3390/antiox7090122
Ke, C., Zhu, X., Zhang, Y., and Shen, Y. (2018). Metabolomic characterization of hypertension and dyslipidemia. Metabolomics 14:117. doi: 10.1007/s11306-018-1408-y
Kim, J. S. (2016). Moyamoya disease: epidemiology, clinical features, and diagnosis. J Stroke 18, 2–11. doi: 10.5853/jos.2015.01627
Kim, E. H., Yum, M. S., Ra, Y. S., Park, J. B., Ahn, J. S., Kim, G. H., et al. (2016). Importance of RNF213 polymorphism on clinical features and long-term outcome in moyamoya disease. J. Neurosurg. 124, 1221–1227. doi: 10.3171/2015.4.jns142900
Klutho, P. J., Pennington, S. M., Scott, J. A., Wilson, K. M., Gu, S. X., Doddapattar, P., et al. (2015). Deletion of methionine sulfoxide reductase a does not affect Atherothrombosis but promotes Neointimal hyperplasia and extracellular signal-regulated kinase 1/2 signaling. Arterioscler. Thromb. Vasc. Biol. 35, 2594–2604. doi: 10.1161/atvbaha.115.305857
Kuroda, S., and Houkin, K. (2008). Moyamoya disease: current concepts and future perspectives. Lancet Neurol. 7, 1056–1066. doi: 10.1016/s1474-4422(08)70240-0
Lancellotti, S., de Filippis, V., Pozzi, N., Peyvandi, F., Palla, R., Rocca, B., et al. (2010). Formation of methionine sulfoxide by peroxynitrite at position 1606 of von Willebrand factor inhibits its cleavage by ADAMTS-13: a new prothrombotic mechanism in diseases associated with oxidative stress. Free Radic. Biol. Med. 48, 446–456. doi: 10.1016/j.freeradbiomed.2009.11.020
Lee, B. C., Lee, S. G., Choo, M. K., Kim, J. H., Lee, H. M., Kim, S., et al. (2017). Selenoprotein MsrB1 promotes anti-inflammatory cytokine gene expression in macrophages and controls immune response in vivo. Sci. Rep. 7:5119. doi: 10.1038/s41598-017-05230-2
Lee, B. C., Lee, Y. K., Lee, H. J., Stadtman, E. R., Lee, K. H., and Chung, N. (2005). Cloning and characterization of antioxidant enzyme methionine sulfoxide-S-reductase from Caenorhabditis elegans. Arch. Biochem. Biophys. 434, 275–281. doi: 10.1016/j.abb.2004.11.012
Lei, K. F., Wang, Y. F., Zhu, X. Q., Lu, P. C., Sun, B. S., Jia, H. L., et al. (2007). Identification of MSRA gene on chromosome 8p as a candidate metastasis suppressor for human hepatitis B virus-positive hepatocellular carcinoma. BMC Cancer 7:172. doi: 10.1186/1471-2407-7-172
Li, Q., Qu, M., Wang, N., Wang, L., Fan, G., and Yang, C. (2022). Betaine protects rats against ischemia/reperfusion injury-induced brain damage. J. Neurophysiol. 127, 444–451. doi: 10.1152/jn.00400.2021
Lourenço Dos Santos, S., Petropoulos, I., and Friguet, B. (2018). The oxidized protein repair enzymes methionine sulfoxide reductases and their roles in protecting against oxidative stress, in ageing and in regulating protein function. Antioxidants 7:191. doi: 10.3390/antiox7120191
Ma, X., Deng, W., Liu, X., Li, M., Chen, Z., He, Z., et al. (2011). A genome-wide association study for quantitative traits in schizophrenia in China. Genes Brain Behav. 10, 734–739. doi: 10.1111/j.1601-183X.2011.00712.x
Makukhin, N., Tretyachenko, V., Moskovitz, J., and Míšek, J. (2016). A Ratiometric fluorescent probe for imaging of the activity of methionine sulfoxide reductase a in cells. Angew. Chem. Int. Ed. Engl. 55, 12727–12730. doi: 10.1002/anie.201605833
Miyatake, S., Miyake, N., Touho, H., Nishimura-Tadaki, A., Kondo, Y., Okada, I., et al. (2012). Homozygous c.14576G>a variant of RNF213 predicts early-onset and severe form of moyamoya disease. Neurology 78, 803–810. doi: 10.1212/WNL.0b013e318249f71f
Moskovitz, J., Bar-Noy, S., Williams, W. M., Requena, J., Berlett, B. S., and Stadtman, E. R. (2001). Methionine sulfoxide reductase (MsrA) is a regulator of antioxidant defense and lifespan in mammals. Proc. Natl. Acad. Sci. U. S. A. 98, 12920–12925. doi: 10.1073/pnas.231472998
Moskovitz, J., Flescher, E., Berlett, B. S., Azare, J., Poston, J. M., and Stadtman, E. R. (1998). Overexpression of peptide-methionine sulfoxide reductase in Saccharomyces cerevisiae and human T cells provides them with high resistance to oxidative stress. Proc. Natl. Acad. Sci. U. S. A. 95, 14071–14075. doi: 10.1073/pnas.95.24.14071
Moskovitz, J., Poston, J. M., Berlett, B. S., Nosworthy, N. J., Szczepanowski, R., and Stadtman, E. R. (2000). Identification and characterization of a putative active site for peptide methionine sulfoxide reductase (MsrA) and its substrate stereospecificity. J. Biol. Chem. 275, 14167–14172. doi: 10.1074/jbc.275.19.14167
Moskovitz, J., and Smith, A. (2021). Methionine sulfoxide and the methionine sulfoxide reductase system as modulators of signal transduction pathways: a review. Amino Acids 53, 1011–1020. doi: 10.1007/s00726-021-03020-9
Moskovitz, J., Weissbach, H., and Brot, N. (1996). Cloning the expression of a mammalian gene involved in the reduction of methionine sulfoxide residues in proteins. Proc. Natl. Acad. Sci. U. S. A. 93, 2095–2099. doi: 10.1073/pnas.93.5.2095
Pichler Hefti, J., Sonntag, D., Hefti, U., Risch, L., Schoch, O. D., Turk, A. J., et al. (2013). Oxidative stress in hypobaric hypoxia and influence on vessel-tone modifying mediators. High Alt. Med. Biol. 14, 273–279. doi: 10.1089/ham.2012.1110
Reiterer, M., Schmidt-Kastner, R., and Milton, S. L. (2019). Methionine sulfoxide reductase (Msr) dysfunction in human brain disease. Free Radic. Res. 53, 1144–1154. doi: 10.1080/10715762.2019.1662899
Rose, A. H., and Hoffmann, P. R. (2015). Selenoproteins and cardiovascular stress. Thromb. Haemost. 113, 494–504. doi: 10.1160/th14-07-0603
Shin, T. H., Lee, D. Y., Basith, S., Manavalan, B., Paik, M. J., Rybinnik, I., et al. (2020). Metabolome changes in cerebral ischemia. Cells 9:1630. doi: 10.3390/cells9071630
Wang, Y. P., Li, J. T., Qu, J., Yin, M., and Lei, Q. Y. (2020). Metabolite sensing and signaling in cancer. J. Biol. Chem. 295, 11938–11946. doi: 10.1074/jbc.REV119.007624
Wishart, D. S. (2019). Metabolomics for investigating physiological and pathophysiological processes. Physiol. Rev. 99, 1819–1875. doi: 10.1152/physrev.00035.2018
Yang, Q. J., Zhao, J. R., Hao, J., Li, B., Huo, Y., Han, Y. L., et al. (2018). Serum and urine metabolomics study reveals a distinct diagnostic model for cancer cachexia. J. Cachexia. Sarcopenia Muscle 9, 71–85. doi: 10.1002/jcsm.12246
Zeng, C., Ge, P., Liu, C., Yu, X., Zhai, Y., Liu, W., et al. (2022). Association of circulating branched-chain amino acids with risk of moyamoya disease. Front. Nutr. 9:994286. doi: 10.3389/fnut.2022.994286
Zhang, Q., Liu, Y., Zhang, D., Wang, R., Zhang, Y., Wang, S., et al. (2017). RNF213 as the major susceptibility gene for Chinese patients with moyamoya disease and its clinical relevance. J. Neurosurg. 126, 1106–1113. doi: 10.3171/2016.2.jns152173
Keywords: moyamoya disease, subtypes, methionine sulfoxide, risk factor, oxidative stress
Citation: Li J, Ge P, He Q, Liu C, Zeng C, Tao C, Zhai Y, Wang J, Zhang Q, Wang R, Zhang Y, Zhang D and Zhao J and (2023) Association between methionine sulfoxide and risk of moyamoya disease. Front. Neurosci. 17:1158111. doi: 10.3389/fnins.2023.1158111
Received: 03 February 2023; Accepted: 16 March 2023;
Published: 12 April 2023.
Edited by:
Wen-Jun Tu, Chinese Academy of Medical Sciences and Peking Union Medical College, ChinaReviewed by:
Jonathan Steven Alexander, Louisiana State University Health Shreveport, United StatesCopyright © 2023 Li, Ge, He, Liu, Zeng, Tao, Zhai, Wang, Zhang, Wang, Zhang, Zhang and Zhao. This is an open-access article distributed under the terms of the Creative Commons Attribution License (CC BY). The use, distribution or reproduction in other forums is permitted, provided the original author(s) and the copyright owner(s) are credited and that the original publication in this journal is cited, in accordance with accepted academic practice. No use, distribution or reproduction is permitted which does not comply with these terms.
*Correspondence: Dong Zhang, emhhbmdkb25nMDY2MEBhbGl5dW4uY29t;; Jizong Zhao, emhhb2ppem9uZ0BianR0aC5vcmc=
†These authors have contributed equally to this work
Disclaimer: All claims expressed in this article are solely those of the authors and do not necessarily represent those of their affiliated organizations, or those of the publisher, the editors and the reviewers. Any product that may be evaluated in this article or claim that may be made by its manufacturer is not guaranteed or endorsed by the publisher.
Research integrity at Frontiers
Learn more about the work of our research integrity team to safeguard the quality of each article we publish.