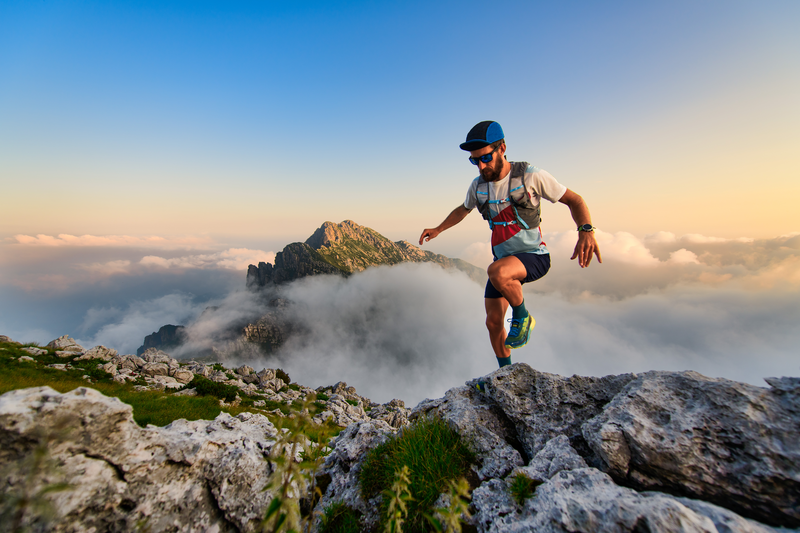
95% of researchers rate our articles as excellent or good
Learn more about the work of our research integrity team to safeguard the quality of each article we publish.
Find out more
REVIEW article
Front. Neurosci. , 22 August 2023
Sec. Translational Neuroscience
Volume 17 - 2023 | https://doi.org/10.3389/fnins.2023.1150868
This article is part of the Research Topic Infection-Triggered Encephalopathy Syndromes as Emerging Pediatric Neuroinflammatory Diseases View all 8 articles
Our goal was to conduct a scoping review of the literature on the treatment of infection-triggered encephalopathy syndrome/acute encephalopathy in children, focusing on treatment targets and treatment initiation timing. We performed literature searches using PubMed for articles reporting treatments of infection-triggered encephalopathy syndrome/acute encephalopathy. We included articles describing specific treatments for acute encephalopathy with control groups. For the purpose of searching new therapies only experimentally tried in the case series, we also included case series studies without control groups in this review, if the studies contained at least two cases with clear treatment goals. Therapies were classified based on their mechanisms of action into brain protection therapy, immunotherapy, and other therapies. We operationally categorized the timing of treatment initiation as T1 (6–12 h), T2 (12–24 h), T3 (24–48 h), and T4 (>48 h) after the onset of seizures and/or impaired consciousness. Thirty articles were included in this review; no randomized control study was found. Eleven retrospective/historical cohort studies and five case–control studies included control groups with or without specific therapies or outcomes. The targeted conditions and treatment timing varied widely across studies. However, the following three points were suggested to be effective in multiple studies: (1) Careful seizure management and targeted temperature management within 12 h (T1) of onset of febrile seizure/prolonged impaired consciousness without multiple organ failure may reduce the development of acute encephalopathy with biphasic seizures and late reduced diffusion; (2) immunotherapy using corticosteroids, tocilizumab, or plasma exchange within 24 h (T1–T2) of onset of acute necrotizing encephalopathy may reduce sequelae; and (3) anakinra therapy and ketogenic diet demonstrate little evidence of neurologic sequelae reduction, but may reduce seizure frequency and allow for weaning from barbiturates, even when administered weeks (T4) after onset in children with febrile infection-related epilepsy syndrome. Although available studies have no solid evidence in the treatment of infection-triggered encephalopathy syndrome/acute encephalopathy, this scoping review lays the groundwork for future prospective clinical trials.
Infection-triggered encephalopathy syndrome (ITES) is characterized by acute encephalopathy (AE). In a national survey in Japan, 34.6% of patients with AE developed mild to severe neurological sequelae (Kasai et al., 2020). AE is a common cause of death in children with ITES. It is defined as syndrome characterized by an acute onset of severe and long-lasting disturbance of consciousness, which typically occurs in previously healthy children (Mizuguchi et al., 2021). In AE, three pathological syndromes interact with each other: excitotoxicity, systemic inflammation/cytokine storm, and metabolic disturbances (Mizuguchi et al., 2007). Excitotoxicity is the main pathological mechanism underlying AE with biphasic seizures and late reduced diffusion (AESD); moreover, systemic inflammation and cytokine storm are associated with acute necrotizing encephalopathy (ANE) and hemorrhagic shock and encephalopathy syndrome (HSES), whereas inflammatory activation in the central nervous system is likely to precede the development of seizures in new-onset refractory status epilepticus (RSE)/febrile infection-related epilepsy syndrome (FIRES) and acute encephalitis with refractory, repetitive partial seizures (AERRPS) (Wickstrom et al., 2022). Specific treatments such as immunotherapy, brain protection treatment, and other treatments have been attempted for AE, taking the abovementioned pathologies into consideration. Moreover, the guidelines for the diagnosis and treatment of AE in childhood recommend the use of corticosteroids, gamma globulin (intravenous immunoglobulin [IVIG]), and cyclosporine for immunotherapy; targeted temperature management (TTM) and osmotic therapy for brain protection; and dextromethorphan, vitamin cocktail therapy, and edaravone for other therapies (Mizuguchi et al., 2021). Real-world surveys have also indicated that these therapies represent treatments of choice (Hayakawa et al., 2020; Murofushi et al., 2022). Making the diagnosis of AE from febrile seizures (FS), especially in patients with AESD, may take several days; hence, the timing and target of each AE treatment modality should be clarified (Yamaguchi et al., 2020; Mizuguchi et al., 2021). Furthermore, the clinical course and final diagnosis can be modified by acute treatment. For example, early intervention for children with febrile status epilepticus may prevent progression to AE (Tokumoto et al., 2022). Notably, interventional studies for AEs vary in terms of the timing of treatment initiation across studies, implying that the purpose of treatment and the implications of treatment efficacy depend not only on the disease condition, but also on the timing of treatment initiation. This poses a challenge for interventional studies. ITES/AE is a rare condition and only a few studies have investigated the efficacy of the abovementioned treatments. Therefore, we conducted a scoping review to systemically map the available research on ITES/AE treatment in children, to determine the extent of the published literature on the efficacy, while focusing on the targeted disease conditions and the timing of treatment initiation.
This scoping review was conducted using the PRISMA Extension for Scoping Reviews (PRISMA-ScR): Checklist and Explanation (Tricco et al., 2018).
The following question was set for this scoping systematic review: What are the effective specific therapies for children with ITES/AE? AE was defined based on the Japanese Society of Child Neurology guidelines for the diagnosis and treatment of AE in childhood (Mizuguchi et al., 2021). The concepts of AE and treatment are shown in Table 1.
We performed literature searches with PubMed for English articles on the treatment of ITES/AE in humans. Searches were performed independently by two authors, HN and MN, using agreed search terms, and the results were combined. To obtain specific AE treatment or management modalities, we searched the following keywords on May 23, 2023: “((“acute encephalopathy” [All fields] OR “acute encephalopathy with biphasic seizures and late reduced diffusion”[All Fields] OR “acute necrotizing encephalopathy”[All fields] OR “Hemorrhagic shock and encephalopathy syndrome”[All fields] OR “acute encephalopathy with febrile convulsive status epilepticus”[All fields] OR “acute encephalitis with refractory repetitive partial seizures”[All fields] OR “Febrile infection-related epilepsy syndrome”[All fields] OR “Reye syndrome”[All fields] OR “Clinically mild encephalitis/encephalopathy with a reversible splenial lesion”[All fields] OR “Acute febrile encephalopathy”[MeSH terms] OR “Hemorrhagic shock and encephalopathy syndrome”[Supplementary concept] OR “Reye syndrome”[MeSH terms]) AND (“treatment”[All fields] OR “medication”[All fields] OR “therapy”[All fields] OR “intervention”[All fields] OR “Targeted temperature management”[All fields] OR “Vitamins”[All fields] OR “Edaravone”[All fields] OR “Cyclosporine”[All fields] OR “Plasmapheresis”[All fields] OR “Anakinra”[All fields] OR “Time to treatment”[MeSH terms] OR “Therapeutics”[MeSH terms] OR “Hypothermia, induced”[MeSH terms] OR “immunoglobulins, intravenous”[MeSH terms] OR “Immunotherapy”[MeSH terms] OR “Mannitol”[MeSH terms] OR “Vitamins”[MeSH terms] OR “Edaravone”[MeSH terms] OR “Cyclosporine”[MeSH terms] OR “Plasmapheresis”[MeSH terms] OR “Tocilizumab”[All fields] OR “Interleukin 1 receptor antagonist protein”[MeSH terms] OR (“dextromethorphan”[MeSH terms] OR “dextromethorphan”[All fields] OR “dextromethorphane”[All fields])) AND ((casereports[Filter] OR clinicalstudy[Filter] OR clinicaltrial[Filter] OR meta-analysis[Filter] OR observationalstudy[Filter] OR randomizedcontrolledtrial[Filter]) AND (humans[Filter]) AND (allchild[Filter])). Searches were also performed manually.
We included articles describing specific treatment modalities for AE with control groups. For the purpose of searching new therapies only experimentally tried in the case series, we also included case series separately from studies with control groups in the review, if they contained two or more cases with clear treatment goals. Eligible articles were identified by performing an initial screen of titles and abstracts, followed by a full review of selected articles. All retrospective and prospective studies that met these criteria were selected.
The following data were extracted from the selected articles: patient demographics, type of study (retrospective cohort study, case control study, and case series), therapies (target, goal, and timing), outcomes, adverse events, and other therapies. When considering specific treatments for AE; moreover, therapies were classified, based on mechanisms of action, into immunotherapy, brain protection therapy, and other therapies. In addition, it is important to clarify the timing and target of each treatment. In this study, we operationally categorized the timing of treatment initiation as T1–T4 (Figure 1): T1 includes the 6–12 h period after the onset of seizures and/or impaired consciousness, when there are no imaging abnormalities, and differentiation between FS and AE is not yet possible. This timing is consistent with the initiation of neuroprotective treatment for most neurological emergencies (Hypothermia after Cardiac Arrest Study Group, 2002; Shankaran et al., 2005; Hutchison et al., 2008; Hemmen et al., 2010; Legriel et al., 2016). If treatment at this stage is successful without imaging or clinical sequelae, the diagnostic criteria for AE are not fulfilled and the goal of treatment in such a study can be said not to treat AE but to prevent AE development. T2 corresponds to 12–24 h after AE onset, whereas T3 represents 24–48 h after AE onset (i.e., when AE was diagnosed by prolonged impaired consciousness longer than 24 h with or without radiological findings). Finally, T4 corresponds to the period after 48 h of AE onset (i.e., when the definitive diagnosis of AESD was established owing to the manifestation of clustered seizures with or without the characteristic magnetic resonance imaging abnormalities) (Takanashi et al., 2009). The aim of treatment at T3 and T4 was to minimize AE-induced neurological sequelae. Herein, we review comparative studies focusing on the mechanisms and the timing of AE treatment.
Figure 1. Schematic diagram of treatment timing for acute encephalopathy. Treatment timing is classified into T1, T2, T3, and T4. T1 is the period from 6 to 12 h after the onset of symptoms such as convulsions and impaired consciousness, when there are no imaging abnormalities and the differentiation between febrile seizures and AE has not yet been established. T2 represents the period from 12 to 24 h after onset, whereas T3 is the 24–48 h period after onset, i.e., when AE is diagnosed by prolonged impaired consciousness longer than 24 h and/or radiological findings. T4 is the period after 48 h of onset, i.e., when late seizures or imaging changes of AESD are observed and subacute phase in children with FIRES/AERRPS. The gray shape next to febrile seizure and acute encephalopathy annotations represents the degree and length of seizure and/or impaired consciousness. The horizontal arrows indicate when treatment and examination should be considered. AERRPS, acute encephalitis with refractory, repetitive partial seizures; AESD, acute encephalopathy with biphasic seizures and late reduced diffusion; ANE, acute necrotizing encephalopathy; CSF, cerebrospinal fluid; DWI, diffusion-weighted imaging; EEG, electroencephalogram; FIRES, febrile infection-related epilepsy syndrome; MRI, magnetic resonance imaging; NCSE, non-convulsive status epilepticus; SRSE, super refractory status epilepticus; TTM, targeted temperature management.
Figure 2 shows a flow diagram depicting the search results. Overall, 477 articles were identified from PubMed search. After screening titles and abstracts, 64 articles were assessed for eligibility. Subsequently, the eligibility criteria were applied to the full text documents, resulting in the identification of 11 articles from the search; moreover, 19 articles were identified manually through reference screening.
Thirty articles were included in the review, none of which was a randomized control study. Eleven retrospective/historical cohort studies and five case–control studies included control groups with or without specific therapies or outcomes (Tables 2, 3). Fourteen studies were case series reports without control groups (Tables 4, 5). Among the 16 comparative studies with control groups, 8 studies were on immunotherapies (5, 1, 1, and 1 studies on corticosteroid, plasma exchange [PE], anakinra, and cyclosporine therapies, respectively), 6 studies were on brain protection therapy (5 and 1 studies on TTM [including therapeutic hypothermia] and barbiturate therapy, respectively), and 2 studies were on other treatments, such as vitamin cocktail treatment for mitochondrial dysfunction. Regarding the 14 case series studies, 3 were on brain protection therapy (2 and 1 studies on TTM and pentobarbital therapy, respectively), 10 were on immunotherapies (4, 2, 2, 1, and 1 studies on corticosteroid, tocilizumab, anakinra, cyclosporine, and PE therapies, respectively), and 4 studies were on other therapies (2, 1, and 1 studies on ketogenic diet [KD], centromedian thalamic nuclei deep brain stimulation [CMN-DBS], and dextromethorphan therapy, respectively). The timing of the treatment varied among studies. Multiple studies suggested that the following three strategies were effective: (1) careful seizure management and TTM within 12 h (T1) of onset of FS or prolonged impaired consciousness without multiple organ failure may reduce AESD incidence (Nishiyama et al., 2015; Murata et al., 2016); (2) immunotherapy using corticosteroids or tocilizumab within 24 h (T1–T2) of ANE onset may reduce sequelae (Okumura et al., 2009; Okajima et al., 2022); and (3) treatments such as anakinra therapy and KD demonstrate little evidence of reducing neurologic sequelae, but may reduce seizure frequency and allow for weaning from barbiturates, even when given weeks after onset in children with FIRES. This scoping review laid the groundwork for future prospective clinical trials.
Table 2. Retrospective cohort studies and case–control studies including control groups (Study characteristics and patient demographics).
Table 3. Retrospective cohort studies and case control studies including control (group therapies, response, outcome, adverse effects therapies for AE/ITES, response, outcome, adverse effects).
Table 4. Case series studies without control groups (pediatric study characteristics and patient demographics).
Since the 1970s, many AE treatment options have been reported in patients with Reye syndrome; however, this scoping review revealed limited evidence for AE treatment efficacy. This review found no randomized control trial evaluating the AE treatment efficacy; only 16 retrospective cohort and case–control studies with control groups as well as 14 case series reports of at least two patients were found. In the present study, we classified the treatment options into brain protection therapy, immunotherapy, and other therapies, based on the mechanism of action of each treatment; moreover, we categorized the timing of treatment initiation after neurological symptom onset as follows: T1 (6–12 h), T2 (12–24 h), T3 (24–48 h), and T4 (>48 h). The timing of treatment initiation, target condition (confirmed diagnoses of AE or high risk of developing AE), and treatment goal (improvement of neurological outcome and freedom from status epilepticus) differed significantly among the studies. We have hereinafter discussed each treatment option based on the mechanism of action, timing of initiation, and target conditions.
Therapeutic hypothermia is theoretically effective for various brain injuries, and its mechanisms of action include reduction of oxygen and nutrient demand, suppression of glutamate and free radical production, suppression of apoptosis, suppression of inflammatory cytokine production, suppression of brain edema, and anticonvulsant effects (Polderman, 2008). A recent study showed no difference between therapeutic hypothermia with TTM at 33°C and a control intervention with TTM at 36°C (Nielsen et al., 2013). To maintain the body temperature at 36°C and avoid hyperthermia is now included in the notion of TTM at normothermia. Two studies have reported AESD incidence reduction and fewer sequelae in patients who underwent TTM within 24 h after onset (T1–T2) compared with patients who did not (Nishiyama et al., 2015; Murata et al., 2016). Nishiyama et al. conducted a retrospective cohort study on TTM at 34.5 ± 0.5°C or 36.0 ± 0.5°C for at least 48 h within 24 h of AE onset (T1–T2) in children at high risk of developing AE-induced neurological sequelae (Nishiyama et al., 2015). In the TTM group, most children were maintained in a hypothermic state until 12 h of onset (T1). They found that 23/23 (100%) and 23/34 (70.6%) of children had good outcomes in the TTM and conventional care groups, respectively (p = 0.004). Eight children with sequelae in the conventional care group fulfilled the diagnostic criteria for AESD, whereas no child without sequelae developed AESD. Murata et al. conducted a historical (retrospective) cohort study on children who underwent TTM at 36.0°C for 12–72 h combined with methylprednisolone (MP) use (TTM/MP) (Murata et al., 2016). For children who developed febrile status epilepticus that was clinically diagnosed as AE, TTM therapy was initiated within 8 h of seizure onset (T1). The conventional treatment group received only MP treatment, and the prognosis at 6 months after onset was good (PCPC = 1) in 10/10 (100%) and 6/10 (60%) patients in the TTM/MP and MP groups, respectively (p = 0.0433). No child (0%) and 4 (40%) children were finally diagnosed as having AESD in the TTM/MP and MP groups, respectively. TTM has a narrow time window; hence, the targeted temperature was reached within 12 h of the onset of impaired consciousness (T1) in many patients in the abovementioned two studies. Kawano et al. (2011) reported that the induction of TTM after 12 h (T2–T4) of AE onset (including final diagnoses of ANE [n = 3/5], HSES [n = 4/1], AE with refractory seizures [n = 11/5], and others [n = 9/5] in patients with hypothermia/normothermia) did not improve outcomes. In a case–control study, Sakata et al. (2020) examined the time to TTM initiation in children having AE with reduced subcortical diffusion (including AESD) with or without neurological sequelae and in those without late seizures. They found that the time from onset of status epilepticus to TTM induction correlated with the outcome at T1, whereas the time from onset of late seizures at T4 to TTM initiation did not correlate with the outcome. Tokumoto et al. (2022) reported the hourly protocol orient management including TTM aiming to start treatment around 6 h after onset (T1) for febrile convulsive status epilepticus reduced neurological sequelae and cases finally diagnosed as AE even though the ratio who underwent TTM were not different. Hoshide et al. (2020) conducted a historical cohort study involving the use of therapeutic hypothermia in children with identified late seizures and a diagnosis of AESD confirmed at T4. They found that the frequency of neurologic sequelae did not differ between the therapeutic hypothermia and conventional treatment groups; nevertheless, the development of post-encephalopathic epilepsy was significantly lower in the therapeutic hypothermia group. Two case series reports have been published on this subject. Lin et al. (2012) reported two cases of patients with FIRES who developed super RSE (SRSE) after admission, which was successfully treated via therapeutic hypothermia at 33°C at 8 h and 12 h after admission (T1). In Boutros et al. (1977) reported a case series of Reye syndrome. Although no specific timing was provided, they performed protocol-based management according to the intracranial pressure (ICP) immediately after admission, including therapeutic hypothermia at 31°C, volume control, and rescue treatment using mannitol. Four of five patients with clinical stage IV Reye syndrome (estimated fatality rate at that time, 50–100%) recovered without sequelae; however, one patient died. In these reports, status epilepticus in the TTM group was assessed using electroencephalographic (EEG) monitoring; moreover, all the reports included patients undergoing immune therapy such as corticosteroid therapy, and hence the impact of such supportive care should be considered. Therefore, although the standard timing for TTM in patients with AE has not yet been established, TTM remains the only effective treatment for AE as reported in multiple comparative studies; furthermore, it seems more appropriate to administer TTM within 12 h of AE onset (T1) to reduce neurological sequelae and AESD development.
Marshall et al. (1978) reported a case series wherein pentobarbital was administered to reduce ICP in seven patients with Reye syndrome, who were classified as stage IV using EEG classification. Phenobarbital was administered when ICP was greater than 30 mmHg for at least 30 min (T1). Pentobarbital administration reduced the amount of mannitol per day required for ICP control from 3.7 ± 0.3 g/kg/day to 0.5 ± 0.2 g/kg/day (p < 0.001). Kuki et al. (2022) conducted a case–control study in patients with HSES in their institution. Univariate analysis comparing between non-death and death groups revealed that barbiturate therapy (85.2% vs. 46.2%; odds ratio [OR], 0.149 [95% confidence interval [CI], 0.033–0.683]; p = 0.014) as well as IVIG (66.7% vs. 30.8%; odds ratio [OR], 0.222 [95% confidence interval [CI], 0.054–0.923]; p = 0.038) and intravenous MP therapies (85.2% vs. 46.2%; odds ratio [OR], 0.149 [95% confidence interval [CI], 0.033–0.683]; p = 0.014) were significantly more applied in the non-death group; however, they did not describe the timing of barbiturate initiation.
Corticosteroid therapy is theoretically effective for AE when the primary pathophysiology is systemic inflammatory response and cytokine storm. Since its recommendation in the Japanese influenza encephalopathy guideline (Morishima, 2006), it has been the most commonly used specific treatment for AE in Japan (Hayakawa et al., 2020). However, only a few comparative studies have investigated the efficacy of corticosteroid therapy. To our knowledge, only our previous study has hitherto compared the use and non-use of corticosteroids in children suspected to be at high risk of inflammation-mediated AE (Ishida et al., 2021). We used corticosteroid pulse therapy in children with aspartate aminotransferase levels >90 U/L within 6 h after AE onset, in whom the suspected pathophysiological mechanism was systemic inflammatory response or cytokine storm, based on our clinical prediction rule (Nagase et al., 2013; Sasaki et al., 2017). In our retrospective cohort study, we investigated the efficacy of corticosteroid pulse therapy within 24 h of AE onset (T1–T2) in children at high risk of cytokine storm-mediated AE (Ishida et al., 2021). The study revealed no difference in the neurological sequelae; good outcome (PCPC = 1–2) was achieved in 5 (38%) and 0 (0%) patients in the corticosteroid and non-corticosteroid pulse groups, respectively. However, there was a trend toward better outcomes in patients who underwent earlier the treatment initiation within several hours of onset (T1) (Ishida et al., 2021). Furthermore, three case–control studies have compared the proportions of corticosteroid-treated children with and without neurological sequelae (Okumura et al., 2009; Hayashi et al., 2012; Zhu et al., 2021). Okumura et al. (2009) conducted a multi-center case–control study on patients with ANE and reported that patients who underwent early corticosteroid therapy (MP pulse or dexamethasone administration) within 24 h (T1–T2) for ANE without brainstem lesions developed fewer moderate or severe sequelae (5 of 12 patients) than those without early corticosteroid therapy (5 of 5 patients) (p = 0.044); nevertheless, the outcome of patients was not correlated with corticosteroid use, corticosteroid pulse therapy, or gammaglobulin use throughout the clinical course at T1–T4. Zhu et al. (2021) conducted a case–control study in children with and without ANE-induced neurological sequelae. They found no difference in the efficacies of corticosteroid pulse therapy and intravenous gammaglobulin use within the periods <24 h (T1–T2) and < 72 h (T1–T4) after onset between groups (Zhu et al., 2021). Hayashi et al. (2012) reported that corticosteroid therapy within 48 h of onset (T1–T3), or during the entire period of late seizures (T1–T4), was not associated with outcome in patients with AESD (Hayashi et al., 2012). In a case–control study of 21 patients with AE associated with E. coli O111 infection, who were classified according to neurological prognosis, Takanashi et al. reported that corticosteroid pulse therapy was associated with prognosis; however, this study was not included in the current analysis because 11 of the 21 participants were adults (Takanashi et al., 2014). Case series studies of corticosteroid therapy without control groups included a study suggesting that patients with ANE who received corticosteroid pulse therapy instead of dexamethasone had fewer sequelae (timing was not indicated) (Seo et al., 2010); a study showing that corticosteroid pulse therapy with IVIG within 24 h of ANE diagnosis (T1–T3) was not associated with mortality (Lee et al., 2014); and a report of two cases of children with ANE treated by intravenous MP administered 5 and 8 h after onset (T1), combined with therapeutic PE and TTM at normothermia, both of whom completely recovered (Okajima et al., 2022). Horino et al. (2021) evaluated the efficacy of corticosteroid use in patients with AEs who underwent intrathecal dexamethasone therapy (IT-DEX) for FIRES. IT-DEX was administered on days 9–59 of FIRES (T4), and all six patients were weaned from anesthesia within a median of 5.5 days after IT-DEX administration. Seizure frequency decreased in 3 (50%) patients after IT-DEX administration. Seizure foci became more localized and background activity improved in all patients. The duration of intensive care unit (ICU) stay was positively correlated with the time from onset to IT-DEX administration (rs = 0.85, p = 0.03) and the duration of ventilation (rs = 0.88, p = 0.03). Therefore, although corticosteroid therapy is theoretically effective for AEs, the disease conditions, disease severity, and timing of treatment that result in clinical efficacy have not been sufficiently clarified. In our studies on the dynamics of inflammatory cytokines in patients with HSES (a cytokine storm-mediated AE) and ICU-treated patients with febrile status epilepticus, inflammatory cytokine levels peaked within 24 h after neurological symptom onset, followed by a gradual decline in cytokine levels (Yamaguchi et al., 2021; Tomioka et al., 2023). Probably, corticosteroids should be administered preferably within 12 h (T1) and at most within 24 h (T1–T2) of onset to improve the outcome of patients with cytokine storm-mediated AEs associated with systemic inflammation, such as ANEs. For conditions such as AERRPS/FIRES, in which cerebral inflammation is a possible pathogenesis, interventions performed later (T4), such as weaning from RSE, may be effective.
IVIG therapy is thought to have an anti-inflammatory action via the suppression of immunocompetent cell activation and inflammatory cytokine production. It is theoretically reasonable to use IVIG in patients with inflammation-associated AE, such as cytokine storm-mediated AE. Thus, IVIG is recommended in AE management guidelines (Mizuguchi et al., 2021) and is the second most used AE treatment after corticosteroid therapy (Hayakawa et al., 2020). However, only one comparative study has demonstrated the efficacy of IVIG. In a case–control study of patients with HSES, Kuki et al. (2022) reported an association of neurological outcome with IVIG use as well as corticosteroid therapy and barbiturate use (non-death group vs. death group, 66.7% vs. 30.8%, respectively; OR, 0.222 [95% CI, 0.054–0.923]; p = 0.038); nevertheless, multivariate analysis showed no significant association. Furthermore, the following three studies have compared outcomes with and without IVIG use in patients with AE: IVIG use for ANE was not associated with outcome (good prognosis in 2 of 5 patients exposed to IVIG use and in 5 of 12 patients without IVIG use) (T1–T4) (Okumura et al., 2009); IVIG use for ANE within 24 h (T1–T2) and within 72 h (T1–T4) were not associated with outcome (Zhu et al., 2021); and IVIG use for AESD within 48 h of onset (T1–T3) (IVIG use: 1 of 18 patients had good outcomes, and 2 of 15 patients had poor outcomes, p = 0.43) or during the entire course (T1–T4) (IVIG use: 3 of 18 patients had good outcomes, and 9 of 15 patients had poor outcomes, p = 0.010) did not effectively improve outcomes (Hayashi et al., 2012).
In a case series study, Lee et al. (2014) demonstrated that IVIG with corticosteroid pulse therapy within 24 h of ANE diagnosis (T1–T3) was not associated with mortality.
PE is also theoretically effective against cytokine storm because of its ability to rapidly remove inflammatory substances from the blood. Li et al. (2021) conducted a retrospective comparative study to investigate the efficacy of PE therapy in patients with ANE. The outcomes of 10 pediatric ICU (PICU)-admitted patients who underwent PE was compared with those of 19 patients without PE. The duration from disturbance of consciousness to the first cycle of PE ranged from 6 h to 78 h, with a median of 32 h (T1–T4), and in 8 (80%) patients consciousness disturbance continued within 24 h (T1–T2) after PICU admission until the first cycle of PE. In the non-PE group, 18/19 children also underwent corticosteroid and IVIG therapy. In-hospital deaths were clearly lower in the PE (0%) than the non-PE (47.4%) group (p = 0.011); however, the mortality rate at the final follow-up was not significantly different between the two groups (33.3 and 58.8% for the PE and non-PE groups, respectively, p = 0.411). The improvement in liver enzymes was more rapid in the PE group. In a case report, two children with ANE were treated using intravenous MP combined with therapeutic PE (which started at 9 h and 16 h (T1–T2) from the onset) and TTM at normothermia, both of whom completely recovered (Okajima et al., 2022).
Recent studies have suggested that neuroinflammation may be involved in the pathogenesis of FIRES/AERRPS (Clarkson et al., 2019). Lai et al. (2020) conducted an international retrospective cohort study of 25 children treated with anakinra, a recombinant interleukin (IL)-1 receptor antagonist, as an immunomodulator for FIRES. Earlier anakinra initiation after seizure onset was associated with shorter durations of mechanical ventilation (r = 0.46, p = 0.03) as well as ICU (r = 0.50, p = 0.01) and hospital length of stay (r = 0.48, p = 0.03). Among children with available seizure frequency data (n = 15), 11 exhibited >50% seizure occurrence reduction at 1 week of anakinra treatment. Although there were no statistically significant differences due to the small sample size, the median interval between seizure onset and anakinra initiation tended to be shorter in children with seizure occurrence reduction (19 days [range, 12–30 days]) than in those without seizure occurrence reduction (27 days [range, 13.5–37.5 days]) (T4). Nevertheless, there was no such tendency in terms of neurological outcomes at discharge (Lai et al., 2020). Shrestha et al. reported a case series of six children with FIRES who underwent anakinra treatment at a median of 11 days of illness (T4). Their seizure burden, which was >10 episodes per day prior to anakinra treatment, reduced to <1–2 episodes per week after anakinra treatment initiation. However, none of the patients returned to their baseline cognitive function, and the median follow-up duration was 40 months (interquartile range, 35–51 months). Of the five patients with serial full-scale intelligence quotient scores, three demonstrated a decline in scores over time (Shrestha et al., 2023). Sa et al. reported two cases of patients with FIRES treated with CMN-DBS and anakinra. In patient 1, CMN-DBS and anakinra treatments were initiated on days 27 and 43 (T4), respectively. In patient 2, CMN-DBS and anakinra treatments were started on days 37 and 22 (T4), respectively. CMN-DBS abolished the occurrence of generalized seizures in both patients. The neurological outcome of patient 1 was good, as the patient was able to attend a mainstream class, whereas patient 2 had a poor outcome, as the patient was in a vegetative state (Sa et al., 2019).
Cyclosporine use can inhibit hypercytokinemia-associated apoptosis and prevent cytotoxicity and tissue damage progressions. Watanabe et al. (2014) reported the efficacy of cyclosporine use in children with AESD without frontal lobe-predominant damage after late seizures (T4). Three of five cyclosporine-treated patients had good prognosis, and two patients had mild sequelae; however, two and three of five non-cyclosporine-treated patients had mild and moderate sequelae, respectively (p = 0.020) (Watanabe et al., 2014). Matsuo et al. reported cases of four patients with AESD treated with cyclosporine and dextromethorphan, an N-methyl-D-aspartate (NMDA) receptor antagonist, after the occurrence of late seizures (T4) (Matsuo et al., 2013). Three patients recovered without sequelae, whereas one patient had mild sequelae. Although the number of patients was small, it is noteworthy that cyclosporine treatment was administered after the occurrence of late seizures (T4) in both studies.
In patients with ANE, brain injury is presumed to result from a cytokine storm; moreover, these patients reportedly have increased blood IL-6 levels in the acute phase (Kawamura et al., 2013). Only two case series reports on the use of tocilizumab, a monoclonal antibody against the IL-6 receptor, for ANE treatment are hitherto available. Koh et al. reported cases of three patients with ANE who received tocilizumab at 18–32 h of onset (T2–T3). Two of the patients recovered without sequelae, and one patient developed mild sequelae (the modified Rankin Scale [mRS] score was 2) (Koh et al., 2019). Hosie et al. reported two ANE cases. The patient in case 1 received IVIG, MP, and tocilizumab at 21, 39, and 53 h of onset (T4 for tocilizumab), respectively. His mRS at discharge was 5 and remained unchanged 2 years later. The patient in case 2 received dexamethasone, MP, and tocilizumab at 1, 21, and 22 h, (T2 for tocilizumab), respectively. Researchers have suggested that the early use of IL-6 blockade as ‘add-on’ immunotherapy within the first 24 h (T1–T2) of onset demonstrates potential for improving the long-term outcome (Hosie et al., 2023).
Energy depletion resulting from decreased mitochondrial function is a potential cause of AE. Omata et al. (2016) conducted a study in which a vitamin cocktail consisting of vitamin B1, vitamin C, biotin, vitamin E, coenzyme Q10, and L-carnitine was administered within 24 h of AE diagnosis (within 48 h of onset, T1–T3) to patients with febrile convulsive status epilepticus. Patients with AE (including AESD and other AEs) who underwent vitamin cocktail treatment within 24 h of diagnosis exhibited better neurological outcomes at hospital discharge than those without vitamin cocktail treatment. Fukui et al. (2019) also reported that patients with AE who received treatment with vitamin B1, vitamin B6, and L-carnitine within 24 h of onset (T1–T2) had improved neurological outcomes (reduced developmental delay and lower incidence of post-encephalopathy epilepsy) compared with those without treatment. Furthermore, fewer patients in the vitamin-treated group were eventually diagnosed with AESD. Vitamin B6 acts as a coenzyme for glutamic acid decarboxylase and is believed to facilitate the conversion of glutamate to gamma-aminobutyric acid, which is a possible mechanism of action for its efficacy.
The KD is an established and effective non-pharmacological treatment for drug-resistant epilepsy. Recently, there has been an increasing number of reports on the efficacy of KD for SRSE. Additionally, the KD has been attempted as a means of seizure control in children with FIRES, a severe form of AE characterized by prolonged RSE triggered by an unknown fever. Nabbout et al. conducted a study involving nine KD-treated patients with FIRES (Nabbout et al., 2010). The KD was initiated between days 4 and 55 (T4), and SRSE was terminated within 2–4 days following the onset of ketonuria. Similarly, Singh et al. reported two cases of RSE wherein FIRES was successfully terminated using the KD, which was initiated on hospitalization days 3 and 13 (Singh et al., 2014). In addition to these studies, several other case series have reported the efficacy of KD therapy for RSE in patients with FIRES; however, these case series are not included in this review as they focused on status epilepticus treatment rather than encephalitis/encephalopathy treatment, and hence are beyond the scope of this review.
A case series report has evaluated the efficacy of dextromethorphan (an NMDA receptor antagonist and sigma-1 receptor agonist for AESD) in four patients with mild consciousness disturbance (Japan Coma Scale score < 30) occurring 24 h after prolonged (>15 min) FS (near-miss AESD) and four patients with AESD. In patients with near-miss AESD, dextromethorphan was started on day 2 or 3 (T3–T4), and no patient developed secondary seizures and AESD. In patients with AESD, dextromethorphan and cyclosporine were started when the secondary seizure was observed on days 4–6 (T4); three of the four patients had normal cognition after 1–3 years of follow-up, whereas one patient showed mild neurological sequelae (hyperactivity) after 3 years. The authors suggested that dextromethorphan and cyclosporine could be effective for the treatment and prevention of AESD (Matsuo et al., 2013). Sa et al. reported two cases of patients with FIRES treated with CMN-DBS and anakinra. CMN-DBS was performed on days 27 and 37 for SRSE control. Generalized seizures were terminated in both patients. One patient had no cognitive sequelae, whereas the other patient had severe sequelae and was in a vegetative state (Sa et al., 2019).
No randomized control trial has evaluated the AE treatment options; only 16 comparative studies with a control group and 14 case series reports with at least two cases are currently available. Disease conditions and the timing of treatment varied widely among the studies. Generally, the studies suggested that the treatments were effective in terms of ameliorating neurologic sequelae when administered within 12–24 h of AE onset. Moreover, treatments often targeted high-risk conditions prior to the diagnosis of AE syndromes. Nevertheless, KD and anakinra therapies may reduce seizure frequency and allow for weaning from barbiturates, even when administered weeks after onset in children with FIRES. This scoping review lays the groundwork for future prospective clinical trials.
HN developed the main concepts and performed the scoping review and wrote the paper. MN performed the scoping review. All the authors reviewed and edited the manuscript and approved the final version.
This study was supported by a Grant-in-Aid for Scientific Research (KAKENHI) from the Ministry of Education, Culture, Sports, Science, and Technology of Japan (Subject ID: 21K09047 to HN) and a Grant-in-Aid for Research on Measures for Intractable Diseases (21FC1005) from the Ministry of Health, Labor, and Welfare, Japan.
The authors declare that the research was conducted in the absence of any commercial or financial relationships that could be construed as a potential conflict of interest.
All claims expressed in this article are solely those of the authors and do not necessarily represent those of their affiliated organizations, or those of the publisher, the editors and the reviewers. Any product that may be evaluated in this article, or claim that may be made by its manufacturer, is not guaranteed or endorsed by the publisher.
Boutros, A., Hoyt, J., Menezes, A., and Bell, W. (1977). Management of Reye's syndrome. A rational approach to a complex problem. Crit. Care Med. 5, 234–238. doi: 10.1097/00003246-197709000-00005
Clarkson, B. D. S., LaFrance-Corey, R. G., Kahoud, R. J., Farias-Moeller, R., Payne, E. T., and Howe, C. L. (2019). Functional deficiency in endogenous interleukin-1 receptor antagonist in patients with febrile infection-related epilepsy syndrome. Ann. Neurol. 85, 526–537. doi: 10.1002/ana.25439
Fukui, K. O., Kubota, M., Terashima, H., Ishiguro, A., and Kashii, H. (2019). Early administration of vitamins B1 and B6 and l-carnitine prevents a second attack of acute encephalopathy with biphasic seizures and late reduced diffusion: a case control study. Brain Dev. 41, 618–624. doi: 10.1016/j.braindev.2019.02.015
Hayakawa, I., Okubo, Y., Nariai, H., Michihata, N., Matsui, H., Fushimi, K., et al. (2020). Recent treatment patterns and variations for pediatric acute encephalopathy in Japan. Brain Dev. 42, 48–55. doi: 10.1016/j.braindev.2019.08.007
Hayashi, N., Okumura, A., Kubota, T., Tsuji, T., Kidokoro, H., Fukasawa, T., et al. (2012). Prognostic factors in acute encephalopathy with reduced subcortical diffusion. Brain Dev. 34, 632–639. doi: 10.1016/j.braindev.2011.11.007
Hemmen, T. M., Raman, R., Guluma, K. Z., Meyer, B. C., Gomes, J. A., Cruz-Flores, S., et al. (2010). Intravenous thrombolysis plus hypothermia for acute treatment of ischemic stroke (ICTuS-L): final results. Stroke 41, 2265–2270. doi: 10.1161/STROKEAHA.110.592295
Horino, A., Kuki, I., Inoue, T., Nukui, M., Okazaki, S., Kawawaki, H., et al. (2021). Intrathecal dexamethasone therapy for febrile infection-related epilepsy syndrome. Ann. Clin. Transl. Neurol. 8, 645–655. doi: 10.1002/acn3.51308
Hoshide, M., Yasudo, H., Inoue, H., Matsushige, T., Sakakibara, A., Nawata, Y., et al. (2020). Efficacy of hypothermia therapy in patients with acute encephalopathy with biphasic seizures and late reduced diffusion. Brain Dev. 42, 515–522. doi: 10.1016/j.braindev.2020.03.007
Hosie, P. H., Lim, C., Scott, T. R. D., Cardamone, M., Farrar, M. A., Frith, C., et al. (2023). Treatment of severe acute necrotizing encephalopathy of childhood with interleukin-6 receptor blockade in the first 24 h as add-on immunotherapy shows favorable long-term outcome at 2 years. Brain Dev. 45, 401–407. doi: 10.1016/j.braindev.2023.03.002
Hutchison, J. S., Ward, R. E., Lacroix, J., Hebert, P. C., Barnes, M. A., Bohn, D. J., et al. (2008). Hypothermia therapy after traumatic brain injury in children. N. Engl. J. Med. 358, 2447–2456. doi: 10.1056/NEJMoa0706930
Hypothermia after Cardiac Arrest Study Group (2002). Mild therapeutic hypothermia to improve the neurologic outcome after cardiac arrest. N. Engl. J. Med. 346, 549–556. doi: 10.1056/NEJMoa012689
Ishida, Y., Nishiyama, M., Yamaguchi, H., Tomioka, K., Takeda, H., Tokumoto, S., et al. (2021). Early steroid pulse therapy for children with suspected acute encephalopathy: an observational study. Medicine 100:e26660. doi: 10.1097/MD.0000000000026660
Kasai, M., Shibata, A., Hoshino, A., Maegaki, Y., Yamanouchi, H., Takanashi, J. I., et al. (2020). Epidemiological changes of acute encephalopathy in Japan based on national surveillance for 2014–2017. Brain Dev. 42, 508–514. doi: 10.1016/j.braindev.2020.04.006
Kawamura, Y., Nakai, H., Sugata, K., Asano, Y., and Yoshikawa, T. (2013). Serum biomarker kinetics with three different courses of HHV-6B encephalitis. Brain Dev. 35, 590–595. doi: 10.1016/j.braindev.2012.08.005
Kawano, G., Iwata, O., Iwata, S., Kawano, K., Obu, K., Kuki, I., et al. (2011). Determinants of outcomes following acute child encephalopathy and encephalitis: pivotal effect of early and delayed cooling. Arch. Dis. Child. 96, 936–941. doi: 10.1136/adc.2009.180554
Koh, J. C., Murugasu, A., Krishnappa, J., and Thomas, T. (2019). Favorable outcomes with early interleukin 6 receptor blockade in severe acute necrotizing encephalopathy of childhood. Pediatr. Neurol. 98, 80–84. doi: 10.1016/j.pediatrneurol.2019.04.009
Kuki, I., Inoue, T., Nukui, M., Okazaki, S., Kawawaki, H., Ishikawa, J., et al. (2022). Effect of higher body temperature and acute brain edema on mortality in hemorrhagic shock and encephalopathy syndrome. J. Neurol. Sci. 439:120321. doi: 10.1016/j.jns.2022.120321
Lai, Y. C., Muscal, E., Wells, E., Shukla, N., Eschbach, K., Hyeong Lee, K., et al. (2020). Anakinra usage in febrile infection related epilepsy syndrome: an international cohort. Ann. Clin. Transl. Neurol. 7, 2467–2474. doi: 10.1002/acn3.51229
Lee, C. G., Kim, J. H., Lee, M., and Lee, J. (2014). Clinical outcome of acute necrotizing encephalopathy in related to involving the brain stem of single institution in Korea. Korean J. Pediatr. 57, 264–270. doi: 10.3345/kjp.2014.57.6.264
Legriel, S., Lemiale, V., Schenck, M., Chelly, J., Laurent, V., Daviaud, F., et al. (2016). Hypothermia for neuroprotection in convulsive status epilepticus. N. Engl. J. Med. 375, 2457–2467. doi: 10.1056/NEJMoa1608193
Li, K., Zhang, T., Liu, G., Jin, P., Wang, Y., Wang, L., et al. (2021). Plasma exchange therapy for acute necrotizing encephalopathy of childhood. Pediatr. Investig. 5, 99–105. doi: 10.1002/ped4.12280
Lin, J. J., Lin, K. L., Hsia, S. H., and Wang, H. S., Group, C.S (2012). Therapeutic hypothermia for febrile infection-related epilepsy syndrome in two patients. Pediatr. Neurol. 47, 448–450. doi: 10.1016/j.pediatrneurol.2012.08.013
Marshall, L. F., Shapiro, H. M., Rauscher, A., and Kaufman, N. M. (1978). Pentobarbital therapy for intracranial hypertension in metabolic coma. Reye's syndrome. Crit. Care Med. 6, 1–5. doi: 10.1097/00003246-197801000-00001
Matsuo, M., Maeda, T., Ono, N., Sugihara, S., Kobayashi, I., Koga, D., et al. (2013). Efficacy of dextromethorphan and cyclosporine a for acute encephalopathy. Pediatr. Neurol. 48, 200–205. doi: 10.1016/j.pediatrneurol.2012.11.003
Mizuguchi, M., Ichiyama, T., Imataka, G., Okumura, A., Goto, T., Sakuma, H., et al. (2021). Guidelines for the diagnosis and treatment of acute encephalopathy in childhood. Brain Dev. 43, 2–31. doi: 10.1016/j.braindev.2020.08.001
Mizuguchi, M., Yamanouchi, H., Ichiyama, T., and Shiomi, M. (2007). Acute encephalopathy associated with influenza and other viral infections. Acta Neurol. Scand. 115, 45–56. doi: 10.1111/j.1600-0404.2007.00809.x
Morishima, T. (2006). Guideline for the management of influenza associated encephalopathy. Nihon Rinsho 64, 1897–1905.
Murata, S., Kashiwagi, M., Tanabe, T., Oba, C., Shigehara, S., Yamazaki, S., et al. (2016). Targeted temperature management for acute encephalopathy in a Japanese secondary emergency medical care hospital. Brain Dev. 38, 317–323. doi: 10.1016/j.braindev.2015.09.003
Murofushi, Y., Sakuma, H., Tada, H., Mizuguchi, M., and Takanashi, J. I. (2022). Changes in the treatment of pediatric acute encephalopathy in Japan between 2015 and 2021: a national questionnaire-based survey. Brain Dev. 45, 153–160. doi: 10.1016/j.braindev.2022.10.008
Nabbout, R., Mazzuca, M., Hubert, P., Peudennier, S., Allaire, C., Flurin, V., et al. (2010). Efficacy of ketogenic diet in severe refractory status epilepticus initiating fever induced refractory epileptic encephalopathy in school age children (FIRES). Epilepsia 51, 2033–2037. doi: 10.1111/j.1528-1167.2010.02703.x
Nagase, H., Nakagawa, T., Aoki, K., Fujita, K., Saji, Y., Maruyama, A., et al. (2013). Therapeutic indicators of acute encephalopathy in patients with complex febrile seizures. Pediatr. Int. 55, 310–314. doi: 10.1111/ped.12046
Nielsen, N., Wetterslev, J., Cronberg, T., Erlinge, D., Gasche, Y., Hassager, C., et al. (2013). Targeted temperature management at 33 degrees C versus 36 degrees C after cardiac arrest. N. Engl. J. Med. 369, 2197–2206. doi: 10.1056/NEJMoa1310519
Nishiyama, M., Tanaka, T., Fujita, K., Maruyama, A., and Nagase, H. (2015). Targeted temperature management of acute encephalopathy without AST elevation. Brain Dev. 37, 328–333. doi: 10.1016/j.braindev.2014.06.005
Okajima, K., Hayakawa, I., Tsuboi, N., Shimura, K., Ishiguro, A., and Abe, Y. (2022). Early therapeutic plasma exchange may lead to complete neurological recovery in moderate to severe influenza-associated acute necrotizing encephalopathy. Brain Dev. 44, 492–497. doi: 10.1016/j.braindev.2022.03.004
Okumura, A., Mizuguchi, M., Kidokoro, H., Tanaka, M., Abe, S., Hosoya, M., et al. (2009). Outcome of acute necrotizing encephalopathy in relation to treatment with corticosteroids and gammaglobulin. Brain Dev. 31, 221–227. doi: 10.1016/j.braindev.2008.03.005
Omata, T., Fujii, K., Takanashi, J., Murayama, K., Takayanagi, M., Muta, K., et al. (2016). Drugs indicated for mitochondrial dysfunction as treatments for acute encephalopathy with onset of febrile convulsive status epileptics. J. Neurol. Sci. 360, 57–60. doi: 10.1016/j.jns.2015.11.043
Polderman, K. H. (2008). Induced hypothermia and fever control for prevention and treatment of neurological injuries. Lancet 371, 1955–1969. doi: 10.1016/s0140-6736(08)60837-5
Sa, M., Singh, R., Pujar, S., D'Arco, F., Desai, N., Eltze, C., et al. (2019). Centromedian thalamic nuclei deep brain stimulation and Anakinra treatment for FIRES - two different outcomes. Eur. J. Paediatr. Neurol. 23, 749–754. doi: 10.1016/j.ejpn.2019.08.001
Sakata, K., Kawano, G., Suda, M., Yokochi, T., Yae, Y., Imagi, T., et al. (2020). Determinants of outcomes for acute encephalopathy with reduced subcortical diffusion. Sci. Rep. 10:9134. doi: 10.1038/s41598-020-66167-7
Sasaki, K., Nagase, H., Maruyama, A., Fujita, K., Nishiyama, M., Tanaka, T., et al. (2017). Clinical prediction rule for neurological sequelae due to acute encephalopathy: a medical community-based validation study in Harima Japan. BMJ Open 7:e016675. doi: 10.1136/bmjopen-2017-016675
Seo, H. E., Hwang, S. K., Choe, B. H., Cho, M. H., Park, S. P., and Kwon, S. (2010). Clinical spectrum and prognostic factors of acute necrotizing encephalopathy in children. J. Korean Med. Sci. 25, 449–453. doi: 10.3346/jkms.2010.25.3.449
Shankaran, S., Laptook, A. R., Ehrenkranz, R. A., Tyson, J. E., McDonald, S. A., Donovan, E. F., et al. (2005). Whole-body hypothermia for neonates with hypoxic-ischemic encephalopathy. N. Engl. J. Med. 353, 1574–1584. doi: 10.1056/NEJMcps050929
Shrestha, A., Wood, E. L., Berrios-Siervo, G., Stredny, C. M., Boyer, K., Vega, C., et al. (2023). Long-term neuropsychological outcomes in children with febrile infection-related epilepsy syndrome (FIRES) treated with anakinra. Front. Neurol. 14:1100551. doi: 10.3389/fneur.2023.1100551
Singh, R. K., Joshi, S. M., Potter, D. M., Leber, S. M., Carlson, M. D., and Shellhaas, R. A. (2014). Cognitive outcomes in febrile infection-related epilepsy syndrome treated with the ketogenic diet. Pediatrics 134, e1431–e1435. doi: 10.1542/peds.2013-3106
Takanashi, J., Tada, H., Terada, H., and Barkovich, A. J. (2009). Excitotoxicity in acute encephalopathy with biphasic seizures and late reduced diffusion. AJNR Am. J. Neuroradiol. 30, 132–135. doi: 10.3174/ajnr.A1247
Takanashi, J., Taneichi, H., Misaki, T., Yahata, Y., Okumura, A., Ishida, Y., et al. (2014). Clinical and radiologic features of encephalopathy during 2011 E coli O111 outbreak in Japan. Neurology 82, 564–572. doi: 10.1212/WNL.0000000000000120
Tokumoto, S., Nishiyama, M., Yamaguchi, H., Tomioka, K., Ishida, Y., Toyoshima, D., et al. (2022). Prognostic effects of treatment protocols for febrile convulsive status epilepticus in children. BMC Neurol. 22:77. doi: 10.1186/s12883-022-02608-2
Tomioka, K., Nishiyama, M., Tokumoto, S., Yamaguchi, H., Aoki, K., Seino, Y., et al. (2023). Time course of serum cytokine level changes within 72 h after onset in children with acute encephalopathy and febrile seizures. BMC Neurol. 23:7. doi: 10.1186/s12883-022-03048-8
Tricco, A. C., Lillie, E., Zarin, W., O'Brien, K. K., Colquhoun, H., Levac, D., et al. (2018). PRISMA extension for scoping reviews (PRISMA-ScR): checklist and explanation. Ann. Intern. Med. 169, 467–473. doi: 10.7326/M18-0850
Watanabe, Y., Motoi, H., Oyama, Y., Ichikawa, K., Takeshita, S., Mori, M., et al. (2014). Cyclosporine for acute encephalopathy with biphasic seizures and late reduced diffusion. Pediatr. Int. 56, 577–582. doi: 10.1111/ped.12288
Wickstrom, R., Taraschenko, O., Dilena, R., Payne, E. T., Specchio, N., Nabbout, R., et al. (2022). International consensus recommendations for management of new onset refractory status epilepticus (NORSE) including febrile infection-related epilepsy syndrome (FIRES): summary and clinical tools. Epilepsia 63, 2827–2839. doi: 10.1111/epi.17391
Yamaguchi, H., Nishiyama, M., Tokumoto, S., Ishida, Y., Tomioka, K., Aoki, K., et al. (2020). Detailed characteristics of acute encephalopathy with biphasic seizures and late reduced diffusion: 18-year data of a single-center consecutive cohort. J. Neurol. Sci. 411:116684. doi: 10.1016/j.jns.2020.116684
Yamaguchi, H., Nishiyama, M., Tokumoto, S., Ishida, Y., Tomioka, K., Aoki, K., et al. (2021). Elevated cytokine, chemokine, and growth and differentiation factor-15 levels in hemorrhagic shock and encephalopathy syndrome: a retrospective observational study. Cytokine 137:155324. doi: 10.1016/j.cyto.2020.155324
Keywords: acute encephalopathy, febrile seizure, status epilepticus, treatment, children
Citation: Nagase H, Yamaguchi H, Tokumoto S, Ishida Y, Tomioka K, Nishiyama M, Nozu K and Maruyama A (2023) Timing of therapeutic interventions against infection-triggered encephalopathy syndrome: a scoping review of the pediatric literature. Front. Neurosci. 17:1150868. doi: 10.3389/fnins.2023.1150868
Received: 25 January 2023; Accepted: 07 August 2023;
Published: 22 August 2023.
Edited by:
Russell Dale, The Children’s Hospital at Westmead, AustraliaReviewed by:
Ceren Günbey, Hacettepe University Hospitals, TürkiyeCopyright © 2023 Nagase, Yamaguchi, Tokumoto, Ishida, Tomioka, Nishiyama, Nozu and Maruyama. This is an open-access article distributed under the terms of the Creative Commons Attribution License (CC BY). The use, distribution or reproduction in other forums is permitted, provided the original author(s) and the copyright owner(s) are credited and that the original publication in this journal is cited, in accordance with accepted academic practice. No use, distribution or reproduction is permitted which does not comply with these terms.
*Correspondence: Hiroaki Nagase, bmFnYXNlQG1lZC5rb2JlLXUuYWMuanA=
Disclaimer: All claims expressed in this article are solely those of the authors and do not necessarily represent those of their affiliated organizations, or those of the publisher, the editors and the reviewers. Any product that may be evaluated in this article or claim that may be made by its manufacturer is not guaranteed or endorsed by the publisher.
Research integrity at Frontiers
Learn more about the work of our research integrity team to safeguard the quality of each article we publish.