- 1Department of Neurology and Psychiatry, Tongji Hospital, Tongji Medical College, Huazhong University of Science and Technology, Wuhan, China
- 2Department of Pharmacology, School of Basic Medical Sciences, Fudan University, Shanghai, China
Background: Observational studies have suggested that peripheral immune disorders are associated with amyotrophic lateral sclerosis (ALS). Previous studies predominantly focused on changes in adaptive immunity. However, emerging evidence showed natural killer (NK) cells, an essential component of innate immunity, were involved in the degeneration of motor neurons. However, the causal relationship between dysregulated NK cells-related immune traits and ALS remains unclear.
Objective: This study aimed to explore the causal relationship between NK cells-related immune traits and the risk of ALS.
Materials and methods: Single nucleotide polymorphisms (SNPs) significantly associated with NK cells-related immune traits were selected as instrumental variables to estimate their causal effects on ALS. SNPs from a genome-wide association study (GWAS) on NK cells-related immune traits were used as exposure instruments, including an absolute NK-cells count, absolute HLA-DR+ NK-cells count, NK cells/lymphocytes, NK cells/CD3– lymphocytes, HLA DR+ NK cells/NK cells, HLA DR+ NK cells/CD3– lymphocytes, and the median fluorescence intensities of CD16–CD56+ on NK cells and HLA-DR+ NK cells. Summary-level GWAS statistics of ALS were used as the outcome data. Exposure and outcome data were analyzed using the two-sample Mendelian randomization (MR) method.
Results: Each one standard deviation increase in the expression levels of CD16–CD56+ on NK cells and HLA-DR+ NK cells were associated with a lower risk of ALS in both the MR-Egger and inverse variance weighted methods (P < 0.05). The results proved robust under all sensitivity analyses. Neither instrumental outliers nor heterogeneity were detected.
Conclusion: Our results suggest that higher expression levels of CD16–CD56+ on NK cells and HLA-DR+ NK cells are associated with a lower risk of ALS.
Introduction
Amyotrophic lateral sclerosis (ALS) is a fatal neurodegenerative disorder characterized by progressive degeneration of the upper and lower motor neurons (Kiernan et al., 2011). Riluzole and edaravone are currently the most widely used medicines for ALS, and they potentially prolong survival by 3–6 months in most patients with ALS (Hardiman et al., 2011; Rothstein, 2017). Although significant effort has been devoted to investigating the pathological mechanisms underlying ALS, with several theories having been proposed, the etiology of ALS remains poorly understood (Geevasinga et al., 2016).
Accumulating evidence implicates neuroimmune crosstalk between the peripheral immune system (PIS) and central immune system (CIS) in ALS, cooperatively contributing to the death of motor neurons (Yu et al., 2022). An increased infiltration of peripheral immune cells into the spinal cord and motor cortex of patients with ALS has been observed, which, together with activated microglia and astrocytes, constitute a pro-inflammatory immune microenvironment (Beers and Appel, 2019). Previous studies have linked changes in the PIS with disease progression in both mouse models and patients with ALS (Zhao et al., 2013; Murdock et al., 2016; McCombe et al., 2020). Specifically, the total leukocytes count is elevated in patients with ALS, and the count of anti-inflammatory regulatory T cells (Tregs) negatively correlates with disease progression (Beers et al., 2017; Sheean et al., 2018). These studies have indicated that peripheral immune disorders and systemic inflammation are associated with ALS. In addition to previous reports on changes in adaptive immunity, a considerable number of studies have recently reported innate immune disorders in ALS, providing insight into the novel pathological mechanisms of ALS.
As an important component of innate immune cells, natural killer (NK) cells are important components of the first line of immune defense in humans and essential regulators of adaptive immunity (Abel et al., 2018). Conventionally, human NK cells are defined by the expression of CD56 and lack of CD3 (Cooper et al., 2001a). Preliminary studies have demonstrated that NK cells may be involved in the development of ALS. Under normal physiological conditions, motor neurons are protected from NK-cells damage by the expression of human leukocyte antigen A–C surface markers, which are lost in spinal cord motor neurons in ALS due to unknown mechanisms, rendering them vulnerable to NK-cells damage (Song et al., 2016). Garofalo et al. (2020) revealed that NK cells in the spinal cord instruct microglia toward an inflammatory phenotype in an interferon-γ (IFN-γ)-dependent manner in a mouse model of ALS (hSOD1G93A). However, it is important to note that not all NK cells are prolific IFN-γ producers, and the functions of NK cells are closely related to the expression of surface markers, such as CD16, CD56, CD57, and CD161. Compared with other subsets of NK cells, CD16–CD56+ NK cells and HLA-DR+ NK cells are two functionally activated subsets associated with high IFN-γ production (Freud et al., 2017; Erokhina et al., 2018, 2021). The currently limited understanding of NK cells in ALS may be due to insufficient discrimination of NK cells-related immune traits in previous studies. Therefore, we emphasize that distinguishing NK cells subsets is an important step in exploring the role of NK cells in ALS.
In medical research, conclusions drawn from observational studies are susceptible to methodological inconsistencies, confounding factors, and selection bias (Sedgwick, 2015). Randomized controlled trials (RCTs) are a promising tool for making causal inferences; however, the implementation of RCTs requires tremendous human and financial effort and is occasionally subject to sample size and ethical issues, especially in the field of rare diseases, such as ALS. Mendelian randomization (MR) is an emerging approach for assessing the causal relationship between exposure and outcome (Emdin et al., 2017). Based on Mendel’s law of inheritance regarding the random assignment of parental alleles to offspring, the MR approach can be considered a “natural” RCT, capable of reducing the conventional bias of observational studies and compensating for the inadequacy of RCTs in investigating rare diseases (Emdin et al., 2017). Therefore, to evaluate the causal relationship between NK cells-related immune traits and ALS, we conducted a MR study. By leveraging the single nucleotide polymorphism (SNP) data from a large genome-wide association study (GWAS) on immune-cells traits and summary-GWAS ALS statistics, we found a higher expression of CD16–CD56+ on NK cells and HLA-DR+ NK cells to be causally associated with a lower risk of ALS.
Materials and methods
Exposure data and instrumental variable selection
The appropriate genetic variants were selected from a previous GWAS study that assessed the impact of natural genetic variation on immune-cells traits (Orrù et al., 2020). In the current study, we obtained summary-level association results for eight independent NK cells-related immune traits, including the absolute counts of NK cells and HLA-DR+ NK cells; ratios of NK cells/lymphocytes, NK cells/CD3– lymphocytes, HLA-DR+ NK cells/NK cells, and HLA-DR+ NK cells/CD3– lymphocytes; and the median fluorescence intensity (MFI) of CD16–CD56+ on NK cells and HLA-DR+ NK cells. The genetic IVs, SNPs in this case, were filtered according to a previously described procedure (Xia et al., 2022). Briefly, SNPs associated with NK cells-related immune traits were extracted as IVs at a genome-wide significance level (P < 5.00E-8). Second, the extracted IVs were subsequently clumped based on the 1000 Genomes Project linkage disequilibrium structure (R2 < 0.001), and only the SNPs with the lowest P-values were retained (Abecasis et al., 2012). Third, certain SNPs that were not available in the summary statistics of the outcome were replaced with proxy SNPs with a high correlation coefficient (R2 ≥ 0.8) based on European ancestry. Fourth, F-statistics for each SNP were calculated to quantify the strength of the instruments, and weak SNPs were excluded (F < 10) (Burgess et al., 2016). Summarized information pertaining to all the IVs included in this study is shown in the Supplementary Table.
Outcome data
Publicly available GWAS summary statistics for ALS were obtained from a previous study involving a sample of 80,810 individuals of European ancestry (20,806 ALS cases and 59,804 control cases) (Nicolas et al., 2018). Kinesin family member 5A (KIF5A) was identified as a novel gene associated with ALS in this GWAS study. All patients with ALS in this GWAS study had been diagnosed with ALS at probable or definite levels according to the El Escorial criteria (Brooks, 1994) and had experienced symptom onset after 18 years of age. The outcome and exposure data were harmonized to exclude strand mismatches and ensure the alignment of SNP effect sizes (Burgess et al., 2019).
Two-sample Mendelian randomization
The theoretical basis of two-sample MR research relies on three core assumptions: (1) the selected genetic variances, as IVs, are associated with the risk factor, that is, NK cells-related immune traits in this case; (2) the selected genetic variances are significantly associated with ALS through the risk factor only; and (3) the selected IVs are not associated with other confounding factors. In MR analysis, when the core assumptions are satisfied, the inverse variance weighted (IVW) method can improve the statistical power and accuracy of the estimation (Burgess et al., 2017); therefore, the IVW method was implemented as the main approach to examining the overall causal relationship between NK cells-related immune traits and ALS based on the effect of IVs. However, when horizontal pleiotropy exists, the causal estimate using the IVW method is potentially biased. Thus, genetic variants may affect ALS susceptibility through pathways other than NK cells-related immune traits. The presence of pleiotropy was detected when the intercept significantly deviated from the origin in the MR-Egger regression method (Hemani et al., 2018). MR-Egger regression and weighted median was implemented as complementary methods to examine the effect of NK cells-related immune traits on ALS. We conducted Cochran’s Q test and leave-one-SNP-out analysis using the IVW method to evaluate the heterogeneity of the IVs. Steiger’s analysis was used to explore whether ALS had a causal impact on NK cells-related immune traits (Hemani et al., 2017). A flow diagram depicting the study process is shown in Figure 1. All analyses were performed using the TwoSampleMR and RadialMR packages in R software (version 4.2.0).
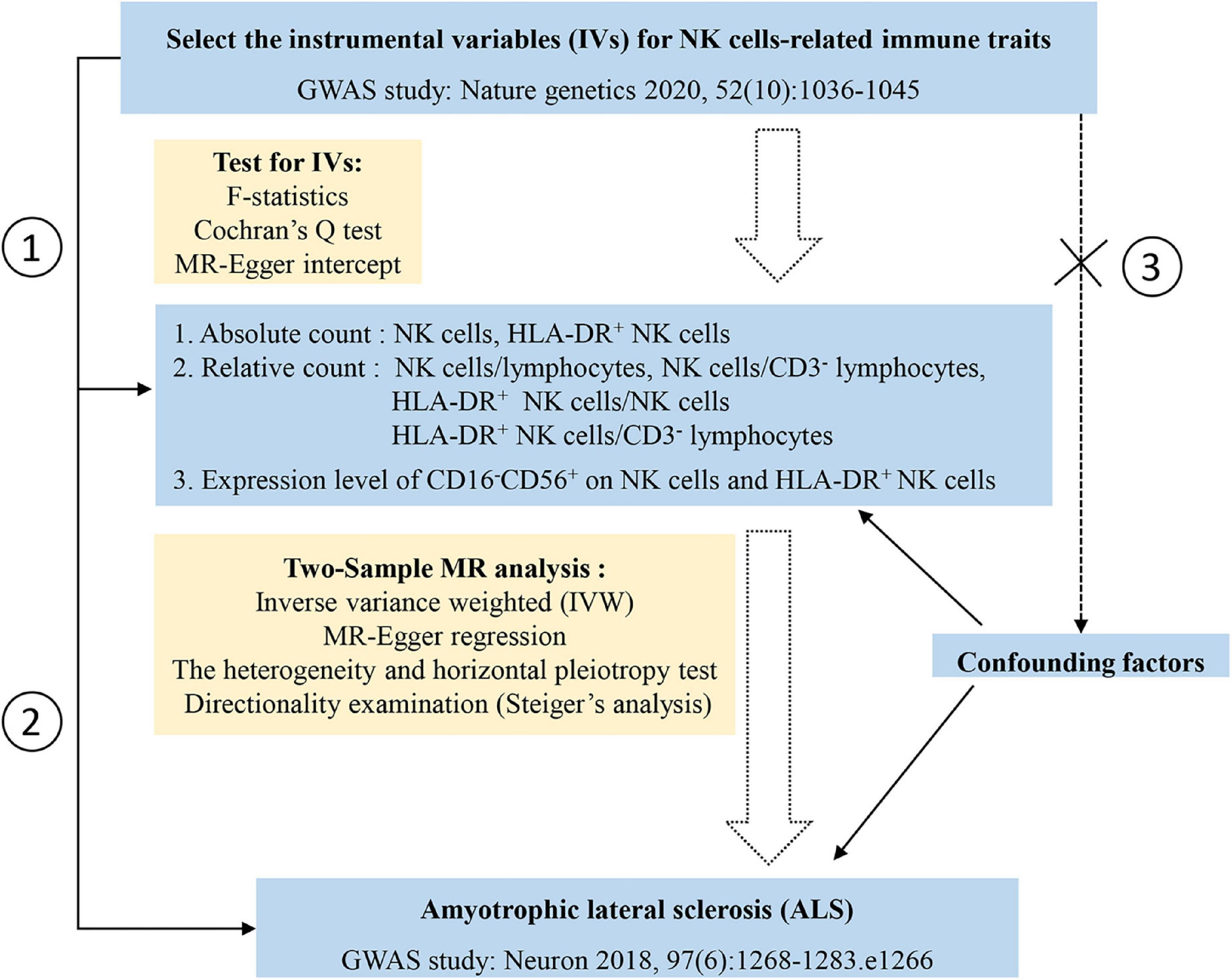
Figure 1. Study design and the flow chart of statistical analysis. Three assumptions of the Mendelian randomization in the current study: assumption 1, the selected genetic variances, as instrumental variables, are associated with the risk factor, that is, natural killer (NK) cells-related immune traits in this case; assumption 2, the selected genetic variances are significantly associated with amyotrophic lateral sclerosis (ALS) through the risk factor only; assumption 3, the selected instrumental variables are not associated other confounding factors (the dashed line and cross symbol indicate no association between selected instrumental variables and confounding factors).
Results
Eight NK cells-related immune traits were analyzed for their association with ALS in the current study. The association statistics are summarized in Table 1 and Figure 2. The results revealed that a one standard deviation (1-SD) increase in the MFI of CD16–CD56+ on NK cells was associated with a lower risk of ALS in both the IVW method (odds ratio [OR] = 0.966, 95% confidence interval [CI] = 0.946–0.987, P = 0.002) and MR-Egger (OR = 0.934, 95% CI = 0.893–0.976, P = 0.005; Figure 3). The MFI of CD16–CD56+ on HLA-DR+ NK cells was also negatively associated with the risk of ALS in the IVW method (OR = 0.919, 95% CI = 0.854–0.989, P = 0.025) and MR-Egger (OR = 0.519, 95% CI = 0.321–0.838, P = 0.036; Supplementary Figure 1). The increased ratio of NK cells/CD3– lymphocytes was negatively associated with the risk of ALS in the IVW method (OR = 0.975, 95% CI = 0.955–0.996, P = 0.020) but not in the MR-Egger method (OR = 0.979, 95% CI = 0.954–1.005, P = 0.124).
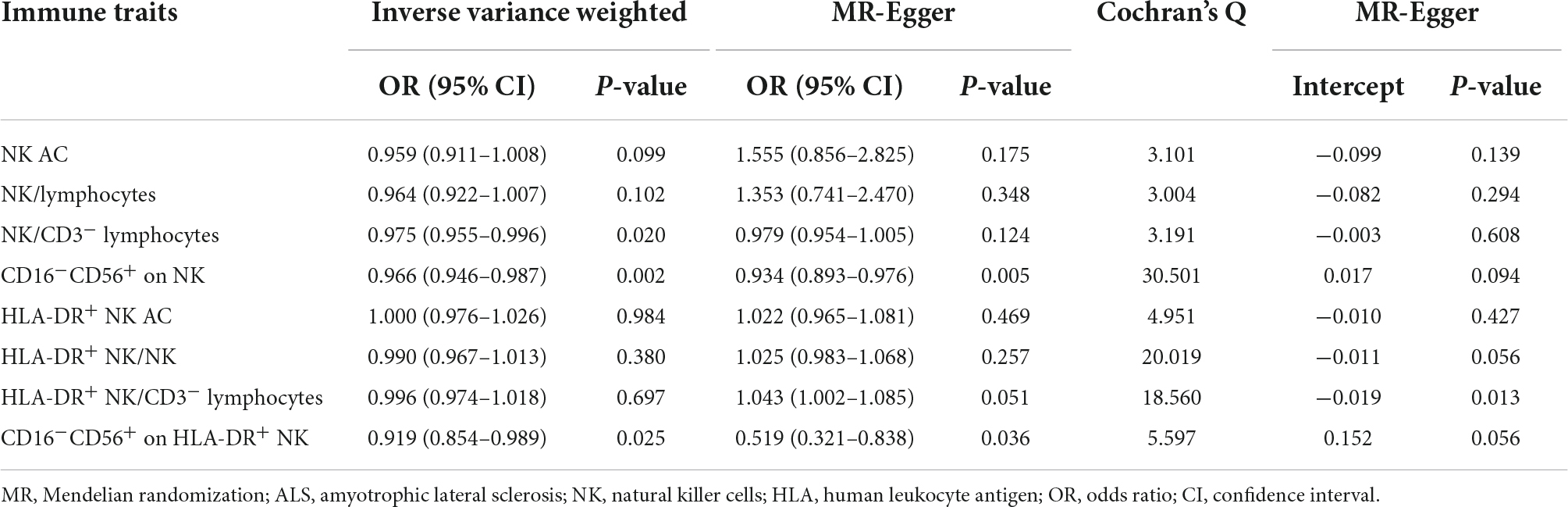
Table 1. Summary of the causal effects of natural killer (NK) cells-related immune traits on amyotrophic lateral sclerosis (ALS) with various Mendelian randomization (MR) methods.
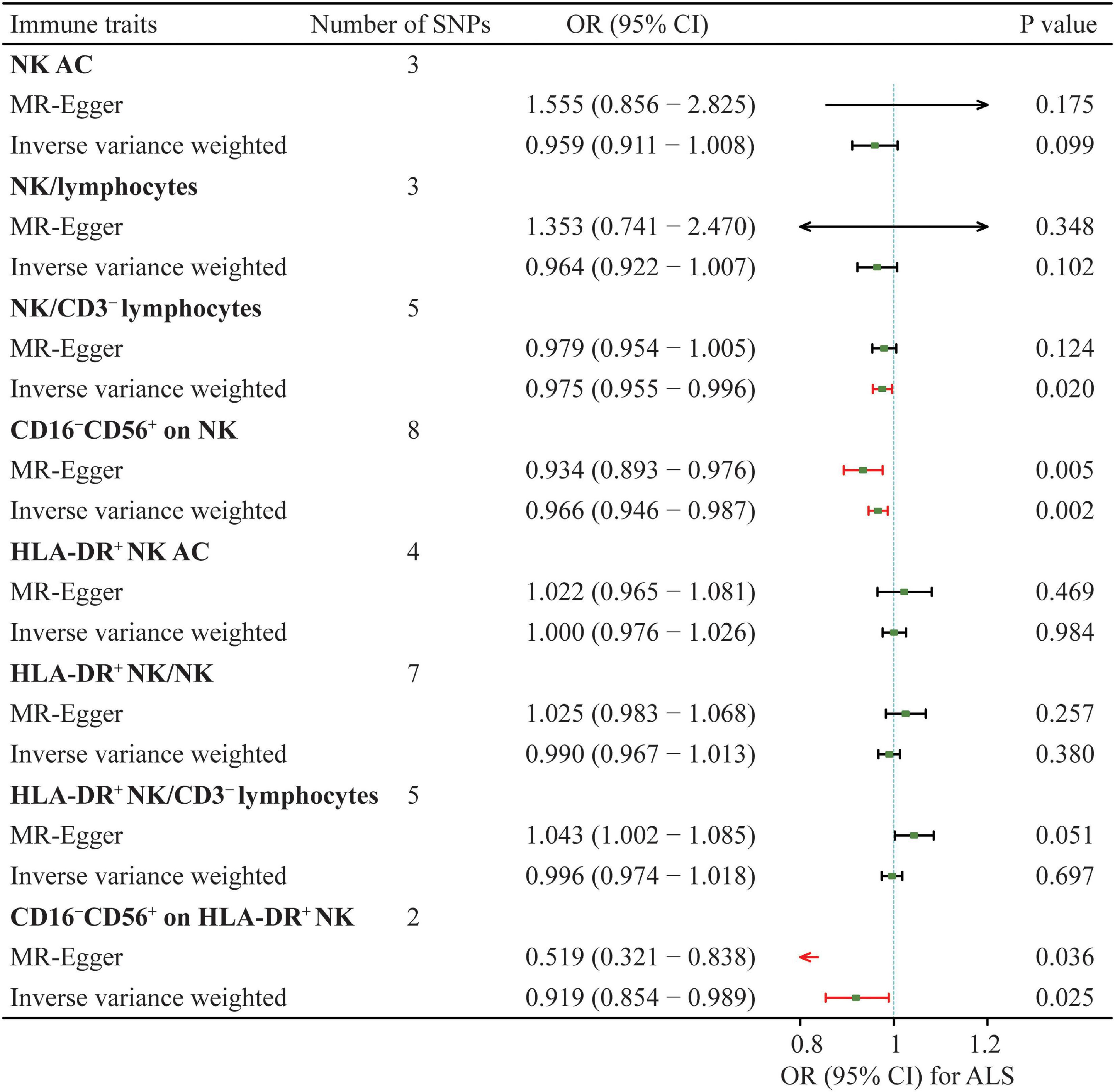
Figure 2. Summary of the causal effects of NK cells-related immune traits on ALS with mendelian randomization. ALS, amyotrophic lateral sclerosis; SNP, single nucleotide polymorphism; NK, natural killer cells; HLA, human leucocyte antigen; OR, odds ratio; CI, confidence interval.
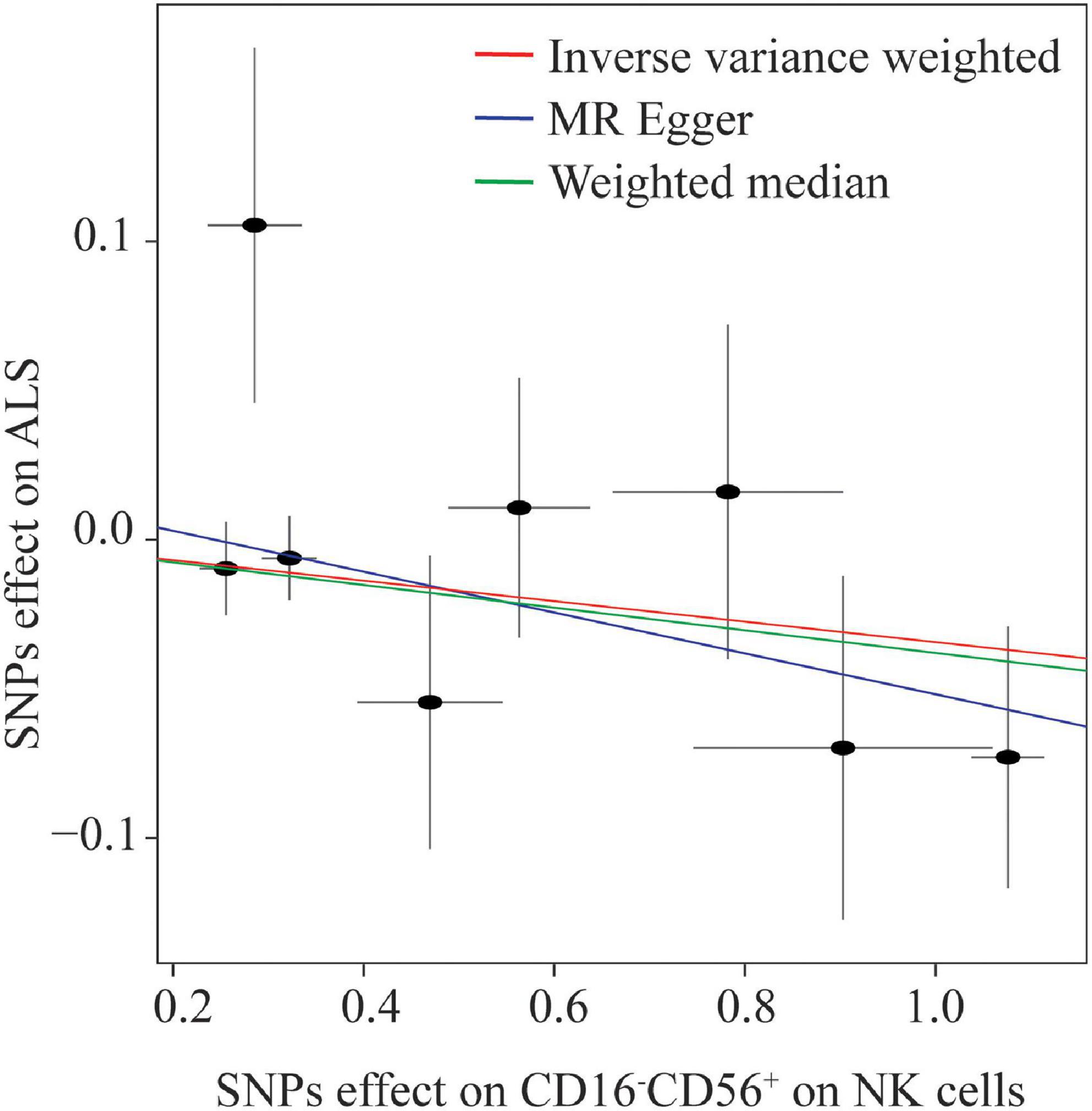
Figure 3. Scatterplot of single nucleotide polymorphism (SNP) effects on the expression level of CD16–CD56+ on natural killer (NK) cells versus amyotrophic lateral sclerosis (ALS), with the slope of each line corresponding to the estimated Mendelian randomization effect per method.
Thereafter, extensive sensitivity analyses were performed to validate the causal association between NK cells-related immune traits (CD16–CD56+ on NK cells and HLA-DR+ NK cells) and ALS. No heterogeneity was detected using Cochran’s Q test (Q pval > 0.05; Table 1). Moreover, the results indicated no horizontal pleiotropy (the intercept of MR-Egger did not significantly deviate from zero, and p-values for an intercept from MR-Egger were > 0.05). No single instrumental variable influenced the estimated causal effects (Figure 4). A directionality examination using Steiger’s analysis did not suggest a violation of causality.
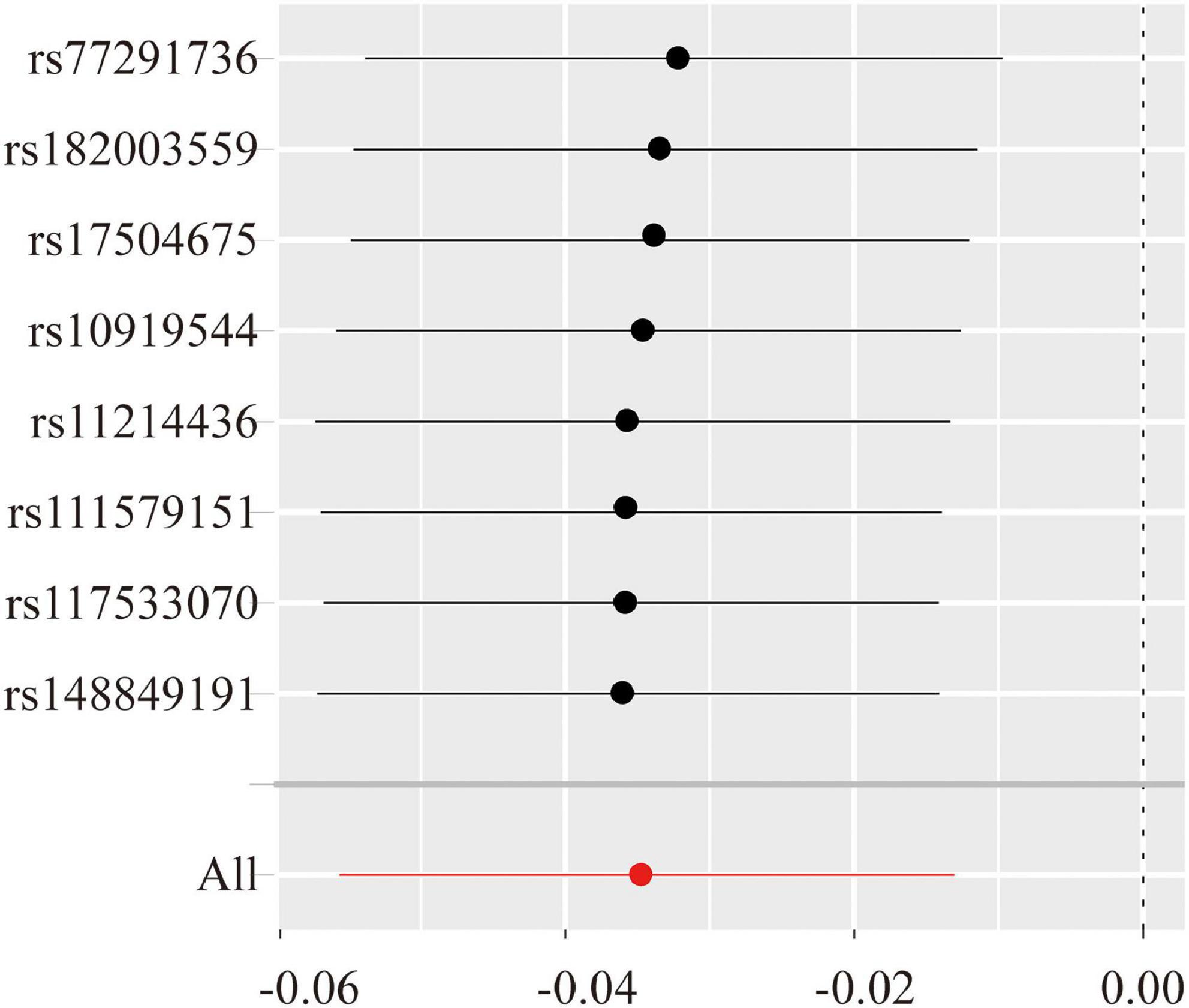
Figure 4. The leave-one-single nucleotide polymorphism (ANP)-out sensitivity analyze for the expression level of CD16–CD56+ on natural killer (NK) cells versus amyotrophic lateral sclerosis (ALS). The Y-axis shows the ID of the SNP to be omitted from analysis and the X-axis represents Mendelian randomization (MR) odds ratios. The red line shows MR estimate including all SNPs.
Discussion
In the past few years, there has been emerging evidence that innate immunity disorders are involved in the occurrence and development of ALS (Chiu et al., 2009; Cirulli et al., 2015; Volpe and Nogueira-Machado, 2015; Oakes et al., 2017). Herein, using a two-sample MR approach, we demonstrated that increased expression levels of CD16–CD56+ on NK cells and HLA-DR+ NK cells were causally associated with a decreased risk of ALS, indicating that these two subsets of NK cells are protective factors against ALS. To the best of our knowledge, this study is the first attempt at elucidating the causal relationship between alterations in NK cells-related immune traits and ALS using a genetic approach based on GWAS summary statistics.
Natural killer cells constitute one of the three major human lymphocyte lineages (T, B, and NK cells), accounting for approximately 5–15% of circulating lymphocytes in healthy adults (Colucci et al., 2003). In addition to being the most important component of innate immunity, NK cells are key players in adaptive immunity (Caligiuri, 2008). Over the past decade, accumulating evidence has revealed that CNS-infiltrating NK cells modulate neuroinflammation, establishing an important role for NK cells in neurodegenerative diseases (Poli et al., 2013; Menees and Lee, 2022; Wang and van de Pavert, 2022). In patients with ALS, NK cells are elevated in the peripheral blood (Gustafson et al., 2017; Murdock et al., 2017). NK cells have also been shown to infiltrate the CNS of both hSOD1G93A mice and patients with ALS (Finkelstein et al., 2011; Garofalo et al., 2020). Recently, IFN-γ-secreting NK cells were shown to colocalize with motor neurons in the spinal cord and motor cortex of hSOD1G93A mice at the early stage of ALS, driving pro-inflammatory microglial activity in the CNS (Garofalo et al., 2020). These findings suggest a potential role and viable therapeutic target for NK cells in ALS.
In clinical practice, a vital problem exists in that the deletion of a classification of immune cells is usually not feasible because it severely disrupts the immune system. To the best of our knowledge, recent studies have only focused on the specific classification of T cells in adaptive immunity in ALS, such as CD4+CD25+Foxp3+ Tregs (Thonhoff et al., 2018). Therefore, probing changes in different subsets of NK cells is critical for a more comprehensive understanding of the peripheral immune abnormalities in ALS. In humans, NK cells exhibit the dynamic expression of surface markers involved in differentiation, trafficking, and cytotoxicity, and different subsets of NK cells possess varying immune functions (O′Brien and Finlay, 2019). In a recent study, changes in trafficking and cytotoxicity markers in NK cells were associated with changes in the revised ALS functional rating scale (ALSFRS-R) (Murdock et al., 2021). Conventionally, according to the differential expression of CD16 and CD56, NK cells in the peripheral blood are classified into two major subsets: CD16+CD56dim NK cells and CD16–CD56+ NK cells (Cooper et al., 2001b; Farag and Caligiuri, 2006). CD16+CD56dim NK cells account for approximately 90% of peripheral NK cells and predominantly exert cytotoxic effects. CD16–CD56+ NK cells only account for approximately 10% of NK cells; however, they play an important role in the regulation of immunity by secreting various cytokines, including IFN-γ, tumor necrosis factor (TNF), and granulocyte colony-stimulating factor (Freud et al., 2017). Accumulating evidence reveals a systematic pro-inflammatory state and the crosstalk between the PIS and CIS in ALS. Elevated levels of blood TNF-α, TNF receptor 1, interleukin 6 (IL-6), IL-1β, IL-8, and vascular endothelial growth factor were observed in patients with ALS compared with those in controls (Hu et al., 2017). As important immunoregulatory and cytokine-secreting cells, CD16–CD56+ NK cells potentially contribute to the systemic inflammatory state and degeneration of motor neurons by regulating the levels of peripheral cytokines. In a study of multiple sclerosis, IFN-γ+ NK cells migrated to the vicinity of the meninges to drive astrocytes to convert to an anti-inflammatory phenotype, reducing the level of neuroinflammation in the spinal cord (Sanmarco et al., 2021). Ziemssen et al. revealed a significantly higher relative amount of CD16brightCD56dim NK cells in patients with ALS (Jin et al., 2020). A recent study demonstrated that lower levels of CD56bright NK cells in the CSF were associated with faster progression in ALS patients (Rolfes et al., 2021), thus supporting our results wherein a per standard deviation increase in CD16–CD56+ NK cells was found to be negatively associated with the risk of ALS.
In addition to classifying NK cells based on T-cell receptor-associated molecules, NK cells can also be subdivided according to other markers, such as NK cell p44-related protein and HLA-DR (a subtype of class II histocompatibility antigens) (Phillips et al., 1984; Vitale et al., 1998). HLA-DR expression in NK cells is a marker of cellular activation. Similar to CD16–CD56+ NK cells, HLA-DR+ NK cells are associated with higher IFN-γ production (Evans et al., 2011; Langers et al., 2014; Erokhina et al., 2018), and IFN-γ induces high HLA-DR expression in NK cells (Yano et al., 1996). In our study, the absolute counts of HLA-DR+ exhibited no causal relationship with the risk of ALS; nonetheless, a higher expression level of CD16–CD56+ was associated with a lower risk of ALS. In fact, a fairly high proportion of CD16–CD56+ NK cells are also HLA-DR+ under normal conditions (Mizrahi et al., 2007). Therefore, we hypothesized that the expression levels of CD16–CD56+ and HLA-DR+ may be related to immune disorders in ALS through the secretion of cytokines, particularly IFN-γ.
The results of our study are reliable, since we enrolled a recently published, high-quality GWAS study on the immune-cells spectrum. Furthermore, the GWAS on ALS selected in the current study was also based on a large sample of the European population. The reliable data sources and study designs provided sufficient statistical power. However, in addition to the limitations of the MR methodology, which have been reviewed previously (Davey Smith and Hemani, 2014), this study has several other limitations: (1) the presently available GWAS data exclusively provided the MFI of CD16–CD56+ on NK cells; the absolute count of CD16–CD56+ NK cells is required in future GWAS studies; (2) in this study, the number of SNPs for some NK cells-related immune traits were relatively small (<5) because of the absence of an available corresponding GWAS; and (3) the immune traits that were measured at a specific time point might have been affected by many temporary factors, such as age and lifestyle, which may not reflect the lifelong immune characteristics determined by the encoding gene.
Conclusion
In conclusion, our MR study suggests that higher expression levels of CD16–CD56+ on NK cells and HLA-DR+ NK cells are associated with a lower risk of ALS. Our work enhances the current understanding of the role of peripheral immune disorders in ALS, especially the role of innate immunity, and provides insight into potential NK cells-based therapeutic approaches in ALS. In the future, more attention should be focused on exploring the potential mechanism of the dysregulation of innate immune-cells subsets in the pathogenesis of ALS, an approach that may provide a theoretical basis for the proposal of new therapeutic strategies.
Data availability statement
All data in this study were obtained from previously published GWAS studies. Data used can be obtained through cited papers. Further inquiries can be directed to the corresponding author.
Author contributions
ZG and LB: investigation, methodology, data acquisition and analysis, and visualization. MZ: conceptualization, funding acquisition. LB and MZ: supervision, data curation, reviewing, and editing. All authors contributed to the article, interpretation of the data, drafting of the manuscript, and approved the submission.
Funding
This study was supported by National Key Research and Development Program of China (2018YFE0118900) and the Clinical Research Project of the Bethune Charitable Foundation.
Acknowledgments
We thank Orrù V. et al. and Nicolas A. et al. for sharing the GWAS summary data from the published manuscript.
Conflict of interest
The authors declare that the research was conducted in the absence of any commercial or financial relationships that could be construed as a potential conflict of interest.
Publisher’s note
All claims expressed in this article are solely those of the authors and do not necessarily represent those of their affiliated organizations, or those of the publisher, the editors and the reviewers. Any product that may be evaluated in this article, or claim that may be made by its manufacturer, is not guaranteed or endorsed by the publisher.
Supplementary material
The Supplementary Material for this article can be found online at: https://www.frontiersin.org/articles/10.3389/fnins.2022.981371/full#supplementary-material
References
Abecasis, G. R., Auton, A., Brooks, L. D., DePristo, M. A., Durbin, R. M., Handsaker, R. E., et al. (2012). An integrated map of genetic variation from 1,092 human genomes. Nature 491, 56–65. doi: 10.1038/nature11632
Abel, A. M., Yang, C., Thakar, M. S., and Malarkannan, S. (2018). Natural killer cells: development, maturation, and clinical utilization. Front. Immunol. 9:1869. doi: 10.3389/fimmu.2018.01869
Beers, D. R., and Appel, S. H. (2019). Immune dysregulation in amyotrophic lateral sclerosis: mechanisms and emerging therapies. Lancet Neurol. 18, 211–220. doi: 10.1016/S1474-4422(18)30394-6
Beers, D. R., Zhao, W., Wang, J., Zhang, X., Wen, S., Neal, D., et al. (2017). ALS patients’ regulatory T lymphocytes are dysfunctional, and correlate with disease progression rate and severity. JCI Insight 2:e89530. doi: 10.1172/jci.insight.89530
Brooks, B. R. (1994). El Escorial World Federation of Neurology criteria for the diagnosis of amyotrophic lateral sclerosis. Subcommittee on Motor Neuron Diseases/Amyotrophic Lateral Sclerosis of the World Federation of Neurology Research Group on Neuromuscular Diseases and the El Escorial “Clinical limits of amyotrophic lateral sclerosis” workshop contributors. J. Neurol. Sci. 124(Suppl.), 96–107. doi: 10.1016/0022-510x(94)90191-0
Burgess, S., Bowden, J., Fall, T., Ingelsson, E., and Thompson, S. G. (2017). Sensitivity analyses for robust causal inference from mendelian randomization analyses with multiple genetic variants. Epidemiology 28, 30–42. doi: 10.1097/EDE.0000000000000559
Burgess, S., Davey Smith, G., Davies, N. M., Dudbridge, F., Gill, D., Glymour, M. M., et al. (2019). Guidelines for performing Mendelian randomization investigations. Wellcome Open Res. 4:186. doi: 10.12688/wellcomeopenres.15555.1
Burgess, S., Davies, N. M., and Thompson, S. G. (2016). Bias due to participant overlap in two-sample Mendelian randomization. Genet. Epidemiol. 40, 597–608. doi: 10.1002/gepi.21998
Chiu, I. M., Phatnani, H., Kuligowski, M., Tapia, J. C., Carrasco, M. A., Zhang, M., et al. (2009). Activation of innate and humoral immunity in the peripheral nervous system of ALS transgenic mice. Proc. Natl. Acad. Sci. U.S.A. 106, 20960–20965. doi: 10.1073/pnas.0911405106
Cirulli, E. T., Lasseigne, B. N., Petrovski, S., Sapp, P. C., Dion, P. A., Leblond, C. S., et al. (2015). Exome sequencing in amyotrophic lateral sclerosis identifies risk genes and pathways. Science 347, 1436–1441. doi: 10.1126/science.aaa3650
Colucci, F., Caligiuri, M. A., and Di Santo, J. P. (2003). What does it take to make a natural killer? Nat. Rev. Immunol. 3, 413–425. doi: 10.1038/nri1088
Cooper, M. A., Fehniger, T. A., and Caligiuri, M. A. (2001a). The biology of human natural killer-cell subsets. Trends Immunol. 22, 633–640. doi: 10.1016/S1471-4906(01)02060-9
Cooper, M. A., Fehniger, T. A., Turner, S. C., Chen, K. S., Ghaheri, B. A., Ghayur, T., et al. (2001b). Human natural killer cells: a unique innate immunoregulatory role for the CD56(bright) subset. Blood 97, 3146–3151. doi: 10.1182/blood.V97.10.3146
Davey Smith, G., and Hemani, G. (2014). Mendelian randomization: genetic anchors for causal inference in epidemiological studies. Hum. Mol. Genet. 23, R89–R98. doi: 10.1093/hmg/ddu328
Emdin, C. A., Khera, A. V., and Kathiresan, S. (2017). Mendelian randomization. JAMA 318, 1925–1926. doi: 10.1001/jama.2017.17219
Erokhina, S. A., Streltsova, M. A., Kanevskiy, L. M., Grechikhina, M. V., Sapozhnikov, A. M., and Kovalenko, E. I. (2021). HLA-DR-expressing NK cells: effective killers suspected for antigen presentation. J. Leukocyte Biol. 109, 327–337. doi: 10.1002/JLB.3RU0420-668RR
Erokhina, S. A., Streltsova, M. A., Kanevskiy, L. M., Telford, W. G., Sapozhnikov, A. M., and Kovalenko, E. I. (2018). HLA-DR(+) NK cells are mostly characterized by less mature phenotype and high functional activity. Immunol. Cell Biol. 96, 212–228.
Evans, J. H., Horowitz, A., Mehrabi, M., Wise, E. L., Pease, J. E., Riley, E. M., et al. (2011). A distinct subset of human NK cells expressing HLA-DR expand in response to IL-2 and can aid immune responses to BCG. Eur. J. Immunol. 41, 1924–1933. doi: 10.1002/eji.201041180
Farag, S. S., and Caligiuri, M. A. (2006). Human natural killer cell development and biology. Blood Rev. 20, 123–137. doi: 10.1016/j.blre.2005.10.001
Finkelstein, A., Kunis, G., Seksenyan, A., Ronen, A., Berkutzki, T., Azoulay, D., et al. (2011). Abnormal changes in NKT cells, the IGF-1 axis, and liver pathology in an animal model of ALS. PLoS One 6:e22374. doi: 10.1371/journal.pone.0022374
Freud, A. G., Mundy-Bosse, B. L., Yu, J., and Caligiuri, M. A. (2017). The broad spectrum of human natural killer cell diversity. Immunity 47, 820–833.
Garofalo, S., Cocozza, G., Porzia, A., Inghilleri, M., Raspa, M., Scavizzi, F., et al. (2020). Natural killer cells modulate motor neuron-immune cell cross talk in models of amyotrophic lateral sclerosis. Nat. Commun. 11:1773. doi: 10.1038/s41467-020-15644-8
Geevasinga, N., Menon, P., Özdinler, P. H., Kiernan, M. C., and Vucic, S. (2016). Pathophysiological and diagnostic implications of cortical dysfunction in ALS. Nat. Rev. Neurol. 12, 651–661. doi: 10.1038/nrneurol.2016.140
Gustafson, M. P., Staff, N. P., Bornschlegl, S., Butler, G. W., Maas, M. L., Kazamel, M., et al. (2017). Comprehensive immune profiling reveals substantial immune system alterations in a subset of patients with amyotrophic lateral sclerosis. PLoS One 12:e0182002. doi: 10.1371/journal.pone.0182002
Hardiman, O., van den Berg, L. H., and Kiernan, M. C. (2011). Clinical diagnosis and management of amyotrophic lateral sclerosis. Nat. Rev. Neurol. 7, 639–649. doi: 10.1038/nrneurol.2011.153
Hemani, G., Bowden, J., and Davey Smith, G. (2018). Evaluating the potential role of pleiotropy in Mendelian randomization studies. Hum. Mol. Genet. 27, R195–R208. doi: 10.1093/hmg/ddy163
Hemani, G., Tilling, K., and Davey Smith, G. (2017). Orienting the causal relationship between imprecisely measured traits using GWAS summary data. PLoS Genet. 13:e1007081. doi: 10.1371/journal.pgen.1007081
Hu, Y., Cao, C., Qin, X. Y., Yu, Y., Yuan, J., Zhao, Y., et al. (2017). Increased peripheral blood inflammatory cytokine levels in amyotrophic lateral sclerosis: a meta-analysis study. Sci. Rep. 7:9094. doi: 10.1038/s41598-017-09097-1
Jin, M., Günther, R., Akgün, K., Hermann, A., and Ziemssen, T. (2020). Peripheral proinflammatory Th1/Th17 immune cell shift is linked to disease severity in amyotrophic lateral sclerosis. Sci. Rep. 10:5941. doi: 10.1038/s41598-020-62756-8
Kiernan, M. C., Vucic, S., Cheah, B. C., Turner, M. R., Eisen, A., Hardiman, O., et al. (2011). Amyotrophic lateral sclerosis. Lancet 377, 942–955. doi: 10.1016/S0140-6736(10)61156-7
Langers, I., Renoux, V., Reschner, A., Touzé, A., Coursaget, P., Boniver, J., et al. (2014). Natural killer and dendritic cells collaborate in the immune response induced by the vaccine against uterine cervical cancer. Eur. J. Immunol. 44, 3585–3595. doi: 10.1002/eji.201444594
McCombe, P. A., Lee, J. D., Woodruff, T. M., and Henderson, R. D. (2020). The peripheral immune system and amyotrophic lateral sclerosis. Front. Neurol. 11:279. doi: 10.3389/fneur.2020.00279
Menees, K. B., and Lee, J. K. (2022). New insights and implications of natural killer cells in Parkinson’s disease. J. Parkinsons Dis. [Epub ahead of print]. doi: 10.3233/JPD-223212
Mizrahi, S., Yefenof, E., Gross, M., Attal, P., Ben Yaakov, A., Goldman-Wohl, D., et al. (2007). A phenotypic and functional characterization of NK cells in adenoids. J. Leukocyte Biol. 82, 1095–1105. doi: 10.1189/jlb.0407205
Murdock, B. J., Bender, D. E., Kashlan, S. R., Figueroa-Romero, C., Backus, C., Callaghan, B. C., et al. (2016). Increased ratio of circulating neutrophils to monocytes in amyotrophic lateral sclerosis. Neurol. Neuroimmunol. Neuroinflamm. 3:e242. doi: 10.1212/NXI.0000000000000242
Murdock, B. J., Famie, J. P., Piecuch, C. E., Raue, K. D., Mendelson, F. E., Pieroni, C. H., et al. (2021). NK cells associate with ALS in a sex- and age-dependent manner. JCI Insight 6:e147129. doi: 10.1172/jci.insight.147129
Murdock, B. J., Zhou, T., Kashlan, S. R., Little, R. J., Goutman, S. A., and Feldman, E. L. (2017). Correlation of peripheral immunity with rapid amyotrophic lateral sclerosis progression. JAMA Neurol. 74, 1446–1454.
Nicolas, A., Kenna, K. P., Renton, A. E., Ticozzi, N., Faghri, F., Chia, R., et al. (2018). Genome-wide analyses identify KIF5A as a novel ALS gene. Neuron 97, 1268.e6–1283.e6. doi: 10.1016/j.neuron.2018.02.027
Oakes, J. A., Davies, M. C., and Collins, M. O. (2017). TBK1: a new player in ALS linking autophagy and neuroinflammation. Mol. Brain 10:5. doi: 10.1186/s13041-017-0287-x
O′Brien, K. L., and Finlay, D. K. (2019). Immunometabolism and natural killer cell responses. Nat. Rev. Immunol. 19, 282–290.
Orrù, V., Steri, M., Sidore, C., Marongiu, M., Serra, V., Olla, S., et al. (2020). Complex genetic signatures in immune cells underlie autoimmunity and inform therapy. Nat. Genet. 52, 1036–1045. doi: 10.1038/s41588-020-0684-4
Phillips, J. H., Le, A. M., and Lanier, L. L. (1984). Natural killer cells activated in a human mixed lymphocyte response culture identified by expression of Leu-11 and class II histocompatibility antigens. J. Exp. Med. 159, 993–1008. doi: 10.1084/jem.159.4.993
Poli, A., Kmiecik, J., Domingues, O., Hentges, F., Bléry, M., Chekenya, M., et al. (2013). NK cells in central nervous system disorders. J. Immunol. 190, 5355–5362. doi: 10.4049/jimmunol.1203401
Rolfes, L., Schulte-Mecklenbeck, A., Schreiber, S., Vielhaber, S., Herty, M., Marten, A., et al. (2021). Amyotrophic lateral sclerosis patients show increased peripheral and intrathecal T-cell activation. Brain Commun. 3:fcab157. doi: 10.1093/braincomms/fcab157
Rothstein, J. D. (2017). Edaravone: a new drug approved for ALS. Cell 171:725. doi: 10.1016/j.cell.2017.10.011
Sanmarco, L. M., Wheeler, M. A., Gutiérrez-Vázquez, C., Polonio, C. M., Linnerbauer, M., Pinho-Ribeiro, F. A., et al. (2021). Gut-licensed IFNγ(+) NK cells drive LAMP1(+)TRAIL(+) anti-inflammatory astrocytes. Nature 590, 473–479. doi: 10.1038/s41586-020-03116-4
Sheean, R. K., McKay, F. C., Cretney, E., Bye, C. R., Perera, N. D., Tomas, D., et al. (2018). Association of regulatory T-cell expansion with progression of amyotrophic lateral sclerosis: a study of humans and a transgenic mouse model. JAMA Neurol. 75, 681–689. doi: 10.1001/jamaneurol.2018.0035
Song, S., Miranda, C. J., Braun, L., Meyer, K., Frakes, A. E., Ferraiuolo, L., et al. (2016). Major histocompatibility complex class I molecules protect motor neurons from astrocyte-induced toxicity in amyotrophic lateral sclerosis. Nat. Med. 22, 397–403. doi: 10.1038/nm.4052
Thonhoff, J. R., Simpson, E. P., and Appel, S. H. (2018). Neuroinflammatory mechanisms in amyotrophic lateral sclerosis pathogenesis. Curr. Opin. Neurol. 31, 635–639. doi: 10.1097/WCO.0000000000000599
Vitale, M., Bottino, C., Sivori, S., Sanseverino, L., Castriconi, R., Marcenaro, E., et al. (1998). NKp44, a novel triggering surface molecule specifically expressed by activated natural killer cells, is involved in non-major histocompatibility complex-restricted tumor cell lysis. J. Exp. Med. 187, 2065–2072. doi: 10.1084/jem.187.12.2065
Volpe, C. M., and Nogueira-Machado, J. A. (2015). Is innate immunity and inflammasomes involved in pathogenesis of amyotrophic lateral sclerosis (ALS)? Recent Patents Endocr. Metab. Immune Drug Discov. 9, 40–45. doi: 10.2174/1872214809666150407111420
Wang, S., and van de Pavert, S. A. (2022). Innate lymphoid cells in the central nervous system. Front. Immunol. 13:837250. doi: 10.3389/fimmu.2022.837250
Xia, K., Zhang, L., Tang, L., Huang, T., and Fan, D. (2022). Assessing the role of blood pressure in amyotrophic lateral sclerosis: a mendelian randomization study. Orphanet J. Rare Dis. 17:56. doi: 10.1186/s13023-022-02212-0
Yano, N., Endoh, M., Nomoto, Y., Sakai, H., and Rifai, A. (1996). Increase of HLA-DR-positive natural killer cells in peripheral blood from patients with IgA nephropathy. Hum. Immunol. 49, 64–70. doi: 10.1016/0198-8859(96)00057-2
Yu, W., He, J., Cai, X., Yu, Z., Zou, Z., and Fan, D. (2022). Neuroimmune crosstalk between the peripheral and the central immune system in amyotrophic lateral sclerosis. Front. Aging Neurosci. 14:890958. doi: 10.3389/fnagi.2022.890958
Keywords: amyotrophic lateral sclerosis (ALS), innate immunity, natural killer cells, Mendelian randomization (MR), genome-wide association study (GWAS)
Citation: Gong Z, Liu Y, Ding F, Ba L and Zhang M (2022) Natural killer cells-related immune traits and amyotrophic lateral sclerosis: A Mendelian randomization study. Front. Neurosci. 16:981371. doi: 10.3389/fnins.2022.981371
Received: 29 June 2022; Accepted: 31 August 2022;
Published: 29 September 2022.
Edited by:
Ji He, Peking University Third Hospital, ChinaReviewed by:
Andrea Markovinovic, King’s College London, United KingdomFabiola De Marchi, Azienda Ospedaliero Universitaria Maggiore della Carità, Italy
Copyright © 2022 Gong, Liu, Ding, Ba and Zhang. This is an open-access article distributed under the terms of the Creative Commons Attribution License (CC BY). The use, distribution or reproduction in other forums is permitted, provided the original author(s) and the copyright owner(s) are credited and that the original publication in this journal is cited, in accordance with accepted academic practice. No use, distribution or reproduction is permitted which does not comply with these terms.
*Correspondence: Li Ba, YmFsaTIwMTVAMTI2LmNvbQ==; Min Zhang, emhhbmdfbWluXzM0NjRAMTI2LmNvbQ==