- 1Department of Otorhinolaryngology Head and Neck Surgery, Tianjin First Central Hospital, Tianjin, China
- 2Institute of Otolaryngology of Tianjin, Tianjin, China
- 3Key Laboratory of Auditory Speech and Balance Medicine, Tianjin, China
- 4Key Medical Discipline of Tianjin (Otolaryngology), Tianjin, China
- 5Quality Control Centre of Otolaryngology, Tianjin, China
Objective: The aim of this study was to observe the 3-dimensional (3D; horizontal, vertical, and torsional) characteristics of nystagmus in patients with posterior semicircular canal canalithiasis (PSC-can)–related benign paroxysmal positional vertigo (BPPV) and investigate its correlation with Ewald’s.
Methods: In all, 84 patients with PSC-can were enrolled. The latency, duration, direction, and slow-phase velocity induced by the Dix-Hallpike test in the head-hanging and sitting positions were recorded using 3D video nystagmography (3D-VNG). The characteristics of the horizontal, vertical, and torsional components of nystagmus were quantitatively analyzed.
Results: 3D-VNG showed that the fast phase of the vertical components and torsional components of left and right ear PSC-can as induced by the head-hanging position of the Dix-Hallpike test were upward, clockwise and counterclockwise, and horizontal components were mainly contralateral. The median slow-phase velocity of each of the three components for consecutive 5 s was 26.3°/s (12.3–45.8), 25.0°/s (15.7–38.9), and 9.2°/s (4.9–13.7). When patients were returned to the sitting position, the fast phase of the vertical and torsional components of nystagmus was reversed. Only 54 patients had horizontal components of nystagmus, and 32 of them remained in the same direction. The median slow-phase velocity of the three components for consecutive 5 s was 9.4°/s (6.0–11.7), 6.8°/s (4.5–11.8), and 4.9°/s (2.8–8.0). The ratios of the slow-phase velocity of the horizontal, vertical, and torsional components of the head-hanging position to the sitting position were close to 1.85 (1.0–6.6), 3.7 (1.9–6.6), and 5.1 (2.6–11.3). The ratios of the slow-phase velocity of the vertical to horizontal component, the torsional to horizontal component, and the vertical to torsional component of the head-hanging position were close to 3.3 (1.7–7.6), 3.9 (1.8–7.6), and 1.0 (0.5–1.8). The ratios of the slow-phase velocity of the vertical to horizontal component, the torsional to horizontal component, and the vertical to torsional component of the sitting position were close to 2.1 (1.1–6.8), 1.5 (1.0–3.8), and 1.2 (0.8–2.8).
Conclusion: There were three components of nystagmus induced by the Dix-Hallpike test in patients with PSC-can. The vertical component was the strongest and the horizontal component was the weakest. The 3D characteristics of nystagmus were consistent with those of physiological nystagmus associated with the same PSC with a single-factor stimulus, in accordance with Ewald’s law.
Introduction
Benign paroxysmal positional vertigo (BPPV) is the most common peripheral vestibular disorder, accounting for 17–42% of patients with vertigo (Power et al., 2020; Kim et al., 2021; Palmeri and Kumar, 2022). Posterior semicircular canal (PSC) involvement is common (Shigeno and Kitaoka, 2020). BPPV is a common peripheral vestibular disease, whose basic pathologic process is caused by the migration of otoconia from the utricular macula to the semicircular canals (Bhattacharyya et al., 2017). The canalith repositioning procedure has confirmed this pathological theory. In 1824 (Shimizu, 2000) and 1892 (Baloh and Honrubia, 1979), Flourens and Ewald explored the physiological mechanism of the vestibular semicircle from the 2 levels of the phenomenon and mechanism, respectively, through animal physiological models (Yang et al., 2017). Their findings formed the basis of Flouren’s law and Ewald’s law, Ewald’s Law perfected Flourens Law from mechanism and phenomenon, and contributed greatly to the understanding of human vestibular physiology and pathology (Baloh et al., 1977). Whether this law is completely consistent with human semicircular canal physiology has remained difficult to verify in the human body for quite some time. In the horizontal semicircular canal canalithiasis/cupulolithiasis, posterior/anterior semicircular canal canalithiasis/cupulolithiasis, or in the roll test left/right head turning and Dix-Hallpike Test head-hanging/sitting position, otoliths are diagnosed and localized by inducing excitatory or inhibitory effects under the action of a single stimulant, a specific otoconia. Therefore, similar to horizontal semicircular canal BPPV (HSC-BPPV) (Zhang et al., 2021), PSC-BPPV also objectively provides a physiologically effective model for understanding and studying the response effect of single semicircular canal stimulation under the action of a “single factor.” BPPV is not so much a disease as a physiological reaction of the vestibular semicircular canal to physiological properties. Through BPPV and positional testing, Flourens and Ewald’s laws from animal physiological models were finally interpreted in relation to the human body. Therefore, BPPV is also the best way to understand human semicircular canal physiology and the semicircular canal nystagmus effect, helping to analyze the functional characteristics of the human semicircular canal and its nystagmus effect.
Currently, the diagnosis of PSC canalithiasis (PSC-can) is mainly based on the naked eye observation of the vertical and torsional components of nystagmus induced by the Dix-Hallpike test (Brevern et al., 2017). The application of traditional videonystagmography (VNG) makes the analysis of BPPV nystagmus and the localization of otolith localization from subjective to objective, but the torsion component of PSC could not be observed (Xu et al., 2019; Zhang et al., 2021). Three dimensional (3D)-VNG can accurately quantify the horizontal, vertical, and torsional components of PSC-can nystagmus, but there were few quantitative studies of 3D-VNG for PSC-can (Fetter and Sievering, 1995a,b; Imai et al., 2009; Ichijo, 2013). In this study, 3D-VNG was used to record and quantitatively analyze the horizontal, vertical, and torsional components of nystagmus induced by the Dix-Hallpike test in patients with PSC-can. Furthermore, we explored the correlation between the nystagmus of PSC-can and Ewald’s law. We hope the findings can contribute to the study of the physiological characteristics of the posterior semicircular canal and make the diagnosis of PSC-can more objective.
Materials and methods
Participants
This was an observational study involving the assessment of 84 patients with PSC-can who were examined at the Ear, Nose, and Throat (ENT) Department of the Tianjin First Central Hospital between September 2021 and January 2022. All of the patients had successfully been treated with the canalith repositioning procedure (CRP), and all provided informed consent prior to their inclusion in the study. The study procedures were approved by the Ethics Committee of the Tianjin First Central Hospital (number: 2020N118KY).
The inclusion criteria were the following: a history of positional vertigo as a predominant symptom, accompanied by autonomic symptoms such as nausea and vomiting; and a diagnosis of PSC-can according to the Bárány guidelines (Brevern et al., 2017) with successful repositioning. Meanwhile, the exclusion criteria were the following: horizontal semicircular canal benign paroxysmal positional vertigo (HSC-BPPV), anterior semicircular canal benign paroxysmal positional vertigo (ASC-BPPV), multiple-canal BPPV, or cupulolithiasis; and other types of peripheral and central vertigo, as identified on neurological and imaging examinations. Vestibular migraine was identified and excluded according to the International vestibular Migraine Guidelines.
Methods
The Dix-Hallpike test was performed to diagnose PSC-can by 3D-VNG (VertiGoggles-M, ZEHNIT Medical Technology-VNG-II, Shanghai, China). The directional characteristics of the torsional and vertical components of nystagmus induced in the left and right head-hanging positions and the sitting position were observed by monitor. The latency, duration, direction, and slow-phase velocity of the horizontal, vertical, and torsional components were simultaneously recorded by 3D-VNG. During the Dix-Hallpike test, the patient’s eyes should remain directly in front of the face (i.e., the eyes should remain in place at all times). For the Dix-Hallpike test, the patient’s eyes should be kept directly in front of the face (i.e., in place at all times).
Based on the Bárány diagnosis and treatment guidelines (Brevern et al., 2015, 2017), from a doctor’s perspective, using the apex of the eyeball as a marker and the patient as a reference, the monitor observed vertical upward nystagmus with a torsional component (i.e., the vertical component was marked by upward movement, and the torsional component was marked by the apex of the eyeball turning to the lower ear) when the affected ear at the head-hanging position was turned to the ground in the Dix-Hallpike test. That is, right (R)-PSC-can induced right torsional nystagmus (counterclockwise), and left (L)-PSC-can induced left torsional nystagmus (clockwise). After the patient had returned to the sitting position, the fast phase of nystagmus was reversed. Therefore, on the 3D-VNG diagram, the left/right horizontal nystagmuses were recorded as downward/upward fast phase nystagmus curves, the downward/upward vertical nystagmuses were recorded in the same direction as the real nystagmus, and the clockwise/counterclockwise torsional nystagmuses were recorded as downward/upward fast phase nystagmus curves (Figures 1, 2). Latency time was recorded as the period from the end of a head turn (in the head-hanging or sitting position) to the onset of continuous nystagmus. Duration was recorded as the period of continuous nystagmus, from its onset to the end. Based on the nystagmus duration of <10 s for some patients, the analysis of slow-phase velocity included the average slow-phase velocity of 5 s, 10 s, and the entire duration. The roll test was used to exclude HSC-BPPV and multiple-canal BPPV.
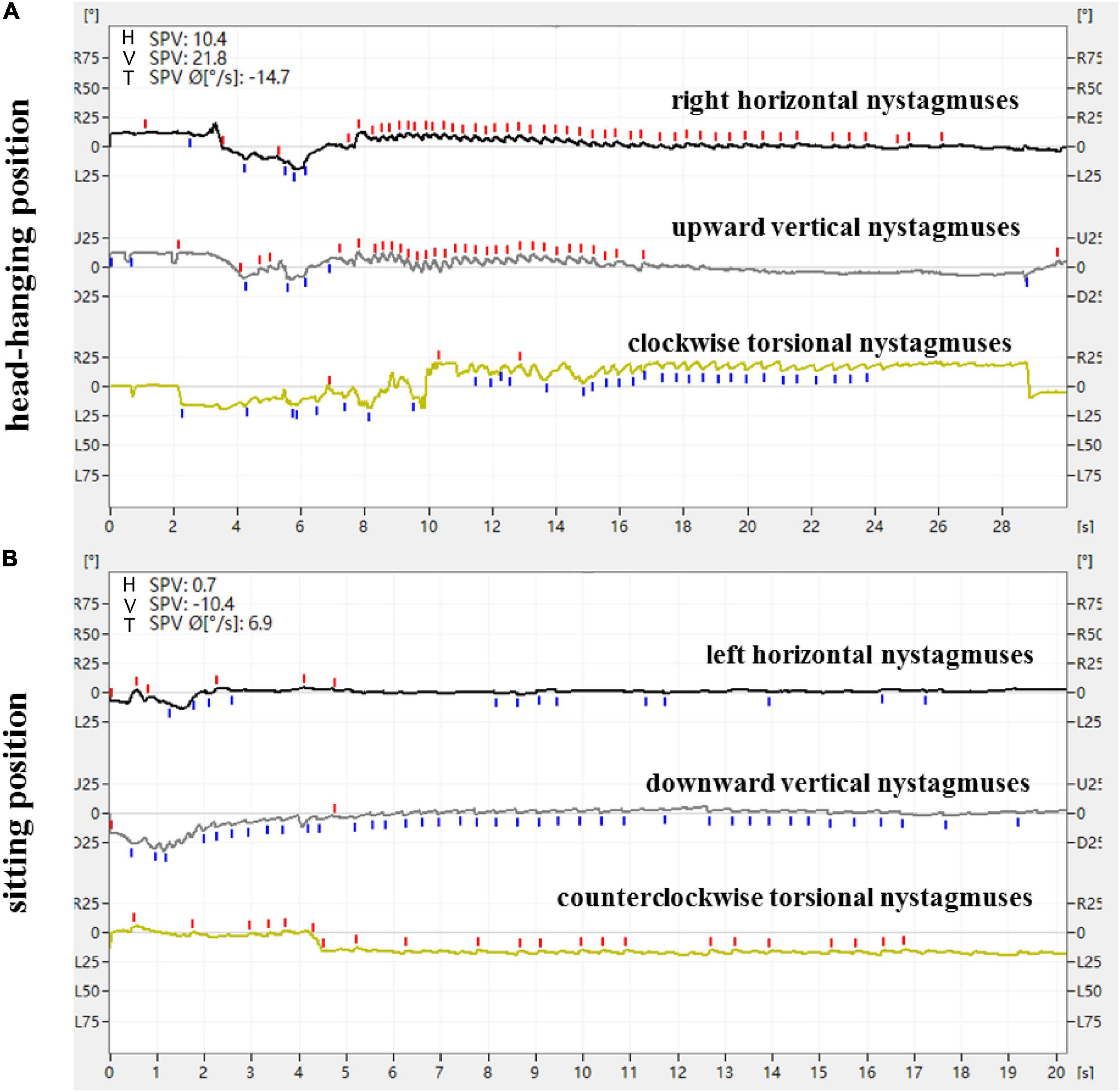
Figure 1. Expression of nystagmus on the 3D-VNG by Dix-Hallpike test of a patient with L-PSC-can. (A) At the head-hanging position, the right horizontal nystagmuses were recorded as upward fast-phase nystagmus curves, the upward vertical nystagmuses were recorded as upward fast-phase nystagmus curves, and the clockwise torsional nystagmuses were recorded as downward fast-phase nystagmus curves. (B) The direction of nystagmus was reversed when the head was recovered from the head-hanging position to the sitting position.
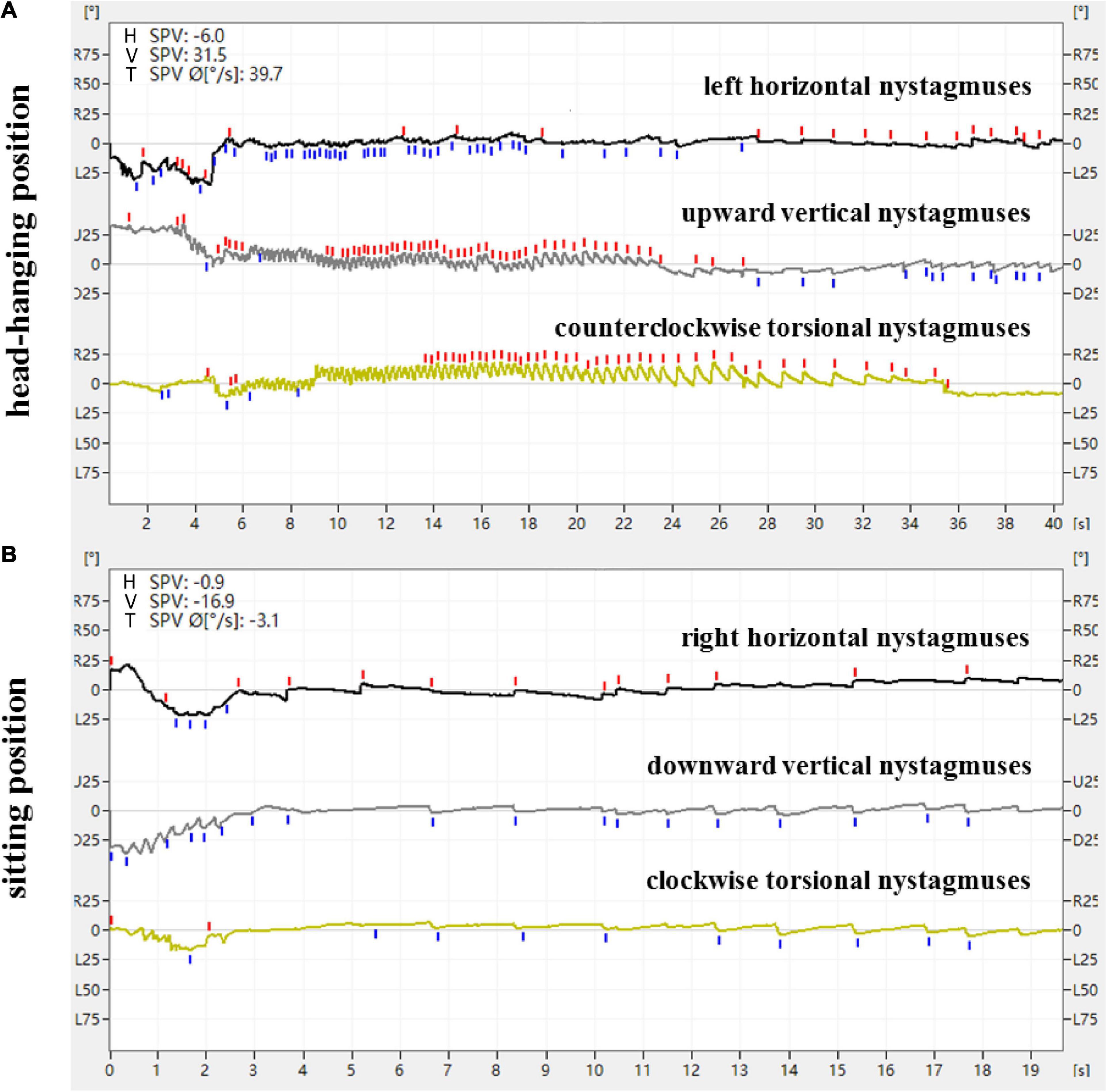
Figure 2. Expression of nystagmus on the 3D-VNG by Dix-Hallpike test of a patient with R-PSC-can. (A) At the head-hanging position, the left horizontal nystagmuses were recorded as downward fast-phase nystagmus curves, the upward vertical nystagmuses were recorded as upward fast-phase nystagmus curves, and the counterclockwise torsional nystagmuses were recorded as upward fast-phase nystagmus curves. (B) The direction of nystagmus was reversed when the head was recovered from the head-hanging position to the sitting position.
Analysis
The parameters of torsional nystagmus induced by the Dix-Hallpike test were compared within and between the groups. Continuous variables are expressed as the means ± SD or medians (interquartile ranges [IQR], Q1-Q3); categorical variables are expressed as frequencies and percentages. Comparisons of means between the two groups were performed using the independent t-test or the Mann-Whitney U test as appropriate. The chi-square test was used to compare proportions in demographics. P-values < 0.05 were considered statistically significant. SPSS Statistics 25 (IBM Corp., Armonk, NY, USA) was used for the statistical analyses, and GraphPad Prism 5 (GraphPad, San Diego, CA, USA) and R scripts were used to generate the figures.
Results
General demographic characteristics of subjects
In all, 84 patients with PSC-can were enrolled in this study, including 29 males and 55 females (age range 25–80 years; mean age 54.7 years). There were 25 patients with L-PSC-can and 59 patients with R-PSC-can. Demographic data for the BPPV patients are summarized in Table 1. The vertigo symptoms of all patients were relieved or disappeared after CRP treatment (Epley, 1992).
The characteristics of nystagmus in patients with PSC-can according to the Dix-Hallpike test
From a doctor’s perspective, with the patient used as the reference and the apex of the eyeball as the marker, the directional characteristics of nystagmus induced by the Dix-Hallpike test in the 84 patients with PSC-can were recorded by nystagmus monitoring and 3D-VNG diagram observation. When the affected ear was tilted to the ground in the head-hanging position of the Dix-Hallpike test, the nystagmus monitors could observe torsional vertical jerk nystagmus (the torsional component turned to the downward ear position, and the vertical component turned to the upward-forehead position), with the duration of nystagmus being <1 min. The fast phase of the torsional component of L-PSC-can and R-PSC-can at the head-hanging position was clockwise and counterclockwise, respectively. The fast phase of nystagmus was reversed when the head was recovered from the head-hanging position to the sitting position, but in only one case did the torsional component remain in the same direction. Under 3D-VNG, the vertical component of nystagmus was recorded, and the clockwise/counterclockwise torsional nystagmuses were recorded as downward/upward fast-phase nystagmus curves. In addition, the horizontal component of nystagmus was also recorded by 3D-VNG in 73 patients with PSC-can, with 63 cases in the contralateral and 10 cases in the ipsilateral (Figures 1, 2). The fast phase of nystagmus was reversed when the patients were returned to the sitting position. The vertical component of nystagmus was observed in 74 patients, the torsional component of nystagmus was observed in 62 patients, and the horizontal component of nystagmus was observed in 54 patients (32 cases were contralateral and 22 cases were ipsilateral).
Under 3D-VNG, 84 patients with PSC-can in the head-hanging position of the Dix-Hallpike test were observed. The median latency and duration of the horizontal component of nystagmus induced by the head-hanging position were 2.0 s (1.0–4.0) and 14.0 s (10.0–21.0), those of the vertical component of nystagmus were 2.0 s (1.0–4.0) and 15.0 s (10.0–19.8), and those the torsional component of nystagmus were 2.0 s (1.0–3.0) and 15.5 s (12.0–21.8), respectively. The median slow-phase velocity of the horizontal component at the entire duration, at the strongest 10 and 5 s were 6.6°/s (3.7–9.4), 7.7°/s (4.0–11), and 9.2°/s (4.9–13.7); those of the vertical component were 19.9°/s (9.8–30.4), 24.5°/s (11.8–36.9), and 26.3°/s (12.3–45.8); and those of the torsion component were 18.3°/s (12.3–26.3), 20.7°/s (13.6–31.6), and 25.0°/s (15.7–38.9), respectively (Table 2). When patients were returned to the sitting position from the head-hanging position, the median latency and duration of the horizontal component of nystagmus were 1.0 s (1.0–2.0) and 15.5 s (12.0–19.3), those of the vertical component of nystagmus were 1.0 s (0.5–2.0) and 16.0 s (11.5–22.3), and those of the torsional component of nystagmus were 1.0 s (0.5–2.0) and 15.0 s (10.0–20.0), respectively. The median slow-phase velocity of the horizontal component at the entire duration, at the strongest 10 and 5 s were 3.5°/s (1.8–4.6), 4.2°/s (2.5–6.6), and 4.9°/s (2.8–8.0); that of the vertical component of nystagmus was 7.2°/s (5.2–9.1), 8.4°/s (6.0–10.5), and 9.4°/s (6.0–11.7); and that of the torsion component of nystagmus was 5.0°/s (3.8–8.9), 5.9°/s (4.0–9.6), and 6.8°/s (4.5–11.8) (Table 3).
The results showed that the vertical and torsional components of nystagmus induced by the Dix-Hallpike test were dominant in PSC-can patients, with the vertical component being the strongest (Figures 3, 4). The fast phase of the horizontal component was mostly contralateral in the head-hanging position, and the fast phase of the horizontal component after the return to the sitting position varied, being either ipsilateral or contralateral. According to the data, the average slow-phase velocity of the consecutive at 5 s was the strongest, so it is suggested that this slow-phase velocity should be used as a standard for quantitative analysis.

Figure 3. Slow-phase velocity of nystagmus in the head-hanging position of patients with PSC-can. The slow-phase velocity of nystagmus differed significantly between the horizontal and vertical components (p < 0.0001), horizontal and torsional components (p < 0.0001). *p < 0.05, **p < 0.01, and ***p < 0.001, compared with respective control.
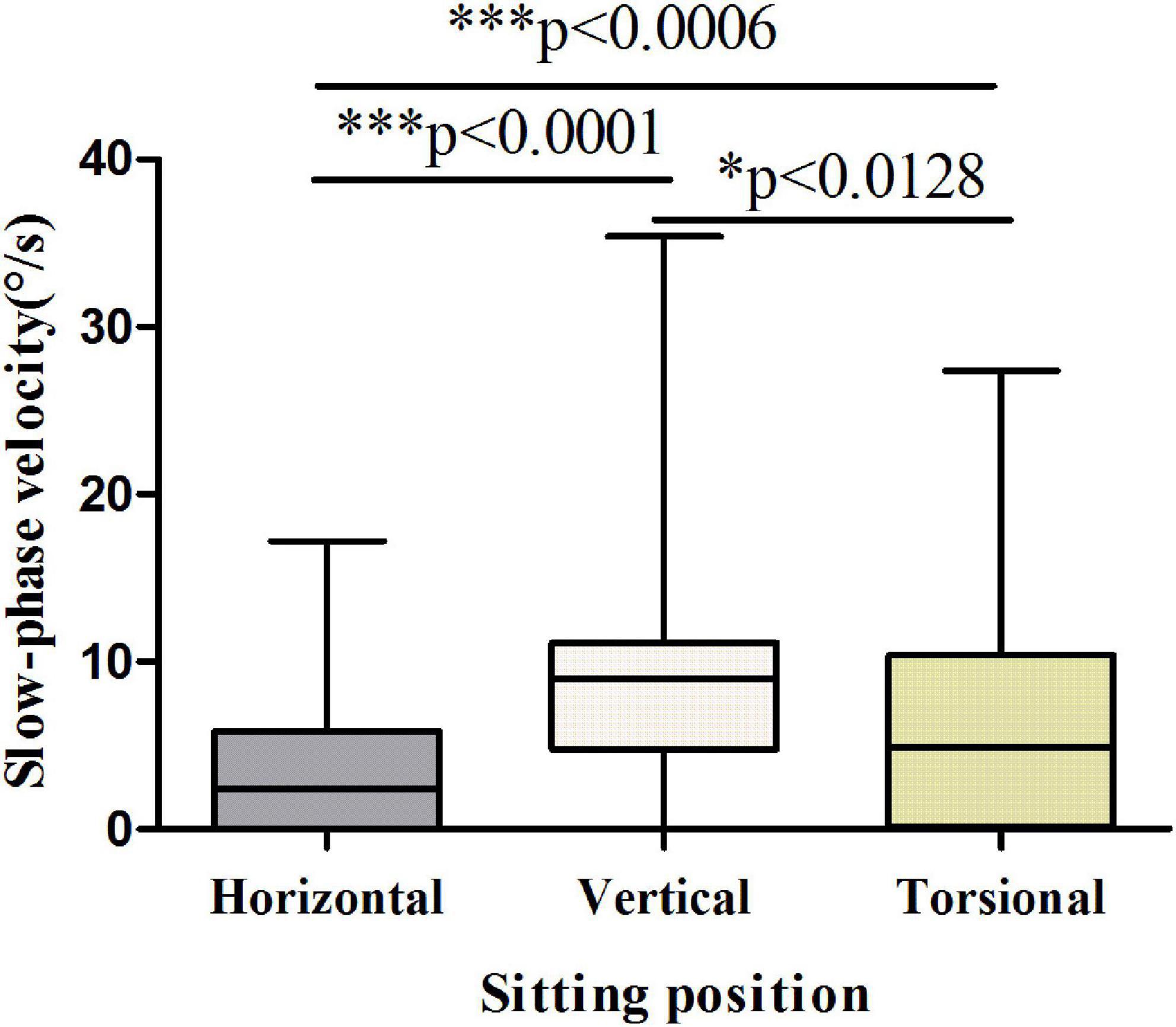
Figure 4. Slow-phase velocity of nystagmus in the sitting position of patients with PSC-can. The slow-phase velocity of nystagmus differed significantly between the horizontal and vertical components (p < 0.0001), horizontal and torsional components (p < 0.0006), vertical and torsional components (p < 0.0128). *p < 0.05, **p < 0.01, and ***p < 0.001, compared with respective control.
Comparison between the head-hanging position and sitting position nystagmus of patients with PSC-can according to the Dix-Hallpike test
The ratio of latency between the head-hanging position and the sitting position was close to 2 (1.0–6.0) (p < 0.001), with the ratio of duration close to 1. The ratios of the slow-phase velocity of the horizontal, vertical, and torsion components of nystagmus for the head-hanging position and the sitting position were close to 1.85 (1.0–6.6), 3.7 (1.9–6.6), and 5.1 (2.6–11.3), respectively (Table 4).

Table 4. Comparison between the head-hanging position and sitting position nystagmus of 84 patients with PSC-can.
The ratio of slow-phase velocity in the head-hanging position and sitting position of patients with PSC-can according to the Dix-Hallpike test
The ratios of slow-phase velocity in the head-hanging position of the vertical to horizontal component, the torsional to horizontal component, and the vertical to torsional component were close to 3.3 (1.7–7.6), 3.9 (1.8–7.6), and 1.0 (0.5–1.8), respectively. The ratios of slow-phase velocity in the sitting position of the vertical to horizontal component, the torsional to horizontal component, and the vertical to torsional component were close to 2.1 (1.1–6.8), 1.5 (1.0–3.8), and 1.2 (0.8–2.8), respectively (Table 5).
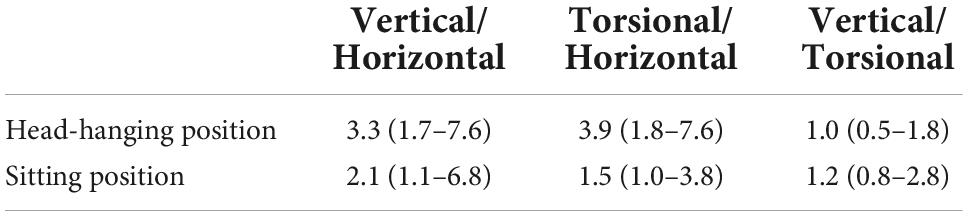
Table 5. The ratio of slow-phase velocity in the head-hanging position and sitting position of 84 patients with PSC-can.
Discussion
According to Flourences and Ewald’s law, the fast phase of nystagmus induced in this study was in the same plane as that of the stimulated semicircular canal. The endolymph in the posterior semicircular canal flows away from the ampulla with excitatory stimulation (Dix and Hallpike, 1952; Honrubia and House, 2001). The posterior semicircular canal is at an angle of about 45° to the sagittal plane, and the position of the ampulla and the common crus were anteriorly inferior and posteriorly superior, respectively. Therefore, if the right posterior semicircular canal was excited under physiological conditions, it is necessary to turn the head to the left and upward. Then, a VOR synthesis vector is generated in which the slow phase eye movement rotates to the lower right, while the fast phase eye movement rotates to the upper left (the direction of head turning is the same as the direction of the induced nystagmus). In physiological state, the stimulation of the right posterior semicircular canal by turning the head to the left and upward was the same as the stimulation of the R-PSC-can in the right Dix-Hallpike test. In 3D-VNG, the horizontal, vertical and torsional components were to the left, upward and counterclockwise, respectively (Wang et al., 2009).
Compared with HSC-BPPV, PSC-BPPV objectively provides a physiological effect model to understand and study the “single semicircular canal stimulation response effect” under the action of “single factor,” but the study of PSC nystagmus mechanism and nystagmus characteristics has been one of the difficulties. In this study, the nystagmus characteristics of 84 patients with PSC-can were analyzed using 3D-VNG. With the patient in the hanging-head position of the Dix-Hallpike test in left ear and right ear PSC-can, the vertical components of nystagmus were upward, the torsional components were clockwise and counterclockwise, respectively, and the horizontal components were mainly contralateral. The median slow-phase velocity of the three components for consecutive 5 s was 26.3°/s (12.3–45.8), 25.0°/s (15.7–38.9) and 9.2°/s (4.9–13.7), respectively. When the patient was returned to the sitting position, the fast phase of the vertical and torsional components of nystagmus was reversed. In only one case the torsional component remained in the same direction. Among 54 patients with horizontal components of nystagmus, 32 patients the fast phase of the nystagmus remained unchanged. The median slow-phase velocity of the three components for consecutive 5 s was 9.4°/s (6.0–11.7), 6.8°/s (4.5–11.8), and 4.9°/s (2.8–8.0), respectively. The fast phase of the vertical and torsional components of nystagmus in patients with PSC-can was basically consistent with that in the BPPV guidelines, but information on the horizontal component of nystagmus was lacking in all of the guidelines. In this study, the horizontal component of nystagmus was not contralateral in 10 patients (13.7%) when the head was at the hanging-head position, and the direction of the horizontal component of nystagmus was not reversed in 32 patients (59.3%) when the head was at the sitting position. The reason leading to this may lies in two aspects: one is the coexistence of other types of vestibular terminal receptor dysfunction; the other is the variation of the anatomy and angle of each individual semicircular canal. For example, the posterior semicircular canal may not be completely perpendicular to the horizontal plane and has a horizontal Angle, resulting in horizontal components in different directions occur at the head-hanging position and sitting position. The 3D-VNG recorded and analyzed the nystagmus of PSC-can patients that was induced by the Dix-Hallpike test, which objectively showed the entirety fast phase of nystagmus, especially, the horizontal component of nystagmus is difficult to see with the naked eye, and more accurately showed the spatial relationship of the posterior semicircular canal plane.
Hiroaki’s (Ichijo, 2013) research results showed that Ewald’s third law is correct in the PSC, and the slow-phase velocity, the head-hanging position and those in the sitting position showed inversion asymmetry. The results of Zhang et al. (2021) showed the fast phase of nystagmus induced by HSC-can to be the same as the direction of head turning, and the slow-phase velocity ratio of the affected side to the healthy side was about 2:1. It has been reported that horizontal semicircular canal BPPV can be used as a single factor in the physiological effect model of a single horizontal semicircular canal stimulation response (Zhang et al., 2021), but no research report on the three-dimensional nystagmosis characteristics of P-BPPV based on this point of view was found. Xu’s (Xu et al., 2019) research results showed that the slow-phase velocity of vertical nystagmus induced by the head-hanging position on the affected side of the PSC-can was significantly greater than that induced by the sitting position, with the ratio of slow-phase velocity being about 2:1. However, the relationship of the slow-phase velocity between each component of PSC-can at two head positions and the relationship of the slow-phase velocity between the three components of each head position have not been reported (Hayashi et al., 2002; Aw et al., 2005; Imai et al., 2009; Eggers et al., 2019). Our results show that the ratios of the slow-phase velocity of the horizontal, vertical, and torsion components between the head-hanging position and sitting position were close to 1.85 (1.0–6.6), 3.7 (1.9–6.6) and 5.1 (2.6–11.3), respectively. The ratios of the slow-phase velocity of the vertical to horizontal component, the torsional to horizontal component, and the vertical to torsional component of the head-hanging position were close to 3.3 (1.7–7.6), 3.9 (1.8–7.6), and 1.0 (0.5–1.8), respectively. The ratios of the slow-phase velocity of the vertical to horizontal component, the torsional to horizontal component, and the vertical to torsional component of the sitting position were close to 2.1 (1.1–6.8), 1.5 (1.0–3.8), and 1.2 (0.8–2.8), respectively. These results suggest that PSC-can is the clinical manifestation of Ewald’s law in the human body. This study not only describes the orientation characteristics of the three components of nystagmus, but also further quantitatively analyzes each component. The results of this study further suggest that the three components of horizontal, vertical and torsional nystagmus induced by PSC-can are the result of the joint action of the PSC with the associated eye muscles dominated by ipsilateral superior/inferior oblique and contralateral superior/inferior rectus, but the weight of each group eye muscles and the interaction mechanism need to be further explored.
The limitation of this study is the absence of any control group. Therefore, normal people and PSC-can patients with unilateral and bilateral vestibular dysfunction should be included in subsequent studies for controlled studies. Because the PSC-can patients in this group did not have a comprehensive vestibular function evaluation, such as the ocular/cervical vestibular evoked myogenic potential (o/cVEMP) evaluation of otolithoid function and multifrequency functional testing of the three semicircular canals, the influence of otolithoid and other frequency functions of the three semicircular canals on nystagmus in PSC-can cannot be ruled out. There are other forms of the horizontal component of PSC-can nystagmus, and whether it can be used as an indication of other semicircular canal or otolith organ injury is worth further study in comprehensive vestibular function assessment. The 3D-VNG can only objectively record the characteristics of nystagmus. The diagnostic and differential diagnostic value of positional vertigo should be further verified by accumulating cases and increasing the time of observation and follow-up.
Conclusion
In this study, 3D-VNG technology was applied to objectively record and analyze the direction, slow-phase velocity, and time parameter characteristics of each component of nystagmus in the head-hanging and sitting positions of patients with PSC-can in the Dix-Hallpike test. BPPV also objectively provides a physiological effect model to understand and study the “response effect of single posterior semicircular canal stimulation” under the action of “single factor on single posterior semicircular canal,” suggesting that PSC-Can is also the embodiment of Ewald’s law of human body. And can be used as a physiological stimulation model to understand the physiological function of the human posterior semicircular canal. In this study, the diagnosis and treatment of PSC-can was measured from naked eye observation to precise quantification, laying a foundation for future objective diagnosis, big data analysis and intelligent analysis of BPPV. At the same time, 3D-VNG can help to understand the mechanism and characteristics of PSC nystagmus.
Data availability statement
The original contributions presented in this study are included in the article/supplementary material, further inquiries can be directed to the corresponding authors.
Ethics statement
This study was reviewed and approved by the Ethics Committee of the Tianjin First Central Hospital (number: 2020N118KY). Written informed consent was obtained from the individual(s) for the publication of any potentially identifiable images or data included in this article.
Author contributions
YL and TC wrote the main manuscript. XZ and QD collected the literature. QL, CW, and WW revised the manuscript. All authors reviewed the manuscript.
Funding
This work was supported by the Tianjin Key Medical Discipline Construction Project (TJYXZDXK-046A), Tianjin Applied Basic Research Multiple Investment Fund Project (21JCQNJC01670, 21JCQNJC01780, 21JCZDJC01200, and 21JCQNJC01770), and Tianjin Health Research Project (TJWJ2022QN028, TJWJ2022QN027, and ZC20010).
Conflict of interest
The authors declare that the research was conducted in the absence of any commercial or financial relationships that could be construed as a potential conflict of interest.
Publisher’s note
All claims expressed in this article are solely those of the authors and do not necessarily represent those of their affiliated organizations, or those of the publisher, the editors and the reviewers. Any product that may be evaluated in this article, or claim that may be made by its manufacturer, is not guaranteed or endorsed by the publisher.
References
Aw, S. T., Todd, M. J., Aw, G. E., McGarvie, L. A., and Halmagyi, G. M. (2005). Benign positional nystagmus: A study of its three-dimensional spatio-temporal characteristics. Neurology 64, 1897–1905. doi: 10.1212/01.WNL.0000163545.57134.3D
Baloh, R. W., and Honrubia, V. (1979). Clinical neurophysiology of the vestibular system. Contemp. Neurol. Ser. 18, 1–21.
Baloh, R. W., Honrubia, V., and Konrad, H. R. (1977). Ewald’s second law re-evaluated. Acta Otolaryngol. 83, 475–479. doi: 10.3109/00016487709128874
Bhattacharyya, N., Gubbels, S., and Schwartz, S. (2017). Clinical practice guideline: Benign paroxysmal positional vertigo. Otolaryngol. Head Neck Surg. 156 (suppl. 3), S1–S47.
Brevern, M. V., Bertholon, P., Brandt, T., Fife, T., Imai, T., Nuti, D., et al. (2017). Benign paroxysmal positional vertigo: Diagnostic criteria consensus document of the committee for the classification of vestibular disorders of the Barany Society. Acta Otorrinolaringol. Esp. 68, 349–360. doi: 10.1016/j.otorri.2017.02.007
Brevern, M. V., Bertholon, P., Brandt, T., Fife, T., Imai, T., Nuti, D., et al. (2015). Benign paroxysmal positional vertigo: Diagnostic criteria. J. Vestib. Res. 25, 105–117. doi: 10.3233/VES-150553
Dix, M. R., and Hallpike, C. S. (1952). The pathology, symptomatology and diagnosis of certain common disorders of the vestibular system. Ann. Otol. Rhinol. Laryngol. 61, 987–1016.
Eggers, S. D., Bisdorff, A., Brevern, M. V., Zee, D. S., Kim, J., Perez-Fernandez, N., et al. (2019). Classification of vestibular signs and examination techniques: Nystagmus and nystagmus-like movements. J. Vestib. Res. 29, 57–87. doi: 10.3233/VES-190658
Epley, J. M. (1992). The canalith repositioning procedure: For treatment of benign paroxysmal positional vertigo. Otolaryngol. Head Neck Surg. 107, 399–404. doi: 10.1177/019459989210700310
Fetter, M., and Sievering, F. (1995a). Three-dimensional (3-D) eye movement analysis in patients with positioning nystagmus. Acta Otolaryngol. Suppl. 520 Pt 2, 369–371. doi: 10.3109/00016489509125273
Fetter, M., and Sievering, F. (1995b). Three-dimensional eye movement analysis in benign paroxysmal positioning vertigo and nystagmus. Acta Otolaryngol. 115, 353–357. doi: 10.3109/00016489509139328
Hayashi, Y., Kanzaki, J., Etoh, N., Higashino, K., Goto, F., Schneider, E., et al. (2002). Three-dimensional analysis of nystagmus in benign paroxysmal positional vertigo. New insights into its pathophysiology. J. Neurol. 249, 1683–1688. doi: 10.1007/s00415-002-0905-z
Honrubia, V., and House, M. (2001). Mechanism of posterior semicircular canal stimulation in patients with benign paroxysmal positional vertigo. Acta Otolaryngol. 121, 234–240. doi: 10.1080/000164801300043640
Ichijo, H. (2013). Asymmetry of positioning nystagmus in posterior canalolithiasis. Acta Otolaryngol. 133, 159–164. doi: 10.3109/00016489.2012.728293
Imai, T., Takeda, N., Ito, M., Sekine, K., Sato, G., Midoh, Y., et al. (2009). 3D analysis of benign positional nystagmus due to cupulolithiasis in posterior semicircular canal. Acta Otolaryngol. 129, 1044–1049. doi: 10.1080/00016480802566303
Kim, H. J., Park, J., and Kim, J. S. (2021). Update on benign paroxysmal positional vertigo. J. Neurol. 268, 1995–2000.
Palmeri, R., and Kumar, A. (2022). Benign paroxysmal positional vertigo. Treasure Island, FL: StatPearls Publishing.
Power, L., Murray, K., and Szmulewicz, D. J. (2020). Characteristics of assessment and treatment in benign paroxysmal positional vertigo (BPPV). J. Vestib. Res. 30, 55–62.
Shigeno, K., and Kitaoka, K. (2020). A new variant of posterior canal-benign paroxysmal positional vertigo-canalolithiasis. Auris Nasus Larynx 47, 924–930.
Wang, N., Chen, T., Lin, P., Song, W., and Dong, H. (2009). [Positioning diagnosis of benign positional paroxysmal vertigo by VNG]. Lin Chung Er Bi Yan Hou Tou Jing Wai Ke Za Zhi 23, 597–600.
Xu, K. X., Chen, T. S., Wang, W., Liu, Q., Wen, C., Li, S. S., et al. (2019). [Objective characteristics of nystagmus in patients with posterior semicircular canal benign paroxysmal positional vertigo]. Zhonghua Er Bi Yan Hou Tou Jing Wai Ke Za Zhi 54, 729–733.
Yang, C. J., Lee, J., Kim, S., Lee, C., and Park, H. J. (2017). Development of a murine model of traumatic benign paroxysmal positional vertigo: A preliminary study. Acta Otolaryngol. 137, 29–34. doi: 10.1080/00016489.2016.1217043
Keywords: benign positional paroxysmal vertigo, three-dimensional video nystagmography, canalithiasis, semicircular canal, Ewald’s law
Citation: Liu Y, Zhang X, Deng Q, Liu Q, Wen C, Wang W and Chen T (2022) The 3D characteristics of nystagmus in posterior semicircular canal benign paroxysmal positional vertigo. Front. Neurosci. 16:988733. doi: 10.3389/fnins.2022.988733
Received: 07 July 2022; Accepted: 22 November 2022;
Published: 13 December 2022.
Edited by:
Sulin Zhang, Huazhong University of Science and Technology, ChinaReviewed by:
Qing Zhang, Shanghai Jiao Tong University School of Medicine, ChinaLorenzo Salerni, University of Siena, Italy
Dan Bing, Huazhong University of Science and Technology, China
Copyright © 2022 Liu, Zhang, Deng, Liu, Wen, Wang and Chen. This is an open-access article distributed under the terms of the Creative Commons Attribution License (CC BY). The use, distribution or reproduction in other forums is permitted, provided the original author(s) and the copyright owner(s) are credited and that the original publication in this journal is cited, in accordance with accepted academic practice. No use, distribution or reproduction is permitted which does not comply with these terms.
*Correspondence: Wei Wang, d3dlaTExMDZAaG90bWFpbC5jb20=; Taisheng Chen, ZmNoX2N0c0BzaW5hLmNvbQ==