- 1College of First Clinical Medicine, Shandong University of Traditional Chinese Medicine, Jinan, China
- 2Wangjing Hospital, China Academy of Chinese Medical Sciences, Beijing, China
- 3Department of Geriatrics, Xiyuan Hospital, China Academy of Chinese Medical Sciences, Beijing, China
- 4Graduate College, Beijing University of Chinese Medicine, Beijing, China
Objective: To analyze the effects and mechanisms of berberine in the treatment of aging-related cognitive dysfunction based on network pharmacology methods, molecular docking techniques, and animal experiments.
Methods: A mouse model of cognitive dysfunction was constructed by subcutaneous injection of D-galactose (D-gal) for 10 weeks, and the neuroprotective effects of berberine on aging-related cognitive dysfunction mice were evaluated by the Morris water maze (MWM) and immunofluorescence staining. The targets of berberine were obtained by SwissTargetPrediction, GeneCards, and PharmMapper. Putative targets of cognitive dysfunction were obtained by GeneCards, TTD, and DrugBank database. The STRING database and Cytoscape software were applied for protein-protein interaction (PPI) analysis and further screening of core targets. The DAVID database was used for Kyoto Encyclopedia of Genes and Genomes (KEGG) and gene ontology (GO) enrichment analysis to clarify the biological processes and pathways involved in the intersection targets, and AutoDockTools was adopted for molecular docking verification of core targets. Finally, the core genes were validated using real-time quantitative PCR.
Results: The MWM results showed that treatment with berberine significantly improved spatial learning and memory in mice with cognitive decline induced by D-gal. Immunofluorescence staining indicated that berberine modified the levels of aging-related markers in the brain. A total of 386 berberine putative targets associated with cognitive dysfunction were identified based on the public database. The core targets of berberine for improving cognitive function, include Mapk1, Src, Ctnnb1, Akt1, Pik3ca, Tp53, Jun, and Hsp90aa1. GO enrichment and KEGG pathway enrichment analyses indicated that the mechanism of berberine in the treatment of aging-related cognitive dysfunction is attributed to pathways such as PI3K-AKT and MAPK pathways. In vivo experiments further confirmed that Akt1, Ctnnb1, Tp53, and Jun were involved in the neuroprotective actions of berberine.
Conclusion: This study reveals the multi-target and multi-pathway effects of berberine on regulating aging-related cognitive dysfunction, which provides preclinical evidence and may promote new drug development in mitigating cognitive dysfunction.
1. Introduction
Cognitive dysfunction, also known as cognitive impairment (CI), is characterized by memory loss, learning disabilities, and a decreased ability to concentrate on a particular task. The spectrum of CI can range from mild cognitive deficits that are not clinically detectable to dementia (Luchsinger, 2012). Dementia is usually diagnosed when acquired cognitive impairment becomes severe enough to impair social and/or occupational functioning (Hugo and Ganguli, 2014). The CI leads to a reduced quality of life in older adults and increases the risk of dementia and mortality (Park et al., 2013; Hu et al., 2020). The population aged 80 years and older is the fastest-growing segment of the global population, and prevention of age-related cognitive dysfunction is one of the greatest challenges facing healthcare today.
Mild cognitive impairment (MCI) is considered to be a state between normal cognitive aging and early dementia (Petersen, 2004). Notably, approximately 16% of subjects diagnosed with MCI returned to normal or near-normal cognition after approximately 1 year (Koepsell and Monsell, 2012). In a meta-analysis evaluating the rate of progression from MCI to dementia in 41 cohort studies stratified by population studies and clinical trials, more than half of the participants did not progress to dementia within 10 years, and the annual conversion rate for dementia and Alzheimer’s disease (AD) was approximately 7% (McGirr et al., 2022). In addition to the modification of some modifiable risk factors, treatment with traditional Chinese medicine (TCM) may play a role in the recovery of cognitive function.
Berberine (BBR) is an isoquinoline alkaloid, mainly found in the rhizome of Coptis sp., and the cortex of Berberis sp., and Phellodendron sp. Numerous studies have demonstrated multiple pharmacological effects of BBR, including anti-inflammatory (Jeong et al., 2009), anti-proliferative (Choi et al., 2008), hypoglycemic (Zhang et al., 2010; Pirillo and Catapano, 2015), hypocholesterolemic (Pirillo and Catapano, 2015), and anti-hypertensive effects (Bova et al., 1992). Previous studies have shown that BBR has antioxidant properties as well as protective effects against neurodegenerative diseases (Cheng et al., 2022), improving the cognitive decline associated with diabetes in db/db mice (Li et al., 2018). Recent studies have highlighted the anti-aging effects of BBR (Xu et al., 2017; Dang et al., 2020), which is considered to be one of the greatest risk factors for neurodegenerative diseases. Taken together, it will be of interest to explore the protective effects of BBR in neurodegenerative diseases.
D-galactose (D-gal) is an aldohexose naturally existing in the body, including in the brain (Nagy and Pohl, 2015). D-gal predisposes to aging and long-term systemic administration has been used to artificially produce brain aging phenotypes in animal models, which causes the onset of behavioral and cognitive deficits (Shwe et al., 2018). Several studies have illustrated that D-gal-induced brain aging not only contributes to memory deficits, neuronal degeneration, and apoptosis, but also increased oxidative stress, and mitochondrial dysfunction, which has many similarities to human brain aging (Banji et al., 2014). All these deficits eventually lead to cognitive decline. The amelioration of cognitive dysfunction by BBR has been observed in animal models of AD (Durairajan et al., 2012; Panahi et al., 2013). BBR has previously been observed to reduce the levels of endogenous oxidants and DNA damage response (Zhao et al., 2013), with potential effects of anti-aging (Dang et al., 2020; Li et al., 2022). However, the effects of BBR on cognitive impairment during aging have not been sufficiently investigated. In the current study, we investigated whether BBR reverses D-gal-induced aging and improves cognitive function in mice. The flow chart of this study is shown in Figure 1.
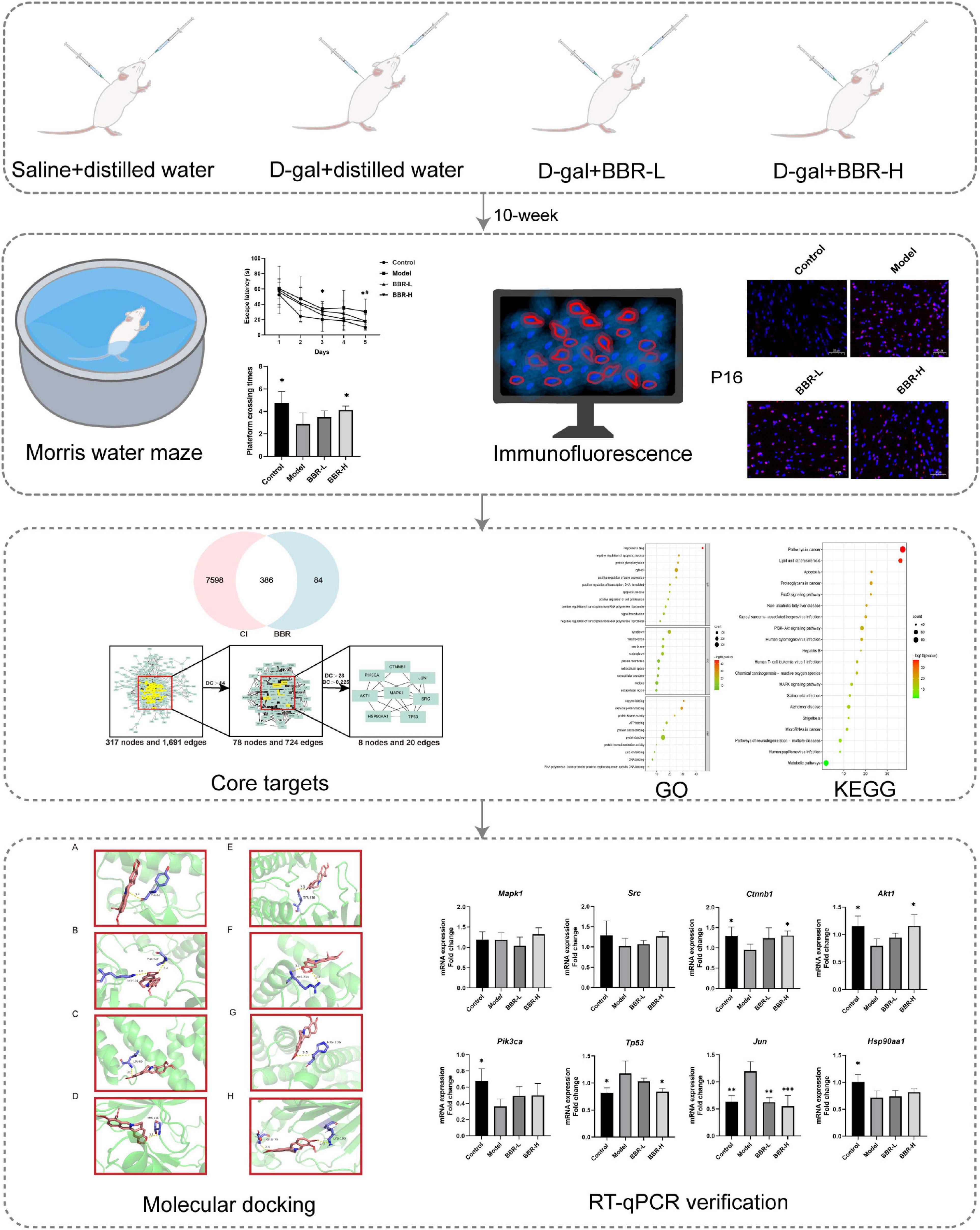
Figure 1. The flowchart of this study. Morris water maze: *p < 0.05 Control vs. Model; #p < 0.05 BBR-L/H vs. Model; RT-qPCR verification: *p < 0.05, **p < 0.01, and ***p < 0.001 vs. Model.
2. Materials and methods
2.1. Animals and general procedures
Eight-week-old male ICR mice (n = 32) were housed in eight cages. All animals were allowed free access to food and water and maintained at constant temperature (22 ± 3°C) and humidity (50 ± 10%) during a 12-h light/dark cycle. The project was authorized by the Ethics Committee of Xiyuan Hospital, China Academy of Chinese Medical Sciences (No. 2021XLC035-3).
After 1 week of adaptive rearing, D-gal-induced cognitive dysfunction was performed by subcutaneous injection of D-gal for 10 weeks according to a previously described procedure (Sun et al., 2018; Daroi et al., 2022). Similarly, BBR was administered orally as previously described (Ma et al., 2021; Wang et al., 2022) to observe the protective benefits against aging-related cognitive impairment. Mice were randomly divided into the following four groups (n = 8 per group). (1) Control: subcutaneous injections of saline and oral distilled water, (2) Model: subcutaneous injections of D-gal (150 mg/kg/d) and oral distilled water, (3) BBR-L: subcutaneous injections of D-gal (150 mg/kg/d) and BBR orally (50 mg/kg), (4) BBR-H: subcutaneous injections of D-gal (150 mg/kg/d) and BBR orally (100 mg/kg).
2.2. Drug
Berberine (BBR), purity ≧98% (LDSW220209-1), provided by Shaanxi Lande Biotechnology Co., Ltd. D- (+) galactose (ST1218-50 g) purchased from Shanghai Beyotime Biotechnology Co., Ltd. Pentobarbital sodium salt (P3761) was purchased from Sigma, USA.
2.3. Morris water maze test
The Morris water maze (MWM) experiment was conducted for 6 days during the 10th week of dosing. The water maze apparatus consisted of a circular pool (120 cm in diameter and 50 cm in height), an automatic camera, and a computerized analysis system. The pool was divided equally into four quadrants, and a 12-cm diameter platform was placed in the first quadrant and filled with an appropriate amount of water so that the top of the platform was 1 cm below the water’s surface. An appropriate amount of ink was poured into the water and mixed, and the water temperature was maintained at (23 ± 1)°C during the experiment. The MWM experiment consisted of two parts: navigation experiments and spatial probe experiments. On the day before the start of the experiment, each mouse was placed in the pool (without a platform) and swam freely for 90 s to adapt to the environment. The experiment started with 5 days of positioning navigation: each mouse was trained four times per day at 20-min intervals, with clockwise changes of entry points during the four training sessions. The mice were placed in the water facing the wall of the pool and the time between entering the water and finding the escape platform was recorded using a video tracking system, i.e., escape latency. If the platform could not be found for 90 s, the mice were manually guided to the platform for 10 s. On day 6, the spatial exploration experiment was started: the escape platform for the positioning navigation experiment was removed, and the mice were placed in the water facing the wall of the pool (the entry point was the midpoint of the third quadrant), and the number of times they crossed the original platform area within 90 s was recorded, i.e., the number of times they crossed the platform.
2.4. Tissue preparation
Brain tissue was taken after MWM. Mice were deeply anesthetized with an intraperitoneal injection of sodium pentobarbital, and the brain was quickly removed from the skull. Three animals in each group were randomly selected. Each brain was divided into left and right halves through a mid-sagittal incision, and the left one was fixed with 4% paraformaldehyde for performing immunofluorescence staining. The remaining cortex was snap-freezing in liquid nitrogen and then transferred to −80°C for further analyses.
2.5. Immunofluorescence staining
Paraffin sections were dewaxed in xylene and rehydrated in ethanol and distilled water. After antigen retrieval with EDTA, sections were blocked in 3% BSA for 30 min at room temperature. The blocked solution was gently shaken off and the primary antibody, namely, Anti -CDKN2A/p16INK4a Rabbit pAb) (GB111143, Servicebio, Wuhan, China) was added to the sections and incubated overnight at 4°C. The next day, a secondary antibody (Cy3 conjugated Goat Anti-Rabbit IgG, GB21303, Servicebio, Wuhan, China) was added and incubated at room temperature for 1 h. After DAPI (G1012, Servicebio, Wuhan, China) stained the nuclei, the sections were dried slightly and then sealed with anti-fluorescence quenched sealing tablets. Images of the cortex were captured at ×20 magnification with a fluorescence microscope (Nikon DS-U3, Nikon).
2.6. Key targets screening for BBR to improve cognitive dysfunction
2.6.1. Identification of candidate targets for cognitive dysfunction
Three public disease-gene-related databases were used to retrieve important targets for Cognitive Dysfunction, including GeneCards1 (Safran et al., 2021), DrugBank2 (Wishart et al., 2018), and TTD (Zhou et al., 2022).3 The search term “cognitive dysfunction” or “cognitive impairment” is used to screen potential disease targets for cognitive dysfunction, and the disease targets obtained from the three databases were combined and de-duplicated. UniProt4 was used to standardize the gene names.
2.6.2. Identification of candidate targets for BBR
To identify the corresponding targets of BBR, target prediction of BBR was performed through three public databases, including GeneCards (see text footnote 1) (Safran et al., 2021), SwissTargetPrediction5 (Daina et al., 2019), and PharmMapper6 (Wang et al., 2017), while all targets were restricted to Homo sapiens. The genes obtained from these three databases were aggregated and duplicates were removed. The UniProt (see text footnote 4) (UniProt Consortium, 2020) database was used to standardize the gene names.
2.6.3. Construction of protein-protein interaction networks
Candidate target genes of BBR were intersected with CI-associated target genes to obtain intersecting genes and draw Venn diagrams, and these genes corresponding to both drugs and diseases were considered potential therapeutic targets. Online STRING 11.57 (Szklarczyk et al., 2021) was used to construct protein-protein interaction (PPI) networks. All networks were built with Cytoscape v3.8.2, software for analyzing and visualizing biological networks. The core networks were analyzed based on degree, betweenness centrality, close-ness centrality, and average shortest path length. In the network, the nodes represent the targets, while the edges represent the connections between them.
2.6.4. Enrichment analysis of GO and KEGG pathways
DAVID Bioinformatics Resource 20218 (Sherman et al., 2022) was used to analyze gene ontology (GO) enrichment and Kyoto Encyclopedia of Genes and Genomes (KEGG) pathway enrichment for key targets involved in biological process, cellular component, molecular function, and signaling pathway. The bioinformatics online analysis platform9 was used to visualize the enrichment analysis results.
2.7. Molecular docking
The molecular structures of BBR were captured in the Traditional Chinese Medicine System Pharmacology database and Analysis Platform (TCMSP)10 (Ru et al., 2014) and the molecular structures (crystal structures) of the target proteins were obtained from the PDB database (Protein Data Bank).11 The molecular structures of BBR were captured in TCMSP (see text footnote 10) and the molecular structures (crystal structures) of the target proteins were obtained from the PDB database (Protein Data Bank, see text footnote 11). Pre-docking molecular processing was performed using AutoDockTools 1.5.7 software, and the pdbqt files were acquired. Molecular docking was performed using AutoDock Vina 1.1.212 software, and the affinity score was calculated, with a smaller value indicating a stronger bonding force. PyMOL2.4.0 software13 was used to visualize the molecular docking results and calculate the Root Mean Square Deviation (RMSD) to verify the reliability, and the docking results were considered reliable with RMSD < 2A.
2.8. RT-qPCR
Total RNA was extracted from the mice’s cerebral cortex using an RNA extraction kit (Servicebio, Wuhan, China, G3640-50T). Reverse-transcription of RNA to cDNA was performed using ReverTra Ace qPCR RT Master Mix (TOYOBO, FSQ-201) according to the manufacturer’s instructions. Real-time qPCR was conducted on an ABI 7500 system using Applied Biosystems SYBR-Green PCR Master mix (Thermo Fisher Scientific, Inc., USA). The total reaction volume was 10 μL and primer sequences are shown in Table 1. The following amplification steps were used: initial denaturation at 95°C for 10 min, followed by 40 denaturation cycles at 95°C for 15 s, and elongation at 60°C for 60 s. Gapdh was used as a housekeeping gene. Relative mRNA expression was calculated using the 2–ΔΔCt method. Three replicates were performed for each group.
3. Statistics
Data were presented as mean ± SEM. MWM task data were analyzed using repeated-measures analysis of variance (ANOVA). The results of Mauchly’s test of sphericity were first used to determine whether there was a correlation between the repeated measures data and if there was a correlation (p ≤ 0.05), a multivariate ANOVA was performed, or the Greenhouse–Geisser correction was applied. Pairwise comparisons between different subgroups at the same time point were performed using multivariate ANOVA. For data that did not involve repeated measures, two independent samples t-test or Mann–Whitney U-test was used for analysis. p < 0.05 was considered statistically significant. All statistical analyses were performed with SPSS software version 26.0. GraphPad Prism 8 was used for generating data plots.
4. Results
4.1. Effects of BBR on cognitive dysfunction
The MWM test is the most widely used laboratory behavioral test to assess cognitive deficits in rodents and the experimental protocol of MWM is as described previously (Vorhees and Williams, 2006). In the navigation test, as shown in Figure 2A, the escape latency of all mice gradually decreased with the increasing number of training sessions, indicating that their ability to locate the platform was enhanced. Compared with the control group, the escape latency of mice in the model group increased, with significant differences on days 3 and 5 (p < 0.05). The escape latency of mice in the BBR-L and BBR-H groups was diminished compared to the model group and exhibited a significant difference on day 5 (p < 0.05). In the exploratory experiment on day 6 (Figure 2B), the times of crossing the original platform were significantly reduced in the model group compared to the control group (p < 0.05). Both the BBR-L and BBR-H groups experienced an elevation in the number of mice crossing the original platform compared to the model group, with the BBR-H group showing a significant difference (p < 0.05). Collectively, these data suggest that we successfully induced aging-related cognitive dysfunction in mice, and more critically, we found that berberine ameliorated this cognitive impairment, although more studies are necessary to clarify the mechanisms involved.
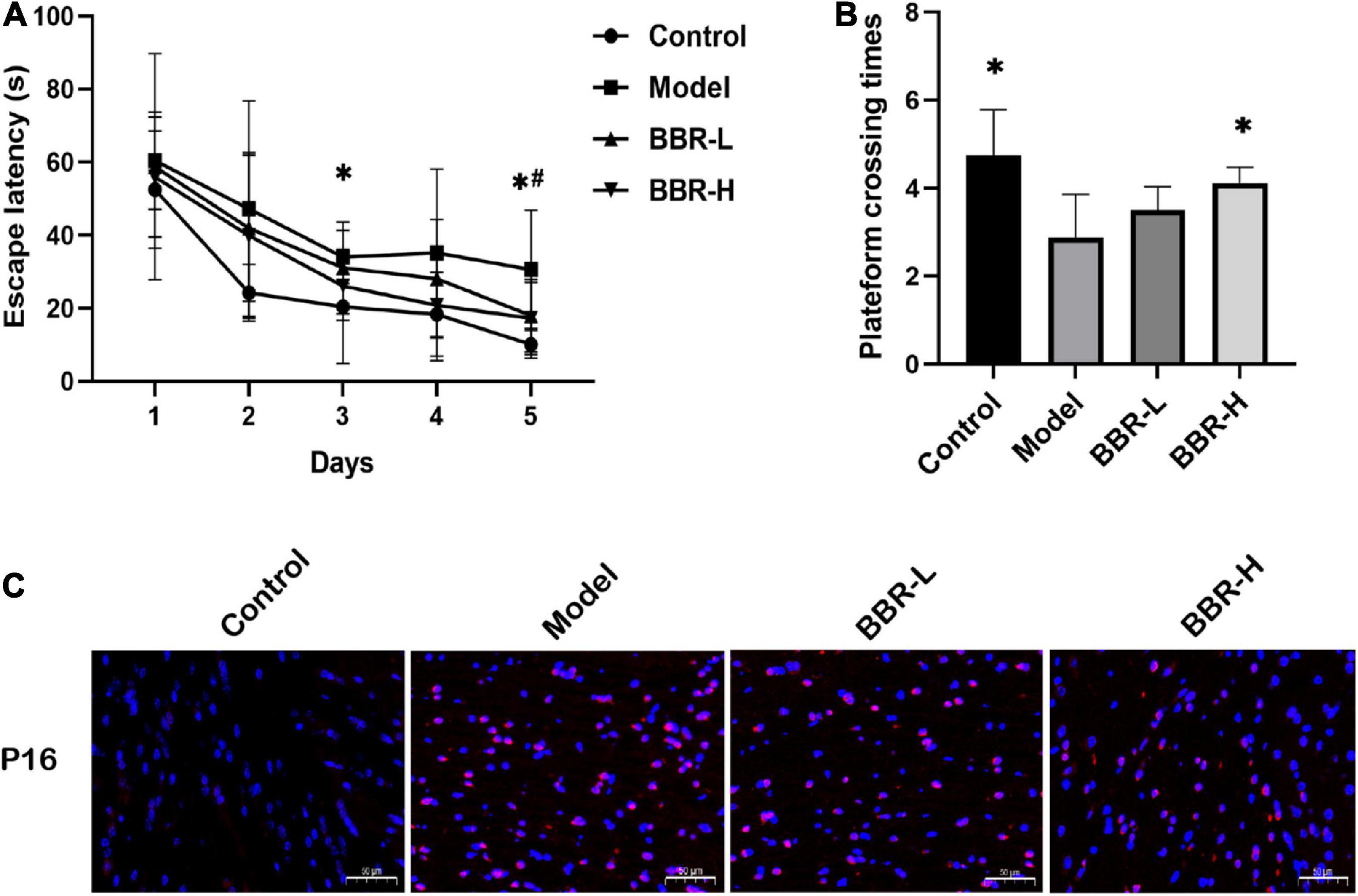
Figure 2. The results of Morris water maze (MWM) and immunofluorescence. (A) The escape latency. (B) The times across the platform (All data are mean ± SEM with n = 8, *p < 0.05 Control vs. Model; #p < 0.05 BBR-L/H vs. Model). (C) Immunofluorescence staining of P16 (×20, red = p16, blue = DAPI, scale bar 50 μm).
4.2. BBR reduces the expression of P16 in brain tissue of cognitive dysfunctions mice
Aging is a common risk factor attributed to various neurodegenerative diseases, and aging of the brain eventually leads to CI. P16, a tumor suppressor gene can be considered the best biomarker of cellular senescence (Kim and Sharpless, 2006). Immunofluorescence staining of brain tissue for P16 at the end of administration to determine that D-gal caused aging-related cognitive dysfunction in mice. As shown in Figure 2C, P16 expression was significantly increased in the D-gal group compared with the control group, indicating that D-gal induced brain aging in mice. However, the administration of BBR altered the expression of senescence marker. Compared with the D-gal group, P16 expression was reduced in both the low- and high-dose groups of BBR. In conclusion, the above results suggest that BBR has anti-aging potential in the brain.
4.3. Results of network pharmacology study
4.3.1. Target network analysis
To further elucidate the mechanisms and targets of BBR for improving cognitive function, we conducted a network pharmacology study. We applied three public disease gene-related databases, including GeneCards, DrugBank, and TTD, to retrieve major targets for CI/cognitive dysfunction. Ultimately, a total of 7,984 recognized CI-associated genes were explored (Supplementary Table 1). To identify the corresponding targets for BBR, we aggregated all predicted targets from GeneCards, SwissTargetPrediction, and PharmMapper. Finally, 470 targets for BBR were obtained (Supplementary Table 2).
4.3.2. PPI network construction and core gene screening for BBR and CI
By intersecting candidate target genes of BBR with CI-related target genes, we identified genes intersecting with drugs and diseases as potential targets. The Venn diagram (Figure 3A) showed that there were 386 potential targets associated with both BBR and CI. Intersecting genes of BBR and CI were imported into the STRING database to construct the PPI network. We set the highest confidence level of 0.900 for the minimum required interaction score and hid the unlinked nodes in the network (Figure 3B). All network visualizations were implemented by Cytoscape. As shown in Figure 3C, the PPI network contains 317 nodes and 1,961 edges. Based on Degree and betweenness centrality, we selected the top 8 genes as hub genes (Figures 3C–E). These hub genes may represent the key molecular targets in the anti-CI action of BBR. Accordingly, eight core target genes were obtained (Figure 3E), including Mapk1, Src, Ctnnb1, Akt1, Pik3ca, Tp53, Jun, and Hsp90aa1.
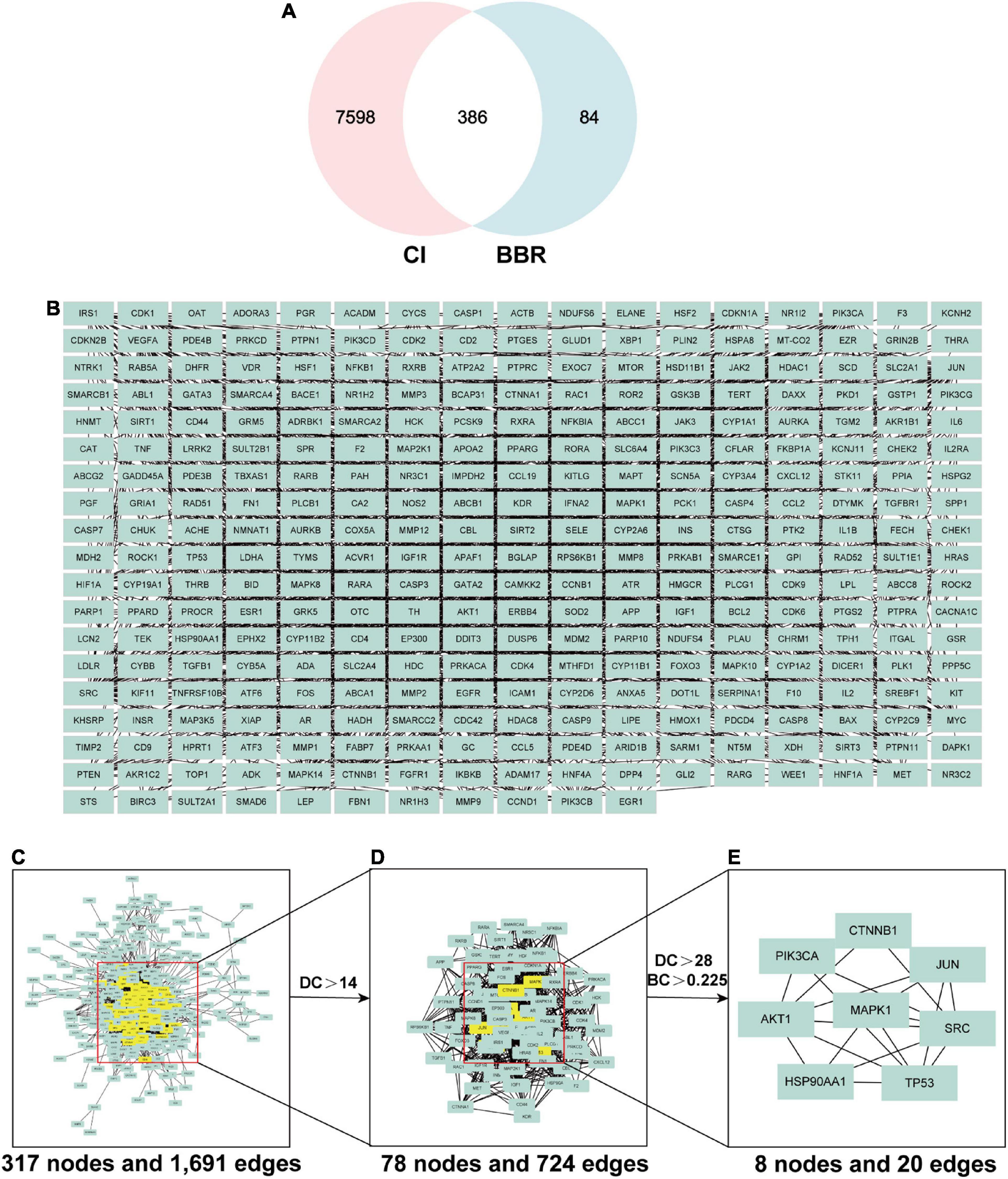
Figure 3. The screening process of core genes between cognitive impairment (CI) and berberine (BBR). (A) Venn diagram of CI and BBR overlapping genes. (B) Intersecting genes after screening. (C) Protein-protein interaction (PPI) network of intersecting genes. (D) PPI network of significant targets extracted from Panel (C). (E) PPI network of candidate BBR targets for CI extracted from Panel (D).
4.3.3. Enrichment analysis of BBR-CI target networks
Gene ontology (GO) analysis is commonly used to comprehensively characterize the contribution of genes in an organism, including biological processes, cellular components, and molecular functions. BBR therapeutic targets associated with CI were included in the analysis, and the results are shown in Figure 4A. KEGG is a database resource for understanding the high-level function and utility of biological systems from genomic and molecular level information. According to the KEGG pathway enrichment analysis (Figure 4B), the PI3K-Akt signaling pathway and MAPK signaling pathway were significantly enriched.
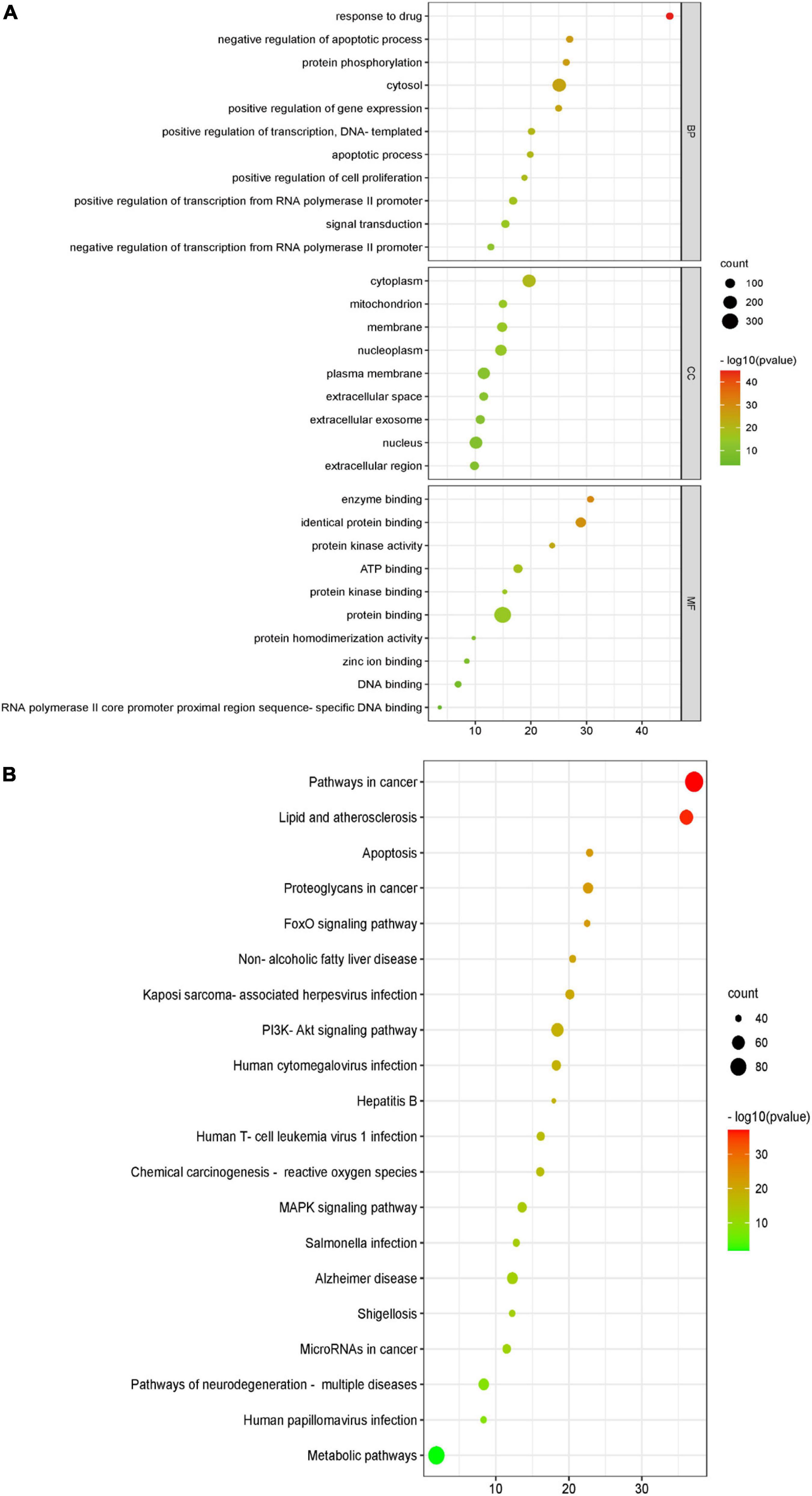
Figure 4. Gene ontology (GO) function and Kyoto Encyclopedia of Genes and Genomes (KEGG) pathway enrichment analyses of berberine (BBR) in the treatment of cognitive impairment (CI). (A) The GO function analysis, includes biological process (BP), cellular component (CC), and molecular function (MF). (B) Top 20 pathways in the BBR anti-CI bubble diagram of KEGG pathway enrichment.
4.4. BBR-core targets molecular docking
Molecular docking of BBR and its corresponding core targets are achieved by AutoDock Vina software. It is generally believed that the smaller the affinity, the more stable the conformation of ligand-receptor binding and the higher the possibility of interaction. The molecular docking results demonstrated that BBR had a small affinity for all eight core targets (Table 2), indicating a favorable binding activity. The docking results are presented in Figure 5.
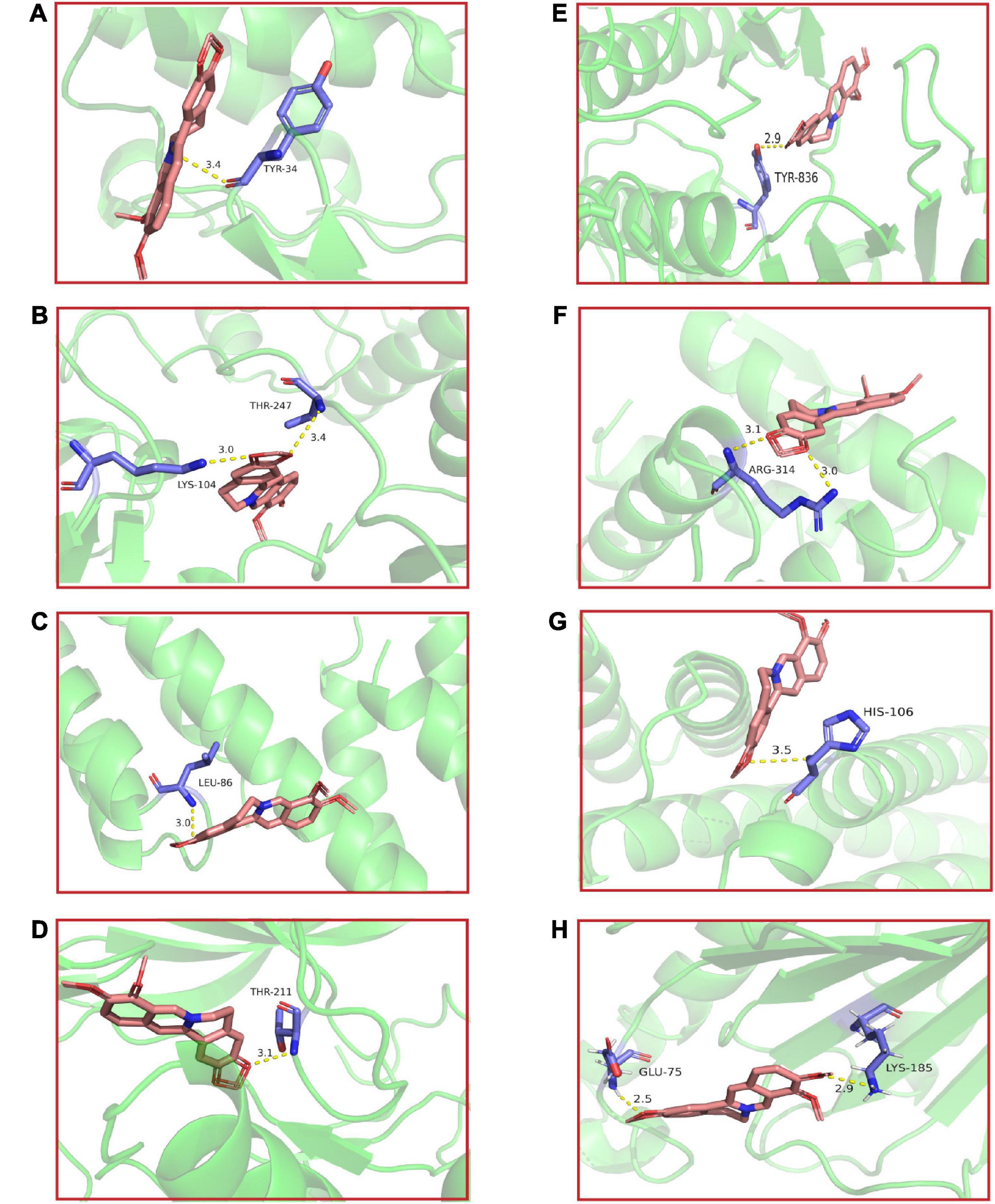
Figure 5. The molecular docking results of berberine (BBR) and key targets. BBR and (A) Mapk1; (B) Src; (C) Ctnnb1; (D) Akt1; (E) Pik3ca; (F) Tp53; (G) Jun; (H) Hsp90aa1.
4.5. RT-qPCR validation of core genes
Further RT-qPCR was performed on brain tissues from mice in the four groups. The results (Figure 6) demonstrated that the expression of Ctnnb1, Akt1, Pik3ca, and Hsp90aa1 was significantly lower in the model group compared with the control group (p < 0.05), the expression of Src showed a decreasing but not significant trend and the expression of Tp53 and Jun was significantly higher (p < 0.05, p < 0.01, respectively). Compared with the model group, the expression of Src, Ctnnb1, Akt1, Pik3ca, and Hsp90aa1 exhibited an increasing tendency in both the BBR-L and BBR-H groups, in which the expression of Ctnnb1 and Akt1 was significantly higher in the BBR-H group (p < 0.05). Tp53 and Jun expression showed a decreasing trend and were significantly lower in the BBR-H group (p < 0.05, p < 0.001, respectively). Unfortunately, statistically significant results for Mapk1 were not observed in our study.
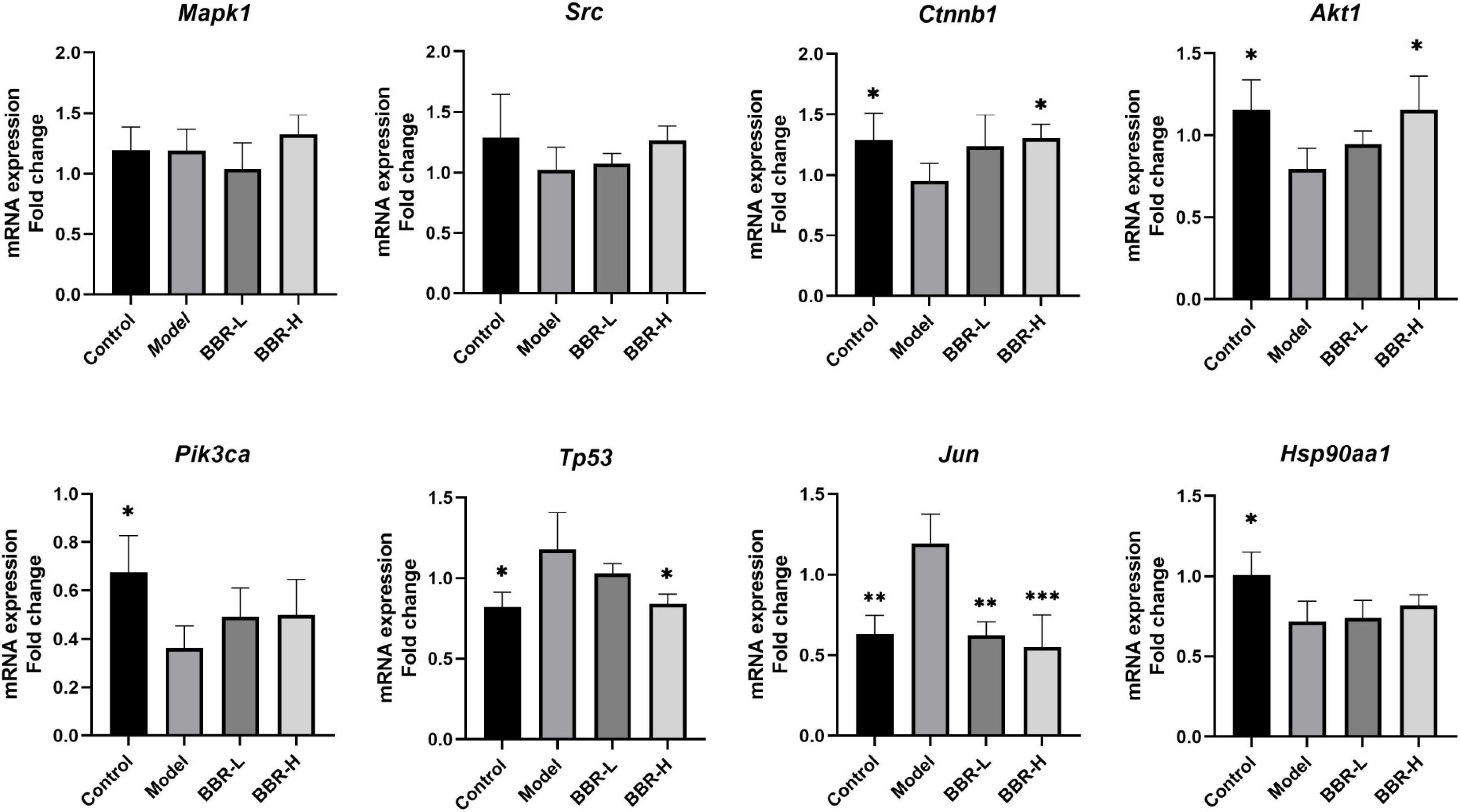
Figure 6. The results of RT-qPCR (All data are mean ± SEM with n = 3, *p < 0.05, **p < 0.01, ***p < 0.001 vs. Model).
5. Discussion
This study investigated the role and mechanism of BBR on aging-related spatial learning memory deficits due to D-gal with the assistance of network pharmacology and molecular docking techniques. Aging is considered to be the most important risk factor for neurodegenerative diseases, including AD. D-gal-induced aging mouse models have been widely used in aging studies, and long-term administration of D-gal induces aging, manifests neurological deficits, and shows significant spatial learning and memory deficits in the MWM test (Wei et al., 2008).
Based on a network pharmacological analysis, we constructed an animal model of aging-related cognitive impairment by subcutaneous injection of D-gal to evaluate the effects of BBR. Chronic administration of low doses of D-gal has been shown to induce changes that mimic the natural aging process in animals, including cognitive impairment (Wei et al., 2005). In our study, MWM behavioral tests were applied to detect changes in cognitive status after 10 weeks of subcutaneous injection of D-gal. We found that BBR treatment improved D-gal-induced learning and memory deficits. Previous studies have shown that BBR displays significant memory improvement activity in multiple animal models of memory deficits through various mechanisms, such as anti-inflammatory, anti-oxidative stress, cholinesterase inhibition, and anti-amyloid effects (Yuan et al., 2019). In addition, we explored the expression levels of aging markers and found that D-gal resulted in increased expression of the aging marker P16, and BBR administration ameliorated these changes. These results are consistent with previous studies (Dang et al., 2020). Administration of BBR for 6 months significantly improved cognitive deficits and insulin resistance in naturally aged rats (Yu et al., 2018). BBR emphasizes the induction of neuroprotective effects against Adriamycin-induced cognitive decline by modulating brain growth factors and exerting anti-inflammatory, anti-apoptotic, and antioxidant effects (Shaker et al., 2021). These results further support the ameliorative role of BBR in aging-related cognitive dysfunction.
Cognitive dysfunction is a common disorder in older individuals, and the incidence of MCI increases significantly with age (Petersen et al., 2018). MCI is a cognitive state between normal aging and dementia, and studies have shown that individuals with MCI are approximately three times more likely to progress to dementia over the next 2–5 years than age-matched controls (Petersen et al., 2018). It is well known that no drug is available to modify the disease process in AD, the most common type of dementia. Of particular note, it has been reported that 12.2% of MCI patients recover normal cognitive function within 3 years (McGirr et al., 2022). There are reasonable grounds to believe that reversing MCI to reduce the burden of dementia is a viable area of interest. There is compelling evidence to suggest that BBR was effective in improving cognitive function in Diabetes-related cognitive impairment and AD (Akbar et al., 2021; Hao et al., 2022). A spectrum of studies has demonstrated the ameliorative role of BBR in AD pathologies and cognitive dysfunction (He et al., 2017; Cai et al., 2018; Lin L. et al., 2020; Wu et al., 2021; Ye et al., 2021). BBR may improve cognitive impairment through a variety of mechanisms, including anti-inflammatory, anti-oxidative stress, improvement of insulin resistance, and inhibition of endoplasmic reticulum stress (Patil et al., 2015; Yu et al., 2018; Wang et al., 2019; Yuan et al., 2019; Shaker et al., 2021; Wu et al., 2021). Our study is the pioneer in exploring the mechanism by which BBR improves cognitive dysfunction with the assistance of network pharmacology and molecular docking techniques. To identify the therapeutic targets of BBR against CI, we identified 7,984 CI-validated targets and 470 BBR therapeutic targets using network pharmacology. We identified 386 potential targets associated with BBR and CI. In addition, BBR therapeutic targets associated with CI were used for GO and KEGG analyses. The results of GO analysis and KEGG analysis showed that BBR may exert its effects through MAPK and PI3K/Akt signaling pathways. Previous studies have shown that BBR can increase the levels of proteins located on neurosynapse through the MAPK signaling pathway (Zhou et al., 2016)and exert neuroprotective effects against tau protein hyperphosphorylation through PI3K/Akt pathway (Lin J. Y. et al., 2020).
The top 8 targets screened by network pharmacology were Mapk1, Src, Ctnnb1, Akt1, Pik3ca, Tp53, Jun, and Hsp90aa1. Molecular docking showed that the core targets dovetailed well with BBR, and RT-qPCR validated that the anti-aging-related cognitive dysfunction of BBR may be achieved by modulating Akt1, Ctnnb1, Tp53, and Jun. PI3K/Akt signaling pathway plays an essential role in regulating cell growth, proliferation, and survival. Akt activated by PI3K promotes cell growth and survival through the phosphorylation of multiple cytoplasmic proteins during senescence (Lin J. Y. et al., 2020). A previous study showed that berberine-induced PI3K/Akt activation exerts a protective effect by causing dephosphorylation of tau proteins and ameliorating neuronal axonal damage (Wang et al., 2018). In addition, BBR blocks Aβ production by activating the PI3K/Akt signaling pathway in vitro (Durairajan et al., 2012). Although our study only detected statistical differences in Akt1, Pik3ca showed the same trend of alteration, and it has also been shown that BBR can reduce tau hyperphosphorylation in 3 × Tg AD mice via the Akt/glycogen synthase kinase-3β (GSK3β) pathway (Chen et al., 2020). The Ctnnb1 gene encodes β-catenin, a pivotal component of the Wnt signaling pathway that plays a significant role in the regulation of cell proliferation, differentiation, and apoptosis (Liu et al., 2022). Wnt signaling declines with age in the rat brain (Jessberger et al., 2009) and has been identified in the cerebral cortex of AD patients (Folke et al., 2019). Targeting recovery of the Wnt/β-catenin signaling pathway is a promising therapeutic strategy for AD (Jia et al., 2019). The Tp53 gene produces the P53 protein, which functions as a transcription factor involved in cell cycle control, DNA repair, and regulation of cellular senescence and body aging (Rufini et al., 2013). A study reveals that BBR can depress the expression of P53 in primary neurons to keep cells in G0/G1 phase (Chai et al., 2013). c-Jun is an inducible transcription factor known to play a key role in neuronal cell death and survival (Raivich et al., 2004). Activated c-Jun expression is increased in the AD brain (Anderson et al., 1994; Raivich et al., 2004) and is present in the neurogenic fiber tangles of the AD brain (Pearson et al., 2006). Our results are in good agreement with previous studies and we support that BBR functions from multiple targets to improve cognitive function. In future studies, it is imperative to validate and explore our results more thoroughly and extensively.
6. Conclusion
Taken together, the results of this study suggest that BBR is able to attenuate spatial learning and memory impairment by inhibiting the expression of aging markers in the brain of the D-gal-induced senescence mice. Further network pharmacology and RT-qPCR validation suggest that this anti-aging effect may be achieved through the regulation of genes such as Akt1, Ctnnb1, Tp53, and Jun. These findings provide new evidence for the anti-aging protective effects of BBR and offer potential therapeutic directions for cognitive dysfunction. However, the specific targets of BBR in its anti-aging effects are not known, which is the main limitation of this study. Therefore, further studies are needed to identify whether BBR plays a role in improving cognitive function through improving the aging of specific cell types in the CNS and to elucidate the mechanism.
Data availability statement
The original contributions presented in this study are included in this article/Supplementary material, further inquiries can be directed to the corresponding authors.
Ethics statement
The animal study was reviewed and approved by Ethics Committee of Xiyuan Hospital, China Academy of Chinese Medical Sciences (No. 2021XLC035-3).
Author contributions
JY and WW performed the experiments and data analysis and wrote the manuscript. JW assisted with the experiments. YC and HL assisted in conceptualizing and revising the manuscript. All authors reviewed and approved the manuscript.
Funding
This study was funded by the Fundamental Research Funds for the Central Public Welfare Research Institutes (ZZ15-YQ-013 and ZZ15-XY-PT-02) and the Science and Technology Innovation Project of Chinese Academy of Traditional Chinese Medicine (CI2021A01401 and CI2021A04618).
Conflict of interest
The authors declare that the research was conducted in the absence of any commercial or financial relationships that could be construed as a potential conflict of interest.
The handling editor declared a shared affiliation with the authors WW and YC at the time of review.
Publisher’s note
All claims expressed in this article are solely those of the authors and do not necessarily represent those of their affiliated organizations, or those of the publisher, the editors and the reviewers. Any product that may be evaluated in this article, or claim that may be made by its manufacturer, is not guaranteed or endorsed by the publisher.
Supplementary material
The Supplementary Material for this article can be found online at: https://www.frontiersin.org/articles/10.3389/fnins.2023.1093180/full#supplementary-material
Footnotes
- ^ https://www.genecards.org/
- ^ https://go.drugbank.com/
- ^ https://db.idrblab.net/ttd/
- ^ https://www.uniprot.org/
- ^ http://swisstargetprediction.ch/
- ^ http://www.lilab-ecust.cn/pharmmapper/
- ^ https://string-db.org/
- ^ https://david.ncifcrf.gov/
- ^ https://www.bioinformatics.com.cn/
- ^ https://tcmsp-e.com/tcmsp.php
- ^ https://www.rcsb.org/
- ^ http://vina.scripps.edu/
- ^ https://pymol.org/2/
References
Akbar, M., Shabbir, A., Rehman, K., Akash, M. S. H., and Shah, M. A. (2021). Neuroprotective potential of berberine in modulating Alzheimer’s disease via multiple signaling pathways. J. Food Biochem. 45:e13936. doi: 10.1111/jfbc.13936
Anderson, A. J., Cummings, B. J., and Cotman, C. W. (1994). Increased immunoreactivity for Jun-and Fos-related proteins in Alzheimer’s disease: association with pathology. Exp. Neurol. 125, 286–295. doi: 10.1006/exnr.1994.1031
Banji, O. J., Banji, D., and Ch, K. (2014). Curcumin and hesperidin improve cognition by suppressing mitochondrial dysfunction and apoptosis induced by D-galactose in rat brain. Food Chem. Toxicol. 74, 51–59. doi: 10.1016/j.fct.2014.08.020
Bova, S., Padrini, R., Goldman, W., Berman, D., and Cargnelli, G. (1992). On the mechanism of vasodilating action of berberine: possible role of inositol lipid signaling system. J. Pharmacol. Exp. Ther. 261, 318–323.
Cai, Z., Wang, C., He, W., and Chen, Y. (2018). Berberine alleviates amyloid-beta pathology in the brain of APP/PS1 transgenic mice via inhibiting β/γ-secretases activity and enhancing α-secretases. Curr. Alzheimer Res. 15, 1045–1052. doi: 10.2174/1567205015666180702105740
Chai, Y. S., Hu, J., Lei, F., Wang, Y. G., Yuan, Z. Y., Lu, X., et al. (2013). Effect of berberine on cell cycle arrest and cell survival during cerebral ischemia and reperfusion and correlations with p53/cyclin D1 and PI3K/Akt. Eur. J. Pharmacol. 708, 44–55. doi: 10.1016/j.ejphar.2013.02.041
Chen, Y., Chen, Y., Liang, Y., Chen, H., Ji, X., and Huang, M. (2020). Berberine mitigates cognitive decline in an Alzheimer’s disease Mouse Model by targeting both tau hyperphosphorylation and autophagic clearance. Biomed. Pharmacother. 121:109670. doi: 10.1016/j.biopha.2019.109670
Cheng, Z., Kang, C., Che, S., Su, J., Sun, Q., Ge, T., et al. (2022). Berberine: a promising treatment for neurodegenerative diseases. Front. Pharmacol. 13:845591. doi: 10.3389/fphar.2022.845591
Choi, M. S., Yuk, D. Y., Oh, J. H., Jung, H. Y., Han, S. B., Moon, D. C., et al. (2008). Berberine inhibits human neuroblastoma cell growth through induction of p53-dependent apoptosis. Anticancer Res. 28, 3777–3784.
Daina, A., Michielin, O., and Zoete, V. (2019). SwissTargetPrediction: updated data and new features for efficient prediction of protein targets of small molecules. Nucleic Acids Res. 47, W357–W364. doi: 10.1093/nar/gkz382
Dang, Y., An, Y., He, J., Huang, B., Zhu, J., Gao, M., et al. (2020). Berberine ameliorates cellular senescence and extends the lifespan of mice via regulating p16 and cyclin protein expression. Aging Cell 19:e13060. doi: 10.1111/acel.13060
Daroi, P. A., Dhage, S. N., and Juvekar, A. R. (2022). p-Coumaric acid protects against D-galactose induced neurotoxicity by attenuating neuroinflammation and apoptosis in mice brain. Metab. Brain Dis. 37, 2569–2579. doi: 10.1007/s11011-022-01007-3
Durairajan, S. S. K., Liu, L.-F., Lu, J.-H., Chen, L.-L., Yuan, Q., Chung, S. K., et al. (2012). Berberine ameliorates β-amyloid pathology, gliosis, and cognitive impairment in an Alzheimer’s disease transgenic mouse model. Neurobiol. Aging 33, 2903–2919. doi: 10.1016/j.neurobiolaging.2012.02.016
Folke, J., Pakkenberg, B., and Brudek, T. (2019). Impaired Wnt signaling in the prefrontal cortex of Alzheimer’s disease. Mol. Neurobiol. 56, 873–891. doi: 10.1007/s12035-018-1103-z
Hao, Y., Li, J., Yue, S., Wang, S., Hu, S., and Li, B. (2022). Neuroprotective effect and possible mechanisms of berberine in diabetes-related cognitive impairment: a systematic review and meta-analysis of animal studies. Front. Pharmacol. 13:917375. doi: 10.3389/fphar.2022.917375
He, W., Wang, C., Chen, Y., He, Y., and Cai, Z. (2017). Berberine attenuates cognitive impairment and ameliorates tau hyperphosphorylation by limiting the self-perpetuating pathogenic cycle between NF-κB signaling, oxidative stress and neuroinflammation. Pharmacol. Rep. 69, 1341–1348. doi: 10.1016/j.pharep.2017.06.006
Hu, C., Wang, L., Guo, Y., Cao, Z., Lu, Y., and Qin, H. (2020). Study of the risk and preventive factors for progress of mild cognitive impairment to dementia. Am. J. Alzheimers Dis. Other Dement. 35:1533317520925324. doi: 10.1177/1533317520925324
Hugo, J., and Ganguli, M. (2014). Dementia and cognitive impairment: epidemiology, diagnosis, and treatment. Clin. Geriatr. Med. 30, 421–442. doi: 10.1016/j.cger.2014.04.001
Jeong, H. W., Hsu, K. C., Lee, J.-W., Ham, M., Huh, J. Y., Shin, H. J., et al. (2009). Berberine suppresses proinflammatory responses through AMPK activation in macrophages. Am. J. Physiol. Endocrinol. Metab. 296, E955–E964.
Jessberger, S., Clark, R. E., Broadbent, N. J., Clemenson, G. D., Consiglio, A., Lie, D. C., et al. (2009). Dentate gyrus-specific knockdown of adult neurogenesis impairs spatial and object recognition memory in adult rats. Learn. Mem. 16, 147–154. doi: 10.1101/lm.1172609
Jia, L., Piña-Crespo, J., and Li, Y. (2019). Restoring Wnt/β-catenin signaling is a promising therapeutic strategy for Alzheimer’s disease. Mol. Brain 12:104. doi: 10.1186/s13041-019-0525-5
Kim, W. Y., and Sharpless, N. E. (2006). The regulation of INK4/ARF in cancer and aging. Cell 127, 265–275. doi: 10.1016/j.cell.2006.10.003
Koepsell, T. D., and Monsell, S. E. (2012). Reversion from mild cognitive impairment to normal or near-normal cognition: risk factors and prognosis. Neurology 79, 1591–1598. doi: 10.1212/WNL.0b013e31826e26b7
Li, C., Jiang, S., Wang, H., Wang, Y., Han, Y., and Jiang, J. (2022). Berberine exerts protective effects on cardiac senescence by regulating the Klotho/SIRT1 signaling pathway. Biomed. Pharmacother. 151:113097. doi: 10.1016/j.biopha.2022.113097
Li, H. Y., Wang, X. C., Xu, Y. M., Luo, N. C., Luo, S., Hao, X. Y., et al. (2018). Berberine improves diabetic encephalopathy through the SIRT1/ER stress pathway in db/db mice. Rejuvenation Res. 21, 200–209. doi: 10.1089/rej.2017.1972
Lin, J. Y., Kuo, W. W., Baskaran, R., Kuo, C. H., Chen, Y. A., Chen, W. S., et al. (2020). Swimming exercise stimulates IGF1/PI3K/Akt and AMPK/SIRT1/PGC1α survival signaling to suppress apoptosis and inflammation in aging hippocampus. Aging 12, 6852–6864. doi: 10.18632/aging.103046
Lin, L., Li, C., Zhang, D., Yuan, M., Chen, C.-H., and Li, M. (2020). Synergic effects of berberine and curcumin on improving cognitive function in an Alzheimer’s disease mouse model. Neurochem. Res. 45, 1130–1141. doi: 10.1007/s11064-020-02992-6
Liu, J., Xiao, Q., Xiao, J., Niu, C., Li, Y., Zhang, X., et al. (2022). Wnt/β-catenin signalling: function, biological mechanisms, and therapeutic opportunities. Signal. Transduct. Target. Ther. 7:3. doi: 10.1038/s41392-021-00762-6
Luchsinger, J. A. (2012). Type 2 diabetes and cognitive impairment: linking mechanisms. J. Alzheimers Dis. 30, S185–S198. doi: 10.3233/JAD-2012-111433
Ma, C. Y., Shi, X. Y., Wu, Y. R., Zhang, Y., Yao, Y. H., Qu, H. L., et al. (2021). Berberine attenuates atherosclerotic lesions and hepatic steatosis in ApoE(−/−) mice by down-regulating PCSK9 via ERK1/2 pathway. Ann. Transl. Med. 9:1517. doi: 10.21037/atm-20-8106
McGirr, A., Nathan, S., Ghahremani, M., Gill, S., Smith, E. E., and Ismail, Z. (2022). Progression to dementia or reversion to normal cognition in mild cognitive impairment as a function of late-onset neuropsychiatric symptoms. Neurology 98, e2132–e2139. doi: 10.1212/wnl.0000000000200256
Nagy, G., and Pohl, N. L. (2015). Complete hexose isomer identification with mass spectrometry. J. Am. Soc. Mass Spectrom. 26, 677–685. doi: 10.1007/s13361-014-1072-z
Panahi, N., Mahmoudian, M., Mortazavi, P., and Hashjin, G. S. (2013). Experimental research effects of berberine on β-secretase activity in a rabbit model of Alzheimer’s disease. Arch. Med. Sci. 9, 146–150. doi: 10.5114/aoms.2013.33354
Park, M. H., Kwon, D. Y., Jung, J. M., Han, C., Jo, I., and Jo, S. A. (2013). Mini-Mental Status Examination as predictors of mortality in the elderly. Acta Psychiatr. Scand. 127, 298–304. doi: 10.1111/j.1600-0447.2012.01918.x
Patil, S., Tawari, S., Mundhada, D., and Nadeem, S. (2015). Protective effect of berberine, an isoquinoline alkaloid ameliorates ethanol-induced oxidative stress and memory dysfunction in rats. Pharmacol. Biochem. Behav. 136, 13–20. doi: 10.1016/j.pbb.2015.07.001
Pearson, A. G., Byrne, U. T. E., MacGibbon, G. A., Faull, R. L. M., and Dragunow, M. (2006). Activated c-Jun is present in neurofibrillary tangles in Alzheimer’s disease brains. Neurosci. Lett. 398, 246–250. doi: 10.1016/j.neulet.2006.01.031
Petersen, R. C. (2004). Mild cognitive impairment as a diagnostic entity. J. Intern. Med. 256, 183–194.
Petersen, R. C., Lopez, O., Armstrong, M. J., Getchius, T. S. D., Ganguli, M., Gloss, D., et al. (2018). Practice guideline update summary: mild cognitive impairment: report of the guideline development, dissemination, and implementation subcommittee of the American academy of neurology. Neurology 90, 126–135. doi: 10.1212/wnl.0000000000004826
Pirillo, A., and Catapano, A. L. (2015). Berberine, a plant alkaloid with lipid- and glucose-lowering properties: from in vitro evidence to clinical studies. Atherosclerosis 243, 449–461. doi: 10.1016/j.atherosclerosis.2015.09.032
Raivich, G., Bohatschek, M., Da Costa, C., Iwata, O., Galiano, M., Hristova, M., et al. (2004). The AP-1 transcription factor c-Jun is required for efficient axonal regeneration. Neuron 43, 57–67. doi: 10.1016/j.neuron.2004.06.005
Ru, J., Li, P., Wang, J., Zhou, W., Li, B., Huang, C., et al. (2014). TCMSP: a database of systems pharmacology for drug discovery from herbal medicines. J. Cheminform. 6:13. doi: 10.1186/1758-2946-6-13
Rufini, A., Tucci, P., Celardo, I., and Melino, G. (2013). Senescence and aging: the critical roles of p53. Oncogene 32, 5129–5143. doi: 10.1038/onc.2012.640
Safran, M., Rosen, N., Twik, M., BarShir, R., Stein, T. I., Dahary, D., et al. (2021). “The genecards suite,” in Practical Guide to Life Science Databases, eds I. Abugessaisa and T. Kasukawa (Singapore: Springer Nature Singapore), 27–56.
Shaker, F. H., El-Derany, M. O., Wahdan, S. A., El-Demerdash, E., and El-Mesallamy, H. O. (2021). Berberine ameliorates doxorubicin-induced cognitive impairment (chemobrain) in rats. Life Sci. 269:119078. doi: 10.1016/j.lfs.2021.119078
Sherman, B. T., Hao, M., Qiu, J., Jiao, X., Baseler, M. W., Lane, H. C., et al. (2022). DAVID: a web server for functional enrichment analysis and functional annotation of gene lists (2021 update). Nucleic Acids Res. 50, W216–W221. doi: 10.1093/nar/gkac194
Shwe, T., Pratchayasakul, W., Chattipakorn, N., and Chattipakorn, S. C. (2018). Role of D-galactose-induced brain aging and its potential used for therapeutic interventions. Exp. Gerontol. 101, 13–36. doi: 10.1016/j.exger.2017.10.029
Sun, K., Yang, P., Zhao, R., Bai, Y., and Guo, Z. (2018). Matrine attenuates D-galactose-induced aging-related behavior in mice via inhibition of cellular senescence and oxidative stress. Oxid. Med. Cell. Longev. 2018:7108604. doi: 10.1155/2018/7108604
Szklarczyk, D., Gable, A. L., Nastou, K. C., Lyon, D., Kirsch, R., Pyysalo, S., et al. (2021). The STRING database in 2021: customizable protein-protein networks, and functional characterization of user-uploaded gene/measurement sets. Nucleic Acids Res. 49, D605–D612. doi: 10.1093/nar/gkaa1074
UniProt Consortium (2020). UniProt: the universal protein knowledgebase in 2021. Nucleic Acids Res. 49, D480–D489. doi: 10.1093/nar/gkaa1100
Vorhees, C. V., and Williams, M. T. (2006). Morris water maze: procedures for assessing spatial and related forms of learning and memory. Nat. Protoc. 1, 848–858. doi: 10.1038/nprot.2006.116
Wang, K., Chen, Q., Wu, N., Li, Y., Zhang, R., Wang, J., et al. (2019). Berberine ameliorates spatial learning memory impairment and modulates cholinergic anti-inflammatory pathway in diabetic rats. Front. Pharmacol. 10:1003. doi: 10.3389/fphar.2019.01003
Wang, Q., Sun, Y. N., Zou, C. M., Zhang, T. L., Li, Z., Liu, M., et al. (2022). Regulation of the kynurenine/serotonin pathway by berberine and the underlying effect in the hippocampus of the chronic unpredictable mild stress mice. Behav. Brain Res. 422:113764. doi: 10.1016/j.bbr.2022.113764
Wang, S., He, B., Hang, W., Wu, N., Xia, L., Wang, X., et al. (2018). Berberine alleviates tau hyperphosphorylation and axonopathy-associated with diabetic encephalopathy via restoring PI3K/Akt/GSK3β pathway. J. Alzheimers Dis. 65, 1385–1400. doi: 10.3233/jad-180497
Wang, X., Shen, Y., Wang, S., Li, S., Zhang, W., Liu, X., et al. (2017). PharmMapper 2017 update: a web server for potential drug target identification with a comprehensive target pharmacophore database. Nucleic Acids Res. 45, W356–W360. doi: 10.1093/nar/gkx374
Wei, H., Cai, Y., Chu, J., Li, C., and Li, L. (2008). Temporal gene expression profile in hippocampus of mice treated with d-galactose. Cell. Mol. Neurobiol. 28, 781–794. doi: 10.1007/s10571-007-9177-6
Wei, H., Li, L., Song, Q., Ai, H., Chu, J., and Li, W. (2005). Behavioural study of the d-galactose induced aging model in C57BL/6J mice. Behav. Brain Res. 157, 245–251. doi: 10.1016/j.bbr.2004.07.003
Wishart, D. S., Feunang, Y. D., Guo, A. C., Lo, E. J., Marcu, A., Grant, J. R., et al. (2018). DrugBank 5.0: a major update to the DrugBank database for 2018. Nucleic Acids Res. 46, D1074–D1082. doi: 10.1093/nar/gkx1037
Wu, Y., Chen, Q., Wen, B., Wu, N., He, B., and Chen, J. (2021). Berberine reduces Aβ(42) deposition and Tau hyperphosphorylation via ameliorating endoplasmic reticulum stress. Front. Pharmacol. 12:640758. doi: 10.3389/fphar.2021.640758
Xu, Z., Feng, W., Shen, Q., Yu, N., Yu, K., Wang, S., et al. (2017). Rhizoma coptidis and berberine as a natural drug to combat aging and aging-related diseases via anti-oxidation and AMPK activation. Aging Dis. 8, 760–777. doi: 10.14336/ad.2016.0620
Ye, C., Liang, Y., Chen, Y., Xiong, Y., She, Y., Zhong, X., et al. (2021). Berberine improves cognitive impairment by simultaneously impacting cerebral blood flow and β-amyloid accumulation in an APP/tau/PS1 mouse model of Alzheimer’s disease. Cells 10:1161. doi: 10.3390/cells10051161
Yu, Y., Zhao, Y., Teng, F., Li, J., Guan, Y., Xu, J., et al. (2018). Berberine improves cognitive deficiency and muscular dysfunction via activation of the AMPK/SIRT1/PGC-1a pathway in skeletal muscle from naturally aging rats. J. Nutr. Health Aging 22, 710–717. doi: 10.1007/s12603-018-1015-7
Yuan, N.-N., Cai, C.-Z., Wu, M.-Y., Su, H.-X., Li, M., and Lu, J.-H. (2019). Neuroprotective effects of berberine in animal models of Alzheimer’s disease: a systematic review of pre-clinical studies. BMC Complement. Altern. Med. 19:109. doi: 10.1186/s12906-019-2510-z
Zhang, H., Wei, J., Xue, R., Wu, J. D., Zhao, W., Wang, Z. Z., et al. (2010). Berberine lowers blood glucose in type 2 diabetes mellitus patients through increasing insulin receptor expression. Metabolism 59, 285–292. doi: 10.1016/j.metabol.2009.07.029
Zhao, H., Halicka, H. D., Li, J., and Darzynkiewicz, Z. (2013). Berberine suppresses gero-conversion from cell cycle arrest to senescence. Aging 5:623. doi: 10.18632/aging.100593
Zhou, J., Du, X., Long, M., Zhang, Z., Zhou, S., Zhou, J., et al. (2016). Neuroprotective effect of berberine is mediated by MAPK signaling pathway in experimental diabetic neuropathy in rats. Eur. J. Pharmacol. 774, 87–94. doi: 10.1016/j.ejphar.2016.02.007
Keywords: berberine, cognitive dysfunction, D-galactose, RT-qPCR, network pharmacology
Citation: Yao J, Wei W, Wen J, Cao Y and Li H (2023) The efficacy and mechanism of berberine in improving aging-related cognitive dysfunction: A study based on network pharmacology. Front. Neurosci. 17:1093180. doi: 10.3389/fnins.2023.1093180
Received: 08 November 2022; Accepted: 06 January 2023;
Published: 20 January 2023.
Edited by:
Dennis Chang, Western Sydney University, AustraliaReviewed by:
Chaohua Li, Peking University, ChinaHe Zhang, Guang’anmen Hospital, China Academy of Chinese Medical Sciences, China
Copyright © 2023 Yao, Wei, Wen, Cao and Li. This is an open-access article distributed under the terms of the Creative Commons Attribution License (CC BY). The use, distribution or reproduction in other forums is permitted, provided the original author(s) and the copyright owner(s) are credited and that the original publication in this journal is cited, in accordance with accepted academic practice. No use, distribution or reproduction is permitted which does not comply with these terms.
*Correspondence: Yu Cao, yu.cao-xiyuan@outlook.com; Hao Li,
xyhplihao1965@126.com
†These authors have contributed equally to this work and share first authorship