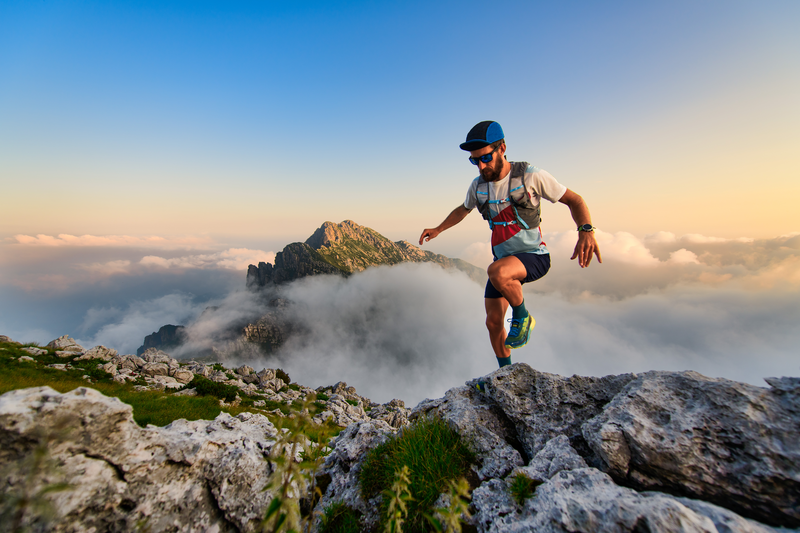
95% of researchers rate our articles as excellent or good
Learn more about the work of our research integrity team to safeguard the quality of each article we publish.
Find out more
ORIGINAL RESEARCH article
Front. Neurosci. , 31 October 2022
Sec. Neuroenergetics and Brain Health
Volume 16 - 2022 | https://doi.org/10.3389/fnins.2022.998875
While prefrontal cortex dysfunction has been implicated in high food cravings, other cortical regions, like the parietal cortex, are potentially also involved in regulating craving. This study explored the effects of stimulating the inferior parietal lobule (IPL) and dorsolateral prefrontal cortex (DLPFC) on food craving state and trait. Transcranial direct current stimulation (tDCS) was administered at 1.5 mA for 5 consecutive days. Participants received 20 min of IPL, DLPFC, or sham stimulation (SHAM) each day which consisted of two rounds of 10-min stimulation, divided by a 10-min mindfulness task break. In addition, we studied inhibition and subjective psychological aspects like body image and self-esteem state and trait. To decompose immediate and cumulative effects, we measured the following on days 1 and 5: inhibition through the Go/No-go task; and food craving, self-esteem, and body appreciation through a battery of questionnaires. We found that false alarm errors decreased in the participants receiving active stimulation in the DLPFC (DLPFC-group). In contrast, false alarm errors increased in participants receiving active stimulation in the IPL (IPL-group). At the same time, no change was found in the participants receiving SHAM (SHAM-group). There was a trending reduction in craving trait in all groups. Momentary craving was decreased in the DLPFC-group and increased in IPL-group, yet a statistical difference was not reached. According to time and baseline, self-esteem and body perception improved in the IPL-group. Furthermore, self-esteem trait significantly improved over time in the DLPFC-group and IPL-group. These preliminary results indicate that tDCS modulates inhibition in frontoparietal areas with opposite effects, enhancing it in DLPFC and impairing it in IPL. Moreover, craving is moderately linked to inhibition, self-esteem, and body appreciation which seem not to be affected by neuromodulation but may rely instead on broader regions as more complex constructs. Finally, the fractionated protocol can effectively influence inhibition with milder effects on other constructs.
Eating is an essential human function linked to survival, pleasure, and reward. An important driving factor of eating is craving, a desire to consume a specific food that is difficult to ignore or satisfy by consuming an alternative (Martin et al., 2011). Food craving has been associated with eating disorder psychopathologies (Davis et al., 2011; Chao et al., 2016; Parker et al., 2021), weight gain (Boswell and Kober, 2016), and addiction relapse (Koob and Volkow, 2010). Therefore, it is essential to study food cravings in the context of preventing obesity and designing efficient interventions, especially when considering the effect of the recent lockdown on eating behaviors (Bhutani et al., 2021).
The research literature exploring brain centers regulating craving behavior focuses on the dorsolateral prefrontal cortex (DLPFC) as one of the critical nodes controlling eating behavior (Lefaucheur et al., 2017; Mostafavi et al., 2020; Saruco and Pleger, 2021). It has been suggested that the involvement of the DLPFC in eating behavior (Gomis-Vicent et al., 2019) is linked to its regulation of reward (McClure et al., 2004; Beaver et al., 2006), decision making (Knoch et al., 2006), and inhibitory control functions (Sridharan et al., 2008). In addition, the prefrontal cortex (PFC) typically orchestrates the brain resources to achieve a specific goal by guiding the activity of more posterior or subcortical areas (Miller and Cohen, 2001)and possibly automating these processes. This points toward the existence of a frontoparietal network, including areas like the DLPFC and inferior parietal lobule (stocktickerIPL) (Fincham et al., 2001; Dosenbach et al., 2007). It has been reported that parietal areas are involved in craving recreational drugs (Garavan et al., 2000; Noori et al., 2016), tobacco (Due et al., 2002; Ghahremani et al., 2018), alcohol (Schacht et al., 2013), and food (Giuliani and Pfeifer, 2015; Han et al., 2018; Stopyra et al., 2021). Furthermore, research combining functional magnetic resonance imaging (fMRI) and machine learning found that connectivity based models outperformed models limited to specific regional activity. This suggest that the regulation of craving is more strongly associated with interactions between brain regions compared to isolated regional activities (Kulkarni et al., 2022).
A recent meta-analysis indicated a more robust effect of right DLPFC stimulation on food craving reduction compared to the left side (Ester and Kullmann, 2022). This seems consistent with the right brain hypothesis of obesity (Alonso-Alonso and Pascual-Leone, 2007), which regards the right PFC as an important area for controlling food intake. Other theories have pointed out the imbalance between activity in the left and right frontal cortex, which is commonly referred to as asymmetric frontal cortical activity. According to this, right frontal cortical activity is associated with avoidance motivation, while left frontal cortical activity is associated with approach (Schutter et al., 2008; Kelley et al., 2017; McGeown and Davis, 2018). As claimed by this theory, stimulating the right side could result in a reduction of craving. Furthermore, attentional bias toward food has been linked with left frontal asymmetry (McGeown and Davis, 2018). While non-invasive brain stimulation (NIBS) has previously reduced the approach motivation and food craving by targeting the right prefrontal areas (Fregni et al., 2008). Regarding the parietal regions, electroencephalography (EEG) studies have linked resting-state right-sided alpha parieto-occipital activation to reduced hedonic food appreciation (van Bochove et al., 2016). Overall, evidence indicates that neuromodulation of the right DLPFC activity effectively reduces food cravings, and that the effects may outlast the intervention. Currently, research has not explored the effects of right IPL neuromodulation as part of a frontoparietal network in food craving.
Over the past decade, several novel approaches emerged, focusing on the modulation of DLPFC activity to enhance the control and reduction of craving (Gluck et al., 2017). Transcranial direct current stimulation (tDCS) is an extensively used neuromodulation technique that delivers a mild electrical current to the cortex via electrodes attached to the scalp (Vance et al., 2016). This electrical current passes through the skull, modifies neuronal trans-membrane potentials (Boes et al., 2018), and ultimately affects neuroplasticity (Nitsche et al., 2008). This method has shown varying degrees of therapeutic effects on multiple neurodegenerative diseases (Brunoni et al., 2016; Saxena and Pal, 2021). Furthermore, tDCS applied over the DLPFC can induce an immediate reduction of acute food craving (Goldman et al., 2011; Martin et al., 2011; Montenegro et al., 2012; Gluck et al., 2015) and elicit long-lasting effects which exceed the intervention (Ljubisavljevic et al., 2016).
One of the critical factors interacting and influencing craving is inhibition. According to the VandenBos and American Psychological Association (2015), inhibition implies the active blocking or delay of a response to a stimulus. The lack of inhibition has been associated with various addictions (Goldstein and Volkow, 2002; Volkow et al., 2016) and poor dietary habits (Mobbs et al., 2011; Forcano et al., 2018; Jones et al., 2018). Prefrontal areas are also established as a hub of inhibitory control (Goldstein and Volkow, 2011; Carr et al., 2021). Most NIBS studies investigating inhibition targeted areas such as the inferior frontal gyrus, DLPFC, and the orbital frontal cortex (Sarkis et al., 2014; Schluter et al., 2018; de Boer et al., 2021). Inhibition has also been linked to parietal brain regions (Menon et al., 2001; Osada et al., 2019; Kolodny et al., 2020; Ouerfelli-Ethier et al., 2021). This highlights the existence of a frontoparietal network that relates to executive control processes (Barbey et al., 2012; Niendam et al., 2012) and inhibition (Miyake et al., 2000).
Craving and obesity have been associated with a general sense of wellbeing (Vallis, 2019) and a physical appearance subdomain of self-esteem (Griffiths et al., 2010), possibly through cognitive biases related to the selective interpretation of body size and shape (Williamson et al., 1999). Empirical data have shown that body image bias is present in healthy subjects (Gagne et al., 2012; Cooper and Wade, 2015) and those with eating pathologies (van den Eynde et al., 2011; Lewer et al., 2017; Strand et al., 2021). Moreover, studies have suggested an interplay of factors like higher BMI, low self-esteem, and weight bias internalization to food addiction (Pape et al., 2021).
Apart from craving and inhibition, frontoparietal areas have been linked to self-awareness (Lavarco et al., 2022), body awareness (Takeuchi et al., 2019), normal bodily self-consciousness (Igelström and Graziano, 2017), self-appraisal (Knyazev et al., 2021), and more specifically to self-esteem (Agroskin et al., 2014). Resting-state fMRI research has revealed positive relations between the DLPFC and trait self-esteem (Pan et al., 2016). Transcranial direct current stimulation (tDCS) of the prefrontal cortex has been found to improve self-esteem through the reduction of rumination (De Raedt et al., 2017), although with varying results (Longe et al., 2010; Jiang et al., 2018). The IPL has been related to self-appraisal (Delahoy et al., 2022) and conscious awareness of the self (Davey et al., 2016). It is also part of the default network (Di and Biswal, 2014) associated with self-referential processing (Raichle, 2015). Thus, it seems plausible to assume that neuromodulation may, by altering the activity of the DLPFC and the IPL, impact self-esteem, body appreciation, and inhibition which may, if affected, interact with craving.
In summary, we explored whether tDCS of the IPL and the DLPFC may be effective in modulating craving. We also hypothesized that neuromodulation of these areas would affect inhibition and constructs like self-esteem and body perception, by potentially interacting with food cravings in high-food-craving participants.
Initially, recruitment was done through a broadcast email to all staff and students of UAEU and a poster displayed outside the lab. A total of 174 subjects responded to our email and completed the first screening questionnaire, 67 were eligible, and 29 subjects participated (Mage = 26.24, SD = 10.44, ranging from 18 to 55 years), 12 females.
Mean BMI was 25.72 kg/m2 (SD = 5.81, minimum value = 17.20, maximum value = 41.3). All the participants were naïve to tDCS stimulation. Power analysis was calculated through G-power 3.1.5 (Erdfelder et al., 2009), which estimated a total sample of 31 would be needed to reach a statistical power of 0.09, and significance was set at 0.05. For practical reasons related to the Covid pandemic, we reached a sample of 29 individuals. Exclusion criteria included: a history of neuropsychiatric or eating disorder, chronic disease, depression, seizures, migraines, pregnancy, alcohol or drug consumption, as well as contraindications for tDCS such as metal implants in the head, scars, surgeries, and previous loss of consciousness, while to be included subjects had to be right-handed and have a high level of craving as measured by reduced Food Cravings Questionnaire-Trait (FCQ-T) (Cepeda-Benito et al., 2000; Meule et al., 2014) as well as a craving visual analog scale (CVAS). Overall scores of both questionnaires ranged from 27 to 150, and the cutoff for inclusion in the study was a score of 100 and above.
All participants signed informed consent, and the study was approved by the HERTC (Human Ethics at UAEU–ERH-2019-5910).
All the initial subjects interested in this study were redirected to a screening questionnaire to collect categorical data such as age, height, weight, and gender. Thereafter the questionnaire continued, and they completed the reduced FCQ-T (Cepeda-Benito et al., 2000; Meule et al., 2014) and the CVAS. In the reduced version of FCQ-T, subjects had to answer 15 questions rated on a 6-point scale ranging from never = 1 to always = 6. Measurement of craving by CVAS is easy to collect and is easily quantified (McMillan and Gilmore-Thomas, 1996). During the CVAS questionnaire, participants were exposed to 12 food pictures (three sweets, three carbohydrates, three fast-food items, and three high fats). Participants had to rate “How much would you like to eat this” on a scale from 1 to 5. The pictures were taken from the foodcast research image database (Foroni et al., 2013), consisting of standardized pictures of food and non-food items.
We applied tDCS over 5 consecutive days, which was administered through a transcranial DC Stimulator (Model 1300A, Soterix Medical Inc., USA) connected to saline-soaked sponge pads (5 × 7 cm). The intensity was set to 1.5 mA with a ramp up and down of 30 s each and a current density of 0.04 mA/cm2.
Stimulation was divided into two sessions of 10 min each with a break of 10 min in between, creating a fractionated tDCS protocol. In the sham stimulation group (SHAM-group), after the initial ramp-up of 30 s, the current intensity was automatically set to zero for 10 min, ending with a ramp-down of 30 s.
The anode was placed over F4, according to the international 10–20 system, on participants who received DLPFC (DLPFC-group) and SHAM (SHAM-group) stimulation. DLPFC position near F4 has been supported by neuronavigation studies (Fitzgerald et al., 2009) and is constantly used in food craving studies (Sedgmond et al., 2019; Ghobadi-Azbari et al., 2022). While the anode was placed over P4 on participants who received IPL (IPL-group) stimulation (Herwig et al., 2003). This position is also widely used in non-navigated NIBS studies (Coulborn et al., 2020; Golaszewski et al., 2021). In all the groups, the cathode was placed in the left supraorbital area, FP1. The use of the 10–20 based system has a considerable degree of face validity as the system accounts for individual variation in head size that is not accounted or in other methods like 5 cm approach (Fitzgerald et al., 2009).
The task employed in study inhibition was the Go/No-go task (Bari and Robbins, 2013) which consisted of showing the participant 4 quadrants on a black screen. The letters “P” or “R” randomly appeared in a quadrant at a time. Subjects were instructed to press a button when they saw a “P” on the screen and refrain from pressing it when seeing the “R.” The order was reversed in the second half of the task. Before each task, there was a short training session. Inhibition parameters followed those described in Bezdjian et al. (2009). The Go/No-go task was administered on a computer through a free program, PEBL (Mueller and Piper, 2014). We analyzed the number of False Alarms (FA) as an indicator of inhibition by quantifying the number of errors when the button was pressed when it was not required.
The Food Cravings Questionnaire-State (FCQ-S) and FCQ-T are two psychometrically sound measures to quantify an individual’s level of food craving, with excellent internal consistency and construct validity (Cepeda-Benito et al., 2000).
The FCQ-T questionnaire consists of 39 statements measuring the stability of craving features over time and situations. Subjects had to rate each statement on a 6-point scale ranging from never = 1 to always = 6, where higher scores indicate a higher level of craving. It measures nine dimensions such as intention and planning to eat, the anticipation of positive reinforcement from eating, anticipation of relief from negative feelings, lack of control, overeating, preoccupation with food, craving as a physiological state, and emotions before or during craving or eating, environmental triggers of craving and guilt resulting from giving in to cravings. Overall, Cronbach’s alpha is 0.97 (Cepeda-Benito et al., 2000).
The FCQ-S questionnaire measures the contextual states of craving. It has five subscales: the desire to eat, the anticipation of positive reinforcement from eating, relief from negative states resulting from eating, obsession over food or lack of control over overeating, and craving as a physiological state. Subjects had to evaluate each statement on a 5-point scale ranging from strongly disagree = 1 to strongly agree = 5. The reported Cronbach’s alpha is 0.94 (Cepeda-Benito et al., 2000).
We selected The Body Appreciation Scale-2 (BAS-2) questionnaire (Tylka and Wood-Barcalow, 2015), a revised version of the Body Appreciation Scale (BAS) (Avalos et al., 2005), to measure personal appreciation and positive attitude toward one’s body. It consists of 10 statements, which subjects had to rate on a scale ranging from 1 = never to 5 = always, measuring dimensions like body esteem, body surveillance, body shame, and psychological wellbeing. Higher scores indicate higher body appreciation. A review of body image measures reported strong internal consistency for BAS-2, with nearly all studies reporting Cronbach’s alpha ≥0.70 (Kling et al., 2019). It significantly correlated with other body image and well-being measures like self-esteem (Kling et al., 2019).
We used a revised version of the Janis & Field Feelings of Inadequacy (Janis and Field, 1959; Fleming and Courtney, 1984). It comprises 36 items, measuring general self-esteem where participants reply choosing from a scale ranging from very often/confident = 1 to not at all often/confident = 5. Higher scores reveal higher self-esteem. Measured factors include self-regard, social confidence, school abilities, physical appearance, and physical abilities. The reliability estimate is 0.91 (Fleming and Courtney, 1984). It is suitable for examining multiple components of self-esteem (Heatherton and Wyland, 2003). For ease, we will refer to this as SE-T (self-esteem trait).
The State Self-Esteem Scale (SSES) measures momentary self-esteem fluctuations. The questionnaire comprises 20 statements scored on a 5-point scale (not at all = 1 to extremely = 5), measuring three dimensions: performance, social, and appearance (Hetherton and Polivy, 1991). Higher scores imply higher self-esteem. We chose this questionnaire, expecting it would be more sensitive to immediate changes resulting from the stimulation, complying with the state FCQ (Cepeda-Benito et al., 2000). The coefficient alpha is 0.92 (Hetherton and Polivy, 1991). For ease, we will refer to this as SE-s (self-esteem state).
The questionnaires mentioned above and the cognitive tasks were administered online to reduce the amount of physical paper used. In addition, we observed physical distancing, used personal protective equipment such as gloves and masks, and controlled entrance to the campus premises upon proof of a negative PCR report and body temperature check. Furthermore, research equipment was disinfection before and after use by following the guidelines for good practice and safety during the COVID-19 pandemic (Bikson et al., 2020).
After completing the initial inclusion questionnaire online, accepted participants were invited to the lab for 5 consecutive days, 2–3 hours after their last meal. On the first day, they signed the informed consent, were screened for inclusion criteria, filled the batteries of questionnaires, and completed the Go/No-go task. They then received tDCS stimulation, and repeated the questionnaires and the Go/No-go task. They also indicated stimulation after-effects on a scale from 1 to 10 for dimensions like headache, neck pain, back pain, blurred vision, scalp irritation, tingling, itching, burning sensation, dizziness, acute mood change, increased heart rate, and anxiety. Finally, they had to reveal whether they believed they were receiving the active or sham stimulation. The protocol was repeated on the last day, day 5. On the other 3 days, tDCS was administered, and the adverse effects and blinding effectiveness were measured. Stimulation was administered for 10-min, followed by a 10-min break, and concluded with another 10-min stimulation. Participants were given a mindfulness script which they had to read and think about during the 10-min break. This was done to obtain standardization over all the groups. Upon completion of the study, participants were debriefed and received a token of appreciation.
Analysis was performed using IBM® SPSS® software. For the main analysis, we chose Generalized Estimating Equation (GEE). This method is adequate for non-normally distributed data and is robust against choosing the wrong correlation structure (Hubbard et al., 2010). The predictors were stimulation, modeled as a factor, and time and baseline as the covariates for each measured outcome (FCQ-T, FCQ-S, SSES, SE-T, BAS-2, FA). The SHAM-group data were used as a reference in all analyses. We computed the main effects and two-way and three-way interaction between all the predictors to better understand if outcomes would change depending on baseline values. As data were not normally distributed, we chose Gamma with Log link. Alpha level was set at 0.05.
Where the Shapiro-Wilks test revealed non-normally distributed data, we adopted the Kruskal-Wallis H test or Fisher’s exact test. Otherwise, we used one-way ANOVA to compare groups. There was no difference in terms of age [X2(2) = 3.249, p = 0.197], BMI [F(2, 26) = 0.230, p = 0.796], gender (Fisher’s exact test, p = 0.452), or baseline as shown in Table 1. The only exception was the inhibition FA, where baseline values were significantly different between groups [F(2, 26) = 6.056, p = 0.007]. For this reason, we choose to enter baseline as a moderator in all the analyses.
It was a single-blind study where the experimenter was aware of the stimulation group, but the participants were not. Most participants guessed that they were in the active group, and their guesses did not differ between groups x2(2) = 4.988, p = 0.083. In the SHAM-group, 68% guessed wrong. Thus, blinding was effective for this group. Overall, 65% guessed right, while 34% guessed wrong over all the groups.
For each side effect, we calculated a mean score of the ratings over the 5 days. Subsequently, we conducted the Independent-Samples Kruskal-Wallis Test since data were not normally distributed. Groups did not differ in adverse effects, which were mild (not greater than 3.8 out of 10).
For trait craving, raw data showed a decrease in all groups across days (Table 2). However, the effect was insignificant (Wald X2 = 5.235, p = 0.073), as shown in Table 3.
Table 2. Craving, self-esteem, body appreciation questionnaires scores as well as results of Go/No-go task.
For the craving state, there was a decreasing trend in craving state scores in the DLPFC-group, while in the IPL-group, there was an increase. In the SHAM-group, the scores initially increased and then decreased. GEE revealed an effect of stimulation (Wald X2 = 8.301, p = 0.016), baseline (Wald X2 = 4.063, p = 0.044) as well as an interaction between stimulation and baseline (Wald X2 = 8.939, p = 0.011). Post-hoc tests did not detect any significance.
Regarding self-esteem trait, the raw data showed a general increase in time across groups. GEE analysis revealed that main effect of baseline (Wald X2 = 4.967, p = 0.026) and effect of both DLPFC (Wald X2 = 8.319, p = 0.004) and IPL (Wald X2 = 5.100, p = 0.024), with a decrease in scores of self-esteem compared to the SHAM. However when we looked at interactions of DLPFC with time (Wald X2 = 63,213, p = 0.013), and IPL with time (Wald X2 = 4.594, p = 0.032) as well as the interaction of DLPFC with baseline (Wald X2 = 9.892, p = 0.002) and IPL with baseline (Wald X2 = 6.827, p = 0.009) there was a significant increase in self-esteem trait scores signifying improvement compared to SHAM.
Regarding self-esteem state, descriptive data showed a trend for increasing scores over time in IPL and SHAM but almost no change in DLPFC. In GEE, we found the main decrease in scores of IPL compared to SHAM (Wald X2 = 21.352, p < 0.001), but if we also take into consideration the two-way interaction with time in IPL (Wald X2 = 5.195, p < 0.023) or with baseline, scores of self-esteem in IPL were higher than SHAM (Wald X2 = 14.789, p < 0.001).
Exploratory data regarding body appreciation showed a decrease in DLPFC and an increase in IPL and SHAM. GEE revealed main effect of time (Wald X2 = 7.567, p = 0.006), main effect of baseline (Wald X2 = 73.250, p = 0.000) and decrease of BA scores in IPL (Wald X2 = 7.227, p = 0.007) compared to SHAM. However, in the interaction between IPL and baseline (Wald X2 = 7.833, p = 0.005), there was an improvement in BA compared to SHAM.
Regarding false alarms (FA), exploratory data showed a decrease in errors in DLPFC, an increase in IPL, and almost no change in SHAM as shown in Figure 1. In GEE analysis we found increase of errors across time (Wald X2 = 6.790, p = 0.009), and effect of baseline (Wald X2 = 22.695, p < 0.001), and more errors in DLPFC compared to SHAM (Wald X2 = 5.663, p = 0.017). However, when we looked at two-way interactions between DLPFC and baseline (Wald X2 = 4.343, p = 0.037), there was a decrease in false alarms signifying improvement compared to SHAM.
Figure 1. False alarms (FA) data, measured pre- and post-stimulation on day 1 and day 5, indicating a decrease in the DLPFC-group, an increase in the IPL-group, and almost no change in the SHAM-group.
Effects of Go/No-go task were stronger compared to other outcomes. Paired-samples post-hoc t-test showed that in either DLPFC and IPL there was significant change from day 1 pre-stimulation to day 5 after stimulation [respectively, t(8) = 2.705, p = 0.027 and t(9) = −2.680, p = 0.025], while there was no significant change in SHAM [t(9) = −1.017, p = 0.336].
The present study sought to investigate whether the geography of cortical stimulation sites could be extended beyond the DLPFC to the IPL area, thus underpinning its involvement in orchestrating control of food cravings. Furthermore, to investigate whether the stimulation of these sites alters control of inhibition, self-esteem, body appreciation, and their interactions with cravings.
Concerning the first aim, the effects of tDCS trended regarding the craving trait. These results did not replicate the general effects of DLPFC stimulation on craving (Fregni et al., 2008; Lefaucheur et al., 2017; Ghobadi-Azbari et al., 2022). However, inconclusive results have been reported in literature, possibly due to variation in stimulation paradigms [see Ester and Kullmann, 2021 for a meta-analysis].
We also found a trend for craving state to be reduced in DLPFC-group and increased in the IPL-group. We speculate that this effect can be linked to the negative relation between IPL stimulation and inhibition as opposed to the enhancement of inhibition in the DLPFC-group. In the case of food cravings, the results might not have reached significance due to the low stimulation intensity and the application of a spaced-stimulation protocol. Furthermore, due to the practical inclusion criteria and challenges related to the COVID pandemic, our sample group comprised of moderate craving subjects. Studies have shown that eating behavior traits significantly predict tDCS effectiveness (Beaumont et al., 2022).
Contradicting our hypothesis, the frontal and parietal areas yielded contrary results. We stimulated the right hemispheres based on the assumption that left frontal activity is associated with approach motivation, while right frontal cortical activity is associated with avoidance motivation (Kelley et al., 2017; McGeown and Davis, 2018). Thus we hypothesized that stimulating the right hemisphere could potentially reduce craving. However, several studies have shown opposite activation of the anterior and posterior cortical regions (Morillas-Romero et al., 2013; van Bochove et al., 2016). van Bochove et al. (2016) reported that resting-state EEG was only linked to the hedonic valuation of food in the parieto-occipital sites and not in the anterior areas. This suggests a functional difference in the lateralization, possibly due to the top-down specialization of the frontal areas as opposed to the more bottom-up properties of the parietal regions (Li et al., 2010).
Finally, there might also be a differential effect of cue-induced as opposed to an abstinence-induced craving, i.e., craving elicited spontaneously from refraining from substance consumption. It has been shown that abstinence-induced craving for smoking was correlated with resting-state cerebral blood flow to the right DLPFC and other areas but not to the parietal regions (Wang et al., 2007). This could possibly be due to abnormal thalamus-DLPFC connectivity linked to attentional biases and reward (Wang et al., 2018). In these studies, abstinence was defined by a 12-h gap from smoking, while our protocol included only 2–3 h of abstinence. Nevertheless, it can be assumed that there might be a difference as opposed to a cue-induced craving, even in our case.
Despite the lack of a significant effect on craving, in this study, stimulation of the DLPFC and the IPL yielded effects on other constructs. This seems to imply that craving can be moderately linked to self-esteem, body appreciation, and inhibition since changes in these outcomes did not necessarily reflect changes in craving or changes of similar strength.
However, we noticed a general trend of an inverse relation between IPL stimulation and DLPFC stimulation, i.e., while DLPFC would inhibit, IPL would activate.
Both stocktickerIPL and DLPFC areas were linked to self-esteem trait. This confirms a right hemispheric dominance observed in neural correlates of self-conscious emotions (Devinsky, 2000; Lavarco et al., 2022). Our findings agree with previous research on self-esteem testing through tDCS (Pan et al., 2016; De Raedt et al., 2017). As well as with tDCS studies linking specific prefrontal areas to factors like unfairness concerning the self (Civai et al., 2014), down-regulation of negative emotions (Peña-Gómez et al., 2011) and rumination (Allaert et al., 2021).
Stimulation of the stocktickerIPL did have a beneficial effect on self-esteem state and body appreciation. Studies show that the stocktickerIPL (Uddin et al., 2005) and the Temporoparietal Junction (TPJ) are related to a sense of self, self-consciousness, self-appraisal, and self-esteem (Knyazev et al., 2021). The authors suggest that the right stocktickerIPL might play a role in self-other discrimination through the frontoparietal mirror neuron network (Uddin et al., 2005). Transcranial direct current stimulation (tDCS) over the TPJ has also been related to mental representations of self and other people (for a review, see Sellaro et al., 2016 and references therein). Meanwhile, stimulation of the DLPFC did not affect state self-esteem, a finding more in line with studies showing a negative effect (Jiang et al., 2018). This might explain the contradicting effects found in the literature about the DLPFC and self-esteem, associated with different aspects of self-esteem, namely state or trait.
The self-esteem state seemed not dependent on craving. Changes after tDCS had the same direction and were increased in the IPL-group conditions and decreased or unaltered in the DLPFC-group. Regarding self-esteem and craving traits, there seemed to be a spontaneous improvement in both constructs in all groups, which was significant for self-esteem but insignificant in the case of craving. Body appreciation followed the same pattern as the self-esteem state, i.e., an increase in the IPL-group and a decrease in the DLPFC-group. In summary, this seems to support that self-esteem and body appreciation is independent of craving and reliant on a paradox of other factors. A more robust connection seems to exist in pathological eating behaviors (Pape et al., 2021).
The DLPFC was more involved in inhibition. Stimulation significantly reduced FA, thus improving inhibition performance. This agrees with previous studies regarding the DLPFC and the PFC as the central hub of cognitive control and inhibition (Cieslik et al., 2013; Salehinejad et al., 2017; Maier et al., 2018; Lavarco et al., 2022). Though more pronounced in the DLPFC, there was also a correlation of the IPL with inhibition. However, it was the opposite effect leading to deterioration in performance. Previous studies have linked the frontoparietal network to inhibition (Garavan et al., 1999; Rubia et al., 2001, 2003; Niendam et al., 2012). Functional connectivity studies show that the frontal-parietal regions are involved in a central executive network activity during cognitive tasks (Seeley et al., 2007) and carry out top-down functions. However, in our study, the effect had the opposite direction. Research reported an effect of tDCS stimulation of the parietal cortex on the increase in the number of false recognitions related to memory (Pergolizzi and Chua, 2015). In this study, the authors attributed the effect to a possible reaction of parietal areas to the perceived oldness of a stimulus, irrespective of the accuracy in relation to the task. Other studies show that anodal tDCS stimulation of the right posterior parietal cortex disrupted the processing of a single stimulus in the contralateral field (Filmer et al., 2015), thus linking it with visuospatial attention. Finally, a study showed that during a task with targets and distractors, attention to highly visible non-targets deactivated parietal regions (Farooqui and Manly, 2018). This implies that in our Go/No-go task, the successful completion would rely on deactivating these areas during No-go cues. Since we artificially activated the IPL region through tDCS, we believe this led to more FA and, thus, more errors.
Concerning the craving state, there seemed to be a link to inhibition. The cravings decreased when inhibition improved, as in the DLPFC-group. Meanwhile, in the IPL-group, craving scores increased with an associated deterioration of inhibitory performance. This connection was not found for the craving trait. Since the craving state is a construct that captures the fluctuations of craving, it is more sensitive to stimulation, unlike the craving trait, which is supposed to be more rigid.
However, the lack of a strong connection between craving and inhibition is puzzling. Even though inhibition was significantly improved in the DLPFC-group and impaired in the IPL-group, this did not affect craving. This might be due to the difference between responders and non-responders potentially included in the study. We hypothesize that craving is a more subjective construct, operationalized through self-report, but might arguably be a more rigid construct. Which possibly relies on a more distributed network involving cortical and subcortical components, which could not be influenced by tDCS to the same extent as inhibition. Perhaps, a potentially stronger effect could have been found if the study focused on food-related inhibition and not on a general inhibition concept.
In this study, we applied a spaced stimulation protocol. The initial research which explored the effects of tDCS on craving applied a continuous stimulation protocol, which, although very well tolerated, was occasionally associated with discomfort or minor adverse effects (Matsumoto and Ugawa, 2017). The space stimulation protocol was based on previously reported observations (Monte-Silva et al., 2010, 2013), which indicated that applying a second stimulation while the after-effects of the first were still ongoing enhances the magnitude and duration of the tDCS-induced effects, and more so than merely prolonging the stimulation. Our results showed that a spaced protocol was effective in the case of inhibition. Monte-Silva et al. (2010, 2013) reported that while exploring a spaced protocol, they kept the stimulation intensity at 2 mA. We reduced the intensity to 1.5 mA to test an even milder protocol. Subsequently, our results showed stronger effects in inhibition measured through the Go/No-go task and milder effects in the other constructs, such as self-esteem and body appreciation. This might be because inhibition was measured more objectively through an operational task, while the other constructs were measured through self-report, thus being more prone to the effect of tiredness and boredom, not proper focus, and potential challenges related to language (del Boca and Noll, 2000; Stanton et al., 2002).
Our results provide a promising new avenue that is much easier for sensitive categories such as the elderly or those with a lower tolerance to potentially adverse effects.
There are potential limitations in this study. The study was conducted as a single-blinded, randomized, parallel-group trial, implying that the researcher administering the tDCS was aware of the modality (i.e., active vs. SHAM). This could potentially impact the results. However, a large study combining 146 meta-analyses has shown that biases associated with lack of blinding are more significant in trials with subjectively assessed outcomes as opposed to objective ones (Wood et al., 2008). The majority of the measures in our study were computer-based without any interference from the researchers. Thus, it is highly unlikely that the researcher’s expectations influenced the outcome (Wallace et al., 2016; Yavari et al., 2018).
Furthermore, the data for each participant were coded for the analysis, removing potential biases. It should also be noted that adequate blinding in tDCS experiments is also affected by other factors related to stimulation that inevitably give the experimenters access to the study group. These are related to skin redness because of stimulation, which has been shown to compromise the adequate blinding of participants (Woods et al., 2016) and the need for continuous monitoring of impedances to ensure effective stimulation. Finally, considering safety measures during the ongoing pandemic, we felt it safer to limit the participant’s contact with multiple researchers.
Another limitation may be related to the fact that despite our attempts to recruit a more inclusive cohort, our sample was comprised mainly of students and their relatives. We cannot discern to what extent it is representative of the population. We also did not explore the long-term effects (preceding 1 month) of tDCS or control factors like boredom.
It should also be noted that the absence of significant tDCS effects on craving may be related to other sources of variability often neglected in the literature (Vergallito et al., 2018) like stable and variable inter-individual differences, such as morphological, genetic, and hormonal features which may partially account for the heterogeneity of responses (i.e., responders vs. non-responders).
To our knowledge, this is the first study where a spaced protocol has been used. It was still effective despite being less “invasive.” Higher doses, higher current densities, and higher stimulation periods have been associated with effects of larger magnitude and duration (Brunoni et al., 2012), which can be associated with skin problems in some cases (Matsumoto and Ugawa, 2017). Decreasing the current might maintain or reduce theoretical risks (Bikson et al., 2016). It would be interesting to apply the same protocol to other operational tasks with established effects in the literature. To the best of our knowledge, this is the first time that outcomes such as self-esteem and body appreciation are studied in relation to the craving, examining the tDCS effects and aiding a better understanding of the complex construct such as craving.
Furthermore, it would be interesting to replicate the study with stronger parameters of tDCS like a 2 mA intensity or rTMS to explore these subjective dimensions. Alternatively, future studies could try and operationalize the same constructs differently rather than through questionnaires. Also, a direct comparison between this kind of stimulation and a continuous one might be informative. Finally, we believe that the results of this study prompt further exploration of the involvement of the DLPFC and the IPL in inhibition and to better understand the dynamics of the frontoparietal networks.
The original contributions presented in the study are included in the article/supplementary material, further inquiries can be directed to the corresponding author.
The studies involving human participants were reviewed and approved by the HERTC (Human Ethics at UAEU–ERH-2019-5910). The patients/participants provided their written informed consent to participate in this study.
ML contributed to the conception and design of the study, and wrote the manuscript. JB collected the data and performed the statistical analysis. FI supervised the findings of this work. All authors contributed to manuscript revision, read and approved the submitted version.
This study was supported by CMHS UAEU grant G00003397 (31M469).
We thank Fransina Christina King for writing assistance and proofreading that greatly improved the manuscript.
The authors declare that the research was conducted in the absence of any commercial or financial relationships that could be construed as a potential conflict of interest.
All claims expressed in this article are solely those of the authors and do not necessarily represent those of their affiliated organizations, or those of the publisher, the editors and the reviewers. Any product that may be evaluated in this article, or claim that may be made by its manufacturer, is not guaranteed or endorsed by the publisher.
Agroskin, D., Klackl, J., and Jonas, E. (2014). The self-liking brain: A VBM study on the structural substrate of self-esteem. PLoS One 9:e86430. doi: 10.1371/journal.pone.0086430
Allaert, J., de Raedt, R., van der Veen, F. M., Baeken, C., and Vanderhasselt, M. A. (2021). Prefrontal tDCS attenuates counterfactual thinking in female individuals prone to self-critical rumination. Sci. Rep. 11:11601. doi: 10.1038/s41598-021-90677-7
Alonso-Alonso, M., and Pascual-Leone, A. (2007). The right brain hypothesis for obesity. JAMA 297, 1819–1822.
Avalos, L., Tylka, T. L., and Wood-Barcalow, N. (2005). The body appreciation scale: Development and psychometric evaluation. Body Image 2, 285–297. doi: 10.1016/j.bodyim.2005.06.002
Barbey, A. K., Colom, R., Solomon, J., Krueger, F., Forbes, C., and Grafman, J. (2012). An integrative architecture for general intelligence and executive function revealed by lesion mapping. Brain 135, 1154–1164. doi: 10.1093/brain/aws021
Bari, A., and Robbins, T. W. (2013). Inhibition and impulsivity: Behavioral and neural basis of response control. Prog. Neurobiol. 108, 44–79. doi: 10.1016/j.pneurobio.2013.06.005
Beaumont, J. D., Smith, N. C., Starr, D., Davis, D., Dalton, M., Nowicky, A., et al. (2022). Modulating eating behavior with transcranial direct current stimulation (tDCS): A systematic literature review on the impact of eating behavior traits. Obes. Rev. 23:e13364. doi: 10.1111/obr.13364
Beaver, J. D., Lawrence, A. D., van Ditzhuijzen, J., Davis, M. H., Woods, A., and Calder, A. J. (2006). Individual differences in reward drive predict neural responses to images of food. J. Neurosci. 26, 5160–5166. doi: 10.1523/JNEUROSCI.0350-06.2006
Bezdjian, S., Baker, L. A., Lozano, D. I., and Raine, A. (2009). Assessing inattention and impulsivity in children during the Go/NoGo task. Br. J. Dev. Psychol. 27, 365–383. doi: 10.1348/026151008X314919
Bhutani, S., Vandellen, M. R., and Cooper, J. A. (2021). Longitudinal weight gain and related risk behaviors during the covid-19 pandemic in adults in the us. Nutrients 13:671. doi: 10.3390/nu13020671
Bikson, M., Grossman, P., Thomas, C., Zannou, A. L., Jiang, J., Adnan, T., et al. (2016). Safety of transcranial direct current stimulation: Evidence based update 2016. Brain Stimul. 9, 641–661. doi: 10.1016/j.brs.2016.06.004
Bikson, M., Hanlon, C. A., Woods, A. J., Gillick, B. T., Charvet, L., Lamm, C., et al. (2020). Guidelines for TMS/tES clinical services and research through the COVID-19 pandemic. Brain Stimul. 13, 1124–1149. doi: 10.1016/j.brs.2020.05.010
Boes, A. D., Kelly, M. S., Trapp, N. T., Stern, A. P., Press, D. Z., and Pascual-Leone, A. (2018). Noninvasive brain stimulation: Challenges and opportunities for a new clinical specialty. J. Neuropsychiatry Clin. Neurosci. 30, 173–179. doi: 10.1176/appi.neuropsych.17110262
Boswell, R. G., and Kober, H. (2016). Food cue reactivity and craving predict eating and weight gain: A meta-analytic review. Obes. Rev. 17, 159–177. doi: 10.1111/obr.12354
Brunoni, A. R., Moffa, A. H., Fregni, F., Palm, U., Padberg, F., Blumberger, D. M., et al. (2016). Transcranial direct current stimulation for acute major depressive episodes: Meta-analysis of individual patient data. Br. J. Psychiatry 208, 522–531. doi: 10.1192/bjp.bp.115.164715
Brunoni, A. R., Nitsche, M. A., Bolognini, N., Bikson, M., Wagner, T., Merabet, L., et al. (2012). Clinical research with transcranial direct current stimulation (tDCS): Challenges and future directions. Brain Stimul. 5, 175–195. doi: 10.1016/j.brs.2011.03.002
Carr, M. M., Wiedemann, A. A., Macdonald-Gagnon, G., and Potenza, M. N. (2021). Impulsivity and compulsivity in binge eating disorder: A systematic review of behavioral studies. Prog. Neuropsychopharmacol. Biol. Psychiatry 110:110318. doi: 10.1016/j.pnpbp.2021.110318
Cepeda-Benito, A., Gleaves, D. H., Williams, T. L., and Erath, S. A. (2000). The development and validation of the state and trait food-cravings questionnaires. Behav. Ther. 31, 151–173. doi: 10.1016/S0005-7894(00)80009-X
Chao, A. M., Grilo, C. M., and Sinha, R. (2016). Food cravings, binge eating, and eating disorder psychopathology: Exploring the moderating roles of gender and race. Eat. Behav. 21, 41–47. doi: 10.1016/j.eatbeh.2015.12.007
Cieslik, E. C., Zilles, K., Caspers, S., Roski, C., Kellermann, T. S., Jakobs, O., et al. (2013). Is there one DLPFC in cognitive action control? evidence for heterogeneity from Co-activation-based parcellation. Cereb. Cortex 23, 2677–2689. doi: 10.1093/cercor/bhs256
Civai, C., Miniussi, C., and Rumiati, R. I. (2014). Medial prefrontal cortex reacts to unfairness if this damages the self: A tDCS study. Soc. Cogn. Affect. Neurosci. 10, 1054–1060. doi: 10.1093/scan/nsu154
Cooper, J. L., and Wade, T. D. (2015). The relationship between memory and interpretation biases. Cognit. Ther. Res. 39, 853–862. doi: 10.1007/s10608-015-9709-1
Coulborn, S., Bowman, H., Miall, R. C., and Fernández-Espejo, D. (2020). Effect of tDCS over the right inferior parietal lobule on mind-wandering propensity. Front. Hum. Neurosci. 14:230. doi: 10.3389/fnhum.2020.00230
Davey, C. G., Pujol, J., and Harrison, B. J. (2016). Mapping the self in the brain’s default mode network. Neuroimage 132, 390–397. doi: 10.1016/j.neuroimage.2016.02.022
Davis, C., Curtis, C., Levitan, R. D., Carter, J. C., Kaplan, A. S., and Kennedy, J. L. (2011). Evidence that “food addiction” is a valid phenotype of obesity. Appetite 57, 711–717. doi: 10.1016/j.appet.2011.08.017
de Boer, N. S., Schluter, R. S., Daams, J. G., van der Werf, Y. D., Goudriaan, A. E., and van Holst, R. J. (2021). The effect of non-invasive brain stimulation on executive functioning in healthy controls: A systematic review and meta-analysis. Neurosci. Biobehav. Rev. 125, 122–147. doi: 10.1016/j.neubiorev.2021.01.013
De Raedt, R., Remue, J., Loeys, T., Hooley, J. M., and Baeken, C. (2017). The effect of transcranial direct current stimulation of the prefrontal cortex on implicit self-esteem is mediated by rumination after criticism. Behav. Res. Ther. 99, 138–146. doi: 10.1016/j.brat.2017.10.009
del Boca, F. K., and Noll, J. A. (2000). Truth or consequences: The validity of self-report data in health services research on addictions. Addiction 95, 347–360. doi: 10.1080/09652140020004278
Delahoy, R., Davey, C. G., Jamieson, A. J., Finlayson-Short, L., Savage, H. S., Steward, T., et al. (2022). Modulation of the brain’s core-self network by self-appraisal processes. Neuroimage 251:118980. doi: 10.1016/j.neuroimage.2022.118980
Devinsky, O. (2000). Right cerebral hemisphere dominance for a sense of corporeal and emotional self. Epilepsy Behav. 1, 60–73. doi: 10.1006/ebeh.2000.0025
Di, X., and Biswal, B. B. (2014). Identifying the default mode network structure using dynamic causal modeling on resting-state functional magnetic resonance imaging. Neuroimage 86, 53–59. doi: 10.1016/j.neuroimage.2013.07.071
Dosenbach, F., Fair, N. U., Miezin, D. A., Cohen, F. M., Wenger, A. L., and Dosenbach, K. K. T. (2007). Distinct brain networks for adaptive and stable task control in humans. Proc. Natl. Acad. Sci.U.S.A. 104, 11073–11078.
Due, D. L., Huettel, S. A., Hall, W. G., and Rubin, D. C. (2002). Article activation in mesolimbic and visuospatial neural circuits elicited by smoking cues: Evidence from functional magnetic resonance imaging. Am. J. Psychiatry 159, 954–960. doi: 10.1176/appi.ajp.159.6.954
Erdfelder, E., FAul, F., Buchner, A., and Lang, A. G. (2009). Statistical power analyses using G*Power 3.1: Tests for correlation and regression analyses. Behav. Res. Methods 41, 1149–1160. doi: 10.3758/BRM.41.4.1149
Ester, T., and Kullmann, S. (2021). Neurobiological regulation of eating behavior: Evidence based on non-invasive brain stimulation. Rev. Endocr. Metab. Disord. 23, 753–772. doi: 10.1007/s11154-021-09697-3
Ester, T., and Kullmann, S. (2022). Neurobiological regulation of eating behavior: Evidence based on non-invasive brain stimulation. Rev. Endocr. Metab. Disord. 23, 753–772.
Farooqui, A. A., and Manly, T. (2018). When attended and conscious perception deactivates fronto-parietal regions. Cortex 107, 166–179. doi: 10.1016/j.cortex.2017.09.004
Filmer, H. L., Dux, P. E., and Mattingley, J. B. (2015). Dissociable effects of anodal and cathodal tDCS reveal distinct functional roles for right parietal cortex in the detection of single and competing stimuli. Neuropsychologia 74, 120–126. doi: 10.1016/j.neuropsychologia.2015.01.038
Fincham, J. M., Carter, C. S., van Veen, V., Stenger, V. A., and Anderson, J. R. (2001). Neural mechanisms of planning: A computational analysis using event-related fMRI. Proc. Natl. Acad. Sci. U.S.A. 99, 3346–3351. doi: 10.1073/pnas.052703399
Fitzgerald, P. B., Maller, J. J., Hoy, K. E., Thomson, R., and Daskalakis, Z. J. (2009). Exploring the optimal site for the localization of dorsolateral prefrontal cortex in brain stimulation experiments. Brain Stimul. 2, 234–237. doi: 10.1016/j.brs.2009.03.002
Fleming, J. S., and Courtney, B. E. (1984). The dimensionality of self-esteem: II. hierarchical facet model for revised measurement scales. J. Pers. Soc. Psychol. 46, 404–421.
Forcano, L., Mata, F., de la Torre, R., and Verdejo-Garcia, A. (2018). Cognitive and neuromodulation strategies for unhealthy eating and obesity: Systematic review and discussion of neurocognitive mechanisms. Neurosci. Biobehav. Rev. 87, 161–191. doi: 10.1016/j.neubiorev.2018.02.003
Foroni, F., Pergola, G., Argiris, G., and Rumiati, R. I. (2013). The foodcast research image database (FRIDa). Front. Hum. Neurosci. 7:51. doi: 10.3389/fnhum.2013.00051
Fregni, F., Orsati, F., Pedrosa, W., Fecteau, S., Tome, F. A. M., Nitsche, M. A., et al. (2008). Transcranial direct current stimulation of the prefrontal cortex modulates the desire for specific foods. Appetite 51, 34–41. doi: 10.1016/j.appet.2007.09.016
Gagne, D. A., von Holle, A., Brownley, K. A., Runfola, C. D., Hofmeier, S., Branch, K. E., et al. (2012). Eating disorder symptoms and weight and shape concerns in a large web-based convenience sample of women ages 50 and above: Results of the gender and body image (GABI) study. Int. J. Eat. Disord. 45, 832–844. doi: 10.1002/eat.22030
Garavan, H., Pankiewicz, J., Bloom, A., Cho, J.-K., Sperry, L., Thomas Ross, M. J., et al. (2000). Cue-induced cocaine craving: Neuroanatomical specificity for drug users and drug stimuli. Am. J. Psychiatry 157, 1789–1798. doi: 10.1176/appi.ajp.157.11.1789
Garavan, H., Ross, T. J., and Stein, E. A. (1999). Right hemispheric dominance of inhibitory control: An event-related functional MRI study. Psychology 96, 8301–8306.
Ghahremani, D. G., Faulkner, P., Cox, C. M., and London, E. D. (2018). Behavioral and neural markers of cigarette-craving regulation in young-adult smokers during abstinence and after smoking. Neuropsychopharmacology 43, 1616–1622. doi: 10.1038/s41386-018-0019-7
Ghobadi-Azbari, P., Malmir, N., Vartanian, M., Mahdavifar-Khayati, R., Robatmili, S., Hadian, V., et al. (2022). Transcranial direct current stimulation to modulate brain reactivity to food cues in overweight and obese adults: Study protocol for a randomized controlled trial with fMRI (NeuroStim-Obesity). Trials 23:297. doi: 10.1186/s13063-022-06234-8
Giuliani, N. R., and Pfeifer, J. H. (2015). Age-related changes in reappraisal of appetitive cravings during adolescence. Neuroimage 108, 173–181. doi: 10.1016/j.neuroimage.2014.12.037
Gluck, M. E., Alonso-Alonso, M., Piaggi, P., Weise, C. M., Jumpertz-Von Schwartzenberg, R., Reinhardt, M., et al. (2015). Neuromodulation targeted to the prefrontal cortex induces changes in energy intake and weight loss in obesity. Obesity 23, 2149–2156. doi: 10.1002/oby.21313
Gluck, M. E., Viswanath, P., and Stinson, E. J. (2017). Obesity, appetite, and the prefrontal cortex. Curr. Obes. Rep. 6, 380–388. doi: 10.1007/s13679-017-0289-0
Golaszewski, S., Kunz, A., Schwenker, K., Sebastianelli, L., Versace, V., Ferrazzoli, D., et al. (2021). Effects of intermittent theta burst stimulation on the clock drawing test performances in patients with Alzheimer’s disease. Brain Topogr. 34, 461–466. doi: 10.1007/s10548-021-00836-2
Goldman, R. L., Borckardt, J. J., Frohman, H. A., O’Neil, P. M., Madan, A., Campbell, L. K., et al. (2011). Prefrontal cortex transcranial direct current stimulation (tDCS) temporarily reduces food cravings and increases the self-reported ability to resist food in adults with frequent food craving. Appetite 56, 741–746. doi: 10.1016/j.appet.2011.02.013
Goldstein, R. Z., and Volkow, N. D. (2002). Reviews and overviews drug addiction and its underlying neurobiological basis: Neuroimaging evidence for the involvement of the frontal cortex. Am. J. Psychiatry 159, 1642–1652. doi: 10.1176/appi.ajp.159.10.1642
Goldstein, R. Z., and Volkow, N. D. (2011). Dysfunction of the prefrontal cortex in addiction: Neuroimaging findings and clinical implications. Nat. Rev. Neurosci. 12, 652–669. doi: 10.1038/nrn3119
Gomis-Vicent, E., Thoma, V., Turner, J. J. D., Hill, K. P., and Pascual-Leone, A. (2019). Review: Non-invasive brain stimulation in behavioral addictions: Insights from direct comparisons with substance use disorders. Am. J. Addict. 28, 431–454. doi: 10.1111/ajad.12945
Griffiths, L. J., Parsons, T. J., and Hill, A. J. (2010). Self-esteem and quality of life in obese children and adolescents: A systematic review. Int. J. Pediatr. Obes. 5, 282–304. doi: 10.3109/17477160903473697
Han, J. E., Boachie, N., Garcia-Garcia, I., Michaud, A., and Dagher, A. (2018). Neural correlates of dietary self-control in healthy adults: A meta-analysis of functional brain imaging studies. Physiol. Behav. 192, 98–108. doi: 10.1016/j.physbeh.2018.02.037
Heatherton, T. F., and Wyland, C. L. (2003). “Assessing self-esteem,” in Positive psychological assessment: A handbook of models and measures, eds S. J. Lopez and C. R. Snyder (Washington, DC: American Psychological Association), 219–233. doi: 10.1037/10612-014
Herwig, U., Satrapi, P., and Schönfeldt-Lecuona, C. (2003). Using the international 10-20 EEG system for positioning of transcranial magnetic stimulation. Brain Topogr. 16, 95–99. doi: 10.1023/b:brat.0000006333.93597.9d
Hetherton, T. F., and Polivy, J. (1991). Development and validation of a scale for measuring state self-esteem. J. Pers. Soc. Psychol. 60, 895–910.
Hubbard, A. E., Ahern, J., Fleischer, N. L., Laan, M., van der Lippman, S. A., Jewell, N., et al. (2010). To GEE or not to GEE: Comparing population average and mixed models for estimating the associations between neighborhood risk factors and health. Epidemiology 21, 467–474. doi: 10.1097/EDE.0b013e3181caeb90
Igelström, K. M., and Graziano, M. S. A. (2017). The inferior parietal lobule and temporoparietal junction: A network perspective. Neuropsychologia 105, 70–83. doi: 10.1016/j.neuropsychologia.2017.01.001
Janis, I. L., and Field, P. B. (1959). “Sex differences and personality factors related to persuasibility,” in Personality and persuasibility, eds C. I. Hovland and I. L. Janis (New Haven, CT: Yale University Press), 55–68.
Jiang, K., Wu, S., Shi, Z., Liu, M., Peng, M., Shen, Y., et al. (2018). Activations of the dorsolateral prefrontal cortex and thalamus during agentic self-evaluation are negatively associated with trait self-esteem. Brain Res. 1692, 134–141. doi: 10.1016/j.brainres.2018.05.017
Jones, A., Hardman, C. A., Lawrence, N., and Field, M. (2018). Cognitive training as a potential treatment for overweight and obesity: A critical review of the evidence. Appetite 124, 50–67. doi: 10.1016/j.appet.2017.05.032
Kelley, N. J., Hortensius, R., Schutter, D. J. L. G., and Harmon-Jones, E. (2017). The relationship of approach/avoidance motivation and asymmetric frontal cortical activity: A review of studies manipulating frontal asymmetry. Int. J. Psychophysiol. 119, 19–30. doi: 10.1016/j.ijpsycho.2017.03.001
Kling, J., Kwakkenbos, L., Diedrichs, P. C., Rumsey, N., Frisén, A., Brandão, M. P., et al. (2019). Systematic review of body image measures. Body Image 30, 170–211. doi: 10.1016/j.bodyim.2019.06.006
Knoch, D., Pascual-Leone, A., Meyer, K., Treyer, V., and Fehr, E. (2006). Diminishing reciprocal fairness by disrupting the right prefrontal cortex. New Ser. 314, 829–832. doi: 10.1126/science.1129156
Knyazev, G. G., Savostyanov, A. N., Bocharov, A. V., and Rudych, P. D. (2021). How self-appraisal is mediated by the brain. Front. Hum. Neurosci. 15:700046. doi: 10.3389/fnhum.2021.700046
Kolodny, T., Mevorach, C., Stern, P., Biderman, N., Ankaoua, M., Tsafrir, S., et al. (2020). Fronto-parietal engagement in response inhibition is inversely scaled with attention-deficit/hyperactivity disorder symptom severity. NeuroImage Clin. 25:102119. doi: 10.1016/j.nicl.2019.102119
Koob, G. F., and Volkow, N. D. (2010). Neurocircuitry of addiction. Neuropsychopharmacology 35, 217–238. doi: 10.1038/npp.2009.110
Kulkarni, K. R., Schafer, M., Berner, L. A., Fiore, V. G., Heflin, M., Hutchison, K., et al. (2022). An interpretable and predictive connectivity-based neural signature for chronic cannabis use. Biol. Psychiatry Cogn. Neurosci. Neuroimaging 7, 1–11. doi: 10.1016/j.bpsc.2022.04.009
Lavarco, A., Ahmad, N., Archer, Q., Pardillo, M., Castaneda, R. N., Minervini, A., et al. (2022). Self-conscious emotions and the right fronto-temporal and right temporal parietal junction. Brain Sci. 12:138. doi: 10.3390/brainsci12020138
Lefaucheur, J. P., Antal, A., Ayache, S. S., Benninger, D. H., Brunelin, J., Cogiamanian, F., et al. (2017). Evidence-based guidelines on the therapeutic use of transcranial direct current stimulation (tDCS). Clin. Neurophys. 128, 56–92. doi: 10.1016/j.clinph.2016.10.087
Lewer, M., Bauer, A., Hartmann, A. S., and Vocks, S. (2017). Different facets of body image disturbance in binge eating disorder: A review. Nutrients 9:1294. doi: 10.3390/nu9121294
Li, L., Gratton, C., Yao, D., and Knight, R. T. (2010). Role of frontal and parietal cortices in the control of bottom-up and top-down attention in humans. Brain Res. 1344, 173–184. doi: 10.1016/j.brainres.2010.05.016
Ljubisavljevic, M., Maxood, K., Bjekic, J., Oommen, J., and Nagelkerke, N. (2016). Long-term effects of repeated prefrontal cortex transcranial direct current stimulation (tDCS) on food craving in normal and overweight young adults. Brain Stimul. 9, 826–833. doi: 10.1016/j.brs.2016.07.002
Longe, O., Maratos, F. A., Gilbert, P., Evans, G., Volker, F., Rockliff, H., et al. (2010). Having a word with yourself: Neural correlates of self-criticism and self-reassurance. Neuroimage 49, 1849–1856. doi: 10.1016/j.neuroimage.2009.09.019
Maier, M. J., Rosenbaum, D., Haeussinger, F. B., Brüne, M., Enzi, B., Plewnia, C., et al. (2018). Forgiveness and cognitive control – provoking revenge via theta-burst-stimulation of the DLPFC. Neuroimage 183, 769–775. doi: 10.1016/j.neuroimage.2018.08.065
Martin, C. K., McClernon, F. J., Chellino, A., and Correa, J. B. (2011). “Food cravings: A central construct in food intake behavior, weight loss, and the neurobiology of appetitive behavior,” in Handbook of behavior, food and nutrition, eds V. Preedy, R. Watson, and C. Martin (New York, NY: Springer), 741–755.
Matsumoto, H., and Ugawa, Y. (2017). Adverse events of tDCS and tACS: A review. Clin. Neurophysiol. Pract. 2, 19–25. doi: 10.1016/j.cnp.2016.12.003
McClure, S. M., Laibson, D. I., Loewenstein, G., and Cohen, J. D. (2004). Separate neural systems value immediate and delayed monetary rewards. Science 306, 503–507. doi: 10.1126/science.1100907
McGeown, L., and Davis, R. (2018). Frontal EEG asymmetry moderates the association between attentional bias towards food and body mass index. Biol. Psychol. 136, 151–160. doi: 10.1016/j.biopsycho.2018.06.001
McMillan, D. E., and Gilmore-Thomas, K. (1996). Stability of opioid craving over time as measured by visual analog scales. Drug Alcohol Depend. 40, 235–239. doi: 10.1016/0376-8716(96)01218-5
Menon, V., Adleman, N. E., White, C. D., Glover, G. H., and Reiss, A. L. (2001). Error-related brain activation during a Go/NoGo response inhibition taskHum. Brain Mapp. 12, 131–143. doi: 10.1002/1097-0193(200103)12:3<131::aid-hbm1010>3.0.co;2-c
Meule, A., Hermann, T., and Kübler, A. (2014). A short version of the food cravings questionnaire-trait: The FCQ-T-reduced. Front. Psychol. 5:190. doi: 10.3389/fpsyg.2014.00190
Miller, E. K., and Cohen, J. D. (2001). An Integrative theory of prefrontal cortex function. Annu. Rev. Neurosci. 24, 167–202.
Miyake, A., Friedman, N. P., Emerson, M. J., Witzki, A. H., Howerter, A., and Wager, T. D. (2000). The unity and diversity of executive functions and their contributions to complex “frontal lobe” tasks: A latent variable analysis. Cogn. Psychol. 41, 49–100. doi: 10.1006/cogp.1999.0734
Mobbs, O., Iglesias, K., Golay, A., and van der Linden, M. (2011). Cognitive deficits in obese persons with and without binge eating disorder. Investigation using a mental flexibility task. Appetite 57, 263–271. doi: 10.1016/j.appet.2011.04.023
Montenegro, R. A., Okano, A. H., Cunha, F. A., Gurgel, J. L., Fontes, E. B., and Farinatti, P. T. V. (2012). Prefrontal cortex transcranial direct current stimulation associated with aerobic exercise change aspects of appetite sensation in overweight adults. Appetite 58, 333–338. doi: 10.1016/j.appet.2011.11.008
Monte-Silva, K., Kuo, M. F., Hessenthaler, S., Fresnoza, S., Liebetanz, D., Paulus, W., et al. (2013). Induction of late LTP-like plasticity in the human motor cortex by repeated non-invasive brain stimulation. Brain Stimul. 6, 424–432. doi: 10.1016/j.brs.2012.04.011
Monte-Silva, K., Kuo, M. F., Liebetanz, D., Paulus, W., and Nitsche, M. A. (2010). Shaping the optimal repetition interval for cathodal transcranial direct current stimulation (tDCS). J. Neurophysiol. 103, 1735–1740. doi: 10.1152/jn.00924.2009
Morillas-Romero, A., Tortella-Feliu, M., Bornas, X., and Aguayo-Siquier, B. (2013). Resting parietal electroencephalogram asymmetries and self-reported attentional control. Clin. EEG Neurosci. 44, 188–192. doi: 10.1177/1550059412465871
Mostafavi, S. A., Khaleghi, A., Mohammadi, M. R., and Akhondzadeh, S. (2020). Is transcranial direct current stimulation an effective modality in reducing food craving? A systematic review and meta-analysis. Nutr. Neurosci. 23, 55–67. doi: 10.1080/1028415X.2018.1470371
Mueller, S. T., and Piper, B. J. (2014). The psychology experiment building language (PEBL) and PEBL test battery. J. Neurosci. Methods 222, 250–259. doi: 10.1016/J.JNEUMETH.2013.10.024
Niendam, T. A., Laird, A. R., Ray, K. L., Dean, Y. M., Glahn, D. C., and Carter, C. S. (2012). Meta-analytic evidence for a superordinate cognitive control network subserving diverse executive functions. Cogn. Affect. Behav. Neurosci. 12, 241–268. doi: 10.3758/s13415-011-0083-5
Nitsche, M. A., Cohen, L. G., Wassermann, E. M., Priori, A., Lang, N., Antal, A., et al. (2008). Transcranial direct current stimulation: State of the art 2008. Brain Stimul. 1, 206–223. doi: 10.1016/j.brs.2008.06.004
Noori, H. R., Cosa Linan, A., and Spanagel, R. (2016). Largely overlapping neuronal substrates of reactivity to drug, gambling, food and sexual cues: A comprehensive meta-analysis. Eur. Neuropsychopharmacol. 26, 1419–1430. doi: 10.1016/j.euroneuro.2016.06.013
Osada, T., Ohta, S., Ogawa, A., Tanaka, M., Suda, A., Kamagata, K., et al. (2019). An essential role of the intraparietal sulcus in response inhibition predicted by parcellation-based network. J. Neurosci. 39, 2509–2521. doi: 10.1523/JNEUROSCI.2244-18.2019
Ouerfelli-Ethier, J., Salemme, R., Fournet, R., Urquizar, C., Pisella, L., and Khan, A. Z. (2021). Impaired spatial inhibition processes for interhemispheric anti-saccades following dorsal posterior parietal lesions. Cereb. Cortex Commun. 2, 1–15. doi: 10.1093/texcom/tgab054
Pan, W., Liu, C., Yang, Q., Gu, Y., Yin, S., and Chen, A. (2016). The neural basis of trait self-esteem revealed by the amplitude of low-frequency fluctuations and resting state functional connectivity. Soc. Cogn. Affect. Neurosci. 11, 367–376. doi: 10.1093/scan/nsv119
Pape, M., Herpertz, S., Schroeder, S., Seiferth, C., Färber, T., Wolstein, J., et al. (2021). Food addiction and its relationship to weight- and addiction-related psychological parameters in individuals with overweight and obesity. Front. Psychol. 12:736454. doi: 10.3389/fpsyg.2021.736454
Parker, M. N., Tanofsky-Kraff, M., Crosby, R. D., Byrne, M. E., LeMay-Russell, S., Swanson, T. N., et al. (2021). Food cravings and loss-of-control eating in youth: Associations with gonadal hormone concentrations. Int. J. Eat. Disord. 54, 1426–1437. doi: 10.1002/eat.23530
Peña-Gómez, C., Vidal-Piñeiro, D., Clemente, I. C., Pascual-Leone, Á, and Bartrés-Faz, D. (2011). Down-regulation of negative emotional processing by transcranial direct current stimulation: Effects of personality characteristics. PLoS One 6:e22812. doi: 10.1371/journal.pone.0022812
Pergolizzi, D., and Chua, E. F. (2015). Transcranial direct current stimulation (tDCS) of the parietal cortex leads to increased false recognition. Neuropsychologia 66, 88–98. doi: 10.1016/j.neuropsychologia.2014.11.012
Raichle, M. E. (2015). The brain’s default mode network. Annu. Rev. Neurosci. 38, 433–447. doi: 10.1146/annurev-neuro-071013-014030
Rubia, K., Russell, T., Overmeyer, S., Brammer, M. J., Bullmore, E. T., Sharma, T., et al. (2001). Mapping motor inhibition: Conjunctive brain activations across different versions of go/no-go and stop tasks. Neuroimage 13, 250–261. doi: 10.1006/nimg.2000.0685
Rubia, K., Smith, A. B., Brammer, M. J., and Taylor, E. (2003). Right inferior prefrontal cortex mediates response inhibition while mesial prefrontal cortex is responsible for error detection. Neuroimage 20, 351–358. doi: 10.1016/S1053-8119(03)00275-1
Salehinejad, M. A., Ghanavai, E., Rostami, R., and Nejati, V. (2017). Cognitive control dysfunction in emotion dysregulation and psychopathology of major depression (MD): Evidence from transcranial brain stimulation of the dorsolateral prefrontal cortex (DLPFC). J. Affect. Disord. 210, 241–248. doi: 10.1016/j.jad.2016.12.036
Sarkis, R. A., Kaur, N., and Camprodon, J. A. (2014). Transcranial direct current stimulation (tDCS): Modulation of executive function in health and disease. Curr. Behav. Neurosci. Rep. 1, 74–85. doi: 10.1007/s40473-014-0009-y
Saruco, E., and Pleger, B. (2021). A systematic review of obesity and binge eating associated impairment of the cognitive inhibition system. Front. Nutr. 8:609012. doi: 10.3389/fnut.2021.609012
Saxena, V., and Pal, A. (2021). Role of transcranial direct current stimulation in the management of Alzheimer’s disease: A meta-analysis of effects, adherence and adverse effects. Clin. Psychopharmacol. Neurosci. 19, 589–599. doi: 10.9758/CPN.2021.19.4.589
Schacht, J. P., Anton, R. F., and Myrick, H. (2013). Functional neuroimaging studies of alcohol cue reactivity: A quantitative meta-analysis and systematic review. Addict. Biol. 18, 121–133. doi: 10.1111/j.1369-1600.2012.00464.x
Schluter, R. S., Daams, J. G., van Holst, R. J., and Goudriaan, A. E. (2018). Effects of non-invasive neuromodulation on executive and other cognitive functions in addictive disorders: A systematic review. Front. Neurosci. 12:642. doi: 10.3389/fnins.2018.00642
Schutter, D. J. L. G., de Weijer, A. D., Meuwese, J. D. I., Morgan, B., and van Honk, J. (2008). Interrelations between motivational stance, cortical excitability, and the frontal electroencephalogram asymmetry of emotion: A transcranial magnetic stimulation study. Hum. Brain Mapp. 29, 574–580. doi: 10.1002/hbm.20417
Sedgmond, J., Lawrence, N. S., Verbruggen, F., Morrison, S., Chambers, C. D., and Adams, R. C. (2019). Prefrontal brain stimulation during food-related inhibition training: Effects on food craving, food consumption and inhibitory control. R. Soc. Open Sci. 6:181186. doi: 10.1098/rsos.181186
Seeley, W. W., Menon, V., Schatzberg, A. F., Keller, J., Glover, G. H., Kenna, H., et al. (2007). Dissociable intrinsic connectivity networks for salience processing and executive control. J. Neurosci. 27, 2349–2356. doi: 10.1523/JNEUROSCI.5587-06.2007
Sellaro, R., Nitsche, M. A., and Colzato, L. S. (2016). The stimulated social brain: Effects of transcranial direct current stimulation on social cognition. Ann. N Y. Acad. Sci. 1369, 218–239. doi: 10.1111/NYAS.13098
Sridharan, D., Levitin, D. J., and Menon, V. (2008). A critical role for the right fronto-insular cortex in switching between central-executive and default-mode networks. Proc. Natl. Acad. Sci. U.S.A. 105, 12569–12574.
Stanton, J. M., Sinar, E. F., Balzer, W. K., and Smith, P. C. (2002). Issues and strategies for reducing the length of self-report scales. Pers. Psychol. 55, 167–194.
Stopyra, M. A., Friederich, H. C., Lavandier, N., Mönning, E., Bendszus, M., Herzog, W., et al. (2021). Homeostasis and food craving in obesity: A functional MRI study. Int. J. Obes. 45, 2464–2470. doi: 10.1038/s41366-021-00920-4
Strand, M., Fredlund, P., Boldemann, C., and Lager, A. (2021). Body image perception, smoking, alcohol use, indoor tanning, and disordered eating in young and middle-aged adults: Findings from a large population-based Swedish study. BMC Public Health 21:128. doi: 10.1186/s12889-021-10158-4
Takeuchi, N., Sudo, T., Oouchida, Y., Mori, T., and Izumi, S. I. (2019). Synchronous neural oscillation between the right inferior fronto-parietal cortices contributes to body awareness. Front. Hum. Neurosci. 13:330. doi: 10.3389/fnhum.2019.00330
Tylka, T. L., and Wood-Barcalow, N. L. (2015). The body appreciation scale-2: Item refinement and psychometric evaluation. Body Image 12, 53–67. doi: 10.1016/j.bodyim.2014.09.006
Uddin, L. Q., Kaplan, J. T., Molnar-Szakacs, I., Zaidel, E., and Iacoboni, M. (2005). Self-face recognition activates a frontoparietal “mirror” network in the right hemisphere: An event-related fMRI study. Neuroimage 25, 926–935. doi: 10.1016/j.neuroimage.2004.12.018
Vallis, M. (2019). Sustained behaviour change in healthy eating to improve obesity outcomes: It is time to abandon willpower to appreciate wanting. Clin. Obes. 9:e12299. doi: 10.1111/cob.12299
van Bochove, M. E., Ketel, E., Wischnewski, M., Wegman, J., Aarts, E., de Jonge, B., et al. (2016). Posterior resting state EEG asymmetries are associated with hedonic valuation of food. Int. J. Psychophysiol. 110, 40–46. doi: 10.1016/j.ijpsycho.2016.10.006
van den Eynde, F., Guillaume, S., Broadbent, H., Stahl, D., Campbell, I. C., Schmidt, U., et al. (2011). Neurocognition in bulimic eating disorders: A systematic review. Acta Psychiatr. Scand. 124, 120–140. doi: 10.1111/j.1600-0447.2011.01701.x
Vance, D., Fazeli, P., Cody, S., Bell, T., and Northcutt, C. (2016). A description and critical analysis of the therapeutic uses of transcranial direct current stimulation: Implications for clinical practice and research. Res. Rev. 6, 23–31. doi: 10.2147/nrr.s115627
VandenBos, G. R. and American Psychological Association (2015). APA dictionary of psychology, 2nd Edn. Washington, DC: American Psychological Association.
Vergallito, A., Riva, P., Pisoni, A., and Romero Lauro, L. J. (2018). Modulation of negative emotions through anodal tDCS over the right ventrolateral prefrontal cortex. Neuropsychologia 119, 128–135. doi: 10.1016/j.neuropsychologia.2018.07.037
Volkow, N. D., Koob, G. F., and McLellan, A. T. (2016). Neurobiologic advances from the brain disease model of addiction. N. Engl. J. Med. 374, 363–371. doi: 10.1056/nejmra1511480
Wallace, D., Cooper, N. R., Paulmann, S., Fitzgerald, P. B., and Russo, R. (2016). Perceived comfort and blinding efficacy in randomised sham-controlled transcranial direct current stimulation (tDCS) trials at 2 mA in young and older healthy adults. PLoS One 11:e0149703. doi: 10.1371/journal.pone.0149703
Wang, C., Zhang, Y., Yan, C., Sun, M., and Cheng, J. (2018). The thalamo-cortical resting state functional connectivity and abstinence-induced craving in young smokers. Brain Imaging Behav. 12, 1450–1456. doi: 10.1007/s11682-017-9809-5
Wang, Z., Faith, M., Patterson, F., Tang, K., Kerrin, K., Wileyto, E. P., et al. (2007). Neural substrates of abstinence-induced cigarette cravings in chronic smokers. J. Neurosci. 27, 14035–14040. doi: 10.1523/JNEUROSCI.2966-07.2007
Williamson, D. A., Muller, S. L., Reas, D. L., and Thaw, J. M. (1999). Cognitive bias in eating disorders: Implications for theory and treatment. Behav. Modif. 23, 556–577.
Wood, L., Egger, M., Gluud, L. L., Schulz, K. F., Jüni, P., Altman, D. G., et al. (2008). Empirical evidence of bias in treatment effect estimates in controlled trials with different interventions and outcomes: Meta-epidemiological study. BMJ 336, 601–605. doi: 10.1136/bmj.39465.451748.AD
Woods, A. J., Antal, A., Bikson, M., Boggio, P. S., Brunoni, A. R., Celnik, P., et al. (2016). A technical guide to tDCS, and related non-invasive brain stimulation tools. Clin. Neurophysiol. 127, 1031–1048. doi: 10.1016/j.clinph.2015.11.012
Keywords: craving, transcranial direct-current stimulation (tDCS), dorsolateral prefrontal cortex (DLPFC), inhibition, self-esteem, body appreciation, inferior parietal lobule (IPL)
Citation: Ljubisavljevic M, Basha J and Ismail FY (2022) The effects of prefrontal vs. parietal cortex transcranial direct current stimulation on craving, inhibition, and measures of self-esteem. Front. Neurosci. 16:998875. doi: 10.3389/fnins.2022.998875
Received: 20 July 2022; Accepted: 10 October 2022;
Published: 31 October 2022.
Edited by:
Yi Zhang, Xidian University, ChinaReviewed by:
Alessia Gallucci, University of Milano-Bicocca, ItalyCopyright © 2022 Ljubisavljevic, Basha and Ismail. This is an open-access article distributed under the terms of the Creative Commons Attribution License (CC BY). The use, distribution or reproduction in other forums is permitted, provided the original author(s) and the copyright owner(s) are credited and that the original publication in this journal is cited, in accordance with accepted academic practice. No use, distribution or reproduction is permitted which does not comply with these terms.
*Correspondence: Milos Ljubisavljevic, bWlsb3NAdWFldS5hYy5hZQ==
Disclaimer: All claims expressed in this article are solely those of the authors and do not necessarily represent those of their affiliated organizations, or those of the publisher, the editors and the reviewers. Any product that may be evaluated in this article or claim that may be made by its manufacturer is not guaranteed or endorsed by the publisher.
Research integrity at Frontiers
Learn more about the work of our research integrity team to safeguard the quality of each article we publish.