- 1Research Center, Sainte-Justine University Hospital Center, Montreal, QC, Canada
- 2Department of Epidemiology, Biostatistics and Occupational Health, Faculty of Medicine, McGill University, Montreal, QC, Canada
- 3Department of Pediatrics, Sainte-Justine University Hospital Center, University of Montreal, Montreal, QC, Canada
- 4School of Rehabilitation, University of Montreal, Montreal, QC, Canada
- 5Institute of Biomedical Engineering, University of Montreal, Montreal, QC, Canada
- 6Unité de Recherche Clinique Appliquée, Sainte-Justine University Hospital Center, Montreal, QC, Canada
- 7Department of Radiology, Radio-Oncology and Nuclear Medicine, University of Montreal, Montreal, QC, Canada
Background: Infants born at 29–36 weeks gestational age (GA) are at risk of experiencing neurodevelopmental challenges. We hypothesize that cerebral hemodynamics and oxygen metabolism measured by bedside optical brain monitoring are potential biomarkers of brain development and are associated with neurological examination at term-equivalent age (TEA).
Methods: Preterm infants (N = 133) born 29–36 weeks GA and admitted in the neonatal intensive care unit were enrolled in this prospective cohort study. Combined frequency-domain near infrared spectroscopy (FDNIRS) and diffuse correlation spectroscopy (DCS) were used from birth to TEA to measure cerebral hemoglobin oxygen saturation and an index of microvascular cerebral blood flow (CBFi) along with peripheral arterial oxygen saturation (SpO2). In combination with hemoglobin concentration in the blood, these parameters were used to derive cerebral oxygen extraction fraction (OEF) and an index of cerebral oxygen metabolism (CMRO2i). The Amiel-Tison and Gosselin Neurological Assessment was performed at TEA. Linear regression models were used to assess the associations between changes in FDNIRS-DCS parameters from birth to TEA and GA at birth. Logistic regression models were used to assess the associations between changes in FDNIRS-DCS parameters from birth to TEA and neurological examination at TEA.
Results: Steeper increases in CBFi (p < 0.0001) and CMRO2i (p = 0.0003) were associated with higher GA at birth. Changes in OEF, CBFi, and CMRO2i from birth to TEA were not associated with neurological examination at TEA.
Conclusion: In this population, cerebral FDNIRS-DCS parameters were not associated with neurological examination at TEA. Larger increases in CBFi and CMRO2i from birth to TEA were associated with higher GA. Non-invasive bedside FDNIRS-DCS monitoring provides cerebral hemodynamic and metabolic parameters that may complement neurological examination to assess brain development in preterm infants.
Introduction
Infants born between 29 and 36 weeks of gestational age (GA) represent the majority of preterm births (Goldenberg et al., 2008), yet determining the ones at greatest risk of neurodevelopmental impairments remains a challenge (Vohr, 2013; Volpe, 2022). By 2 years of corrected age (CA), 25–45% of these infants have developmental delay (Simard et al., 2012; Cheong et al., 2017). Population-based data indicate that infants born 29–36 weeks GA have 1.4–2.3 times the risk of facing major disabilities affecting their long-term functioning (Moster et al., 2008). A better insight into their brain development is needed to improve early screening strategies and identify those the most vulnerable for neurodevelopmental impairment. Studies using magnetic resonance imaging (MRI) have shown smaller brain volumes and altered white matter microstructure in moderate to late preterm infants at term-equivalent age (TEA) (Kelly et al., 2016; Thompson et al., 2019; Volpe, 2022). While MRI provides high-resolution images of the brain microstructure, it is less feasible for early stratification due to high scanning cost and the high number of moderate to late preterm infants.
An alternative modality to assess brain health at the bedside is cerebral near infrared spectroscopy (NIRS). NIRS has the potential to identify infants at higher risk of neurodevelopmental impairment as early as in the neonatal period (Tataranno et al., 2021). Combining frequency-domain NIRS (FDNIRS) and diffuse correlation spectroscopy (DCS) allows to measure cerebral hemoglobin oxygen saturation (Fantini et al., 1995) and an index of microvascular cerebral blood flow (CBFi) (Boas and Yodh, 1997), respectively. Using hemoglobin concentration measurements and peripheral arterial oxygen saturation, FDNIRS-DCS parameters can be used to derive cerebral oxygen extraction fraction (OEF) and an index of the cerebral metabolic rate of oxygen consumption (CMRO2i). This technique was previously used to monitor early brain development in preterm infants (Roche-Labarbe et al., 2010; Lin et al., 2013a,b, 2016), and showed good agreement with gold-standard imaging metrics including MRI and contrast-based optical techniques (Carp et al., 2010; Durduran et al., 2010; Kim et al., 2010; Diop et al., 2011; Buckley et al., 2012; Jain et al., 2014; Milej et al., 2020). Previous studies also examined how NIRS parameters were related with electroencephalographic (EEG) activity in the preterm population, and demonstrated associations between OEF stability and more mature EEG activity (El-Dib et al., 2016), as well as relationships between increase in relative CBF and CMRO2 and EEG patterns such as spontaneous physiological neuronal bursts of activity (Nourhashemi et al., 2020).
How FDNIRS-DCS parameters relate to clinical findings in the preterm population is, however, less documented. Data suggest that brain lesions resulting in poorer developmental outcomes in the preterm population are associated with altered cerebral blood flow and oxygen metabolism (Lin et al., 2016; McLachlan et al., 2017). It was shown that even mild intraventricular hemorrhage is associated with lower CBFi and CMRO2i (Lin et al., 2016), and that severe cases leading to post-hemorrhagic ventricular dilatation have lower CBF before vs after a therapeutic intervention (McLachlan et al., 2017). FDNIRS-DCS also has the potential to inform on brain growth and maturation, including myelination and synaptogenesis (Roche-Labarbe et al., 2012; Lin et al., 2013b,2016). As the preterm infant grows, cerebral blood flow and oxygen metabolism would likely increase to reflect brain development and increased brain volumes (Roche-Labarbe et al., 2010, 2012). In a previous study, the increase in brain volumes in preterm infants born before 32 weeks GA was lower than in healthy control fetuses during the third trimester, suggesting impaired brain growth (Bouyssi-Kobar et al., 2016). In the current study, we hypothesized that increases in CBFi and CMRO2i from birth to TEA would be associated with normal neurological examination at TEA. The aim of the present study was to assess changes in FDNIRS-DCS parameters from birth to TEA and their associations with GA at birth and neurological examination at TEA in infants born between 29 and 36 weeks GA.
Materials and methods
Study population
This is a single-center prospective observational cohort study at Sainte-Justine University Hospital Center, a tertiary referral hospital in Montreal, Canada. From August 2018 to April 2020, we consecutively recruited infants born between 29 and 36 6/7 weeks GA who were admitted for at least 48 h at the neonatal intensive care unit. Exclusion criteria were major chromosomal anomaly and congenital malformations, moderate to severe hypoxic ischemic encephalopathy, and neonatal stroke, as these infants already benefit from neurodevelopmental follow-up in specialized clinics; moribund infants; and infants under child protection services due to consent issues. The study (#2019-1936) was approved by the institutional review board of the Comité d’éthique de la recherche at Sainte-Justine University Hospital Center, named by the Quebec Government (#FWA00021692) and acts in accordance with Quebec and Canada laws, and the Code of Federal Regulations in the USA. Parental written informed consent was obtained for each patient.
Data collection
Parents completed a questionnaire for demographic data. Perinatal and neonatal characteristics were retrieved from medical charts. Peripheral arterial oxygen saturation (SpO2, %) was measured from preductal pulse oximetry at an upper limb. We used hemoglobin concentration in blood (HGB, g/dl) measured for clinical purposes throughout the hospital stay.
FDNIRS-DCS monitoring and data analysis
FDNIRS-DCS monitoring was performed with a commercial system (MetaOx, ISS Inc., Champaign, IL, USA) (Buckley et al., 2014; Carp et al., 2017). The system was approved by Health Canada for research purpose only. Optical energy exposure satisfied the American National Standards Institute (ANSI) standard for Safe Use of Lasers and related regulations (ANSI Z136 Standards, 2017). The optical sensor was designed and developed to image the neonatal cortex (Dehaes et al., 2011). The sensor was positioned on the middle frontal location of the head and signals were recorded at least 5 times by repositioning the sensor slightly to account for local inhomogeneities. Left and right frontal locations were assessed in sensitivity analyses. The source-detector separations were 10, 15, 20, and 25 mm for FDNIRS, and 22 mm for DCS (eight collocated detecting optical fibers). This geometry was optimized to probe the brain and minimized signal contamination due to head curvature (Dehaes et al., 2011). Analysis was performed offline by averaging middle frontal values. Data quality assessment and data rejection were performed based on published criteria prior to averaging (Roche-Labarbe et al., 2010, 2012; Lin et al., 2013a; Dehaes et al., 2014, 2015). FDNIRS and DCS systems have a distinct data quality assessment. Rejection criteria included data affected by hair, inadequate head-sensor coupling, bending of the probe, and artifacts due to motion (Roche-Labarbe et al., 2010).
Brain monitoring was performed from the first week of age to TEA, defined as between 37 and 40 weeks of post-menstrual age. The number of monitoring sessions varied between neonates and depended on GA and the length of hospital stay. Each session required 15–20 min. For each patient and FDNIRS-DCS parameter, temporal change was calculated by the difference between the value at (or near) TEA and the value measured at the first visit normalized by the temporal period that separated the two measurements (in weeks).
Absolute cerebral oxygen hemoglobin saturation (SO2, %) was calculated by the ratio between oxyhemoglobin (HbO2, μmol/L) and total hemoglobin (HbT, μmol/L) derived from FDNIRS signals. OEF was defined by the ratio between the arterio-venous O2 saturation difference (SpO2 – SvO2) and SpO2, where SvO2 was estimated via an arterial:venous contribution ratio of 0.25:0.75 as in previous studies (Watzman et al., 2000; Roche-Labarbe et al., 2012; Lin et al., 2013a; Dehaes et al., 2014, 2015). CBFi was derived from DCS signals (Boas and Yodh, 1997; Cheung et al., 2001). The calculation of CMRO2i was performed via the Fick’s principle (Kety and Schmidt, 1948) as previously described (Roche-Labarbe et al., 2012; Lin et al., 2013a,b; Dehaes et al., 2014, 2015) and estimated by the product of CBFi, HGB, OEF, and the theoretical maximum oxygen carrying capacity (γ = 1.39 ml O2/g of HGB). These calculations were performed in MATLAB (Mathworks, Natick, MA, USA).
Neurological examination
At TEA, the Amiel-Tison and Gosselin Neurological Assessment (ATGNA) was performed by a trained member of the research team blinded to the medical history and FDNIRS-DCS results. The ATGNA is a standardized 5 min exam consisting of 35 items evaluating head growth, cranial sutures, alertness, passive muscle tone of the limbs and axis, and axial motor activity. Results are synthesized in clusters to provide a final categorization: optimal (normal) exam defined as no neurological sign, or non-optimal (abnormal) exam with presence of mild/moderate or severe neurological signs (Gosselin et al., 2005). Previous works have demonstrated high inter-observer reliability (k = 0.92) (Simard et al., 2009), stability of results over time (Cochran’s Q = 7.224) (Simard et al., 2010), and ability to discriminate preterm born infants with developmental delay (Simard et al., 2011) and suboptimal neuromotor status (Leroux et al., 2013).
Statistical analysis
Descriptive statistics are reported as means (standard deviations) and proportions. The association of FDNIRS-DCS first measure and temporal changes with GA at birth was assessed using univariate linear regression models. We further examined the effect of sex using multiple linear regression models. We performed logistic regression models to estimate odds ratios (OR) and 95% confidence intervals (CI) for the associations between FDNIRS-DCS parameters and the neurological examination at TEA. Models for the relationship between FDNIRS-DCS parameters at first visit and neurological exam at TEA were adjusted for post-menstrual age at the time of measurement, as it did not systematically occur at the same post-menstrual age for each patient. We performed subgroup analyses stratifying by GA (29–32 vs 33–36 weeks GA) and by sex (females vs males). We also examined how cerebral SO2 measures were associated with the neurological examination by performing logistic regression models. In sensitivity analyses, we assessed the association of FDNIRS-DCS temporal changes measured on the left and right frontal regions with GA at birth and with neurological exam at TEA using linear and logistic regression models, respectively. P-values of <0.05 were considered statistically significant, and analyses were conducted using SAS version 9.4 (SAS Institute Inc., Cary, NC).
Results
Among 150 infants initially recruited, 133 completed the neurological assessment at TEA and were included in the study (Figure 1). Participants who were lost to follow-up were comparable to infants who completed the neurological assessment in terms of clinical characteristics, except for higher mean birth weight and birth weight Z-Score (Supplementary Table 1). The cohort was composed of 60 females (45%) and 73 males (55%), with a mean GA of 33 weeks and mean birth weight of 1831 grams. Two neonates included in the study had a suspected mild hypoxic ischemic encephalopathy. At TEA, 71 (53%) infants had an abnormal neurological examination. Infants with normal vs abnormal neurological examination were comparable for mean gestational age, birth weight, head circumference, antenatal corticosteroid, and surfactant administration (Table 1). There were more multiple births among those with an abnormal neurological examination (41% vs 19%), as well as more males (62% vs 47%), and urgent cesarean sections (56% vs 40%).
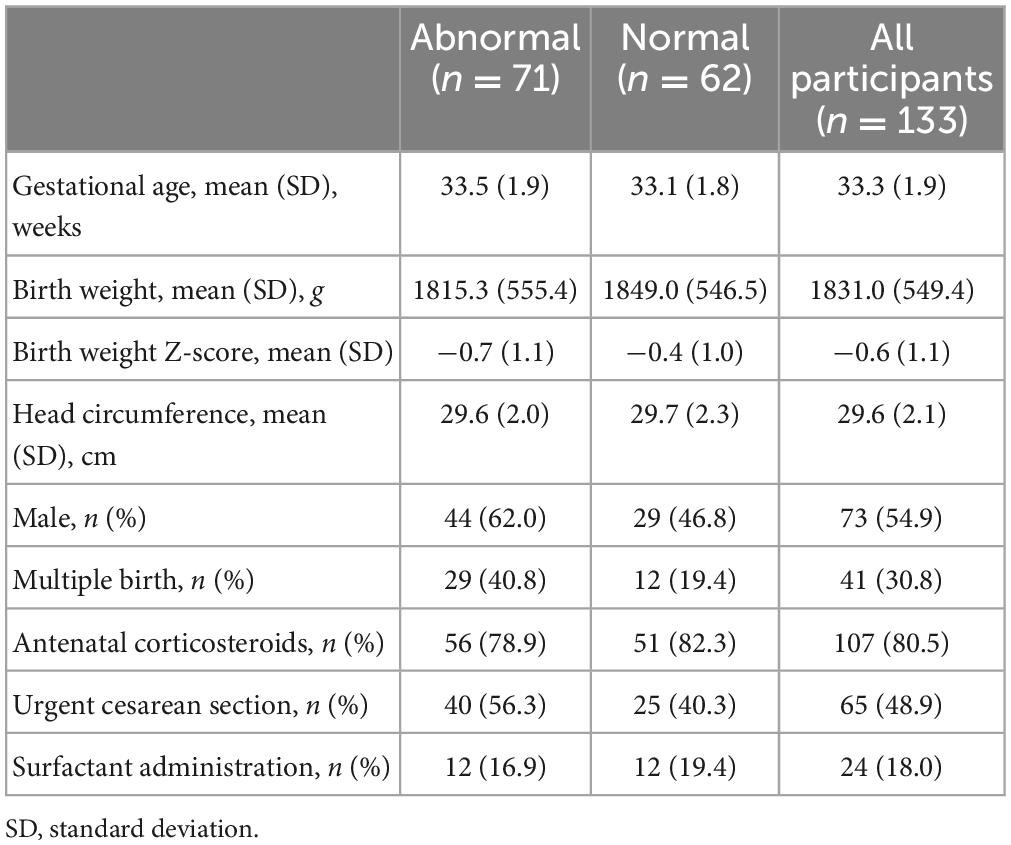
Table 1. Neonatal characteristics of the study population according to abnormal or normal neurological examination at term-equivalent age.
After data quality assessment of FDNIRS and DCS data separately, CMRO2i data at TEA were available in 90 patients, for CBFi in 92 patients, and for OEF in 98 patients. When considering FDNIRS-DCS temporal change, CMRO2i data were available in 71 patients, for CBFi in 74 patients, and for OEF in 93 patients. The average time between birth and consent was 4.13 days (SD = 2.21), and the average time between birth and first FDNIRS-DCS measurement was 4.88 days (SD = 2.94). Mean time interval between first and last FDNIRS-DCS measurements was 4.45 weeks (SD = 2.77).
Steeper increases in CBFi (p < 0.0001) and CMRO2i (p = 0.0003) were associated with higher GA at birth (Figure 2). However, there was no significant association between temporal change in OEF and GA (R2 = 0.04; p = 0.05). In contrast, there was no significant association between CMRO2i and CBFi at first visit and GA at birth. The relationship between OEF first measurement and GA reached statistical significance (R2 = 0.05, p = 0.02), but the magnitude of association was very weak (Supplementary Figure 1). In adjusted linear models, infant sex was not significantly associated with the temporal change in FDNIRS-DCS parameters (CBFi: p = 0.26; CMRO2i: p = 0.38; OEF: p = 0.72).
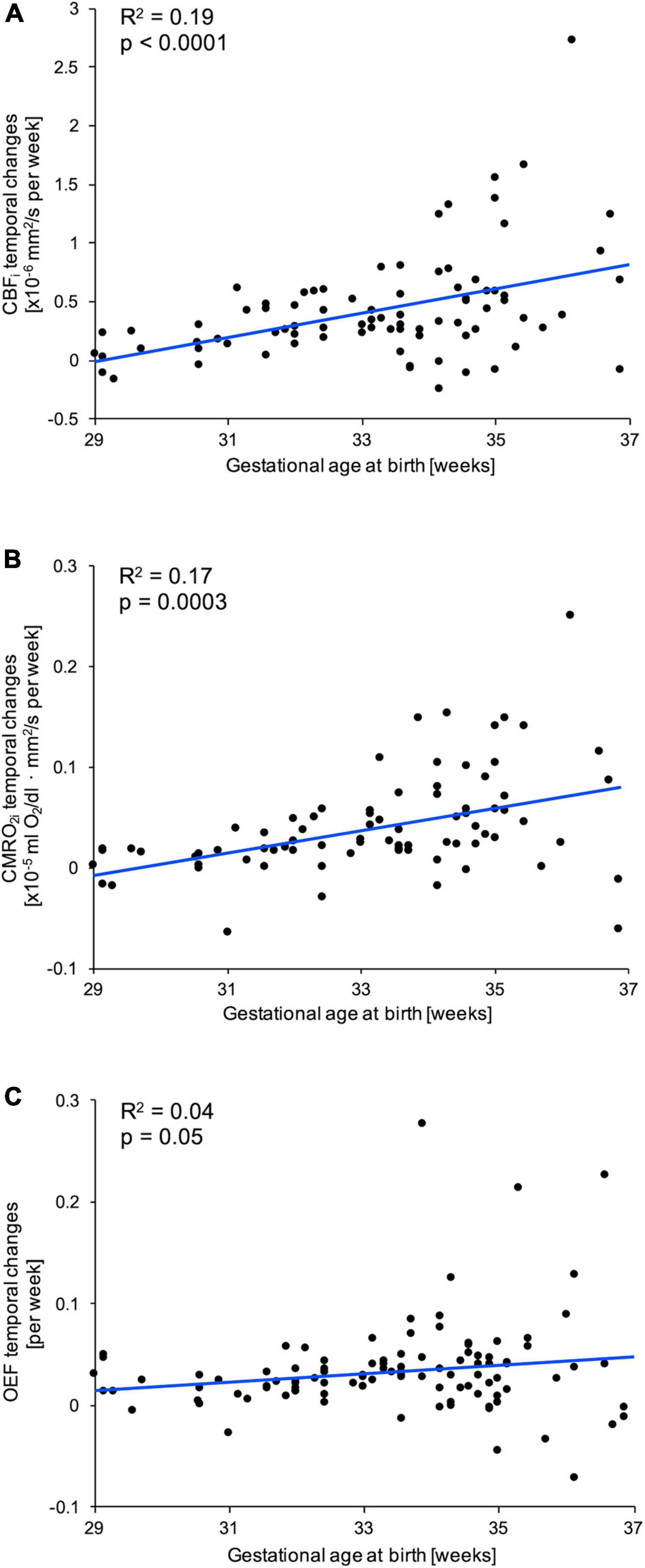
Figure 2. Associations between temporal changes of (A) cerebral blood flow index (CBFi), (B) cerebral metabolic rate of oxygen consumption index (CMRO2i), and (C) cerebral oxygen extraction fraction (OEF) with gestational age at birth (GA). For each parameter, a linear fit (blue line) and corresponding correlation coefficient (R2) and p-value are provided.
We did not identify any significant association between FDNIRS-DCS parameters and neurological examination at TEA, whether examining OR considering the first and last measurements, or the temporal change in FDNIRS-DCS parameters (Table 2). Stratifying by sex (females vs males) or by GA groups (29–32 vs 33–36 weeks GA) did not change this relationship. There were also no significant associations between cerebral SO2 at first and last visits or temporal change in SO2 and neurological examination at TEA (Supplementary Table 2).
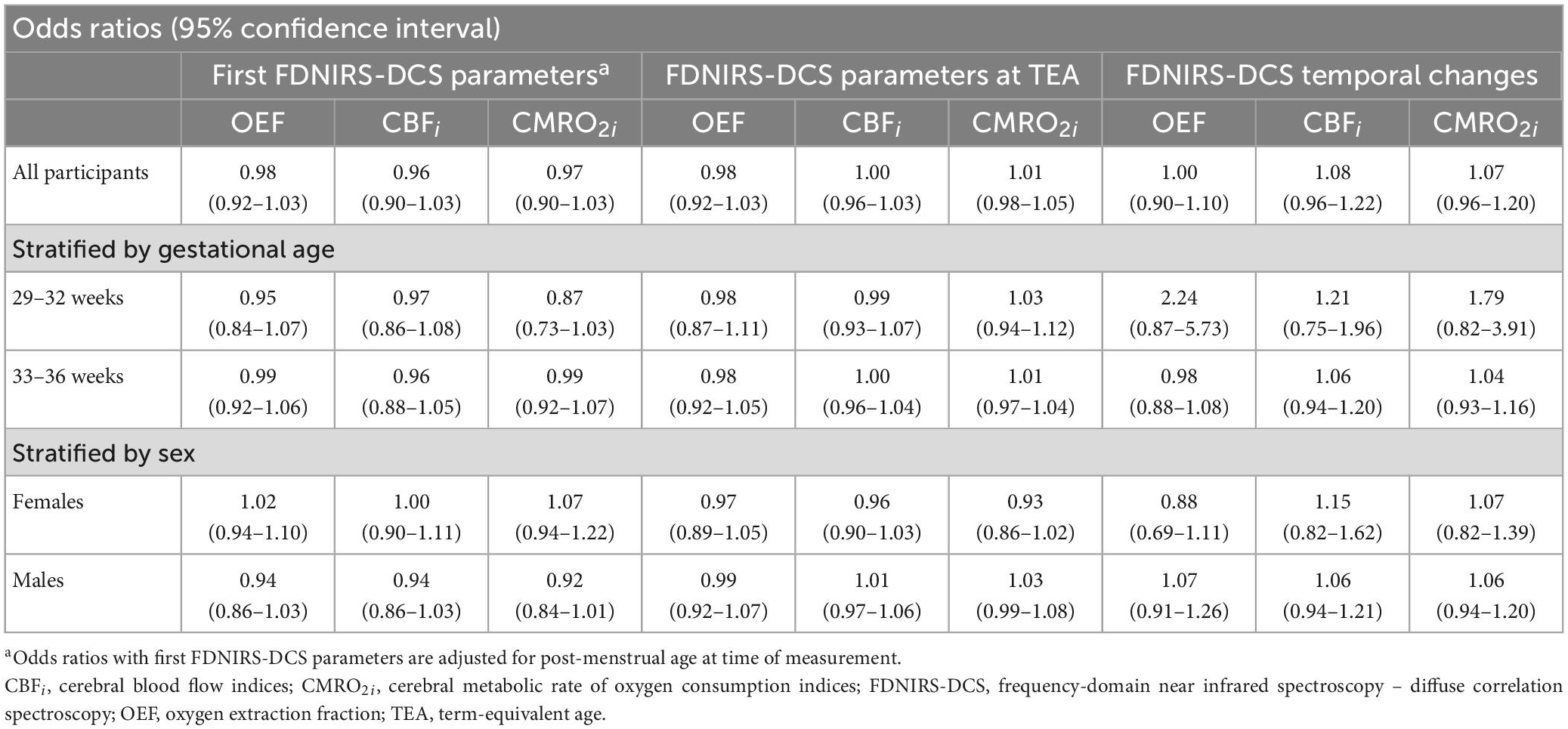
Table 2. Associations between FDNIRS-DCS parameters and temporal changes and neurological examination at term-equivalent age.
In sensitivity analyses, models considering FDNIRS-DCS measurements on the left and right frontal regions yielded results that were comparable to our main analyses examining the middle frontal location. A steeper rise in CBFi (R2 = 0.43; p < 0.0001) and CMRO2i (R2 = 0.28, p = 0.02) was significantly associated with higher GA at birth, but there was no association between temporal change in OEF and GA (R2 = 0.03, p = 0.74). Similar to our main analyses, there was no significant association with neurological exam at TEA, whether considering the temporal change in CMRO2i (OR 1.11; 95% CI 0.95–1.30), CBFi (OR 1.07; 95% CI 0.91–1.24), or OEF (OR 1.04; 95% CI 0.96–1.12).
Discussion
In this cohort of 133 infants born between 29 and 36 6/7 weeks GA, there was a significant association between steeper rises in CMRO2i and CBFi over time and increasing GA at birth. There was no association between FDNIRS-DCS parameters at TEA or temporal changes and the neurological examination at TEA. To our knowledge, this is the first study assessing the relationship between serial FDNIRS-DCS measurements of brain hemodynamics and oxygen metabolism and a standardized neurological examination at TEA in infants born between 29 and 36 6/7 weeks GA. These findings suggest that FDNIRS-DCS and the neurological exam may be complementary, informing on brain hemodynamics and metabolism and neurological status at TEA, respectively. These data highlight the need to further explore how FDNIRS-DCS could help identifying infants at high neurodevelopmental risk who would benefit from targeted intervention.
There is a growing interest in the use of cerebral NIRS monitoring to improve neurodevelopmental outcomes of infants born preterm. Studies have so far investigated how measures of SO2 and OEF obtained in the first days of life are associated with later neurodevelopmental outcomes in infants born very preterm with inconsistent results. Two cohort studies of infants born before 32 weeks GA indicated that a lower cerebral SO2 in the first 72 h of life was associated with lower cognitive scores at 24 months CA (Alderliesten et al., 2019; Katheria et al., 2021). In a study of 67 infants born before 32 weeks GA, lower SO2 in the first 2 weeks of life was associated with less optimal neurodevelopment at 2–3 years of age, while higher OEF on the first day of life was associated with lower motor scores (Verhagen et al., 2015). Also, a randomized control trial of 115 infants born very preterm assessed the impact of cerebral SO2 monitoring on neurodevelopment and found no association between SO2 in the first 72 h of life and neurological outcomes at 2 years CA (Plomgaard et al., 2019).
Evidence from diverse clinical settings indicates that FDNIRS-DCS monitoring may provide a more complete assessment of the brain’s state than SO2 or OEF alone. This is because conventional NIRS measure of SO2 values cannot distinguish whether changes occur in cerebral metabolism or delivery, or both. OEF also reflects a combination of oxygen delivery and consumption, which may limit its interpretation, while FDNIRS-DCS allows to differentiate oxygen demand (metabolism) and supply (flow), and may therefore determine their independent contribution (Lin et al., 2016). In the preterm population, research suggests that CBFi and CMRO2i may inform more accurately on early cerebral physiological and metabolic changes occurring through brain development (Roche-Labarbe et al., 2012; Lin et al., 2016). A study of 56 infants born between 24 and 37 weeks GA reported that repeated measures of CBFi and CMRO2i had a better correlation with post-menstrual age compared to SO2 (Roche-Labarbe et al., 2012). In a study monitoring infants born extremely preterm until TEA, those with germinal matrix or intraventricular hemorrhage had consistently lower CBFi and CMRO2i, compared to infants with no hemorrhage, but there was no difference in cerebral SO2 (Lin et al., 2016). FDNIRS-DCS parameters may also be insightful when neonates undergo clinical intervention that may alter cerebral hemodynamics and metabolism (Levy et al., 2021). A previous study described brain monitoring in infants born extremely preterm before and after patent ductus arteriosus treatment and demonstrated that even if CBF drops significantly, CMRO2 can be preserved with a compensatory increase of OEF (Arora et al., 2013). In newborns with single ventricle congenital heart disease, it was shown that CBFi and CMRO2i monitoring can capture unstable postoperative states and indicate lower values compared with stable states, while SO2 may remain the same regardless of the clinical state (Dehaes et al., 2015). Similarly, in a study of newborns with hypoxic ischemic encephalopathy undergoing therapeutic hypothermia, CBFi and CMRO2i were lower during treatment compared to after, but SO2 values were not significantly different between these thermic states (Dehaes et al., 2014). These findings point to a more complete and accurate assessment of cerebral hemodynamics and oxygen metabolism using CBFi and CMRO2i in comparison to SO2 alone.
In the present study, we found that increases in CBFi and CMRO2i values over time were significantly higher with increasing GA at birth, which is biologically plausible. A previous study using arterial spin labeling perfusion magnetic resonance imaging to quantify CBF found a global and regional increase in CBF values, especially in the frontal area, from 32 to 45 weeks of post-menstrual age, suggesting the high metabolic demand associated with brain growth in the third trimester (Ouyang et al., 2017). Another MRI-based study reported significant increases in global CMRO2 between 35 and 42 weeks of post-menstrual age (Liu et al., 2014). Similarly, our study using FDNIRS-DCS indicated that cerebral blood flow and oxygen metabolism increased before TEA, but also demonstrated that this increase occurred at a significantly higher rate with increasing GA at birth. However, first measurements did not correlate with GA at birth. Our findings suggest that the FDNIRS-DCS temporal changes may be more informative than a single timepoint measurement. With the growth and physiological maturation of the newborn brain over time, we would expect an increase in both cerebral blood flow and oxygen metabolism (Roche-Labarbe et al., 2010). With increasing GA, we would also expect that infants would be born with an already more mature and more voluminous brain (Fenton and Kim, 2013), resulting in higher cerebral blood flow and oxygen metabolism. In contrast to CBFi and CMRO2i, we found no association between GA and OEF temporal change. Since OEF represents the fraction of oxygen extracted from arterial blood in the brain, variations in OEF would reflect transient compensatory mechanisms to maintain cerebral oxygenation rather than brain maturation (Brown et al., 2003).
Several factors may explain why FDNIRS-DCS parameters were not associated with the neurological examination at TEA. It is possible that the standardized neurological assessment captured subtle abnormalities that were not reflected on brain hemodynamics and oxygen metabolism, or vice-versa. Also, the neurological examination and FDNIRS-DCS measures may not assess the same processes of infant brain development. FDNIRS-DCS monitoring provides information on brain hemodynamics and oxygen metabolism, which likely reflects brain growth in preterm infants (Roche-Labarbe et al., 2010). A comparative study of brain volumes extracted from structural MRI would help correlating the two modalities for brain growth. In addition, FDNIRS-DCS reflects maturational processes fueled by nutrients such as oxygen, including neuronal proliferation and migration, myelination, and synaptogenesis (Roche-Labarbe et al., 2012; Lin et al., 2013b,2016). The assessment of cerebral oxygen metabolism and blood flow evolution before TEA, a critical time window for brain developmental processes (Volpe, 2009), is thus informative. Standardized neurological examinations at TEA mirror the global and structural integrity of the brain and have been shown to correlate with neuroanatomical abnormalities or injuries identified on cerebral MRI in very low birth weight infants (Woodward et al., 2004). The ATGNA provides information on cortical and upper structure integrity such as axial tone, cranial signs, and alertness level, but also considers brainstem function via the assessment of primitive reflexes and passive limb flexor tone (Simard et al., 2011). A study including 38 infants born before 32 weeks GA found that lower brainstem, corticospinal and corticopontine pathways volumes on MRI were associated with less optimal ATGNA scores at TEA (Raguž et al., 2021). It was also shown that the ATGNA at TEA identified children at highest risk of poor developmental outcomes at 2 years CA (Simard et al., 2011). Therefore, combining the two modalities, the neurological examination and FDNIRS-DCS, could offer a thorough understanding of the neurological state, and may enhance risk stratification for neurodevelopmental impairment. This hypothesis requires long-term neurodevelopmental follow-up assessment to be confirmed.
Limitations must be acknowledged when interpreting these findings. FDNIRS-DCS data quality may be affected when measurements are performed in infants with small heads, which represents a challenge in preterm populations. The interpretation of our findings is limited to the frontal cerebral physiology as none of the measurements were performed in other cerebral regions, and therefore is not reflecting whole brain physiology. We focused on the middle frontal region in our main analyses to optimize data quality, but sensitivity analyses indicated that associations were similar whether considering the middle or the left and right frontal locations. Also, previous studies demonstrated that results were consistent when considering a single location vs multiple positions (Roche-Labarbe et al., 2012), and showed little variation in FDNIRS-DCS parameters between locations, including no significant difference in CMRO2i between the left and right frontal hemispheres (Lin et al., 2013b). The estimation of SO2 was based on assumed arterial:venous ratio previously used in premature infants with similar post-menstrual age as well as in newborns and children with congenital heart disease aged <8 years with varying arterial oxygen saturations (Watzman et al., 2000). While this ratio has been used for a broad range of ages, it may vary between birth and TEA, which could introduce a bias when interpreting OEF and CMRO2i results. However, ranges of cerebral SO2 is narrower from preterm to term gestational ages, compared with the more variable mesenteric or renal SO2 values (Alderliesten et al., 2016; Van Meurs, 2016), thus limiting the magnitude of bias. The clinical state and activity level of the newborn at time of measurement may have affected the results. We performed repeated FDNIRS-DCS measurements and considered temporal changes in our analyses, but timepoint measurements may be influenced by transient variations that do not fully reflect the baseline cerebral state. Although the ATGNA provides results that are stable over time (Simard et al., 2010), a one-time neurological examination at TEA may capture transient neurological signs or miss abnormalities that would only manifest later. Future studies examining the association between FDNIRS-DCS parameters and serial neurological examinations are needed.
Conclusion
This study shows that temporal changes in CMRO2i and CBFi are associated with GA at birth and may represent potential indicators of brain development and growth in preterm infants. At TEA, there was no association between FDNIRS-DCS parameters and the neurological examination. Whether these two measures provide different, perhaps complementary information on the infant brain needs to be further investigated.
Data availability statement
The raw data supporting the conclusions of this article will be made available by the authors, without undue reservation.
Ethics statement
The studies involving human participants were reviewed and approved by the Institutional Review Board of the Comité d’Éthique de la Recherche at CHU Sainte-Justine, named by the Quebec Government (#FWA00021692), in accordance with Quebec and Canada Laws, and the Code of Federal Regulations in the United States. Written informed consent to participate in this study was provided by the participants’ legal guardian/next of kin.
Author contributions
GC-C, M-NS, MD, and TML conceived and designed the study. OB, RC, and MD contributed to the FDNIRS-DCS assessment. M-MG, MG, and TML performed the neurological examinations. CB contributed to the clinical data collection. GC-C and OL performed the statistical analyses with input from M-NS, MD, and TML. GC-C drafted the original manuscript with critical revisions from M-NS, AMN, MD, and TML. All authors contributed to the acquisition, analysis, and interpretation of data, read, and approved the final manuscript.
Funding
This work was supported by the Canadian Institutes of Health Research (CIHR) Project Grant #156216 (to M-NS, MD, and TML), the Fonds de Recherche du Québec – Santé (FRQS) grants #299326 (to TML) and #32600 (to MD), the Natural Sciences and Engineering Research Council of Canada (NSERC) grant #RGPIN-2015-04672 (to MD), and a grant from the Ordre Professionnel de la Physiothérapie du Québec (to M-MG).
Acknowledgments
We thank Imen Ben Hmida, Anik Cloutier, Anurudhya Karthikeyan, Bohdana Marandyuk, Mi-Suk Kang Dufour, and Guylaine Aubé for their assistance during the project as well as all participating families. This manuscript is in memory of Dr. Ala Birca, pediatric neurologist and clinical researcher who contributed to the conception and design of the study.
Conflict of interest
The authors declare that the research was conducted in the absence of any commercial or financial relationships that could be construed as a potential conflict of interest.
Publisher’s note
All claims expressed in this article are solely those of the authors and do not necessarily represent those of their affiliated organizations, or those of the publisher, the editors and the reviewers. Any product that may be evaluated in this article, or claim that may be made by its manufacturer, is not guaranteed or endorsed by the publisher.
Supplementary material
The Supplementary Material for this article can be found online at: https://www.frontiersin.org/articles/10.3389/fnins.2023.1105638/full#supplementary-material
References
Alderliesten, T., Dix, L., Baerts, W., Caicedo, A., van Huffel, S., Naulaers, G., et al. (2016). Reference values of regional cerebral oxygen saturation during the first 3 days of life in preterm neonates. Pediatr. Res. 79, 55–64. doi: 10.1038/pr.2015.186
Alderliesten, T., van Bel, F., van der Aa, N. E., Steendijk, P., van Haastert, I. C., de Vries, L. S., et al. (2019). Low cerebral oxygenation in preterm infants is associated with adverse neurodevelopmental outcome. J. Pediatr. 207, 109–116.e2. doi: 10.1016/j.jpeds.2018.11.038
ANSI Z136 Standards (2017). The Laser Institute. Available online at: https://www.lia.org/resources/laser-safety-information/laser-safety-standards/ansi-z136-standards (accessed October 17, 2022).
Arora, R., Ridha, M., Lee, D. S. C., Elliott, J., Rosenberg, H. C., Diop, M., et al. (2013). Preservation of the metabolic rate of oxygen in preterm infants during indomethacin therapy for closure of the ductus arteriosus. Pediatr. Res. 73, 713–718. doi: 10.1038/pr.2013.53
Boas, D. A., and Yodh, A. G. (1997). Spatially varying dynamical properties of turbid media probed with diffusing temporal light correlation. J. Opt. Soc. Am. Opt. Image Sci. Vis. 14, 192–215. doi: 10.1364/JOSAA.14.000192
Bouyssi-Kobar, M., du Plessis, A. J., McCarter, R., Brossard-Racine, M., Murnick, J., Tinkleman, L., et al. (2016). Third trimester brain growth in preterm infants compared with in utero healthy fetuses. Pediatrics 138:e20161640. doi: 10.1542/peds.2016-1640
Brown, D. W., Hadway, J., and Lee, T.-Y. (2003). Near-infrared spectroscopy measurement of oxygen extraction fraction and cerebral metabolic rate of oxygen in newborn piglets. Pediatr. Res. 54, 861–867. doi: 10.1203/01.PDR.0000090928.93045.BE
Buckley, E. M., Carp, S., Lin, I., Nakaji, H., Dubb, J., Heuber, D., et al. (2014). “A novel combined frequency-domain near-infrared spectroscopy and diffuse correlation spectroscopy system,” in Paper presented by Biomedical Optics 2014, paper BM3A.17, (Washington, DC: Optica Publishing Group). doi: 10.1364/BIOMED.2014.BM3A.17
Buckley, E. M., Hance, D., Pawlowski, T., Lynch, J., Wilson, F. B., Mesquita, R. C., et al. (2012). Validation of diffuse correlation spectroscopic measurement of cerebral blood flow using phase-encoded velocity mapping magnetic resonance imaging. J. Biomed. Opt. 17:037007. doi: 10.1117/1.JBO.17.3.037007
Carp, S. A., Dai, G. P., Boas, D. A., Franceschini, M. A., and Kim, Y. R. (2010). Validation of diffuse correlation spectroscopy measurements of rodent cerebral blood flow with simultaneous arterial spin labeling MRI; towards MRI-optical continuous cerebral metabolic monitoring. Biomed. Opt. Express 1, 553–565. doi: 10.1364/BOE.1.000553
Carp, S. A., Farzam, P., Redes, N., Hueber, D. M., and Franceschini, M. A. (2017). Combined multi-distance frequency domain and diffuse correlation spectroscopy system with simultaneous data acquisition and real-time analysis. Biomed. Opt. Express 8, 3993–4006. doi: 10.1364/BOE.8.003993
Cheong, J. L., Doyle, L. W., Burnett, A. C., Lee, K. J., Walsh, J. M., Potter, C. R., et al. (2017). Association between moderate and late preterm birth and neurodevelopment and social-emotional development at age 2 years. JAMA Pediatr. 171:e164805. doi: 10.1001/jamapediatrics.2016.4805
Cheung, C., Culver, J. P., Takahashi, K., Greenberg, J. H., and Yodh, A. G. (2001). In vivo cerebrovascular measurement combining diffuse near-infrared absorption and correlation spectroscopies. Phys. Med. Biol. 46, 2053–2065. doi: 10.1088/0031-9155/46/8/302
Dehaes, M., Aggarwal, A., Lin, P.-Y., Rosa Fortuno, C., Fenoglio, A., Roche-Labarbe, N., et al. (2014). Cerebral oxygen metabolism in neonatal hypoxic ischemic encephalopathy during and after therapeutic hypothermia. J. Cereb. Blood Flow Metab. 34, 87–94. doi: 10.1038/jcbfm.2013.165
Dehaes, M., Cheng, H. H., Buckley, E. M., Lin, P.-Y., Ferradal, S., Williams, K., et al. (2015). Perioperative cerebral hemodynamics and oxygen metabolism in neonates with single-ventricle physiology. Biomed. Opt. Express 6, 4749–4767. doi: 10.1364/BOE.6.004749
Dehaes, M., Grant, P. E., Sliva, D. D., Roche-Labarbe, N., Pienaar, R., Boas, D. A., et al. (2011). Assessment of the frequency-domain multi-distance method to evaluate the brain optical properties: monte Carlo simulations from neonate to adult. Biomed. Opt. Express 2, 552–567. doi: 10.1364/BOE.2.000552
Diop, M., Verdecchia, K., Lee, T.-Y., and St Lawrence, K. (2011). Calibration of diffuse correlation spectroscopy with a time-resolved near-infrared technique to yield absolute cerebral blood flow measurements. Biomed. Opt. Express 2, 2068–2081. doi: 10.1364/BOE.2.002068
Durduran, T., Zhou, C., Buckley, E. M., Kim, M. N., Yu, G., Choe, R., et al. (2010). Optical measurement of cerebral hemodynamics and oxygen metabolism in neonates with congenital heart defects. J. Biomed. Opt. 15:037004. doi: 10.1117/1.3425884
El-Dib, M., Govindan, R., Aly, S., Mohamed, M., du Plessis, A., and Aly, H. (2016). EEG maturation and stability of cerebral oxygen extraction in very low birth weight infants. J. Perinatol. 36, 311–316. doi: 10.1038/jp.2015.200
Fantini, S., Franceschini-Fantini, M. A., Maier, J. S., Walker, S. A., Barbieri, B. F., and Gratton, E. (1995). Frequency-domain multichannel optical detector for noninvasive tissue spectroscopy and oximetry. Optical Eng. 34, 32–42. doi: 10.1117/12.183988
Fenton, T. R., and Kim, J. H. (2013). A systematic review and meta-analysis to revise the Fenton growth chart for preterm infants. BMC Pediatr. 13:59. doi: 10.1186/1471-2431-13-59
Goldenberg, R. L., Culhane, J. F., Iams, J. D., and Romero, R. (2008). Epidemiology and causes of preterm birth. Lancet 371, 75–84. doi: 10.1016/S0140-6736(08)60074-4
Gosselin, J., Gahagan, S., and Amiel-Tison, C. (2005). The Amiel-Tison neurological assessment at term: conceptual and methodological continuity in the course of follow-up. Ment. Retard. Dev. Disabil. Res. Rev. 11, 34–51. doi: 10.1002/mrdd.20049
Jain, V., Buckley, E. M., Licht, D. J., Lynch, J. M., Schwab, P. J., Naim, M. Y., et al. (2014). Cerebral oxygen metabolism in neonates with congenital heart disease quantified by MRI and optics. J. Cereb. Blood. Flow Metab. 34, 380–388. doi: 10.1038/jcbfm.2013.214
Katheria, A. C., Stout, J., Morales, A. L., Poeltler, D., Rich, W. D., Steen, J., et al. (2021). Association between early cerebral oxygenation and neurodevelopmental impairment or death in premature infants. J. Perinatol. 41, 743–748. doi: 10.1038/s41372-021-00942-w
Kelly, C. E., Cheong, J. L. Y., Gabra Fam, L., Leemans, A., Seal, M. L., Doyle, L. W., et al. (2016). Moderate and late preterm infants exhibit widespread brain white matter microstructure alterations at term-equivalent age relative to term-born controls. Brain Imaging Behav. 10, 41–49. doi: 10.1007/s11682-015-9361-0
Kety, S. S., and Schmidt, C. F. (1948). The nitrous oxide method for the quantitative determination of cerebral blood flow in man: theory, procedure and normal values. J. Clin. Invest. 27, 476–483. doi: 10.1172/JCI101994
Kim, M. N., Durduran, T., Frangos, S., Edlow, B. L., Buckley, E. M., Moss, H. E., et al. (2010). Noninvasive measurement of cerebral blood flow and blood oxygenation using near-infrared and diffuse correlation spectroscopies in critically brain-injured adults. Neurocrit. Care 12, 173–180. doi: 10.1007/s12028-009-9305-x
Leroux, B. G., N’guyen The Tich, S., Branger, B., Gascoin, G., Rouger, V., Berlie, I., et al. (2013). Neurological assessment of preterm infants for predicting neuromotor status at 2 years: results from the LIFT cohort. BMJ Open 3:e002431. doi: 10.1136/bmjopen-2012-002431
Levy, P. T., Pellicer, A., Schwarz, C. E., Neunhoeffer, F., Schuhmann, M. U., Breindahl, M., et al. (2021). Near-infrared spectroscopy for perioperative assessment and neonatal interventions. Pediatr. Res. doi: 10.1038/s41390-021-01791-1 [Epub ahead of print].
Lin, P.-Y., Hagan, K., Fenoglio, A., Grant, P. E., and Franceschini, M. A. (2016). Reduced cerebral blood flow and oxygen metabolism in extremely preterm neonates with low-grade germinal matrix- intraventricular hemorrhage. Sci. Rep. 6:25903. doi: 10.1038/srep25903
Lin, P.-Y., Roche-Labarbe, N., Dehaes, M., Carp, S., Fenoglio, A., Barbieri, B., et al. (2013a). Non-invasive optical measurement of cerebral metabolism and hemodynamics in infants. J. Vis. Exp. e4379. doi: 10.3791/4379
Lin, P.-Y., Roche-Labarbe, N., Dehaes, M., Fenoglio, A., Grant, P. E., and Franceschini, M. A. (2013b). Regional and hemispheric asymmetries of cerebral hemodynamic and oxygen metabolism in newborns. Cereb. Cortex 23, 339–348. doi: 10.1093/cercor/bhs023
Liu, P., Huang, H., Rollins, N., Chalak, L. F., Jeon, T., Halovanic, C., et al. (2014). Quantitative assessment of global cerebral metabolic rate of oxygen (CMRO2) in neonates using MRI. NMR Biomed. 27, 332–340. doi: 10.1002/nbm.3067
McLachlan, P. J., Kishimoto, J., Diop, M., Milej, D., Lee, D. S. C., de Ribaupierre, S., et al. (2017). Investigating the effects of cerebrospinal fluid removal on cerebral blood flow and oxidative metabolism in infants with post-hemorrhagic ventricular dilatation. Pediatr. Res. 82, 634–641. doi: 10.1038/pr.2017.131
Milej, D., He, L., Abdalmalak, A., Baker, W. B., Anazodo, U. C., Diop, M., et al. (2020). Quantification of cerebral blood flow in adults by contrast-enhanced near-infrared spectroscopy: validation against MRI. J. Cereb. Blood Flow Metab. 40, 1672–1684. doi: 10.1177/0271678X19872564
Moster, D., Lie, R. T., and Markestad, T. (2008). Long-term medical and social consequences of preterm birth. N. Engl. J. Med. 359, 262–273. doi: 10.1056/NEJMoa0706475
Nourhashemi, M., Mahmoudzadeh, M., Goudjil, S., Kongolo, G., and Wallois, F. (2020). Neurovascular coupling in the developing neonatal brain at rest. Hum. Brain Mapp. 41, 503–519. doi: 10.1002/hbm.24818
Ouyang, M., Liu, P., Jeon, T., Chalak, L., Heyne, R., Rollins, N. K., et al. (2017). Heterogeneous increases of regional cerebral blood flow during preterm brain development: preliminary assessment with pseudo-continuous arterial spin labeled perfusion MRI. Neuroimage 147, 233–242. doi: 10.1016/j.neuroimage.2016.12.034
Plomgaard, A. M., Alderliesten, T., van Bel, F., Benders, M., Claris, O., Cordeiro, M., et al. (2019). No neurodevelopmental benefit of cerebral oximetry in the first randomised trial (SafeBoosC II) in preterm infants during the first days of life. Acta Paediatr. 108, 275–281. doi: 10.1111/apa.14463
Raguž, M., Radoš, M., Kostović Srzetić, M., Kovačić, N., Žunić Išasegi, I., Benjak, V., et al. (2021). Structural changes in the cortico-ponto-cerebellar axis at birth are associated with abnormal neurological outcomes in childhood. Clin. Neuroradiol. 31, 1005–1020. doi: 10.1007/s00062-021-01017-1
Roche-Labarbe, N., Carp, S. A., Surova, A., Patel, M., Boas, D. A., Grant, P. E., et al. (2010). Noninvasive optical measures of CBV, StO(2), CBF index, and rCMRO(2) in human premature neonates’ brains in the first six weeks of life. Hum. Brain Mapp. 31, 341–352. doi: 10.1002/hbm.20868
Roche-Labarbe, N., Fenoglio, A., Aggarwal, A., Dehaes, M., Carp, S. A., Franceschini, M. A., et al. (2012). Near-infrared spectroscopy assessment of cerebral oxygen metabolism in the developing premature brain. J. Cereb. Blood Flow Metab. 32, 481–488. doi: 10.1038/jcbfm.2011.145
Simard, M.-N., Lambert, J., Lachance, C., Audibert, F., and Gosselin, J. (2009). Interexaminer reliability of Amiel-Tison neurological assessments. Pediatr. Neurol. 41, 347–352. doi: 10.1016/j.pediatrneurol.2009.05.010
Simard, M.-N., Lambert, J., Lachance, C., Audibert, F., and Gosselin, J. (2010). Stability of neurocranial signs in the first two years of life in infants at risk. Early Hum. Dev. 86, 473–478. doi: 10.1016/j.earlhumdev.2010.06.007
Simard, M.-N., Lambert, J., Lachance, C., Audibert, F., and Gosselin, J. (2011). Prediction of developmental performance in preterm infants at two years of corrected age: contribution of the neurological assessment at term age. Early Hum. Dev. 87, 799–804. doi: 10.1016/j.earlhumdev.2011.06.004
Simard, M.-N., Luu, T. M., and Gosselin, J. (2012). Concurrent validity of ages and stages questionnaires in preterm infants. Pediatrics 130, e108–e114. doi: 10.1542/peds.2011-3532
Tataranno, M. L., Vijlbrief, D. C., Dudink, J., and Benders, M. J. N. L. (2021). Precision medicine in neonates: a tailored approach to neonatal brain injury. Front. Pediatr. 9:634092. doi: 10.3389/fped.2021.634092
Thompson, D. K., Kelly, C. E., Chen, J., Beare, R., Alexander, B., Seal, M. L., et al. (2019). Characterisation of brain volume and microstructure at term-equivalent age in infants born across the gestational age spectrum. Neuroimage Clin. 21:101630. doi: 10.1016/j.nicl.2018.101630
Van Meurs, K. (2016). Near-infrared spectroscopy (NIRS): principles, evidence and clinical applications. Available online at: https://www.perinatal.com.br/simposio2016/pdf/NIRS%20in%20the%20NICU%206%2017%202016.pdf (accessed January 30, 2023).
Verhagen, E. A., Van Braeckel, K. N. J. A., van der Veere, C. N., Groen, H., Dijk, P. H., Hulzebos, C. V., et al. (2015). Cerebral oxygenation is associated with neurodevelopmental outcome of preterm children at age 2 to 3 years. Dev. Med. Child. Neurol. 57, 449–455. doi: 10.1111/dmcn.12622
Vohr, B. (2013). Long-term outcomes of moderately preterm, late preterm, and early term infants. Clin. Perinatol. 40, 739–751.
Volpe, J. J. (2009). Brain injury in premature infants: a complex amalgam of destructive and developmental disturbances. Lancet Neurol. 8, 110–124. doi: 10.1016/S1474-4422(08)70294-1
Volpe, J. J. (2022). Commentary - The late preterm infant: vulnerable cerebral cortex and large burden of disability. J. Neonatal Perinatal Med. 15, 1–5. doi: 10.3233/NPM-210803
Watzman, H. M., Kurth, C. D., Montenegro, L. M., Rome, J., Steven, J. M., and Nicolson, S. C. (2000). Arterial and venous contributions to near-infrared cerebral oximetry. Anesthesiology 93, 947–953. doi: 10.1097/00000542-200010000-00012
Keywords: frequency-domain near infrared spectroscopy, diffuse correlation spectroscopy, preterm birth, brain development, neurological examination, cerebral blood flow, cerebral oxygen metabolism
Citation: Côté-Corriveau G, Simard M-N, Beaulieu O, Chowdhury RA, Gagnon M-M, Gagnon M, Ledjiar O, Bernard C, Nuyt AM, Dehaes M and Luu TM (2023) Associations between neurological examination at term-equivalent age and cerebral hemodynamics and oxygen metabolism in infants born preterm. Front. Neurosci. 17:1105638. doi: 10.3389/fnins.2023.1105638
Received: 22 November 2022; Accepted: 17 February 2023;
Published: 02 March 2023.
Edited by:
Ardalan Aarabi, University of Picardie Jules Verne, FranceReviewed by:
Dengrong Jiang, Johns Hopkins University, United StatesStefan Carp, Harvard Medical School and Massachusetts General Hospital, United States
Copyright © 2023 Côté-Corriveau, Simard, Beaulieu, Chowdhury, Gagnon, Gagnon, Ledjiar, Bernard, Nuyt, Dehaes and Luu. This is an open-access article distributed under the terms of the Creative Commons Attribution License (CC BY). The use, distribution or reproduction in other forums is permitted, provided the original author(s) and the copyright owner(s) are credited and that the original publication in this journal is cited, in accordance with accepted academic practice. No use, distribution or reproduction is permitted which does not comply with these terms.
*Correspondence: Mathieu Dehaes, bWF0aGlldS5kZWhhZXNAdW1vbnRyZWFsLmNh; Thuy Mai Luu, dGh1eS5tYWkubHV1QHVtb250cmVhbC5jYQ==
†These authors have contributed equally to this work and share senior authorship