- College of Acupuncture, Moxibustion, and Tuina, Chengdu University of Traditional Chinese Medicine, Chengdu, China
Neurodegenerative diseases (ND) have received increasing attention due to their irreversibility, but there is still no means to completely cure ND in clinical practice. Mindfulness therapy (MT), including Qigong, Tai Chi, meditation, and yoga, etc., has become an effective complementary treatment modality in solving clinical and subclinical problems due to its advantages of low side effects, less pain, and easy acceptance by patients. MT is primarily used to treat mental and emotional disorders. In recent years, evidence has shown that MT has a certain therapeutic effect on ND with a potential molecular basis. In this review, we summarize the pathogenesis and risk factors of Alzheimer’s disease (AD), Parkinson’s disease (PD), and amyotrophic lateral sclerosis (ALS), relating to telomerase activity, epigenetics, stress, and the pro-inflammatory transcription factor nuclear factor kappa B (NF-κB) mediated inflammatory response, and analyze the molecular mechanism basis of MT to prevent and treat ND, to provide possible explanations for the potential of MT treatments for ND.
1. Introduction
Neurodegenerative diseases (ND), primarily caused by the loss of specific neurons in the central nervous system, include AD, PD, ALS, and other disorders. The etiology is primarily linked to abnormal protein accumulation, gene mutation, increased reactive oxygen species, neuroinflammation, mitochondrial dysfunction, and apoptosis (Perry et al., 2010; Gómez-Gómez and Zapico, 2019; Hou et al., 2019; Irwin and Vitiello, 2019; Wu et al., 2019; Madore et al., 2020). ND primarily affects individuals over the age of 65, and symptoms generally worsen over time (Qiu and Fratiglioni, 2018). More than 10 million people worldwide now suffer from ND annually, and this number is rising each year along with the world’s aging population (Behl et al., 2021). While ND poses a great threat to human health, it also increases the burden on the healthcare system. There is currently no means to completely cure ND in clinical practice, and most drugs can only slow the rate of ND decline and improve the quality of patient survival. The possibility of traditional TCM non-drug therapy for ND is being studied in an increasing number of research studies as TCM gains popularity (Liu et al., 2022).
MT includes qigong, Tai Chi, meditation, yoga, and other forms of physical and mental activities. Even 5,000 years ago, the ancient Chinese had mastered the self-exercise method for optimizing body and mind fitness through meditation and breath regulation. Tai Chi, meditation, yoga, and other forms of alternative medicine have progressively emerged. In a broad sense, mindfulness has been defined as a type of present-centered awareness that is unelaborate, nonjudgmental, and accepts every thought, feeling, or sensation as it arises in the attentional field (Bishop et al., 2004). The MT recommends that practitioners cultivate this awareness through meditation, actively and objectively attend to the present moment with an attitude of acceptance (not over-identification) rather than a reaction attitude, and concurrently collaborate with specific operations to achieve body and mind in order to achieve physical and mental balance. MT has been widely employed in the treatment of depression, anxiety, chronic pain, and sleep disturbances (Figure 1). Research conducted in 2013 by Gao and Xu discovered that long-term regular practice of Qigong exercise by the elderly may slow the rate of mental decline (Gao and Xu, 2013) and that MT has significant efficacy in the treatment of cognitive disorders (Rawtaer et al., 2015; Fam et al., 2020; Jin et al., 2020).
In recent years, the significance of MT in the prevention and treatment of ND has gotten a lot of attention (Dong et al., 2016; Song et al., 2017; Brasure et al., 2018; Kwok et al., 2019; Deuel and Seeberger, 2020; Guzman-Martinez et al., 2021), but its molecular mechanism has not been systematically summarized. Therefore, this paper begins with the proven molecular mechanism of MT and the risk factors for ND in order to investigate the potential molecular mechanism of MT to prevent ND.
2. Brief overview of ND
The term “ND” refers to a broad range of disabling and frequently unpredictable disease groupings that are all caused by neuronal loss and degeneration (Behl et al., 2021).
AD is the most common form of progressive ND, which is usually characterized by physical dysfunction, cognitive dysfunction, memory loss, and a progressive loss of self-care capacity. AD is the most common cause of dementia, accounting for 50–75% of all dementia patients (Yoshiyama et al., 2013). In the world, there are approximately 50 million people who have AD, and the frequency of the disease is increasing due to an aging population, according to a 2018 report from the Alzheimer’s Association. There will be 152 million people living with dementia (Livingston et al., 2020). Extracellular β-amyloid (Aβ) deposition and intracellular neurofibrillary tangles are the two pathologically distinctive abnormalities in AD (Yin et al., 2017). Among them, Aβ begins to build up nearly 20 years before dementia manifests, and the ensuing hard plaques interact with acetylcholine to cause inflammatory reactions and impair synaptic transmission, which may further cause the particular protein (tau) to degrade and exacerbate AD.
Neuroinflammation, neuronal loss and death, gliosis, synaptic loss, and impaired main synaptic function are pathogenic features of AD (Tönnies and Trushina, 2017). Along with atrophy in areas like the hippocampus, temporal lobe, parietal lobe, frontal cortex, and thalamus (Knight et al., 2016). AD is also linked to increased oxidative stress, which is considered to be a central factor in AD (Bai et al., 2022), dysregulated gene expression, cytokines, neurotrophins, and stress markers Telomere shortening and deterioration of brain connectivity are also linked to the disease’s pathophysiology (Ng et al., 2021).
After AD, PD is the most common ND and the most common severe mobility disability globally (de Lau and Breteler, 2006). It primarily affects older persons and affects 8–18 per 100,000 people annually (de Lau and Breteler, 2006). The prevalence of PD increases with age (Pringsheim et al., 2014). Dyskinesia, with progressive bradykinesia, rigidity, resting tremor, and abnormal posture and gait as its main manifestations, as well as non-motor disorders like hyposmia, constipation, sleep disturbance, depression, and cognitive impairment, are the main clinical characteristics of Parkinson’s disease (Greenamyre and Hastings, 2004). PD is a form of multisystem alpha-synucleinopathies characterized by selective loss of dopaminergic neurons and deposition of Lewy bodies in the substantia nigra, resulting in extensive involvement of other central nervous system structures and peripheral tissues (Cacabelos, 2017). Aging appears to be the only significant risk factor for PD development (Rokad et al., 2017). In addition, α-synuclein accumulation, mitochondrial dysfunction, autophagy impairment, and oxidative stress are common factors in PD pathogenesis. Factors such as oxidative stress play a central role in PD (de Lau and Breteler, 2006; Cacabelos, 2017).
ALS is a specific form of ND, but the etiology still remains unclear. ALS impacts the muscles and central nervous system (van Es et al., 2017). The majority of ALS patients, are middle-aged or elderly and above the age of 40. It is a deadly and somewhat uncommon ND. Approximately 3–6 persons out of every 100,000 people have ALS (Chiò et al., 2013). Motor neurons in the spinal cord, brain stem, and motor cortex are the main targets of ALS (Heiman-Patterson et al., 2015). Memory loss, cognitive decline, and decreased speech, swallowing, and respiratory function are all caused by neuronal death (Brown and Al-Chalabi, 2017). Statistics show that Cu/Zn superoxide dismutase gene mutations account for 20% of familial ALS cases (Heiman-Patterson et al., 2015). Aggregation and buildup of the ubiquitinated protein inclusion body TDP-43 in motor neurons are the neuropathological signs of ALS (van Es et al., 2017). The pathogenesis of ALS is also tightly linked to aging, oxidative stress (Aborode et al., 2022), RNA damage repair and axonal development, mitochondrial malfunction and autophagy, and acquired living conditions. And Oxidative stress is one major contributor to ALS pathogenesis. The result is an increased intracellular level of highly reactive free radicals, combined with defective antioxidant compensation systems that produce oxidative stress.
The aberrant buildup of cytoplasmic or nuclear proteins in the brain is the common pathogenic mechanism for various disorders, despite the fact that their etiologies and sites of lesions differ. Common risk factors include aging, genetic mutations, inflammation, and stress. Early treatment of these risk factors may lower the likelihood of developing ND and/or postpone the disease’s onset.
3. Molecular mechanism of MT
3.1. MT lengthens telomeres
MT appears to be a telomere-protective agent. Qigong (Baduanjin) (Tiwari et al., 2014), mindfulness meditation, and yoga (Schutte and Malouff, 2014; Rathore and Abraham, 2018; Dasanayaka et al., 2022) can lengthen telomeres and boost telomerase activity in both white blood cells and peripheral blood mononuclear cells (BPMC). Telomeres are eukaryotic chromosome ends that have repeated DNA sequences and unique cap-like structures that support chromosomal integrity. Telomeres are not fully reproduced because DNA polymerases are unable to complete the replication of the ends of linear molecules. As a result, telomeres shorten with each replication (Shay, 2018), and when they are sufficiently shortened, cells stall until senescence. Despite the fact that short telomeres are a pathogenic cause of senescence, telomerase re-expression can prevent premature senescence caused by telomerase deficiency and the specificity of short telomeres (Bär and Blasco, 2016).
Telomerase is the fundamental nuclear protein reverse transcriptase. Telomerase increases the amount of telomeric DNA at the ends of eukaryotic chromosomes to maintain the length of the telomeres (Epel et al., 2009). MT promotes telomeric DNA synthesis and cell division, counteracts telomere depletion due to cell division, and interrupts the telomere shortening process or lengthens telomeres by increasing telomerase activity and causing telomerase re-expression (Smith et al., 2020). Telomerase re-expression lengthens telomeres, guards against fusion and degeneration of chromosome ends, and is crucial for chromosome placement, replication, and protection, as well as for the regulation of cell growth and lifespan (Anitha et al., 2019).
3.2. MT and DNA methylation
One of the most well researched epigenetic processes, DNA methylation modifies chromatin structure without changing nucleotide base sequences. Particularly in the human brain and blood (García-Campayo et al., 2018), DNA methylation is crucial for controlling the expression of genes (Martínez-González et al., 2020). Long-term MT can methylate genes including FKBP5 (Bishop et al., 2018), SCL6A4 (Stoffel et al., 2019), NR4A2 (García-Campayo et al., 2018), and CLU (Huang et al., 2016), influencing the proteins encoded by these genes, controlling the dynamic process of methylation and demethylation, and enhancing the organism’s benefit from the epigenetic process.
3.3. MT and inflammation
Numerous trials have demonstrated that yoga, Tai Chi, and meditation practices can prevent the accumulation of ROS in cells by upregulating the activity of ROS-degrading enzymes through meditation with rhythmic breathing exercises and reducing oxidative stress markers such as ROS and 8-hydroxy-2-deoxyguanosine (Huang et al., 2014; Kumar et al., 2015; Gagrani et al., 2018) and maintaining brain homeostasis. Furthermore, meditation (Wetherell et al., 2017), Tai Chi and qigong (Campo et al., 2015; Klein et al., 2016; Larkey et al., 2016) can reduce stress and lower salivary cortisol levels in people with high baseline levels (Moraes et al., 2018), while having no effect on baseline levels in the normal population. A randomized clinical trial also confirmed that long-term mindfulness training can reduce the accumulation of cortisol and saliva in the hair (Puhlmann et al., 2021), resulting in increased immune responsiveness (Tang et al., 2009; Svetlov et al., 2019). In addition, short-term meditation has been shown to increase side-sympathetic nerve tension and decrease the activity of the sympathetic nervous system. While the reaction of the sympathetic nervous system can drive inflammation, the side neurosympathetic system can inhibit NF-κB and inflammatory reactions by activating acetylcholine (Haroon et al., 2012).
Several studies using gene expression analysis MT have identified downregulation of NF-κB target genes, which can be interpreted as a reversal of the molecular signature of chronic stress effects (Buric et al., 2017). Several randomized controlled trials have confirmed that the expression of the pro-inflammatory genes RIPK2 and COX2 (Kaliman et al., 2014), as well as NF-κB activity, are significantly reduced in PBMC in long-term meditators (Creswell et al., 2012; Black et al., 2015; Bower et al., 2015). In addition, Tai Chi (Irwin et al., 2014; Buric et al., 2017), and yoga (Bower et al., 2015) also reduced levels of the inflammatory markers C-reactive protein (CRP), tumor necrosis factor (TNF-α), and interleukin 6 (IL-6), and had downregulation of several genes involved in leukocyte production and inflammation (Buric et al., 2017).
4. Possible therapeutic mechanisms of MT against ND
4.1. MT delays aging and has the potential to combat ND cognitive impairment
The largest risk factor for the majority of ND is aging. Each lower motor unit cell type is prone to its own unique collection of aging-related phenotypes that may exacerbate the course of the ND disease (Guo and Yu, 2019; Hou et al., 2019; Pandya and Patani, 2020; Liu, 2022). The physiological process of aging produces a multitude of molecular and cellular abnormalities. DNA damage, mitochondrial dysfunction, telomere length loss, and oxidative stress are the four main causes of aging (Wyss-Coray, 2016; Hernandez-Segura et al., 2018), and telomere loss is thought to be the primary cause of aging. Age-related cognitive decline is also linked to shorter telomeres (Bär and Blasco, 2016), and Mendelian randomization research also found a causal link between shorter telomeres and increased risk of AD (Guo and Yu, 2019).
MT delays cellular senescence by increasing telomerase activity to extend telomeres (Campisi and d'Adda di Fagagna, 2007; Blackburn et al., 2015). Genetically or pharmacologically, the reduction of senescent cells improves Aβ peptide and tau protein-induced neuropathology and improves memory in AD model mice (Bussian et al., 2018; Zhang et al., 2019), thus achieving a counteracting effect on cognitive impairment (Hou et al., 2019). Notably, studies have confirmed increased gray matter volume and significantly reduced brain atrophy in the hippocampus and prefrontal cortex in expert meditators (aged 22–77) (Chételat et al., 2017), and Wolkowitz et al. hypothesized that BPMC telomerase activity may correlate with hippocampal enzyme activity and hippocampal volume (Wolkowitz et al., 2015; Deng et al., 2016). Then we can venture to speculate that meditation may have the effect of treating cognitive impairment and promoting memory by increasing telomerase activity and increasing prefrontal cortex gray matter volume as well as hippocampal volume (Cheng et al., 2013), thus reducing the risk of developing AD and/or delaying disease onset. However, this hypothesis is currently controversial and needs further validation.
4.2. The epigenetic potential of ND is controlled by MT
Epigenetic dysregulation can lead to cognitive impairment and neuronal death associated with ND (Hwang et al., 2017).
Long-term meditators’ genome-wide alterations in DNA methylation were examined by García-Campayo et al. (2018). The 64 differently methylated areas that meditators produced compared to non-meditators belong to 43 genes, and 48.4% of these regions were determined to be directly related to common human disorders, of which 9 (14%) were in genes linked to ND (AD, PD, and ALS) (García-Campayo et al., 2018). And among these related genes, nuclear receptor family 4 group A member 2 (Nr4a2) is the most differentially methylated. This gene encodes a nuclear transcriptional regulator that has been identified as a key regulator of dopaminergic (DA) neuronal differentiation, survival, and maintenance and as being essential for neuronal development, particularly for the maintenance of the DA system (Jakaria et al., 2019).
Nr4a2 prevents inflammation-mediated DA neuron death and is crucial for hippocampus synaptic plasticity and memory formation (Català-Solsona et al., 2021). It may be possible to treat DA dysfunction-related disorders like PD by promoting the methylation of this gene through meditation. It’s interesting to note that new research has discovered that altered Nr4a2 expression is likewise linked to the course of AD, and Nr4a2 agonists can speed up the degradation of Aβ by considerably reducing γ-secretase activity by upregulating an Aβ-degrading enzyme (insulin-degrading enzyme). The characteristic AD symptoms were significantly reduced in the agonist-treated mouse model of AD, and cognitive performance was significantly enhanced (Jakaria et al., 2019; Moon et al., 2019).
Additionally, the CpG sites of FKBP5 GREs in intron 7 and the promoter region speed up age-related demethylation in AD patients, increasing the expression of FKBP51 mRNA and protein with aging. Tau cannot be separated into less toxic tangles due to FKBP51’s interference with tau degradation and promotion of tau oligomer formation. This increases neurotoxic tau, which advances AD (Blair et al., 2013). Long-term meditation increases FKBP5 DNA methylation and decreases FKBP51 expression, which reduces tau neurotoxicity and slows the course of AD. In contrast, decreased FKBP5 DNA methylation increases FKBP51 expression.
Epel et al. also discovered that CLU gene expression and PSEN1 gene expression were both decreased following meditation (Epel et al., 2016; Huang et al., 2016). Reduced CLU gene expression can lower the risk of AD and PD, according to genome-wide correlation studies that have identified the CLU gene as a well-established risk gene related to AD and PD (Karch and Goate, 2015; Lin et al., 2021). Although PSEN1 encodes the -secretase necessary for the synthesis of Aβ peptides, PSEN1’s decreased expression leads to insufficient synthesis of the γ-secretase complex, which may be caused by a decrease in the synthesis of Aβ peptides as a result of meditation and a relative decrease in the γ-secretase needed for the hydrolysis of Aβ peptides.
By examining blood markers after meditation, Epel et al. also discovered that the level of Aβ40 in the blood of meditators decreased (Epel et al., 2016). Since CLU induces the deposition and removal of Aβ (Maturana-Candelas et al., 2021), meditation may lower the level of Aβ40 by reducing the expression of the CLU gene, thereby lowering the Aβ42 /Aβ40 ratio and lowering the risk of AD (Chouraki et al., 2015). The epigenetic modifications in subtelomeric areas may be related to telomere length, and DNA methylation may also be implicated in the stability of telomere length in long-term meditators’ specific subtelomeric regions (Mendioroz et al., 2020).
Long-term meditators, compared to non-meditators, have different areas that are methylated in pathways related to cellular senescence, neurotransmission, lipid and glucose metabolism, immunology, and inflammation (Kaliman, 2019). These genes’ methylation may affect ND directly or indirectly. Reduced Aβ deposition has a protective or mitigating effect on cognitive impairment caused by AD and PD. This effect is due to the methylation of ND-related genes resulting from MT, such as the Nr4a2 gene and the CLU gene, whose expression is strongly associated with the deposition and clearance of Aβ and tau.
Through the epigenetic process of DNA methylation, meditation may control the expression of ND-related genes, alleviating symptoms and slowing the course of the disease. However, long-term meditation accumulation might be necessary to regulate gene expression, which short-term meditation cannot do. This hypothesis has to be proven in trials using larger sample sizes.
4.3. MT’s potential to combat ND-related neuroinflammation
Chronic oxidative stress results in the accumulation of reactive oxygen species, which damages target molecules like DNA, proteins, and lipid structures. An imbalance in the antioxidant system is one of the key mechanisms causing ND (Tönnies and Trushina, 2017). The antioxidant system may become unbalanced, the brain’s equilibrium may be lost, and ND may result if the balance between the production and consumption of reactive oxygen species is upset (Radi et al., 2014; Nissanka and Moraes, 2018; Stefanatos and Sanz, 2018; Collin, 2019; Yeung et al., 2021).
Chronic stress may promote the loss of nigrostriatal cells in PD, hastening the disease’s course (van der Heide et al., 2021), and stressful conditions may intensify the condition’s motor symptoms, such as tremor.
Additionally, in human ALS fibroblasts and pluripotent stem cell iPSC-motoneurons, prolonged stress stimulates the production of stress granules and pathogenic TDP-43 aggregates, accelerating the course of ALS (Ratti et al., 2020).
Stress also leads to a reduction in hippocampal volume and a decrease in the number of glucocorticoid receptors (GR) in the hippocampus (Frodl and O’Keane, 2013), as well as stimulation of the hypothalamic–pituitary–adrenal (HPA) axis and sympathetic nervous system, which promotes the release of glucocorticoids (GC) and catecholamines. The decrease in the number of GR and increased GC release leads to elevated GC levels and brain atrophy, such as in the hippocampus, due to prolonged high levels of GC stimulation, which puts the person in a state of extreme stress and anxiety and exacerbates the progression of AD (Boutrup et al., 2019).
In addition, high levels of GC initiate an immune response in brain microglia, making them pro-inflammatory and promoting a neurotoxic response (Milligan Armstrong et al., 2021). In an AD rat experiment, it was demonstrated that Aβ25-35 amyloid toxicity affects the adaptive response of the HPA axis to stress (Brureau et al., 2013), resulting in chronically high levels of cortisol in patients (Vyas and Maatouk, 2013).
The HPA axis is a crucial neuroendocrine signaling system that regulates physiological homeostasis and stress reactions. It is a well-known characteristic of AD to have a very active HPA axis (Notarianni, 2013), which is indicated by excessive cortisol output. The HPA axis’s overproduction of cortisol affects somatic tissues through blood flow, and elevated levels of GC, including cortisol, lead to hemodynamic, endocrine, and immune system problems as well as increased accumulation of Aβ and tau, which cause increased brain atrophy, behavioral deficits, mood disorders (Du and Pang, 2015), and/or cognitive decline (Milligan Armstrong et al., 2021), all of which accelerate the progression of AD and PD (de Pablos et al., 2014; Ennis et al., 2017).
Additionally, it has been demonstrated that chronic inflammation raises the risk of ND (Buric et al., 2017). It is well established that the nuclear factor NF-κB, a transcription factor that promotes inflammation, can be inhibited by the cortisol-GR complex, preventing the transcription of genes that promote inflammation. NF-κB can transcriptionally regulate the expression of cytokines. The transcription factor is closely linked to mammalian aging, inflammation, and stress. As a molecular indicator of chronic stress (Buric et al., 2017), the upregulation of pro-inflammatory genes is thought to cause dysregulation of GC secretion, decreased sensitivity to GR in the brain and immune cells, and a lack of suppression of NF-κB-mediated inflammation (Boutrup et al., 2019). This vicious cycle ultimately increases the risk of developing ND.
Neuroinflammation is associated with ND and is one of the important mechanisms in the development of ND (Kwon and Koh, 2020), and both central inflammation and systemic inflammation can accelerate the progression of ND (Perry et al., 2010; Shih et al., 2015). MT delays aging due to DNA damage by inhibiting NF-κB activity (Tilstra et al., 2012), which in turn delays aging-related neurodegeneration (Tilstra et al., 2012; Álvarez-López et al., 2022).
Long-term practice of meditation, yoga, Tai Chi, and qigong induces downregulation of NF-κB-related upstream and downstream targets, which may reduce stress and inflammatory responses by inhibiting the NF-κB pathway through the RIPK2 pathway (Bhasin et al., 2013), delaying the onset of ND. Animal studies have shown that acute activation of pro-inflammatory cytokine signaling in the brain in response to peripheral immune activation is associated with deficits in hippocampal-dependent memory (Dantzer et al., 2008). MT reduction in stress can reduce the hyperactivity of the HPA axis, resulting in a decrease in the level of GC accumulated in the nuclei of neurons in different brain regions, especially the hippocampus, hypothalamus, and amygdala, which are rich in GR. Brain shrinkage and memory impairment may be delayed as a result of MT’s lowering of pro-inflammatory cytokines. Regular MT training can have beneficial effects on ND by improving neuroendocrine stress responses, improving HPA axis stress responses (Khalsa, 2015), and nuclear receptor-mediated transcriptional changes to reduce neuroinflammation (Figure 2).
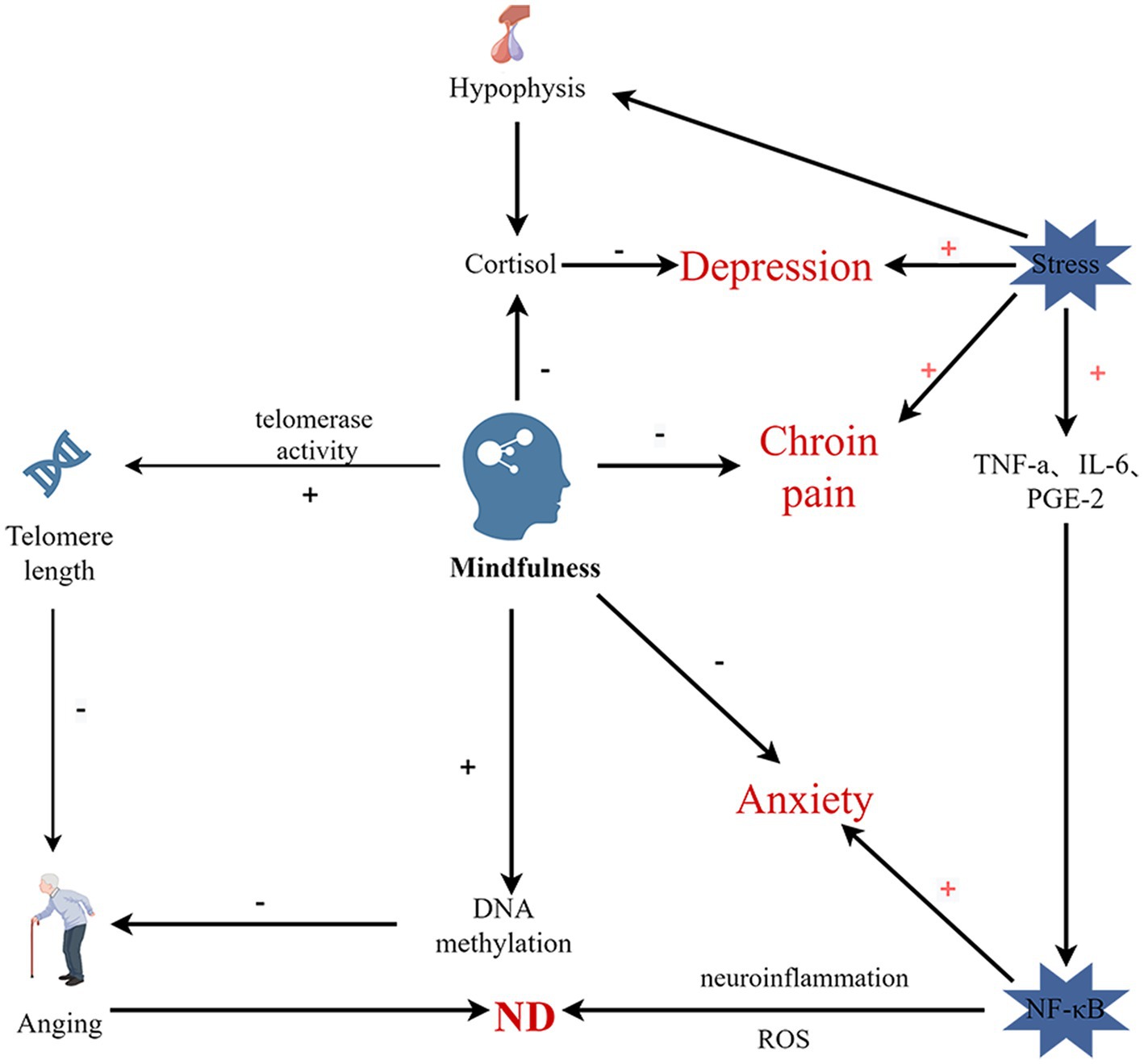
Figure 2. Schematic diagram of hypothesized pathway of the effects of MT on mental and physical wellbeing and aging. NF-kB-Nuclear Factor kappa B; IL-6-Interleukin-6; TNF-α-Tumor Necrosis Factor-alpha; PGE-2-prostaglandin E2; ROS-Reactive Oxygen Species. Stress activates the HPV axis, releasing cortisol, and increased cortisol and oxidative stress lead to increased stress. MT can prevent and treat ND by promoting telomerase activity, thereby lengthening telomeres and slowing aging, and by promoting DNA methylation associated with ND and reducing neuroinflammation (By Figdraw).
5. Discussion
Current research finds that the mechanisms affecting the onset and development of diseases mainly include: incorrect folding and aggregation of proteins, neuropathy, cellular procedural death and aging. Circadian rhythm disorder, nutritional inadequacies, stress, inflammatory reactions, age, and gene mutations are typical risk factors for ND (Perry et al., 2010; Gómez-Gómez and Zapico, 2019; Hou et al., 2019; Irwin and Vitiello, 2019; Wu et al., 2019; Madore et al., 2020). MT can intervene in these risk variables to prevent and treat ND. By increasing telomerase activity to postpone aging, lowering anxiety and depression to regulate circadian rhythms (Yingwei et al., 2019), lowering stress and NF-κB-induced neuroinflammatory responses, and changing DNA methylation relevant to ND to regulate gene expression, MT can prevent and reduce the progression of ND. In turn, these processes engage in mutually beneficial interactions. Telomerase, for instance, possesses antioxidant, anti-apoptotic, neurotrophic, and neurogenesis-promoting properties that help restore brain cell suppleness and viability in addition to lengthening telomeres to delay aging. Additionally, oxidative stress and inflammation can shorten telomeres and speed up aging (Bär and Blasco, 2016). Antioxidants have neuroprotective effects (Hou et al., 2019), and MT can reduce the occurrence of neuroinflammation and slow down the aging process by reducing oxidative stress. Meanwhile, bioinformatic analysis predicted that epigenetic responses to MT exercises may regulate inflammatory pathways dependent on tumor necrosis factor α and NF-κB signaling. Through these mechanisms, MT can create a positive cycle that will improve the symptoms of ND and slow the ND process.
The clinical effects of MT on ND have demonstrated that MT can enhance patients’ quality of life, improve symptoms like anxiety and depression, and slow the progression of the disease. However, the majority of these trials evaluated the effectiveness using a scale. According to the current study of mind-based brain area research, inflammatory factor research, and the subjective score table (Newberg et al., 2014; Lou, 2017; Kwok et al., 2019), and less frequently with biological markers. Extensive experimental research is still needed to understand the mechanism of MT activity in ND. Future research should explore if MT intervention at a younger age would lower the incidence of ND. More clinical trials are also required to determine whether traditional Chinese medicine offers unique, superior benefits for ND prevention and treatment. Mind-based physical and mental therapy is an interesting and beneficial way, in the modern society, where public health awareness is generally enhanced, early and continuous mind intervention will play a role in disease with age to prevent and delay illness, and it is worth our study.
Author contributions
CW drafted the manuscript. YF revised the manuscript. All authors contributed to the article and approved the submitted version.
Funding
This research was supported by the National Natural Science Foundation of China (grant number: 82074575).
Conflict of interest
The authors declare that the research was conducted in the absence of any commercial or financial relationships that could be construed as a potential conflict of interest.
Publisher’s note
All claims expressed in this article are solely those of the authors and do not necessarily represent those of their affiliated organizations, or those of the publisher, the editors and the reviewers. Any product that may be evaluated in this article, or claim that may be made by its manufacturer, is not guaranteed or endorsed by the publisher.
References
Aborode, A. T., Pustake, M., Awuah, W. A., Alwerdani, M., Shah, P., Yarlagadda, R., et al. (2022). Targeting oxidative stress mechanisms to treat Alzheimer’s and Parkinson’s disease: a critical review. Oxidative Med. Cell. Longev. 2022, 7934442–7934449. doi: 10.1155/2022/7934442
Álvarez-López, M. J., Conklin, Q. A., Cosín-Tomás, M., Shields, G. S., King, B. G., Zanesco, A. P., et al. (2022). Changes in the expression of inflammatory and epigenetic-modulatory genes after an intensive meditation retreat. Compr. Psychoneuroendocrinol. 11:100152. doi: 10.1016/j.cpnec.2022.100152
Anitha, A., Thanseem, I., Vasu, M. M., Viswambharan, V., and Poovathinal, S. A. (2019). Telomeres in neurological disorders. Adv. Clin. Chem. 90, 81–132. doi: 10.1016/bs.acc.2019.01.003
Bai, R., Guo, J., Ye, X.-Y., Xie, Y., and Xie, T. (2022). Oxidative stress: the core pathogenesis and mechanism of Alzheimer’s disease. Ageing Res. Rev. 77:101619. doi: 10.1016/j.arr.2022.101619
Bär, C., and Blasco, M. A. (2016). Telomeres and telomerase as therapeutic targets to prevent and treat age-related diseases. F1000Res 5 5:F1000 Faculty Rev-89. doi: 10.12688/f1000research.7020.1
Behl, T., Kaur, G., Sehgal, A., Singh, S., Bhatia, S., Al-Harrasi, A., et al. (2021). Elucidating the multi-targeted role of nutraceuticals: a complementary therapy to starve neurodegenerative diseases. Int. J. Mol. Sci. 22:4045. doi: 10.3390/ijms22084045
Bhasin, M. K., Dusek, J. A., Chang, B.-H., Joseph, M. G., Denninger, J. W., Fricchione, G. L., et al. (2013). Relaxation response induces temporal transcriptome changes in energy metabolism, insulin secretion and inflammatory pathways. PLoS One 8:e62817. doi: 10.1371/journal.pone.0062817
Bishop, S. R., Lau, M., Shapiro, S., Carlson, L., Anderson, N. D., Carmody, J., et al. (2004). Mindfulness: a proposed operational definition. Clin. Psychol. Sci. Pract. 11, 230–241. doi: 10.1093/clipsy.bph077
Bishop, J. R., Lee, A. M., Mills, L. J., Thuras, P. D., Eum, S., Clancy, D., et al. (2018). Methylation of FKBP5 and SLC6A4 in relation to treatment response to mindfulness based stress reduction for posttraumatic stress disorder. Front. Psych. 9:418. doi: 10.3389/fpsyt.2018.00418
Black, D. S., O’Reilly, G. A., Olmstead, R., Breen, E. C., and Irwin, M. R. (2015). Mindfulness meditation and improvement in sleep quality and daytime impairment among older adults with sleep disturbances: a randomized clinical trial. JAMA Intern. Med. 175, 494–501. doi: 10.1001/jamainternmed.2014.8081
Blackburn, E. H., Epel, E. S., and Lin, J. (2015). Human telomere biology: a contributory and interactive factor in aging, disease risks, and protection. Science 350, 1193–1198. doi: 10.1126/science.aab3389
Blair, L. J., Nordhues, B. A., Hill, S. E., Scaglione, K. M., O’Leary, J. C., Fontaine, S. N., et al. (2013). Accelerated neurodegeneration through chaperone-mediated oligomerization of tau. J. Clin. Invest. 123, 4158–4169. doi: 10.1172/JCI69003
Boutrup, R. J., Jørgensen, M. M., Gregersen, N., Frost, L., Aagaard, H., Djernis, D., et al. (2019). Psykologiske, neurologiske og cellulære virkningsmekanismer ved mindfulnesstræning, vol. 4.
Bower, J. E., Crosswell, A. D., Stanton, A. L., Crespi, C. M., Winston, D., Arevalo, J., et al. (2015). Mindfulness meditation for younger breast cancer survivors: a randomized controlled trial. Cancer 121, 1231–1240. doi: 10.1002/cncr.29194
Brasure, M., Desai, P., Davila, H., Nelson, V. A., Calvert, C., Jutkowitz, E., et al. (2018). Physical activity interventions in preventing cognitive decline and Alzheimer-type dementia: a systematic review. Ann. Intern. Med. 168, 30–38. doi: 10.7326/M17-1528
Brown, R. H., and Al-Chalabi, A. (2017). Amyotrophic lateral sclerosis. N. Engl. J. Med. 377, 162–172. doi: 10.1056/NEJMra1603471
Brureau, A., Zussy, C., Delair, B., Ogier, C., Ixart, G., Maurice, T., et al. (2013). Deregulation of hypothalamic-pituitary-adrenal axis functions in an Alzheimer’s disease rat model. Neurobiol. Aging 34, 1426–1439. doi: 10.1016/j.neurobiolaging.2012.11.015
Buric, I., Farias, M., Jong, J., Mee, C., and Brazil, I. A. (2017). What is the molecular signature of mind-body interventions? A systematic review of gene expression changes induced by meditation and related practices. Front. Immunol. 8:670. doi: 10.3389/fimmu.2017.00670
Bussian, T. J., Aziz, A., Meyer, C. F., Swenson, B. L., van Deursen, J. M., and Baker, D. J. (2018). Clearance of senescent glial cells prevents tau-dependent pathology and cognitive decline. Nature 562, 578–582. doi: 10.1038/s41586-018-0543-y
Cacabelos, R. (2017). Parkinson’s disease: from pathogenesis to pharmacogenomics. Int. J. Mol. Sci. 18:E551. doi: 10.3390/ijms18030551
Campisi, J., and d'Adda di Fagagna, F. (2007). Cellular senescence: when bad things happen to good cells. Nat. Rev. Mol. Cell Biol. 8, 729–740. doi: 10.1038/nrm2233
Campo, R. A., Light, K. C., O’Connor, K., Nakamura, Y., Lipschitz, D., LaStayo, P. C., et al. (2015). Blood pressure, salivary cortisol, and inflammatory cytokine outcomes in senior female cancer survivors enrolled in a tai chi chih randomized controlled trial. J. Cancer Surviv. 9, 115–125. doi: 10.1007/s11764-014-0395-x
Català-Solsona, J., Miñano-Molina, A. J., and Rodríguez-Álvarez, J. (2021). Nr4a2 transcription factor in hippocampal synaptic plasticity, memory and cognitive dysfunction: a perspective review. Front. Mol. Neurosci. 14:786226. doi: 10.3389/fnmol.2021.786226
Cheng, G. L. F., Zeng, H., Leung, M.-K., Zhang, H.-J., Lau, B. W. M., Liu, Y.-P., et al. (2013). Heroin abuse accelerates biological aging: a novel insight from telomerase and brain imaging interaction. Transl. Psychiatry 3:e260. doi: 10.1038/tp.2013.36
Chételat, G., Mézenge, F., Tomadesso, C., Landeau, B., Arenaza-Urquijo, E., Rauchs, G., et al. (2017). Reduced age-associated brain changes in expert meditators: a multimodal neuroimaging pilot study. Sci. Rep. 7:10160. doi: 10.1038/s41598-017-07764-x
Chiò, A., Logroscino, G., Traynor, B. J., Collins, J., Simeone, J. C., Goldstein, L. A., et al. (2013). Global epidemiology of amyotrophic lateral sclerosis: a systematic review of the published literature. Neuroepidemiology 41, 118–130. doi: 10.1159/000351153
Chouraki, V., Beiser, A., Younkin, L., Preis, S. R., Weinstein, G., Hansson, O., et al. (2015). Plasma amyloid-β and risk of Alzheimer’s disease in the Framingham heart study. Alzheimers Dement. 11, 249–257.e1. doi: 10.1016/j.jalz.2014.07.001
Collin, F. (2019). Chemical basis of reactive oxygen species reactivity and involvement in neurodegenerative diseases. Int. J. Mol. Sci. 20:E2407. doi: 10.3390/ijms20102407
Creswell, J. D., Irwin, M. R., Burklund, L. J., Lieberman, M. D., Arevalo, J. M. G., Ma, J., et al. (2012). Mindfulness-based stress reduction training reduces loneliness and pro-inflammatory gene expression in older adults: a small randomized controlled trial. Brain Behav. Immun. 26, 1095–1101. doi: 10.1016/j.bbi.2012.07.006
Dantzer, R., O’Connor, J. C., Freund, G. G., Johnson, R. W., and Kelley, K. W. (2008). From inflammation to sickness and depression: when the immune system subjugates the brain. Nat. Rev. Neurosci. 9, 46–56. doi: 10.1038/nrn2297
Dasanayaka, N. N., Sirisena, N. D., and Samaranayake, N. (2022). Impact of meditation-based lifestyle practices on mindfulness, wellbeing, and plasma telomerase levels: a case-control study. Front. Psychol. 13:846085. doi: 10.3389/fpsyg.2022.846085
de Lau, L. M. L., and Breteler, M. M. B. (2006). Epidemiology of Parkinson’s disease. Lancet Neurol. 5, 525–535. doi: 10.1016/S1474-4422(06)70471-9
de Pablos, R. M., Herrera, A. J., Espinosa-Oliva, A. M., Sarmiento, M., Muñoz, M. F., Machado, A., et al. (2014). Chronic stress enhances microglia activation and exacerbates death of nigral dopaminergic neurons under conditions of inflammation. J. Neuroinflammation 11:34. doi: 10.1186/1742-2094-11-34
Deng, W., Cheung, S. T., Tsao, S. W., Wang, X. M., and Tiwari, A. F. Y. (2016). Telomerase activity and its association with psychological stress, mental disorders, lifestyle factors and interventions: a systematic review. Psychoneuroendocrinology 64, 150–163. doi: 10.1016/j.psyneuen.2015.11.017
Deuel, L. M., and Seeberger, L. C. (2020). Complementary therapies in Parkinson disease: a review of acupuncture, tai chi, Qi gong, yoga, and Cannabis. Neurotherapeutics 17, 1434–1455. doi: 10.1007/s13311-020-00900-y
Dong, J., Cui, Y., Li, S., and Le, W. (2016). Current pharmaceutical treatments and alternative therapies of Parkinson’s disease. Curr. Neuropharmacol. 14, 339–355. doi: 10.2174/1570159x14666151120123025
Du, X., and Pang, T. Y. (2015). Is dysregulation of the HPA-Axis a Core pathophysiology mediating co-morbid depression in neurodegenerative diseases? Front. Psych. 6:32. doi: 10.3389/fpsyt.2015.00032
Ennis, G. E., An, Y., Resnick, S. M., Ferrucci, L., O’Brien, R. J., and Moffat, S. D. (2017). Long-term cortisol measures predict Alzheimer disease risk. Neurology 88, 371–378. doi: 10.1212/WNL.0000000000003537
Epel, E., Daubenmier, J., Moskowitz, J. T., Folkman, S., and Blackburn, E. (2009). Can meditation slow rate of cellular aging? Cognitive stress, mindfulness, and telomeres. Ann. N. Y. Acad. Sci. 1172, 34–53. doi: 10.1111/j.1749-6632.2009.04414.x
Epel, E. S., Puterman, E., Lin, J., Blackburn, E. H., Lum, P. Y., Beckmann, N. D., et al. (2016). Meditation and vacation effects have an impact on disease-associated molecular phenotypes. Transl. Psychiatry 6:e880. doi: 10.1038/tp.2016.164
Fam, J., Sun, Y., Qi, P., Lau, R. C., Feng, L., Kua, E. H., et al. (2020). Mindfulness practice alters brain connectivity in community-living elders with mild cognitive impairment. Psychiatry Clin. Neurosci. 74, 257–262. doi: 10.1111/pcn.12972
Frodl, T., and O’Keane, V. (2013). How does the brain deal with cumulative stress? A review with focus on developmental stress, HPA axis function and hippocampal structure in humans. Neurobiol. Dis. 52, 24–37. doi: 10.1016/j.nbd.2012.03.012
Gagrani, M., Faiq, M. A., Sidhu, T., Dada, R., Yadav, R. K., Sihota, R., et al. (2018). Meditation enhances brain oxygenation, upregulates BDNF and improves quality of life in patients with primary open angle glaucoma: a randomized controlled trial. Restor. Neurol. Neurosci. 36, 741–753. doi: 10.3233/RNN-180857
Gao, L., and Xu, S. (2013). Investigation and research on the effect of health qigong on delaying the mental decline of the elderly. Sports Cult. Guide 14, 32–34+39. doi: 10.3969/j.issn.1671-1572.2013.07.009
García-Campayo, J., Puebla-Guedea, M., Labarga, A., Urdánoz, A., Roldán, M., Pulido, L., et al. (2018). Epigenetic response to mindfulness in peripheral blood leukocytes involves genes linked to common human diseases. Mindfulness 9, 1146–1159. doi: 10.1007/s12671-017-0851-6
Gómez-Gómez, M. E., and Zapico, S. C. (2019). Frailty, cognitive decline, neurodegenerative diseases and nutrition interventions. Int. J. Mol. Sci. 20:E2842. doi: 10.3390/ijms20112842
Greenamyre, J. T., and Hastings, T. G. (2004). Biomedicine. Parkinson’s--divergent causes, convergent mechanisms. Science 304, 1120–1122. doi: 10.1126/science.1098966
Guo, Y., and Yu, H. (2019). Leukocyte telomere length shortening and Alzheimer’s disease etiology. J. Alzheimers Dis. 69, 881–885. doi: 10.3233/JAD-190134
Guzman-Martinez, L., Calfío, C., Farias, G. A., Vilches, C., Prieto, R., and Maccioni, R. B. (2021). New Frontiers in the prevention, diagnosis, and treatment of Alzheimer’s disease. J. Alzheimers Dis. 82, S51–S63. doi: 10.3233/JAD-201059
Haroon, E., Raison, C. L., and Miller, A. H. (2012). Psychoneuroimmunology meets neuropsychopharmacology: translational implications of the impact of inflammation on behavior. Neuropsychopharmacology 37, 137–162. doi: 10.1038/npp.2011.205
Heiman-Patterson, T. D., Blankenhorn, E. P., Sher, R. B., Jiang, J., Welsh, P., Dixon, M. C., et al. (2015). Genetic background effects on disease onset and lifespan of the mutant dynactin p150Glued mouse model of motor neuron disease. PLoS One 10:e0117848. doi: 10.1371/journal.pone.0117848
Hernandez-Segura, A., Nehme, J., and Demaria, M. (2018). Hallmarks of cellular senescence. Trends Cell Biol. 28, 436–453. doi: 10.1016/j.tcb.2018.02.001
Hou, Y., Dan, X., Babbar, M., Wei, Y., Hasselbalch, S. G., Croteau, D. L., et al. (2019). Ageing as a risk factor for neurodegenerative disease. Nat. Rev. Neurol. 15, 565–581. doi: 10.1038/s41582-019-0244-7
Huang, X.-Y., Eungpinichpong, W., Silsirivanit, A., Nakmareong, S., and Wu, X.-H. (2014). Tai chi improves oxidative stress response and DNA damage/repair in young sedentary females. J. Phys. Ther. Sci. 26, 825–829. doi: 10.1589/jpts.26.825
Huang, F., Shang, Y., Luo, Y., Wu, P., Huang, X., Tan, X., et al. (2016). Lower prevalence of Alzheimer’s disease among Tibetans: association with religious and genetic factors. J. Alzheimers Dis. 50, 659–667. doi: 10.3233/JAD-150697
Hwang, J.-Y., Aromolaran, K. A., and Zukin, R. S. (2017). The emerging field of epigenetics in neurodegeneration and neuroprotection. Nat. Rev. Neurosci. 18, 347–361. doi: 10.1038/nrn.2017.46
Irwin, M. R., Olmstead, R., Breen, E. C., Witarama, T., Carrillo, C., Sadeghi, N., et al. (2014). Tai chi, cellular inflammation, and transcriptome dynamics in breast cancer survivors with insomnia: a randomized controlled trial. J. Natl. Cancer Inst. Monogr. 2014, 295–301. doi: 10.1093/jncimonographs/lgu028
Irwin, M. R., and Vitiello, M. V. (2019). Implications of sleep disturbance and inflammation for Alzheimer’s disease dementia. Lancet Neurol. 18, 296–306. doi: 10.1016/S1474-4422(18)30450-2
Jakaria, M., Haque, M. E., Cho, D.-Y., Azam, S., Kim, I.-S., and Choi, D.-K. (2019). Molecular insights into NR4A2 (Nurr1): an emerging target for neuroprotective therapy against Neuroinflammation and neuronal cell death. Mol. Neurobiol. 56, 5799–5814. doi: 10.1007/s12035-019-1487-4
Jin, J., Wu, Y., Li, S., Jin, S., Wang, L., Zhang, J., et al. (2020). Effect of 1 year of qigong exercise on cognitive function among older Chinese adults at risk of cognitive decline: a cluster randomized controlled trial. Front. Psychol. 11:546834. doi: 10.3389/fpsyg.2020.546834
Kaliman, P. (2019). Epigenetics and meditation. Curr. Opin. Psychol. 28, 76–80. doi: 10.1016/j.copsyc.2018.11.010
Kaliman, P., Alvarez-López, M. J., Cosín-Tomás, M., Rosenkranz, M. A., Lutz, A., and Davidson, R. J. (2014). Rapid changes in histone deacetylases and inflammatory gene expression in expert meditators. Psychoneuroendocrinology 40, 96–107. doi: 10.1016/j.psyneuen.2013.11.004
Karch, C. M., and Goate, A. M. (2015). Alzheimer’s disease risk genes and mechanisms of disease pathogenesis. Biol. Psychiatry 77, 43–51. doi: 10.1016/j.biopsych.2014.05.006
Khalsa, D. S. (2015). Stress, meditation, and Alzheimer’s disease prevention: where the evidence stands. J. Alzheimers Dis. 48, 1–12. doi: 10.3233/JAD-142766
Klein, P. J., Schneider, R., and Rhoads, C. J. (2016). Qigong in cancer care: a systematic review and construct analysis of effective qigong therapy. Support Care Cancer 24, 3209–3222. doi: 10.1007/s00520-016-3201-7
Knight, M. J., McCann, B., Kauppinen, R. A., and Coulthard, E. J. (2016). Magnetic resonance imaging to detect early molecular and cellular changes in Alzheimer’s disease. Front. Aging Neurosci. 8:139. doi: 10.3389/fnagi.2016.00139
Kumar, A., Singh, A., and Ekavali, (2015). A review on Alzheimer’s disease pathophysiology and its management: an update. Pharmacol. Rep. 67, 195–203. doi: 10.1016/j.pharep.2014.09.004
Kwok, J. Y. Y., Kwan, J. C. Y., Auyeung, M., Mok, V. C. T., Lau, C. K. Y., Choi, K. C., et al. (2019). Effects of mindfulness yoga vs stretching and resistance training exercises on anxiety and depression for people with Parkinson disease: a randomized clinical trial. JAMA Neurol. 76, 755–763. doi: 10.1001/jamaneurol.2019.0534
Kwon, H. S., and Koh, S.-H. (2020). Neuroinflammation in neurodegenerative disorders: the roles of microglia and astrocytes. Transl. Neurodegener. 9:42. doi: 10.1186/s40035-020-00221-2
Larkey, L., Huberty, J., Pedersen, M., and Weihs, K. (2016). Qigong/tai chi easy for fatigue in breast cancer survivors: rationale and design of a randomized clinical trial. Contemp. Clin. Trials 50, 222–228. doi: 10.1016/j.cct.2016.08.002
Lin, Y., Lu, L., Zhou, M., Liu, H., Ye, P., Zhang, W., et al. (2021). Association of CLU gene polymorphism with Parkinson’s disease in the Chinese Han population. J. Gene Med. 23:e3302. doi: 10.1002/jgm.3302
Liu, R.-M. (2022). Aging, cellular senescence, and Alzheimer’s disease. Int. J. Mol. Sci. 23:1989. doi: 10.3390/ijms23041989
Liu, H., Zheng, Y., and Huang, S. (2022). Research progress on the treatment of neurodegenerative diseases with acupuncture for clearing the governor and regulating the mind. Chin. J. Gerontol. 42, 4119–4123. doi: 10.3969/j.issn.1005-9202.2022.16.070
Livingston, G., Huntley, J., Sommerlad, A., Ames, D., Ballard, C., Banerjee, S., et al. (2020). Dementia prevention, intervention, and care: 2020 report of the lancet commission. Lancet 396, 413–446. doi: 10.1016/S0140-6736(20)30367-6
Lou, J. S. (2017). Mindfulness, depression and quality of life in amyotrophic lateral sclerosis. Eur. J. Neurol. 24, 881–882. doi: 10.1111/ene.13309
Madore, C., Yin, Z., Leibowitz, J., and Butovsky, O. (2020). Microglia, lifestyle stress, and neurodegeneration. Immunity 52, 222–240. doi: 10.1016/j.immuni.2019.12.003
Martínez-González, K., Islas-Hernández, A., Martínez-Ezquerro, J. D., Bermúdez-Rattoni, F., and Garcia-delaTorre, P. (2020). Telomere length and oxidative stress variations in a murine model of Alzheimer’s disease progression. Eur. J. Neurosci. 52, 4863–4874. doi: 10.1111/ejn.14877
Maturana-Candelas, A., Gómez, C., Poza, J., Rodríguez-González, V., Pablo, V. G., Lopes, A. M., et al. (2021). Influence of PICALM and CLU risk variants on beta EEG activity in Alzheimer’s disease patients. Sci. Rep. 11:20465. doi: 10.1038/s41598-021-99589-y
Mendioroz, M., Puebla-Guedea, M., Montero-Marín, J., Urdánoz-Casado, A., Blanco-Luquin, I., Roldán, M., et al. (2020). Telomere length correlates with subtelomeric DNA methylation in long-term mindfulness practitioners. Sci. Rep. 10:4564. doi: 10.1038/s41598-020-61241-6
Milligan Armstrong, A., Porter, T., Quek, H., White, A., Haynes, J., Jackaman, C., et al. (2021). Chronic stress and Alzheimer’s disease: the interplay between the hypothalamic-pituitary-adrenal axis, genetics and microglia. Biol. Rev. Camb. Philos. Soc. 96, 2209–2228. doi: 10.1111/brv.12750
Moon, M., Jung, E. S., Jeon, S. G., Cha, M.-Y., Jang, Y., Kim, W., et al. (2019). Nurr1 (NR4A2) regulates Alzheimer’s disease-related pathogenesis and cognitive function in the 5XFAD mouse model. Aging Cell 18:e12866. doi: 10.1111/acel.12866
Moraes, L. J., Miranda, M. B., Loures, L. F., Mainieri, A. G., and Mármora, C. H. C. (2018). A systematic review of psychoneuroimmunology-based interventions. Psychol. Health Med. 23, 635–652. doi: 10.1080/13548506.2017.1417607
Newberg, A. B., Serruya, M., Wintering, N., Moss, A. S., Reibel, D., and Monti, D. A. (2014). Meditation and neurodegenerative diseases. Ann. N. Y. Acad. Sci. 1307, 112–123. doi: 10.1111/nyas.12187
Ng, T. K. S., Feng, L., Fam, J., Rawtaer, I., Kumar, A. P., Rane, G., et al. (2021). Mindfulness awareness practice (MAP) to prevent dementia in older adults with mild cognitive impairment: protocol of a randomized controlled trial and implementation outcomes. Int. J. Environ. Res. Public Health 18:10205. doi: 10.3390/ijerph181910205
Nissanka, N., and Moraes, C. T. (2018). Mitochondrial DNA damage and reactive oxygen species in neurodegenerative disease. FEBS Lett. 592, 728–742. doi: 10.1002/1873-3468.12956
Notarianni, E. (2013). Hypercortisolemia and glucocorticoid receptor-signaling insufficiency in Alzheimer’s disease initiation and development. Curr. Alzheimer Res. 10, 714–731. doi: 10.2174/15672050113109990137
Pandya, V. A., and Patani, R. (2020). Decoding the relationship between ageing and amyotrophic lateral sclerosis: a cellular perspective. Brain 143, 1057–1072. doi: 10.1093/brain/awz360
Perry, V. H., Nicoll, J. A. R., and Holmes, C. (2010). Microglia in neurodegenerative disease. Nat. Rev. Neurol. 6, 193–201. doi: 10.1038/nrneurol.2010.17
Pringsheim, T., Jette, N., Frolkis, A., and Steeves, T. D. L. (2014). The prevalence of Parkinson’s disease: a systematic review and meta-analysis. Mov. Disord. 29, 1583–1590. doi: 10.1002/mds.25945
Puhlmann, L. M. C., Vrtička, P., Linz, R., Stalder, T., Kirschbaum, C., Engert, V., et al. (2021). Contemplative mental training reduces hair glucocorticoid levels in a randomized clinical trial. Psychosom. Med. 83, 894–905. doi: 10.1097/PSY.0000000000000970
Qiu, C., and Fratiglioni, L. (2018). Aging without dementia is achievable: current evidence from epidemiological research. J. Alzheimers Dis. 62, 933–942. doi: 10.3233/JAD-171037
Radi, E., Formichi, P., Battisti, C., and Federico, A. (2014). Apoptosis and oxidative stress in neurodegenerative diseases. J. Alzheimers Dis. 42, S125–S152. doi: 10.3233/JAD-132738
Rathore, M., and Abraham, J. (2018). Implication of asana, pranayama and meditation on telomere stability. Int. J. Yoga 11, 186–193. doi: 10.4103/ijoy.IJOY_51_17
Ratti, A., Gumina, V., Lenzi, P., Bossolasco, P., Fulceri, F., Volpe, C., et al. (2020). Chronic stress induces formation of stress granules and pathological TDP-43 aggregates in human ALS fibroblasts and iPSC-motoneurons. Neurobiol. Dis. 145:105051. doi: 10.1016/j.nbd.2020.105051
Rawtaer, I., Mahendran, R., Yu, J., Fam, J., Feng, L., and Kua, E. H. (2015). Psychosocial interventions with art, music, tai chi and mindfulness for subsyndromal depression and anxiety in older adults: a naturalistic study in Singapore. Asia Pac. Psychiatry 7, 240–250. doi: 10.1111/appy.12201
Rokad, D., Ghaisas, S., Harischandra, D. S., Jin, H., Anantharam, V., Kanthasamy, A., et al. (2017). Role of Neurotoxicants and traumatic brain injury in α-Synuclein protein Misfolding and aggregation. Brain Res. Bull. 133, 60–70. doi: 10.1016/j.brainresbull.2016.12.003
Schutte, N. S., and Malouff, J. M. (2014). A meta-analytic review of the effects of mindfulness meditation on telomerase activity. Psychoneuroendocrinology 42, 45–48. doi: 10.1016/j.psyneuen.2013.12.017
Shay, J. W. (2018). Telomeres and aging. Curr. Opin. Cell Biol. 52, 1–7. doi: 10.1016/j.ceb.2017.12.001
Shih, R.-H., Wang, C.-Y., and Yang, C.-M. (2015). NF-kappaB signaling pathways in neurological inflammation: a Mini review. Front. Mol. Neurosci. 8:77. doi: 10.3389/fnmol.2015.00077
Smith, E. M., Pendlebury, D. F., and Nandakumar, J. (2020). Structural biology of telomeres and telomerase. Cell. Mol. Life Sci. 77, 61–79. doi: 10.1007/s00018-019-03369-x
Song, R., Grabowska, W., Park, M., Osypiuk, K., Vergara-Diaz, G. P., Bonato, P., et al. (2017). The impact of tai chi and Qigong mind-body exercises on motor and non-motor function and quality of life in Parkinson’s disease: a systematic review and meta-analysis. Parkinsonism Relat. Disord. 41, 3–13. doi: 10.1016/j.parkreldis.2017.05.019
Stefanatos, R., and Sanz, A. (2018). The role of mitochondrial ROS in the aging brain. FEBS Lett. 592, 743–758. doi: 10.1002/1873-3468.12902
Stoffel, M., Aguilar-Raab, C., Rahn, S., Steinhilber, B., Witt, S. H., Alexander, N., et al. (2019). Effects of mindfulness-based stress prevention on serotonin transporter gene methylation. Psychother. Psychosom. 88, 317–319. doi: 10.1159/000501646
Svetlov, A. S., Nelson, M. M., Antonenko, P. D., McNamara, J. P. H., and Bussing, R. (2019). Commercial mindfulness aid does not aid short-term stress reduction compared to unassisted relaxation. Heliyon 5:e01351. doi: 10.1016/j.heliyon.2019.e01351
Tang, Y.-Y., Ma, Y., Fan, Y., Feng, H., Wang, J., Feng, S., et al. (2009). Central and autonomic nervous system interaction is altered by short-term meditation. Proc. Natl. Acad. Sci. U. S. A. 106, 8865–8870. doi: 10.1073/pnas.0904031106
Tilstra, J. S., Robinson, A. R., Wang, J., Gregg, S. Q., Clauson, C. L., Reay, D. P., et al. (2012). NF-κB inhibition delays DNA damage-induced senescence and aging in mice. J. Clin. Invest. 122, 2601–2612. doi: 10.1172/JCI45785
Tiwari, A., Chan, C. L. W., Ho, R. T. H., Tsao, G. S. W., Deng, W., Hong, A. W. L., et al. (2014). Effect of a qigong intervention program on telomerase activity and psychological stress in abused Chinese women: a randomized, wait-list controlled trial. BMC Complement. Altern. Med. 14:300. doi: 10.1186/1472-6882-14-300
Tönnies, E., and Trushina, E. (2017). Oxidative stress, synaptic dysfunction, and Alzheimer’s disease. J. Alzheimers Dis. 57, 1105–1121. doi: 10.3233/JAD-161088
van der Heide, A., Meinders, M. J., Speckens, A. E. M., Peerbolte, T. F., Bloem, B. R., and Helmich, R. C. (2021). Stress and mindfulness in Parkinson’s disease: clinical effects and potential underlying mechanisms. Mov. Disord. 36, 64–70. doi: 10.1002/mds.28345
van Es, M. A., Hardiman, O., Chio, A., Al-Chalabi, A., Pasterkamp, R. J., Veldink, J. H., et al. (2017). Amyotrophic lateral sclerosis. Lancet 390, 2084–2098. doi: 10.1016/S0140-6736(17)31287-4
Vyas, S., and Maatouk, L. (2013). Contribution of glucocorticoids and glucocorticoid receptors to the regulation of neurodegenerative processes. CNS Neurol. Disord. Drug Targets 12, 1175–1193. doi: 10.2174/187152731131200125
Wetherell, J. L., Hershey, T., Hickman, S., Tate, S. R., Dixon, D., Bower, E. S., et al. (2017). Mindfulness-based stress reduction for older adults with stress disorders and neurocognitive difficulties: a randomized controlled trial. J. Clin. Psychiatry 78, e734–e743. doi: 10.4088/JCP.16m10947
Wolkowitz, O. M., Mellon, S. H., Lindqvist, D., Epel, E. S., Blackburn, E. H., Lin, J., et al. (2015). PBMC telomerase activity, but not leukocyte telomere length, correlates with hippocampal volume in major depression. Psychiatry Res. 232, 58–64. doi: 10.1016/j.pscychresns.2015.01.007
Wu, H., Dunnett, S., Ho, Y.-S., and Chang, R. C.-C. (2019). The role of sleep deprivation and circadian rhythm disruption as risk factors of Alzheimer’s disease. Front. Neuroendocrinol. 54:100764. doi: 10.1016/j.yfrne.2019.100764
Wyss-Coray, T. (2016). Ageing, neurodegeneration and brain rejuvenation. Nature 539, 180–186. doi: 10.1038/nature20411
Yeung, A. W. K., Tzvetkov, N. T., Georgieva, M. G., Ognyanov, I. V., Kordos, K., Jóźwik, A., et al. (2021). Reactive oxygen species and their impact in neurodegenerative diseases: literature landscape analysis. Antioxid. Redox Signal. 34, 402–420. doi: 10.1089/ars.2019.7952
Yin, Z., Raj, D., Saiepour, N., Van Dam, D., Brouwer, N., Holtman, I. R., et al. (2017). Immune hyperreactivity of Aβ plaque-associated microglia in Alzheimer’s disease. Neurobiol. Aging 55, 115–122. doi: 10.1016/j.neurobiolaging.2017.03.021
Yingwei, W., Xiudong, L., and Xuejing, B. (2019). Effects of Baduanjin health qigong on sleep quality and memory function of the elderly in the community. Chin. J. Gerontol. 39, 3435–3437. doi: 10.3969/j.issn
Yoshiyama, Y., Lee, V. M. Y., and Trojanowski, J. Q. (2013). Therapeutic strategies for tau mediated neurodegeneration. J. Neurol. Neurosurg. Psychiatry 84, 784–795. doi: 10.1136/jnnp-2012-303144
Zhang, P., Kishimoto, Y., Grammatikakis, I., Gottimukkala, K., Cutler, R. G., Zhang, S., et al. (2019). Senolytic therapy alleviates Aβ-associated oligodendrocyte progenitor cell senescence and cognitive deficits in an Alzheimer’s disease model. Nat. Neurosci. 22, 719–728. doi: 10.1038/s41593-019-0372-9
Keywords: mindfulness therapy, neurodegenerative disease, Parkinson’s disease, Alzheimer’s disease, amyotrophic lateral sclerosis, molecular mechanism
Citation: Wu C and Feng Y (2023) Exploring the potential of mindfulness-based therapy in the prevention and treatment of neurodegenerative diseases based on molecular mechanism studies. Front. Neurosci. 17:1097067. doi: 10.3389/fnins.2023.1097067
Edited by:
Min Fang, Shanghai University of Traditional Chinese Medicine, ChinaReviewed by:
Stanislaw Szlufik, Warszawski Uniwersytet Medyczny, PolandDhiraj, National Eye Institute (NIH), United States
Copyright © 2023 Wu and Feng. This is an open-access article distributed under the terms of the Creative Commons Attribution License (CC BY). The use, distribution or reproduction in other forums is permitted, provided the original author(s) and the copyright owner(s) are credited and that the original publication in this journal is cited, in accordance with accepted academic practice. No use, distribution or reproduction is permitted which does not comply with these terms.
*Correspondence: Yue Feng, ZmVuZ3l1ZTcxNEAxNjMuY29t