- 1School of Chemistry, Chemical Engineering and Life Sciences, Wuhan University of Technology, Wuhan, China
- 2School of Public Health, Tongji Medical College, Huazhong University of Science and Technology, Wuhan, China
- 3State Key Laboratory of Advanced Technology for Materials Synthesis and Processing, Wuhan University of Technology, Wuhan, China
Schizophrenia (SCZ) is a serious mental illness that affects 1% of people worldwide. SCZ is associated with a higher risk of developing metabolic disorders such as obesity. Antipsychotics are the main treatment for SCZ, but their side effects include significant weight gain/obesity. Despite extensive research, the underlying mechanisms by which SCZ and antipsychotic treatment induce weight gain/obesity remain unclear. Hypothalamic endoplasmic reticulum (ER) stress is one of the most important pathways that modulates inflammation, neuronal function, and energy balance. This review aimed to investigate the role of hypothalamic ER stress in SCZ and antipsychotic-induced weight gain/obesity. Preliminary evidence indicates that SCZ is associated with reduced dopamine D2 receptor (DRD2) signaling, which significantly regulates the ER stress pathway, suggesting the importance of ER stress in SCZ and its related metabolic disorders. Antipsychotics such as olanzapine activate ER stress in hypothalamic neurons. These effects may induce decreased proopiomelanocortin (POMC) processing, increased neuropeptide Y (NPY) and agouti-related protein (AgRP) expression, autophagy, and leptin and insulin resistance, resulting in hyperphagia, decreased energy expenditure, and central inflammation, thereby causing weight gain. By activating ER stress, antipsychotics such as olanzapine activate hypothalamic astrocytes and Toll-like receptor 4 signaling, thereby causing inflammation and weight gain/obesity. Moreover, evidence suggests that antipsychotic-induced ER stress may be related to their antagonistic effects on neurotransmitter receptors such as DRD2 and the histamine H1 receptor. Taken together, ER stress inhibitors could be a potential effective intervention against SCZ and antipsychotic-induced weight gain and inflammation.
Introduction
Schizophrenia (SCZ) is a severe mental disorder that affects approximately 1% of people worldwide. This disease is characterized by positive symptoms (e.g., hallucinations, delusion, thought disturbances, and disorganized speech and behavior); negative symptoms (e.g., poverty of speech, anhedonia, and apathy); and cognitive impairment associated with memory, attention, and executive function (McCutcheon et al., 2020). These symptoms impair social and occupational functioning and increase the risk for suicide, substantially reducing the lifespan of patients with SCZ. A body of evidence has revealed that dysregulation of neurotransmission pathways such as dopaminergic, glutamatergic, gamma-aminobutyric acid (GABA)-ergic, serotonergic, opioid, and cholinergic plays an important role in the pathogenesis of SCZ (McCutcheon et al., 2020). However, the etiology of SCZ remains elusive and new therapeutic strategies are urgently needed. Additionally, clinical evidence has consistently demonstrated that patients with SCZ have a higher risk of developing metabolic disorders such as obesity, glucose metabolic disorders, dyslipidemia, and hyperinsulinemia (Harris et al., 2013). Numerous studies have demonstrated that these metabolic side effects are mainly caused by antipsychotic drug treatment. However, growing evidence has revealed that patients with SCZ have an intrinsic metabolic risk (Freyberg et al., 2017). Researchers have reported that drug-naïve patients or patients from the pre-medication era have metabolic disorders such as a higher body mass index (BMI), waist circumference, and low-density lipoprotein (LDL) levels; hyperinsulinemia; and abnormalities in insulin secretion (Harris et al., 2013; Freyberg et al., 2017). There have been similar findings in youth (12–17 years old) compared with controls. Drug-naïve patients experiencing their first psychotic episode have a larger waist circumference and LDL levels (Jensen et al., 2017). However, the mechanisms underlying these abnormalities are not clear. The hypothalamus is well known to control a variety of body functions such as hunger, energy expenditure, the sleep/wake cycle, stress response, and reproduction, among others. Research suggests that the hypothalamus also mediates cognitive performance and psychosocial health (Burdakov and Peleg-Raibstein, 2020). Accumulated evidence indicates that patients with SCZ have abnormalities in gross anatomic regions (hypothalamus, the third ventricle, and the hypothalamic subregions such as the paraventricular nucleus [PVN]) as well as changes at the cellular level (reduction of PVN neurons, peptides, and neurotransmitters) (Bernstein et al., 2021). A recent clinical study reported a reduction in hypothalamic dopamine D2/D3 receptor (DRD2/DRD3) availability (examined by 18F-fluorodeoxyglucose and 18F-fallypride positron emission tomography [PET] imaging) in unmedicated patients with SCZ (Mitelman et al., 2020), suggesting the involvement of hypothalamic DRD2/DRD3 signaling in SCZ. Reduced DRD2 signaling in the hypothalamus is known to increase food intake, body weight, and regulate glucose metabolism (Ikeda et al., 2020), suggesting that the reduced DRD2 signaling may also be related to SCZ-related metabolic disorders such as increased BMI and abnormalities in glucose metabolism. However, the mechanisms are currently incompletely understood.
Antipsychotics are the mainstay of treatment for SCZ. However, almost all antipsychotics are associated with varying degrees of weight gain and even obesity (Barton et al., 2020). Clinical studies have reported weight gain ranging from 0.09 to 12.4 kg in patients taking antipsychotics such as olanzapine, clozapine, quetiapine, ziprasidone, risperidone, and haloperidol for 6 weeks to 1 year (Nasrallah, 2008; Barton et al., 2020). The weight gain liability of antipsychotics is clozapine ≈ olanzapine > zotepine > quetiapine > risperidone > ziprasidone > aripiprazole (Nasrallah, 2008; Stogios et al., 2022). Olanzapine is one of the most obesogenic antipsychotics. Previous studies have shown that approximately 67–90% of patients taking olanzapine gain at least 3.3–12 kg of body weight after 8 weeks to 12 months of olanzapine treatment (Eder et al., 2001; Nasrallah, 2008). Antipsychotic-induced weight gain/obesity is an important risk factor for type II diabetes, cardiovascular disease, stroke, and patient noncompliance, and leads to decreased life expectancy and increased mortality in patients with SCZ.
Significant efforts have been made to uncover the underlying mechanisms of antipsychotic-induced weight gain/obesity. Several neurotransmitter receptors that regulate food intake and energy expenditure are involved in antipsychotic-induced weight gain including the histamine H1 receptor (H1R), the serotonin 2C receptor (5-HT2CR), DRD2, the α2 adrenergic receptor, the muscarinic M3 receptor (M3R), the cannabinoid type 1 receptor (CB1R), the GABA type A receptor, and the melanocortin 4 receptor (MC4R) (Nasrallah, 2008). It has been reported that olanzapine, clozapine, risperidone, or quetiapine could block hypothalamic H1R or 5-HT2CR, thereby activating adenosine 5′-monophosphate (AMP)-activated protein kinase (AMPK) signaling and increasing neuropeptide Y (NPY) expression, which in turn leads to increased food intake, weight gain, and glucose intolerance (Ikegami et al., 2013; He et al., 2014; Wan et al., 2020). Another study reported that acute olanzapine and clozapine treatment tends but does not significantly induce AMPK activation in the hypothalamus (Fernø et al., 2011). However, pharmacological studies have shown that H1R agonists such as 2-((3-trifluoromethyl)phenyl) histamine dimaleate (FMPH) cannot pass through the brain-blood barrier (BBB) (Malmberg-Aiello et al., 1998), which has limited the use of H1R agonists in reducing antipsychotic-induced weight gain. Activation of hypothalamic H1R by using betahistine, an H1R agonist/H3R antagonist, partially inhibits olanzapine-induced weight gain in patients (Poyurovsky et al., 2013) and rodents (Deng et al., 2012). Our recent study reported that gold nanoclusters (AuNCs) could eliminate olanzapine-induced food intake and obesity in rats partly via affecting H1R-AMPK signaling (He et al., 2022b). Moreover, a 5-HT2CR-specific agonist, lorcaserin, decreases risperidone- and olanzapine-induced overeating and weight gain in rats (Lord et al., 2017; Wan et al., 2020). Co-treatment with cevimeline, an M3R agonist, attenuates olanzapine-induced weight gain via M3R-AMPK signaling in female rats (Han et al., 2022). Olanzapine treatment also decreases CB1R expression in the dorsal vagal complex (DVC) and the hypothalamus (Weston-Green et al., 2012; Lazzari et al., 2017). Co-treatment with a neutral CB1R antagonist and an inverse agonist, rimonabant and NESS06SM, respectively, significantly reduces olanzapine-induced weight gain in rats (Lazzari et al., 2017).
Antipsychotic-induced weight gain/obesity is also associated with several neuropeptides or hormones that regulate energy balance and neuroendocrine function such as proopiomelanocortin (POMC), NPY, AgRP, insulin, leptin, and ghrelin (Ballon et al., 2014). Olanzapine, clozapine, and risperidone treatment in rodents has been associated with increased expression of NPY and AgRP or decreased expression of POMC in the hypothalamus (Kirk et al., 2006; Fernø et al., 2011; Lian et al., 2015). Infusing an MC4R agonist, setmelanotide, reduces hyperphagia in risperidone-fed mice (Li L. et al., 2021). In rats, olanzapine treatment upregulates the messenger RNA (mRNA) and protein expression of growth hormone secretagogue receptor 1a (GHS-R1a), a receptor of ghrelin in the hypothalamus (Zhang et al., 2014). Cerebroventricular injection of a GHS-R1a antagonist, D-Lys3-GHRP-6, suppresses olanzapine-induced hyperphagia in rats, suggesting the involvement of ghrelin signaling in antipsychotic-induced weight gain (Zhang et al., 2014). A recent study reported that olanzapine activates hypothalamic NPY-AMPK signaling by disrupting the GHSR-H1R interaction, and this effect contributes to olanzapine-induced weigh gain (Chen et al., 2020). Antipsychotic-induced weight gain is associated with glucose metabolism disorders (Zhang et al., 2017a). Olanzapine, clozapine, risperidone, and quetiapine treatment has been associated with weight gain and abnormal blood glucose and leptin levels in patients (Doane et al., 2022) and rodents (Cope et al., 2005; Kirk et al., 2009). Anti-diabetic drugs such as metformin partially reduce olanzapine- or clozapine-induced weight gain in patients (Ellul et al., 2018) and rodents (Hu et al., 2014). Interestingly, evidence suggests that gut microbiome alterations are largely associated with the pathophysiology of SCZ as well as antipsychotic-induced obesity (Singh et al., 2022). Indeed, obesogenic antipsychotic treatment causes gut microbiota imbalance in both rodents and humans (Singh et al., 2022). Co-administration of the prebiotic Bimuno galacto-oligosaccharides (B-GOS®) alleviates olanzapine-induced weight gain in rats (Kao et al., 2018). Despite significant progress on the underlying mechanisms of antipsychotic-induced weight gain, there is still a lack of effective drugs for the treatment of antipsychotic-induced obesity.
Endoplasmic reticulum (ER) stress refers to physiological or pathological states in which proteins are over unfolded or misfolded in the ER. Hypothalamic ER stress plays a critical role in mediating neuroinflammation and neuronal injury (Yi et al., 2019), as well as regulating food intake, energy expenditure, and body weight (Ramírez and Claret, 2015). Several studies have investigated the association between ER stress and SCZ and have reported that genotypes of ER stress-related genes, including X-box-binding protein 1 (XBP-1) 116C/G and 197C/G, are causative factors of SCZ (Chen et al., 2004; Kakiuchi et al., 2004). An ER stress inhibitor 4-phenylbutyric acid (4-PBA) has been suggested as an important therapy to treat SCZ-related manifestations (Patel et al., 2017). The alterations of ER stress in the hypothalamus of patients with SCZ are not clear. SCZ has been reported to be accompanied by reduced hypothalamic DRD2 signaling (Mitelman et al., 2020), which is largely implicated in ER stress (Song et al., 2017), suggesting that hypothalamic ER stress may be involved in SCZ pathology and its associated metabolic disturbances. Moreover, the most widely used but obesogenic antipsychotics such as olanzapine induce activation of hypothalamic ER stress in rodents (He et al., 2019). Inhibition of ER stress suppresses olanzapine-induced hyperphagia and weight gain (He et al., 2019). These findings suggest the importance of hypothalamic ER stress in obesity induced by antipsychotics such as olanzapine. This review systematically elucidates the association between hypothalamic ER stress in SCZ and antipsychotic-induced weight gain/obesity, discussing the possible underlying molecular mechanisms and providing insights into the search for targets that could alleviate SCZ and antipsychotic-induced obesity.
The role of hypothalamic endoplasmic reticulum stress in body weight control
The hypothalamus is an indispensable “headquarters” for regulating energy homeostasis (Tran et al., 2022). It contains a number of nuclei such as the arcuate nucleus (ARC), the PVN, the ventromedial hypothalamus (VMH), the dorsomedial hypothalamus (DMH), and the lateral hypothalamus (LH) to respond to hormones and nutrients and to regulate food intake and energy expenditure. The ARC which contains two major populations of “first-order” neurons with opposing effects on energy homeostasis: POMC neurons that express the anorexigenic peptides POMC and cocaine- and amphetamine-regulated transcript, and NPY/AgRP neurons that express the orexigenic peptides NPY and AgRP (Vohra et al., 2022). NPY/AgRP and POMC neurons send projections to “second-order” neurons in other hypothalamic regions such as the VMH, PVN, and LH, regulating body weight and glucose homeostasis (Vohra et al., 2022). Leptin and insulin are two important hormones that inhibit food intake and body weight by acting on the hypothalamus. Insulin and leptin receptors are expressed on POMC and AgRP neurons (Shin et al., 2017; Üner et al., 2019). By binding to their receptors, insulin and leptin directly stimulate anorexigenic POMC neurons and inhibit orexigenic NPY/AgRP neurons in the ARC to suppress food intake and to increase energy expenditure. Treatment with leptin or insulin increases POMC expression but decreases the release of AgRP (Breen et al., 2005). Mechanically, leptin and insulin reduce food intake and stimulate energy expenditure partly through AMPK, phosphoinositide 3-kinase (PI3K), suppressor of cytokine signaling 3 (SOCS3), protein tyrosine phosphatase 1B (PTP1B), and mammalian target of rapamycin (mTOR) signaling in the hypothalamus (Thon et al., 2016), and these effects play an essential role in leptin- and insulin-derived AgRP and POMC expression. Furthermore, other neurons such as dopaminergic and histaminergic neurons in the hypothalamus play an important role in regulating food intake and weight gain (Panula et al., 2000; Yonemochi et al., 2019). Hypothalamic dopamine suppresses food intake and weight gain by acting on postsynaptic DRD2 (Meguid et al., 2000). Hypothalamic histamine inhibits food intake and weight gain by activating H1R in the ARC and PVN (Panula et al., 2000). It has been reported that leptin and insulin regulate food intake and body weight partly by communicating with the hypothalamic DRD2 (Kleinridders and Pothos, 2019) and H1R pathways (Yoshimatsu et al., 1999).
In addition to neurons, growing evidence indicates that hypothalamic glia cells such as the astrocytes and microglia have a critical role in body weight regulation. Hypothalamic astrocytes and microglia accumulate and are activated in response to high-fat diet (HFD) (Zhang et al., 2017b; Valdearcos et al., 2019). Pharmacological deletion of astrocytes and microglia or reducing their capability for activating inflammation in the hypothalamus reduces food intake and weight gain and enhances leptin signaling in HFD-fed rodents (Valdearcos et al., 2019; Varela et al., 2021). Reactive astrocytes and microglia activate inflammatory signals such as inhibitor of nuclear factor kappa-B kinase subunit beta (IKKβ)/nuclear factor κB (NF-κB) signaling and produce inflammatory cytokines during HFD feeding (Valdearcos et al., 2019; Sa et al., 2022). Moreover, hypothalamic astrocytes reduce ghrelin-induced food intake by affecting AgRP neurons (Yang L. et al., 2015). Deletion of angiopoietin-like 4 in astrocytes increases the excitability and insulin sensitivity of POMC neurons and alleviates HFD-induced weight gain in mice (Varela et al., 2021). These findings suggest that astrocytes may partly regulate food intake and body weight by interacting with POMC and AgRP neurons.
Hypothalamic neuronal endoplasmic reticulum stress and weight gain
Endoplasmic reticulum stress is an adaptive mechanism that refers to the pathophysiological process in which ER function is disturbed under conditions of hypoxia, oxidative stress, nutritional imbalance, or calcium homeostasis dysfunction (Schönthal, 2012). Mechanically, ER stress is mediated by protein kinase R-like ER kinase (PERK), activating transcription factor 6 (ATF6), and inositol requiring enzyme 1 (IRE1) (Cnop et al., 2012). Under unstressed conditions, these proteins bind to the chaperone BiP, also known GRP78 (Cnop et al., 2012). When ER stress is activated, GRP78 dissociates from PERK, IRE1, and ATF6. PERK is subsequently activated by phosphorylation. PERK phosphorylates the downstream eukaryotic initiation factor 2α (eIF2α) to increase the translation of activating transcription factor 4 (ATF4). Upon ER stress, ATF6 relocated to the Golgi apparatus and is processed by site 1 and 2 proteases; these changes form active ATF6 (p50), which is then transported to the nucleus to activate gene transcription (Matos et al., 2015). IRE1 could be activated by phosphorylation. Phospho-IRE1 cleaves 26 nucleotides from the mRNA of XBP1 and produces an active spliced form of XBP1 (Park et al., 2021). Then, these proteins activate comprehensive transcriptional and translational signaling, resulting in up- or downregulation of their downstream expression of signaling pathways (Kroeger et al., 2019; Figure 1).
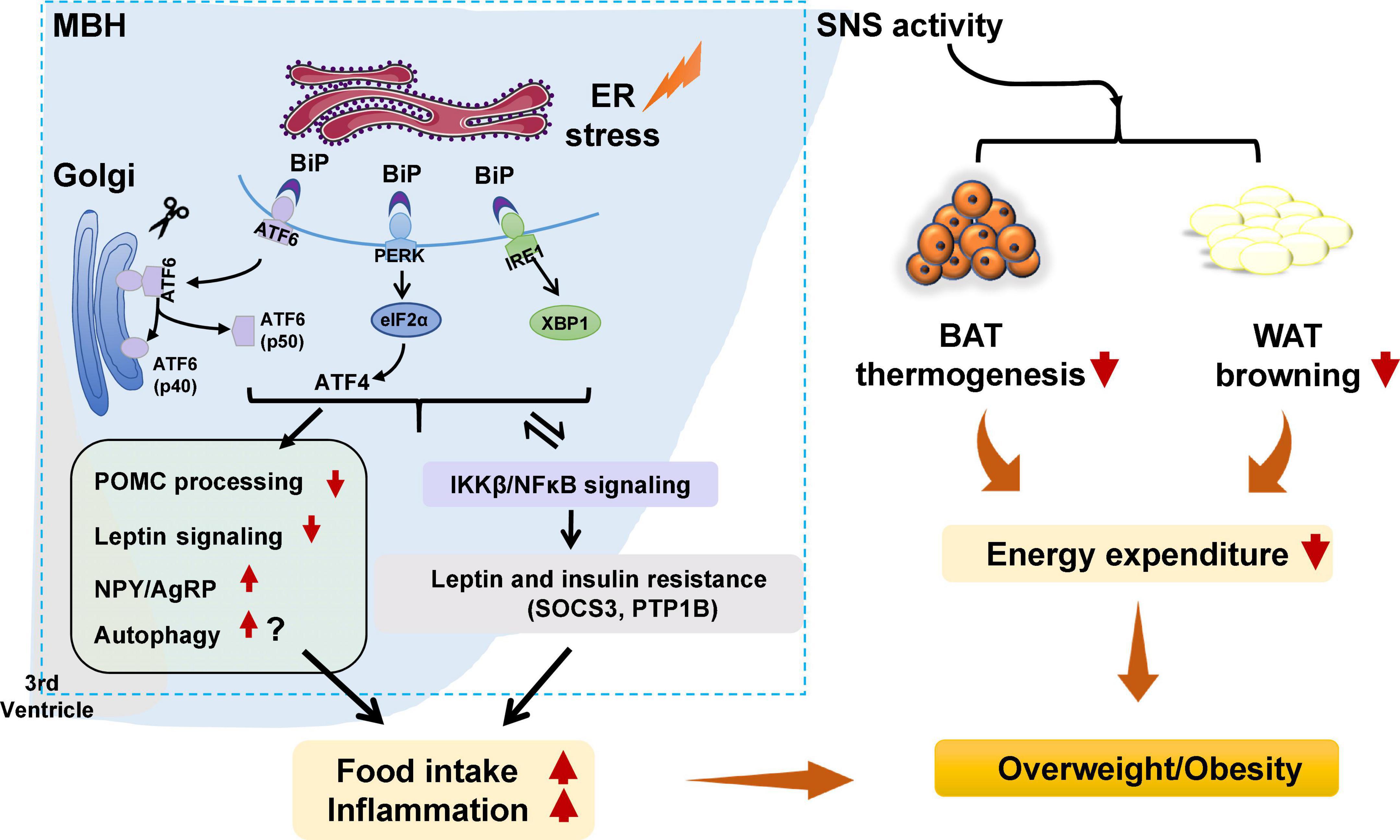
Figure 1. Potential role of hypothalamic endoplasmic reticulum (ER) stress in obesity and inflammation. Under certain stimuli, such as high-fat diet (HFD) feeding, drug treatment, or infection, ER stress is activated, and binding immunoglobulin protein (BiP) dissociates from protein kinase R-like ER kinase (PERK), inositol requiring enzyme 1 (IRE1), and activating transcription factor 6 (ATF6), resulting in the release of those three proteins. PERK is then activated by phosphorylation and p-PERK phosphorylates eukaryotic initiation factor 2α (eIF2α) and increases the translation of ATF4. ATF6 relocates to the Golgi apparatus and is processed by site 1 and 2 proteases, resulting in ATF6 activation. IRE1 is activated by phosphorylation. pIRE1 catalyzes X-box-binding protein 1 (XBP1) mRNA splicing, resulting in increased production of active spliced XBP1. These effects could (1) activate the hypothalamic autophagy signaling, impede proopiomelanocortin (POMC) processing, attenuate leptin signaling, and possibly increase neuropeptide Y (NPY), and agouti-related peptide (AgRP) expression, leading to increased food intake, decreased energy expenditure, inflammation, and weight gain; (2) decrease white adipose tissue (WAT) browning and brown adipose tissue (BAT) thermogenesis by affecting sympathetic nervous system (SNS) activity, resulting in lower energy expenditure and weight gain; and (3) trigger activation of the inhibitor of nuclear factor kappa-B kinase subunit beta (IKKβ)/nuclear factor κB (NF-κB) signaling pathway, resulting in hypothalamic leptin and insulin resistance (by affecting suppressor of cytokine signaling 3 [SOCS3] and protein tyrosine phosphatase 1B [PTP1B]). These effects could increase food intake, decrease energy expenditure, and promote inflammation, thus resulting in weight gain. Furthermore, activated IKKβ/NF-κB signaling could lead to ER stress in the hypothalamus and worsen hypothalamic inflammation.
Hypothalamic ER stress plays a crucial role in the development of obesity (Table 1). Mice with diet-induced obesity (DIO) have increased expression of hypothalamic ER stress markers, including phospho-PERK (p-PERK), phospho-eukaryotic initiation factor-2α (p-eIF2α), and phospho-IRE1 (p-IRE1) (Won et al., 2009; Cakir et al., 2013). Overnutrition activates hypothalamic PERK and promotes leptin resistance during obesity development in mice (Zhang et al., 2008). Pharmacologic activation of hypothalamic ER stress in rats results in hyperphagia and weight gain, and promotes leptin resistance (Ozcan et al., 2009). On the contrary, suppression of hypothalamic ER stress with ER stress inhibitors including tauroursodeoxycholic acid (TUDCA) or 4-PBA suppresses food intake in DIO mice (Ozcan et al., 2006). The mechanisms by which ER stress mediates feeding and body weight are not fully understood. Previous studies suggest that hypothalamic POMC neurons may be important response neurons. Diet-induced ER stress obstructs post-translational processing of POMC in mice (Cakir et al., 2013). Deletion of ATF4 from hypothalamic POMC neurons protects mice from obesity, glucose intolerance, and leptin resistance during HFD feeding (Xiao et al., 2017b). ATF4 also impairs hypothalamic α-melanocyte-stimulating hormone (α-MSH) production (Xiao et al., 2017a). Hypothalamic induction of ER stress attenuates POMC processing and decreases α-MSH levels by decreasing pro-converting enzyme 2 (Cakir et al., 2013). Hypothalamic ER stress could favor a positive energy balance by attenuating its response to an anorectic hormone leptin, whose receptors are expressed on POMC neurons (Cakir and Nillni, 2019). Activation of ER stress inhibits acute leptin signaling in arcuate POMC neurons (Williams et al., 2014). Constitutive expression of a dominant XBP1s form in POMC neurons protects mice from gaining weight by increasing energy expenditure and leptin sensitivity (Williams et al., 2014). Moreover, a recent study reported that genetic deletion of autophagy in POMC neurons of ob/ob mice worsens hyperphagia and reduces POMC neuronal projections to the PVN, characterized by less dense α-MSH-immunoreactive fibers (Park et al., 2020). The mechanisms by which autophagy reduces POMC neurons appear to involve inhibition of mTOR signaling, which is important for regulating cell growth and proliferation (Jaboin et al., 2007). Reduced POMC neuron projections would result in decreased expression of POMC and POMC-derived peptides such as α-MSH, leading to leptin resistance, hyperphagia, and weight gain (Oh et al., 2016). Neonatal TUDCA treatment ameliorates loss of autophagy-induced hyperphagia, weight gain, and the reduction in POMC neuronal projections is ameliorated (Park et al., 2020), suggesting that the ER stress-autophagy pathway in POMC neurons controls hypothalamic development and energy balance (Figure 1). Therefore, hypothalamic ER stress could mediate food intake and body weight by reducing POMC processing, activating autophagy, and inhibiting leptin signaling (Figure 1). Furthermore, ER stress could regulate food intake by affecting NPY/AgRP expression and neuronal function. NPY and AgRP are expressed in particular hypothalamic neurons that play an important role in feeding control (Morton and Schwartz, 2001). Induction of ER stress by tunicamycin significantly increases NPY and AgRP mRNA in the mouse hypothalamus (Ozcan et al., 2009). In AgRP neurons, RNA-seq revealed that ER-stress-related genes such as BiP and ATF6 are significantly activated during food deprivation (Henry et al., 2015). ATF4 knockout in AgRP neurons protects mice from weight gain by decreasing food intake and increasing energy expenditure (Deng et al., 2017). Additionally, in rodents fed a HFD, inhibition of ER stress reduces orexigenic NPY gene expression in the amygdala, suggesting a role for brain ER stress-NPY in regulating feeding (Areias and Prada, 2015). These findings suggest that ER stress could also regulate food intake via affecting AgRP and NPY.
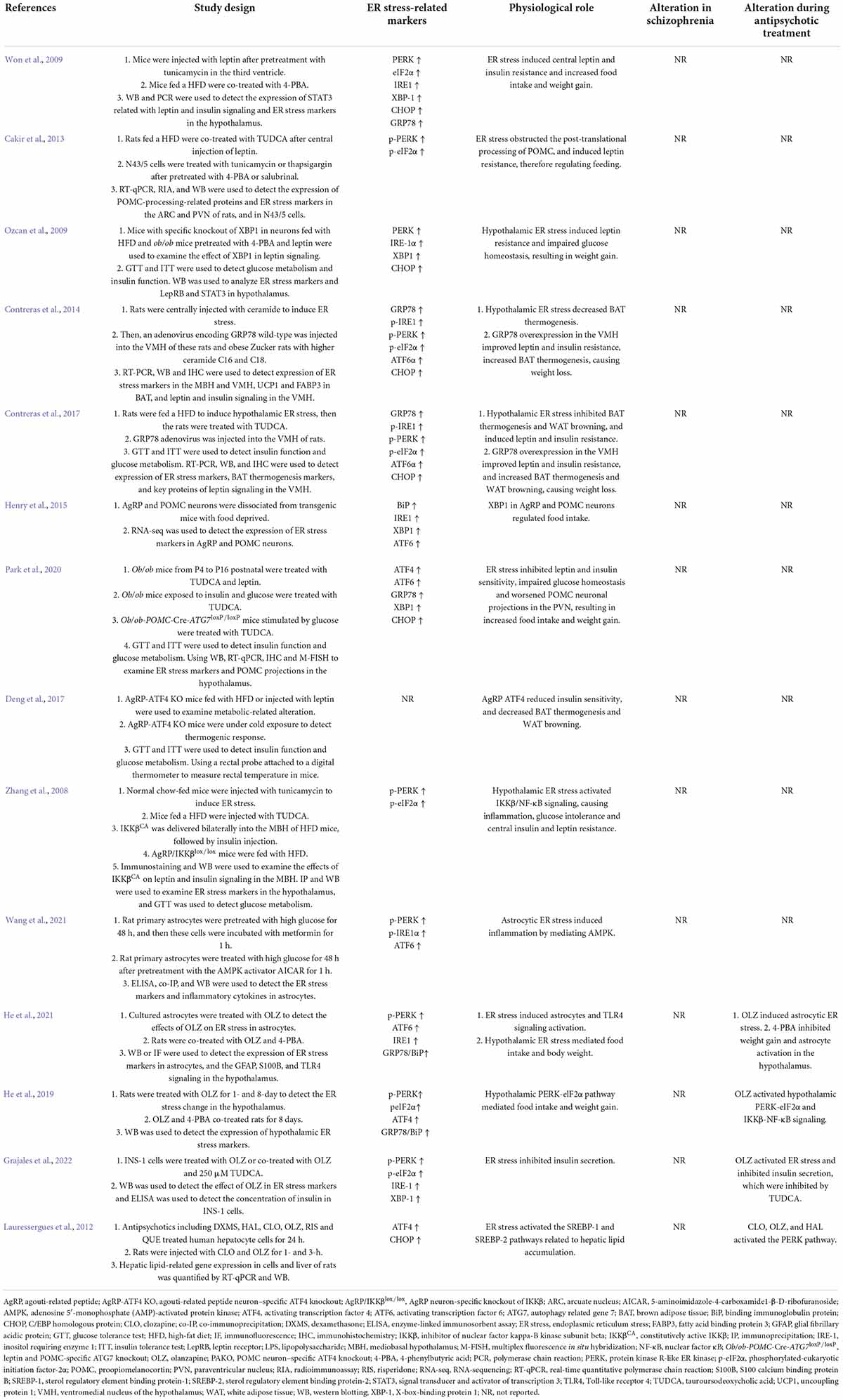
Table 1. Findings highlighting role of hypothalamic ER stress-related proteins in mediating appetite and weight gain and their modifications by antipsychotic drugs.
The role of ER stress in other hypothalamic neuronal systems involved in metabolism and body weight regulation have not been studied extensively. The available evidence suggests that ER stress is related to several neurotransmitter receptors that play essential roles in regulating food intake and body weight such as DRD2, H1R, and CB1R. One study reported that the DRD2 agonist (bromocriptine) decreases the expression of GRP78/BiP in cultured cells, indicating that ER stress could be inhibited by DRD2 activation (Henderson et al., 2021). A study also reported that H1R antagonism induces ER stress in cultured cells (Jakhar et al., 2016). The CB1R agonist arachidonyl-2′-chloroethylamide (ACEA) attenuates ER stress and inflammation in Neuro-2a neuroblastoma cells (Vrechi et al., 2018). These findings suggest that the ER stress could be the downstream effect of DRD2, H1R, and CB1R signaling. Future studies should investigate whether hypothalamic ER stress could be mediated by altering hypothalamic DRD2, H1R, and CB1R signaling and its potential role in food intake and body weight regulation.
Hypothalamic ER stress also drives obesity by reducing energy expenditure (Table 1). Pharmacological activation of ER stress in the hypothalamus by ceramides effectively reduces brown adipose tissue (BAT) thermogenesis, resulting in weight gain (Contreras et al., 2014). Genetic overexpression of GRP78 in the VMH reduces ceramide-induced ER stress and increases BAT thermogenesis, resulting in weight loss in rats (Contreras et al., 2014). In HFD-induced obese rats, overexpression of GRP78 in the VMH attenuates ER stress, increases BAT thermogenesis, and stimulates white adipose tissue (WAT) browning, ultimately attenuating HFD-induced obesity (Contreras et al., 2017). Moreover, the study revealed that ER stress in the VMH decreases sympathetic nervous system (SNS) activity to inhibit BAT function and increases WAT browning, and these effects could be independent of feeding and leptin (Contreras et al., 2017). Treatment with TUDCA, an ER stress inhibitor, reduces food intake and tends to increase oxygen consumption in DIO mice (Cakir et al., 2013). Intracerebroventricular (ICV) administration of TUDCA induces weight loss, decreases hypothalamic ER stress, and elevates BAT temperature in rats (González-García et al., 2018). Furthermore, hypothalamic POMC neuron-specific ATF4 knockout protects DIO mice from obesity by increasing BAT thermogenesis and increasing oxygen consumption (Xiao et al., 2017b). ATF4 knockout in AgRP neurons promotes mouse fat loss mainly by increasing energy expenditure (Deng et al., 2017). Induction of XBP1s in POMC neurons significantly increases the metabolic rate to mediate thermogenesis in both BAT and inguinal WAT (Williams et al., 2014), therefore protecting mice against DIO. These findings suggest that hypothalamic ER-stress-induced reductions in energy expenditure may be at least partially related to the hypothalamic POMC and AgRP (Figure 1).
In the pathogenesis of obesity, hypothalamic ER stress is significantly linked to inflammation. Activation of hypothalamic inflammation leads to central metabolic dysregulations, uncoupling of food intake and energy expenditure, and weight gain (Jais and Brüning, 2017; Le Thuc et al., 2017). ER stress markers including PERK, ATF6, and IRE1 participate in activating the inflammatory processes (Garg et al., 2012). In the progression of obesity, a HFD could induce hypothalamic ER stress, and this effect could promote hypothalamic inflammation in mice (Zhang et al., 2008) and rats (Ropelle et al., 2010). Activation of hypothalamic ER stress via overnutrition activates IKKβ-NF-κB signaling, whereas inhibition of ER stress via intraventricular injection of TUDCA suppresses hypothalamic IKKβ-NF-κB signaling, reduces food intake, and induces weight loss in DIO mice (Zhang et al., 2008). This study also revealed that activation of IKKβ-NF-κB signaling by ER stress induces leptin and insulin resistance by affecting SOCS3, and these effects cause energy imbalance and weight gain (Zhang et al., 2008). Interestingly, ER stress is also regulated by inflammation. A study revealed that administration of a small molecule inhibitor of NF-κB, withaferin A (WA), reduces the expression of ER stress hallmarkers including PERK, XBP1, and ATF6 in the mouse pancreas (Kanak et al., 2017). An intraperitoneal injection of active IKKβ (IKKβCA) lentivirus increases phosphorylation of PERK and eIF2α (Zhang et al., 2008). In rats, a single intraperitoneal injection of tumor necrosis factor alpha (TNF-α) increases expression of p-PERK, IRE1α, and GRP78 in the hypothalamus (Denis et al., 2010). Palmitate and TNF-α treatment upregulate NF-κB expression and ER stress-related gene expression in hypothalamic appetite-stimulating NPY/AgRP neurons (Dalvi et al., 2017). Therefore, ER stress and NF-κB signaling positively regulate each other during HFD feeding, and these effects induce a positive energy balance and cause obesity (Zhang et al., 2008; Figure 1).
Hypothalamic astrocytic endoplasmic reticulum stress and weight gain
In addition to ER stress in hypothalamic neurons, ER stress in hypothalamic astrocytes has recently gained attention for its important role in the development of obesity and inflammation (Figure 2). Astrocytes are the most abundant glial cells in the central nervous system (CNS). Numerous studies have demonstrated the importance of hypothalamic astrocytes in regulating feeding (Kim J. G. et al., 2014), energy expenditure (Manaserh et al., 2020), and inflammation (Douglass et al., 2017). For example, fasting activates hypothalamic astrocytes (Chen et al., 2016). Astrocyte insulin receptor deletion in mice reduces energy expenditure and temperature (Manaserh et al., 2020). Researchers have reported that chronic and acute (single) HFD exposure in rodents induce inflammation and astrocytes activation in the hypothalamus (Zhang et al., 2017b; Cansell et al., 2021). Mice fed a HFD for 28 days had increased TNF-α mRNA expression in hypothalamic astrocytes (Sugiyama et al., 2020).
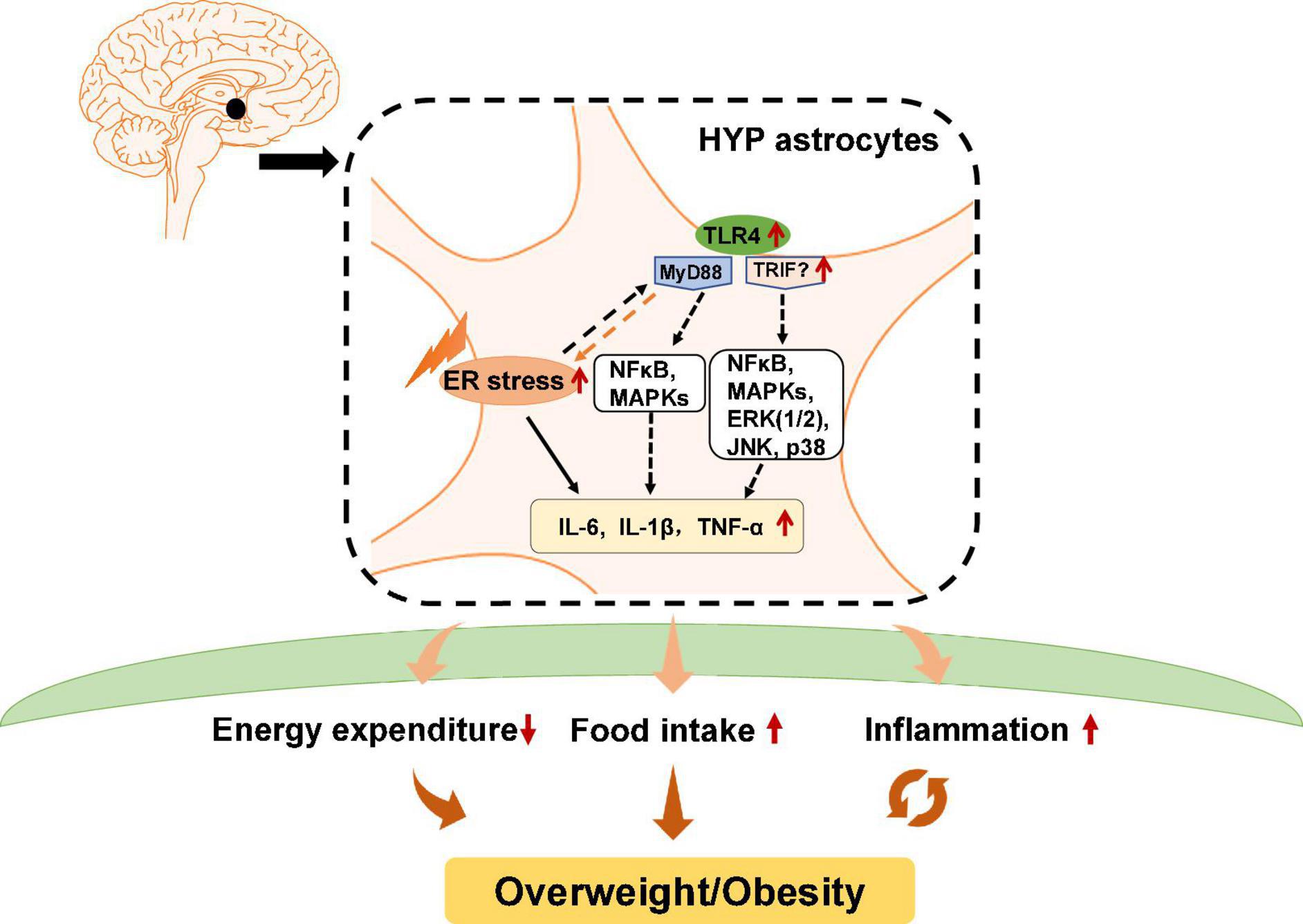
Figure 2. Potential role of astrocytic endoplasmic reticulum (ER) stress and Toll-like receptor 4 (TLR4) signaling in obesity and inflammation. In hypothalamic astrocytes, ER stress may activate TLR4 that then activates myeloid differentiation primary response 88 (MyD88)-independent and MyD88-dependent signaling, which increases the expression of nuclear factor κB (NF-κB), mitogen-activated protein kinases (MAPKs), extracellular signal-regulated kinase 1/2 (ERK1/2), c-Jun N-terminal kinase (JNK), and p38. These effects upregulate pro-inflammatory cytokines such as interleukin 6 (IL-6), IL-1β, and tumor necrosis factor alpha (TNF-α), and causing central inflammation. Activated astrocytic TLR4 signaling could also lead to decreased energy expenditure and promote ER stress activation, which increases food intake, eventually leading to obesity.
Previous studies have reported that in astrocytes, TLR4 signaling plays an essential role in obesity pathogenesis and inflammation (Figure 2). TLR4 is expressed by activated astrocytes (Shen et al., 2016). Growing evidence suggests that astrocytic TLR4 could be activated in response to natural ligands and existing compounds, such as tenascin C, damage-associated molecular patterns (DAMPS), lipopolysaccharide (LPS), and saturated fatty acids (SFA), which are well known to promote inflammation or induce weight gain. For example, tenascin C, an endogenous activator of TLR4, induces IL-6 expression in primary cortical astrocytes (Krasovska and Doering, 2018). LPS, which induces hypothalamic inflammation (Rorato et al., 2017), acts on the TLR4-myeloid differentiation factor 2 (MD2) complex (the binding site of LPS) to activate TLR4 and enhances IL-1β, IL-6, and TNF-α expression (Wu et al., 2012). DAMPs such as the high mobility group box protein 1 (HMGB-1) has been reported to activate TLR4 in mixed cultures of astrocytes and microglia, thereby inducing NF-κB activation (Rosciszewski et al., 2019). SFA, which is known to cause hypothalamic inflammation and obesity, activates TLR4 to induce an inflammatory response (Milanski et al., 2009). Moreover, a clinical study reported that obese patients have increased tenascin C and TLR4 levels in visceral adipose tissue (Catalán et al., 2012). LPS, total free fatty acid levels, and TLR4 mRNA are significantly increased in the plasma of patients with nonalcoholic steatohepatitis (Sharifnia et al., 2015). Serum from obese patients shows TLR4/NF-κB signaling activation in THP-1 monocytes (Yao et al., 2010). SFA induces pro-inflammatory cytokines secretion including TNF-α, IL-6, and IL-8 in human placental cells via activating TLR4 signaling (Yang X. et al., 2015). These findings suggest the importance of TLR4 in the pathogenesis of inflammation and obesity in humans. However, the role of hypothalamic astrocyte TLR4 signaling in inflammation and obesity is not clear in humans.
Toll-like receptor 4 has a cytoplasmic Toll/interleukin-1 receptor/resistance protein (TIR) domain and interacts with two adaptor molecules, namely myeloid differentiation primary response 88 (MyD88) (Medzhitov et al., 1998) and TIR domain-containing adapter protein-inducing interferon-β (TRIF) (Zuany-Amorim et al., 2002). In the MyD88-dependent pathway, TLR4 activation induces early recruitment of MyD88 and rapidly activates NF-κB and mitogen-activated protein kinases (MAPKs), therefore inducing inflammatory factors expression such as TNF-α and interleukin (IL)-1β, IL-6, and IL-15 (Gorina et al., 2011). In the MyD88-independent pathway, TLR4 interacts with TRIF and activates interferon regulatory factor 3 (IRF3), NF-κB, and MAPKs, including extracellular signal-regulated kinase 1/2 (ERK1/2), c-Jun N-terminal kinase (JNK), and p38, among others, thereby inducing the secretion of inflammatory factors such as TNF-α, IL-1β, and IL-6. Pharmacologic inhibition of TLR4, TLR4 knockout, TLR4-interactor knockdown, or hypothalamic ARC-restricted TLR4 knockdown reduces food intake, increases whole-body energy expenditure, reduces hypothalamic inflammation, and protects rodents from HFD-induced obesity (Milanski et al., 2009; Camandola and Mattson, 2017; Zhao et al., 2017; de Vicente et al., 2021). MyD88 deficiency in the mouse CNS (MyD88ΔCNS) or astrocyte-specific MyD88 knockout protects mice from chronic HFD-induced obesity, and mice exhibit ameliorated hypothalamic reactive gliosis and inflammation (Jin et al., 2020). Trif deficient (Trif–/–) mice show increased food intake and weight gain (Yang and Fukuchi, 2020). However, the role of hypothalamic TRIF signaling in food intake and weight gain is unclear. Moreover, activation of astrocytic IKKβ-NF-κB signaling increases fat mass and causes weight gain (Zhang et al., 2017b). Astrocytic IKKβ-NF-κB loss of function counteracts DIO in mice (Zhang et al., 2017b). Astrocyte-specific deletion of IKKβ in mice after 6 weeks of HFD feeding decreases hypothalamic inflammation and astrocytosis in the hypothalamic ARC, therefore reducing food intake and increased energy expenditure (Douglass et al., 2017). These findings suggest that astrocytes may regulate food intake, energy expenditure and inflammation development at least partly via TLR4 signaling, which involves the participation of MyD88, TRIF, and IKKβ-NF-κB signaling (Figure 2).
It is worth noting that TLR4 activation is associated with ER stress. TLR4 activation in HFD-induced obese mice could precede hypothalamic ER stress (Milanski et al., 2009). Inhibition of TLR4 signaling by anti-TLR4 antibodies (Milanski et al., 2009) or TLR4 knockout (Pierre et al., 2013) attenuates ER stress and reduces HFD-induced ER stress. Downstream molecules of TLR4 including MAPK and NF-κB, and the expression of inflammatory factors such as IL-1β and IL-6, could be activated by ER stress (Darling and Cook, 2014; Kim S. et al., 2014). Moreover, ER stress could mediate inflammation via TLR4 signaling during the development of obesity (Li, 2018). HFD-induced obese mice show significantly increased mRNA and protein expression of TLR4, TNF-α, and IL-6 in adipose tissue, while these changes are suppressed by treatment with the ER stress inhibitor 4-PBA (Li, 2018). TUDCA inhibits NF-κB activation in astrocytes induced by combined stimulation of LPS and interferon-γ (IFN-γ) (Yanguas-Casás et al., 2014), suggesting that inhibition of ER stress decreases astrocyte inflammation. These studies clarify the important relationship between ER stress and TLR4 signaling (Figure 2). In conclusion, hypothalamic ER stress in neurons and astrocytes has an important role in the regulation of body weight and inflammatory responses.
While it is known that ER stress induces inflammation in neurons and astrocytes, are these effects dependent or independent? Studies in cultured neurons have revealed that ER stress activates NF-κB signaling and increases expression of pro-inflammatory cytokines, and these effects could be inhibited by co-treatment with ER stress inhibitors such as 4-PBA (Wang et al., 2016; He et al., 2019). Studies in astrocytes have shown the same results: Activation of ER stress triggers an inflammatory response, which is inhibited by co-treatment with ER stress inhibitors (Yanguas-Casás et al., 2014; He et al., 2021). These findings suggest that ER-stress-induced inflammation in neurons and astrocytes could be independent. However, astrocytes and neurons are known to communicate with each other (Paixão and Klein, 2010). Research has proved that ER stress is transmissible between astrocytes and neurons (Sprenkle et al., 2019). ER stress in astrocytes/neurons could regulate inflammatory and ER stress in other astrocytes/neurons (Sprenkle et al., 2019). In astrocytes, ER stress activation augments inflammatory signaling and increases the expression and secretion of pro-inflammatory cytokines such as IL-6 and IL-1β (Wang et al., 2018). The receptors for IL-1β, and TNF-α are expressed on neurons (Friedman, 2001; Chadwick et al., 2008). It is possible that pro-inflammatory cytokines secreted by astrocytes can act on their receptors on neurons to stimulate neuronal ER stress, triggering neuronal inflammatory responses. It has been suggested that overnutrition/HFD activates astrocytes and microglia to release cytokines, and these cytokines then mediate inflammation in POMC and AgRP neurons and cause leptin and insulin resistance, resulting in impaired metabolism and weight gain (Ullah et al., 2021). Furthermore, the receptors for the above pro-inflammatory cytokines are also expressed on astrocytes (Friedman, 2001; Bobbo et al., 2021). Therefore, pro-inflammatory cytokines including IL-6, IL-1β, and TNF-α secreted by neurons may also activate ER stress in astrocytes to induce an inflammatory response. Taken together, during obesity development, hypothalamic inflammation could be a combined effects of ER stress activation in both neurons and astrocytes, and they could both trigger each other.
Besides astrocytes, other glia cells such as microglia, hypothalamic neural stem cells, and NG2 cells play a role in metabolic inflammation. Obesity activates microglia and inflammation in the hypothalamus (Mendes et al., 2018), whereas deletion of hypothalamic microglia abrogates inflammation in rodents (Valdearcos et al., 2014). NG2 glia cells, which express inflammatory cytokines such as IL-1β (Galichet et al., 2021), are increased in the brain of HFD-fed mice (Xiao et al., 2018). Neural stem cells, which express IL-6, IL-1β, and TNF-α (Chang and Kong, 2019), are reduced by HFD exposure via IKKβ/NF-κB signaling (Livesey, 2012). The role of ER stress in mediating inflammation in microglia, N2 glia, and neural stem cells has not been fully studied; and the available information is inconsistent. Increased ER stress in microglia contributes to neuroinflammation induced by paraquat (an herbicide) (Yang et al., 2020). Inhibition of ER stress via TUDCA reduces activation of microglial NF-κB induced by LPS and IFN-γ (Yanguas-Casás et al., 2014). However, LPS-induced increased IL-1β, IL-6, and TNF-α expression in microglia are partially reversed by tunicamycin (an ER stress inducer) (Wang et al., 2017). Inhibition of ER stress by 4-PBA promotes LPS-induced inflammation in cultured microglia (Wang et al., 2017). Furthermore, whether ER stress in hypothalamic microglia, N2 glia, and neural stem cells plays a role in metabolic inflammation and obesity remains to be uncovered. How antipsychotics affect ER stress in these glia cells and its potential role in antipsychotic-related inflammation is unknown. Astrocytes, which make up the largest glial population, are related to ER stress signaling in inflammatory regulation (Martin-Jiménez et al., 2017). Obesogenic antipsychotics such as olanzapine activate astrocytic ER stress, leading to weight gain (He et al., 2021). Therefore, the next section focuses on the role of astrocytic ER stress in antipsychotic-related inflammation and weight gain.
The role of hypothalamic endoplasmic reticulum stress in schizophrenia and antipsychotic-induced weight gain
There are numerous studies reporting that SCZ is associated with metabolic disorders. Almost all antipsychotics cause weight gain/obesity, and this effect is the main cause of metabolic disorders in SCZ patients. Hypothalamic ER stress is well known in regulating food intake, energy expenditure, inflammation, and insulin and leptin signaling (Cakir and Nillni, 2019). In this section, we discuss the relationship between ER stress and SCZ and antipsychotic-induced weight gain.
Insights from clinical studies on endoplasmic reticulum stress in schizophrenia and antipsychotic-induced weight gain
Several studies have investigated the relationship between ER stress and SCZ (Table 2). A study in B lymphocytes from patients with bipolar disorder reported that XBP1 and CHOP are upregulated upon treatment with ER stress inducers (thapsigargin and tunicamycin) (So et al., 2007), suggesting the ER stress could occur in patients with psychiatric disorders. XBP1 polymorphisms are associated with psychiatric disorders including SCZ, depression, and bipolar disorder in patients with or without antipsychotic treatment (Table 2). GRP78/BiP is an essential HSP70 resident protein in the ER. HSP70 is overexpressed in patients with SCZ and is suggested to be involved in the pathology of the disease (Table 2). Another study in a Korean population showed that HSP70-2 gene polymorphism might be related to the pathogenesis of SCZ (Table 2). Moreover, an autopsy study reported that in the dorsolateral prefrontal cortex of antipsychotic-treated SCZ patients, expression of GRP78/BiP and sXbp1/uXbp1 were increased (Table 2). These findings suggest that brain ER stress may play an important role in the pathophysiology of SCZ. However, in these studies certain subjects had been treated with antipsychotics; therefore, antipsychotics could have affected these results. Currently, there is a lack of direct clinical evidence regarding whether hypothalamic ER stress is related to the pathophysiology of SCZ. It has been reported that DRD2/DRD3 availability is significantly decreased in the hypothalamus of SCZ patients not receiving antipsychotic treatment (Mitelman et al., 2020). In mice lacking the dysbindin-1 gene, an animal model of SCZ, there are reduced dopamine levels in the hypothalamus (Hattori et al., 2008). These findings suggest that SCZ reduces DRD2/DRD3 signaling in the hypothalamus. Given that ER stress could work downstream of DRD2 (Song et al., 2017), it is possible that DRD2-ER stress pathway mediates SCZ. Moreover, it is currently unclear that how antipsychotics affect the hypothalamic ER stress in SCZ patients and whether it is involved in antipsychotic-induced obesity. The most widely used antipsychotics such as olanzapine, quetiapine and risperidone are known DRD2 antagonists. Therefore, it is possible that antipscyhotics may mediate the hypothalamic ER stress in SCZ patients. Furthermore, there is no clinical evidence of how hypothalamic astrocytes would be affected in patients with SCZ. The role of astrocytic ER stress in this population is currently not clear.
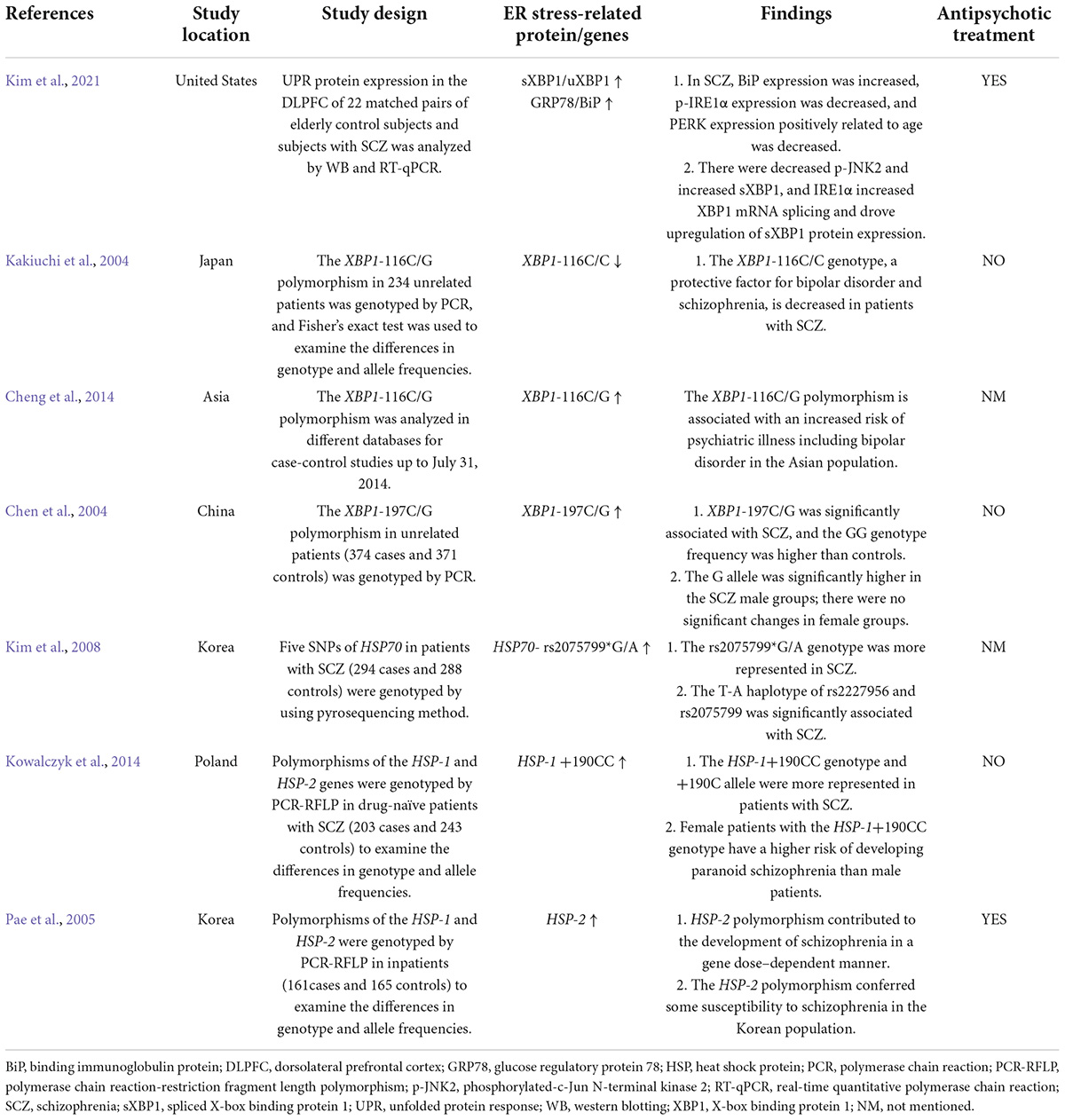
Table 2. Clinical studies of ER stress proteins in schizophrenia patients treated with or without antipsychotics.
A possible role of hypothalamic endoplasmic reticulum stress in schizophrenia-associated metabolic disorder?
Hypothalamic DRD2 signaling plays an essential role in the regulation of food intake, body weight, and glucose metabolism (Yonemochi et al., 2019; Ikeda et al., 2020). Several studies have proved that a decline in central dopaminergic activity significantly influences metabolic parameters such as BMI, glucose, and lipid metabolism in humans (Brunerova et al., 2013). Treatment with a DRD2 antagonist (L-741) but not a DRD3 antagonist could increase food intake and body weight of mice during anorexia (Klenotich et al., 2015). Mice with insulin receptors knocked out in the brain show reduced dopamine signaling, leading to behavioral disturbances (Kleinridders et al., 2015). Mice with Drd2 gene knocked out exhibit impaired insulin secretion and glucose intolerance (García-Tornadú et al., 2010). Moreover, it has been proved that ER stress is involved in the dopaminergic neuronal function. In the substantia nigra pars compacta (SNpc) of Drd2-knockout mice, increased phospho-eIF2α was observed, showing that DRD2 inhibition may activate ER stress eIF2α signaling (Tinsley et al., 2009). Researchers reported that the DRD2 antagonist haloperidol increases PERK expression in a hepatocyte cell model (Lauressergues et al., 2012). On the contrary, activating DRD2 by levodopa attenuated α-syn-induced increased ER stress markers including ATF4, C/EBP homologous protein expression (CHOP), GRP78/BiP and XBP1 in SH-SY5Y neuronal cells (Song et al., 2017). These evidences suggested that reduced DRD2 signaling induced ER stress and these effects could be inhibited by DRD2 activation. Due to the importance of ER stress in regulating food intake, body weight and insulin secretion (Kim et al., 2012; Ajoolabady et al., 2022), it is suggested that in SCZ, reduced DRD2 signaling may lead to induction of ER stress, and these effects may largely contribute to SCZ-associated metabolic disorders such as increased BMI and glucose metabolic disorders (Figure 3).
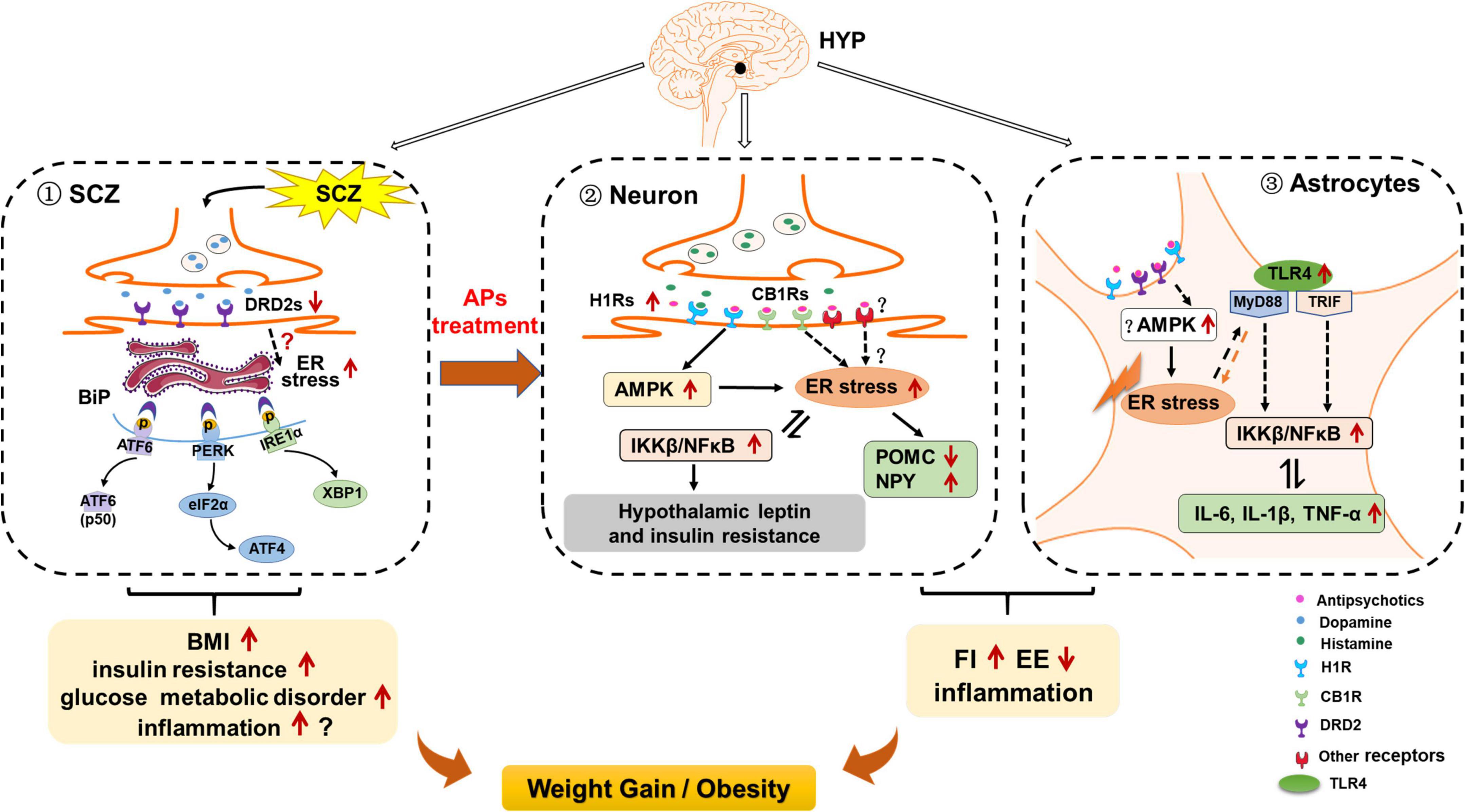
Figure 3. Possible role of hypothalamic endoplasmic reticulum (ER) stress in schizophrenia (SCZ)- and antipsychotic-induced weight gain/obesity. (1) Hypothalamic ER stress may be activated by decreased dopamine receptor D2 (DRD2) signaling, and these effects may be related to SCZ-related upregulation of body mass index (BMI), insulin resistance, and other metabolic disorders. Activated ER stress may also induce neuroinflammation; these effects may also be related to SCZ and its associated metabolic disorders. (2) In hypothalamic neurons, ER stress may contribute to antipsychotic-induced weight gain by inducing hyperphagia, decreasing energy expenditure, and inducing inflammation. Antipsychotics such as olanzapine and clozapine may activate hypothalamic ER stress by affecting H1 receptors (H1R) and CB1 receptors (CB1R), and activating adenosine 5′-monophosphate (AMP)-activated protein kinase (AMPK). On the one hand, activation of ER stress may inhibit proopiomelanocortin (POMC) processing, attenuate leptin signaling, and increase neuropeptide Y (NPY) and agouti-related protein (AgRP) expression, leading to increased food intake; reduced brown adipose tissue (BAT) thermogenesis and white adipose tissue (WAT) browning; increased inflammation; and thus weight gain/obesity Moreover, antipsychotic-induced ER stress activates inhibitor of nuclear factor kappa-B kinase subunit beta (IKKβ)/nuclear factor κB (NF-κB) signaling, leading to insulin and leptin resistance, and increased expression of pro-inflammatory cytokines such as interleukin 6 (IL-6), IL-1β, and tumor necrosis factor alpha (TNF-α). These effects may also contribute to antipsychotic-induced weight gain. (3) In hypothalamic astrocytes, antipsychotics such as olanzapine may induce ER stress possibly by acting on H1R, DRD2, and AMPK signaling. These effects may cause hyperphagia and decreased energy expenditure, resulting in weight gain. Antipsychotic-induced ER stress also leads to the increased TLR4 expression and activation of myeloid differentiation primary response 88 (MyD88)-independent/dependent signaling, which could stimulate the downstream IKKβ-NF-κB signaling activation and further causing the increased expression of pro-inflammatory cytokines such as TNF-α, IL-6, and IL-1β. These effects may be important for antipsychotic-induced obesity development.
Although there are controversial results, a meta-analysis suggests that SCZ is associated with the propensity to produce pro-inflammatory cytokines such as TNF-α, IL-6, and IL-1β in the brain (Momtazmanesh et al., 2019). Evidence has shown that DRD2 pathway regulates inflammation, and this effect cause metabolic disorders such as weight gain (Leite and Ribeiro, 2020). Drd2 knockout in mice produces a remarkable inflammatory response in the CNS (Shao et al., 2013). The DRD2 antagonist haloperidol increases TNF-α, IL-1β, and IL-6 expression via activating NF-κB signaling in SH-SY5Y cells exposed to hypoglycemia and hypoxia (Yang et al., 2022). Quinpirole, a DRD2 agonist, inhibits TLR4/NF-κB signaling and suppresses the expression of pro-inflammatory cytokines including TNF-α, IL-6, and IL-1β in the brain of an allergic rhinitis mouse model (Liu et al., 2021). ER stress via the IRE1-XBP1 and PERK pathways promotes inflammatory NF-κB signaling and increases TNF-α, IL-6, and IL-1β expression (Chipurupalli et al., 2021). Therefore, it is also possible that in SCZ, reduced DRD2 signaling activates ER stress, and these effects could activate inflammatory response and regulate body weight. Additional studies are necessary to evaluate this eventuality.
Hypothalamic neuronal endoplasmic reticulum stress and antipsychotic-induced weight gain
Studies have revealed that treatment with antipsychotic drugs including olanzapine, haloperidol, clozapine, and aripiprazole increases the expression of IRE1 and PERK in human and mouse hepatocytes (Lauressergues et al., 2012; Weston-Green et al., 2018; Forno et al., 2020). Olanzapine or risperidone treatment causes significant ER stress in the pancreatic β-cell line of hamsters (Ozasa et al., 2013). This evidence suggests that antipsychotics activate ER stress. Our previous study showed that in human neuroblastoma SH-SY5Y cells, olanzapine treatment induces ER stress and activation of IKKβ-NF-κB signaling and secretion of the pro-inflammatory cytokines including TNF-α, IL-6, and IL-1β (He et al., 2019). Hence, olanzapine could directly act on neurons to induce ER stress and inflammation (He et al., 2019). The fact that the ER stress inhibitor 4-PBA suppresses olanzapine-induced ER stress and inflammation in SH-SY5Y cells suggests that olanzapine-induced inflammation is at least partly regulated by ER stress (He et al., 2019). In rats, both acute (1 day) and short-term (8 days) olanzapine treatment induce ER stress via PERK-eIF2α signaling; activate inflammatory IKKβ-NF-κB signaling; and augment TNF-α, IL-6, and IL-1β expression in the hypothalamus (He et al., 2019). These results suggest that olanzapine-induced ER stress and inflammation occur before rats are obese and could be a significant contributor rather than a consequence of obesity. Moreover, co-treatment with 4-PBA reduces olanzapine-induced hyperphagia and weight gain and inhibits olanzapine-induced ER stress and inflammation (He et al., 2019; Table 1). These findings indicate that olanzapine treatment activates hypothalamic neuronal ER stress and its related inflammatory IKKβ-NF-κB signaling, and these effects could lead to weight gain partly by increasing food intake. Hypothalamic ER stress also plays an important role in modulating energy expenditure. Numerous animal studies have revealed that obesogenic antipsychotics including olanzapine, clozapine, and risperidone reduce energy expenditure by inhibiting BAT thermogenesis, reducing tail artery vasoconstriction, and decreasing oxygen consumption and locomotor activity, all of which promote weight gain (Stefanidis et al., 2009; Bahr et al., 2015; Blessing et al., 2017). A study in humans reported that chronic olanzapine, risperidone, and quetiapine treatment (1, 3, 6, and 12 months) promotes weight gain with a hypometabolic state (Cuerda et al., 2011). Therefore, a reduction in energy expenditure induced by antipsychotics might be related to activation of hypothalamic ER stress. This eventuality should be evaluated in future studies. For example, investigating whether central inhibition of ER stress could reverse the antipsychotic-induced reduction in BAT thermogenesis and suppress the hypometabolic state in rodents would help to understand the role of ER stress in antipsychotic-induced decreased energy expenditure.
Hypothalamic astrocyte endoplasmic reticulum stress and antipsychotic-induced weight gain
We recently found that olanzapine treatment also causes ER stress in cultured astrocytes, and induces activation of astrocytes in the rat hypothalamus (He et al., 2021). Astrocyte activation during olanzapine treatment occurs before weight gain onset, which indicates that astrocytic ER stress may be a contributor to antipsychotic-induced obesity (He et al., 2021). Moreover, we found upregulated expression of p-NF-κB, p-p38 (a MAPK), TLR4, MyD88, and p-ERK1/2 in olanzapine-treated cultured astrocytes. In rats, both short- and long-term olanzapine treatment significantly increase food intake and weight gain, accompanied by activated astrocytes and TLR4 signaling in the hypothalamus. In a pair-fed experiment, we found that olanzapine-treated rats do not exhibit significantly increased weight gain because of their limited access to food. However, the pair-fed olanzapine rats still exhibit activated astrocytes and TLR4 signaling in the hypothalamus, demonstrating that these changes are primarily caused by olanzapine rather than the secondary effects of hyperphagia or weight gain. Moreover, 4-PBA co-treatment inhibits olanzapine-induced hyperphagia, weight gain, astrocyte activation, and TLR4 signaling in the hypothalamus (He et al., 2021). These findings suggest that weight gain induced by olanzapine treatment may also be related to the hypothalamic astrocytes and TLR4 signaling, and these effects are mediated by the hypothalamic ER stress. After olanzapine activates ER stress, the TLR4 signaling pathway may be activated and thereby induce hyperphagia and weight gain (Figure 2). It is important to investigate whether astrocytic TLR4 knockout affects the hyperphagic and obesogenic effects of olanzapine in rodents.
The effects of other antipsychotics on astrocytes, ER stress, and TLR4 signaling, as well as their role in antipsychotic-induced obesity, are controversial. It has been reported that both acute (120 min) and short-term (7 days) treatment of clozapine, quetiapine, and brexpiprazole induce astroglial L-glutamate release and connexin 43 expression, suggesting that most second-generation antipsychotics affect astrocyte activity (Fukuyama and Okada, 2021). Clozapine treatment increases intracellular Ca2+ concentrations and decreases Ca2+ reentry in cultured cortical astrocytes and C6 cells (Kanda et al., 2016). Quetiapine treatment upregulates adenosine triphosphate (ATP) synthesis in astrocytes (Wang et al., 2014). Studies have reported that quetiapine inhibits astrocyte activation in APP/PS1 mice (Zhu et al., 2014) and in streptozotocin-induced diabetic mice (Wang et al., 2019). A previous study reported that chronic exposure to olanzapine (17–27 month period) in macaques significantly reduces the number of astrocytes in parietal gray matter by 20.5% (Dorph-Petersen et al., 2005). Moreover, a study in C6 astroglial cells reported that haloperidol increases extracellular levels of TNF-α and IL-1β and decreases IL-10, while risperidone decreases the release of TNF-α and IL-1β (Bobermin et al., 2018). This evidence suggests that antipsychotic drugs induce variable effects depending on the brain region, treatment period, animal models, and cell lines. Future studies should investigate the effect of antipsychotics such as olanzapine, risperidone and quetiapine on astrocytic ER stress in the hypothalamus and its role in antipsychotic-induced weight gain.
As previously mentioned, microglia also mediate inflammation and obesity development, and there is evidence that antipsychotics interact with microglia. Olanzapine and haloperidol activate microglia in the rat brain (Cotel et al., 2015). Risperidone inhibits microglial activation induced by IFN-γ (Racki et al., 2021). Clozapine reduces microglial activation induced by LPS in neuron-glia cultures (Hu et al., 2012). Clozapine, risperidone, and haloperidol do not affect cytokine expression levels in the absence of external stimuli to microglia (Giridharan et al., 2020). After induction of inflammation in microglia by poly (I:C), clozapine, risperidone, and haloperidol decrease the expression of IL-1α and IL-1β, and risperidone and haloperidol (but not clozapine) increase the expression of IL-6, IL-10, and TNF-α (Giridharan et al., 2020). Microglial ER stress and TLR4 signaling play a crucial role in the development of inflammation and obesity (Masson et al., 2015; Reis et al., 2015). Modulation of ER stress regulates the production of pro-inflammatory cytokines in microglia (Wang et al., 2017). Activation of TLR4 signaling by LPS induced a pro-inflammatory response in microglia (Zusso et al., 2019). TLR4 neutralization inhibited hypoxia-induced secretion of TNF-α and IL-1β in primary cultured microglia (Yao et al., 2013). However, the effects of antipsychotics on microglial ER stress and TLR4 signaling are currently unknown.
Potential mechanisms in schizophrenia- and antipsychotic-induced endoplasmic reticulum stress during obesity development
As discussed above, hypothalamic ER stress may be activated by reduced DRD2 signaling that is part of the pathogenesis of SCZ and SCZ-related metabolic disorders, but additional studies are needed. The mechanisms by which reduced DRD2 signaling leads to ER stress activation are unknown. It has been suggested that in SCZ, DRD2 controls Ca2+ upregulation or release in neurons (Frégeau et al., 2013). Ca2+ is an important inducer of cellular ER stress (Krebs et al., 2015). Hence, in SCZ, DRD2 might regulate ER stress by affecting Ca2+. Studies that investigate alterations in hypothalamic ER stress markers and the Ca2+ level, DRD2 signaling in SCZ, and whether these effects could be inhibited by ER stress inhibitors are warranted. Moreover, we have reported that antipsychotics such as olanzapine activate hypothalamic ER stress in neurons (He et al., 2019) and astrocytes (He et al., 2021). Antipsychotics largely affect the energy sensor, AMPK, and have affinity for different neurotransmitter receptors such as DRD2 and H1R, which are expressed on both neurons and astrocytes and are related to ER stress. Could antipsychotic-induced ER stress be related to AMPK or these neurotransmitter receptors? In this section, we discuss the mechanisms by which antipsychotics induce ER stress.
The possible mechanisms of antipsychotic-induced endoplasmic reticulum stress in neurons
Growing evidence suggests that AMPK may play a role in antipsychotic-induced ER stress. A previous study showed that ceramide-induced reduction in ER stress is regulated by AMPK in the VMH, but the exact mechanism is unclear (Martinez-Sanchez et al., 2017). Inactivation of AMPK within the VMH reduces ER stress, whereas constitutive activation of AMPK prevents triiodothyronine (T3)-induced inhibition of ER stress (Liu et al., 2020). These results suggest that AMPK could act as an upstream regulator of ER stress in the VMH (Liu et al., 2020). Previous studies have revealed that obesogenic antipsychotics such as olanzapine, clozapine, risperidone, and quetiapine activate hypothalamic AMPK and cause weight gain (Kim et al., 2007; He et al., 2014), but there are currently no data demonstrating that antipsychotics trigger hypothalamic ER stress via AMPK signaling. Furthermore, it is unclear which receptors antipsychotics affect to induce ER stress. Antipsychotics including olanzapine, clozapine, and quetiapine induce AMPK activation by blocking H1R (Kim et al., 2007). A previous study reported that H1R antagonism induces ER stress in MCF-7 cells (Jakhar et al., 2016). Therefore, antipsychotic-induced ER stress may be partly related to antagonism of hypothalamic H1R (Figure 1). More studies should investigate whether H1R activation could counteract antipsychotic-induced ER stress. Moreover, it has been reported that ACEA, a CB1R agonist, attenuates ER stress and inflammation in Neuro-2a neuroblastoma cells (Vrechi et al., 2018). Olanzapine treatment significantly reduces CB1R expression in the rat hypothalamus (Weston-Green et al., 2012). These findings indicate that ER stress may also be activated by antipsychotics such as olanzapine via CB1R pathways. Activation of hypothalamic ER stress leads to decreased POMC processing and disrupts leptin signaling. Previous studies have reported that olanzapine reduced the expression of POMC and leptin receptor as well as increased the expression of NPY and AgRP in the hypothalamus of rats (He et al., 2014; Zhang et al., 2014). Risperidone upregulated the mRNA expression of H1R, NPY, and AgRP in the hypothalamus (Lian et al., 2015). Hence, olanzapine and risperidone may activate ER stress by affecting H1R, CB1R, and AMPK. These effects may result in decreased POMC expression, increased NPY and AgRP expression and disrupted leptin signaling, therefore caused hyperphagia, decreased energy expenditure, weight gain, and inflammation (Figure 2). Furthermore, activation of IKKβ-NF-κB signaling through ER stress in the hypothalamus is associated with dysfunction of SOCS3 signaling (Zhang et al., 2008), which means central leptin and insulin resistance. Previous studies have suggested that antipsychotic treatment is associated with leptin resistance and insulin resistance at least partly by activating on H1R and DRD2 (Kim et al., 2007; Hahn et al., 2011); however, the molecular mechanisms are unknown. Antipsychotic-induced leptin and insulin resistance may be related to elevated hypothalamic ER stress. It is well known that leptin and insulin resistance are important contributors to hyperphagia, thermogenesis dysfunction and inflammation. Therefore, antipsychotic-induced ER stress may also cause leptin and insulin resistance, and these effects may worsen obesity and inflammation development during antipsychotic treatment (Figure 2).
The potential mechanisms of antipsychotic-induced endoplasmic reticulum stress in astrocytes
The mechanisms by which antipsychotics induce inflammation, including secretion of pro-inflammatory factors and activation of TLR4 signaling in astrocytes, are not fully understood. Previous studies have reported that AMPK subunits such as α2, β2, and β1 are expressed in astrocytes (Turnley et al., 1999). Metformin reduces high-glucose-induced ER stress and inflammation partly by inhibiting AMPK signaling in primary cultured rat astrocytes (Wang et al., 2021). These results suggest that in astrocytes, AMPK may act upstream of ER stress. Therefore, it is possible that in astrocytes, olanzapine-induced ER stress may be related to AMPK during the development of inflammation and obesity (Figure 3). Future studies should explore whether inhibition of astrocytic AMPK suppresses antipsychotic-induced ER stress. Moreover, the receptors involved in antipsychotic-induced ER stress in astrocytes are unknown. As described above, in the hypothalamus, antipsychotics (e.g., olanzapine, clozapine, and quetiapine) induce AMPK activation by antagonizing H1R (Kim et al., 2007). A previous study reported that H1R is expressed in astrocytes (Xu et al., 2018). Histamine inhibits the secretion of pro-inflammatory factors including TNF-α and IL-1β, and these effects are completely abolished by co-treatment with the H1R antagonist cetirizine in primary cultured astrocytes (Xu et al., 2018). Hence, antipsychotic-induced activation of the inflammatory pathways and ER stress may be largely associated with the antagonism of astrocytic H1R.
Previous studies have proved that DRD2 inhibition is related to astrocytic inflammation. In LPS-stimulated astrocytes, bromocriptine, a DRD2 agonist, suppresses the expression of caspase-1, IL-1β, and TNF-α, changes that suggest DRD2 activation could reduce astrocytic inflammation (Tanaka et al., 2011; Zhu et al., 2018). In a Parkinson’s disease mouse model, the DRD2 agonist LY171555 specifically inhibits astrocytic inflammation by inducing the interaction of β-arrestin 2 and nod-like receptor protein 3 (NLRP3) (Zhu et al., 2018). In contrast, there are marked inflammatory responses in different brain regions of Drd2-deficient mice, including the hippocampus, spinal cord, striatum, and ventral midbrain (Shao et al., 2013). Ablation of Drd2 in astrocytes activates astrocytes in the mouse substantia nigra and increased the mRNA expression of pro-inflammatory factors including IL-1β, IL-6, and cyclooxygenase 2 (COX-2) (Shao et al., 2013). Based on these findings, astrocytic DRD2 might be involved in astrocytic inflammation. Furthermore, in the SNpc of Drd2-knockout mice, there is significantly increased p-eIF2α, suggesting that DRD2 inhibition could activate ER stress (Tinsley et al., 2009). On the contrary, in SH-SY5Y cells, the DRD2 agonist bromocriptine reduces the expression of BiP, indicating that ER stress could be inhibited by DRD2 activation (Henderson et al., 2021). Given that olanzapine, haloperidol, risperidone, and ziprasidone block DRD2, antipsychotics may induce ER stress in astrocytes by antagonizing DRD2, resulting in activation of TLR4 and IKKβ-NF-κB signaling, and secretion of pro-inflammatory markers such as IL-6 and IL-1β, ultimately leading to inflammation and weight gain. Moreover, H1R, CB1R, and DRD2 are expressed on microglia (Dong et al., 2014; Huck et al., 2015; De Meij et al., 2021). H1R, CB1R, and DRD2 signaling in microglia mediates the expression of pro-inflammatory cytokines (Dong et al., 2014; Huck et al., 2015; De Meij et al., 2021), although the exact mechanisms are not clear. As has been mentioned, ER stress could work as a downstream of H1R and DRD2 signaling in astrocytes. However, in microglia, whether ER stress plays a role in H1R-, CB1R-, and DRD2-related inflammatory response is unknown. It is possible that antipsychotics affect microglial ER stress by antagonizing H1R, CB1R, and DRD2, therefore affecting the inflammatory response and body weight. Future studies should investigate whether inhibition of ER stress affects inflammatory cytokine expression induced by activation of H1R, CB1R, and DRD2 in microglia, and how ER stress inhibitors affect the effects of antipsychotics on microglial cytokine expression.
It is noteworthy that the reported effects of antipsychotics on inflammation are inconsistent and complex. In patients with SCZ, both stimulatory and inhibitory effects on inflammatory cytokines have been reported from studies on olanzapine, haloperidol, clozapine, and risperidone (Drzyzga et al., 2006). In rodents, clozapine increases the expression of IL-1β in rat serum (Sernoskie et al., 2022). Olanzapine induces the expression of IL-1β, IL-6, and TNF-α in the rat hypothalamus (He et al., 2019). The role of other antipsychotics on hypothalamic inflammation has not been elucidated fully. In astrocytes, olanzapine, risperidone, quetiapine, and haloperidol increase IL-1β (He et al., 2022a). In microglia, as has been reviewed, haloperidol and risperidone reduce IL-1β induced by poly (I:C) treatment, but increase IL-6 and TNF-α (Giridharan et al., 2020). These findings suggest that the effects of antipsychotics on inflammatory markers vary by cell type. The reasons are likely complicated. For example, antipsychotics such as clozapine, olanzapine, and quetiapine have affinity for DRD2 and H1R, which regulate inflammatory pathways in astrocytes and microglia. However, these receptors differentially regulate inflammatory pathways in different cell types. H1R activation decreases TNF-α and IL-1β in astrocytes (Xu et al., 2018), whereas H1R activation increases TNF-α and IL-6 in microglia (Dong et al., 2014). Activation of DRD2 suppresses the upregulation of IL-1β induced by LPS + ATP in primary cultured astrocytes (Zhu et al., 2018), whereas activation of DRD2 increases nitrite production in microglia (Huck et al., 2015). ER stress signaling could be downstream of H1R and DRD2 (Jakhar et al., 2016; Song et al., 2017). Studies suggest that ER stress differentially mediates inflammation in astrocytes and microglia. Inhibition of ER stress in astrocytes suppresses NF-κB activation induced by LPS and IFN-γ (Yanguas-Casás et al., 2014). However, in microglia, activation of ER stress by tunicamycin suppresses LPS-induced increases in IL-1β, IL-6, and TNF-α, whereas 4-PBA suppresses ER stress and promotes LPS-induced inflammation (Wang et al., 2017). Another study reported that suppression of ER stress by oxytocin inhibits TNF-α and IL-6 in microglia (Inoue et al., 2019). Therefore, the exact role of ER stress in microglia in regulating inflammation requires further investigation. The available evidence suggests that antipsychotics have differential effects on microglial and astrocytic inflammation by affecting H1R and DRD2 and their associated ER stress pathways. This may be an important reason why antipsychotics have been reported to produce pro- and anti-inflammatory effects in different studies. The overall effect of antipsychotics on inflammation in the hypothalamus is a combination of effects on neurons, astrocytes, and microglia. We suggest that ER stress in hypothalamic neurons and astrocytes may be related to antipsychotic-induced inflammation and obesity. Additional studies are needed to unravel how different doses of antipsychotics for different periods of time affect the astrocytic and microglial ER stress-inflammation pathways in the hypothalamus, to understand the mechanisms by which antipsychotics shows pro- or anti-inflammatory effects during different conditions.
Endoplasmic reticulum stress inhibitors as therapeutic alternatives in managing metabolic alterations associated with schizophrenia and antipsychotic treatment
Schizophrenia is accompanied by metabolic disorders such as weight gain/obesity, glucose metabolism disorder, and leptin and insulin resistance. Antipsychotics are the main cause of these side effects. As discussed above, activation of hypothalamic ER stress via reduced DRD2 signaling may contribute to SCZ-associated increased BMI and insulin signaling dysfunction. Olanzapine activates ER stress in both neurons and astrocytes in the hypothalamus, and these effects could be related to olanzapine-induced weight gain/obesity (He et al., 2019, 2021). Clozapine, haloperidol, and risperidone activate ER stress, and these effects may involve antipsychotic-induced metabolic side effects (Lauressergues et al., 2012). These findings suggest that ER stress inhibitors are potential effective interventions against SCZ and antipsychotic related metabolic disorders such as weight gain/obesity and insulin signaling dysfunction. Previous studies have demonstrated that inhibition of ER stress by TUDCA or 4-PBA significantly reduces food intake, weight gain, abnormal glucose metabolism, and insulin and leptin resistance in obese rodents (Basseri et al., 2009; Zhou et al., 2016). Of note, 4-PBA and TUDCA have a very good safety profile and are used medications for patients. TUDCA and PBA could improve β-cell function in humans and increase insulin sensitivity in obese women and men (Kars et al., 2010; Sarvani et al., 2017). In antipsychotic-induced obese rodents, 4-PBA reverses olanzapine-induced weight gain and inflammation by inhibiting hypothalamic ER stress (He et al., 2019). Metformin, which suppresses weight gain at least partly by inhibiting ER stress (Li X. et al., 2021), inhibits olanzapine-induced weight gain in patients (Wu et al., 2008) and rodents (Hu et al., 2014). TUDCA inhibits olanzapine-induced insulin secretion (stimulated by glucose) in pancreatic β cells (Grajales et al., 2022). Overall, the ER stress inhibitors may be useful therapeutic alternatives to manage metabolic alterations associated with SCZ and antipsychotic treatment. Future studies should investigate the effects of TUDCA or 4-PBA on clozapine-, risperidone-, and quetiapine-induced weight gain and other metabolic disorders.
As has been reviewed, ER stress may also play an important role in the pathology of SCZ. ER stress inhibitors including 4-PBA and TUDCA are neuroprotective and significantly ameliorate the cognitive disorders in various neuropsychiatric disorders such as Alzheimer’s disease (Cuadrado-Tejedor et al., 2011; Dionísio et al., 2015) and cerebral ischemic injury (Qi et al., 2004). Pioglitazone, an antidiabetic agent, is an ER stress inhibitor and has been found to reduce the negative symptoms in patients with SCZ (Iranpour et al., 2016). Morin, an ER stress inhibitor, inhibits the IRE1-sXBP-1 signaling pathway induced by tunicamycin (an ER stress inducer) (Mo et al., 2019) and attenuates LPS + ketamine-induced SCZ-like behaviors in mice (Ben-Azu et al., 2019). The ER stress inhibitor, 4-PBA, could be a potential therapy for SCZ (Patel et al., 2017). These findings suggest that, in addition to managing SCZ-related metabolic disorders, the ER stress inhibitors may also effective in treating SCZ.
Conclusion
The role of ER stress in SCZ and antipsychotic-induced weight gain/obesity has not been explored extensively. Based on evidence from clinical and pre-clinical studies, ER stress may be an important underlying mechanism for SCZ and antipsychotic-induced weight gain/obesity. Patients with SCZ show a reduction in hypothalamic DRD2 signaling, which may lead to activation of ER stress, thereby causing weight gain and dysregulated glucose metabolism. Antipsychotic drugs, in particular olanzapine, induce hypothalamic ER stress, and this effect is associated with inflammation and weight gain. Antipsychotics seems to induce hypothalamic ER stress by (1) obstructing POMC processing, attenuating leptin signaling, and increasing the expression of NPY/AgRP, therefore resulting in hyperphagia; (2) decreasing WAT browning and BAT thermogenesis, which inhibit energy expenditure; and (3) activating the MyD88-independent and MyD88-dependent pathways in astrocytes, resulting in increased secretion of pro-inflammatory cytokines. Based on the published evidence, antipsychotic-induced ER stress and the resulting inflammation may be related to the antipsychotic antagonism of H1R and DRD2. Taken together, hypothalamic ER stress could be a valuable target for mitigating SCZ and antipsychotic-induced metabolic side effects such as weight gain/obesity.
Author contributions
MH and RZ wrote the manuscript. RZ, JF, RL, YZ, and BL revised the manuscript and figures. MH, GG, and TS provided the funding. All authors have contributed to and have approved the final manuscript.
Funding
This work was supported by the National Natural Science Foundation of China (21975191), the Natural Science Foundation of Hubei Province (2021CFB301 and 2021CFB299), and the State Key Laboratory of Advanced Technology for Materials Synthesis and Processing (WUT) (2022-KF-27).
Conflict of interest
The authors declare that the research was conducted in the absence of any commercial or financial relationships that could be construed as a potential conflict of interest.
Publisher’s note
All claims expressed in this article are solely those of the authors and do not necessarily represent those of their affiliated organizations, or those of the publisher, the editors and the reviewers. Any product that may be evaluated in this article, or claim that may be made by its manufacturer, is not guaranteed or endorsed by the publisher.
References
Ajoolabady, A., Liu, S., Klionsky, D. J., Lip, G. Y. H., Tuomilehto, J., Kavalakatt, S., et al. (2022). ER stress in obesity pathogenesis and management. Trends Pharmacol. Sci. 43, 97–109. doi: 10.1016/j.tips.2021.11.011
Areias, M. F. C., and Prada, P. O. (2015). Mechanisms of insulin resistance in the amygdala: Influences on food intake. Behav. Brain Res. 282, 209–217. doi: 10.1016/j.bbr.2015.01.003
Bahr, S. M., Weidemann, B. J., Castro, A. N., Walsh, J. W., deLeon, O., Burnett, C. M., et al. (2015). Risperidone-induced weight gain is mediated through shifts in the gut microbiome and suppression of energy expenditure. EBioMedicine 2, 1725–1734. doi: 10.1016/j.ebiom.2015.10.018
Ballon, J. S., Pajvani, U., Freyberg, Z., Leibel, R. L., and Lieberman, J. A. (2014). Molecular pathophysiology of metabolic effects of antipsychotic medications. Trends Endocrinol. Metab. 25, 593–600. doi: 10.1016/j.tem.2014.07.004
Barton, B. B., Segger, F., Fischer, K., Obermeier, M., and Musil, R. (2020). Update on weight-gain caused by antipsychotics: A systematic review and meta-analysis. Expert Opin. Drug Saf. 19, 295–314. doi: 10.1080/14740338.2020.1713091
Basseri, S., Lhoták, S., Sharma, A. M., and Austin, R. C. (2009). The chemical chaperone 4-phenylbutyrate inhibits adipogenesis by modulating the unfolded protein response. J. Lipid Res. 50, 2486–2501. doi: 10.1194/jlr.M900216-JLR200
Ben-Azu, B., Aderibigbe, A. O., Ajayi, A. M., Eneni, A. O., Omogbiya, I. A., Owoeye, O., et al. (2019). Morin decreases cortical pyramidal neuron degeneration via inhibition of neuroinflammation in mouse model of schizophrenia. Int. Immunopharmacol. 70, 338–353. doi: 10.1016/j.intimp.2019.02.052
Bernstein, H. G., Keilhoff, G., and Steiner, J. (2021). The implications of hypothalamic abnormalities for schizophrenia. Handb. Clin. Neurol. 182, 107–120. doi: 10.1016/b978-0-12-819973-2.00008-3
Blessing, W. W., Blessing, E. M., Mohammed, M., and Ootsuka, Y. (2017). Clozapine, chlorpromazine and risperidone dose-dependently reduce emotional hyperthermia, a biological marker of salience. Psychopharmacology 234, 3259–3269. doi: 10.1007/s00213-017-4710-x
Bobbo, V. C., Engel, D. F., Jara, C. P., Mendes, N. F., Haddad-Tovolli, R., Prado, T. P., et al. (2021). Interleukin-6 actions in the hypothalamus protects against obesity and is involved in the regulation of neurogenesis. J. Neuroinflamm. 18:192. doi: 10.1186/s12974-021-02242-8
Bobermin, L. D., da Silva, A., Souza, D. O., and Quincozes-Santos, A. (2018). Differential effects of typical and atypical antipsychotics on astroglial cells in vitro. Int. J. Dev. Neurosci. 69, 1–9. doi: 10.1016/j.ijdevneu.2018.06.001
Breen, T. L., Conwell, I. M., and Wardlaw, S. L. (2005). Effects of fasting, leptin, and insulin on AGRP and POMC peptide release in the hypothalamus. Brain Res. 1032, 141–148. doi: 10.1016/j.brainres.2004.11.008
Brunerova, L., Potockova, J., Horacek, J., Suchy, J., and Andel, M. (2013). Central dopaminergic activity influences metabolic parameters in healthy men. Neuroendocrinology 97, 132–138. doi: 10.1159/000338405
Burdakov, D., and Peleg-Raibstein, D. (2020). The hypothalamus as a primary coordinator of memory updating. Physiol. Behav. 223:112988. doi: 10.1016/j.physbeh.2020.112988
Cakir, I., and Nillni, E. A. (2019). Endoplasmic reticulum stress, the hypothalamus, and energy balance. Trends Endocrinol. Metab. 30, 163–176. doi: 10.1016/j.tem.2019.01.002
Cakir, I., Cyr, N. E., Perello, M., Litvinov, B. P., Romero, A., Stuart, R. C., et al. (2013). Obesity induces hypothalamic endoplasmic reticulum stress and impairs proopiomelanocortin (POMC) post-translational processing. J. Biol. Chem. 288, 17675–17688. doi: 10.1074/jbc.M113.475343
Camandola, S., and Mattson, M. P. (2017). Toll-like receptor 4 mediates fat, sugar, and umami taste preference and food intake and body weight regulation. Obesity 25, 1237–1245. doi: 10.1002/oby.21871
Cansell, C., Stobbe, K., Sanchez, C., Le Thuc, O., Mosser, C. A., Ben-Fradj, S., et al. (2021). Dietary fat exacerbates postprandial hypothalamic inflammation involving glial fibrillary acidic protein-positive cells and microglia in male mice. Glia 69, 42–60. doi: 10.1002/glia.23882
Catalán, V., Gómez-Ambrosi, J., Rodríguez, A., Ramírez, B., Rotellar, F., Valentí, V., et al. (2012). Increased tenascin C and Toll-like receptor 4 levels in visceral adipose tissue as a link between inflammation and extracellular matrix remodeling in obesity. J. Clin. Endocrinol. Metab. 97, E1880–E1889. doi: 10.1210/jc.2012-1670
Chadwick, W., Magnus, T., Martin, B., Keselman, A., Mattson, M. P., and Maudsley, S. (2008). Targeting TNF-alpha receptors for neurotherapeutics. Trends Neurosci. 31, 504–511. doi: 10.1016/j.tins.2008.07.005
Chang, Y., and Kong, R. (2019). Ganoderic acid A alleviates hypoxia-induced apoptosis, autophagy, and inflammation in rat neural stem cells through the PI3K/AKT/mTOR pathways. Phytother. Res. 33, 1448–1456. doi: 10.1002/ptr.6336
Chen, N., Sugihara, H., Kim, J., Fu, Z., Barak, B., Sur, M., et al. (2016). Direct modulation of GFAP-expressing glia in the arcuate nucleus bi-directionally regulates feeding. Elife 5:e18716. doi: 10.7554/eLife.18716
Chen, W., Duan, S., Zhou, J., Sun, Y., Zheng, Y., Gu, N., et al. (2004). A case–control study provides evidence of association for a functional polymorphism –197C/G in XBP1 to schizophrenia and suggests a sex-dependent effect. Biochem. Biophys. Res. Commun. 319, 866–870. doi: 10.1016/j.bbrc.2004.05.060
Chen, X., Yu, Y., Zheng, P., Jin, T., He, M., Zheng, M., et al. (2020). Olanzapine increases AMPK-NPY orexigenic signaling by disrupting H1R-GHSR1a interaction in the hypothalamic neurons of mice. Psychoneuroendocrinology 114:104594. doi: 10.1016/j.psyneuen.2020.104594
Cheng, D., Zhang, K., Zhen, G., and Xue, Z. (2014). The -116c/G polymorphism in Xbp1 gene is associated with psychiatric illness in asian population: A meta-analysis. Am. J. Med. Genet B Neuropsychiatry Genet. 165b, 665–672. doi: 10.1002/ajmg.b.32271
Chipurupalli, S., Samavedam, U., and Robinson, N. (2021). Crosstalk between er stress, autophagy and inflammation. Front. Med. 8:758311. doi: 10.3389/fmed.2021.758311
Cnop, M., Foufelle, F., and Velloso, L. A. (2012). Endoplasmic reticulum stress, obesity and diabetes. Trends Mol. Med. 18, 59–68. doi: 10.1016/j.molmed.2011.07.010
Contreras, C., Gonzalez-Garcia, I., Martinez-Sanchez, N., Seoane-Collazo, P., Jacas, J., Morgan, D. A., et al. (2014). Central ceramide-induced hypothalamic lipotoxicity and ER stress regulate energy balance. Cell Rep. 9, 366–377. doi: 10.1016/j.celrep.2014.08.057
Contreras, C., Gonzalez-Garcia, I., Seoane-Collazo, P., Martinez-Sanchez, N., Linares-Pose, L., Rial-Pensado, E., et al. (2017). Reduction of hypothalamic endoplasmic reticulum stress activates browning of white fat and ameliorates obesity. Diabetes 66, 87–99. doi: 10.2337/db15-1547
Cope, M. B., Nagy, T. R., Fernández, J. R., Geary, N., Casey, D. E., and Allison, D. B. (2005). Antipsychotic drug-induced weight gain: Development of an animal model. Int. J. Obes. 29, 607–614. doi: 10.1038/sj.ijo.0802928
Cotel, M. C., Lenartowicz, E. M., Natesan, S., Modo, M. M., Cooper, J. D., Williams, S. C., et al. (2015). Microglial activation in the rat brain following chronic antipsychotic treatment at clinically relevant doses. Eur. Neuropsychopharmacol. 25, 2098–2107. doi: 10.1016/j.euroneuro.2015.08.004
Cuadrado-Tejedor, M., García-Osta, A., Ricobaraza, A., Oyarzabal, J., and Franco, R. (2011). Defining the mechanism of action of 4-phenylbutyrate to develop a small-molecule-based therapy for Alzheimer’s disease. Curr. Med. Chem. 18, 5545–5553. doi: 10.2174/092986711798347315
Cuerda, C., Merchan-Naranjo, J., Velasco, C., Gutierrez, A., Leiva, M., de Castro, M. J., et al. (2011). Influence of resting energy expenditure on weight gain in adolescents taking second-generation antipsychotics. Clin. Nutr. 30, 616–623. doi: 10.1016/j.clnu.2011.03.007
Dalvi, P. S., Chalmers, J. A., Luo, V., Han, D. Y., Wellhauser, L., Liu, Y., et al. (2017). High fat induces acute and chronic inflammation in the hypothalamus: Effect of high-fat diet, palmitate and TNF-α on appetite-regulating NPY neurons. Int. J. Obes. 41, 149–158. doi: 10.1038/ijo.2016.183
Darling, N. J., and Cook, S. J. (2014). The role of MAPK signalling pathways in the response to endoplasmic reticulum stress. Biochim. Biophys. Acta 1843, 2150–2163. doi: 10.1016/j.bbamcr.2014.01.009
De Meij, J., Alfanek, Z., Morel, L., Decoeur, F., Leyrolle, Q., Picard, K., et al. (2021). Microglial cannabinoid type 1 receptor regulates brain inflammation in a sex-specific manner. Cannabis Cannabinoid Res. 6, 488–507. doi: 10.1089/can.2020.0170
de Vicente, L. G., Muñoz, V. R., Pinto, A. P., Rovina, R. L., da Rocha, A. L., Marafon, B. B., et al. (2021). TLR4 deletion increases basal energy expenditure and attenuates heart apoptosis and ER stress but mitigates the training-induced cardiac function and performance improvement. Life Sci. 285:119988. doi: 10.1016/j.lfs.2021.119988
Deng, C., Lian, J., Pai, N., and Huang, X. F. (2012). Reducing olanzapine-induced weight gain side effect by using betahistine: A study in the rat model. J. Psychopharmacol. 26, 1271–1279. doi: 10.1177/0269881112449396
Deng, J., Yuan, F., Guo, Y., Xiao, Y., Niu, Y., Deng, Y., et al. (2017). Deletion of ATF4 in AgRP neurons promotes fat loss mainly via increasing energy expenditure. Diabetes 66, 640–650. doi: 10.2337/db16-0954
Denis, R. G., Arruda, A. P., Romanatto, T., Milanski, M., Coope, A., Solon, C., et al. (2010). TNF-α transiently induces endoplasmic reticulum stress and an incomplete unfolded protein response in the hypothalamus. Neuroscience 170, 1035–1044. doi: 10.1016/j.neuroscience.2010.08.013
Dionísio, P. A., Amaral, J. D., Ribeiro, M. F., Lo, A. C., D’Hooge, R., and Rodrigues, C. M. (2015). Amyloid-β pathology is attenuated by tauroursodeoxycholic acid treatment in APP/PS1 mice after disease onset. Neurobiol. Aging 36, 228–240. doi: 10.1016/j.neurobiolaging.2014.08.034
Doane, M. J., Bessonova, L., Friedler, H. S., Mortimer, K. M., Cheng, H., Brecht, T., et al. (2022). Weight gain and comorbidities associated with oral second-generation antipsychotics: Analysis of real-world data for patients with schizophrenia or bipolar I disorder. BMC Psychiatry 22:114. doi: 10.1186/s12888-022-03758-w
Dong, H., Zhang, W., Zeng, X., Hu, G., Zhang, H., He, S., et al. (2014). Histamine induces upregulated expression of histamine receptors and increases release of inflammatory mediators from microglia. Mol. Neurobiol. 49, 1487–1500. doi: 10.1007/s12035-014-8697-6
Dorph-Petersen, K. A., Pierri, J. N., Perel, J. M., Sun, Z., Sampson, A. R., and Lewis, D. A. (2005). The influence of chronic exposure to antipsychotic medications on brain size before and after tissue fixation: A comparison of haloperidol and olanzapine in macaque monkeys. Neuropsychopharmacology 30, 1649–1661. doi: 10.1038/sj.npp.1300710
Douglass, J. D., Dorfman, M. D., Fasnacht, R., Shaffer, L. D., and Thaler, J. P. (2017). Astrocyte IKKβ/NF-κB signaling is required for diet-induced obesity and hypothalamic inflammation. Mol. Metab. 6, 366–373. doi: 10.1016/j.molmet.2017.01.010
Drzyzga, L., Obuchowicz, E., Marcinowska, A., and Herman, Z. S. (2006). Cytokines in schizophrenia and the effects of antipsychotic drugs. Brain Behav. Immun. 20, 532–545. doi: 10.1016/j.bbi.2006.02.002
Eder, U., Mangweth, B., Ebenbichler, C., Weiss, E., Hofer, A., Hummer, M., et al. (2001). Association of olanzapine-induced weight gain with an increase in body fat. Am. J. Psychiatry 158, 1719–1722. doi: 10.1176/appi.ajp.158.10.1719
Ellul, P., Delorme, R., and Cortese, S. (2018). Metformin for weight gain associated with second-generation antipsychotics in children and adolescents: A systematic review and meta-analysis. CNS Drugs 32, 1103–1112. doi: 10.1007/s40263-018-0571-z
Fernø, J., Varela, L., Skrede, S., Vázquez, M. J., Nogueiras, R., Diéguez, C., et al. (2011). Olanzapine-induced hyperphagia and weight gain associate with orexigenic hypothalamic neuropeptide signaling without concomitant AMPK phosphorylation. PLoS One 6:e20571. doi: 10.1371/journal.pone.0020571
Forno, F., Maatuf, Y., Boukeileh, S., Dipta, P., Mahameed, M., Darawshi, O., et al. (2020). Aripiprazole cytotoxicity coincides with activation of the unfolded protein response in human hepatic cells. J. Pharmacol. Exp. Ther. 374, 452–461. doi: 10.1124/jpet.119.264481
Frégeau, M. O., Carrier, M., and Guillemette, G. (2013). Mechanism of dopamine D2 receptor-induced Ca(2+) release in PC-12 cells. Cell Signal. 25, 2871–2877. doi: 10.1016/j.cellsig.2013.08.021
Freyberg, Z., Aslanoglou, D., Shah, R., and Ballon, J. S. (2017). Intrinsic and antipsychotic drug-induced metabolic dysfunction in schizophrenia. Front. Neurosci. 11:432. doi: 10.3389/fnins.2017.00432
Friedman, W. J. (2001). Cytokines regulate expression of the type 1 interleukin-1 receptor in rat hippocampal neurons and glia. Exp. Neurol. 168, 23–31. doi: 10.1006/exnr.2000.7595
Fukuyama, K., and Okada, M. (2021). Effects of atypical antipsychotics, clozapine, quetiapine and brexpiprazole on astroglial transmission associated with connexin43. Int. J. Mol. Sci. 22:5623. doi: 10.3390/ijms22115623
Galichet, C., Clayton, R. W., and Lovell-Badge, R. (2021). Novel tools and investigative approaches for the study of oligodendrocyte precursor cells (NG2-Glia) in CNS development and disease. Front. Cell. Neurosci. 15:673132. doi: 10.3389/fncel.2021.673132
García-Tornadú, I., Ornstein, A. M., Chamson-Reig, A., Wheeler, M. B., Hill, D. J., Arany, E., et al. (2010). Disruption of the dopamine d2 receptor impairs insulin secretion and causes glucose intolerance. Endocrinology 151, 1441–1450. doi: 10.1210/en.2009-0996
Garg, A. D., Kaczmarek, A., Krysko, O., Vandenabeele, P., Krysko, D. V., and Agostinis, P. (2012). ER stress-induced inflammation: Does it aid or impede disease progression? Trends Mol. Med. 18, 589–598. doi: 10.1016/j.molmed.2012.06.010
Giridharan, V. V., Scaini, G., Colpo, G. D., Doifode, T., Pinjari, O. F., Teixeira, A. L., et al. (2020). Clozapine prevents poly (I:C) induced inflammation by modulating NLRP3 pathway in microglial cells. Cells 9:577. doi: 10.3390/cells9030577
González-García, I., Contreras, C., Estévez-Salguero, Á, Ruíz-Pino, F., Colsh, B., Pensado, I., et al. (2018). Estradiol regulates energy balance by ameliorating hypothalamic ceramide-induced ER stress. Cell Rep. 25, 413–423.e5. doi: 10.1016/j.celrep.2018.09.038
Gorina, R., Font-Nieves, M., Marquez-Kisinousky, L., Santalucia, T., and Planas, A. M. (2011). Astrocyte TLR4 activation induces a proinflammatory environment through the interplay between MyD88-dependent NFkappaB signaling, MAPK, and Jak1/Stat1 pathways. Glia 59, 242–255. doi: 10.1002/glia.21094
Grajales, D., Vázquez, P., Alén, R., Hitos, A. B., and Valverde, ÁM. (2022). Attenuation of olanzapine-induced endoplasmic reticulum stress improves insulin secretion in pancreatic beta cells. Metabolites 12:433. doi: 10.3390/metabo12050443
Hahn, M., Chintoh, A., Giacca, A., Xu, L., Lam, L., Mann, S., et al. (2011). Atypical antipsychotics and effects of muscarinic, serotonergic, dopaminergic and histaminergic receptor binding on insulin secretion in vivo: An animal model. Schizophr. Res. 131, 90–95. doi: 10.1016/j.schres.2011.06.004
Han, M., Lian, J., Su, Y., and Deng, C. (2022). Cevimeline co-treatment attenuates olanzapine-induced metabolic disorders via modulating hepatic M3 muscarinic receptor: AMPKα signalling pathway in female rats. J. Psychopharmacol. 36, 202–213. doi: 10.1177/02698811211050549
Harris, L. W., Guest, P. C., Wayland, M. T., Umrania, Y., Krishnamurthy, D., Rahmoune, H., et al. (2013). Schizophrenia: Metabolic aspects of aetiology, diagnosis and future treatment strategies. Psychoneuroendocrinology 38, 752–766. doi: 10.1016/j.psyneuen.2012.09.009
Hattori, S., Murotani, T., Matsuzaki, S., Ishizuka, T., Kumamoto, N., Takeda, M., et al. (2008). Behavioral abnormalities and dopamine reductions in sdy mutant mice with a deletion in Dtnbp1, a susceptibility gene for schizophrenia. Biochem. Biophys. Res. Commun. 373, 298–302. doi: 10.1016/j.bbrc.2008.06.016
He, M., Fan, J., Zhou, R., Gao, G., Li, R., Zuo, Y., et al. (2022a). NLRP3/Caspase-1-mediated pyroptosis of astrocytes induced by antipsychotics is inhibited by a histamine H1 receptor-selective agonist. Front. Aging Neurosci. 14:847561. doi: 10.3389/fnagi.2022.847561
He, M., Huang, X. F., Gao, G., Zhou, T., Li, W., Hu, J., et al. (2019). Olanzapine-induced endoplasmic reticulum stress and inflammation in the hypothalamus were inhibited by an ER stress inhibitor 4-phenylbutyrate. Psychoneuroendocrinology 104, 286–299. doi: 10.1016/j.psyneuen.2019.03.017
He, M., Qian, K., Zhang, Y., Huang, X.-F., Deng, C., Zhang, B., et al. (2021). Olanzapine-induced activation of hypothalamic astrocytes and toll-like receptor-4 signaling via endoplasmic reticulum stress were related to olanzapine-induced weight gain. Front. Neurosci. 14:589650. doi: 10.3389/fnins.2020.589650
He, M., Yao, J., Zhang, Z., Zhang, Y., Chen, R., Gu, Z., et al. (2022b). Gold nanoclusters eliminate obesity induced by antipsychotics. Sci. Rep. 12:5502. doi: 10.1038/s41598-022-09541-x
He, M., Zhang, Q., Deng, C., Wang, H., Lian, J., and Huang, X. F. (2014). Hypothalamic histamine H1 receptor-AMPK signaling time-dependently mediates olanzapine-induced hyperphagia and weight gain in female rats. Psychoneuroendocrinology 42, 153–164. doi: 10.1016/j.psyneuen.2014.01.018
Henderson, M. J., Trychta, K. A., Yang, S. M., Back, S., Yasgar, A., Wires, E. S., et al. (2021). A target-agnostic screen identifies approved drugs to stabilize the endoplasmic reticulum-resident proteome. Cell Rep. 35:109040. doi: 10.1016/j.celrep.2021.109040
Henry, F. E., Sugino, K., Tozer, A., Branco, T., and Sternson, S. M. (2015). Cell type-specific transcriptomics of hypothalamic energy-sensing neuron responses to weight-loss. Elife 4:e09800. doi: 10.7554/eLife.09800
Hu, X., Zhou, H., Zhang, D., Yang, S., Qian, L., Wu, H. M., et al. (2012). Clozapine protects dopaminergic neurons from inflammation-induced damage by inhibiting microglial overactivation. J. Neuroimmune Pharmacol. 7, 187–201. doi: 10.1007/s11481-011-9309-0
Hu, Y., Young, A. J., Ehli, E. A., Nowotny, D., Davies, P. S., Droke, E. A., et al. (2014). Metformin and berberine prevent olanzapine-induced weight gain in rats. PLoS One 9:e93310. doi: 10.1371/journal.pone.0093310
Huck, J. H., Freyer, D., Böttcher, C., Mladinov, M., Muselmann-Genschow, C., Thielke, M., et al. (2015). De novo expression of dopamine D2 receptors on microglia after stroke. J. Cereb. Blood. Flow. Metab. 35, 1804–1811. doi: 10.1038/jcbfm.2015.128
Ikeda, H., Yonemochi, N., Mikami, R., Abe, M., Kawamura, M., Natsume, R., et al. (2020). Central dopamine D(2) receptors regulate plasma glucose levels in mice through autonomic nerves. Sci. Rep. 10:22347. doi: 10.1038/s41598-020-79292-0
Ikegami, M., Ikeda, H., Ishikawa, Y., Ohsawa, M., Ohashi, T., Kai, M., et al. (2013). Olanzapine induces glucose intolerance through the activation of AMPK in the mouse hypothalamus. Eur. J. Pharmacol. 718, 376–382.
Inoue, T., Yamakage, H., Tanaka, M., Kusakabe, T., Shimatsu, A., and Satoh-Asahara, N. (2019). Oxytocin suppresses inflammatory responses induced by lipopolysaccharide through inhibition of the eIF-2-ATF4 pathway in mouse microglia. Cells 8:527. doi: 10.3390/cells8060527
Iranpour, N., Zandifar, A., Farokhnia, M., Goguol, A., Yekehtaz, H., Khodaie-Ardakani, M. R., et al. (2016). The effects of pioglitazone adjuvant therapy on negative symptoms of patients with chronic schizophrenia: A double-blind and placebo-controlled trial. Hum. Psychopharmacol. 31, 103–112. doi: 10.1002/hup.2517
Jaboin, J. J., Shinohara, E. T., Moretti, L., Yang, E. S., Kaminski, J. M., and Lu, B. (2007). The role of mTOR inhibition in augmenting radiation induced autophagy. Technol. Cancer Res. Treat. 6, 443–447. doi: 10.1177/153303460700600510
Jais, A., and Brüning, J. C. (2017). Hypothalamic inflammation in obesity and metabolic disease. J. Clin. Invest. 127, 24–32. doi: 10.1172/jci88878
Jakhar, R., Paul, S., Bhardwaj, M., and Kang, S. C. (2016). Astemizole-Histamine induces Beclin-1-independent autophagy by targeting p53-dependent crosstalk between autophagy and apoptosis. Cancer Lett. 372, 89–100. doi: 10.1016/j.canlet.2015.12.024
Jensen, K. G., Correll, C. U., Rudå, D., Klauber, D. G., Stentebjerg-Olesen, M., Fagerlund, B., et al. (2017). Pretreatment cardiometabolic status in youth with early-onset psychosis: Baseline results from the TEA trial. J. Clin. Psychiatry 78, e1035–e1046. doi: 10.4088/JCP.15m10479
Jin, S., Kim, K. K., Park, B. S., Kim, D. H., Jeong, B., Kang, D., et al. (2020). Function of astrocyte MyD88 in high-fat-diet-induced hypothalamic inflammation. J. Neuroinflamm. 17:195. doi: 10.1186/s12974-020-01846-w
Kakiuchi, C., Ishiwata, M., Umekage, T., Tochigi, M., Kohda, K., Sasaki, T., et al. (2004). Association of the XBP1-116C/G polymorphism with schizophrenia in the Japanese population. Psychiatry. Clin. Neurosci. 58, 438–440. doi: 10.1111/j.1440-1819.2004.01280.x
Kanak, M. A., Shahbazov, R., Yoshimatsu, G., Levy, M. F., Lawrence, M. C., and Naziruddin, B. (2017). A small molecule inhibitor of NFκB blocks ER stress and the NLRP3 inflammasome and prevents progression of pancreatitis. J. Gastroenterol. 52, 352–365. doi: 10.1007/s00535-016-1238-5
Kanda, Y., Okada, M., Ikarashi, R., Morioka, E., Kondo, T., and Ikeda, M. (2016). Bimodal modulation of store-operated Ca(2+) channels by clozapine in astrocytes. Neurosci. Lett. 635, 56–60. doi: 10.1016/j.neulet.2016.10.027
Kao, A. C., Spitzer, S., Anthony, D. C., Lennox, B., and Burnet, P. W. J. (2018). Prebiotic attenuation of olanzapine-induced weight gain in rats: Analysis of central and peripheral biomarkers and gut microbiota. Transl. Psychiatry 8:66. doi: 10.1038/s41398-018-0116-8
Kars, M., Yang, L., Gregor, M. F., Mohammed, B. S., Pietka, T. A., Finck, B. N., et al. (2010). Tauroursodeoxycholic Acid may improve liver and muscle but not adipose tissue insulin sensitivity in obese men and women. Diabetes 59, 1899–1905. doi: 10.2337/db10-0308
Kim, J. G., Suyama, S., Koch, M., Jin, S., Argente-Arizon, P., Argente, J., et al. (2014). Leptin signaling in astrocytes regulates hypothalamic neuronal circuits and feeding. Nat. Neurosci. 17, 908–910. doi: 10.1038/nn.3725
Kim, M. K., Kim, H. S., Lee, I. K., and Park, K. G. (2012). Endoplasmic reticulum stress and insulin biosynthesis: A review. Exp. Diabetes Res. 2012:509437. doi: 10.1155/2012/509437
Kim, S. F., Huang, A. S., Snowman, A. M., Teuscher, C., and Snyder, S. H. (2007). From the cover: Antipsychotic drug-induced weight gain mediated by histamine H1 receptor-linked activation of hypothalamic AMP-kinase. Proc. Natl. Acad. Sci. U.S.A. 104, 3456–3459. doi: 10.1073/pnas.0611417104
Kim, S., Joe, Y., Jeong, S. O., Zheng, M., Back, S. H., Park, S. W., et al. (2014). Endoplasmic reticulum stress is sufficient for the induction of IL-1β production via activation of the NF-κB and inflammasome pathways. Innate Immun. 20, 799–815. doi: 10.1177/1753425913508593
Kim, J. J., Mandelli, L., Lim, S., Lim, H. K., Kwon, O. J., Pae, C. U., et al. (2008). Association analysis of heat shock protein 70 gene polymorphisms in schizophrenia. Eur. Arch. Psychiatry Clin. Neurosci. 258, 239–244. doi: 10.1007/s00406-007-0791-6
Kim, P., Scott, M. R., and Meador-Woodruff, J. H. (2021). Dysregulation of the unfolded protein response (UPR) in the dorsolateral prefrontal cortex in elderly patients with schizophrenia. Mol. Psychiatry 26, 1321–1331. doi: 10.1038/s41380-019-0537-7
Kirk, S. L., Cahir, M., and Reynolds, G. P. (2006). Clozapine, but not haloperidol, increases neuropeptide Y neuronal expression in the rat hypothalamus. J. Psychopharmacol. 20, 577–579. doi: 10.1177/0269881106061199
Kirk, S. L., Glazebrook, J., Grayson, B., Neill, J. C., and Reynolds, G. P. (2009). Olanzapine-induced weight gain in the rat: Role of 5-HT2C and histamine H1 receptors. Psychopharmacology 207:119. doi: 10.1007/s00213-009-1639-8
Kleinridders, A., and Pothos, E. N. (2019). Impact of brain insulin signaling on dopamine function, food intake, reward, and emotional behavior. Curr. Nutr. Rep. 8, 83–91. doi: 10.1007/s13668-019-0276-z
Kleinridders, A., Cai, W., Cappellucci, L., Ghazarian, A., Collins, W. R., Vienberg, S. G., et al. (2015). Insulin resistance in brain alters dopamine turnover and causes behavioral disorders. Proc. Natl. Acad. Sci. U.S.A. 112, 3463–3468. doi: 10.1073/pnas.1500877112
Klenotich, S. J., Ho, E. V., McMurray, M. S., Server, C. H., and Dulawa, S. C. (2015). Dopamine D2/3 receptor antagonism reduces activity-based anorexia. Transl. Psychiatry 5:e613. doi: 10.1038/tp.2015.109
Kowalczyk, M., Owczarek, A., Suchanek, R., Paul-Samojedny, M., Fila-Danilow, A., Borkowska, P., et al. (2014). Heat shock protein 70 gene polymorphisms are associated with paranoid schizophrenia in the polish population. Cell Stress Chaperones 19, 205–215. doi: 10.1007/s12192-013-0446-7
Krasovska, V., and Doering, L. C. (2018). Regulation of IL-6 secretion by astrocytes via TLR4 in the fragile X mouse model. Front. Mol. Neurosci. 11:272. doi: 10.3389/fnmol.2018.00272
Krebs, J., Agellon, L. B., and Michalak, M. (2015). Ca(2+) homeostasis and endoplasmic reticulum (ER) stress: An integrated view of calcium signaling. Biochem. Biophys. Res. Commun. 460, 114–121. doi: 10.1016/j.bbrc.2015.02.004
Kroeger, H., Chiang, W. C., Felden, J., Nguyen, A., and Lin, J. H. (2019). ER stress and unfolded protein response in ocular health and disease. FEBS J. 286, 399–412. doi: 10.1111/febs.14522
Lauressergues, E., Bert, E., Duriez, P., Hum, D., Majd, Z., Staels, B., et al. (2012). Does endoplasmic reticulum stress participate in APD-induced hepatic metabolic dysregulation? Neuropharmacology 62, 784–796. doi: 10.1016/j.neuropharm.2011.08.048
Lazzari, P., Serra, V., Marcello, S., Pira, M., and Mastinu, A. (2017). Metabolic side effects induced by olanzapine treatment are neutralized by CB1 receptor antagonist compounds co-administration in female rats. Eur. Neuropsychopharmacol. 27, 667–678. doi: 10.1016/j.euroneuro.2017.03.010
Le Thuc, O., Stobbe, K., Cansell, C., Nahon, J. L., Blondeau, N., and Rovère, C. (2017). Hypothalamic inflammation and energy balance disruptions: Spotlight on chemokines. Front. Endocrinol. 8:197. doi: 10.3389/fendo.2017.00197
Leite, F., and Ribeiro, L. (2020). Dopaminergic pathways in obesity-associated inflammation. J. Neuroimmune Pharmacol. 15, 93–113. doi: 10.1007/s11481-019-09863-0
Li, L., Yoo, E. S., Li, X., Wyler, S. C., Chen, X., Wan, R., et al. (2021). The atypical antipsychotic risperidone targets hypothalamic melanocortin 4 receptors to cause weight gain. J. Exp. Med. 218:e20202484. doi: 10.1084/jem.20202484
Li, X. (2018). Endoplasmic reticulum stress regulates inflammation in adipocyte of obese rats via toll-like receptors 4 signaling. Iran. J. Basic Med. Sci. 21, 502–507. doi: 10.22038/ijbms.2018.27346.6674
Li, X., Cai, Y., Luo, J., Ding, J., Yao, G., Xiao, X., et al. (2021). Metformin attenuates hypothalamic inflammation via downregulation of RIPK1-independent microglial necroptosis in diet-induced obese mice. Cell Death Discov. 7:338. doi: 10.1038/s41420-021-00732-5
Lian, J., De Santis, M., He, M., and Deng, C. (2015). Risperidone-induced weight gain and reduced locomotor activity in juvenile female rats: The role of histaminergic and NPY pathways. Pharmacol. Res. 9, 20–26. doi: 10.1016/j.phrs.2015.03.004
Liu, H., Xu, Y., and Hu, F. (2020). AMPK in the ventromedial nucleus of the hypothalamus: A key regulator for thermogenesis. Front. Endocrinol. 11:578830. doi: 10.3389/fendo.2020.578830
Liu, P., Qin, D., Lv, H., Fan, W., Zhou, F., Gao, Z., et al. (2021). Activation of dopamine D2 receptor alleviates neuroinflammation in a mouse model of allergic rhinitis with olfactory dysfunction. Allergy Asthma Immunol. Res. 13, 882–895. doi: 10.4168/aair.2021.13.6.882
Livesey, F. J. (2012). A potential link between obesity and neural stem cell dysfunction. Nat. Cell Biol. 14, 987–989. doi: 10.1038/ncb2599
Lord, C. C., Wyler, S. C., Wan, R., Castorena, C. M., Ahmed, N., Mathew, D., et al. (2017). The atypical antipsychotic olanzapine causes weight gain by targeting serotonin receptor 2C. J. Clin. Invest. 127, 3402–3406. doi: 10.1172/JCI93362
Malmberg-Aiello, P., Lamberti, C., Ipponi, A., Bartolini, A., and Schunack, W. (1998). Evidence for hypernociception induction following histamine H1 receptor activation in rodents. Life Sci. 63, 463–476. doi: 10.1016/s0024-3205(98)00295-1
Manaserh, I. H., Maly, E., Jahromi, M., Chikkamenahalli, L., Park, J., and Hill, J. (2020). Insulin sensing by astrocytes is critical for normal thermogenesis and body temperature regulation. J. Endocrinol. 247, 39–52. doi: 10.1530/joe-20-0052
Martinez-Sanchez, N., Seoane-Collazo, P., Contreras, C., Varela, L., Villarroya, J., Rial-Pensado, E., et al. (2017). Hypothalamic AMPK-ER stress-JNK1 axis mediates the central actions of thyroid hormones on energy balance. Cell Metab. 26, 212–229.e12. doi: 10.1016/j.cmet.2017.06.014
Martin-Jiménez, C. A., García-Vega, Á, Cabezas, R., Aliev, G., Echeverria, V., González, J., et al. (2017). Astrocytes and endoplasmic reticulum stress: A bridge between obesity and neurodegenerative diseases. Prog. Neurobiol. 158, 45–68. doi: 10.1016/j.pneurobio.2017.08.001
Masson, G. S., Nair, A. R., Dange, R. B., Silva-Soares, P. P., Michelini, L. C., and Francis, J. (2015). Toll-like receptor 4 promotes autonomic dysfunction, inflammation and microglia activation in the hypothalamic paraventricular nucleus: Role of endoplasmic reticulum stress. PLoS One 10:e0122850. doi: 10.1371/journal.pone.0122850
Matos, L., Gouveia, A. M., and Almeida, H. (2015). ER stress response in human cellular models of senescence. J. Gerontol. A Biol. Sci. Med. Sci. 70, 924–935. doi: 10.1093/gerona/glu129
McCutcheon, R. A., Reis Marques, T., and Howes, O. D. (2020). Schizophrenia-an overview. JAMA Psychiatry 77, 201–210. doi: 10.1001/jamapsychiatry.2019.3360
Medzhitov, R., Preston-Hurlburt, P., Kopp, E., Stadlen, A., Chen, C., Ghosh, S., et al. (1998). MyD88 is an adaptor protein in the hToll/IL-1 receptor family signaling pathways. Mol. Cell 2, 253–258. doi: 10.1016/s1097-2765(00)80136-7
Meguid, M. M., Fetissov, S. O., Varma, M., Sato, T., Zhang, L., Laviano, A., et al. (2000). Hypothalamic dopamine and serotonin in the regulation of food intake. Nutrition 16, 843–857. doi: 10.1016/s0899-9007(00)00449-4
Mendes, N. F., Kim, Y. B., Velloso, L. A., and Araújo, E. P. (2018). Hypothalamic microglial activation in obesity: A mini-review. Front. Neurosci. 12:846. doi: 10.3389/fnins.2018.00846
Milanski, M., Degasperi, G., Coope, A., Morari, J., Denis, R., Cintra, D. E., et al. (2009). Saturated fatty acids produce an inflammatory response predominantly through the activation of TLR4 signaling in hypothalamus: Implications for the pathogenesis of obesity. J. Neurosci. 29, 359–370. doi: 10.1523/jneurosci.2760-08.2009
Mitelman, S. A., Buchsbaum, M. S., Christian, B. T., Merrill, B. M., Buchsbaum, B. R., Mukherjee, J., et al. (2020). Positive association between cerebral grey matter metabolism and dopamine D(2)/D(3) receptor availability in healthy and schizophrenia subjects: An (18)F-fluorodeoxyglucose and (18)F-fallypride positron emission tomography study. World J. Biol. Psychiatry 21, 368–382. doi: 10.1080/15622975.2019.1671609
Mo, J. S., Choi, D., Han, Y. R., Kim, N., and Jeong, H. S. (2019). Morin has protective potential against ER stress induced apoptosis in renal proximal tubular HK-2 cells. Biomed. Pharmacother. 112:108659. doi: 10.1016/j.biopha.2019.108659
Momtazmanesh, S., Zare-Shahabadi, A., and Rezaei, N. (2019). Cytokine alterations in schizophrenia: An updated review. Front. Psychiatry 10:892. doi: 10.3389/fpsyt.2019.00892
Morton, G. J., and Schwartz, M. W. (2001). The NPY/AgRP neuron and energy homeostasis. Int. J. Obes. 25, S56–S62. doi: 10.1038/sj.ijo.0801915
Nasrallah, H. A. (2008). Atypical antipsychotic-induced metabolic side effects: Insights from receptor-binding profiles. Mol. Psychiatry 13, 27–35. doi: 10.1038/sj.mp.4002066
Oh, T. S., Cho, H., Cho, J. H., Yu, S. W., and Kim, E. K. (2016). Hypothalamic AMPK-induced autophagy increases food intake by regulating NPY and POMC expression. Autophagy 12, 2009–2025. doi: 10.1080/15548627.2016.1215382
Ozasa, R., Okada, T., Nadanaka, S., Nagamine, T., Zyryanova, A., Harding, H., et al. (2013). The antipsychotic olanzapine induces apoptosis in insulin-secreting pancreatic β cells by blocking PERK-mediated translational attenuation. Cell Struct. Funct. 38, 183–195. doi: 10.1247/csf.13012
Ozcan, L., Ergin, A. S., Lu, A., Chung, J., Sarkar, S., Nie, D., et al. (2009). Endoplasmic reticulum stress plays a central role in development of leptin resistance. Cell Metab. 9, 35–51. doi: 10.1016/j.cmet.2008.12.004
Ozcan, U., Yilmaz, E., Ozcan, L., Furuhashi, M., Vaillancourt, E., Smith, R. O., et al. (2006). Chemical chaperones reduce ER stress and restore glucose homeostasis in a mouse model of type 2 diabetes. Science 313, 1137–1140. doi: 10.1126/science.1128294
Pae, C. U., Kim, T. S., Kwon, O. J., Artioli, P., Serretti, A., Lee, C. U., et al. (2005). Polymorphisms of heat shock protein 70 gene (Hspa1a, Hspa1b and Hspa1l) and schizophrenia. Neurosci. Res. 53, 8–13. doi: 10.1016/j.neures.2005.05.004
Paixão, S., and Klein, R. (2010). Neuron-astrocyte communication and synaptic plasticity. Curr. Opin. Neurobiol. 20, 466–473. doi: 10.1016/j.conb.2010.04.008
Panula, P., Karlstedt, K., Sallmen, T., Peitsaro, N., Kaslin, J., Michelsen, K. A., et al. (2000). The histaminergic system in the brain: Structural characteristics and changes in hibernation. J. Chem. Neuroanat. 18, 65–74. doi: 10.1016/s0891-0618(99)00052-6
Park, S. M., Kang, T. I., and So, J. S. (2021). Roles of XBP1s in transcriptional regulation of target genes. Biomedicines 9:791. doi: 10.3390/biomedicines9070791
Park, S., Aintablian, A., Coupe, B., and Bouret, S. G. (2020). The endoplasmic reticulum stress-autophagy pathway controls hypothalamic development and energy balance regulation in leptin-deficient neonates. Nat. Commun. 11:1914. doi: 10.1038/s41467-020-15624-y
Patel, S., Sharma, D., Kalia, K., and Tiwari, V. (2017). Crosstalk between endoplasmic reticulum stress and oxidative stress in schizophrenia: The dawn of new therapeutic approaches. Neurosci. Biobehav. Rev. 83, 589–603. doi: 10.1016/j.neubiorev.2017.08.025
Pierre, N., Deldicque, L., Barbé, C., Naslain, D., Cani, P. D., and Francaux, M. (2013). Toll-like receptor 4 knockout mice are protected against endoplasmic reticulum stress induced by a high-fat diet. PLoS One 8:e65061. doi: 10.1371/journal.pone.0065061
Poyurovsky, M., Fuchs, C., Pashinian, A., Levi, A., Weizman, R., and Weizman, A. (2013). Reducing antipsychotic-induced weight gain in schizophrenia: A double-blind placebo-controlled study of reboxetine-betahistine combination. Psychopharmacology 226, 615–622. doi: 10.1007/s00213-012-2935-2
Qi, X., Hosoi, T., Okuma, Y., Kaneko, M., and Nomura, Y. (2004). Sodium 4-phenylbutyrate protects against cerebral ischemic injury. Mol. Pharmacol. 66, 899–908. doi: 10.1124/mol.104.001339
Racki, V., Marcelic, M., Stimac, I., Petric, D., and Kucic, N. (2021). Effects of haloperidol, risperidone, and aripiprazole on the immunometabolic properties of BV-2 microglial cells. Int. J. Mol. Sci. 22:4399. doi: 10.3390/ijms22094399
Ramírez, S., and Claret, M. (2015). Hypothalamic ER stress: A bridge between leptin resistance and obesity. FEBS Lett. 589, 1678–1687. doi: 10.1016/j.febslet.2015.04.025
Reis, W. L., Yi, C. X., Gao, Y., Tschöp, M. H., and Stern, J. E. (2015). Brain innate immunity regulates hypothalamic arcuate neuronal activity and feeding behavior. Endocrinology 156, 1303–1315. doi: 10.1210/en.2014-1849
Ropelle, E. R., Flores, M. B., Cintra, D. E., Rocha, G. Z., Pauli, J. R., Morari, J., et al. (2010). IL-6 and IL-10 anti-inflammatory activity links exercise to hypothalamic insulin and leptin sensitivity through IKKbeta and ER stress inhibition. PLoS Biol. 8:e1000465. doi: 10.1371/journal.pbio.1000465
Rorato, R., Borges, B. C., Uchoa, E. T., Antunes-Rodrigues, J., Elias, C. F., and Elias, L. L. K. (2017). LPS-induced low-grade inflammation increases hypothalamic JNK expression and causes central insulin resistance irrespective of body weight changes. Int. J. Mol. Sci. 18:1431. doi: 10.3390/ijms18071431
Rosciszewski, G., Cadena, V., Auzmendi, J., Cieri, M. B., Lukin, J., Rossi, A. R., et al. (2019). Detrimental effects of HMGB-1 require microglial-astroglial interaction: Implications for the status epilepticus -induced neuroinflammation. Front. Cell. Neurosci. 13:380. doi: 10.3389/fncel.2019.00380
Sa, M., Park, M. G., and Lee, C. J. (2022). Role of hypothalamic reactive astrocytes in diet-induced obesity. Mol. Cells 45, 65–75. doi: 10.14348/molcells.2022.2044
Sarvani, C., Sireesh, D., and Ramkumar, K. M. (2017). Unraveling the role of ER stress inhibitors in the context of metabolic diseases. Pharmacol. Res. 119, 412–421. doi: 10.1016/j.phrs.2017.02.018
Schönthal, A. H. (2012). Endoplasmic reticulum stress: Its role in disease and novel prospects for therapy. Scientifica 2012:857516. doi: 10.6064/2012/857516
Sernoskie, S. C., Lobach, A. R., Kato, R., Jee, A., Weston, J. K., and Uetrecht, J. (2022). Clozapine induces an acute proinflammatory response that is attenuated by inhibition of inflammasome signaling: Implications for idiosyncratic drug-induced agranulocytosis. Toxicol. Sci. 186, 70–82. doi: 10.1093/toxsci/kfab154
Shao, W., Zhang, S. Z., Tang, M., Zhang, X. H., Zhou, Z., Yin, Y. Q., et al. (2013). Suppression of neuroinflammation by astrocytic dopamine D2 receptors via αB-crystallin. Nature 494, 90–94. doi: 10.1038/nature11748
Sharifnia, T., Antoun, J., Verriere, T. G., Suarez, G., Wattacheril, J., Wilson, K. T., et al. (2015). Hepatic TLR4 signaling in obese NAFLD. Am. J. Physiol. Gastrointest. Liver Physiol. 309, G270–G278. doi: 10.1152/ajpgi.00304.2014
Shen, Y., Qin, H., Chen, J., Mou, L., He, Y., Yan, Y., et al. (2016). Postnatal activation of TLR4 in astrocytes promotes excitatory synaptogenesis in hippocampal neurons. J. Cell Biol. 215, 719–734. doi: 10.1083/jcb.201605046
Shin, A. C., Filatova, N., Lindtner, C., Chi, T., Degann, S., Oberlin, D., et al. (2017). Insulin receptor signaling in POMC, but not AgRP, neurons controls adipose tissue insulin action. Diabetes 66, 1560–1571. doi: 10.2337/db16-1238
Singh, R., Stogios, N., Smith, E., Lee, J., Maksyutynsk, K., Au, E., et al. (2022). Gut microbiome in schizophrenia and antipsychotic-induced metabolic alterations: A scoping review. Ther. Adv. Psychopharmacol. 12:20451253221096525. doi: 10.1177/20451253221096525
So, J., Warsh, J. J., and Li, P. P. (2007). Impaired endoplasmic reticulum stress response in B-lymphoblasts from patients with bipolar-I disorder. Biol. Psychiatry 62, 141–147. doi: 10.1016/j.biopsych.2006.10.014
Song, J., Kim, B. C., Nguyen, D. T., Samidurai, M., and Choi, S. M. (2017). Levodopa (L-DOPA) attenuates endoplasmic reticulum stress response and cell death signaling through DRD2 in SH-SY5Y neuronal cells under alpha-synuclein-induced toxicity. Neuroscience 358, 336–348. doi: 10.1016/j.neuroscience.2017.06.060
Sprenkle, N. T., Lahiri, A., Simpkins, J. W., and Meares, G. P. (2019). Endoplasmic reticulum stress is transmissible in vitro between cells of the central nervous system. J. Neurochem. 148, 516–530. doi: 10.1111/jnc.14642
Stefanidis, A., Verty, A. N., Allen, A. M., Owens, N. C., Cowley, M. A., and Oldfield, B. J. (2009). The role of thermogenesis in antipsychotic drug-induced weight gain. Obesity 17, 16–24. doi: 10.1038/oby.2008.468
Stogios, N., Smith, E., Bowden, S., Tran, V., Asgariroozbehani, R., McIntyre, W. B., et al. (2022). Metabolic adverse effects of off-label use of second-generation antipsychotics in the adult population: A systematic review and meta-analysis. Neuropsychopharmacology 47, 664–672. doi: 10.1038/s41386-021-01163-7
Sugiyama, M., Banno, R., Yaginuma, H., Taki, K., Mizoguchi, A., Tsunekawa, T., et al. (2020). Hypothalamic glial cells isolated by MACS reveal that microglia and astrocytes induce hypothalamic inflammation via different processes under high-fat diet conditions. Neurochem. Int. 136:104733. doi: 10.1016/j.neuint.2020.104733
Tanaka, K., Kanno, T., Yanagisawa, Y., Yasutake, K., Hadano, S., Yoshii, F., et al. (2011). Bromocriptine methylate suppresses glial inflammation and moderates disease progression in a mouse model of amyotrophic lateral sclerosis. Exp. Neurol. 232, 41–52. doi: 10.1016/j.expneurol.2011.08.001
Thon, M., Hosoi, T., and Ozawa, K. (2016). Possible integrative actions of leptin and insulin signaling in the hypothalamus targeting energy homeostasis. Front. Endocrinol. 7:138. doi: 10.3389/fendo.2016.00138
Tinsley, R. B., Bye, C. R., Parish, C. L., Tziotis-Vais, A., George, S., Culvenor, J. G., et al. (2009). Dopamine D2 receptor knockout mice develop features of Parkinson disease. Ann. Neurol. 66, 472–484. doi: 10.1002/ana.21716
Tran, L. T., Park, S., Kim, S. K., Lee, J. S., Kim, K. W., and Kwon, O. (2022). Hypothalamic control of energy expenditure and thermogenesis. Exp. Mol. Med. 54, 358–369. doi: 10.1038/s12276-022-00741-z
Turnley, A. M., Stapleton, D., Mann, R. J., Witters, L. A., Kemp, B. E., and Bartlett, P. F. (1999). Cellular distribution and developmental expression of AMP-activated protein kinase isoforms in mouse central nervous system. J. Neurochem. 72, 1707–1716. doi: 10.1046/j.1471-4159.1999.721707.x
Ullah, R., Rauf, N., Nabi, G., Yi, S., Yu-Dong, Z., and Fu, J. (2021). Mechanistic insight into high-fat diet-induced metabolic inflammation in the arcuate nucleus of the hypothalamus. Biomed. Pharmacother. 142:112012. doi: 10.1016/j.biopha.2021.112012
Üner, A. G., Keçik, O., Quaresma, P. G. F., De Araujo, T. M., Lee, H., Li, W., et al. (2019). Role of POMC and AgRP neuronal activities on glycaemia in mice. Sci. Rep. 9:13068. doi: 10.1038/s41598-019-49295-7
Valdearcos, M., Myers, M. G. Jr., and Koliwad, S. K. (2019). Hypothalamic microglia as potential regulators of metabolic physiology. Nat. Metab. 1, 314–320. doi: 10.1038/s42255-019-0040-0
Valdearcos, M., Robblee, M. M., Benjamin, D. I., Nomura, D. K., Xu, A. W., and Koliwad, S. K. (2014). Microglia dictate the impact of saturated fat consumption on hypothalamic inflammation and neuronal function. Cell Rep. 9, 2124–2138. doi: 10.1016/j.celrep.2014.11.018
Varela, L., Kim, J. G., Fernández-Tussy, P., Aryal, B., Liu, Z. W., Fernández-Hernando, C., et al. (2021). Astrocytic lipid metabolism determines susceptibility to diet-induced obesity. Sci. Adv. 7:eabj2814. doi: 10.1126/sciadv.abj2814
Vohra, M. S., Benchoula, K., Serpell, C. J., and Hwa, W. E. (2022). AgRP/NPY and POMC neurons in the arcuate nucleus and their potential role in treatment of obesity. Eur. J. Pharmacol. 915:174611. doi: 10.1016/j.ejphar.2021.174611
Vrechi, T. A., Crunfli, F., Costa, A. P., and Torrão, A. S. (2018). Cannabinoid receptor type 1 agonist ACEA protects neurons from death and attenuates endoplasmic reticulum stress-related apoptotic pathway signaling. Neurotox. Res. 33, 846–855. doi: 10.1007/s12640-017-9839-1
Wan, X. Q., Zeng, F., Huang, X. F., Yang, H. Q., Wang, L., Shi, Y. C., et al. (2020). Risperidone stimulates food intake and induces body weight gain via the hypothalamic arcuate nucleus 5-HT2c receptor-NPY pathway. CNS Neurosci. Ther. 26, 558–566. doi: 10.1111/cns.13281
Wang, G., Cui, W., Chen, S., Shao, Z., Li, Y., Wang, W., et al. (2021). Metformin alleviates high glucose-induced ER stress and inflammation by inhibiting the interaction between caveolin1 and AMPKα in rat astrocytes. Biochem. Biophys. Res. Commun. 534, 908–913. doi: 10.1016/j.bbrc.2020.10.075
Wang, J., Zhu, S., Wang, H., He, J., Zhang, Y., Adilijiang, A., et al. (2014). Astrocyte-dependent protective effect of quetiapine on GABAergic neuron is associated with the prevention of anxiety-like behaviors in aging mice after long-term treatment. J. Neurochem. 130, 780–789. doi: 10.1111/jnc.12771
Wang, K., Song, F., Wang, H., Wang, J. H., and Sun, Y. (2019). Quetiapine attenuates the neuroinflammation and executive function deficit in streptozotocin-induced diabetic mice. Mediators Inflamm. 2019:1236082. doi: 10.1155/2019/1236082
Wang, Y. W., Zhou, Q., Zhang, X., Qian, Q. Q., Xu, J. W., Ni, P. F., et al. (2017). Mild endoplasmic reticulum stress ameliorates lipopolysaccharide-induced neuroinflammation and cognitive impairment via regulation of microglial polarization. J. Neuroinflamm. 14:233. doi: 10.1186/s12974-017-1002-7
Wang, Y., Chen, Y., Zhou, Q., Xu, J., Qian, Q., Ni, P., et al. (2018). Mild endoplasmic reticulum stress protects against lipopolysaccharide-induced astrocytic activation and blood-brain barrier hyperpermeability. Front. Cell. Neurosci. 12:222. doi: 10.3389/fncel.2018.00222
Wang, Z., Huang, Y., Cheng, Y., Tan, Y., Wu, F., Wu, J., et al. (2016). Endoplasmic reticulum stress-induced neuronal inflammatory response and apoptosis likely plays a key role in the development of diabetic encephalopathy. Oncotarget 7, 78455–78472. doi: 10.18632/oncotarget.12925
Weston-Green, K., Babic, I., de Santis, M., Pan, B., Montgomery, M. K., Mitchell, T., et al. (2018). Disrupted sphingolipid metabolism following acute clozapine and olanzapine administration. J. Biomed. Sci. 25:40. doi: 10.1186/s12929-018-0437-1
Weston-Green, K., Huang, X. F., and Deng, C. (2012). Alterations to melanocortinergic, GABAergic and cannabinoid neurotransmission associated with olanzapine-induced weight gain. PLoS One 7:e33548. doi: 10.1371/journal.pone.0033548
Williams, K. W., Liu, T., Kong, X., Fukuda, M., Deng, Y., Berglund, E. D., et al. (2014). Xbp1s in Pomc neurons connects ER stress with energy balance and glucose homeostasis. Cell Metab. 20, 471–482. doi: 10.1016/j.cmet.2014.06.002
Won, J. C., Jang, P. G., Namkoong, C., Koh, E. H., Kim, S. K., Park, J. Y., et al. (2009). Central administration of an endoplasmic reticulum stress inducer inhibits the anorexigenic effects of leptin and insulin. Obesity 17, 1861–1865. doi: 10.1038/oby.2009.194
Wu, R. R., Zhao, J. P., Guo, X. F., He, Y. Q., Fang, M. S., Guo, W. B., et al. (2008). Metformin addition attenuates olanzapine-induced weight gain in drug-naive first-episode schizophrenia patients: A double-blind, placebo-controlled study. Am. J. Psychiatry 165, 352–358. doi: 10.1176/appi.ajp.2007.07010079
Wu, Y., Li, W., Zhou, C., Lu, F., Gao, T., Liu, Y., et al. (2012). Ketamine inhibits lipopolysaccharide-induced astrocytes activation by suppressing TLR4/NF-κB pathway. Cell Physiol. Biochem. 30, 609–617. doi: 10.1159/000341442
Xiao, G., Burguet, J., Kawaguchi, R., Havton, L., and Hinman, J. (2018). Obesity blocks oligodendrocyte precursor cell differentiation and impedes repair after white matter stroke. bioRxiv [Preprint] doi: 10.1101/283184
Xiao, Y., Deng, Y., Yuan, F., Xia, T., Liu, H., Li, Z., et al. (2017a). An ATF4-ATG5 signaling in hypothalamic POMC neurons regulates obesity. Autophagy 13, 1088–1089. doi: 10.1080/15548627.2017.1307488
Xiao, Y., Deng, Y., Yuan, F., Xia, T., Liu, H., Li, Z., et al. (2017b). ATF4/ATG5 signaling in hypothalamic proopiomelanocortin neurons regulates fat mass via affecting energy expenditure. Diabetes 66, 1146–1158. doi: 10.2337/db16-1546
Xu, J., Zhang, X., Qian, Q., Wang, Y., Dong, H., Li, N., et al. (2018). Histamine upregulates the expression of histamine receptors and increases the neuroprotective effect of astrocytes. J. Neuroinflamm. 15:41. doi: 10.1186/s12974-018-1068-x
Yang, J., and Fukuchi, K. I. (2020). TIR-domain-containing adaptor-inducing interferon-β (TRIF) is involved in glucose metabolism in adipose tissue through the insulin/AKT signaling pathway. Int. J. Endocrinol. 2020:6942307. doi: 10.1155/2020/6942307
Yang, L., Qi, Y., and Yang, Y. (2015). Astrocytes control food intake by inhibiting AGRP neuron activity via adenosine A1 receptors. Cell Rep. 11, 798–807. doi: 10.1016/j.celrep.2015.04.002
Yang, X., Haghiac, M., Glazebrook, P., Minium, J., Catalano, P. M., and Hauguel-de Mouzon, S. (2015). Saturated fatty acids enhance TLR4 immune pathways in human trophoblasts. Hum. Reprod. 30, 2152–2159. doi: 10.1093/humrep/dev173
Yang, Y., Song, J., Liu, N., Wei, G., Liu, S., Zhang, S., et al. (2022). Salvianolic acid A relieves cognitive disorder after chronic cerebral ischemia: Involvement of Drd2/Cryab/NF-κB pathway. Pharmacol. Res. 175:105989. doi: 10.1016/j.phrs.2021.105989
Yang, Z., Shao, Y., Zhao, Y., Li, Q., Li, R., Xiao, H., et al. (2020). Endoplasmic reticulum stress-related neuroinflammation and neural stem cells decrease in mice exposure to paraquat. Sci. Rep. 10:17757. doi: 10.1038/s41598-020-74916-x
Yanguas-Casás, N., Barreda-Manso, M. A., Nieto-Sampedro, M., and Romero-Ramírez, L. (2014). Tauroursodeoxycholic acid reduces glial cell activation in an animal model of acute neuroinflammation. J. Neuroinflamm. 11:50. doi: 10.1186/1742-2094-11-50
Yao, L., Kan, E. M., Lu, J., Hao, A., Dheen, S. T., Kaur, C., et al. (2013). Toll-like receptor 4 mediates microglial activation and production of inflammatory mediators in neonatal rat brain following hypoxia: Role of TLR4 in hypoxic microglia. J. Neuroinflamm. 10:785. doi: 10.1186/1742-2094-10-23
Yao, L., Xiao, Y., Liu, S. P., Xu, A. M., and Zhou, Z. G. (2010). [Serum of obesity induce the activation of TLR4/NF-κB signaling pathway on THP-1 cell line]. Zhonghua Yi Xue Za Zhi 90, 3119–3123.
Yi, S., Chen, K., Zhang, L., Shi, W., Zhang, Y., Niu, S., et al. (2019). Endoplasmic reticulum stress is involved in stress-induced hypothalamic neuronal injury in rats via the PERK-ATF4-CHOP and IRE1-ASK1-JNK pathways. Front. Cell. Neurosci. 13:190. doi: 10.3389/fncel.2019.00190
Yonemochi, N., Ardianto, C., Yang, L., Yamamoto, S., Ueda, D., Kamei, J., et al. (2019). Dopaminergic mechanisms in the lateral hypothalamus regulate feeding behavior in association with neuropeptides. Biochem. Biophys. Res. Commun. 519, 547–552. doi: 10.1016/j.bbrc.2019.09.037
Yoshimatsu, H., Itateyama, E., Kondou, S., Tajima, D., Himeno, K., Hidaka, S., et al. (1999). Hypothalamic neuronal histamine as a target of leptin in feeding behavior. Diabetes 48, 2286–2291. doi: 10.2337/diabetes.48.12.2286
Zhang, Q., He, M., Deng, C., Wang, H., Lian, J., and Huang, X. F. (2014). Hypothalamic ghrelin signalling mediates olanzapine-induced hyperphagia and weight gain in female rats. Int. J. Neuropsychopharmacol. 17, 807–818. doi: 10.1017/S1461145713001697
Zhang, X., Zhang, G., Zhang, H., Karin, M., Bai, H., and Cai, D. (2008). Hypothalamic IKKβ/NF-κB and ER stress link overnutrition to energy imbalance and obesity. Cell 135, 61–73. doi: 10.1016/j.cell.2008.07.043
Zhang, Y., Liu, Y., Su, Y., You, Y., Ma, Y., Yang, G., et al. (2017a). The metabolic side effects of 12 antipsychotic drugs used for the treatment of schizophrenia on glucose: A network meta-analysis. BMC Psychiatry 17:373. doi: 10.1186/s12888-017-1539-0
Zhang, Y., Reichel, J. M., Han, C., Zuniga-Hertz, J. P., and Cai, D. (2017b). Astrocytic process plasticity and IKKbeta/NF-kappaB in central control of blood glucose, blood pressure, and body weight. Cell Metab. 25, 1091–1102.e4. doi: 10.1016/j.cmet.2017.04.002
Zhao, Y., Li, G., Li, Y., Wang, Y., and Liu, Z. (2017). Knockdown of Tlr4 in the arcuate nucleus improves obesity related metabolic disorders. Sci. Rep. 7:7441. doi: 10.1038/s41598-017-07858-6
Zhou, L., Ding, S., Li, Y., Wang, L., Chen, W., Bo, T., et al. (2016). Endoplasmic reticulum stress may play a pivotal role in lipid metabolic disorders in a novel mouse model of subclinical hypothyroidism. Sci. Rep. 6:31381. doi: 10.1038/srep31381
Zhu, J., Hu, Z., Han, X., Wang, D., Jiang, Q., Ding, J., et al. (2018). Dopamine D2 receptor restricts astrocytic NLRP3 inflammasome activation via enhancing the interaction of beta-arrestin2 and NLRP3. Cell Death Differ. 25, 2037–2049. doi: 10.1038/s41418-018-0127-2
Zhu, S., Shi, R., Li, V., Wang, J., Zhang, R., Tempier, A., et al. (2014). Quetiapine attenuates glial activation and proinflammatory cytokines in APP/PS1 transgenic mice via inhibition of nuclear factor-κB pathway. Int. J. Neuropsychopharmacol. 18:pyu022. doi: 10.1093/ijnp/pyu022
Zuany-Amorim, C., Hastewell, J., and Walker, C. (2002). Toll-like receptors as potential therapeutic targets for multiple diseases. Nat. Rev. Drug. Discov. 1, 797–807. doi: 10.1038/nrd914
Keywords: antipsychotics, schizophrenia, ER stress, obesity, inflammation, astrocytes
Citation: Zhou R, He M, Fan J, Li R, Zuo Y, Li B, Gao G and Sun T (2022) The role of hypothalamic endoplasmic reticulum stress in schizophrenia and antipsychotic-induced weight gain: A narrative review. Front. Neurosci. 16:947295. doi: 10.3389/fnins.2022.947295
Received: 18 May 2022; Accepted: 29 August 2022;
Published: 16 September 2022.
Edited by:
Barbara Budzynska, Medical University of Lublin, PolandCopyright © 2022 Zhou, He, Fan, Li, Zuo, Li, Gao and Sun. This is an open-access article distributed under the terms of the Creative Commons Attribution License (CC BY). The use, distribution or reproduction in other forums is permitted, provided the original author(s) and the copyright owner(s) are credited and that the original publication in this journal is cited, in accordance with accepted academic practice. No use, distribution or reproduction is permitted which does not comply with these terms.
*Correspondence: Meng He, menghe@whut.edu.cn; Guanbin Gao, gbgao@whut.edu.cn; Taolei Sun, suntl@whut.edu.cn
†These authors have contributed equally to this work