- 1Institute of Pharmacology, Toxicology, and Pharmacy, Ludwig Maximilian University of Munich, Munich, Germany
- 2Hannover Medical School, Institute for Laboratory Animal Science, Hanover, Germany
- 3Office for Animal Welfare and 3Rs, University of Zurich, Zurich, Switzerland
Experimental craniotomies are a common surgical procedure in neuroscience. Because inadequate analgesia appears to be a problem in animal-based research, we conducted this review and collected information on management of craniotomy-associated pain in laboratory mice and rats. A comprehensive search and screening resulted in the identification of 2235 studies, published in 2009 and 2019, describing craniotomy in mice and/or rats. While key features were extracted from all studies, detailed information was extracted from a random subset of 100 studies/year. Reporting of perioperative analgesia increased from 2009 to 2019. However, the majority of studies from both years did not report pharmacologic pain management. Moreover, reporting of multimodal treatments remained at a low level, and monotherapeutic approaches were more common. Among drug groups, reporting of pre- and postoperative administration of non-steroidal anti-inflammatory drugs, opioids, and local anesthetics in 2019 exceeded that of 2009. In summary, these results suggest that inadequate analgesia and oligoanalgesia are persistent issues associated with experimental intracranial surgery. This underscores the need for intensified training of those working with laboratory rodents subjected to craniotomies.
Systematic review registration: https://osf.io/7d4qe.
1. Introduction
Lack of analgesia and oligoanalgesia (failure to provide adequate analgesia in patients) remains a persistent problem in animal-based research (Richardson and Flecknell, 2005; Stokes et al., 2009; Jirkof, 2017; Flecknell, 2018; Foley et al., 2019). While the type and severity of pain can be anticipated in the context of surgical interventions in experimental animals, scientists often refrain from the application of an adequate analgesia regimen because of concerns about an effect of analgesic drugs on readout parameters (Jirkof, 2017; Peterson et al., 2017; Jirkof and Potschka, 2021). However, it needs to be considered that there is a multitude of analgesic compounds from different drug classes, providing the option to carefully choose the regimen based on the expected interfering effects (Vadivelu et al., 2016; Jirkof, 2017; Jirkof and Potschka, 2021). Moreover, study design can be adjusted to prevent interference, for example with an extension of recovery phases or the inclusion of respective control groups (Jirkof, 2017; Moser, 2020; Jirkof and Potschka, 2021). Most importantly, it should be kept in mind that uncontrolled or insufficiently controlled pain itself, with all its physiological consequences, can have a major impact on research parameters, thereby limiting study quality and rigor of research data (Jirkof, 2017; Peterson et al., 2017; Flecknell, 2018; Jirkof and Potschka, 2021). Thus, with lack of adequate analgesia and oligoanalgesia scientists on one hand violate the 3R principle, which calls for refinement, i.e., a minimization of suffering and pain. On the other hand, they also risk poor data quality, which in turn might affect subsequent planning of animal-based studies.
In neuroscientific research, mice and rats represent the most widely used species. Intracranial implants are often required for recordings, stimulations, or local administration procedures. In this context, reports about undertreatment of pain associated with craniotomy in human patients raise concerns about respective pain management in laboratory rodents with central nervous system (CNS) implants (Dunn et al., 2016; Lutman et al., 2018; Bello et al., 2022). In human patients, oligoanalgesia for neurosurgical procedures is related to concerns about an impact of analgesic drugs on hemorrhage risks and intracranial pressure (Basali et al., 2000; Vadivelu et al., 2016; Bello et al., 2022). Different reviews have discussed to what extent these concerns are justified and how one can optimize the analgesic regimen without an increased risk of postsurgical complications (Dunn et al., 2016; Lutman et al., 2018; Bello et al., 2022). A systematic review from 2017 revealed that there is a significant divergence in studies conducted to explore the efficacy of different regimens, concluding that it is difficult to provide recommendations except for the application of a regional scalp block with local anesthetics (Tsaousi et al., 2017). It has been emphasized that undertreatment of pain in the context of craniotomy procedures can result in prolonged postsurgical pain including headache (Dunn et al., 2016; Lutman et al., 2018; Thapa and Euasobhon, 2018), a risk that has been previously underestimated (Kaur et al., 2000; Gottschalk et al., 2007). Experts of the American College of Laboratory Animal Medicine (ACLAM) Task Force have stated that intracerebral electrode implantation in laboratory rodents is associated with minimal to mild pain only (Kohn et al., 2007). Considering the experience from human patients, this expert opinion might have underestimated the risk of postsurgical pain associated with intracranial procedures.
Stokes et al. (2009) analyzed the literature of analgesic and anesthetic procedures in rodents undergoing surgical interventions. They used a structured multiphase search approach and included 172 papers. While they found improvements from 2000–2001 to 2005–2006, their findings suggest that less than 25% of laboratory rodents receive analgesic drugs in the perioperative phase (Stokes et al., 2009). Since then, several authors and groups have emphasized the need for adequate analgesia in laboratory rodents, have discussed the negative implications of oligoanalgesia for animal welfare and study quality, and have provided guidelines and recommendations for choice of analgesic regimen for different experimental procedures in mice and rats (Gargiulo et al., 2012; Jirkof et al., 2013; Carbone and Austin, 2016; Jirkof, 2017; Flecknell, 2018; Cho et al., 2019; Foley et al., 2019; Jirkof and Potschka, 2021). We hypothesized that these publications and recommendations contributed to an improvement of perioperative pain management in experimental animals and tested this hypothesis with a systematic scoping review. Perioperative anesthetic and analgesic management must be planned as a whole, and the analgesic regimen should take the anesthesia into account. Thus, we decided to assess the complete anesthetic and perioperative pain management approaches in mice and rats undergoing craniotomy described in literature. To allow for completion of our analyses in a reasonable time span, we used a partial literature sample from the years 2009 and 2019. These years allow for analyses of the development during the decade following the report by Stokes et al. (2009).
2. Materials and methods
2.1. Protocol registration
The study protocol was published on the Open Science Framework database (doi: 10.17605/OSF.IO/G5F6K)1 on December 3rd, 2020, prior to starting the screening process. The SYRCLE (Systematic Review Centre for Laboratory animal Experimentation’s) protocol template was used to create the study protocol (de Vries, 2015). The study protocol can also be found in Supplementary Methods 1. This publication follows the PRISMA (Preferred Reporting Items for Systematic Reviews and Meta-Analyses) guidelines; the checklist, including the extension for scoping reviews can be found in Supplementary Methods 2. The review question was: What are the common approaches to analgesic and anesthetic management for experimental craniotomy in mice and rats?
2.2. Data source and search strategy
A comprehensive search string was constructed for PubMed and used to identify relevant studies (M.R. under supervision from H.P. and C.L.). The search string consisted of two main elements, the investigated population, mice and rats, and the investigated procedure, craniotomy. The search string was constructed to include relevant plural terms, alternative spellings and relevant synonyms for terms, combined with “OR” for each element. The elements population and procedure were combined with “AND.” The final search string and a full overview of all included terms can be found in Supplementary Methods 3.
The final PubMed search was performed on October 19th, 2020. All retrieved studies were saved in a bibliographic reference manager (EndNote™ X9.3.3). The review focused on the years 2009 and 2019, with the latter selected as the most recent completed year prior to the search. The year 2009 was selected so that differences and changes in the approach could be assessed over one decade. The retrieved references from these years were uploaded to Rayyan, an online tool supporting systematic reference screening (Ouzzani et al., 2016).
2.3. Study selection
Study screening was performed in two separate phases: first screening of titles and abstracts, then screening of full texts. Inclusion and exclusion criteria were predefined in the study protocol and are listed in Table 1. Both phases were completed by two independent reviewers. Before screening, reviewers were trained with SYRCLE’s e-learning for preclinical systematic reviews and by completing a pre-screened training set of 50 abstracts, of which 95% had to be screened according to requirements.
For title and abstract screening, H.K. screened all studies, while the reference set was divided among six reviewers as the second screener (K.A., M.R., M.B., A.G., H.S., P.J.). During title and abstract screening, we included studies published in English language describing craniotomy and/or any type of surgery or other procedure indicating craniotomy in mice and/or rats. For full-text screening, H.K. screened all studies, while the reference set was divided among eight scientists (N.M., H.S., K.A., P.J., M.B., A.G., L.S. and K.S.). During full text screening, we included primary in vivo studies, published in English, and describing craniotomy in mice and/or rats. For both screening phases, discrepancies were discussed and solved without the need for a third reviewer.
2.4. Data extraction
Data extraction was carried out in two phases. First, comprehensive data were extracted from a random subset of 200 out of 2235 references (Supplementary Tables 1, 2). Studies were initially sorted by year and then by alphabet (for first author) before import to Excel. Using a randomization tool,2 a sequence was generated with the length corresponding to the number of included studies from each year. The first 100 numbers from each sequence determined the random sample. Study characteristics to be extracted (Table 2) were predefined in the study protocol. Prior to data extraction, a test extraction of ten studies per year was performed, which served as the basis for the extensions and adjustment of the features to be extracted (H.K. with support of C.L. and H.P). Data were extracted from included references in alphabetical (for first author) order from text and graph, alternating between 2009 and 2019 to prevent confounding by extractor learning effects. The final extraction sheet can be found in Supplementary Tables 1, 2. Relevant information from referencing to another publication were tracked and included for one level i.e., if a referenced publication referenced yet another publication the latter was not retrieved. If information was not provided in the original or directly referenced publication, it was recorded as not reported.
During data extraction, we identified a study that was erroneously included during screening (it had no craniotomy, only subcutaneous electroencephalography electrodes were implanted) (Elmorsy et al., 2019). This study was subsequently excluded, and the next number from the random number sequence of the respective year (the 101st) was substituted.
The second part of data extraction was restricted to a smaller set of key study characteristics, which was extracted from all included studies (2235 studies) (Supplementary Table 3). This second phase was later added to our original plans and has not been described in our protocol. The characteristics extracted can be found in Table 3. Data were extracted from text and graphs and recorded in the Excel spreadsheet. Relevant information from referencing to another publication was again tracked, as described above.
Extracted data from a random subset of 5% of all included studies were quality checked by a second reviewer; ten studies for the first part by A.G., 112 for the second part by L.S. The random subsets for quality checking were again generated using random.org.
2.5. Data synthesis and analysis
The unit of analysis for this review was the reported analgesic and anesthetic treatment per paper; if a paper described different analgesic or anesthetic procedures for different groups of animals, these were separately included in our analyses. For our review, we separated analgesic-antipyretic agents into analgesic-antipyretic agents with and without an anti-inflammatory effect. In the following, we refer to analgesic-antipyretic agents with an anti-inflammatory effect as non-steroidal anti-inflammatory drugs (NSAIDs) and to analgesic-antipyretic agents without a relevant anti-inflammatory effect as antipyretic analgesics.
Extracted data were tabulated using Excel and Word, and evaluated for the descriptive overview in the section “3. Results.” Data were quantitatively analyzed and plotted using Excel’s Pivot table tool. Graphs and figures were created using Excel and PowerPoint.
Differences between the years were analyzed using Fisher’s exact test and Chi-square test. Two-sided testing, with confidence intervals of 95%, was performed using GraphPad PRISM (GraphPad Software version 5.04, San Diego, USA). Complete information on all calculations performed can be found in Supplementary Methods 4.
3. Results
3.1. Identification of relevant references
Our search identified a total of 65,507 references. After removing all references that were not published in the years of 2009 or 2019 (k = 61314), a total of 4,193 references remained for screening. Following title and abstract screening, 2,925 references remained that were subjected to full-text screening. In the end, full-text screening resulted in 2,234 relevant references. Information about key study characteristics was extracted from all these included references; 911 from 2009 and 1323 from 2019 (which described 2,247 treatment regimens). As per protocol, more in depth data extraction was restricted to a random subset of 200 references (100 per year). An overview of the study flow can be found in Figure 1. A list of all included references and extracted parameters can be found in Supplementary Tables 1–3.
3.2. Key characteristics from all 2235 references
3.2.1. General study characteristics
In total, 911 references were published in 2009, and 1,324 references were published in 2019, which were included in our review. Seven of the 2019 references described multiple experimental groups in which animals received different compounds for pain management, or one group received a compound, and the other group did not. These groups were separately included in our analyses.
3.2.2. Analgesia in 2009 and 2019 – key characteristics
The perioperative use of analgesics or local anesthetics was reported in 111 of 911 studies from 2009 and in 335 of 1,333 studies from 2019 (Figures 2A, B). Perioperative administration of analgesics or local anesthetics was more frequently reported in 2019 than 2009 (X2 (1) = 56.15, p < 0.001).
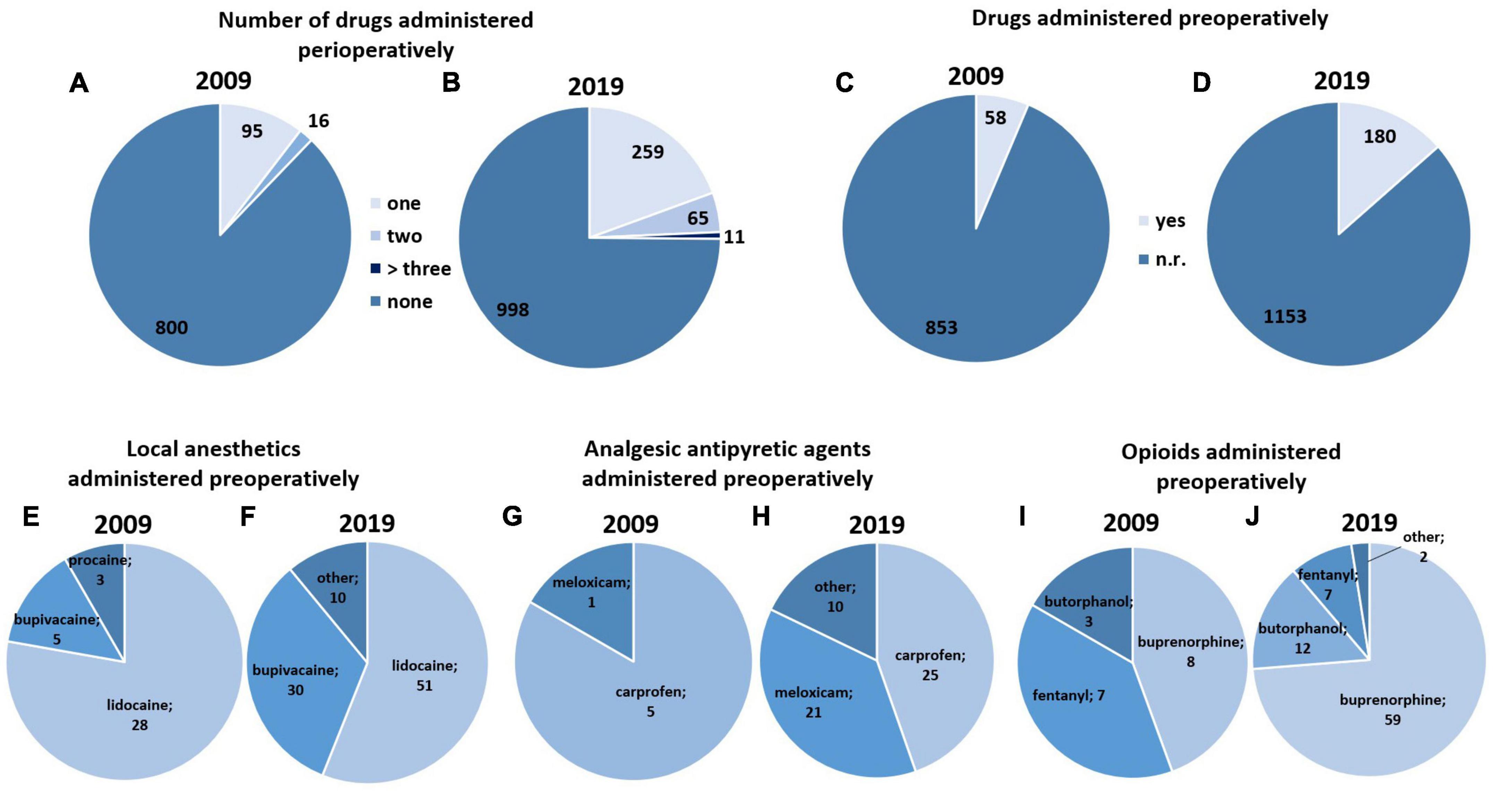
Figure 2. Analgesics and local anesthetics administered perioperatively and preoperatively (k = 2244 studies). (A,B) Number of drugs (analgesics and local anesthetics) administered perioperatively in 2009 (A) and 2019 (B). (C,D) Drugs (analgesics and local anesthetics) administered preoperatively in 2009 (C) and 2019 (D). (E,F) Local anesthetics administered preoperatively in 2009 (E) and 2019 (F). Other = drugs used in up to four studies. (G,H) Analgesic antipyretic agents administered preoperatively in 2009 (G) and 2019 (H). Other = drugs used in up to seven studies. (I,J) Opioids administered preoperatively in 2009 (I) and 2019 (J). Other = drugs used in up to two studies. “Preoperatively” was defined as all timepoints before skin incision.
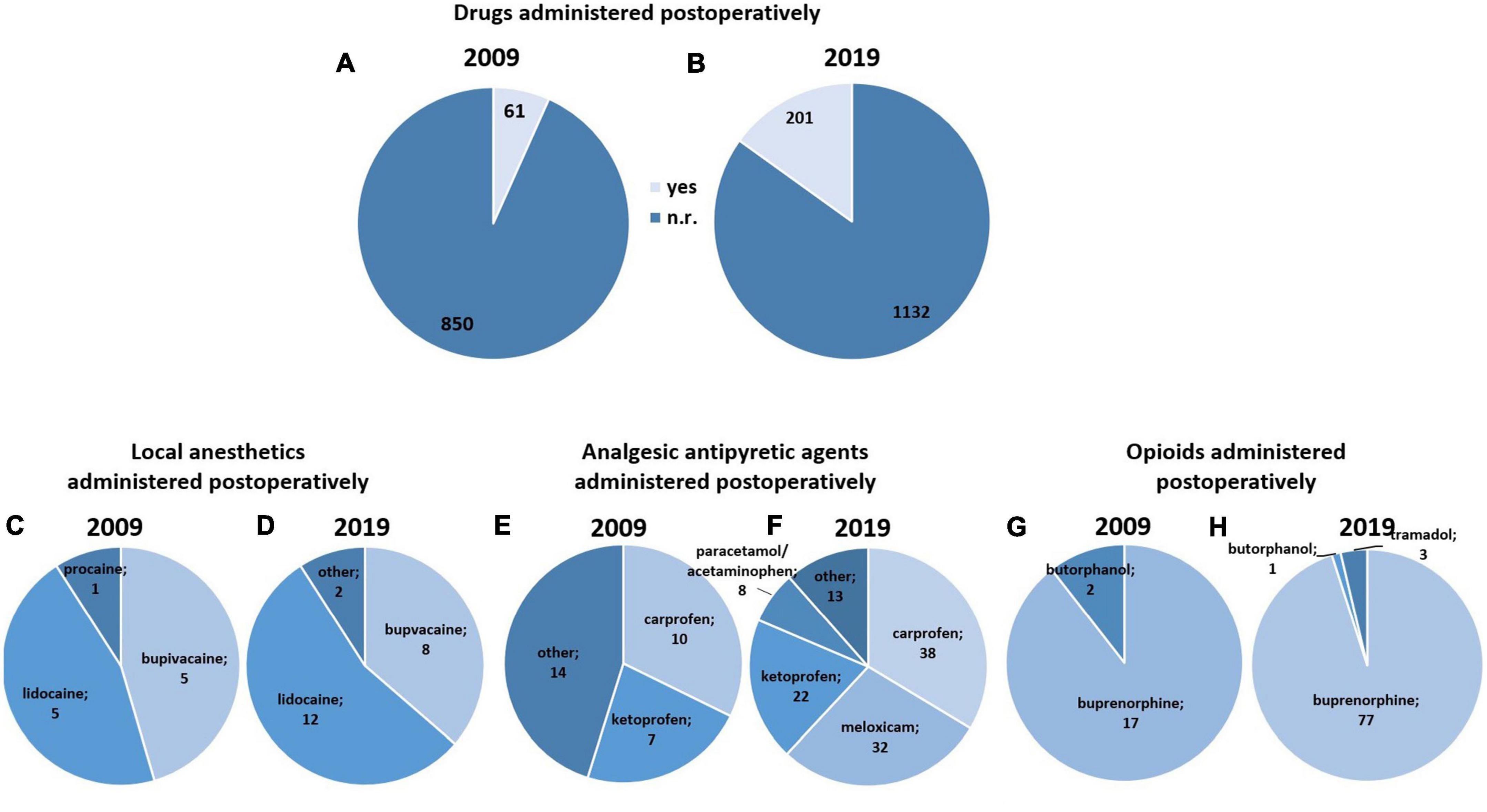
Figure 3. Analgesics and local anesthetics administered postoperatively (k = 2244 studies). (A,B) Drugs (analgesics and local anesthetics) administered postoperatively in 2009 (A) and 2019 (B). (C,D) Local anesthetics administered postoperatively in 2009 (C) and 2019 (D). Other = drugs used in up to two studies. (E,F) Analgesic antipyretic agents administered postoperatively in 2009 (E) and 2019 (F). Other = drugs used in up to five studies. (G,H) Opioids administered postoperatively in 2009 (G) and 2019 (H). “Postoperatively” was defined as all timepoints after finishing surgery and closing of incision.
The number of studies not reporting administration of analgesics or local anesthetics amounted to 87.8% (800/911) in 2009 and 74.8% (998/1333) in 2019.
While 95 of 911 studies from 2009 described the use of a single analgesic drug, only 16 of 911 studies from this year reported using two drugs. There was an increase in the relative number of studies reporting administration of more than one analgesic or local anesthetic from 2009 to 2019 (X2 (1) = 20.43, p < 0.001). 259 out of 1,333 studies described the use of a single drug, 65 out of 1,333 studies described use of two drugs, ten out of 1333 studies described use of three drugs, and one out of 1,333 studies described use of four drugs (Figures 2A, B). Please note that the number of drugs provided refers to the type of drug administered, and not the number of drug administrations, i.e., if the same drug was repeatedly applied, (e.g., once before and once after surgery), it was counted as one drug.
In 2009, two of 911 studies reported administering either an analgesic or local anesthetic, but the authors did not report which substance was used. The same applied to seven of 1,333 studies from 2019.
3.2.2.1. Preoperative analgesia - key characteristics
The number of studies reporting administration of analgesics or local anesthetics before surgery increased from 2009 to 2019 (X2 (1) = 21.38, p < 0.001). In the studies describing administration of local anesthetics prior to surgery, lidocaine was the most commonly used local anesthetic (2009: 28/911 studies; 2019: 51/1333 studies), and bupivacaine the second most commonly used (2009: 5/911 studies; 2019: 30/1333 studies) (Figures 2E, F). The comparison between data sets from 2009 and 2019 indicated an increase in the use of local anesthetics before surgery (X2 (1) = 7.85, p = 0.005).
The two most common NSAIDs administered before surgery in both years of interest were carprofen (2009: 5/911 studies; 2019: 25/1333 studies) and meloxicam (2009: 1/911 studies; 2019: 21/1333 studies) (Figures 2G, H). The reporting of preoperative administration of NSAIDs reached higher levels in 2019 than in 2009 (X2 (1) = 23.31, p < 0.001). One of 1333 studies in 2019 reported use of the antipyretic analgesic metamizole/dipyrone.
The most common opioids administered before surgery were buprenorphine (2009: 8/911 studies; 2019: 59/1333 studies), fentanyl (2009: 7/911 studies; 2019: 7/1333 studies), and butorphanol (2009: 3/911 studies; 2019: 12/1333 studies) (Figures 2I, J). Preoperative opioid administration significantly increased from 2009 to 2019 (X2 (1) = 17.53, p < 0.001).
Full information on all drugs used for pain management before surgery is provided in Table 4 and Supplementary Table 3.
3.2.2.2. Intraoperative analgesia - key characteristics
Only two studies from each year reported administering analgesics or local anesthetics during surgery. The drugs administered during surgery comprised bupivacaine and fentanyl (one study each of 911 studies in 2009), as well as buprenorphine and meloxicam (one study each of 1333 studies in 2019) (Table 4). Please note that the information provided here focused on administration during the actual surgical procedure [infusion or injection from the first incision through wound closure by primary intention (edges of wound are brought and held together by e.g., suturing, gluing, or stapling)]. In this context, it should be remembered that the duration of action of some drugs administered before surgery extends into the surgical phase.
3.2.2.3. Postoperative analgesia - key characteristics
The use of local anesthetics or analgesics after surgery was reported in 61 of 911 studies in 2009 and 201 of 1333 studies in 2019 (Figures 3A, B). The most commonly used local anesthetics in 2009 were bupivacaine (2009: 5/911 studies; 2019: 8/1333 studies) and lidocaine (2009: 5/911 studies; 2019: 12/1333 studies) (Figures 3C, D). We did not observe a significant change in the postoperative use of local anesthetics from 2009 to 2019 (X2 (1) = 0.46, p = 0.498). Among NSAIDs, the most commonly used substances in 2009 and 2019 were carprofen (2009: 10/911 studies; 2019: 38/1333 studies), ketoprofen (2009: 7/911 studies; 2019: 22/1333 studies, and meloxicam (2009: 2/911 studies; 2019: 32/1333 studies) (Figures 3E, F). Acetaminophen/paracetamol was the most frequently reported antipyretic analgesic in both years (2009: 3/911 studies; 2019: 8/1333 studies). One of 911 studies in 2009 and four of 1333 studies in 2019 reported use of metamizole/dipyrone. We found evidence for a significant increase in the postoperative use of NSAIDs from 2009 to 2019 (X2 (1) = 17.30, p < 0.001), but no change in the postoperative use of antipyretic analgesics (X2 (1) = 1.04, p = 0.308). Postsurgical administration of opioids comprised the use of buprenorphine (2009: 17/911 studies; 2019: 77/1333 studies), butorphanol (2009: 2/911 studies; 2019: 1/1333 study) and tramadol (2019: 3/1333 studies) (Figures 3G, H). The postoperative administration of opioids increased from 2009 to 2019 (X2 (1) = 19.32, p < 0.001).
For an overview of all drugs administered after surgery as reported in the 2009 and 2019 references, please see Table 4 and Supplementary Table 3.
3.2.3. Multimodal approaches - key characteristics
Multimodal approaches (use of >2 substances) for perioperative pain management were reported in 16 of 911 studies in 2009 (two substances) and 76 of 1,333 studies (two substances: 65/1333 studies; three substances: 10/1333 studies; four substances: 1/1333 studies) in 2019 (Figures 2A, B and Table 4). The reporting of multimodal approaches increased from 2009 to 2019 (X2 (1) = 20.43, p < 0.001).
In 2009, six of 911 studies reported administering a local anesthetic in combination with an opioid. Another four of 911 studies reported administering an opioid and an NSAID, whereas two of 911 studies reported the administration of a local anesthetic and an NSAID and one of 911 studies reported the administration of a local anesthetic and an antipyretic analgesic. Two of 911 studies reported administering two different NSAIDs and one study reported administering two opioids.
In 2019, the most commonly reported combinations of two substances were a local anesthetic with an NSAID (24/1333 studies), followed by opioid plus NSAID (16/1333 studies), and local anesthetic plus opioid (16/1333 studies). Two further studies reported the administration of an antipyretic analgesic and an NSAID, while another study reported the administration of an antipyretic analgesic and an opioid. The majority of studies reporting the administration of three substances (7/10 studies) described using a local anesthetic, an NSAID and an opioid.
3.2.4. Anesthesia in 2009 and 2019 - key characteristics
The administration of anesthetic drugs was reported in all 912 studies in 2009 and in 1,329 of 1,330 studies in 2019 (Figure 4). For these analyses, experimental groups receiving different anesthetics, described within publications, were separately analyzed; the treatment regime was our unit of analysis. Because one reference in 2009 described two experimental groups, and five references in 2019 described two or more experimental groups, we now refer to 912 studies in 2009 and 1,330 studies in 2019.
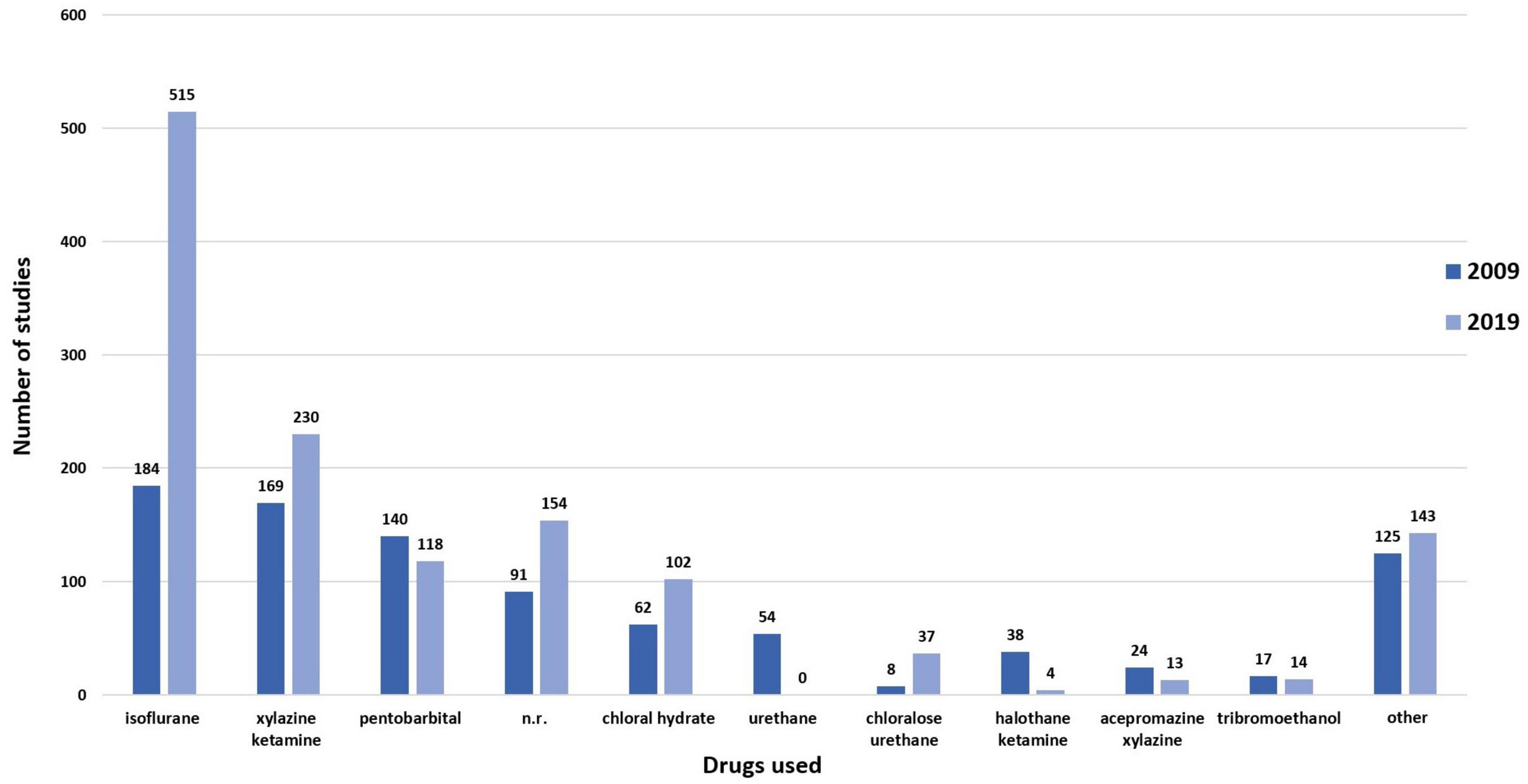
Figure 4. Anesthetics drugs (k = 2241 studies). Administration of anesthetic drugs in 2009 (k = 911) and 2019 (k = 1330). N.r., not reported. Note that one of the included papers from 2019 did not use anesthesia; the surgeries were performed on conscious animals.
In 2009, three of 912 studies reported inducing hypothermia in neonatal pups instead of using an anesthetic drug. Another study reported using either hypothermia in neonatal pups or a mixture of ketamine and xylazine. In 2019, two of 1330 publications described using hypothermia in neonatal pups. One additional manuscript reported not administering any substances to induce anesthesia; the animals remained conscious for the duration of the surgery. No explanation was provided as to why the animals remained conscious during the surgical procedure. In 2009, 91 of 912 studies did not report which drug was used to induce general anesthesia. In 2019, this was the case for 154 of 1330 studies.
The most commonly used drug to induce general anesthesia in both years of interest was isoflurane. It was administered in 184 of 912 studies in 2009 and in 515 of 1330 studies in 2019 (Figure 4). In both years, isoflurane was used in combination with ketamine and xylazine in several studies (2009: 7/912 studies; 2019: 17/1330 studies). Use of isoflurane was more frequently reported in 2019 than in 2009 (X2 (1) = 85.65, p < 0.001). Moreover, there was a general increase in the use of inhalational anesthesia compared to the use of injectable anesthesia (X2 (1) = 22.70, p < 0.001). Full information on all calculations is provided in Supplementary Methods 4.
The combination of ketamine and xylazine was the second most commonly used form for induction of general anesthesia. Use of this combination was reported in 169 of 912 studies in 2009 and 230 of 1330 studies in 2019 (Figure 4). There was no significant difference between 2009 and 2019 (X2 (1) = 0.33, p = 0.564). Full information on all calculations is provided in Supplementary Methods 4. Ketamine and xylazine were also combined with further drugs, the most frequently reported combination being ketamine, xylazine, and acepromazine (2009: 24/912 studies; 2019: 13/1330 studies).
The third most commonly used substance for general anesthesia in both years was pentobarbital (2009: 140/912 studies; 2019: 118/1330 studies). There was a significant decrease in the use of pentobarbital from 2009 to 2019 (X2 (1) = 21.67, p < 0.001). Combinations of pentobarbital with other substances were reported by 30 of 912 studies in 2009 and twelve of 1330 studies in 2019. Drugs used for combination with pentobarbital comprised chloral hydrate, diethyl ether, isoflurane, ketamine, and xylazine.
Other drugs used to induce anesthesia included chloral hydrate (2009: 62/912 studies; 2019: 102/1330 studies), halothane (2009: 38/911 studies; 2019: 4/1330 studies), urethane (2009: 54/912 studies) urethane in combination with chloralose (2009: 8/912 studies; 2019: 37/1330 studies), and tribromoethanol (2009: 17/912 studies; 2019: 7/1330 studies).
Full information on all anesthetic drugs used is provided in Table 5 and Supplementary Table 3.
3.2.5. Anesthesia and analgesia in combination - key characteristics
Conclusions about pain management need to consider that some drugs used for general anesthesia can exert analgesic effects themselves (e.g., ketamine, alpha-sympathomimetic drugs including xylazine, and urethane). Thus, we additionally analyzed the combination of specific drugs used for general anesthesia with analgesics or local anesthetics.
In 2009, 184 of 912 studies reported administering isoflurane as the only drug used to induce general anesthesia. Here, 35 studies described the additional perioperative administration of one or more drugs used for pain management. The list of added drugs comprised: lidocaine, bupivacaine, meloxicam, carprofen, ketoprofen, ibuprofen, flunixin, acetaminophen/paracetamol, buprenorphine, and butorphanol. The remaining 149 of 184 studies did not mention administering any analgesics or local anesthetics.
For 2019, 515 of 1330 studies reported administering isoflurane as the only drug used to induce general anesthesia. Here, 188 studies reported the additional perioperative administration of one or more analgesics or local anesthetics (including lidocaine, bupivacaine, ropivacaine, marcaine, meloxicam, carprofen, ketoprofen, ibuprofen, flunixin, acetaminophen/paracetamol, metamizole/dipyrone, buprenorphine, butorphanol, fentanyl, and piritramide). The remaining 327 of 515 studies using isoflurane as the only substance to induce general anesthesia did not mention administering any additional analgesics or local anesthetics.
In 2009, 169 of 912 studies reported use of a ketamine and xylazine combination to induce general anesthesia. Twenty-five of these studies reported the additional perioperative administration of one or more analgesics or local anesthetics (including lidocaine, procaine, carprofen, ketoprofen, metamizole/dipyrone, buprenorphine, and butorphanol). In 2019, 230 of 1330 studies reported use of a combination of ketamine and xylazine to induce general anesthesia. Sixty-three of these studies reported the additional perioperative administration of one or more analgesics or local anesthetics (including lidocaine, bupivacaine, procaine, ropivacaine, carprofen, meloxicam, ketoprofen, flunixin, metamizole/dipyrone, buprenorphine, butorphanol, fentanyl, and tramadol).
Among studies using pentobarbital to induce anesthesia, in 2009, three of 140 studies reported additional pre- and/or postoperative analgesia. In 2019, ten of 118 studies described pre- or postoperative administration of an analgesic.
In 2009, 62 of 912 studies reported using chloral hydrate to induce anesthesia. Only three of these studies reported an additional pre- or postoperative use of an analgesic or local anesthetic. In 2019, five of 102 studies using chloral hydrate described additional pre- and/or postoperative administration of an analgesic or local anesthetic.
Urethane was used in 54 of 912 studies in 2009. Five of these studies reported the administration of an analgesic or local anesthetic before surgery. In 2019, four studies from a total of 37 studies using urethane described the use of an analgesic drug before surgery.
Among studies using halothane (2009: 38/912 studies; 2019: 4/1330 studies) to induce anesthesia, in 2009, three studies reported administering an analgesic or local anesthetic before or following surgery. In 2019 one of the studies described administering two local anesthetics before surgery.
Please note that information on drugs used to induce anesthesia reported in 24 or fewer studies were not considered in this paragraph. Full information about all drugs and combinations used can be found in Supplementary Table 3.
3.3. Detailed data for the random subset of 200 references
For the purpose of feasibility, more detailed information was only collected from a random subset of 200 references (100 references/year). The additional information described below comprises study, animal and intervention characteristics, treatment protocols, outcome measures and risk of bias parameters. These additional data for the random subset allowed us to get an impression of dosage and frequency of the described anesthetics and analgesics.
Full information on all extracted parameters is provided in Supplementary Tables 1, 2.
3.3.1. General study-/animal characteristics – detailed analysis of random subset of 200 studies
From the subset of 200 studies, for 2009, 22 of 100 studies using mice and 79 of 100 studies using rats were identified. In one reference from 2009 (Merkler et al., 2009), both mice and rats were used. In 2019, the number of studies using mice was 43 of 100, and 57 of 100 used rats (Figures 5A, B). The list of studies using mice indicated that the most commonly used strain was C57BL/6 in both years of interest (2009: 4/100 studies; 2019: 13/100 studies). Further information regarding the substrains were generally not reported in these studies. An exception was the C57BL/6J strain, which was specifically mentioned in selected studies from both years (2009: 2/100 studies; 2019: 5/100 studies). The list of studies using rats indicated that the most commonly used strain was Sprague–Dawley in both years of interest (2009: 36/100 studies; 2019: 33/100 studies). The second most commonly used strain was Wistar in both years (2009: 24/100 studies; 2019: 15/100 studies). Further information on strain specificity was not reported.
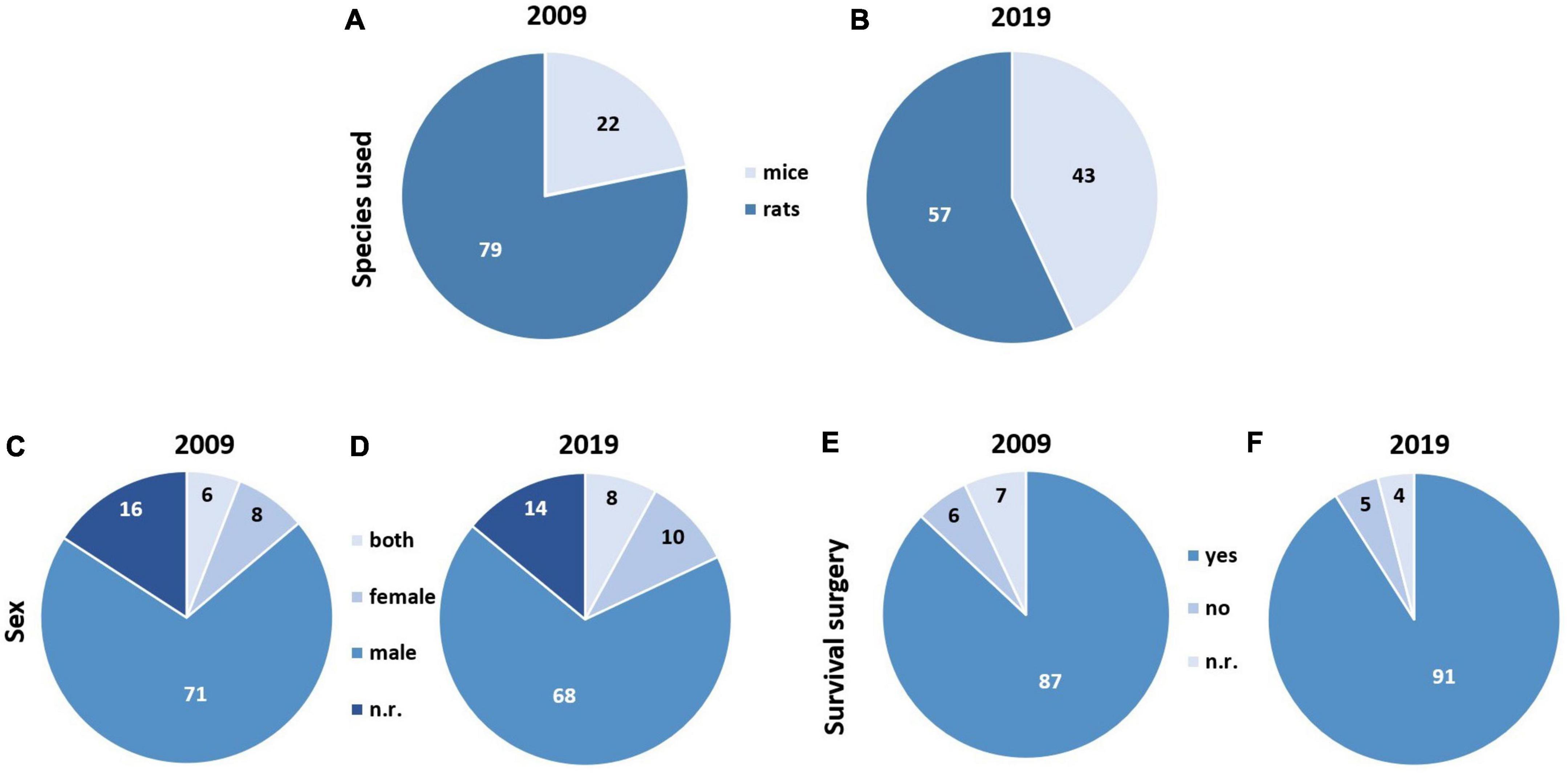
Figure 5. Animal model characteristics: species and sex and intervention characteristic survival surgery in the subset of 200 studies. (A) Studies using mice and rats in 2009 (k = 101 studies). (B) Studies using mice and rats in 2019 (k = 100 studies). (C,D) Studies reporting sex in 2009 (C; k = 101 studies) and 2019 (D; k = 100 studies). (E,F) Studies reporting if animals survived surgery in 2009 (E; k = 100 studies) and 2019 (F; k = 100 studies). n.r., not reported.
In both years, the majority of studies focused on male animals (2009: 71/101 studies; 2019: 68/100 studies). Female animals were used much less frequently (2009: 8/101 studies; 2019: 10/100 studies) (Figures 5C, D). Studies reporting both sexes were relatively uncommon in both years (2009: 6/101 studies; 2019: 8/100 studies). In 2009, 16 of 101 studies did not report the sex of the used animals; in 2019 this was 14 of 100 studies. Please note that we here refer to 101 studies from 2009, since one reference reported using mice and rats, but only provided information about the sex for rats.
For detailed information on further animals’ characteristics, housing, and husbandry see Supplementary Tables 1, 2.
For the purpose of this study, country of origin was defined as the country of the institute associated with the first author at the time of publication. The majority of the studies were from the United States of America (2009: 37/100 studies; 2019: 28/100 studies). There were no major differences in analgesic or anesthetic approaches between countries or continents. Full information on all countries of origin can be found in Supplementary Tables 1, 2.
3.3.2. Intervention characteristics – detailed analysis of random subset of 200 studies
Deep electrode implantations were the most common surgical procedure reported in both years of interest (2009: 19/102 studies; 2019: 17/102 studies). Please note that we here refer to 102 studies from each year, since in two references from each year, two groups with different surgical procedures were reported.
For both years, the second most commonly reported surgical intervention was the transient insertion of an intracerebral injection cannula (2009: 16/102 studies; 2019: 16/102 studies). For further information on other surgical procedures, see Supplementary Tables 1, 2.
There were no major differences between years in analgesic or anesthetic approaches for different surgical interventions. Almost all studies from both years reported survival surgeries (2009: 87/100 studies; 2019: 91/100 studies) (Figures 5E, F). Further studies either reported experiments during surgery, killing the animals directly afterward, or survival for less than 24 h post-surgery.
Specific monitoring during surgery was reported in 25 of 100 studies from 2009 and 24 of 100 studies from 2019. Monitoring during surgery comprised any monitoring measure, such as vital parameters (heart rate, breathing, blood pressure, body temperature, blood oxygen), reflexes (e.g., corneal reflex, pedal reflex), movements of the animal, and ECG (Electrocardiogram).
Perioperative care was reported for 23 of 100 studies from 2009 and 30 of 100 studies from 2019. Perioperative care was defined as any measure during surgery aimed at improving animal wellbeing or the outcome of surgery. These measures comprised maintaining of body temperature and application of eye ointment.
Non-pharmacological measures for pain management (e.g., cooling of incision to aid healing, massage, physical therapy) were reported neither for the year 2009 nor for 2019.
3.3.3. Pain-related outcome measures – detailed analysis of random subset of 200 studies
None of the studies published in 2009 reported an assessment of analgesic efficacy. In 2019, only one of 100 studies (Stanchi et al., 2019) reported assessing the analgesic efficacy post-surgery. Here, the well-being of mice was assessed daily by inspecting the animals. Parameters testing the efficacy of pain-reducing measures post-surgery, such as the mouse grimace score, were not reported within the sample.
3.3.4. Analgesia – detailed analysis of random subset of 200 studies
The use of analgesics or local anesthetics was reported in twelve of 100 studies in 2009 and 33 of 103 studies in 2019. For the subset of 200 studies, data regarding characteristics of the analgesic and anesthetic treatment protocol, e.g., dosage, administration interval, route of application, were extracted.
3.3.4.1. Local anesthetics
In 2009, six studies from the subset of 100 reported using local anesthetics (see Table 6). The substances used were lidocaine (2/100 studies), procaine (2/100 studies), and bupivacaine (1/100 studies). One study did not report which substance was used. Information about dosing was not provided in the majority (4/6 studies) of studies (Table 6 and Supplementary Tables 1, 2). For all local anesthetics, a single application was reported.
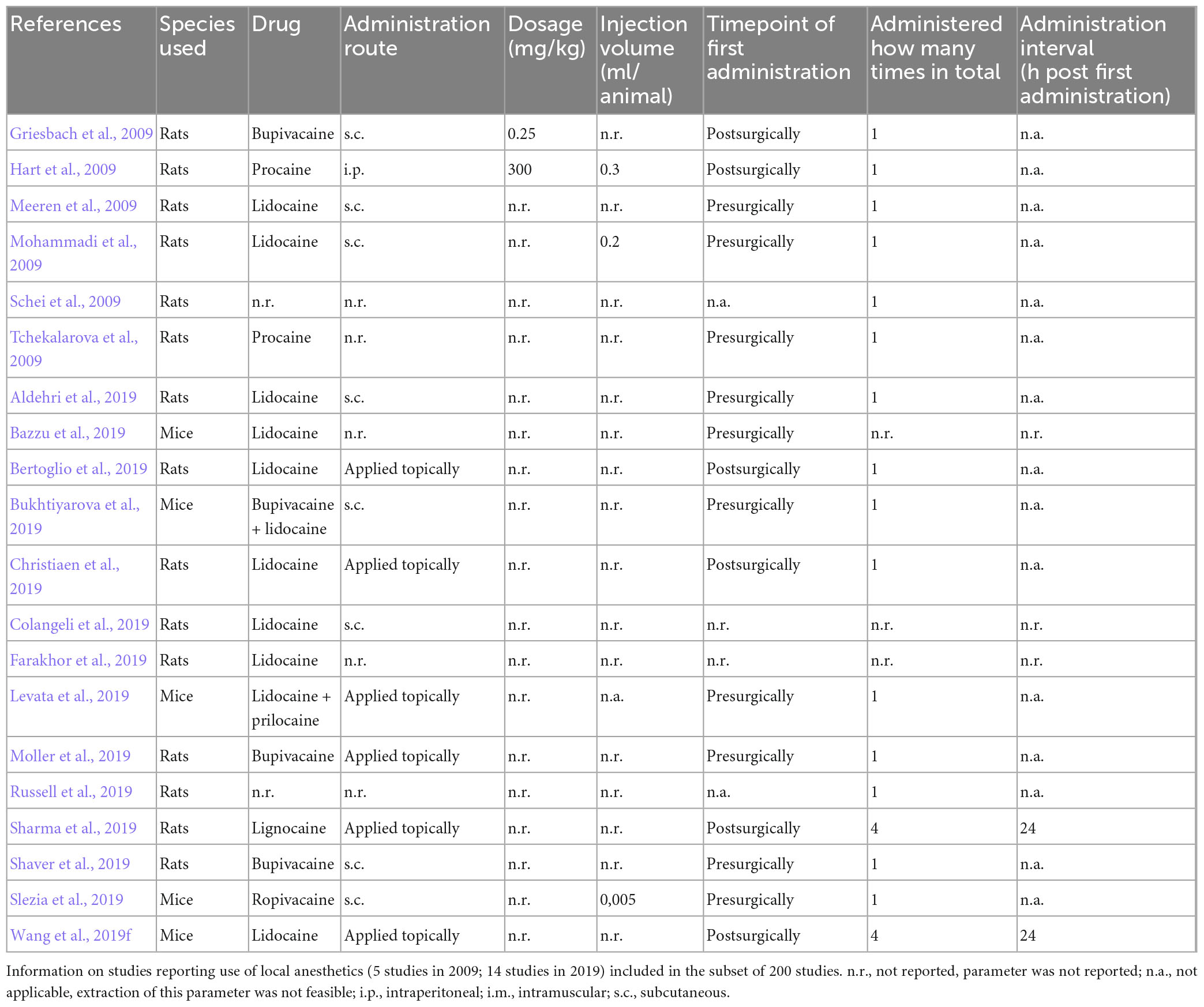
Table 6. Studies reporting use of local anesthesia from 2009 and 2019 included in the subset of 200 studies.
In 2019, 14 of 103 studies reported administration of a local anesthetic (see Table 6), with seven studies reporting administration before, four studies after surgery, and three studies not providing information on the time of administration. The most used local anesthetic was lidocaine, with eight of 103 studies reporting its use, whereas the second most used drug was bupivacaine, being used in two of 103 studies. Further local anesthetics used were ropivacaine, lignocaine, and prilocaine, all three substances being used in one study each. One study did not provide information on which local anesthetic was used and one study reported administering a combination of bupivacaine and lidocaine. None of the studies provided dosing information. Most studies (12/14) reported administering the local anesthetic once. Only two studies reported multiple applications with either lidocaine or lignocaine administered four times every 24 h.
For complete information on all drugs used, please refer to Table 6 and Supplementary Tables 1, 2.
3.3.4.2. Analgesic antipyretic agents administered
The administration of analgesic antipyretic agents was reported in five of 100 studies from 2009 and 16 of 103 studies from 2019 (see Table 7). Most studies (4/5) in 2009 and all (Kaur et al., 2000) studies in 2019 reported use of NSAIDs. The remaining study in 2009 used an antipyretic analgesic. In one reference from 2019, two experimental subgroups were described with animals receiving either an NSAID or an opioid.
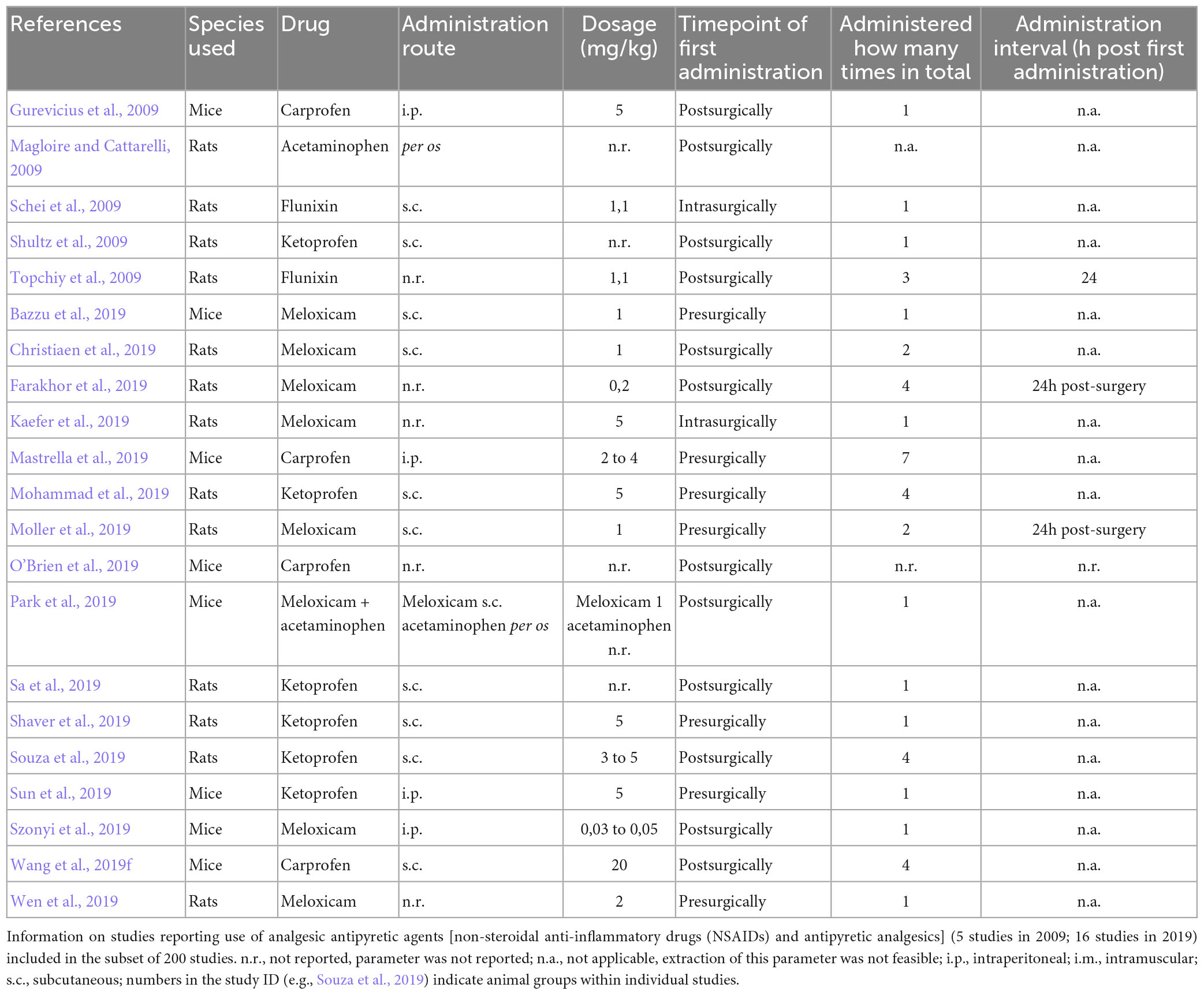
Table 7. Studies reporting use of analgesic antipyretic agents from 2009 (k = 100) and 2019 (k = 100) included in the subset of 200 studies.
For 2009, one study reported administering an NSAID during, and three studies after surgery, with flunixin being administered in two of 100 studies. The dose used in both studies was 1.1 mg/kg body weight (BW). One study administered the substance once subcutaneously, whereas the second study reported administering the substance three times with an application interval of 24 h but provided no information on the administration route. Other NSAIDs used were carprofen and ketoprofen. One study reported using the antipyretic analgesic acetaminophen/paracetamol. All substances were reported once, with no information on used doses for acetaminophen and ketoprofen. Carprofen was administered intraperitoneally at a dose of 5 mg/kg BW. All three analgesics were administered once.
In 2019, seven studies reported administering the NSAID before, one study during and eight studies after surgery. The most commonly used NSAID was meloxicam. Eight of 103 studies reported using it, with doses ranging from 0.03 to 5 mg/kg BW. Two studies administered the substance twice with an interval of 24 h and one study administered it four times with an interval of 24 h. The remaining studies reported administering meloxicam once. Of these eight studies, three did not report how the NSAID was administered. Four studies reported a subcutaneous administration. Here, doses ranged from 1 to 5 mg/kg BW. One study reported intraperitoneal administration at a dose of 0.03 to 0.05 mg/kg BW. The second most commonly used NSAID was ketoprofen, being used in five of 103 studies, with two studies both reporting application four times with an interval of 24 h. The remaining studies reported administering ketoprofen once. Four studies reported a subcutaneous administration of doses ranging from 3 to 5 mg/kg BW. The fifth study reported intraperitoneal administration of a dose of 5 mg/kg BW. Carprofen was used in three of 103 studies with doses ranging from 2 to 20 mg/kg BW, being administered seven times with an interval of 12 h in one study, and four times with an interval of 24 h in another study. The third study did not provide information on how often the substance was administered. Of these three studies, one reported subcutaneous administration of 20 mg/kg BW, another study reported intraperitoneal administration of 2 to 4 mg/kg BW while the third study did not provide information on how the substance was administered.
For complete information on all drugs used, please refer to Table 7 and Supplementary Tables 1, 2.
3.3.4.3. Opioids
Two of the 100 studies from 2009, and 14 of the 103 studies from 2019, reported opioid administration. In one reference from 2019, animals were allocated to two groups receiving either an NSAID or an opioid.
The opioid administered in both studies from 2009 and almost all studies from 2019 (13/14 studies) was buprenorphine (2009: doses ranged from 0.02 to 1 mg/kg BW applied subcutaneously; 2019: doses ranged from 0.01 to 2 mg/kg BW applied subcutaneously and 0.01 to 0.1 mg/kg BW applied intraperitoneally). In three of 14 studies from 2019, doses were reported without information about the route of administration. One additional study reported neither dose nor route of administration. Buprenorphine was administered once in all studies from 2009 and the majority of studies from 2019 (10/13 studies). Two studies from 2019 described repeated administration with either seven administrations every 12 h, or ten administrations every 8 h. One study reported a postoperative administration every 6–12 h but provided no information on how often the opioid was administered. Use of butorphanol was reported in one study in 2019, with an intraperitoneal administration of a dose of 2.5 mg/kg BW.
For complete information on all drugs used, please refer to Table 8 and Supplementary Tables 1, 2.
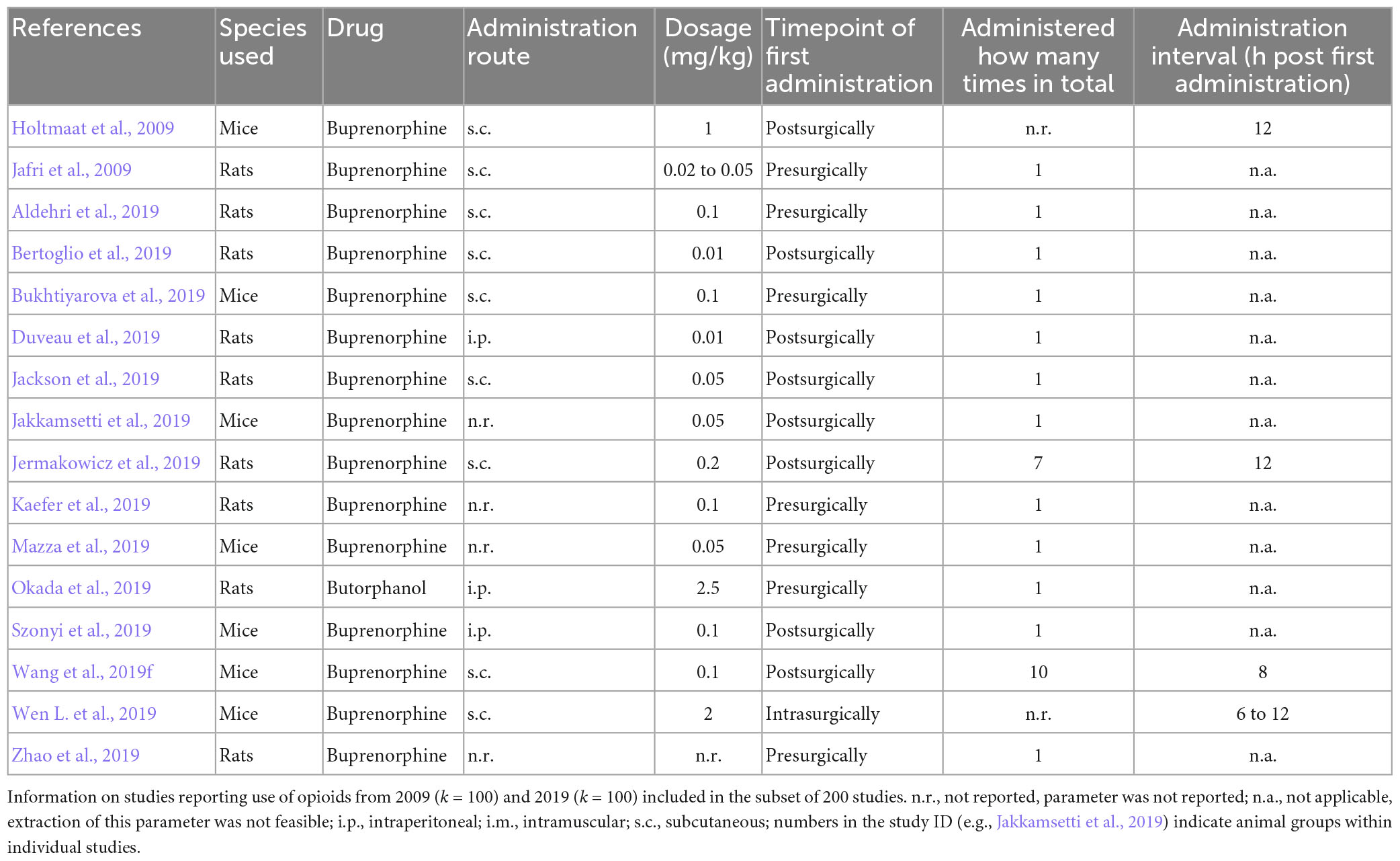
Table 8. Studies reporting use of opioids from 2009 (k = 100) and 2019 (k = 100) included in the subset of 200 studies.
3.3.5. Anesthesia – detailed analysis of random subset of 200 studies
In this section, we refer to 103 studies from 2009 and 101 studies from 2019, as several references reported forming groups with animals receiving different approaches to induce general anesthesia.
In 2009, the most frequently applied general anesthesia approach was a combination of ketamine and xylazine (25/103 studies), with the following dose ranges: 25 to 120 mg/kg BW for ketamine and 2.5 to 110 mg/kg BW for xylazine. Two studies did not report the doses used.
The second most common substance to induce general anesthesia in 2009 was isoflurane (19/103 studies). Six studies provided information about the concentrations used, which ranged from 1 to 3.5 vol.%. Another six studies reported administering different concentrations for induction (range: 2.5–5 vol.%) and maintenance (range: 1–3 vol.%) of anesthesia. Seven studies did not provide information on concentrations. Other drugs used to induce anesthesia were: pentobarbital (15/103 studies), halothane (5/103 studies), and urethane (5/103 studies).
Full information on all substances used to induce general anesthesia can be found in Table 9 and Supplementary Tables 1, 2.

Table 9. Type of anesthesia reported in studies from 2009 (k = 100) and 2019 (k = 100) included in the subset of 200 studies.
Isoflurane was the most frequently reported substance used for anesthesia in 2019 (44/101 studies). Twenty-four studies reported a different concentration for induction (range 2.5–5 vol.%) and maintenance (range 0.5–5 vol.%) of general anesthesia, whereas 15 studies did not specify separate concentrations for induction and maintenance of general anesthesia (concentrations ranging from 0.5 to 4 vol.%). Five studies did not provide information on concentration at all.
In 2019, the second most common approach was the combination of ketamine and xylazine with 19 of 101 studies reporting this combination. The doses ranged from 7.5 to 120 mg/kg BW for ketamine and 3–100 mg/kg BW for xylazine. Further anesthetic drugs comprised pentobarbital (7/101 studies) and chloral hydrate (5/101 studies).
Full information on all substances used to induce general anesthesia can be found in Table 9 and Supplementary Tables 1, 2.
3.3.6. Risk of bias – detailed analysis of random subset of 200 studies
Blinding was reported in 16 of 100 studies in 2009 (blinding of data analysis: 13 studies; during experimental procedure: two studies; during experimental procedure and data analysis: one study) and 16 of 100 studies in 2019 (of data analysis: 13 studies; during experimental procedure and data analysis: three studies). Randomization was reported in 14 of 100 studies published in 2009 (randomized group allocation: twelve studies; randomized data analysis: two studies) and 38 of 100 studies published in 2019 (group allocation: 36 studies; conducting of experimental procedure: one study; randomized data analysis: one study). In 2009, two of 100 studies reported conducting a power analysis, but none reported doing so in 2019. Reporting of doses and routes of application from studies reporting use of analgesics or local anesthetics was incomplete (no dose and/or route of application reported) in seven of eleven studies from 2009 and 23 of 32 studies from 2019. Regarding used anesthetics, 32 of 103 studies from 2009 and 30 of 101 studies from 2019 provided insufficient information on used doses or routes of application. To conclude, the majority of our sample did not report adequate blinding and randomization to prevent bias affecting their outcomes. Underreporting of doses and routes of administration was also common.
4. Discussion
This systematic scoping review provides representative information about common anesthetic and analgesic regimens applied perioperatively for craniotomy in mice and rats in 2009 and 2019. The extracted data inform us about the development of analgesic approaches over a decade since the report by Stokes et al. (2009), which described the lack of adequate analgesic regimens in the vast majority of mouse and rat studies with surgical interventions. While our analyses showed an increase in the application of analgesic drugs and local anesthetics in mouse and rat studies with intracranial surgery in 2019 versus 2009, the issue of undertreatment of surgical pain seems to persist, at least in the context of craniotomies. Moreover, the proportion of studies reporting multimodal analgesic regimens remains low. Please note that our data does not allow a conclusion on whether an analgesic regimen was sufficient or insufficient in specific studies, as neither a low nor high number of analgesics alone aligns with sufficient or insufficient analgesia. Aspects like dosage, administration interval and frequency of administration also have to be considered for the interpretation regarding sufficiency of the used analgesic regimen. The majority of studies from both years did not report the administration of any analgesic or local anesthetic, which suggests a lack of efforts to control postsurgical pain in the majority of experimental mice and rats undergoing craniotomy. This finding confirms recent statements by laboratory animal science experts (Jirkof, 2017; Flecknell, 2018; Foley et al., 2019). The high number of studies without description of analgesic regimen is alarming. Adequate treatment and prevention of pain is an ethical and, with most national regulations, also a legal requirement for any intervention in experimental animals (European Union, 2010; Jirkof, 2017; Prescott and Lidster, 2017). It is well known from human patients that uncontrolled pain can, among others, contribute to distress, negatively impact the affective state, disturb sleep patterns, prolong post-surgical recovery and healing phases, and increase risk of post-surgical complications and morbidity (Basali et al., 2000; Ortiz-Cardona and Bendo, 2007; Flexman et al., 2010; Dahl and Kehlet, 2011; Hansen et al., 2013). Studies focusing on rodents demonstrated that pain can for instance affect activity, behavioral patterns, circadian rhythmicity, and sleep duration (Carstens and Moberg, 2000; Jirkof et al., 2010, 2012, 2013). In the context of surgical procedures, it is of particular relevance that the uncontrolled activation of the nociceptive system can result in its sensitization at different levels, thereby contributing to prolonged and more severe post-surgical pain states with hyperalgesia and allodynia, and a risk for chronic pain (Kaur et al., 2000; Joshi and Ogunnaike, 2005; Latremoliere and Woolf, 2009; Dahl and Kehlet, 2011; Thapa and Euasobhon, 2018). Thus, the high number of publications not reporting the use of analgesics raises particular concerns regarding animal welfare. While exceptions might be defendable under very exceptional circumstances, the available multitude of analgesic drugs and local anesthetics should allow for an optimal analgesic regimen for the vast majority of studies without confounding the readout parameters (Peterson et al., 2017; Flecknell, 2018). In addition, an adjusted study design with longer post-surgical recovery phases and comparisons with substance-control groups can help to ensure data quality and correct interpretation (Moser, 2020; Jirkof and Potschka, 2021).
It can obviously not be emphasized enough that uncontrolled or insufficiently controlled pain and excessive activation of the nociceptive system exerts a multitude of effects. For instance, it can result in alterations of neurotransmitters, hormones, enzymes, metabolites, an impact on immune responses, and on sympathetic activity with a pronounced influence on cardiovascular function (Carstens and Moberg, 2000; Page, 2003; Arras et al., 2007; Jirkof, 2017; Jirkof and Potschka, 2021). These examples indicate that insufficiently controlled pain can influence a multitude of scientific readout parameters, with a detrimental impact on precision, reproducibility and external validity of the data (Jirkof, 2017; Peterson et al., 2017; Jirkof and Potschka, 2021).
As already mentioned, undertreatment of craniotomy-associated pain has also been reported in humans, and related to concerns about adverse effects comprising hypercapnia, hypertension, nausea and vomiting, sedation, and reduced blood coagulation, which might compromise neurological assessment or contribute to complications in the post-surgical phase (Leslie and Williams, 2005; Vadivelu et al., 2016). It should be taken into account that uncontrolled pain will also result in effects which can increase the risks in the post-craniotomy phase (Basali et al., 2000). The undertreatment of human patients undergoing craniotomy results from the idea that intracranial procedures are less painful than other surgeries (Dunbar et al., 1999). However, recent studies have demonstrated otherwise. Many patients reported moderate to severe pain in the early post-surgical phase (De Benedittis et al., 1996; Gottschalk et al., 2007; Mordhorst et al., 2010; Dunn et al., 2016; Bello et al., 2022), and acute post-surgical headache may persist or recur chronically in a subgroup of patients (Kaur et al., 2000; Vadivelu et al., 2016; Lutman et al., 2018). Thus, classifying the pain potentially associated with intracerebral electrode implantation in laboratory rodents as minimal to mild, as previously suggested in 2007 by ACLAM Task Force members (Kohn et al., 2007), is questionable. However, the expert group did already emphasize that the suggested categories should be considered as movable sliding scales. While previous findings from our group following implantation of electrodes in sham control groups argued against any long-term pain states and chronic issues, assessment in the early post-surgical phase confirmed the need for an efficacious analgesic regimen following craniotomy in mice and rats (Moller et al., 2018; Koska et al., 2019; Seiffert et al., 2019; Boldt et al., 2021; Buchecker et al., 2022). The need for analgesia has been further supported by Cho et al. (2019), who assessed the efficacy of meloxicam, carprofen and buprenorphine in comparison with a control group without analgesia in mice following craniotomy using the mouse grimace scale (MGS). In that study, all analgesics and routes of administration led to significant reductions in MGS scores compared to the control group.
The use of analgesic drugs increased from 2009 to 2019. There was no evidence for a preference of non-opioid analgesics versus opioid analgesics. The group of non-opioid analgesics comprised various drugs including antipyretic analgesics without a relevant anti-inflammatory effect, such as metamizole/dipyrone or acetaminophen/paracetamol, as well as NSAIDs such as carprofen, meloxicam and ketoprofen. While the choice of drugs with an anti-inflammatory effect can be advantageous in the postsurgical phase, as the limitation of inflammatory signaling will limit the activation of nociceptors, the specific risks associated with craniotomy procedures need to be taken into account. In this context, it is of interest that the additional use of paracetamol is also recommended to control post-craniotomy headache in human patients by different experts (Kotak et al., 2009; Dunn et al., 2016; Lutman et al., 2018). While NSAIDs are routinely used by different neurosurgeons, the avoidance of NSAIDs and preference of antipyretic analgesics might offer advantages concerning the risk for intracranial hemorrhage (Palmer et al., 1994; Vadivelu et al., 2016; Lutman et al., 2018). Thus, the use of antipyretic analgesics might also be justified in the context of craniotomies in mice and rats despite the lack of anti-inflammatory effects. On the other hand, the efficacy of the different drugs for different types of pain should be considered. For instance, it should be questioned whether metamizole/dipyrone achieves opioid-like efficacy in animals undergoing craniotomy, as the spasmolytic effect could contribute to a favorable efficacy profile in laparotomy procedures but may not reach an analgesic effect in craniotomy-associated pain as, for example, an opioid would.
The selection of NSAIDs used in studies from 2009 and 2019 was limited to traditional NSAIDs, without reported use of cyclooxygenase-2 inhibitors, i.e., coxibs. While these increase the risks for cardio- and cerebrovascular events in human patients (Kearney et al., 2006; Arfè et al., 2016; Fanelli et al., 2017; Gunter et al., 2017), coxibs are frequently used in veterinary medicine, and they might offer advantages for craniotomy procedures in experimental animals as they are not associated with enhanced bleeding risk (Lutman et al., 2018). Thus, it would be of particular future interest to conduct studies directly comparing traditional NSAIDs with coxibs.
The most common opioid used in mice and rats with craniotomy was buprenorphine. Other opioids administered were butorphanol, tramadol, fentanyl and piritramide. In comparison with buprenorphine, butorphanol and tramadol both have a lower efficacy. While adverse effects of opioids have limited their use in human patients with craniotomy (Leslie and Williams, 2005; Ortiz-Cardona and Bendo, 2007; Dunn et al., 2016; Vadivelu et al., 2016; Ban et al., 2019), it is nevertheless surprising to see that only one of the studies reported the use of intrasurgical administration of fentanyl, and only 14 out of 2234 studies across both years reported its presurgical administration. Likewise, only a handful of studies reported the perioperative administration of tramadol, piritramide and other full μ receptor agonists.
In laboratory rodents, one major issue with opioid use is nausea, pica behavior, loss of appetite, and weight loss (Tallett et al., 2009; Sarabia-Estrada et al., 2017). In this respect, slow-release preparations might be superior to regular formulations (Foley, 2014), which are unfortunately not yet licensed at a global level.
Intraoperative skin infiltration and scalp blockade by injecting local anesthetics into the skin surrounding the area of incision or near nerves innervating the operating field are considered as highly efficacious measures in human patients with craniotomy; their efficacy has been confirmed in several clinical studies and a meta-analysis (Guilfoyle et al., 2013; Vallapu et al., 2018). Thus, it is promising that the number of studies reporting the application of local anesthesia for intracranial surgery in mice and rats was higher in 2019 than in 2009. On the other hand, the overall rate of local anesthesia use still seems to be very low. This is unfortunate, as local anesthetics can efficaciously block transmission of nociceptive signals and can therefore prevent sensitization of the nociceptive system (Haldar et al., 2015; Vadivelu et al., 2016).
In human patients, a relatively new approach to perioperative analgesia is the administration of gabapentinoids (e.g., gabapentin, pregabalin) in addition to other opioid or non-opioid analgesics. These gabapentinoids are antiseizure medications which also exert antihyperalgesic and antinociceptive effects, and thereby decrease opioid requirements (Vadivelu et al., 2016; Tsaousi et al., 2017). In our data set, the administration of gabapentinoids for perioperative pain-management was not reported. This indicates that the use of these drugs in rodent models of craniotomy is not yet common practice.
The timing of the administration of analgesics and local anesthetics is of particular relevance for efficacy. It is strongly recommended to guarantee a continuous limitation of the activation of the nociceptive system throughout the surgical and post-surgical phase, to avoid any breakthrough pain and reduce sensitization processes contributing to hyperalgesia and allodynia (Joshi and Ogunnaike, 2005; Latremoliere and Woolf, 2009). Intrasurgical administration of opioids and non-opioid analgesics was only reported by very few studies (k = 4). It is emphasized that their administration during surgery can imply the risk that the nociceptive system has already been activated prior to analgesic drug exposure if no analgesic management has been applied before surgery. A preventive application of analgesics or local anesthetics can inhibit the activation of the nociceptive system, as an analgesic treatment is started before onset of the first painful stimulus, which leads to an inhibited nociceptive transmission and thereby contributes to a reduced central sensitization (Dahl and Kehlet, 2011; Vadivelu et al., 2014).
Multimodal analgesia regimens are strongly recommended in human and veterinary medicine for several reasons. In line with this, scientists have also been encouraged to implement the multimodal analgesia regimen in experimental studies (White and Kehlet, 2010; Barazanchi et al., 2018; Flecknell, 2018; Foley et al., 2019). The combination of different analgesics from different drug classes allows interference with nociceptive signaling at different levels of the nociceptive system (Kehlet and Dahl, 1993; Corletto, 2007). This renders multimodal approaches more efficacious based on additive or synergistic effects (Kehlet and Dahl, 1993; Schug, 2006; Girard et al., 2016). In addition, the doses of the different components of a multimodal regimen can often be reduced, thereby resulting in an advantageous tolerability profile of multimodal pain management approaches (White, 2008; Gritsenko et al., 2014; Rafiq et al., 2014; Foley et al., 2019). The advantage of the minimization of opioid doses by multimodal regimens has also been specifically discussed for craniotomy procedures in humans (Ban et al., 2019). Thus, it seems unfortunate that this has not yet been widely implemented for intracranial surgery in mice and rats. However, it is encouraging to see that there is an increase from 2009 to 2019 in using more than one analgesic drug.
Careful monitoring of vital functions during surgery, perioperative care comprising maintenance of body temperature and application of eye ointment, as well as non-pharmacological measures to control pain, can improve surgical outcomes and recovery, and can limit distress and pain (Schuler et al., 2009). However, the detailed assessment of a random sample of studies from 2009 and 2019 confirmed a low reporting rate for these adjunctive measures.
The design of an optimal analgesic regimen needs to go hand in hand with the selection of the anesthetic drugs. The comparison between data from 2009 and 2019 revealed an increase in the use of inhalational anesthesia, with isoflurane being the most commonly used anesthetic. Inhalational anesthesia offers several advantages compared to systemic anesthesia, including excellent controllability associated with good tolerability and low anesthesia risks (Hildebrandt et al., 2008; Lerman and Jöhr, 2009; Jedlicka et al., 2021). Unfortunately, the commonly used vaporous inhalation anesthetics do not exert relevant analgesic effects themselves. While this is not of immediate relevance during surgery, when the animals have lost consciousness and are in a tolerance state, the lack of antinociceptive impact raises the chance of sensitization, which can result in increased severity of post-surgical pain (Latremoliere and Woolf, 2009). While the same considerations apply for pentobarbital, which has still been used in some studies in 2009 and 2019, any combination comprising ketamine and an alpha2-sympathomimetic (such as xylazine or medetomidine) might benefit from the analgesic effects of the two drugs. On the other hand, these systemic combinations have clear disadvantages regarding controllability and anesthesia risks in comparison with inhalation anesthesia.
The selection of an appropriate anesthesia and analgesia regimen also needs to take the type of surgery into account. A different level of invasiveness, as well as the specific characteristics of the intervention, might result in different levels and types of post-surgical pain. For example, surgical procedures in the context of traumatic brain injury and ischemic or hemorrhagic stroke may put the animals at risk for higher levels of pain related to increases in intracranial pressure. Our data sets did not show a clear relationship between pain management approaches and interventions. However, a conclusive focused analysis would require a larger sample of studies.
Our work included an assessment of a small subset of parameters associated with study quality and the risk of bias. In line with various previous studies (Haahr and Hróbjartsson, 2006; Moja et al., 2014; Barcot et al., 2019), in our sample the risk of bias seemed to be poorly controlled, with basic principles such as blinding and randomization only reported for a small number of studies. While this is not directly relevant to our description of anesthetic and analgesic procedures, it raises concerns because there is evidence that the lack of randomization and blinding can result in inflated effect sizes (McCann et al., 2014; Saltaji et al., 2018).
As we extracted data from literature, our results are limited by the incomplete reporting by the original authors; their descriptions of the experimental methods could lack details. Thus, additional anesthetic and analgesic drugs may have been used compared to our findings. In spite of the introduction of the ARRIVE guidelines (Animal Research: Reporting of In Vivo Experiments), reporting of experimental details for primary studies is often far from complete. With our results, we cannot distinguish underreporting from undertreatment. Moreover, the preference for male animals is still evident in our sample of studies from 2019. Thus, the majority of the here included studies seem to ignore the National Institutes of Health recommendations from 2014 to perform studies in both sexes, as long as both are relevant for the research question (Clayton and Collins, 2014). While there is a lag between performing and publishing studies, and while recommendations cannot be applied retrospectively, we would still have expected to see some improvement in 2019. Lastly, it must be noted that we performed a scoping review; not a full systematic review. In line with the scoping approach, we only searched a single database; PubMed, and did not aim to include all relevant literature. It is likely that studies not indexed in PubMed were not retrieved by our search, but as PubMed is one of the largest medical databases, we do not expect our sample to be biased.
In conclusion, analysis of studies with craniotomy in mice and rats published in 2009 and 2019 revealed a slight increase in the reporting of perioperative administration of analgesics and local anesthetics. However, the high number of studies without any description of efforts to control pain suggests that inadequate analgesia is a persistent issue in the context of intracranial surgery in laboratory rodents. The persistently rare reporting of multimodal approaches, local anesthetic procedures, and adjunctive care measures, underscores the need for an intensified training and education of those working with animals subjected to craniotomies in the adequate application and reporting of anesthesia and analgesia.
Data availability statement
The original contributions presented in this study are included in the article/Supplementary material, further inquiries can be directed to the corresponding author.
Author contributions
HP, MB, and AB acquired the funding. HP supervised the review. HP, CL, MB, and AB conceptualized the review. MR wrote the review protocol and the search string under the supervision from HP and CL. HK, KA, MR, NM, AG, MB, HS, KS, LS, and PJ performed the screening. AG and LS performed the quality checks. HK performed the statistical analyses. HK, CL, and HP wrote the manuscript. All authors read and approved the manuscript.
Funding
This work was supported by grants of Deutsche Forschungsgemeinschaft (FOR-2591, GZ: PO681/9-2, FOR-2591, GZ: BA5768/2-1, and FOR-2591, GZ: BL953/11-2).
Acknowledgments
We thank Agnes Geißelmann for her excellent help with constructing the search string and performing the searches.
Conflict of interest
The authors declare that the research was conducted in the absence of any commercial or financial relationships that could be construed as a potential conflict of interest.
Publisher’s note
All claims expressed in this article are solely those of the authors and do not necessarily represent those of their affiliated organizations, or those of the publisher, the editors and the reviewers. Any product that may be evaluated in this article, or claim that may be made by its manufacturer, is not guaranteed or endorsed by the publisher.
Supplementary material
The Supplementary Material for this article can be found online at: https://www.frontiersin.org/articles/10.3389/fnins.2023.1143109/full#supplementary-material
Footnotes
References
Aldehri, M., Temel, Y., Jahanshahi, A., and Hescham, S. (2019). Fornix deep brain stimulation induces reduction of hippocampal synaptophysin levels. J. Chem. Neuroanat. 96, 34–40. doi: 10.1016/j.jchemneu.2018.12.001
Arfè, A., Scotti, L., Varas-Lorenzo, C., Nicotra, F., Zambon, A., Kollhorst, B., et al. (2016). Non-steroidal anti-inflammatory drugs and risk of heart failure in four European countries: Nested case-control study. BMJ 354:i4857. doi: 10.1136/bmj.i4857
Arras, M., Rettich, A., Cinelli, P., Kasermann, H. P., and Burki, K. (2007). Assessment of post-laparotomy pain in laboratory mice by telemetric recording of heart rate and heart rate variability. BMC Vet. Res. 3:16. doi: 10.1186/1746-6148-3-16
Asan, A. S., and Sahin, M. (2019). Modulation of multiunit spike activity by transcranial AC stimulation (tACS) in the rat cerebellar cortex. Annu. Int. Conf. IEEE Eng. Med. Biol. Soc. 2019, 5192–5195. doi: 10.1109/EMBC.2019.8856830
Ban, V. S., Bhoja, R., and McDonagh, D. L. (2019). Multimodal analgesia for craniotomy. Curr. Opin. Anaesthesiol. 32, 592–599. doi: 10.1097/ACO.0000000000000766
Barazanchi, A. W. H., MacFater, W. S., Rahiri, J. L., Tutone, S., Hill, A. G., and Joshi, G. P. (2018). Evidence-based management of pain after laparoscopic cholecystectomy: A PROSPECT review update. Br. J. Anaesth. 121, 787–803. doi: 10.1016/j.bja.2018.06.023
Barcot, O., Boric, M., Dosenovic, S., Poklepovic Pericic, T., Cavar, M., and Puljak, L. (2019). Risk of bias assessments for blinding of participants and personnel in Cochrane reviews were frequently inadequate. J. Clin. Epidemiol. 113, 104–113. doi: 10.1016/j.jclinepi.2019.05.012
Bartolomucci, A., Bresciani, E., Bulgarelli, I., Rigamonti, A. E., Pascucci, T., Levi, A., et al. (2009). Chronic intracerebroventricular injection of TLQP-21 prevents high fat diet induced weight gain in fast weight-gaining mice. Genes Nutr. 4, 49–57. doi: 10.1007/s12263-009-0110-0
Basali, A., Mascha, E. J., Kalfas, I., and Schubert, A. (2000). Relation between perioperative hypertension and intracranial hemorrhage after craniotomy. Anesthesiology 93, 48–54. doi: 10.1097/00000542-200007000-00012
Baud, M. O., Ghestem, A., Benoliel, J. J., Becker, C., and Bernard, C. (2019). Endogenous multidien rhythm of epilepsy in rats. Exp. Neurol. 315, 82–87. doi: 10.1016/j.expneurol.2019.02.006
Bazzu, G., Serra, P. A., Hamelink, R., Feenstra, M. G. P., Willuhn, I., and Denys, D. (2019). Monitoring deep brain stimulation by measuring regional brain oxygen responses in freely moving mice. J. Neurosci. Methods 317, 20–28. doi: 10.1016/j.jneumeth.2019.01.012
Behrend, C. E., Cassim, S. M., Pallone, M. J., Daubenspeck, J. A., Hartov, A., Roberts, D. W., et al. (2009). Toward feedback controlled deep brain stimulation: Dynamics of glutamate release in the subthalamic nucleus in rats. J. Neurosci. Methods 180, 278–289. doi: 10.1016/j.jneumeth.2009.04.001
Bello, C., Andereggen, L., Luedi, M. M., and Beilstein, C. M. (2022). Postcraniotomy headache: Etiologies and treatments. Curr. Pain Headache Rep. 26, 357–364. doi: 10.1007/s11916-022-01036-8
Bertoglio, D., Jonckers, E., Ali, I., Verhoye, M., Van der Linden, A., and Dedeurwaerdere, S. (2019). In vivo measurement of brain network connectivity reflects progression and intrinsic disease severity in a model of temporal lobe epilepsy. Neurobiol. Dis. 127, 45–52. doi: 10.1016/j.nbd.2019.02.012
Biella, G. E., Trevisan, S., and Giardini, M. E. (2009). Probing for local activity-related modulation of the infrared backscattering of the brain cortex. J. Biophotonics 2, 588–595. doi: 10.1002/jbio.200810067
Bleimeister, I. H., Wolff, M., Lam, T. R., Brooks, D. M., Patel, R., Cheng, J. P., et al. (2019). Environmental enrichment and amantadine confer individual but nonadditive enhancements in motor and spatial learning after controlled cortical impact injury. Brain Res. 1714, 227–233. doi: 10.1016/j.brainres.2019.03.007
Boldt, L., Koska, I., Maarten van Dijk, R., Talbot, S. R., Miljanovic, N., and Palme, R. (2021). Toward evidence-based severity assessment in mouse models with repeated seizures: I. Electrical kindling. Epilepsy Behav. 115:107689. doi: 10.1016/j.yebeh.2020.107689
Boni, L. J., Ploug, K. B., Olesen, J., Jansen-Olesen, I., and Gupta, S. (2009). The in vivo effect of VIP, PACAP-38 and PACAP-27 and mRNA expression of their receptors in rat middle meningeal artery. Cephalalgia 29, 837–847. doi: 10.1111/j.1468-2982.2008.01807.x
Bramlett, H. M., Furones-Alonso, O., Lotocki, G., Rodriguez-Paez, A., Sanchez-Molano, J., and Keane, R. W. (2009). Sex differences in XIAP cleavage after traumatic brain injury in the rat. Neurosci. Lett. 461, 49–53. doi: 10.1016/j.neulet.2009.05.071
Buchecker, V., Koska, I., Pace, C., Talbot, S. R., Palme, R., Bleich, A., et al. (2022). Toward evidence-based severity assessment in mouse models with repeated seizures: (II.) impact of surgery and intrahippocampal kainate. Eur. Surg. Res. 64, 89–107. doi: 10.1159/000522156
Bukhtiyarova, O., Soltani, S., Chauvette, S., and Timofeev, I. (2019). Slow wave detection in sleeping mice: Comparison of traditional and machine learning methods. J. Neurosci. Methods 316, 35–45. doi: 10.1016/j.jneumeth.2018.08.016
Burgdorf, J. S., Christian, E. P., Sorensen, L., Stanton, P. K., Leaderbrand, K., Madsen, T. M., et al. (2019). A translational EEG-based approach to assess modulation of long-lasting NMDAR-dependent synaptic plasticity. Psychopharmacology 236, 3687–3693. doi: 10.1007/s00213-019-05341-w
Byun, J. S., Lee, S. H., Jeon, S. H., Kwon, Y. S., Lee, H. J., Kim, S. S., et al. (2009). Kainic acid-induced neuronal death is attenuated by aminoguanidine but aggravated by L-NAME in mouse hippocampus. Korean J. Physiol. Pharmacol. 13, 265–271. doi: 10.4196/kjpp.2009.13.4.265
Caltana, L., Merelli, A., Lazarowski, A., and Brusco, A. (2009). Neuronal and glial alterations due to focal cortical hypoxia induced by direct cobalt chloride (CoCl2) brain injection. Neurotox. Res. 15, 348–358. doi: 10.1007/s12640-009-9038-9
Carbone, L., and Austin, J. (2016). Pain and laboratory animals: Publication practices for better data reproducibility and better animal welfare. PLoS One 11:e0155001. doi: 10.1371/journal.pone.0155001
Carcak, N., Ferrandon, A., Koning, E., Aker, R. G., Ozdemir, O., Onat, F. Y., et al. (2009). Effect of stage 2 kindling on local cerebral blood flow rates in rats with genetic absence epilepsy. Epilepsia 50, 33–43. doi: 10.1111/j.1528-1167.2008.01712.x
Carstens, E., and Moberg, G. P. (2000). Recognizing pain and distress in laboratory animals. ILAR J. 41, 62–71. doi: 10.1093/ilar.41.2.62
Casanova-Carvajal, O., Urbano-Bojorge, A. L., Ramos, M., Serrano-Olmedo, J. J., and Martinez-Murillo, R. (2019). Slowdown intracranial glioma progression by optical hyperthermia therapy: Study on a CT-2A mouse astrocytoma model. Nanotechnology 30:355101. doi: 10.1088/1361-6528/ab2164
Cemil, B., Tun, K., Kaptanoglu, E., Cevirgen, B., Gurcay, A. G., Kaymaz, F., et al. (2009). The effect of mitomycin C as fibrosis preventive agent during craniectomies. Br. J. Neurosurg. 23, 304–308. doi: 10.1080/02688690802603913
Chen, G., Liu, C., Meng, G., Zhang, C., Chen, F., Tang, S., et al. (2019). Neuroprotective effect of mogrol against Abeta1-42 -induced memory impairment neuroinflammation and apoptosis in mice. J. Pharm. Pharmacol. 71, 869–877. doi: 10.1111/jphp.13056
Chen, C., Zhong, X., Smith, D. K., Tai, W., Yang, J., Zou, Y., et al. (2019). Astrocyte-specific deletion of Sox2 promotes functional recovery after traumatic brain injury. Cereb. Cortex 29, 54–69. doi: 10.1093/cercor/bhx303
Chen, F., Weng, Z., Xia, Q., Cao, C., Leak, R. K., Han, L., et al. (2019). Intracerebroventricular delivery of recombinant NAMPT deters inflammation and protects against cerebral ischemia. Transl. Stroke Res. 10, 719–728. doi: 10.1007/s12975-019-00692-0
Chen, T., Zhu, J., Hang, C. H., and Wang, Y. H. (2019). The potassium SK channel activator NS309 protects against experimental traumatic brain injury through anti-inflammatory and immunomodulatory mechanisms. Front. Pharmacol. 10:1432. doi: 10.3389/fphar.2019.01432
Chen, J., Li, C., Pei, D. S., Han, D., Liu, X. M., Jiang, H. X., et al. (2009). GluR6-containing KA receptor mediates the activation of p38 MAP kinase in rat hippocampal CA1 region during brain ischemia injury. Hippocampus 19, 79–89. doi: 10.1002/hipo.20479
Chen, M., and Bi, L. L. (2019). Optogenetic long-term depression induction in the PVT-CeL circuitry mediates decreased fear memory. Mol. Neurobiol. 56, 4855–4865. doi: 10.1007/s12035-018-1407-z
Chitturi, J., Santhakumar, V., and Kannurpatti, S. S. (2019). Beneficial effects of kaempferol after developmental traumatic brain injury is through protection of mitochondrial function, oxidative metabolism, and neural viability. J. Neurotrauma 36, 1264–1278. doi: 10.1089/neu.2018.6100
Cho, C., Michailidis, V., Lecker, I., Collymore, C., Hanwell, D., Loka, M., et al. (2019). Evaluating analgesic efficacy and administration route following craniotomy in mice using the grimace scale. Sci. Rep. 9:359. doi: 10.1038/s41598-018-36897-w
Christiaen, E., Goossens, M. G., Raedt, R., Descamps, B., Larsen, L. E., Craey, E., et al. (2019). Alterations in the functional brain network in a rat model of epileptogenesis: A longitudinal resting state fMRI study. Neuroimage 202:116144. doi: 10.1016/j.neuroimage.2019.116144
Cifani, C., Durocher, Y., Pathak, A., Penicaud, L., Smih, F., Massi, M., et al. (2009). Possible common central pathway for resistin and insulin in regulating food intake. Acta Physiol. 196, 395–400. doi: 10.1111/j.1748-1716.2008.01949.x
Clayton, J. A., and Collins, F. S. (2014). Policy: NIH to balance sex in cell and animal studies. Nature 509, 282–283. doi: 10.1038/509282a
Colangeli, R., Di Maio, R., Pierucci, M., Deidda, G., Casarrubea, M., and Di Giovanni, G. (2019). Synergistic action of CB1 and 5-HT2B receptors in preventing pilocarpine-induced status epilepticus in rats. Neurobiol. Dis. 125, 135–145. doi: 10.1016/j.nbd.2019.01.026
Corletto, F. (2007). Multimodal and balanced analgesia. Vet. Res. Commun. 31(Suppl. 1), 59–63. doi: 10.1007/s11259-007-0085-5
Cunningham, M. G., Connor, C. M., Carlezon, W. A. Jr., and Meloni, E. (2009). Amygdalar GABAergic-rich neural grafts attenuate anxiety-like behavior in rats. Behav. Brain Res. 205, 146–153. doi: 10.1016/j.bbr.2009.06.015
da Silva Pacheco, S., Araujo Rondini, T., Cioni Bittencourt, J., and Fuzeti Elias, C. (2019). Neurons expressing estrogen receptor alpha differentially innervate the periaqueductal gray matter of female rats. J. Chem. Neuroanat. 97, 33–42. doi: 10.1016/j.jchemneu.2019.01.004
Daglas, M., Draxler, D. F., Ho, H., McCutcheon, F., Galle, A., Au, A. E., et al. (2019). Activated CD8(+) T cells cause long-term neurological impairment after traumatic brain injury in mice. Cell Rep. 29, 1178–91.e6. doi: 10.1016/j.celrep.2019.09.046
Dahl, J. B., and Kehlet, H. (2011). Preventive analgesia. Curr. Opin. Anaesthesiol. 24, 331–338. doi: 10.1097/ACO.0b013e328345afd9
Dal-Pont, G. C., Resende, W. R., Bianchini, G., Gava, F. F., Peterle, B. R., Trajano, K. S., et al. (2019). Tamoxifen has an anti-manic effect but not protect the brain against oxidative stress in an animal model of mania induced by ouabain. J. Psychiatr. Res. 113, 181–189. doi: 10.1016/j.jpsychires.2019.03.020
Datta, S., Siwek, D. F., and Stack, E. C. (2009). Identification of cholinergic and non-cholinergic neurons in the pons expressing phosphorylated cyclic adenosine monophosphate response element-binding protein as a function of rapid eye movement sleep. Neuroscience 163, 397–414. doi: 10.1016/j.neuroscience.2009.06.035
De Benedittis, G., Lorenzetti, A., Migliore, M., Spagnoli, D., Tiberio, F., and Villani, R. M. (1996). Postoperative pain in neurosurgery: A pilot study in brain surgery. Neurosurgery 38, 466–9; discussion9–70. doi: 10.1227/00006123-199603000-00008
de Vries, R. (2015). A protocol format for the preparation, registration and publication of systematic reviews of animal intervention studies. Evid. Based Preclin. Med. 2, 1–9. doi: 10.1002/ebm2.7
Delaney, L. J., Ciraku, L., Oeffinger, B. E., Wessner, C. E., Liu, J. B., Li, J., et al. (2019). Breast cancer brain metastasis response to radiation after microbubble oxygen delivery in a murine model. J. Ultrasound Med. 38, 3221–3228. doi: 10.1002/jum.15031
Diesch, T. J., Mellor, D. J., Johnson, C. B., and Lentle, R. G. (2009). Electroencephalographic responses to tail clamping in anaesthetized rat pups. Lab. Anim. 43, 224–231. doi: 10.1258/la.2008.0080083
Diguet, E., Petit, F., Escartin, C., Cambon, K., Bizat, N., Dufour, N., et al. (2009). Normal aging modulates the neurotoxicity of mutant huntingtin. PLoS One 4:e4637. doi: 10.1371/journal.pone.0004637
Ding, Z. M., Rodd, Z. A., Engleman, E. A., and McBride, W. J. (2009). Sensitization of ventral tegmental area dopamine neurons to the stimulating effects of ethanol. Alcohol. Clin. Exp. Res. 33, 1571–1581. doi: 10.1111/j.1530-0277.2009.00985.x
Doan, B. T., Autret, G., Mispelter, J., Meric, P., Meme, W., Montecot-Dubourg, C., et al. (2009). Simultaneous two-voxel localized (1)H-observed (13)C-edited spectroscopy for in vivo MRS on rat brain at 9.4T: Application to the investigation of excitotoxic lesions. J. Magn. Reson. 198, 94–104. doi: 10.1016/j.jmr.2009.01.023
Doretto, M. C., Cortes-de-Oliveira, J. A., Rossetti, F., and Garcia-Cairasco, N. (2009). Role of the superior colliculus in the expression of acute and kindled audiogenic seizures in Wistar audiogenic rats. Epilepsia 50, 2563–2574. doi: 10.1111/j.1528-1167.2009.02164.x
Dreier, J. P., Major, S., Lemale, C. L., Kola, V., Reiffurth, C., Schoknecht, K., et al. (2019). Correlates of spreading depolarization, spreading depression, and negative ultraslow potential in epidural versus subdural electrocorticography. Front. Neurosci. 13:373. doi: 10.3389/fnins.2019.00373
Du, R., Zhu, X., Wu, S., Zhang, X., He, Y., Zhang, K., et al. (2019). PET imaging of metabolic changes after neural stem cells and GABA progenitor cells transplantation in a rat model of temporal lobe epilepsy. Eur. J. Nucl. Med. Mol. Imaging 46, 2392–2397. doi: 10.1007/s00259-019-04408-2
Dunbar, P. J., Visco, E., and Lam, A. M. (1999). Craniotomy procedures are associated with less analgesic requirements than other surgical procedures. Anesth. Analg. 88, 335–340. doi: 10.1213/00000539-199902000-00021
Dunn, L. K., Naik, B. I., Nemergut, E. C., and Durieux, M. E. (2016). Post-craniotomy pain management: Beyond opioids. Curr. Neurol. Neurosci. Rep. 16:93. doi: 10.1007/s11910-016-0693-y
Duveau, V., Buhl, D. L., Evrard, A., Ruggiero, C., Mande-Niedergang, B., Roucard, C., et al. (2019). Pronounced antiepileptic activity of the subtype-selective GABAA -positive allosteric modulator PF-06372865 in the GAERS absence epilepsy model. CNS Neurosci. Ther. 25, 255–260. doi: 10.1111/cns.13046
Dux, M., Rosta, J., Santha, P., and Jancso, G. (2009). Involvement of capsaicin-sensitive afferent nerves in the proteinase-activated receptor 2-mediated vasodilatation in the rat dura mater. Neuroscience 161, 887–894. doi: 10.1016/j.neuroscience.2009.04.010
Echegoyen, J., Armstrong, C., Morgan, R. J., and Soltesz, I. (2009). Single application of a CB1 receptor antagonist rapidly following head injury prevents long-term hyperexcitability in a rat model. Epilepsy Res. 85, 123–127. doi: 10.1016/j.eplepsyres.2009.02.019
Ehrlichman, R. S., Gandal, M. J., Maxwell, C. R., Lazarewicz, M. T., Finkel, L. H., Contreras, D., et al. (2009). N-methyl-d-aspartic acid receptor antagonist-induced frequency oscillations in mice recreate pattern of electrophysiological deficits in schizophrenia. Neuroscience 158, 705–712. doi: 10.1016/j.neuroscience.2008.10.031
Elmorsy, S. A., Soliman, G. F., Rashed, L. A., and Elgendy, H. (2019). Dexmedetomidine and propofol sedation requirements in an autistic rat model. Korean J. Anesthesiol. 72, 169–177. doi: 10.4097/kja.d.18.00005
Etholm, L., and Heggelund, P. (2009). Seizure elements and seizure element transitions during tonic-clonic seizure activity in the synapsin I/II double knockout mouse: A neuroethological description. Epilepsy Behav. 14, 582–590. doi: 10.1016/j.yebeh.2009.02.021
Etter, G., van der Veldt, S., Manseau, F., Zarrinkoub, I., Trillaud-Doppia, E., and Williams, S. (2019). Optogenetic gamma stimulation rescues memory impairments in an Alzheimer’s disease mouse model. Nat. Commun. 10:5322. doi: 10.1038/s41467-019-13260-9
European Union (2010). Directive 2010/63/EU of the European parliament and of the council of 22 September 2010 on the protection of animals used for scientific purposes. Brussels: European Union.
Ewell, L. A., Fischer, K. B., Leibold, C., Leutgeb, S., and Leutgeb, J. K. (2019). The impact of pathological high-frequency oscillations on hippocampal network activity in rats with chronic epilepsy. Elife 8:e42148. doi: 10.7554/eLife.42148
Fanelli, A., Ghisi, D., Aprile, P. L., and Lapi, F. (2017). Cardiovascular and cerebrovascular risk with nonsteroidal anti-inflammatory drugs and cyclooxygenase 2 inhibitors: Latest evidence and clinical implications. Ther. Adv. Drug Saf. 8, 173–182. doi: 10.1177/2042098617690485
Farakhor, S., Shalchyan, V., and Daliri, M. R. (2019). Adaptation effects of medial forebrain bundle micro-electrical stimulation. Bioengineered 10, 78–86. doi: 10.1080/21655979.2019.1599628
Farias, S., Frey, L. C., Murphy, R. C., and Heidenreich, K. A. (2009). Injury-related production of cysteinyl leukotrienes contributes to brain damage following experimental traumatic brain injury. J. Neurotrauma 26, 1977–1986. doi: 10.1089/neu.2009.0877
Farooq, U., and Dragoi, G. (2019). Emergence of preconfigured and plastic time-compressed sequences in early postnatal development. Science 363, 168–173. doi: 10.1126/science.aav0502
Fiath, R., Raducanu, B. C., Musa, S., Andrei, A., Lopez, C. M., Welkenhuysen, M., et al. (2019). Fine-scale mapping of cortical laminar activity during sleep slow oscillations using high-density linear silicon probes. J. Neurosci. Methods 316, 58–70. doi: 10.1016/j.jneumeth.2018.08.020
Flecknell, P. (2018). Rodent analgesia: Assessment and therapeutics. Vet. J. 232, 70–77. doi: 10.1016/j.tvjl.2017.12.017
Flexman, A. M., Ng, J. L., and Gelb, A. W. (2010). Acute and chronic pain following craniotomy. Curr. Opin. Anaesthesiol. 23, 551–557. doi: 10.1097/ACO.0b013e32833e15b9
Foley, P. L. (2014). Current options for providing sustained analgesia to laboratory animals. Lab. Anim. 43, 364–371. doi: 10.1038/laban.590
Foley, P. L., Kendall, L. V., and Turner, P. V. (2019). Clinical management of pain in rodents. Comp. Med. 69, 468–489. doi: 10.30802/AALAS-CM-19-000048
Fortress, A. M., Avcu, P., Wagner, A. K., Dixon, C. E., and Pang, K. C. H. (2019). Experimental traumatic brain injury results in estrous cycle disruption, neurobehavioral deficits, and impaired GSK3beta/beta-catenin signaling in female rats. Exp. Neurol. 315, 42–51. doi: 10.1016/j.expneurol.2019.01.017
Foti, S. B., Samulski, R. J., and McCown, T. J. (2009). Delivering multiple gene products in the brain from a single adeno-associated virus vector. Gene Ther. 16, 1314–1319. doi: 10.1038/gt.2009.106
Francois, J., Ferrandon, A., Koning, E., Angst, M. J., Sandner, G., and Nehlig, A. (2009). Selective reorganization of GABAergic transmission in neonatal ventral hippocampal-lesioned rats. Int. J. Neuropsychopharmacol. 12, 1097–1110. doi: 10.1017/S1461145709009985
Fritsch, B., Qashu, F., Figueiredo, T. H., Aroniadou-Anderjaska, V., Rogawski, M. A., and Braga, M. F. (2009). Pathological alterations in GABAergic interneurons and reduced tonic inhibition in the basolateral amygdala during epileptogenesis. Neuroscience 163, 415–429. doi: 10.1016/j.neuroscience.2009.06.034
Gargiulo, S., Greco, A., Gramanzini, M., Esposito, S., Affuso, A., Brunetti, A., et al. (2012). Mice anesthesia, analgesia, and care, part I: Anesthetic considerations in preclinical research. ILAR J. 53, E55–E69. doi: 10.1093/ilar.53.1.55
Girard, P., Chauvin, M., and Verleye, M. (2016). Nefopam analgesia and its role in multimodal analgesia: A review of preclinical and clinical studies. Clin. Exp. Pharmacol. Physiol. 43, 3–12. doi: 10.1111/1440-1681.12506
Good, L. B., Sabesan, S., Marsh, S. T., Tsakalis, K., Treiman, D., and Iasemidis, L. (2009). Control of synchronization of brain dynamics leads to control of epileptic seizures in rodents. Int. J. Neural Syst. 19, 173–196. doi: 10.1142/S0129065709001951
Gottschalk, A., Berkow, L. C., Stevens, R. D., Mirski, M., Thompson, R. E., White, E. D., et al. (2007). Prospective evaluation of pain and analgesic use following major elective intracranial surgery. J. Neurosurg. 106, 210–216. doi: 10.3171/jns.2007.106.2.210
Griesbach, G. S., Hovda, D. A., and Gomez-Pinilla, F. (2009). Exercise-induced improvement in cognitive performance after traumatic brain injury in rats is dependent on BDNF activation. Brain Res. 1288, 105–115. doi: 10.1016/j.brainres.2009.06.045
Gritsenko, K., Khelemsky, Y., Kaye, A. D., Vadivelu, N., and Urman, R. D. (2014). Multimodal therapy in perioperative analgesia. Best Pract. Res. Clin. Anaesthesiol. 28, 59–79. doi: 10.1016/j.bpa.2014.03.001
Guidine, P. A., Mesquita, M. B., Moraes-Santos, T., Massensini, A. R., and Moraes, M. F. (2009). Electroencephalographic evidence of brainstem recruitment during scorpion envenomation. Neurotoxicology 30, 90–96. doi: 10.1016/j.neuro.2008.10.001
Guilfoyle, M. R., Helmy, A., Duane, D., and Hutchinson, P. J. A. (2013). Regional scalp block for postcraniotomy analgesia: A systematic review and meta-analysis. Anesth. Analg. 116, 1093–1102. doi: 10.1213/ANE.0b013e3182863c22
Gunter, B. R., Butler, K. A., Wallace, R. L., Smith, S. M., and Harirforoosh, S. (2017). Non-steroidal anti-inflammatory drug-induced cardiovascular adverse events: A meta-analysis. J. Clin. Pharm. Ther. 42, 27–38. doi: 10.1111/jcpt.12484
Gurevicius, K., Kuang, F., Stoenica, L., Irintchev, A., Gureviciene, I., Dityatev, A., et al. (2009). Genetic ablation of tenascin-C expression leads to abnormal hippocampal CA1 structure and electrical activity in vivo. Hippocampus 19, 1232–1246. doi: 10.1002/hipo.20585
Haahr, M. T., and Hróbjartsson, A. (2006). Who is blinded in randomized clinical trials? A study of 200 trials and a survey of authors. Clin. Trials 3, 360–365. doi: 10.1177/1740774506069153
Haldar, R., Kaushal, A., Gupta, D., Srivastava, S., and Singh, P. K. (2015). Pain following craniotomy: Reassessment of the available options. Biomed Res. Int. 2015:509164. doi: 10.1155/2015/509164
Hansen, M. S., Brennum, J., Moltke, F. B., and Dahl, J. B. (2013). Suboptimal pain treatment after craniotomy. Dan. Med. J. 60:A4569.
Hart, G., Harris, J. A., and Westbrook, R. F. (2009). Systemic or intra-amygdala injection of a benzodiazepine (midazolam) impairs extinction but spares re-extinction of conditioned fear responses. Learn. Mem. 16, 53–61. doi: 10.1101/lm.1154409
Harvey, C. D., Collman, F., Dombeck, D. A., and Tank, D. W. (2009). Intracellular dynamics of hippocampal place cells during virtual navigation. Nature 461, 941–946. doi: 10.1038/nature08499
Hernandez-Gonzalez, M., Martinez-Pelayo, M., Arteaga Silva, M., Bonilla-Jaime, H., and Guevara, M. A. (2009). Ethanol changes the electroencephalographic correlation of the ventral tegmental area and nucleus accumbens, components of the mesoaccumbens system in rats. Pharmacol. Biochem. Behav. 92, 124–130. doi: 10.1016/j.pbb.2008.10.020
Hildebrandt, I. J., Su, H., and Weber, W. A. (2008). Anesthesia and other considerations for in vivo imaging of small animals. ILAR J. 49, 17–26. doi: 10.1093/ilar.49.1.17
Ho, S. K., Kovacevic, N., Henkelman, R. M., Boyd, A., Pawson, T., and Henderson, J. T. (2009). EphB2 and EphA4 receptors regulate formation of the principal inter-hemispheric tracts of the mammalian forebrain. Neuroscience 160, 784–795. doi: 10.1016/j.neuroscience.2009.03.013
Holtmaat, A., Bonhoeffer, T., Chow, D. K., Chuckowree, J., De Paola, V., Hofer, S. B., et al. (2009). Long-term, high-resolution imaging in the mouse neocortex through a chronic cranial window. Nat. Protoc. 4, 1128–1144. doi: 10.1038/nprot.2009.89
Hrncic, D., Rasic-Markovic, A., Susic, V., Djuric, D., and Stanojlovic, O. (2009). Influence of NR2B-selective NMDA antagonist on lindane-induced seizures in rats. Pharmacology 84, 234–239. doi: 10.1159/000238055
Hu, L., Li, P., You, Z., Zhang, Z., Jin, H., and Huang, L. (2019). Effects of cellular prion protein on rapid eye movement sleep deprivation-induced spatial memory impairment. J. Integr. Neurosci. 18, 439–444. doi: 10.31083/j.jin.2019.04.1163
Huguet, G., Aldavert-Vera, L., Kadar, E., Pena de Ortiz, S., Morgado-Bernal, I., and Segura-Torres, P. (2009). Intracranial self-stimulation to the lateral hypothalamus, a memory improving treatment, results in hippocampal changes in gene expression. Neuroscience 162, 359–374. doi: 10.1016/j.neuroscience.2009.04.074
Ilieva, K., Tchekalarova, J., Atanasova, D., Kortenska, L., and Atanasova, M. (2019). Antidepressant agomelatine attenuates behavioral deficits and concomitant pathology observed in streptozotocin-induced model of Alzheimer’s disease in male rats. Horm. Behav. 107, 11–19. doi: 10.1016/j.yhbeh.2018.11.007
Ishida, T., Obara, Y., and Kamei, C. (2009). Effects of some antipsychotics and a benzodiazepine hypnotic on the sleep-wake pattern in an animal model of schizophrenia. J. Pharmacol. Sci. 111, 44–52. doi: 10.1254/jphs.09142FP
Ito, K., Hirooka, Y., and Sunagawa, K. (2009). Acquisition of brain Na sensitivity contributes to salt-induced sympathoexcitation and cardiac dysfunction in mice with pressure overload. Circ. Res. 104, 1004–1011. doi: 10.1161/CIRCRESAHA.108.188995
Itoh, T., Satou, T., Nishida, S., Tsubaki, M., Hashimoto, S., and Ito, H. (2009b). The novel free radical scavenger, edaravone, increases neural stem cell number around the area of damage following rat traumatic brain injury. Neurotox. Res. 16, 378–389. doi: 10.1007/s12640-009-9081-6
Itoh, T., Satou, T., Nishida, S., Tsubaki, M., Hashimoto, S., and Ito, H. (2009a). Expression of amyloid precursor protein after rat traumatic brain injury. Neurol. Res. 31, 103–109. doi: 10.1179/016164108X323771
Jackson, T. M., Ostrowski, T. D., and Middlemas, D. S. (2019). Intracerebroventricular ghrelin administration increases depressive-like behavior in male juvenile rats. Front. Behav. Neurosci. 13:77. doi: 10.3389/fnbeh.2019.00077
Jafri, M. S., Tang, R., and Tang, C. M. (2009). Optical coherence tomography guided neurosurgical procedures in small rodents. J. Neurosci. Methods 176, 85–95. doi: 10.1016/j.jneumeth.2008.08.038
Jakkamsetti, V., Marin-Valencia, I., Ma, Q., Good, L. B., Terrill, T., Rajasekaran, K., et al. (2019). Brain metabolism modulates neuronal excitability in a mouse model of pyruvate dehydrogenase deficiency. Sci. Transl. Med. 11:eaan0457. doi: 10.1126/scitranslmed.aan0457
Jedlicka, J. F., Groene, P., Linhart, J., Raith, E., Mustapha, D., and Conzen, P. F. (2021). Inhalational anaesthetics: An update on mechanisms of action and toxicity. J. Exp. Neurol. 70, 343–355. doi: 10.1007/s00101-020-00908-1
Jermakowicz, W. J., Sloley, S. S., Dan, L., Vitores, A., Carballosa-Gautam, M. M., and Hentall, I. D. (2019). Cellular changes in injured rat spinal cord following electrical brainstem stimulation. Brain Sci. 9:124. doi: 10.3390/brainsci9060124
Jirkof, P. (2017). Side effects of pain and analgesia in animal experimentation. Lab. Anim. 46, 123–128. doi: 10.1038/laban.1216
Jirkof, P., and Potschka, H. (2021). “Effects of untreated pain, anesthesia, and analgesia in animal experimentation,” in Experimental design and reproducibility in preclinical animal studies. 1, ed. J. M. Sánchez Morgado (Cham: Springer), 105–126. doi: 10.1007/978-3-030-66147-2_5
Jirkof, P., Cesarovic, N., Rettich, A., Fleischmann, T., and Arras, M. (2012). Individual housing of female mice: Influence on postsurgical behaviour and recovery. Lab. Anim. 46, 325–334. doi: 10.1258/la.2012.012027
Jirkof, P., Cesarovic, N., Rettich, A., Nicholls, F., Seifert, B., and Arras, M. (2010). Burrowing behavior as an indicator of post-laparotomy pain in mice. Front. Behav. Neurosci. 4:165. doi: 10.3389/fnbeh.2010.00165
Jirkof, P., Fleischmann, T., Cesarovic, N., Rettich, A., Vogel, J., and Arras, M. (2013). Assessment of postsurgical distress and pain in laboratory mice by nest complexity scoring. Lab. Anim. 47, 153–161. doi: 10.1177/0023677213475603
Joshi, G. P., and Ogunnaike, B. O. (2005). Consequences of inadequate postoperative pain relief and chronic persistent postoperative pain. Anesthesiol. Clin. North Am. 23, 21–36. doi: 10.1016/j.atc.2004.11.013
Kaefer, K., Malagon-Vina, H., Dickerson, D. D., O’Neill, J., Trossbach, S. V., Korth, C., et al. (2019). Disrupted-in-schizophrenia 1 overexpression disrupts hippocampal coding and oscillatory synchronization. Hippocampus 29, 802–816. doi: 10.1002/hipo.23076
Kalauzi, A., Kesic, S., and Saponjic, J. (2009). Cortico-pontine theta synchronization phase shift following monoaminergic lesion in rat. J. Physiol. Pharmacol. 60, 79–84.
Katagiri, M., Iida, K., Ishihara, K., Nair, D., Harada, K., Kagawa, K., et al. (2019). Anti-seizure effect and neuronal activity change in the genetic-epileptic model rat with acute and chronic vagus nerve stimulation. Epilepsy Res. 155:106159. doi: 10.1016/j.eplepsyres.2019.106159
Katz, L. M., Frank, J. E., Dvorak, A., Finch, A., Szymanowski, A., and Gordon, C. J. (2009). Independence of brain and trunk temperature during hypothermic preconditioning in rats. J. Neurosci. Methods 179, 179–183. doi: 10.1016/j.jneumeth.2009.01.025
Kaur, A., Selwa, L., Fromes, G., and Ross, D. A. (2000). Persistent headache after supratentorial craniotomy. Neurosurgery 47, 633–636. doi: 10.1227/00006123-200009000-00020
Kearney, P. M., Baigent, C., Godwin, J., Halls, H., Emberson, J. R., and Patrono, C. (2006). Do selective cyclo-oxygenase-2 inhibitors and traditional non-steroidal anti-inflammatory drugs increase the risk of atherothrombosis? Meta-analysis of randomised trials. BMJ 332, 1302–1308. doi: 10.1136/bmj.332.7553.1302
Kehlet, H., and Dahl, J. B. (1993). The value of “multimodal” or “balanced analgesia” in postoperative pain treatment. Anesth. Analg. 77, 1048–1056. doi: 10.1213/00000539-199311000-00030
Kenny, E. M., Fidan, E., Yang, Q., Anthonymuthu, T. S., New, L. A., Meyer, E. A., et al. (2019). Ferroptosis contributes to neuronal death and functional outcome after traumatic brain injury. Crit. Care Med. 47, 410–418. doi: 10.1097/CCM.0000000000003555
Kim, J. H., and Ong, W. Y. (2009). Localization of the transcription factor, sterol regulatory element binding protein-2 (SREBP-2) in the normal rat brain and changes after kainate-induced excitotoxic injury. J. Chem. Neuroanat. 37, 71–77. doi: 10.1016/j.jchemneu.2008.12.004
Kim, Y. C., and Narayanan, N. S. (2019). Prefrontal D1 Dopamine-receptor neurons and delta resonance in interval timing. Cereb. Cortex 29, 2051–2060. doi: 10.1093/cercor/bhy083
Kohn, D., Martin, T., Foley, P., Morris, T., Swindle, M., Vogler, G., et al. (2007). Guidelines for the assessment and management of pain in rodents and rabbits. J. Am. Assoc. Lab. Anim. Sci. 46, 97–108.
Koska, I., van Dijk, R. M., Seiffert, I., Di Liberto, V., Möller, C., Palme, R., et al. (2019). Toward evidence-based severity assessment in rat models with repeated seizures: II. Chemical post-status epilepticus model. Epilepsia 60, 2114–2127. doi: 10.1111/epi.16330
Kotak, D., Cheserem, B., and Solth, A. (2009). A survey of post-craniotomy analgesia in British neurosurgical centres: Time for perceptions and prescribing to change? Br. J. Neurosurg. 23, 538–542. doi: 10.1080/02688690903100595
Kunori, N., and Takashima, I. (2019). An implantable cranial window using a collagen membrane for chronic voltage-sensitive dye imaging. Micromachines 10:789. doi: 10.3390/mi10110789
Kyyriainen, J., Bolkvadze, T., Koivisto, H., Lipponen, A., Perez, L. O., Ekolle Ndode-Ekane, X., et al. (2019). Deficiency of urokinase-type plasminogen activator and its receptor affects social behavior and increases seizure susceptibility. Epilepsy Res. 151, 67–74. doi: 10.1016/j.eplepsyres.2019.02.009
Lackovic, Z., Rebic, V., and Riederer, P. F. (2009). Single intracerebroventricular injection of botulinum toxin type A produces slow onset and long-term memory impairment in rats. J. Neural Transm. 116, 1273–1280. doi: 10.1007/s00702-009-0285-y
Latremoliere, A., and Woolf, C. J. (2009). Central sensitization: A generator of pain hypersensitivity by central neural plasticity. J. Pain 10, 895–926. doi: 10.1016/j.jpain.2009.06.012
Lee, C., and Agoston, D. V. (2009). Inhibition of VEGF receptor 2 increased cell death of dentate hilar neurons after traumatic brain injury. Exp. Neurol. 220, 400–403. doi: 10.1016/j.expneurol.2009.08.019
Lee, M., Shin, H. S., and Choi, J. H. (2009). Simultaneous recording of brain activity and functional connectivity in the mouse brain. Annu. Int. Conf. IEEE Eng. Med. Biol. Soc. 2009, 2934–2936.
Lee, W. R., Im, C., Park, H. Y., Seo, J. M., and Kim, J. M. (2019). Fabrication of convex PDMS-parylene microstructures for conformal contact of planar micro-electrode array. Polymers 11:1436. doi: 10.3390/polym11091436
Lerman, J., and Jöhr, M. (2009). Inhalational anesthesia vs total intravenous anesthesia (TIVA) for pediatric anesthesia. Paediatr. Anaesth. 19, 521–534. doi: 10.1111/j.1460-9592.2009.02962.x
Leslie, K., and Williams, D. L. (2005). Postoperative pain, nausea and vomiting in neurosurgical patients. Curr. Opin. Anaesthesiol. 18, 461–465. doi: 10.1097/01.aco.0000182564.25057.fa
Levata, L., Dore, R., Johren, O., Schwaninger, M., Schulz, C., and Lehnert, H. (2019). Nesfatin-1 acts centrally to induce sympathetic activation of brown adipose tissue and non-shivering thermogenesis. Horm. Metab. Res. 51, 678–685. doi: 10.1055/a-0985-4272
Li, F., Wang, X., Zhang, Z., Gao, P., and Zhang, X. (2019). Breviscapine provides a neuroprotective effect after traumatic brain injury by modulating the Nrf2 signaling pathway. J. Cell. Biochem. 120, 14899–14907. doi: 10.1002/jcb.28751
Li, Y. T., Chen, S. C., Yang, L. Y., Hsieh, T. H., and Peng, C. W. (2019). Designing and implementing a novel transcranial electrostimulation system for neuroplastic applications: A preliminary study. IEEE Trans. Neural Syst. Rehabil. Eng. 27, 805–813. doi: 10.1109/TNSRE.2019.2908674
Li, Q., Yang, C., Zhang, X., Yang, Z., and Zhang, T. (2019). Arginine vasopressin attenuates dysfunction of hippocampal theta and gamma oscillations in chronic cerebral hypoperfusion via V1a receptor. Brain Res. Bull. 153, 84–92. doi: 10.1016/j.brainresbull.2019.08.012
Li, R. B., Guo, X. C., Liang, H. X., Wang, F. Y., and Zhu, B. L. (2009). Study on changes of MMP-3 expression after brain contusion in rats. Leg. Med. 11, S176–S179. doi: 10.1016/j.legalmed.2009.02.029
Liu, D. S., O’Brien, T. J., Williams, D. A., Hicks, R. J., and Myers, D. E. (2009). Lamina-specific changes in hippocampal GABA(A)/cBZR and mossy fibre sprouting during and following amygdala kindling in the rat. Neurobiol. Dis. 35, 337–347. doi: 10.1016/j.nbd.2009.05.009
Lopez-Martin, E., Bregains, J., Relova-Quinteiro, J. L., Cadarso-Suarez, C., Jorge-Barreiro, F. J., and Ares-Pena, F. J. (2009). The action of pulse-modulated GSM radiation increases regional changes in brain activity and c-Fos expression in cortical and subcortical areas in a rat model of picrotoxin-induced seizure proneness. J. Neurosci. Res. 87, 1484–1499. doi: 10.1002/jnr.21951
Lu, X. C., Si, Y., Williams, A. J., Hartings, J. A., Gryder, D., and Tortella, F. C. (2009). NNZ-2566, a glypromate analog, attenuates brain ischemia-induced non-convulsive seizures in rats. J. Cereb. Blood Flow Metab. 29, 1924–1932. doi: 10.1038/jcbfm.2009.109
Lundblad, C., Grande, P. O., and Bentzer, P. (2009). Hemodynamic and histological effects of traumatic brain injury in eNOS-deficient mice. J. Neurotrauma 26, 1953–1962. doi: 10.1089/neu.2009.0955
Luo, P., Li, X., Wu, X., Dai, S., Yang, Y., Xu, H., et al. (2019). Preso regulates NMDA receptor-mediated excitotoxicity via modulating nitric oxide and calcium responses after traumatic brain injury. Cell Death Dis. 10:496. doi: 10.1038/s41419-019-1731-x
Lutman, B., Bloom, J., Nussenblatt, B., and Romo, V. A. (2018). Contemporary perspective on the management of post-craniotomy headache and pain. Curr. Pain Headache Rep. 22:69. doi: 10.1007/s11916-018-0722-4
Lv, X., Tang, R., Gao, Z., Hu, D., Li, G., Lang, Y., et al. (2019). Activation of the primary motor cortex using fully-implanted electrical sciatic nerve stimulation. Exp. Ther. Med. 18, 3357–3364. doi: 10.3892/etm.2019.7993
Ma, Y., Liu, T., Fu, J., Fu, S., Hu, C., Sun, B., et al. (2019). Lactobacillus acidophilus exerts neuroprotective effects in mice with traumatic brain injury. J. Nutr. 149, 1543–1552. doi: 10.1093/jn/nxz105
Magloire, V., and Cattarelli, M. (2009). Delayed changes of sleep duration after rewarded olfactory discrimination learning in the rat. Behav. Brain Res. 205, 568–571. doi: 10.1016/j.bbr.2009.08.039
Mark, A. L., Agassandian, K., Morgan, D. A., Liu, X., Cassell, M. D., and Rahmouni, K. (2009). Leptin signaling in the nucleus tractus solitarii increases sympathetic nerve activity to the kidney. Hypertension 53, 375–380. doi: 10.1161/HYPERTENSIONAHA.108.124255
Mastrella, G., Hou, M., Li, M., Stoecklein, V. M., Zdouc, N., Volmar, M. N. M., et al. (2019). Targeting APLN/APLNR improves antiangiogenic efficiency and blunts proinvasive side effects of VEGFA/VEGFR2 blockade in glioblastoma. Cancer Res. 79, 2298–2313. doi: 10.1158/0008-5472.CAN-18-0881
Mazza, M., Ahmad, H., Hadjidemetriou, M., Agliardi, G., Pathmanaban, O. N., King, A. T., et al. (2019). Hampering brain tumor proliferation and migration using peptide nanofiber:siPLK1/MMP2 complexes. Nanomedicine 14, 3127–3142. doi: 10.2217/nnm-2019-0298
McCann, R. A., Armstrong, C. M., Skopp, N. A., Edwards-Stewart, A., Smolenski, D. J., June, J. D., et al. (2014). Virtual reality exposure therapy for the treatment of anxiety disorders: An evaluation of research quality. J. Anxiety Disord. 28, 625–631. doi: 10.1016/j.janxdis.2014.05.010
McCracken, C. B., and Grace, A. A. (2009). Nucleus accumbens deep brain stimulation produces region-specific alterations in local field potential oscillations and evoked responses in vivo. J. Neurosci. 29, 5354–5363. doi: 10.1523/JNEUROSCI.0131-09.2009
Meeren, H. K., Veening, J. G., Moderscheim, T. A., Coenen, A. M., and van Luijtelaar, G. (2009). Thalamic lesions in a genetic rat model of absence epilepsy: Dissociation between spike-wave discharges and sleep spindles. Exp. Neurol. 217, 25–37. doi: 10.1016/j.expneurol.2009.01.009
Merkler, D., Klinker, F., Jurgens, T., Glaser, R., Paulus, W., Brinkmann, B. G., et al. (2009). Propagation of spreading depression inversely correlates with cortical myelin content. Ann. Neurol. 66, 355–365. doi: 10.1002/ana.21746
Mian, A., Sultana, T., Georgiev, D., Witte, R., Herfurth, H., Auner, G., et al. (2009). Postimplantation pressure testing and characterization of laser bonded glass/polyimide microjoints. J. Biomed. Mater. Res. B Appl. Biomater. 90, 614–620. doi: 10.1002/jbm.b.31325
Mittal, K., Eremenko, E., Berner, O., Elyahu, Y., Strominger, I., Apelblat, D., et al. (2019). CD4 T cells induce a subset of MHCII-expressing microglia that attenuates Alzheimer pathology. iScience 16, 298–311. doi: 10.1016/j.isci.2019.05.039
Mo, M., Eyo, U. B., Xie, M., Peng, J., Bosco, D. B., Umpierre, A. D., et al. (2019). Microglial P2Y12 receptor regulates seizure-induced neurogenesis and immature neuronal projections. J. Neurosci. 39, 9453–9464. doi: 10.1523/JNEUROSCI.0487-19.2019
Mohammad, H., Sekar, S., Wei, Z., Moien-Afshari, F., and Taghibiglou, C. (2019). Perampanel but not amantadine prevents behavioral alterations and epileptogenesis in pilocarpine rat model of status epilepticus. Mol. Neurobiol. 56, 2508–2523. doi: 10.1007/s12035-018-1230-6
Mohammadi, S., Pavlik, A., Krajci, D., and Al-Sarraf, H. (2009). NMDA preconditioning and neuroprotection in vivo: Delayed onset of kainic acid-induced neurodegeneration and c-Fos attenuation in CA3a neurons. Brain Res. 1256, 162–172. doi: 10.1016/j.brainres.2008.12.019
Mohammadipoor-Ghasemabad, L., Sangtarash, M. H., Sheibani, V., Sasan, H. A., and Esmaeili-Mahani, S. (2019). Hippocampal microRNA-191a-5p regulates BDNF expression and shows correlation with cognitive impairment induced by paradoxical sleep deprivation. Neuroscience 414, 49–59. doi: 10.1016/j.neuroscience.2019.06.037
Mohammadpoory, Z., Nasrolahzadeh, M., Mahmoodian, N., Sayyah, M., and Haddadnia, J. (2019). Complex network based models of ECoG signals for detection of induced epileptic seizures in rats. Cogn. Neurodyn. 13, 325–339. doi: 10.1007/s11571-019-09527-y
Moja, L., Pecoraro, V., Ciccolallo, L., Dall’Olmo, L., Virgili, G., and Garattini, S. (2014). Flaws in animal studies exploring statins and impact on meta-analysis. Eur. J. Clin. Invest. 44, 597–612. doi: 10.1111/eci.12264
Mollazadeh, M., Murari, K., Cauwenberghs, G., and Thakor, N. (2009). Micropower CMOS integrated low-noise amplification, filtering, and digitization of multimodal neuropotentials. IEEE Trans. Biomed. Circuits Syst. 3, 1–10. doi: 10.1109/TBCAS.2008.2005297
Moller, C., van Dijk, R. M., Wolf, F., Keck, M., Schonhoff, K., Bierling, V., et al. (2019). Impact of repeated kindled seizures on heart rate rhythms, heart rate variability, and locomotor activity in rats. Epilepsy Behav. 92, 36–44. doi: 10.1016/j.yebeh.2018.11.034
Moller, C., Wolf, F., van Dijk, R. M., Di Liberto, V., Russmann, V., Keck, M., et al. (2018). Toward evidence-based severity assessment in rat models with repeated seizures: I. Electrical kindling. Epilepsia 59, 765–777. doi: 10.1111/epi.14028
Mordhorst, C., Latz, B., Kerz, T., Wisser, G., Schmidt, A., Schneider, A., et al. (2010). Prospective assessment of postoperative pain after craniotomy. J. Neurosurg. Anesthesiol. 22, 202–206. doi: 10.1097/ANA.0b013e3181df0600
Moser, P. (2020). “Out of control? Managing baseline variability in experimental studies with control groups,” in Good research practice in non-clinical pharmacology and biomedicine, eds A. Bespalov, M. C. Michel, and T. Steckler (Cham: Springer International Publishing), 101–117. doi: 10.1007/164_2019_280
Mukherjee, S., and Simasko, S. M. (2009). Chronic alcohol treatment in rats alters sleep by fragmenting periods of vigilance cycling in the light period with extended wakenings. Behav. Brain Res. 198, 113–124. doi: 10.1016/j.bbr.2008.10.026
Murai, N., Kondo, Y., Akuzawa, S., Mihara, T., Shiraishi, N., Kakimoto, S., et al. (2019). A novel GABAB receptor positive allosteric modulator, ASP8062, exerts analgesic effects in a rat model of fibromyalgia. Eur. J. Pharmacol. 865:172750. doi: 10.1016/j.ejphar.2019.172750
Nehlig, A., Dufour, F., Klinger, M., Willing, L. B., Simpson, I. A., and Vannucci, S. J. (2009). The ketogenic diet has no effect on the expression of spike-and-wave discharges and nutrient transporters in genetic absence epilepsy rats from Strasbourg. J. Neurochem. 109, 207–213. doi: 10.1111/j.1471-4159.2009.05938.x
Njoku, I., Radabaugh, H. L., Nicholas, M. A., Kutash, L. A., O’Neil, D. A., Marshall, I. P., et al. (2019). Chronic treatment with galantamine rescues reversal learning in an attentional set-shifting test after experimental brain trauma. Exp. Neurol. 315, 32–41. doi: 10.1016/j.expneurol.2019.01.019
Nuki, Y., Tsou, T. L., Kurihara, C., Kanematsu, M., Kanematsu, Y., and Hashimoto, T. (2009). Elastase-induced intracranial aneurysms in hypertensive mice. Hypertension 54, 1337–1344. doi: 10.1161/HYPERTENSIONAHA.109.138297
O’Brien, C. B., Baghdoyan, H. A., and Lydic, R. (2019). Computer-based multitaper spectrogram program for electroencephalographic data. J. Vis. Exp. doi: 10.3791/60333
Ogun, M. N., Cetinkaya, A., and Beyazcicek, E. (2019). The effect of vortioxetine on penicillin-induced epileptiform activity in rats. Arq. Neuropsiquiatr. 77, 412–417. doi: 10.1590/0004-282x20190064
Okada, S., Katagiri, A., Saito, H., Lee, J., Ohara, K., Iinuma, T., et al. (2019). Functional involvement of nucleus tractus solitarii neurons projecting to the parabrachial nucleus in trigeminal neuropathic pain. J. Oral Sci. 61, 370–378. doi: 10.2334/josnusd.18-0355
Onyszchuk, G., LeVine, S. M., Brooks, W. M., and Berman, N. E. (2009). Post-acute pathological changes in the thalamus and internal capsule in aged mice following controlled cortical impact injury: A magnetic resonance imaging, iron histochemical, and glial immunohistochemical study. Neurosci. Lett. 452, 204–208. doi: 10.1016/j.neulet.2009.01.049
Ortiz-Cardona, J., and Bendo, A. A. (2007). Perioperative pain management in the neurosurgical patient. Anesthesiol. Clin. 25, 655–74, xi. doi: 10.1016/j.anclin.2007.05.003
Oshima, T., Lee, S., Sato, A., Oda, S., Hirasawa, H., and Yamashita, T. (2009). TNF-alpha contributes to axonal sprouting and functional recovery following traumatic brain injury. Brain Res. 1290, 102–110. doi: 10.1016/j.brainres.2009.07.022
Ouzzani, M., Hammady, H., Fedorowicz, Z., and Elmagarmid, A. (2016). Rayyan—a web and mobile app for systematic reviews. Syst. Rev. 5:210. doi: 10.1186/s13643-016-0384-4
Palmer, J. D., Sparrow, O. C., and Iannotti, F. (1994). Postoperative hematoma: A 5-year survey and identification of avoidable risk factors. Neurosurgery 35, 1061–4; discussion4–5. doi: 10.1097/00006123-199412000-00007
Park, H., You, N., Lee, J., and Suh, M. (2019). Longitudinal study of hemodynamics and dendritic membrane potential changes in the mouse cortex following a soft cranial window installation. Neurophotonics 6:015006. doi: 10.1117/1.NPh.6.1.015006
Peterson, N. C., Nunamaker, E. A., and Turner, P. V. (2017). To treat or not to treat: The effects of pain on experimental parameters. Comp. Med. 67, 469–482.
Pettibone, J. R., Yu, J. Y., Derman, R. C., Faust, T. W., Hughes, E. D., Filipiak, W. E., et al. (2019). Knock-in rat lines with Cre recombinase at the dopamine D1 and adenosine 2a receptor loci. eNeuro 6, 163–219. doi: 10.1523/ENEURO.0163-19.2019
Pfluger, P., Pinnell, R. C., Martini, N., and Hofmann, U. G. (2019). Chronically implanted microelectrodes cause c-fos expression along their trajectory. Front. Neurosci. 13:1367. doi: 10.3389/fnins.2019.01367
Potts, M. B., Rola, R., Claus, C. P., Ferriero, D. M., Fike, J. R., and Noble-Haeusslein, L. J. (2009). Glutathione peroxidase overexpression does not rescue impaired neurogenesis in the injured immature brain. J. Neurosci. Res. 87, 1848–1857. doi: 10.1002/jnr.21996
Prescott, M. J., and Lidster, K. (2017). Improving quality of science through better animal welfare: The NC3Rs strategy. Lab. Anim. 46, 152–156. doi: 10.1038/laban.1217
Qiao, S., Qian, Y., Xu, G., Luo, Q., and Zhang, Z. (2019). Long-term characterization of activated microglia/macrophages facilitating the development of experimental brain metastasis through intravital microscopic imaging. J. Neuroinflammation 16:4. doi: 10.1186/s12974-018-1389-9
Qing, W. G., Dong, Y. Q., Ping, T. Q., Lai, L. G., Fang, L. D., Min, H. W., et al. (2009). Brain edema after intracerebral hemorrhage in rats: The role of iron overload and aquaporin 4. J. Neurosurg. 110, 462–468. doi: 10.3171/2008.4.JNS17512
Rafiq, S., Steinbrüchel, D. A., Wanscher, M. J., Andersen, L. W., Navne, A., Lilleoer, N. B., et al. (2014). Multimodal analgesia versus traditional opiate based analgesia after cardiac surgery, a randomized controlled trial. J. Cardiothorac. Surg. 9:52. doi: 10.1186/1749-8090-9-52
Rahim, A. A., Wong, A. M., Howe, S. J., Buckley, S. M., Acosta-Saltos, A. D., Elston, K. E., et al. (2009). Efficient gene delivery to the adult and fetal CNS using pseudotyped non-integrating lentiviral vectors. Gene Ther. 16, 509–520. doi: 10.1038/gt.2008.186
Richardson, C. A., and Flecknell, P. A. (2005). Anaesthesia and post-operative analgesia following experimental surgery in laboratory rodents: Are we making progress? Altern. Lab. Anima. ATLA 33, 119–127. doi: 10.1177/026119290503300207
Rimoli, M. G., Russo, E., Cataldi, M., Citraro, R., Ambrosino, P., Melisi, D., et al. (2009). T-type channel blocking properties and antiabsence activity of two imidazo[1,2-b]pyridazine derivatives structurally related to indomethacin. Neuropharmacology 56, 637–646. doi: 10.1016/j.neuropharm.2008.11.003
Roiko, S. A., Harris, A. C., LeSage, M. G., Keyler, D. E., and Pentel, P. R. (2009). Passive immunization with a nicotine-specific monoclonal antibody decreases brain nicotine levels but does not precipitate withdrawal in nicotine-dependent rats. Pharmacol. Biochem. Behav. 93, 105–111. doi: 10.1016/j.pbb.2009.04.011
Romoli, M., Krashia, P., Sen, A., Franciotta, D., Gastaldi, M., Nobili, A., et al. (2019). Hippocampal epileptogenesis in autoimmune encephalitis. Ann. Clin. Transl. Neurol. 6, 2261–2269. doi: 10.1002/acn3.50919
Rudnick, N. D., Koehler, C., Picciotto, M. R., and Siegel, S. J. (2009). Role of beta2-containing nicotinic acetylcholine receptors in auditory event-related potentials. Psychopharmacology 202, 745–751. doi: 10.1007/s00213-008-1358-6
Russell, N. H., Black, R. T., Lee, N. N., Doperalski, A. E., Reeves, T. M., and Phillips, L. L. (2019). Time-dependent hemeoxygenase-1, lipocalin-2 and ferritin induction after non-contusion traumatic brain injury. Brain Res. 1725:146466. doi: 10.1016/j.brainres.2019.146466
Sa, J. M., Barbosa, R. M., Menani, J. V., De Luca, L. A. Jr., Colombari, E., and Almeida Colombari, D. S. (2019). Cardiovascular and hidroelectrolytic changes in rats fed with high-fat diet. Behav. Brain Res. 373:112075. doi: 10.1016/j.bbr.2019.112075
Sahin, D., Ilbay, G., Imal, M., Bozdogan, O., and Ates, N. (2009). Vagus nerve stimulation suppresses generalized seizure activity and seizure-triggered postictal cardiac rhythm changes in rats. Physiol. Res. 58, 345–350. doi: 10.33549/physiolres.931344
Saltaji, H., Armijo-Olivo, S., Cummings, G. G., Amin, M., da Costa, B. R., and Flores-Mir, C. (2018). Influence of blinding on treatment effect size estimate in randomized controlled trials of oral health interventions. BMC Med. Res. Methodol. 18:42. doi: 10.1186/s12874-018-0491-0
Samnick, S., Romeike, B. F., Lehmann, T., Israel, I., Rube, C., Mautes, A., et al. (2009). Efficacy of systemic radionuclide therapy with p-131I-iodo-L-phenylalanine combined with external beam photon irradiation in treating malignant gliomas. J. Nucl. Med. 50, 2025–2032. doi: 10.2967/jnumed.109.066548
Sarabia-Estrada, R., Cowan, A., Tyler, B. M., and Guarnieri, M. (2017). Association of nausea with buprenorphine analgesia for rats. Lab. Animl. 46, 242–244. doi: 10.1038/laban.1277
Sasaki, T., Takeda, Y., Taninishi, H., Arai, M., Shiraishi, K., and Morita, K. (2009). Dynamic changes in cortical NADH fluorescence in rat focal ischemia: Evaluation of the effects of hypothermia on propagation of peri-infarct depolarization by temporal and spatial analysis. Neurosci. Lett. 449, 61–65. doi: 10.1016/j.neulet.2008.10.054
Schei, J. L., Foust, A. J., Rojas, M. J., Navas, J. A., and Rector, D. M. (2009). State-dependent auditory evoked hemodynamic responses recorded optically with indwelling photodiodes. Appl. Opt. 48, D121–D129. doi: 10.1364/AO.48.00D121
Schmid, A. W., Lynch, M. A., and Herron, C. E. (2009). The effects of IL-1 receptor antagonist on beta amyloid mediated depression of LTP in the rat CA1 in vivo. Hippocampus 19, 670–676. doi: 10.1002/hipo.20542
Schug, S. A. (2006). Combination analgesia in 2005—a rational approach: Focus on paracetamol-tramadol. Clin. Rheumatol. 25(Suppl. 1), S16–S21. doi: 10.1007/s10067-006-0202-9
Schuler, B., Rettich, A., Vogel, J., Gassmann, M., and Arras, M. (2009). Optimized surgical techniques and postoperative care improve survival rates and permit accurate telemetric recording in exercising mice. BMC Vet. Res. 5:28. doi: 10.1186/1746-6148-5-28
Seiffert, I., van Dijk, R. M., Koska, I., Di Liberto, V., Moller, C., Palme, R., et al. (2019). Toward evidence-based severity assessment in rat models with repeated seizures: III. Electrical post-status epilepticus model. Epilepsia 60, 1539–1551. doi: 10.1111/epi.16095
Sekiya, T., Canlon, B., Viberg, A., Matsumoto, M., Kojima, K., Ono, K., et al. (2009). Selective vulnerability of adult cochlear nucleus neurons to de-afferentation by mechanical compression. Exp. Neurol. 218, 117–123. doi: 10.1016/j.expneurol.2009.04.014
Sharma, R., Rahi, S., and Mehan, S. (2019). Neuroprotective potential of solanesol in intracerebroventricular propionic acid induced experimental model of autism: Insights from behavioral and biochemical evidence. Toxicol. Rep. 6, 1164–1175. doi: 10.1016/j.toxrep.2019.10.019
Shaver, T. K., Ozga, J. E., Zhu, B., Anderson, K. G., Martens, K. M., and Vonder Haar, C. (2019). Long-term deficits in risky decision-making after traumatic brain injury on a rat analog of the Iowa gambling task. Brain Res. 1704, 103–113. doi: 10.1016/j.brainres.2018.10.004
Sher, F., van Dam, G., Boddeke, E., and Copray, S. (2009). Bioluminescence imaging of Olig2-neural stem cells reveals improved engraftment in a demyelination mouse model. Stem Cells 27, 1582–1591. doi: 10.1002/stem.76
Shiuchi, T., Miyatake, Y., Otsuka, A., Chikahisa, S., Sakaue, H., and Sei, H. (2019). Role of orexin in exercise-induced leptin sensitivity in the mediobasal hypothalamus of mice. Biochem. Biophys. Res. Commun. 514, 166–172. doi: 10.1016/j.bbrc.2019.04.145
Shultz, S. R., Macfabe, D. F., Martin, S., Jackson, J., Taylor, R., Boon, F., et al. (2009). Intracerebroventricular injections of the enteric bacterial metabolic product propionic acid impair cognition and sensorimotor ability in the long-evans rat: Further development of a rodent model of autism. Behav. Brain Res. 200, 33–41. doi: 10.1016/j.bbr.2008.12.023
Silvani, A., Bastianini, S., Berteotti, C., Franzini, C., Lenzi, P., Lo Martire, V., et al. (2009). Sleep modulates hypertension in leptin-deficient obese mice. Hypertension 53, 251–255. doi: 10.1161/HYPERTENSIONAHA.108.125542
Simader, E., Budinsky, L., Helbich, T. H., Sherif, C., Hoftberger, R., Kasprian, G., et al. (2019). Subarachnoid hemorrhage in rats—visualizing blood distribution in vivo using gadolinium-enhanced magnetic resonance imaging: Technical note. J. Neurosci. Methods 325:108370. doi: 10.1016/j.jneumeth.2019.108370
Sinton, C. M., Kovakkattu, D., and Friese, R. S. (2009). Validation of a novel method to interrupt sleep in the mouse. J. Neurosci. Methods 184, 71–78. doi: 10.1016/j.jneumeth.2009.07.026
Slezia, A., Proctor, C. M., Kaszas, A., Malliaras, G. G., and Williamson, A. (2019). Electrophoretic delivery of gamma-aminobutyric acid (GABA) into epileptic focus prevents seizures in mice. J. Vis. Exp. doi: 10.3791/59268-v
Song, G., and Poon, C. S. (2009). Lateral parabrachial nucleus mediates shortening of expiration during hypoxia. Respir. Physiol. Neurobiol. 165, 1–8. doi: 10.1016/j.resp.2008.10.007
Souza, G., Stornetta, R. L., Stornetta, D. S., Abbott, S. B. G., and Guyenet, P. G. (2019). Contribution of the retrotrapezoid nucleus and carotid bodies to hypercapnia- and hypoxia-induced arousal from sleep. J. Neurosci. 39, 9725–9737. doi: 10.1523/JNEUROSCI.1268-19.2019
Stanchi, F., Matsumoto, K., and Gerhardt, H. (2019). Imaging glioma progression by intravital microscopy. Methods Mol. Biol. 1862, 227–243. doi: 10.1007/978-1-4939-8769-6_16
Stanojlovic, M., Pallais Yllescas, J. P. Jr., Mavanji, V., and Kotz, C. (2019). Chemogenetic activation of orexin/hypocretin neurons ameliorates aging-induced changes in behavior and energy expenditure. Am. J. Physiol. Regul. Integr. Comp. Physiol. 316, R571–R583. doi: 10.1152/ajpregu.00383.2018
Stokes, E. L., Flecknell, P. A., and Richardson, C. A. (2009). Reported analgesic and anaesthetic administration to rodents undergoing experimental surgical procedures. Lab. Anim. 43, 149–154. doi: 10.1258/la.2008.008020
Sun, W., Suzuki, K., Toptunov, D., Stoyanov, S., Yuzaki, M., Khiroug, L., et al. (2019). In vivo two-photon imaging of anesthesia-specific alterations in microglial surveillance and photodamage-directed motility in mouse cortex. Front. Neurosci. 13:421. doi: 10.3389/fnins.2019.00421
Suzuki, T., Takizawa, T., Kamio, Y., Qin, T., Hashimoto, T., Fujii, Y., et al. (2019). Noninvasive vagus nerve stimulation prevents ruptures and improves outcomes in a model of intracranial aneurysm in mice. Stroke 50, 1216–1223. doi: 10.1161/STROKEAHA.118.023928
Szonyi, A., Zicho, K., Barth, A. M., Gonczi, R. T., Schlingloff, D., Torok, B., et al. (2019). Median raphe controls acquisition of negative experience in the mouse. Science 366:eaay8746. doi: 10.1126/science.aay8746
Takahashi, M., Vattanajun, A., Umeda, T., Isa, K., and Isa, T. (2009). Large-scale reorganization of corticofugal fibers after neonatal hemidecortication for functional restoration of forelimb movements. Eur. J. Neurosci. 30, 1878–1887. doi: 10.1111/j.1460-9568.2009.06989.x
Tallett, A. J., Blundell, J. E., and Rodgers, R. J. (2009). Effects of acute low-dose combined treatment with naloxone and AM 251 on food intake, feeding behaviour and weight gain in rats. Pharmacol. Biochem. Behav. 91, 358–366. doi: 10.1016/j.pbb.2008.08.007
Tanida, M., Shen, J., and Nagai, K. (2009). Possible role of the histaminergic system in autonomic and cardiovascular responses to neuropeptide Y. Neuropeptides 43, 21–29. doi: 10.1016/j.npep.2008.09.007
Tchekalarova, J., Kubova, H., and Mares, P. (2009). Postnatal caffeine treatment affects differently two pentylenetetrazol seizure models in rats. Seizure 18, 463–469. doi: 10.1016/j.seizure.2009.04.002
Thapa, P., and Euasobhon, P. (2018). Chronic postsurgical pain: Current evidence for prevention and management. Korean J. Pain 31, 155–173. doi: 10.3344/kjp.2018.31.3.155
Thomas, M., Tyers, P., Lazic, S. E., Caldwell, M. A., Barker, R. A., Beazley, L., et al. (2009). Graft outcomes influenced by co-expression of Pax7 in graft and host tissue. J. Anat. 214, 396–405. doi: 10.1111/j.1469-7580.2009.01049.x
Tomov, N., Surchev, L., Wiedenmann, C., Dobrossy, M., and Nikkhah, G. (2019). Roscovitine, an experimental CDK5 inhibitor, causes delayed suppression of microglial, but not astroglial recruitment around intracerebral dopaminergic grafts. Exp. Neurol. 318, 135–144. doi: 10.1016/j.expneurol.2019.04.013
Topchiy, I. A., Wood, R. M., Peterson, B., Navas, J. A., Rojas, M. J., and Rector, D. M. (2009). Conditioned lick behavior and evoked responses using whisker twitches in head restrained rats. Behav. Brain Res. 197, 16–23. doi: 10.1016/j.bbr.2008.07.032
Touzani, K., Bodnar, R. J., and Sclafani, A. (2009). Dopamine D1-like receptor antagonism in amygdala impairs the acquisition of glucose-conditioned flavor preference in rats. Eur. J. Neurosci. 30, 289–298. doi: 10.1111/j.1460-9568.2009.06829.x
Tsanov, M., and Manahan-Vaughan, D. (2009). Long-term plasticity is proportional to theta-activity. PLoS One 4:e5850. doi: 10.1371/journal.pone.0005850
Tsaousi, G. G., Logan, S. W., and Bilotta, F. (2017). Postoperative pain control following craniotomy: A systematic review of recent clinical literature. Pain Pract. 17, 968–981. doi: 10.1111/papr.12548
Vadivelu, N., Kai, A. M., Tran, D., Kodumudi, G., Legler, A., and Ayrian, E. (2016). Options for perioperative pain management in neurosurgery. J. Pain Res. 9, 37–47. doi: 10.2147/JPR.S85782
Vadivelu, N., Mitra, S., Schermer, E., Kodumudi, V., Kaye, A. D., and Urman, R. D. (2014). Preventive analgesia for postoperative pain control: A broader concept. Local Reg. Anesth. 7, 17–22. doi: 10.2147/LRA.S62160
Vallapu, S., Panda, N. B., Samagh, N., and Bharti, N. (2018). Efficacy of dexmedetomidine as an adjuvant to local anesthetic agent in scalp block and scalp infiltration to control postcraniotomy pain: A double-blind randomized trial. J. Neurosci. Rural Pract. 9, 73–79. doi: 10.4103/jnrp.jnrp_310_17
Villa-Cedillo, S. A., Soto-Dominguez, A., Rodriguez-Rocha, H., Garcia-Garcia, A., de Jesus Loera-Arias, M., Rivera-Chavez, L. F., et al. (2019). The mRVG-9R peptide as a potential therapeutic vector to the central nervous system cells. Cell Biol. Int. 43, 809–819. doi: 10.1002/cbin.11161
Villasana, L. E., Peters, A., McCallum, R., Liu, C., and Schnell, E. (2019). Diazepam inhibits post-traumatic neurogenesis and blocks aberrant dendritic development. J. Neurotrauma 36, 2454–2467. doi: 10.1089/neu.2018.6162
Wagner, A. K., Sokoloski, J. E., Chen, X., Harun, R., Clossin, D. P., Khan, A. S., et al. (2009). Controlled cortical impact injury influences methylphenidate-induced changes in striatal dopamine neurotransmission. J. Neurochem. 110, 801–810. doi: 10.1111/j.1471-4159.2009.06155.x
Wan, Z., Ristagno, G., Sun, S., Li, Y., Weil, M. H., and Tang, W. (2009). Preserved cerebral microcirculation during cardiogenic shock. Crit. Care Med. 37, 2333–2337. doi: 10.1097/CCM.0b013e3181a3a97b
Wang, S., Levesque, M., and Avoli, M. (2019f). Transition from status epilepticus to interictal spiking in a rodent model of mesial temporal epilepsy. Epilepsy Res. 152, 73–76. doi: 10.1016/j.eplepsyres.2019.03.005
Wang, T., Wang, H. L., Liu, R., Wang, H., Zhang, Y., Sun, Y. X., et al. (2019g). Early-life stress alters sleep structure and the excitatory-inhibitory balance in the nucleus accumbens in aged mice. Chin. Med. J. 132, 1582–1590. doi: 10.1097/CM9.0000000000000279
Wang, G., Ding, L., Gao, C., Zhang, N., Gan, D., Sun, Y., et al. (2019a). Neuroprotective effect of l-serine against white matter demyelination by harnessing and modulating inflammation in mice. Neuropharmacology 146, 39–49. doi: 10.1016/j.neuropharm.2018.11.025
Wang, X., Yang, X. L., Kong, W. L., Zeng, M. L., Shao, L., Jiang, G. T., et al. (2019h). TRPV1 translocated to astrocytic membrane to promote migration and inflammatory infiltration thus promotes epilepsy after hypoxic ischemia in immature brain. J. Neuroinflammation 16:214. doi: 10.1186/s12974-019-1618-x
Wang, J., Lombardi, F., Zhang, X., Anaclet, C., and Ivanov, P. C. (2019b). Non-equilibrium critical dynamics of bursts in theta and delta rhythms as fundamental characteristic of sleep and wake micro-architecture. PLoS Comput. Biol. 15:e1007268. doi: 10.1371/journal.pcbi.1007268
Wang, L., Shi, H., Kang, Y., and Guofeng, W. (2019c). Hippocampal low-frequency stimulation improves cognitive function in pharmacoresistant epileptic rats. Epilepsy Res. 168:106194. doi: 10.1016/j.eplepsyres.2019.106194
Wang, L., Song, L. F., Chen, X. Y., Ma, Y. L., Suo, J. F., Shi, J. H., et al. (2019d). MiR-181b inhibits P38/JNK signaling pathway to attenuate autophagy and apoptosis in juvenile rats with kainic acid-induced epilepsy via targeting TLR4. CNS Neurosci. Ther. 25, 112–122. doi: 10.1111/cns.12991
Wang, M. J., Jiang, L., Chen, H. S., and Cheng, L. (2019e). Levetiracetam protects against cognitive impairment of subthreshold convulsant discharge model rats by activating protein kinase C (PKC)-growth-associated protein 43 (GAP-43)-calmodulin-dependent protein kinase (CaMK) signal transduction pathway. Med. Sci. Monit. 25, 4627–4638. doi: 10.12659/MSM.913542
Wen, Y., Wu, K., Xie, Y., Dan, W., Zhan, Y., and Shi, Q. (2019). Inhibitory effects of glucagon-like peptide-1 receptor on epilepsy. Biochem. Biophys. Res. Commun. 511, 79–86. doi: 10.1016/j.bbrc.2019.02.028
Wen, L., You, W., Wang, Y., Zhu, Y., Wang, H., and Yang, X. (2019). Investigating Alterations in caecum microbiota after traumatic brain injury in mice. J. Vis. Exp. doi: 10.3791/59410-v
White, P. F. (2008). Multimodal analgesia: Its role in preventing postoperative pain. Curr. Opin. Investig. Drugs 9, 76–82.
White, P. F., and Kehlet, H. (2010). Improving postoperative pain management: What are the unresolved issues? Anesthesiology 112, 220–225. doi: 10.1097/ALN.0b013e3181c6316e
Wigren, H. K., Rytkonen, K. M., and Porkka-Heiskanen, T. (2009). Basal forebrain lactate release and promotion of cortical arousal during prolonged waking is attenuated in aging. J. Neurosci. 29, 11698–11707. doi: 10.1523/JNEUROSCI.5773-08.2009
Worthen, D. R., Bence, A. K., Stables, J. P., and Crooks, P. A. (2009). In vivo evaluation of diaminodiphenyls: Anticonvulsant agents with minimal acute neurotoxicity. Bioorg. Med. Chem. Lett. 19, 5012–5015. doi: 10.1016/j.bmcl.2009.07.059
Xu, S. Y., Liu, M., Gao, Y., Cao, Y., Bao, J. G., Lin, Y. Y., et al. (2019). Acute histopathological responses and long-term behavioral outcomes in mice with graded controlled cortical impact injury. Neural Regen. Res. 14, 997–1003. doi: 10.4103/1673-5374.250579
Xue, M., Hollenberg, M. D., Demchuk, A., and Yong, V. W. (2009). Relative importance of proteinase-activated receptor-1 versus matrix metalloproteinases in intracerebral hemorrhage-mediated neurotoxicity in mice. Stroke 40, 2199–2204. doi: 10.1161/STROKEAHA.108.540393
Yang, Z., Zhu, T., Mondello, S., Akel, M., Wong, A. T., Kothari, I. M., et al. (2019). Serum-based phospho-neurofilament-heavy protein as theranostic biomarker in three models of traumatic brain injury: An operation brain trauma therapy study. J. Neurotrauma 36, 348–359. doi: 10.1089/neu.2017.5586
Yeung, J. H. Y., Palpagama, T. H., Tate, W. P., Peppercorn, K., Waldvogel, H. J., Faull, R. L. M., et al. (2019). The acute effects of amyloid-beta1-42 on glutamatergic receptor and transporter expression in the mouse hippocampus. Front. Neurosci. 13:1427. doi: 10.3389/fnins.2019.01427
Yoon, S. R., Jo, Y. J., Yang, S., Kim, Y. B., Nam, S. Y., Kim, H. C., et al. (2009). Sanjoinine A isolated from semen zizyphi spinosi protects against kainic acid-induced convulsions. Arch. Pharm. Res. 32, 1515–1523. doi: 10.1007/s12272-009-2103-3
Young, C. K., Koke, S. J., Kiss, Z. H., and Bland, B. H. (2009). Deep brain stimulation of the posterior hypothalamic nucleus reverses akinesia in bilaterally 6-hydroxydopamine-lesioned rats. Neuroscience 162, 1–4. doi: 10.1016/j.neuroscience.2009.04.053
Yu, D., Yin, S., and Chen, Z. (2009). Effect of baclofen on neuronal activity in the medial vestibular nucleus after unilateral surgical labyrinthectomy in rats. Acta Otolaryngol. 129, 735–740. doi: 10.1080/00016480802369286
Yurek, D. M., Flectcher, A. M., Kowalczyk, T. H., Padegimas, L., and Cooper, M. J. (2009). Compacted DNA nanoparticle gene transfer of GDNF to the rat striatum enhances the survival of grafted fetal dopamine neurons. Cell Transplant. 18, 1183–1196. doi: 10.3727/096368909X12483162196881
Zeng, L. H., Rensing, N. R., and Wong, M. (2009). The mammalian target of rapamycin signaling pathway mediates epileptogenesis in a model of temporal lobe epilepsy. J. Neurosci. 29, 6964–6972. doi: 10.1523/JNEUROSCI.0066-09.2009
Zhang, Y., Fu, B., Liu, C., Yu, S., Luo, T., Zhang, L., et al. (2019c). Activation of noradrenergic terminals in the reticular thalamus delays arousal from propofol anesthesia in mice. FASEB J. 33, 7252–7260. doi: 10.1096/fj.201802164RR
Zhang, Y., Chopp, M., Rex, C. S., Simmon, V. F., Sarraf, S. T., Zhang, Z. G., et al. (2019b). A small molecule spinogenic compound enhances functional outcome and dendritic spine plasticity in a rat model of traumatic brain injury. J. Neurotrauma 36, 589–600. doi: 10.1089/neu.2018.5790
Zhang, F., Wang, F., Yue, L., Zhang, H., Peng, W., and Hu, L. (2019a). Cross-species investigation on resting state electroencephalogram. Brain Topogr. 32, 808–824. doi: 10.1007/s10548-019-00723-x
Keywords: neuroscience, multimodal analgesia, pain, animal welfare, refinement, surgery, postoperative pain, neurosurgery
Citation: King H, Reiber M, Philippi V, Stirling H, Aulehner K, Bankstahl M, Bleich A, Buchecker V, Glasenapp A, Jirkof P, Miljanovic N, Schönhoff K, von Schumann L, Leenaars C and Potschka H (2023) Anesthesia and analgesia for experimental craniotomy in mice and rats: a systematic scoping review comparing the years 2009 and 2019. Front. Neurosci. 17:1143109. doi: 10.3389/fnins.2023.1143109
Received: 12 January 2023; Accepted: 27 March 2023;
Published: 03 May 2023.
Edited by:
Nick Andrews, Salk Institute for Biological Studies, United StatesReviewed by:
Bamidele Victor Owoyele, University of Ilorin, NigeriaJia Yan, Shanghai Jiao Tong University, China
Copyright © 2023 King, Reiber, Philippi, Stirling, Aulehner, Bankstahl, Bleich, Buchecker, Glasenapp, Jirkof, Miljanovic, Schönhoff, von Schumann, Leenaars and Potschka. This is an open-access article distributed under the terms of the Creative Commons Attribution License (CC BY). The use, distribution or reproduction in other forums is permitted, provided the original author(s) and the copyright owner(s) are credited and that the original publication in this journal is cited, in accordance with accepted academic practice. No use, distribution or reproduction is permitted which does not comply with these terms.
*Correspondence: Heidrun Potschka, cG90c2Noa2FAcGhhcm10b3gudmV0bWVkLnVuaS1tdWVuY2hlbi5kZQ==
†These authors share last authorship