- 1Department of Rehabilitation Medicine, The People’s Hospital of Suzhou New District, Suzhou, China
- 2Department of Rehabilitation Medicine, Fudan University Huashan Hospital, Shanghai, China
- 3National Center for Neurological Disorders, Shanghai, China
- 4National Clinical Research Center for Aging and Medicine, Fudan University Huashan Hospital, Shanghai, China
Background: Respiratory muscle ultrasound is a widely available, highly feasible technique that can be used to study the contribution of the individual respiratory muscles related to respiratory dysfunction. Stroke disrupts multiple functions, and the respiratory function is often significantly decreased in stroke patients.
Method: A search of the MEDLINE, Web of Science, and PubMed databases was conducted. We identified studies measuring respiratory muscles in healthy and patients by ultrasonography. Two reviewers independently extracted and documented data regarding to the criteria. Data were extracted including participant demographics, ultrasonography evaluation protocol, subject population, reference values, etc.
Result: A total of 1954 participants from 39 studies were included. Among them, there were 1,135 participants from 19 studies on diaphragm, 259 participants from 6 studies on extra-diaphragmatic inspiratory muscles, and 560 participants from 14 studies on abdominal expiratory muscles. The ultrasonic evaluation of diaphragm and abdominal expiratory muscle thickness had a relatively typically approach, while, extra-diaphragmatic inspiratory muscles were mainly used in ICU that lack of a consistent paradigm.
Conclusion: Diaphragm and expiratory muscle ultrasound has been widely used in the assessment of respiratory muscle function. On the contrary, there is not enough evidence to assess extra-diaphragmatic inspiratory muscles by ultrasound. In addition, the thickness of the diaphragm on the hemiplegic side was lower than that on the non-hemiplegic side in stroke patients. For internal oblique muscle (IO), rectus abdominis muscle (RA), transversus abdominis muscle (TrA), and external oblique muscle (EO), most studies showed that the thickness on the hemiplegic side was lower than that on the non-hemiplegic side.
Clinical Trial Registration: The protocol of this review was registered in the PROSPERO database (CRD42022352901).
1. Introduction
The respiratory muscle pump consists of three primary groups controlling ventilation: the primary muscle of inspiration, the accessory inspiratory, and the expiratory muscles (Shi et al., 2021). The primary muscle of inspiration is the diaphragm, a thin dome-shaped muscle positioned between the chest and abdomen. As the most important respiratory muscle, the diaphragm contributes 60–80% of the ventilation needs of the human body. However, the diaphragm is not the only inspiratory muscle involved in ventilation. When the load imposed on the diaphragm increases, the accessory inspiratory muscles, such as the parasternal intercostal muscles, external intercostal muscles, scalene muscles, and sternocleidomastoid muscles, are recruited to assist in inspiration (Tuinman et al., 2020). With further loading, the expiratory muscles are activated in a fixed hierarchy to assist expiration (Shi et al., 2019). The role of expiratory muscle includes reducing end-expiratory lung volume, reducing transpulmonary pressure, and increasing inspiratory muscle volume (Shi et al., 2019). There are also studies (Dres et al., 2020) showing that the muscle fibers of the parasternal intercostal muscles contract during inspiration, which expands the thoracic cavity, thereby increasing the tidal volume. Generally, during tidal ventilation, the diaphragm works in synergy with the scalene and external intercostal muscles to trigger inspiration, as well as with the dilator muscles of the upper airway. In cases of respiratory distress, the sternocleidomastoid muscles and the trapezius are also recruited (Vivier and Mekontso Dessap, 2020).
Ultrasonography can assess the mechanics, thickness, and strength of all the respiratory muscles (Matamis et al., 2013), and it may be possible to provide valuable information in this context to complement clinical examination. Recent studies proved that ultrasound permits the quantitative assessment of the excursion and thickness of the respiratory muscles to quantify their function (Cala et al., 1998; Sferrazza Papa et al., 2016; Yoshida et al., 2019; Bedewi et al., 2021; Kang et al., 2021). Different ultrasonic techniques have been validated through several studies (Adigozali et al., 2016; Tahan et al., 2016; Bedewi et al., 2021). Stroke disrupts multiple functions (Benditt and Boitano, 2013). The respiratory function is often significantly decreased in stroke patients, and the respiratory intensity is only about 50% of the normal population. The respiratory dysfunction can be attributed to the affected respiratory central nervous system and respiratory muscles (Rochester and Mohsenin, 2002; Jandt et al., 2011). In this context, the present review has two objectives. The first is to review the ultrasound assessment of respiratory muscles. The second objective is the clinical application of respiratory muscle ultrasound in stroke patients.
2. Methods
2.1. Selection of studies
The review was according to the PRISMA 2020 flow diagram (Figure 1 and Supplementary material 3). We intended to identify studies measuring respiratory muscles in healthy and patients by ultrasonography. Based on the suggestions of thoracic ultrasound from European Respiratory Society Statement (Laursen et al., 2021) and previous review on ultrasonography measurement (Sferrazza Papa et al., 2016), we chose studies based on the following criteria: (1) Study subjects were healthy or patients with respiratory muscle disorders for various causes, the subjects included in the part of the clinical application were stroke patients. (2) The ultrasonography evaluation of the respiratory muscle was clearly described with integrated protocol, the ultrasound equipment, experience of the operator, probe frequency selection, subject’s measurement position, selection of measuring points/ultrasound probe placement position, respiratory status during measurement, and whether the subject cooperated, and respiratory muscle’s values were recorded. (3) All included studies were published in English with full text (English articles that were not published in full text were removed), including original studies and clinical trials.
2.2. Data source and search strategy
We searched articles published in the MEDLINE, Web of Science, and PubMed databases with no date restrictions up to 19 November 2022 (Supplementary material 1). The following keywords were used: Respiratory muscle ultrasonography-related words: ultrasonography, ultrasound, echotomography, sonography, echography, diaphragm, respiratory muscles, ventilatory muscles, and intercostal muscles based on title, abstract, and MeSH terms, and trapezius, sternocleidomastoid muscles, scalene muscles, parasternal intercostal muscle, inspiratory muscle, and expiratory muscle based on title and abstract. Stroke-related words were stroke, hemiplegia, cerebrovascular, CVA, apoplexy, vascular, brain, cerebral, intracerebral, hemorrhage, infarct, and ischemia based on title, abstract, and MeSH terms. The search strategy was created based on the PICO strategy: P (patient)—adults (healthy or diseases); I (intervention)—ultrasonography of the respiratory muscles (e.g., diaphragm, extra-diaphragmatic inspiratory muscles, abdominal muscles); C (comparator)—none; O (outcomes)—methodology and reference value.
2.3. Literature review and data extraction
Two review authors independently read the titles and abstracts of all literatures searched by the databases. Unified standards were used to extract relevant information from the full text of articles that met the inclusions. Data were extracted including participant demographics, ultrasonography evaluation protocol, subject population, reference values, etc.
3. Ultrasonography evaluation of respiratory muscles
3.1. Inspiratory muscle
3.1.1. Diaphragm
The diaphragm is a dome-shaped, fibromuscular partition between the thoracic and abdominal cavities (Gerscovich et al., 2001) that composed of four components: the transverse septum, pleuroperitoneal folds, esophageal mesentery, and muscular body wall laterally that separates the chest from the abdominal cavity (Sarwal et al., 2013). It is the most important inspiratory muscle and plays a major role in maintaining ventilation (Sferrazza Papa et al., 2016). Diaphragmatic ultrasound has been widely used in healthy individuals (Ueki et al., 1995) and clinical practice (Sferrazza Papa et al., 2016), and a wide range of normal and abnormal values has been reported (Gottesman and McCool, 1997; Boussuges et al., 2009; Sferrazza Papa et al., 2016). In general, there are two major forms of ultrasonographic assessment of the diaphragm: diaphragm excursion and thickness (Supinski et al., 2018).
Diaphragm excursion measurement (Table 1): 2–5 MHz transducer with M-mode ultrasonography is used to measure the diaphragm excursion. The participants are in the supine or standing position during ultrasonic measurement. Usually, the liver is used as a window on the right side, and the diaphragm is examined from the anterior subcostal approach (the right costal margin between the midclavicular and anterior axillary lines). While the spleen is used for the left side, the diaphragm was examined from a subcostal or low intercostal approach (between the anterior axillary and mid-axillary lines). One study has shown that supine position is preferred for the study of diaphragmatic excursion because of less variability in observations (Gerscovich et al., 2001). Visualization of the left diaphragm is more difficult because of the smaller window of the spleen, but can be facilitated by a more coronal approach and by paralleling the ribs (Sarwal et al., 2013). In addition to the common approaches described, several other approaches have been showed in previous studies. Among them, the posterior subcostal approach may not be practical in critically ill or mechanically ventilated patients (Fedullo et al., 1992). However, the subxiphoid approach provides another option for measuring excursion, and it is particularly useful in children (Chavhan et al., 2010). The excursion of diaphragm is usually measured during quiet breathing, deep breathing, or the sniff test.
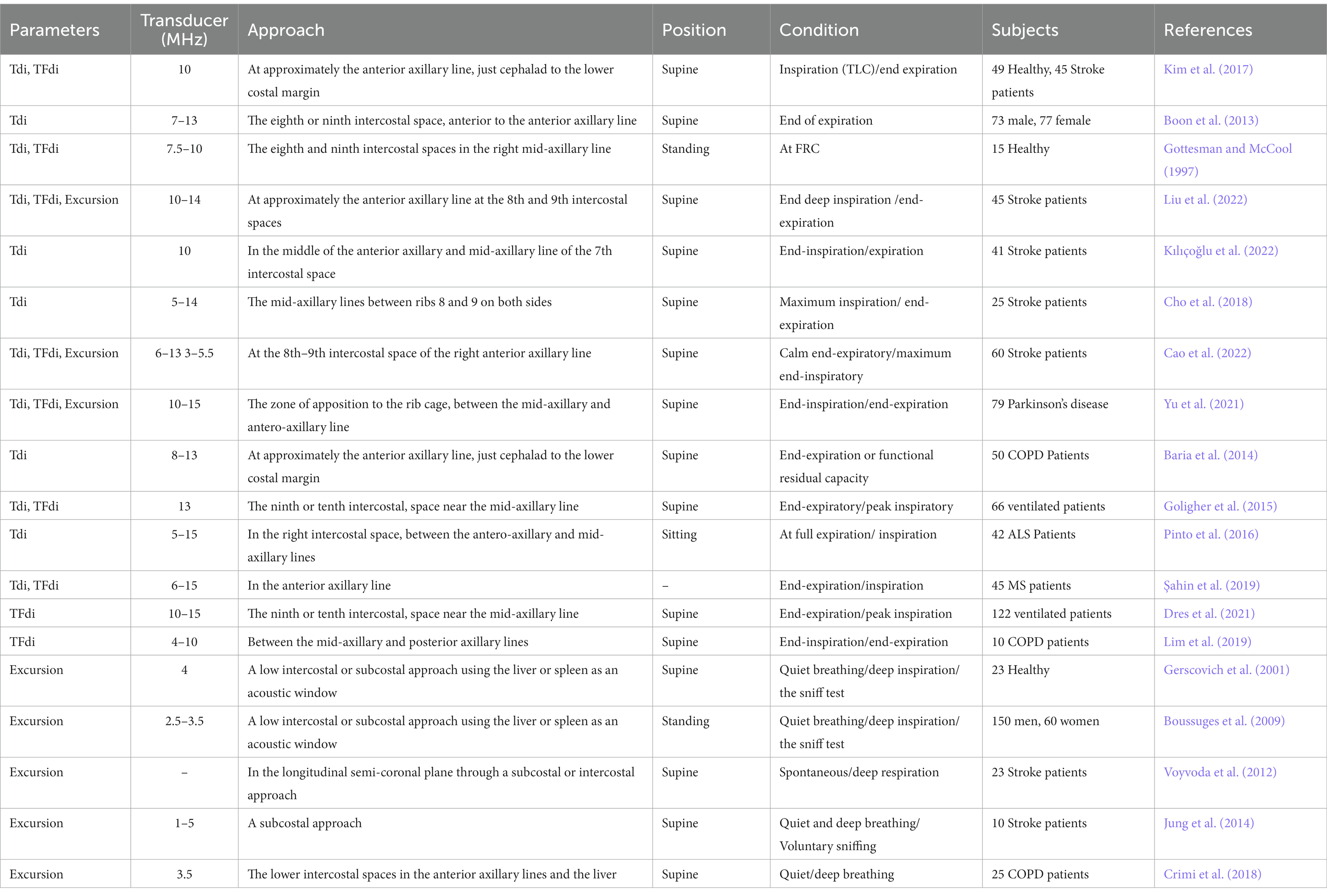
Table 1. Selected studies providing direct visualization of ultrasonographic assessment of the diaphragm.
Diaphragm thickness measurement (Table 1): With the participants in the standing, semi-recumbent, or supine position, 7–13 or 6–15 MHz linear-array transducer with B-mode or M-mode ultrasonography is used to measure the diaphragm thickness at the zone of apposition during inspiration or expiration. The probe is positioned at approximately the anterior axillary line or just cephalad to the lower costal margin at the eighth and ninth intercostal spaces. The diaphragm can be visualized as a three-layered structure consisting of a relatively non-echogenic muscular layer bounded by echogenic membranes of the peritoneum and diaphragmatic pleura with the probe perpendicular to two ribs (Supinski et al., 2018). According to the diaphragm thickness at the end-inspiration and end-expiration, the thickening fraction of the diaphragm (TFdi) can be calculated by the formula. TFdi = (end-inspiratory thickness - end-expiratory thickness)/end-expiratory thickness × 100%. Thickening fraction (TFdi) reflects on tractile activity that can be used to assess muscle function (Wait et al., 1989; Goligher et al., 2015). The ultrasound criteria of diaphragm thickness < 2.0 mm and thickening fraction (TFdi) < 20% was diagnostic of diaphragm paralysis in previous research (Gottesman and McCool, 1997).
3.1.2. Extra-diaphragmatic inspiratory muscles
3.1.2.1. Parasternal intercostal muscle and intercostal muscles
Together with the diaphragm, extra-diaphragmatic inspiratory muscles participate in the generation of the tidal volume (Formenti et al., 2020). The intercostal muscles can be directly visualized between the ribs (Laursen et al., 2021) and the European Respiratory Society recommends ultrasound monitoring of the intercostal muscles to assess respiratory function (Laveneziana et al., 2019). The intercostal muscles are composed of three thin layers of muscle fibers occupying each of the intercostal spaces (Formenti et al., 2020). The outer layer is external intercostal; in contrast, the layer is internal intercostal (De Troyer et al., 2005), and the inner layer is the innermost intercostal muscle (Estenne et al., 1985). The intercostal spaces contain two layers of intercostal muscle in their lateral portion but a single layer in their ventral and sometimes in their dorsal portions. Between the sternum and the chondrocostal junctions, the external intercostals are replaced by a fibrous aponeurosis, and this portion of the internal intercostals on the ventral side is conventionally called the Parasternal intercostals (De Troyer et al., 2005). The inspiratory contraction of the Parasternal intercostal muscle involves muscle shortening, acting to elevate the rib cage and expand the lung (Cala et al., 1998). Because their mass remains constant, increases in thickness can be observed using ultrasound imaging during inspiration (Vivier and Mekontso Dessap, 2020). Ultrasound has been used to investigate parasternal intercostal muscles in the easily accessible anterior parasternal region (Cala et al., 1998; Diab et al., 1998). Unlike the parasternal intercostal muscles, in the lateral and in the posterior part of the intercostal space, the internal and external intercostal muscles often overlap, making the ultrasound detection of both muscle layers impossible (Formenti et al., 2020). Therefore, ultrasonography of the intercostal muscles generally measures both the internal and external intercostal muscles. The extra-diaphragmatic respiratory muscles recruitment is a mechanism of compensation that can be activated in presence of diaphragm dysfunction (Dres et al., 2020). The parasternal intercostal muscle, as the main auxiliary inspiratory muscle, has a broad application prospect in mechanically ventilated patients, especially those with diaphragmatic dysfunction. The extra-diaphragmatic respiratory muscles recruitment is a mechanism of compensation that can be activated in presence of diaphragm dysfunction (Dres et al., 2020). The parasternal intercostal muscle, as the main auxiliary inspiratory muscle, has a broad application prospect in mechanically ventilated patients, especially those with diaphragmatic dysfunction.
Parasternal intercostal muscle thickness measurement (Table 2). Parasternal intercostal muscle ultrasound is performed with a 6–14 or 10–15 MHz linear-array transducer positioned in cranio-caudal direction at the second intercostal space, approximately 3–5 cm or 6–8 cm lateral to the sternal edge with a window visualizing between the 2nd and the 3rd rib. The supine position is usually used, and some studies used the participant at 45°. The second parasternal intercostal muscle was identified as a three-layered biconcave structure: two linear hyperechoic membranes running, respectively, from the anterior and posterior aspects of the adjoining ribs, and a medial portion with muscle echotexture. Using B-Mode or M-mode, the thickness of the parasternal intercostal muscle was measured on frozen images at end expiration and at peak inspiration during tidal breathing or total lung capacity. Change in thickness determined the thickening fraction of the parasternal intercostal muscle (TFic) as follows: TFic = (end-inspiration thickness – end-expiratory thickness)/end-expiratory thickness×100% (Dres et al., 2020, 2021). Previous research showed that a value of TFic less than 10%, associated with a TFdi greater than 20% during mechanical ventilation indicates a successful weaning trial (Formenti et al., 2020).
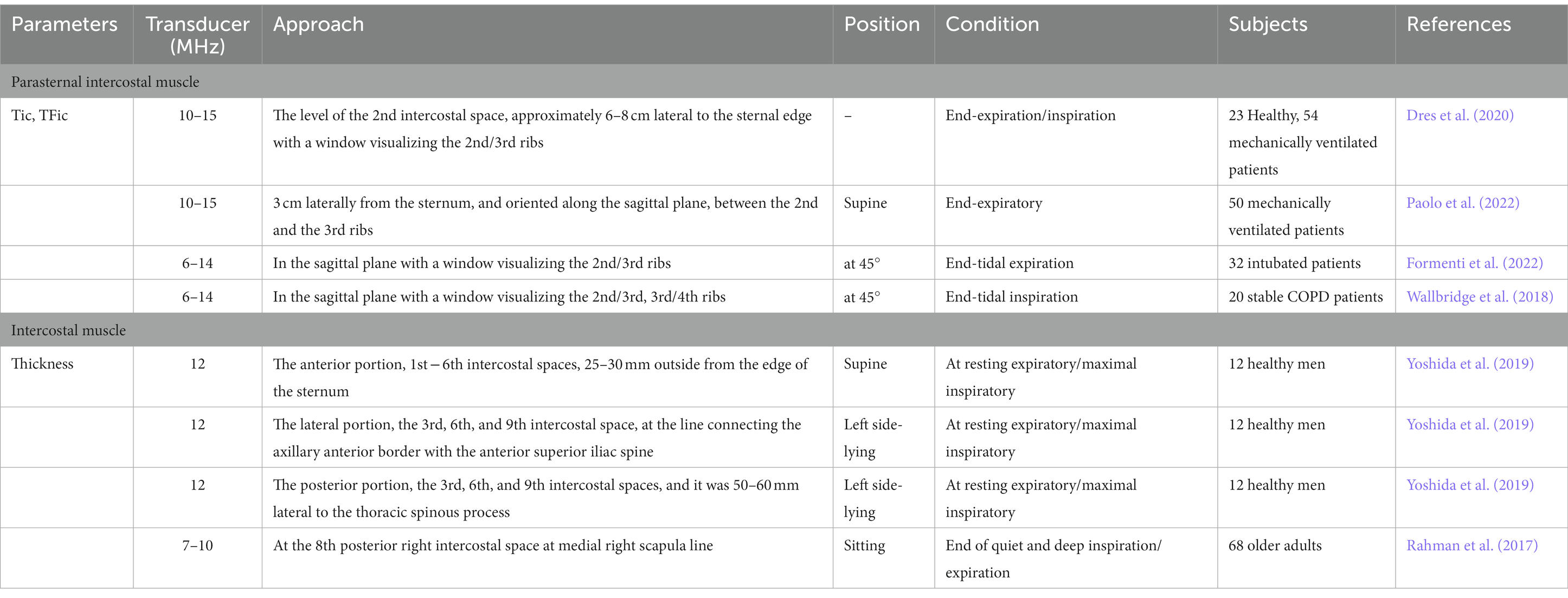
Table 2. Selected studies providing direct visualization of ultrasonographic assessment of the parasternal intercostal muscle and intercostal muscles.
Intercostal muscles thickness measurement (Table 2): Using B-mode ultrasound with 7–12 Hz linear probe to measure contractions of the intercostal muscle. The participants’ measurement used the supine position when measuring the anterior intercostal space, while the left side-lying position was used in the right lateral intercostal space and the posterior intercostal space, and the other research chose the sitting position. The measurement of anterior part of the intercostal muscle was the 1st − 6th intercostal spaces and 25–30 mm outside from the right edge of the sternum. The lateral part was the 3rd, 6th, and 9th intercostal space, at the line connecting the axillary anterior border with the anterior superior iliac spine. The posterior part was the 3rd, 6th, and 9th intercostal spaces, and it was 50–60 mm lateral to the thoracic spinous process. To measure the 8th posterior intercostal space, place the ultrasound probe at medial scapula line. Participants were in a supine position and the probe was placed at the level of the mid-axillary line and measurements were made at the 5th and 6th intercostal space (Pietton et al., 2021). One study determined the area of the intercostal muscles at maximal inhalation in one adult person and it was found that measurements at maximal inhalation were more accurate than those taken at maximal exhalation (Diab et al., 1998). Summary of the literature, previous studies have focused on the right intercostal muscles, the structure and contractile function of the bilateral intercostal muscles by ultrasound need to be further studied. Meanwhile, reference values need to be determined.
3.2. The scalene muscles, sternocleidomastoid muscles and the trapezius
The human inspiratory muscles in the neck include the scalene and sternomastoid. These muscles have similar respiratory actions on the chest wall and cause cranial displacement of the sternum and ribcage (Hudson et al., 2007). The human scalene are obligatory inspiratory muscles that have a greater mechanical advantage than sternomastoid. Lee et al. (2016) found that stretching of the scalene muscles improved vital capacity. Several studies used computed tomographic scan images to measure the changes in muscle length, muscle mass and size of the sternocleidomastoid muscle (Peche et al., 1996; Legrand et al., 2003).
One study evaluated the reliability of shear-wave elastography (SWE) to assess the anterior and middle scalene muscles in healthy adult subjects. Ultrasound examinations of the scalene muscles were performed by an L18–4 MHz linear-array transducer. The ultrasound transducer was placed just lateral to the thyroid lobe (Bedewi et al., 2021). However, the limitations of this study were the small sample size, and the lack of comparison with pathological tissue. Several studies used B mode linear probe of ultrasonography with frequency of 7–11 or 8.5–10.0 MHz for imaging of the trapezius (Adigozali et al., 2016; Kisilewicz et al., 2020). Subjects were asked to sit on the chair while they were in an upright and relaxed position (Adigozali et al., 2016). In order to measure the thickness and SWE of the trapezius, the position of the ultrasound probe was generally chosen the midpoint of the spinous process of 7th cervical vertebra and acromion process of right scapula were determined by palpation. However, due to the peculiarities of anatomy and the limitations of ultrasound technology, there were few ultrasound studies on the scalene, sternocleidomastoid muscles and the trapezius.
3.3. Expiratory muscle
The expiratory muscles include the abdominal wall muscles (transversus abdominis muscle (TrA), internal oblique muscle (IO), external oblique muscle (EO), and rectus abdominis muscle (RA)), and some of the rib cage ones (e.g., the internal intercostal muscles and the triangularis sterni muscle) (De Troyer et al., 1998; Wilson et al., 2001; De Troyer et al., 2005; De Troyer and Boriek, 2011). During tidal breathing, the expiratory muscles are largely inactive (Shi et al., 2019), although the transversus abdominis muscle may occasionally show some activity during quiet breathing (De Troyer et al., 1990). Previous study has shown that transversus abdominis has an important role in posture (Belavý et al., 2017). The contraction of the transversus abdominis muscle with the other muscles of the abdominal cavity has also been shown to increase intra-abdominal pressure (Hodges and Gandevia, 2000). Activation of the expiratory muscles during breathing occurs when the load imposed on the inspiratory muscle increases (Shi et al., 2019). In the presence of an imbalance between inspiratory muscle load and capacity, the abdominal wall muscles are recruited during expiration in a fixed hierarchy (Aliverti et al., 1997; Parthasarathy et al., 2007): initially, the transversus abdominis muscle, followed by the internal oblique muscle and the external oblique muscle, and finally the rectus abdominis muscle (Abe et al., 1996; Suzuki et al., 1999). Abdominal ultrasound allows direct visualization of the three layers of the abdominal wall muscles (Misuri et al., 1997; McMeeken et al., 2004; Rankin et al., 2006; Tahan et al., 2016). In healthy subjects, the thickness of individual abdominal wall muscles follows a certain pattern: transversus abdominis < external oblique < internal oblique < rectus abdominis (Tahan et al., 2016).
Expiratory muscle thickness measurement (Table 3): Using 7.5 or 10–15 MHz linear probe in B-mode condition positioned perpendicular to the abdominal wall. Measurements were performed with the subjects in a supine or semi-recumbent position with knees bent and the hips at 45°. To visualize the rectus abdominis muscle, the transducer is positioned in a transverse orientation approximately 2–3 cm above the umbilicus and 2–3 cm lateral from the midline. The external oblique, internal oblique, and transversus abdominis muscles can be identified as three parallel layers, usually at the anterior axillary line, midway between the inferior border of the rib cage and the iliac crest. Several studies measured rectus abdominis at 3 cm or 4 cm lateral to the umbilicus; the external oblique, internal oblique, and transverse abdominis were measured at 2.5 cm anterior to the mid-axillary line and at the midpoint between the inferior rib and iliac crest. The pressure applied to the probe should be kept to a minimum to prevent compression of the abdominal wall as this may alter the shape/thickness of the underlying muscles. Abdominal muscle thickness was performed at the end of a relaxed expiration or at end-inspiration. Thickening fraction of the expiratory abdominal muscles (TFabd) can be calculated as the magnitude of thickness increase during expiration. Change in thickness determined the thickening fraction of the expiratory abdominal muscles (TFadb) as follows: TFadb = (end-expiratory thickness − end-inspiratory thickness)/end-inspiratory thickness × 100% (Tuinman et al., 2020).
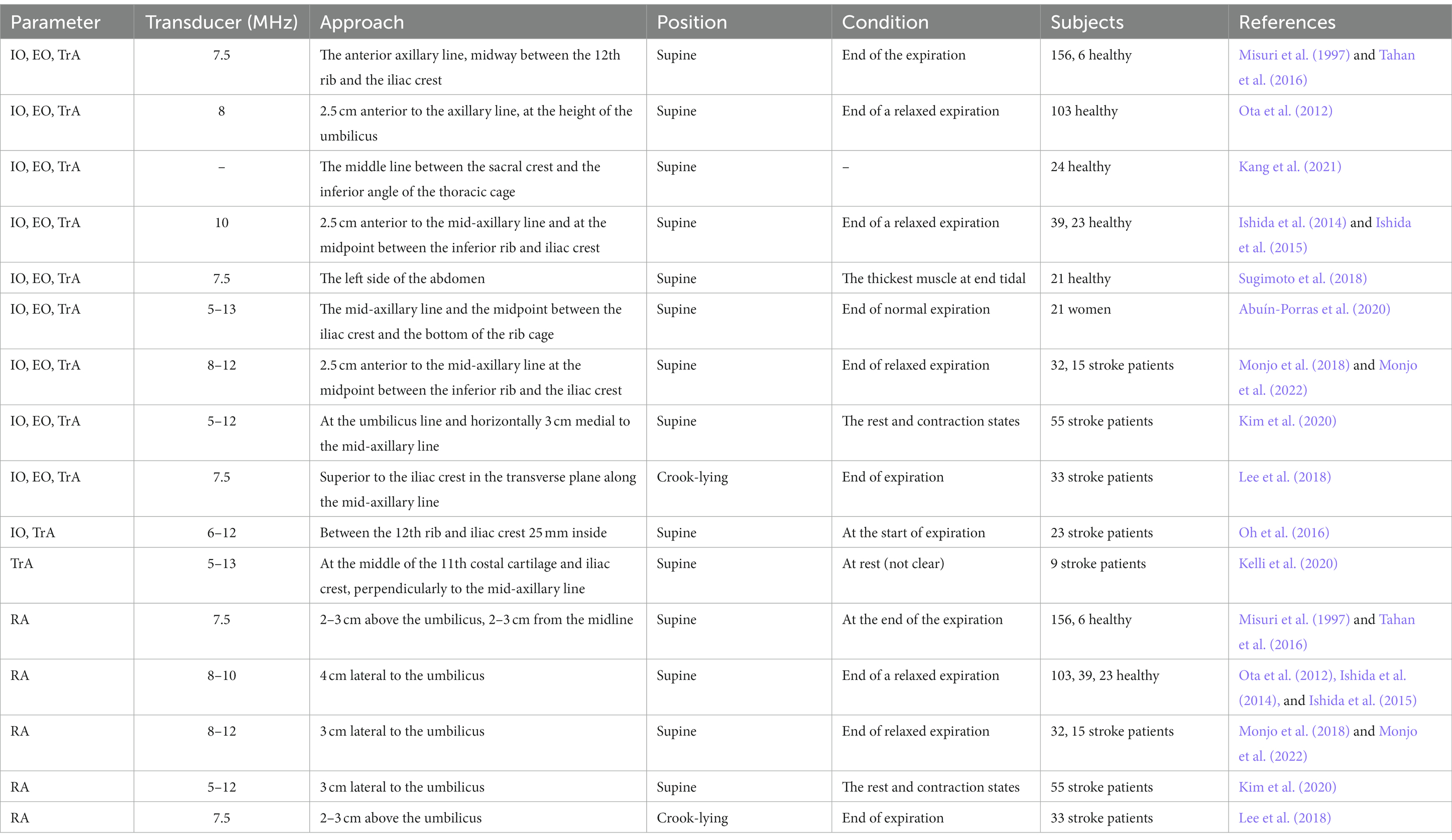
Table 3. Selected studies providing direct visualization of ultrasonographic assessment of the expiratory muscles’ thickness.
3.4. Feasibility and reliability of the ultrasound measurements
To be useful, ultrasound measures should be reliable and with good reproducibility, which means that they are stable over time, have minimal variability, and have enough sensitivity to detect clinically important changes (Lexell and Downham, 2005). There were many studies on the feasibility and reliability of the diaphragmatic ultrasound measurements (Baldwin et al., 2011; Goligher et al., 2015). Ultrasonographic technique of diaphragm was previously reported to be reliable, with high intra-class correlation coefficient for intra-rater and inter-rater reliability (Gottesman and McCool, 1997; Boussuges et al., 2009). Diaphragm motion depend on the position of the subject in the study (Sarwal et al., 2013). The supine position is preferred, because there is less overall variability, less side-to-side variability, and greater reproducibility (Gerscovich et al., 2001). Baldwin et al. (2011) also found that ultrasound technique has good reliability in recumbent positions. In addition, the relationship between inspired volume and diaphragmatic motion was found to be linear (Houston et al., 1994).
On the contrary, very few studies have applied ultrasound to evaluate the extra-diaphragmatic inspiratory muscles. Parasternal intercostal muscle ultrasound may be a useful tool in the evaluation of the respiratory muscle in future studies (Tuinman et al., 2020). Dres et al. (2020) have proposed a technique to measure the parasternal intercostal muscle by ultrasonography, while their results need to be confirmed in a larger number of patients (Vivier and Mekontso Dessap, 2020). Intercostal muscle ultrasound offers a repeatable and radiation-free alternative, however requires validation. Wallbridge et al. (2018) found that the inter-rater reliability was not as strong for thickness, particularly in the third intercostal spaces bilaterally. The results of the research demonstrated that real time ultrasonography was a reliable method for measurement of upper trapezius (Adigozali et al., 2016). Other extra-diaphragmatic inspiratory muscles, such as the scalene muscles and sternocleidomastoid muscles, requires further study on their ultrasound approach, reliability, and reproducibility.
The measurement of abdominal wall muscles’ thickness was feasible in almost all healthy subjects and patients. A large number of studies evaluated the reproducibility of ultrasound imaging measures of abdominal muscle activity (McMeeken et al., 2004; English et al., 2012). Reliability in the measurement of abdominal muscle thickness was assessed in several studies and their results indicated an excellent inter-and intra-rater reproducibility in measuring the muscle thickness of these muscles (Ota et al., 2012; Shi et al., 2021).
3.5. Limitations of respiratory muscle ultrasonography
The operator skills, technical aspects are related to ultrasound physics, and patient characteristics, e.g., probe orientation difficulties, the small muscles, muscle edema, and the pressure of the transducer and manipulator’s hand on the wall can all affect measurements (Tuinman et al., 2020; Vivier and Mekontso Dessap, 2020). Furthermore, the spatial axial resolution of the probe plays a critical role. Comparing individual patient results should be done with a degree of caution and only after adequate training (Tuinman et al., 2020).
Previous studies have some methods to reduce variability, such as the same normalized position of the subject and the exact position of the transducer were maintained during the measurement, use the minimum amount of pressure needed to get a clear image while preventing pressure that could alter the shape or thickness of the muscle (Tuinman et al., 2020), the same observer for three different cycles of measurement, and a unified standard for measurement software, etc. (Liu et al., 2022).
3.6. Risk of bias
The risk of selection, performance, detection, and reporting bias in the included studies are specified in Table 4.
4. Clinical applications of the patients with stroke
4.1. Role of diaphragm ultrasound in stroke
The human diaphragm represents one of the muscles that are controlled by an automatic as well as voluntary motor system (Nakayama et al., 2004). It is thought that bilateral hemi-diaphragms are controlled by the contralateral primary motor cortex and, thus, in the presence of paralysis, the diaphragm is also affected on the same side as the paralysis (Aminoff and Sears, 1971; Cohen et al., 1994). The etiology of the paralysis is multiple (Gerscovich et al., 2001). Central nervous system disease, including brain infarction, may impair diaphragmatic motion. Because ultrasonography can distinguish a functioning from a nonfunctioning diaphragm, it can be used to diagnose both unilateral and bilateral diaphragmatic paralysis and to monitor recovery of the paralyzed diaphragm (Gottesman and McCool, 1997; Summerhill et al., 2008). Respiratory exercises could contribute to the well-being of the stroke patients and this contribution could be followed up by diaphragm ultrasound (Kılıçoğlu et al., 2022).
Hemiplegic side of the diaphragm had reduced thickness and motion during voluntary inspiration on the same side of the body paralysis in patients with stroke (Laroche et al., 1988; Kim et al., 2017) and this finding was not seen during quiet breathing (Cohen et al., 1994). Our results of previous study were consistent with (Liu et al., 2022). However, there was controversy about the function of the hemiplegic side and non-hemiplegic side of the diaphragm after stroke. Some researchers showed that the motion of the diaphragm on the hemiplegic side decreases after stroke, and the contralateral showed a larger excursion compensatively (Cohen et al., 1994). However, Houston et al. (1995) found bilaterally decreased volitive diaphragmatic motion in acute cerebral infarction. Recent studies have found that the thickness and motion of the bilateral diaphragm in stroke patients were decreased (Caleffi-Pereira et al., 2018), while diaphragmatic dysfunction was more severe on the hemiplegic side (Catalá-Ripoll et al., 2020). In view of this, we reviewed the diaphragm thickness in hemiplegic patients after stroke at the subacute stage (almost within 1–6 months from the onset) with ultrasound in the literature, as shown in Figure 2. In most of the studies, the thickness of the diaphragm on the hemiplegic side of stroke patients is lower than that on the non-hemiplegic side, no matter at the end of inspiration or end of expiration (Supplementary material 2).
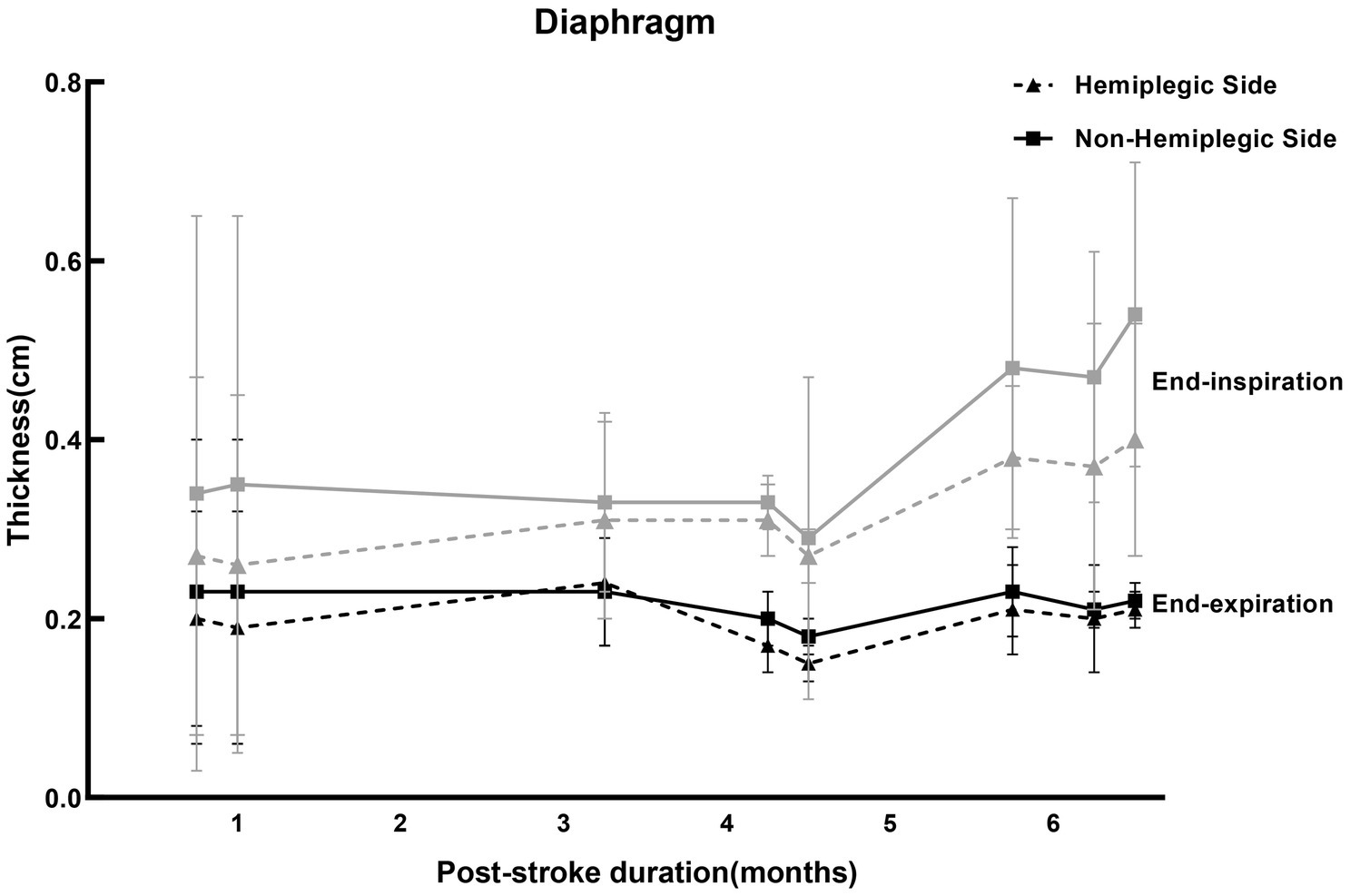
Figure 2. Studies for the thickness of the diaphragm ultrasound in stroke patients. The reference values of the diaphragm thickness of the stroke patients at the subacute stage at end-expiration and end-inspiration measured by ultrasound in different studies in Supplementary material 2, presented by the mean ± standard deviation. Where the gray curves (solid and dotted lines) represented the diaphragm thickness at the end of inspiration, and the black curves (solid and dotted lines) were the thickness of the diaphragm at the end of expiration. The solid lines were the thickness of the diaphragm on non-hemiplegic side, while the dotted lines were the hemiplegic side. The values on the abscissa represented the average duration of post-stroke (months).
4.2. Role of expiratory muscles ultrasound in stroke
The function of hemiplegic expiratory muscles may be affected in stroke patients. It is therefore important to determine the quantitative and qualitative changes in abdominal muscles in stroke survivors. The hemiplegic side has been found to exhibit negative changes in abdominal muscle quantity and quality compared with the non-hemiplegic side or healthy controls (Monjo et al., 2022). One study has shown that the TrA on the hemiplegic side was 16% lower in the stroke patients compared to the matched side in the healthy people (Marsden et al., 2013). However, Kelli et al. (2020) found that bilateral TrA thickness decreased in the hemiplegia patients, suggesting muscle atrophy on both sides of the trunk. Kim et al. (2020) revealed that contractility of RA and EO at the paretic side was significantly lower than at the non-paretic side, while there were no significant difference between non-paretic and paretic sides at rest. Monjo et al. (2018) indicated that changes on the hemiplegic side in stroke survivors might not occur in the abdominal muscles. Regarding the reliability of ultrasound measurements, studies have shown that ultrasound is considered a reliable method for measuring muscle thickness in acute stroke patients (English et al., 2012). We summarized the thickness of abdominal respiratory muscles in hemiplegic patients after stroke in the literature, as shown in Figure 3. In the studies included, the average post-stroke duration of the expiratory muscles-related patients with hemiplegia ranged from months to years. Due to a large duration span, there was a lack of evaluation of possible bias. Consequently, we indicated reference numbers of different studies on the abscissa replaced the average duration of post-stroke in Figure 3. For IO and RA, two of three studies indicated that the thickness of the hemiplegic side of stroke patients was lower than that of the non-hemiplegia side, and one study found the opposite conclusion. For TrA, most studies showed that the thickness on the hemiplegic side was lower than that on the non-hemiplegia side; while for EO, all three studies showed that the thickness on the hemiplegic side was lower than that on the non-hemiplegic side (Supplementary material 2).
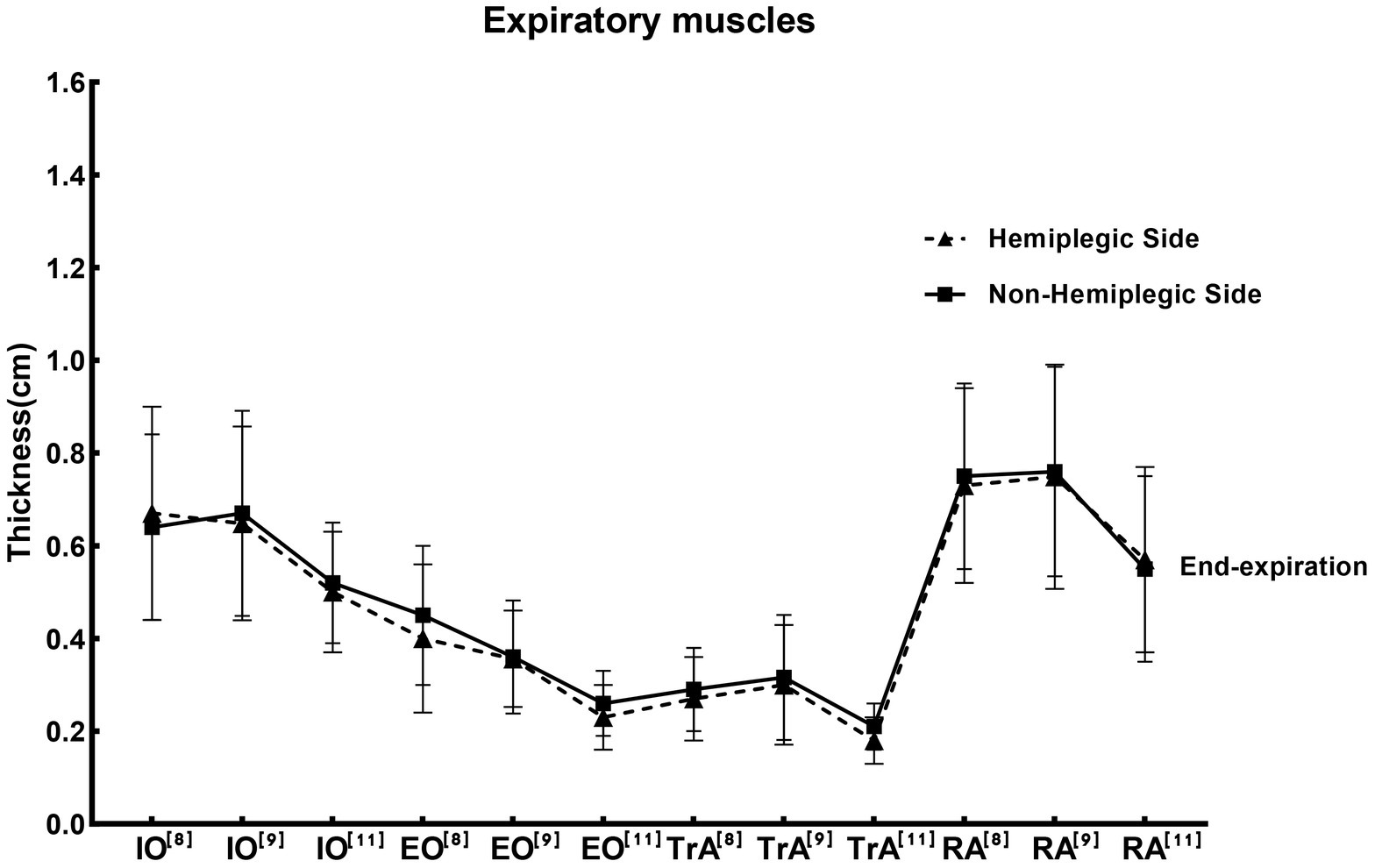
Figure 3. Studies for the thickness of the expiratory muscle ultrasound in stroke patients. The reference values of the thickness of expiratory muscle at end-expiration measured by ultrasound in different studies in Supplementary material 2, presented by the mean ± standard deviation. Where the solid line was the thickness of the expiratory muscles on non-hemiplegic side, while the dotted line was the hemiplegic side. Labels at the top-right of the abbreviations on the abscissa axis, such as [8],[9],[11], are shown in Supplementary material 2. The abbreviations on the abscissa represented different studies. IO, Internal oblique muscle; EO, External oblique muscle; RA, Rectus abdominis muscle; TrA, Transversus abdominis muscle.
5. Discussion
It is necessary to assess the muscles of respiratory to measure respiratory function. Respiratory muscle ultrasound is a widely available, highly feasible, non-invasive bedside radiation-free technique that can be easily applied in the clinic. It can provide information about the structure and function of the respiratory muscle. Moreover, ultrasonography can be used to study the contribution of the individual respiratory muscles related to pulmonary dysfunction. At present, diaphragm and expiratory muscle ultrasound has been widely used in the assessment of respiratory muscle function in healthy people, and there have been some studies on its repeatability and reliability. However, its application in diseases and the outlier values need more studies to verify. In addition, there is not enough evidence to use ultrasound measurements to assess extra-diaphragmatic inspiratory muscles, and further studies using standardized methodology are needed. The impairment of respiratory function is a frequent and serious complication for stroke patients (Kim et al., 2015). Early detection of respiratory dysfunction is important for protecting patients from comorbid pulmonary problems (Kılıçoğlu et al., 2022). By summarizing the function of respiratory muscle on hemiplegic side and non-hemiplegic side of stroke patients in the studies included, we found that the average thickness of diaphragm on the hemiplegic side of stroke patients was significantly lower than that on the non-hemiplegic side, while the average thickness of abdominal expiratory muscles on the hemiplegic side were mostly lower than that on the non-hemiplegic side. However, there are several limitations in this review. We summarized and described the applications of ultrasonic measurement of respiratory muscle according to different parameters; but there was a lack of evaluation of differences and possible bias. In addition, due to the inclusion of fewer studies, the included stroke patients were not distinguished by age, gender, type of stroke, etc., at the clinical application of this review. Due to the inconsistency of indicators reflecting diaphragmatic contractility in the included studies, diaphragmatic thickness at the end-inspiratory and end-expiratory was used instead of diaphragmatic thickening fraction in Figure 2. Moreover, the average post-stroke duration of the expiratory muscles-related patients with hemiplegia ranged from months to years in the studies included. Future research can analyze and summarize these influencing factors before elaboration.
Author contributions
XL conceived and designed the review. XL and YY drafted the manuscript, designed the tables, and designed the figures. XL, YY, and JJ modified the language and checked the text. XL and JJ checked and confirmed the information. All authors read and approved the final manuscript.
Funding
This research was funded by National Key Research and Development Program of the Ministry of Science and Technology of the People’s Republic of China, grant numbers 2018YFC2002300 and 2018YFC2002301.
Conflict of interest
The authors declare that the research was conducted in the absence of any commercial or financial relationships that could be construed as a potential conflict of interest.
Publisher’s note
All claims expressed in this article are solely those of the authors and do not necessarily represent those of their affiliated organizations, or those of the publisher, the editors and the reviewers. Any product that may be evaluated in this article, or claim that may be made by its manufacturer, is not guaranteed or endorsed by the publisher.
Supplementary material
The Supplementary material for this article can be found online at: https://www.frontiersin.org/articles/10.3389/fnins.2023.1132335/full#supplementary-material
References
Abe, T., Kusuhara, N., Yoshimura, N., Tomita, T., and Easton, P. A. (1996). Differential respiratory activity of four abdominal muscles in humans. J. Appl. Physiol. 80, 1379–1389. doi: 10.1152/jappl.1996.80.4.1379
Abuín-Porras, V., Maldonado-Tello, P., de la Cueva-Reguera, M., Rodríguez-Sanz, D., Calvo-Lobo, C., López-López, D., et al. (2020). Comparison of lateral abdominal musculature activation during expiration with an expiratory flow control device versus the abdominal drawing-in maneuver in healthy women: a cross-sectional observational pilot study. Medicina 56:84. doi: 10.3390/medicina56020084
Adigozali, H., Shadmehr, A., Ebrahimi, E., Rezasoltani, A., and Naderi, F. (2016). Ultrasonography for the assessment of the upper trapezius properties in healthy females: a reliability study. Muscles Ligaments Tendons J. 6, 167–172. doi: 10.11138/mltj/2016.6.1.167
Aliverti, A., Cala, S. J., Duranti, R., Ferrigno, G., Kenyon, C. M., Pedotti, A., et al. (1997). Human respiratory muscle actions and control during exercise. J. Appl. Physiol. 83, 1256–1269. doi: 10.1152/jappl.1997.83.4.1256
Aminoff, M. J., and Sears, T. A. (1971). Spinal integration of segmental, cortical and breathing inputs to thoracic respiratory motoneurones. J. Physiol. 215, 557–575. doi: 10.1113/jphysiol.1971.sp009485
Baldwin, C. E., Paratz, J. D., and Bersten, A. D. (2011). Diaphragm and peripheral muscle thickness on ultrasound: intra-rater reliability and variability of a methodology using non-standard recumbent positions. Respirology 16, 1136–1143. doi: 10.1111/j.1440-1843.2011.02005.x
Baria, M. R., Shahgholi, L., Sorenson, E. J., Harper, C. J., Lim, K. G., Strommen, J. A., et al. (2014). B-mode ultrasound assessment of diaphragm structure and function in patients with COPD. Chest 146, 680–685. doi: 10.1378/chest.13-2306
Bedewi, M. A., Alhariqi, B. A., Aldossary, N. M., Gaballah, A. H., and Sandougah, K. J. (2021). Shear wave elastography of the scalene muscles in healthy adults. Medicine 100:e26891. doi: 10.1097/MD.0000000000026891
Belavý, D. L., Gast, U., and Felsenberg, D. (2017). Exercise and transversus abdominis muscle atrophy after 60-d bed rest. Med. Sci. Sports Exerc. 49, 238–246. doi: 10.1249/MSS.0000000000001096
Benditt, J. O., and Boitano, L. J. (2013). Pulmonary issues in patients with chronic neuromuscular disease. Am. J. Resp. Crit. Care. 187, 1046–1055. doi: 10.1164/rccm.201210-1804CI
Boon, A. J., Harper, C. J., Ghahfarokhi, L. S., Strommen, J. A., Watson, J. C., and Sorenson, E. J. (2013). Two-dimensional ultrasound imaging of the diaphragm: quantitative values in normal subjects. Muscle Nerve 47, 884–889. doi: 10.1002/mus.23702
Boussuges, A., Gole, Y., and Blanc, P. (2009). Diaphragmatic motion studied by m-mode ultrasonography: methods, reproducibility, and normal values. Chest 135, 391–400. doi: 10.1378/chest.08-1541
Cala, S. J., Kenyon, C. M., Lee, A., Watkin, K., Macklem, P. T., and Rochester, D. F. (1998). Respiratory ultrasonography of human parasternal intercostal muscle in vivo. Ultrasound Med. Biol. 24, 313–326. doi: 10.1016/s0301-5629(97)00271-8
Caleffi-Pereira, M., Pletsch-Assunção, R., Cardenas, L. Z., Santana, P. V., Ferreira, J. G., Iamonti, V. C., et al. (2018). Unilateral diaphragm paralysis: a dysfunction restricted not just to one hemidiaphragm. BMC Pulm. Med. 18:126. doi: 10.1186/s12890-018-0698-1
Cao, H., Chen, X., Ren, X., Chen, Z., Liu, C., Ni, J., et al. (2022). Repetitive transcranial magnetic stimulation combined with respiratory muscle training for pulmonary rehabilitation after ischemic stroke—a randomized, case-control study. Front. Aging Neurosci. 14:1006696. doi: 10.3389/fnagi.2022.1006696
Catalá-Ripoll, J. V., Monsalve-Naharro, J. Á., and Hernández-Fernández, F. (2020). Incidence and predictive factors of diaphragmatic dysfunction in acute stroke. BMC Neurol. 20:79. doi: 10.1186/s12883-020-01664-w
Chavhan, G. B., Babyn, P. S., Cohen, R. A., and Langer, J. C. (2010). Multimodality imaging of the pediatric diaphragm: anatomy and pathologic conditions. Radiographics 30, 1797–1817. doi: 10.1148/rg.307105046
Cho, J. E., Lee, H. J., Kim, M. K., and Lee, W. H. (2018). The improvement in respiratory function by inspiratory muscle training is due to structural muscle changes in patients with stroke: a randomized controlled pilot trial. Top. Stroke Rehabil. 25, 37–43. doi: 10.1080/10749357.2017.1383681
Cohen, E., Mier, A., Heywood, P., Murphy, K., Boultbee, J., and Guz, A. (1994). Diaphragmatic movement in hemiplegic patients measured by ultrasonography. Thorax 49, 890–895. doi: 10.1136/thx.49.9.890
Crimi, C., Heffler, E., Augelletti, T., Campisi, R., Noto, A., Vancheri, C., et al. (2018). Utility of ultrasound assessment of diaphragmatic function before and after pulmonary rehabilitation in COPD patients. Int. J. Chron. Obstruct. Pulmon. Dis. 13, 3131–3139. doi: 10.2147/COPD.S171134
De Troyer, A., and Boriek, A. M. (2011). Mechanics of the respiratory muscles. Compr. Physiol. 1, 1273–1300. doi: 10.1002/cphy.c100009
De Troyer, A., Estenne, M., Ninane, V., Van Gansbeke, D., and Gorini, M. (1990). Transversus abdominis muscle function in humans. J. Appl. Physiol. 68, 1010–1016. doi: 10.1152/jappl.1990.68.3.1010
De Troyer, A., Kirkwood, P. A., and Wilson, T. A. (2005). Respiratory action of the intercostal muscles. Physiol. Rev. 85, 717–756. doi: 10.1152/physrev.00007.2004
De Troyer, A., Legrand, A., Gevenois, P. A., and Wilson, T. A. (1998). Mechanical advantage of the human parasternal intercostal and triangularis sterni muscles. J. Physiol. 513, 915–925. doi: 10.1111/j.1469-7793.1998.915ba.x
Diab, K. M., Shalabi, A., Sevastik, J. A., and Guntner, P. (1998). A method for morphometric study of the intercostal muscles by high-resolution ultrasound. Eur. Spine J. 7, 224–228. doi: 10.1007/s005860050061
Dres, M., Dubé, B., Goligher, E., Vorona, S., Demiri, S., Morawiec, E., et al. (2020). Usefulness of parasternal intercostal muscle ultrasound during weaning from mechanical ventilation. Anesthesiology 132, 1114–1125. doi: 10.1097/ALN.0000000000003191
Dres, M., Similowski, T., Goligher, E. C., Pham, T., Sergenyuk, L., Telias, I., et al. (2021). Dyspnoea and respiratory muscle ultrasound to predict extubation failure. Eur. Respir. J. 58:2100002. doi: 10.1183/13993003.00002-2021
English, C. K., Thoirs, K. A., Fisher, L., McLennan, H., and Bernhardt, J. (2012). Ultrasound is a reliable measure of muscle thickness in acute stroke patients, for some, but not all anatomical sites: a study of the intra-rater reliability of muscle thickness measures in acute stroke patients. Ultrasound Med. Biol. 38, 368–376. doi: 10.1016/j.ultrasmedbio.2011.12.012
Estenne, M., Yernault, J. C., and De Troyer, A. (1985). Rib cage and diaphragm-abdomen compliance in humans: effects of age and posture. J. Appl. Physiol. 59, 1842–1848. doi: 10.1152/jappl.1985.59.6.1842
Fedullo, A. J., Lerner, R. M., Gibson, J., and Shayne, D. S. (1992). Sonographic measurement of diaphragmatic motion after coronary artery bypass surgery. Chest 102, 1683–1686. doi: 10.1378/chest.102.6.1683
Formenti, P., Umbrello, M., Castagna, V., Cenci, S., Bichi, F., Pozzi, T., et al. (2022). Respiratory and peripheral muscular ultrasound characteristics in ICU COVID 19 ARDS patients. J. Crit. Care 67, 14–20. doi: 10.1016/j.jcrc.2021.09.007
Formenti, P., Umbrello, M., Dres, M., and Chiumello, D. (2020). Ultrasonographic assessment of parasternal intercostal muscles during mechanical ventilation. Ann. Intensive Care 10:120. doi: 10.1186/s13613-020-00735-y
Gerscovich, E. O., Cronan, M., McGahan, J. P., Jain, K., Jones, C. D., and McDonald, C. (2001). Ultrasonographic evaluation of diaphragmatic motion. J. Ultrasound Med. 20, 597–604. doi: 10.7863/jum.2001.20.6.597
Goligher, E. C., Laghi, F., Detsky, M. E., Farias, P., Murray, A., Brace, D., et al. (2015). Measuring diaphragm thickness with ultrasound in mechanically ventilated patients: feasibility, reproducibility and validity. Intens. Care Med. 41, 642–649. doi: 10.1007/s00134-015-3687-3
Gottesman, E., and McCool, F. D. (1997). Ultrasound evaluation of the paralyzed diaphragm. Am. J. Resp. Crit. Care. 155, 1570–1574. doi: 10.1164/ajrccm.155.5.9154859
Hodges, P. W., and Gandevia, S. C. (2000). Changes in intra-abdominal pressure during postural and respiratory activation of the human diaphragm. J. Appl. Physiol. 89, 967–976. doi: 10.1152/jappl.2000.89.3.967
Houston, J. G., Angus, R. M., Cowan, M. D., McMillan, N. C., and Thomson, N. C. (1994). Ultrasound assessment of normal hemidiaphragmatic movement: relation to inspiratory volume. Thorax 49, 500–503. doi: 10.1136/thx.49.5.500
Houston, J. G., Morris, A. D., Grosset, D. G., Lees, K. R., McMillan, N., and Bone, I. (1995). Ultrasonic evaluation of movement of the diaphragm after acute cerebral infarction. J. Neurol. Neurosurg. Psychiatry 58, 738–741. doi: 10.1136/jnnp.58.6.738
Hudson, A. L., Gandevia, S. C., and Butler, J. E. (2007). The effect of lung volume on the co-ordinated recruitment of scalene and sternomastoid muscles in humans. J. Physiol. 584, 261–270. doi: 10.1113/jphysiol.2007.137240
Ishida, H., Kobara, K., Osaka, H., Suehiro, T., Ito, T., Kurozumi, C., et al. (2014). Correlation between peak expiratory flow and abdominal muscle thickness. J. Phys. Ther. Sci. 26, 1791–1793. doi: 10.1589/jpts.26.1791
Ishida, H., Suehiro, T., Kurozumi, C., Ono, K., and Watanabe, S. (2015). Correlation between abdominal muscle thickness and maximal expiratory pressure. J. Ultras. Med. 34, 2001–2005. doi: 10.7863/ultra.14.12006
Jandt, S. R., Da Sil Caballero, R. M., Junior, L. A. F., and Dias, A. S. (2011). Correlation between trunk control, respiratory muscle strength and spirometry in patients with stroke: an observational study. Physiother. Res. Int. 16, 218–224. doi: 10.1002/pri.495
Jung, K., Park, J., Hwang, D., Kim, J., and Kim, J. (2014). Ultrasonographic diaphragmatic motion analysis and its correlation with pulmonary function in hemiplegic stroke patients. Ann. Rehabil. Med. 38, 29–37. doi: 10.5535/arm.2014.38.1.29
Kang, K. W., Kwon, Y. H., and Son, S. M. (2021). Ultrasound measurement of the transverse abdominis, internal oblique, and external oblique muscles associated with forward head posture and reduced Cranio-vertebral angle. Med. Sci. Monitor. 27:e928987. doi: 10.12659/MSM.928987
Kelli, A., Kellis, E., Galanis, N., Dafkou, K., Sahinis, C., and Ellinoudis, A. (2020). Transversus abdominis thickness at rest and exercise in individuals with poststroke hemiparesis. Sports 8:86. doi: 10.3390/sports8060086
Kılıçoğlu, M. S., Yurdakul, O. V., Çelik, Y., and Aydın, T. (2022). Investigating the correlation between pulmonary function tests and ultrasonographic diaphragm measurements and the effects of respiratory exercises on these parameters in hemiplegic patients. Top. Stroke Rehabil. 29, 218–229. doi: 10.1080/10749357.2021.1911748
Kim, C., Lee, J., Kim, H., and Kim, I. (2015). Effects of the combination of respiratory muscle training and abdominal drawing-in maneuver on respiratory muscle activity in patients with post-stroke hemiplegia: A pilot randomized controlled trial. Top. Stroke Rehabil. 22, 262–270. doi: 10.1179/1074935714Z.0000000020
Kim, Y., Kim, J., Nam, H., Kim, H. D., Eom, M. J., Jung, S. H., et al. (2020). Ultrasound imaging of the trunk muscles in acute stroke patients and relations with balance scales. Ann. Rehabil. Med. 44, 273–283. doi: 10.5535/arm.19125
Kim, M., Lee, K., Cho, J., and Lee, W. (2017). Diaphragm thickness and inspiratory muscle functions in chronic stroke patients. Med. Sci. Monitor. 23, 1247–1253. doi: 10.12659/MSM.900529
Kisilewicz, A., Madeleine, P., Ignasiak, Z., Ciszek, B., Kawczynski, A., and Larsen, R. G. (2020). Eccentric exercise reduces upper trapezius muscle stiffness assessed by shear wave elastography and myotonometry. Front. Bioeng. Biotechnol. 8:928. doi: 10.3389/fbioe.2020.00928
Laroche, C. M., Mier, A. K., Moxham, J., and Green, M. (1988). Diaphragm strength in patients with recent hemidiaphragm paralysis. Thorax 43, 170–174. doi: 10.1136/thx.43.3.170
Laursen, C. B., Clive, A., Hallifax, R., Pietersen, P. I., Asciak, R., Davidsen, J. R. M., et al. (2021). European respiratory society statement on thoracic ultrasound. Eur. Respir. J. 57:2001519. doi: 10.1183/13993003.01519-2020
Laveneziana, P., Albuquerque, A., Aliverti, A., Babb, T., Barreiro, E., Dres, M., et al. (2019). ERS statement on respiratory muscle testing at rest and during exercise. Eur. Respir. J. 53:1801214. doi: 10.1183/13993003.01214-2018
Lee, K., Cho, J., Hwang, D., and Lee, W. (2018). Decreased respiratory muscle function is associated with impaired trunk balance among chronic stroke patients: a cross-sectional study. Tohoku J. Exp. Med. 245, 79–88. doi: 10.1620/tjem.245.79
Lee, J., Hwang, S., Han, S., and Han, D. (2016). Effects of stretching the scalene muscles on slow vital capacity. J. Phys. Ther. Sci. 28, 1825–1828. doi: 10.1589/jpts.28.1825
Legrand, A., Schneider, E., Gevenois, P., and De Troyer, A. (2003). Respiratory effects of the scalene and sternomastoid muscles in humans. J. Appl. Physiol. 94, 1467–1472. doi: 10.1152/japplphysiol.00869.2002
Lexell, J. E., and Downham, D. Y. (2005). How to assess the reliability of measurements in rehabilitation [retracted]. Am. J. Phys. Med. Rehab. 84, 719–723. doi: 10.1097/01.phm.0000176452.17771.20
Lim, S. Y., Lim, G., Lee, Y. J., Cho, Y. J., Park, J. S., Yoon, H. I., et al. (2019). Ultrasound assessment of diaphragmatic function during acute exacerbation of chronic obstructive pulmonary disease: a pilot study. Int. J. Chron. Obstruct. Pulmon. Dis. 14, 2479–2484. doi: 10.2147/COPD.S214716
Liu, X., Qu, Q., Deng, P., Zhao, Y., Liu, C., Fu, C., et al. (2022). Assessment of diaphragm in hemiplegic patients after stroke with ultrasound and its correlation of extremity motor and balance function. Brain Sci. 12:882. doi: 10.3390/brainsci12070882
Marsden, J. F., Hough, A., Shum, G., Shaw, S., and Freeman, J. A. (2013). Deep abdominal muscle activity following supratentorial stroke. J. Electromyogr. Kines. 23, 985–990. doi: 10.1016/j.jelekin.2013.04.003
Matamis, D., Soilemezi, E., Tsagourias, M., Akoumianaki, E., Dimassi, S., Boroli, F., et al. (2013). Sonographic evaluation of the diaphragm in critically ill patients. Technique and clinical applications. Intensive Care Med. 39, 801–810. doi: 10.1007/s00134-013-2823-1
McMeeken, J. M., Beith, I. D., Newham, D. J., Milligan, P., and Critchley, D. J. (2004). The relationship between EMG and change in thickness of transversus abdominis. Clin. Biomech. 19, 337–342. doi: 10.1016/j.clinbiomech.2004.01.007
Misuri, G., Colagrande, S., Gorini, M., Iandelli, I., Mancini, M., Duranti, R., et al. (1997). In vivo ultrasound assessment of respiratory function of abdominal muscles in normal subjects. Eur. Respir. J. 10, 2861–2867. doi: 10.1183/09031936.97.10122861
Monjo, H., Fukumoto, Y., Asai, T., Ohshima, K., Kubo, H., Tajitsu, H., et al. (2022). Changes in muscle thickness and echo intensity in chronic stroke survivors: a 2-year longitudinal study. J. Clin. Neurol. 18, 308–314. doi: 10.3988/jcn.2022.18.3.308
Monjo, H., Fukumoto, Y., Asai, T., and Shuntoh, H. (2018). Muscle thickness and echo intensity of the abdominal and lower extremity muscles in stroke survivors. J. Clin. Neurol. 14, 549–554. doi: 10.3988/jcn.2018.14.4.549
Nakayama, T., Fujii, Y., Suzuki, K., Kanazawa, I., and Nakada, T. (2004). The primary motor area for voluntary diaphragmatic motion identified by high field fMRI. J. Neurol. 251, 730–735. doi: 10.1007/s00415-004-0413-4
Oh, D., Kim, G., Lee, W., and Shin, M. M. (2016). Effects of inspiratory muscle training on balance ability and abdominal muscle thickness in chronic stroke patients. J. Phys. Ther. Sci. 28, 107–111. doi: 10.1589/jpts.28.107
Ota, M., Ikezoe, T., Kaneoka, K., and Ichihashi, N. (2012). Age-related changes in the thickness of the deep and superficial abdominal muscles in women. Arch. Gerontol. Geriat. 55, e26–e30. doi: 10.1016/j.archger.2012.03.007
Paolo, F., Valentina, D. G., Silvia, C., Tommaso, P., Elena, C., Martin, D., et al. (2022). The possible predictive value of muscle ultrasound in the diagnosis of ICUAW in long-term critically ill patients. J. Crit. Care 71:154104. doi: 10.1016/j.jcrc.2022.154104
Parthasarathy, S., Jubran, A., Laghi, F., and Tobin, M. J. (2007). Sternomastoid, rib cage, and expiratory muscle activity during weaning failure. J. Appl. Physiol. 103, 140–147. doi: 10.1152/japplphysiol.00904.2006
Peche, R., Estenne, M., Gevenois, P. A., Brassinne, E., Yernault, J. C., and De Troyer, A. (1996). Sternomastoid muscle size and strength in patients with severe chronic obstructive pulmonary disease. Am. J. Resp. Crit. Care. 153, 422–425. doi: 10.1164/ajrccm.153.1.8542153
Pietton, R., David, M., Hisaund, A., Langlais, T., Skalli, W., Vialle, R., et al. (2021). Biomechanical evaluation of intercostal muscles in healthy children and adolescent idiopathic scoliosis: a preliminary study. Ultrasound Med. Biol. 47, 51–57. doi: 10.1016/j.ultrasmedbio.2020.09.011
Pinto, S., Alves, P., Pimentel, B., Swash, M., and de Carvalho, M. (2016). Ultrasound for assessment of diaphragm in ALS. Clin. Neurophysiol. 127, 892–897. doi: 10.1016/j.clinph.2015.03.024
Rahman, N. N., Singh, D., and Lee, R. Y. (2017). Correlation between thoracolumbar curvatures and respiratory function in older adults. Clin. Interv. Aging 12, 523–529. doi: 10.2147/CIA.S110329
Rankin, G., Stokes, M., and Newham, D. J. (2006). Abdominal muscle size and symmetry in normal subjects. Muscle Nerve 34, 320–326. doi: 10.1002/mus.20589
Rochester, C. L., and Mohsenin, V. (2002). Respiratory complications of stroke. Semin. Respir. Crit. Care Med. 23, 248–260. doi: 10.1055/s-2002-33033
Şahin, H., Doğan, A., and Ekiz, T. (2019). Ultrasonographic evaluation of the diaphragm thickness in patients with multiple sclerosis. Mult. Scler. Relat. Disord. 36:101369. doi: 10.1016/j.msard.2019.08.011
Sarwal, A., Walker, F. O., and Cartwright, M. S. (2013). Neuromuscular ultrasound for evaluation of the diaphragm. Muscle Nerve 47, 319–329. doi: 10.1002/mus.23671
Sferrazza Papa, G. F., Pellegrino, G. M., Di Marco, F., Imeri, G., Brochard, L., Goligher, E., et al. (2016). A review of the ultrasound assessment of diaphragmatic function in clinical practice. Respiration 91, 403–411. doi: 10.1159/000446518
Shi, Z., de Vries, H., de Grooth, H., Jonkman, A. H., Zhang, Y., Haaksma, M., et al. (2021). Changes in respiratory muscle thickness during mechanical ventilation: focus on expiratory muscles. Anesthesiology 134, 748–759. doi: 10.1097/ALN.0000000000003736
Shi, Z., Jonkman, A., de Vries, H., Jansen, D., Ottenheijm, C., Girbes, A., et al. (2019). Expiratory muscle dysfunction in critically ill patients: towards improved understanding. Intens. Care Med. 45, 1061–1071. doi: 10.1007/s00134-019-05664-4
Sugimoto, T., Yokogawa, M., Miaki, H., Madokoro, S., and Nakagawa, T. (2018). Changes in thickness of the transversus abdominis during the abdominal drawing-in manoeuvre and expiratory muscle training in elderly people. J. Phys. Ther. Sci. 30, 119–123. doi: 10.1589/jpts.30.119
Summerhill, E. M., El-Sameed, Y. A., Glidden, T. J., and McCool, F. D. (2008). Monitoring recovery from diaphragm paralysis with ultrasound. Chest 133, 737–743. doi: 10.1378/chest.07-2200
Supinski, G. S., Morris, P. E., Dhar, S., and Callahan, L. A. (2018). Diaphragm dysfunction in critical illness. Chest 153, 1040–1051. doi: 10.1016/j.chest.2017.08.1157
Suzuki, J., Tanaka, R., Yan, S., Chen, R., Macklem, P. T., and Kayser, B. (1999). Assessment of abdominal muscle contractility, strength, and fatigue. Am. J. Respir. Crit. Care Med. 159, 1052–1060. doi: 10.1164/ajrccm.159.4.9803025
Tahan, N., Khademi-Kalantari, K., Mohseni-Bandpei, M. A., Mikaili, S., Baghban, A. A., and Jaberzadeh, S. (2016). Measurement of superficial and deep abdominal muscle thickness: an ultrasonography study. J. Physiol. Anthropol. 35:17. doi: 10.1186/s40101-016-0106-6
Tuinman, P. R., Jonkman, A. H., Dres, M., Shi, Z., Goligher, E. C., Goffi, A., et al. (2020). Respiratory muscle ultrasonography: methodology, basic and advanced principles and clinical applications in ICU and ED patients—a narrative review. Intens. Care Med. 46, 594–605. doi: 10.1007/s00134-019-05892-8
Ueki, J., De Bruin, P. F., and Pride, N. B. (1995). In vivo assessment of diaphragm contraction by ultrasound in normal subjects. Thorax 50, 1157–1161. doi: 10.1136/thx.50.11.1157
Vivier, E., and Mekontso Dessap, A. (2020). Bedside ultrasound for weaning from mechanical ventilation. Anesthesiology 132, 947–948. doi: 10.1097/ALN.0000000000003235
Voyvoda, N., Yücel, C., Karatas, G., Oguzulgen, I., and Oktar, S. (2012). An evaluation of diaphragmatic movements in hemiplegic patients. Brit. J. Radiol. 85, 411–414. doi: 10.1259/bjr/71968119
Wait, J. L., Nahormek, P. A., Yost, W. T., and Rochester, D. P. (1989). Diaphragmatic thickness-lung volume relationship in vivo. J. Appl. Physiol. 67, 1560–1568. doi: 10.1152/jappl.1989.67.4.1560
Wallbridge, P., Parry, S. M., Das, S., Law, C., Hammerschlag, G., Irving, L., et al. (2018). Parasternal intercostal muscle ultrasound in chronic obstructive pulmonary disease correlates with spirometric severity. Sci. Rep. 8:15274. doi: 10.1038/s41598-018-33666-7
Wilson, T. A., Legrand, A., Gevenois, P. A., and Troyer, A. (2001). Respiratory effects of the external and internal intercostal muscles in humans. J. Physiol. 530, 319–330. doi: 10.1111/j.1469-7793.2001.0319l.x
Yoshida, R., Tomita, K., Kawamura, K., Nozaki, T., Setaka, Y., Monma, M., et al. (2019). Measurement of intercostal muscle thickness with ultrasound imaging during maximal breathing. J. Phys. Ther. Sci. 31, 340–343. doi: 10.1589/jpts.31.340
Keywords: diaphragm, evaluation, ultrasonography, stroke, respiratory muscle
Citation: Liu X, Yang Y and Jia J (2023) Respiratory muscle ultrasonography evaluation and its clinical application in stroke patients: A review. Front. Neurosci. 17:1132335. doi: 10.3389/fnins.2023.1132335
Edited by:
Kota Bokuda, Tokyo Metropolitan Neurological Hospital, JapanReviewed by:
Ryo Morishima, Tokyo Metropolitan Institute of Medical Science, JapanJacqueline Roots, Queensland University of Technology, Australia
Copyright © 2023 Liu, Yang and Jia. This is an open-access article distributed under the terms of the Creative Commons Attribution License (CC BY). The use, distribution or reproduction in other forums is permitted, provided the original author(s) and the copyright owner(s) are credited and that the original publication in this journal is cited, in accordance with accepted academic practice. No use, distribution or reproduction is permitted which does not comply with these terms.
*Correspondence: Jie Jia, shannonjj@126.com