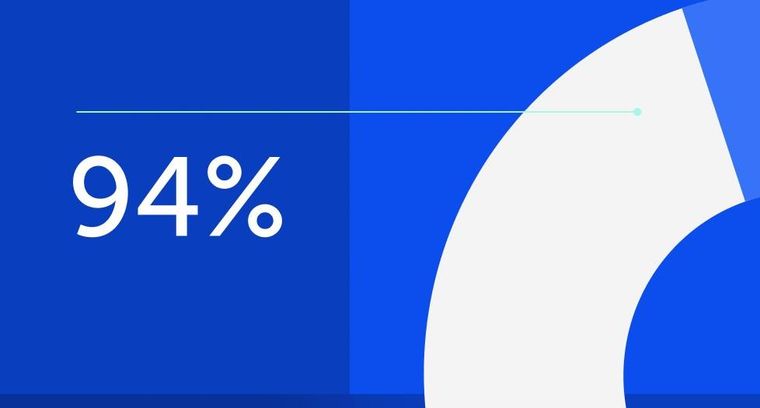
94% of researchers rate our articles as excellent or good
Learn more about the work of our research integrity team to safeguard the quality of each article we publish.
Find out more
MINI REVIEW article
Front. Neurosci., 20 September 2022
Sec. Gut-Brain Axis
Volume 16 - 2022 | https://doi.org/10.3389/fnins.2022.946601
This article is part of the Research TopicInsights in Gut-Brain Axis: 2021View all 9 articles
Despite brain physiological functions or pathological dysfunctions relying on the activity of neuronal/non-neuronal populations, over the last decades a plethora of evidence unraveled the essential contribution of the microbial populations living and residing within the gut, called gut microbiota. The gut microbiota plays a role in brain (dys)functions, and it will become a promising valuable therapeutic target for several brain pathologies. In the present mini-review, after a brief overview of the role of gut microbiota in normal brain physiology and pathology, we focus on the role of the bacterium Clostridioides difficile, a pathogen responsible for recurrent and refractory infections, in people with neurological diseases, summarizing recent correlative and causative evidence in the scientific literature and highlighting the potential of microbiota-based strategies targeting this pathogen to ameliorate not only gastrointestinal but also the neurological symptoms.
The brain is an organ composed of neuronal and non-neuronal cell populations extremely interconnected in complex structural and functional networks that generate and control our behaviors from perception to motor action. Being so complex, it does not come as a surprise that a number of human pathological conditions involve in fact brain dysfunctions. Although genetic and experience-dependent actors surely control, modulate, and influence brain physiology and pathology, over the last decades it became clear that the brain is not alone. Starting from the pioneering studies on microbial ecology back in the 70s (Savage, 1977), the following scientific research on the microbiome (or biome) shed light and revealed the essential contribution of the microbial population living within the human body to the general physiological functions and health of an individual (Liang et al., 2018). In particular, those living and residing in the gastrointestinal tract (GIT) are referred to as gut microbiota, weights in total approximately akin to the human brain and communicating with the latter via the gut–brain axis where (i) microbial signals and metabolites are transmitted across the intestinal epithelium and via different pathways such as the trimethylamine (TMA)/trimethylamine N-oxide (TMAO), the short-chain fatty acids (SCFAs), and the primary and secondary bile acid (BAs) pathways; (ii) the vagus nerve acts as communication pathway (Rhee et al., 2009; Grenham et al., 2011); (iii) moreover, bacteria themselves are able to synthesize and then regulate the level of many neurotransmitters in the brain including major (GABA and glutamate) and neuromodulatory ones (serotonin, dopamine, and norepinephrine) (Desbonnet et al., 2010; Yano et al., 2015; Liu et al., 2017; Strandwitz et al., 2019). For these reasons, the gut microbiota and the brain influence each other via a bidirectional axis (the gut–brain axis), where the gut microbiota can exert control on brain functions in normal physiological conditions (from neurodevelopment till adulthood) and contribute to neurodevelopmental disorders (i.e., autism, schizophrenia, and Rett syndrome) and major brain diseases (i.e., Alzheimer's and Parkinson's diseases) (Grenham et al., 2011; Biagi et al., 2014; Mulak and Bonaz, 2015; Strati et al., 2016; Bonfili et al., 2017; Borghi et al., 2017; Vogt et al., 2017; Scheperjans et al., 2018; Winter et al., 2018; Ma et al., 2019; Deidda and Biazzo, 2021).
Prenatally (in utero), the microbiota derived from the mother can influence (i) fetal brain development by means of metabolites reaching the fetus (Gomez De Aguero et al., 2016; Li et al., 2020), (ii) the development of the brain–blood barrier (Braniste et al., 2014), (iii) brain microglia cells (Thion et al., 2018), and (vi) thalamo-cortical axons growth (Vuong et al., 2020). Early in the postnatal life, the microbial colonization of the infant gut, who inherits the own microbiota via the first swallow and breastfeeding, keeps playing a role in normal brain development and maturation of adult brain functions. It has been demonstrated that animals with absent microbiota throughout life (so-called germ-free animals) show alterations in cognition, social behavior, and stress response in the adult life (Cryan and Dinan, 2012). Later in life, the gut microbiota can influence complex brain functions, such as social interactions by acting on the nutritional behavior of individual animals (Pasquaretta et al., 2018) and regulating pain, anxiety, mood and cognition (Cryan and Dinan, 2012). In mice, administration of Campylobacter jejuni triggers anxiety-like behaviors through a vagal-mediated pathway (Goehler et al., 2005). Patients with inflammatory bowel diseases display altered (i) microbial diversity and (ii) anxiety and depression behaviors (Barberio et al., 2021; Dubinsky et al., 2021).
Defective brain developments had been associated with a number of neurodevelopmental disorders, including, among others, autism spectrum disorder (ASD), schizophrenia spectrum disorders and Down syndrome (Di Cristo, 2007; Deidda et al., 2014, 2021). ASD is characterized by deficits in social communication, social interaction and restricted/repetitive behavioral patterns. GIT symptoms represent common comorbidity in ASD (Molloy and Manning-Courtney, 2003) and can worsen irritability and self-injury in patients (Carr and Owen-Deschryver, 2007). GIT symptom severity increases with the severity of ASD symptoms (Wang et al., 2011; Kho and Lal, 2018). Navarro et al. (2016) described that children with ASD represent a high percentage of patients that reports also GIT dysfunctions (Navarro et al., 2016). Apart from GIT symptoms, ASD is characterized also by an altered gut microbiota profile (Cao et al., 2013) showing higher levels of Desulfovibrio species, Bacteroides vulgatus, and Clostridium bolteae (Finegold et al., 2010; Wang et al., 2012; Pequegnat et al., 2013). Schizophrenia spectrum disorders are characterized by positive symptoms, negative symptoms, and cognitive symptoms. Gut microbiota alteration was described in several clinical trials finding an increase in Lactobacillus, Saccharophagus, Ochrobactrum, Tropheryma, Halothiobacillus, Deferribacter, and Halorubrum (at the genus level) (Schwarz et al., 2018). These trials found also a decrease in Anabaena, Nitrosospira, and Gallionella (at the genus level) (Schwarz et al., 2018) and an increase in Candida albicans fungal species specifically in males (Severance et al., 2016). In Rett syndrome, an X chromosome-linked dominant neurodevelopmental disorder sharing features with ASD, GIT and nutritional problems are common (Motil et al., 2012), as well gut microbiota dysbiosis in terms of relative abundances of both bacterial and fungal components (Strati et al., 2016). Also, people with Down syndrome, the most frequent genetic cause of intellectual disability caused by the trisomy of human chromosome 21 (Bittles et al., 2007; Dierssen, 2012; Deidda et al., 2015; Contestabile et al., 2017), show gut microbiota dysbiosis with differences in Parasporobacterium spp. and Sutterella spp, Veillonellaceae (Biagi et al., 2014).
Not only in neurodevelopmental disorders but gut microbiota dysbiosis was widely reported also in major neurodegenerative diseases. For example, Alzheimer's disease is the first most common form of dementia in elderly life characterized by irreversible neurodegeneration impacting learning and memory functions (Wimo et al., 2017). In this pathology, the gut microbiota composition shows a decreased number of Firmicutes and Actinobacteria and an increased number of bacteria belonging to the Proteobacteria and Bacteroidetes phyla (Vogt et al., 2017). Strikingly, microbiota alterations might arise earlier than Alzheimer's clinical symptoms (Li et al., 2019).
Parkinson's disease is the second most common neurodegenerative disorder of aging (Disease et al., 2016), it results primarily from the death of dopaminergic neurons in the substantia nigra pars compacta in the basal ganglia, and it impacts motor movements (Moore et al., 2005; Antony et al., 2013). GIT symptoms are common including abdominal pain, bloating, and constipation (Abbott et al., 2001; Fasano et al., 2015; Georgescu et al., 2016; Santos et al., 2019). Also, gut microbiome dysfunctions are common (Hamano et al., 1993; Clairembault et al., 2015; Hasegawa et al., 2015; Keshavarzian et al., 2015; Klingelhoefer and Reichmann, 2015; Mulak and Bonaz, 2015; Unger et al., 2016; Bedarf et al., 2017; Hill-Burns et al., 2017; Parashar and Udayabanu, 2017; Scheperjans et al., 2018; Sun and Shen, 2018; Breen et al., 2019; Mihaila et al., 2019; Santos et al., 2019) with changes in the gut bacterial abundances of microbes (such as Prevotellaceae and Enterobacteriaceae) (Scheperjans et al., 2015), reduced content of Dorea, Bacteroides, Prevotella, Faecalibacterium, Bacteroides massiliensis, Stoquefichus massiliensis, Bacteroides coprocola, Blautia glucerasea, Dorea longicatena, Bacteroides dorei, Bacteroides plebeius, Prevotella copri, Coprococcus eutactus, and Ruminococcus callidus, and an increased content of Ruminococcus bromii, Christensenella, Catabacter, Lactobacillus, Oscillospira, Bifidobacterium, Christensenella minuta, Catabacter hongkongensis, Lactobacillus mucosae, and Papillibacter cinnamivorans (Bedarf et al., 2017; Petrov et al., 2017). Thereby, a plethora of evidence indicates that gut microbiota dysfunctions populate brain pathologies in humans. These pieces of evidence are more correlative than causative; in fact, direct strong causative evidence is still lacking in the clinic scientific literature and requires more investigations.
As far as the mechanisms underlying the link between the gut microbiota and neurodegenerative disorders (Parkinson's and Alzheimer's diseases) are concerned, these include gut microbiota-induced chronic inflammation, autoimmune dysregulation, protein misfolding, and defective protein clearance (Padhi et al., 2022). The gut dysbiosis would ultimately be responsible for triggering immune cell activation leading to the disruption of the intestinal and the blood–brain barrier permeability. In particular, the increased gut permeability would allow to pathogenic bacteria to infiltrate and release metabolites and endotoxins (e.g., the lipopolysaccharide, LPS) that can trigger chronic systemic inflammation and neurodegeneration (Brown, 2019).
The concept that gut microbiota affects also the brain and that brain–gut communication dysfunctions might play a role in the pathogenesis of neurological psychiatric disorders brought to a general interest into the search for new therapeutic solutions to tackle these pathologies, including prebiotics, synbiotics and probiotics (Wang et al., 2016; Markowiak and Slizewska, 2017; Deidda and Biazzo, 2021). For example, clinical studies exploited the use of probiotics in ameliorating the core symptoms of children with ASD: i) Kaluzna-Czaplinska and Blaszczyk (2012) showed that probiotic Lactobacillus acidophilus controls the level of D-arabinitol (a metabolite of most pathogenic Candida species) (Kaluzna-Czaplinska and Blaszczyk, 2012), ii) Shaaban et al. (2018) showed that probiotics Lactobacillus acidophilus, Lactobacillus rhamnosus, and Bifidobacteria longum improved the severity of autism and GIT symptoms (Shaaban et al., 2018), while iii) Santocchi et al. (2020) showed that the De Simone probiotic formulation (containing an eight-strain cocktail of probiotics belonging to Lactobacillus, Bifidobacterium, and Streptococcus genus) decreased the severity of ASD score in the group of children without GIT symptoms (Santocchi et al., 2020).
In clinical trials in people with Parkinson's disease, (i) Lactobacillus casei shirota improved stool consistency and defecation (Cassani et al., 2011), (ii) Lactobacillus acidophilus and Bifidobacterium infantis improved abdominal pain and bloating (Georgescu et al., 2016), and (iii) a probiotic mixture containing Lactobacillus acidophilus, Lactobacillus reuteri, Bifidobacterium bifidum, and Lactobacillus fermentum improved movement and metabolic parameters (Tamtaji et al., 2019). In animal models of Parkinson's disease, Lacticaseibacillus rhamnosus HA-114 improved hippocampal-dependent cognition deficits (Xie and Prasad, 2020).
In clinical trials in people with Alzheimer's disease, a mixed-species probiotic (including Lactobacillus acidophilus, Lactobacillus casei, Bifidobacterium bifidum, and Lactobacillus fermentum) improved the mini-mental state examination (MMSE) scores (Akbari et al., 2016). Recent meta-analysis studies showed an improvement in cognitive performance in people with Alzheimer's disease or mild cognitive impairment administered with probiotics (Den et al., 2020). In animal models of Alzheimer's disease, Bifidobacterium breve strain A1 prevented the cognitive dysfunctions induced by Aβ (Kobayashi et al., 2017), while Bonfili et al. (2017) showed that the SLAB51 probiotic formulation decreased the cognitive decline by means of a reduction in brain damage (Bonfili et al., 2017).
Clostridioides difficile (C. difficile) is an anaerobic gram-positive spore-forming bacterium emerging as an important pathogen in humans and being responsible for major infections in hospitalized patients (Eyre et al., 2013). It produces toxins (enterotoxin and cytotoxin which disrupts cytoskeleton signal transductions) that give rise to diarrhea, inflammation, dehydration, abdominal pain, loss of appetite, and nausea in infected patients (Di Bella et al., 2016). C. difficile eradication is difficult to achieve because of its high resistance to antibiotics that only recently begins to be elucidated (O'grady et al., 2021; Wickramage et al., 2021). Fecal microbiota transplantation (FMT), namely the transplant of fecal stools from healthy donors into infected patients (Zhang et al., 2018; Biazzo and Deidda, 2022), represents nowadays the tool used for the eradication of recurrent and refractory (where antibiotic treatment has failed) C. difficile infections in the GIT (Rossen et al., 2015), and also in other pathological conditions such as in ulcerative colitis and obesity (Carlucci et al., 2016).
As far as C. difficile in brain pathologies is concerned (Table 1), different studies found out a major dysbiosis in the gut microbiota composition in children with ASD in comparison with control (Finegold et al., 2002; Navarro et al., 2016) with an overrepresentation of Clostridioides species and underrepresentation of Bifidobacteria (Finegold et al., 2002; Song et al., 2004; Critchfield et al., 2011). The main limitation of these studies relies on the fact that pyrosequencing studies were exploited reporting alterations at the level of the Clostridium genus without verifying whether there is an actual alteration in the relative abundance of C. difficile.
Table 1. Summary of the studies related to the potential involvement of C. difficile in brain pathologies.
The link between C. difficile infection and ASD is far to be elucidated. In mankind, administration of vancomycin, the drug of choice for C. difficile infections, improved diarrhea, but also communication and behavioral symptoms in children with severe ASD; however, these improvements regressed after drug withdrawal (Molloy and Manning-Courtney, 2003) suggesting that the C. difficile infection might be in part responsible. Studies in animal models found out that (i) mice treated with the short-chain fatty acid called propionic acid, a metabolite produced by Clostridioides species, develop autistic-like behaviors (Shultz et al., 2015), thereby identifying a possible causal link between the pathogen and ASD. However, propionic acid is produced by several taxa, not just C. difficile, thereby limiting the result of the study.
Concerning major neurodegenerative diseases, a Swedish population-based cohort study investigated whether C. difficile could represent a causative agent for Parkinson's disease by exploring the association between infection history and future disease risk. The study found out that people within 2 years from the first diagnosis with C. difficile infection were at increased risk to develop the disease in comparison with the group without the infection. Instead, in longer follow-up (more than 2 years), the infection was not associated with Parkinson's disease occurrence (Kang et al., 2020).
The link between C. difficile and brain pathologies has not been elucidated yet, but possible mechanisms recently come out in the spotlight. For example, it has been recently shown that C. difficile alters the metabolism of the neurotransmitter dopamine in the mouse brain, thus interfering with those cognitive functions that involve the neuromodulatory action of dopamine, such as motivation and memory consolidation in rodents (Vinithakumari et al., 2022). Thus, C. difficile might exert a control on the brain with a mechanism similar to those one exploited already by other micro-organisms that alter the level of neurotransmitters, for example, Lactococcus bacteria (Strandwitz et al., 2019), Bifidobacterium infantis (Desbonnet et al., 2010), or Toxoplasma gondii (Luder et al., 1999). Moreover, being C. difficile a pathogen synthetizing toxins, the mechanisms that ultimately lead impacting the brain might likely imply those already described for other bacteria which toxins can lead to a proinflammatory state (Brown, 2019).
As far as microbiota-based strategies targeting C. difficile are concerned, when used to resolve infections caused by C. difficile in people with neurological diseases, FMT proved to be effective also in ameliorating neurological symptoms (Evrensel and Ceylan, 2016; Wortelboer et al., 2019; Vendrik et al., 2020; Xu et al., 2021).
Few clinical trials exploited FMT intervention in ASD and reported improvements in GIT and behavioral symptoms (Kang et al., 2017, 2019), although the authors did not specifically enroll children with ASD affected also by C. difficile infection.
Following a study in a mouse model of Alzheimer's disease showing positive results on Aβ and tau pathology obtained after FMT from control mice (Sun et al., 2019), Park et al. (2021) very recently exploited the FMT strategy in a person with Alzheimer's disease diagnosed with C. difficile infection. Since the classic antibiotic therapy failed in resolving the infection, the patient received two FMTs using fecal samples obtained from a healthy donor. After the transplantation, the author reported a general improvement of GIT symptoms together with elimination of C. difficile in the stools. More interestingly, to what it concerns the neurological aspect, the authors reported a general improvement in cognitive and mood symptoms (i.e., non-verbal learning, short-term memory and attention) (Park et al., 2021). Afterward, another work recapitulated the latter findings in a person with Alzheimer's disease who received FMT from his wife (Hazan, 2020). Altogether, these studies show that FMT might be taken into account when considering strategies aimed at efficiently tackling C. difficile infection and also improving GIT and cognitive deficits in people with Alzheimer's disease.
As far as multiple sclerosis is concerned, two studies explored FMT effects in four patients affected also by C. difficile infection (Borody et al., 2011; Makkawi et al., 2018). As reported in Alzheimer's disease, fecal stools derived from healthy donors resolved not only the infection caused by C. difficile but also improved constipation and neurological symptoms. Surprisingly, some patients showed also progressive improvement of leg paranesthesia and were eventually able to walk for long distances without help.
Antibiotic use is surely a primary risk factor for infection caused by C. difficile, but whether other medications could have an impact is not fully explored yet. In a recent study, Lalani et al. (2020) investigated in a group of US veterans whether the use of antidepressant and GABAergic drugs could contribute as a risk factor for the development of infections caused by C. difficile. The study found that antidepressant drug use was not correlated with risk of infection; instead, GABAergic drug use (benzodiazepines in particular) was associated (Lalani et al., 2020). C. difficile also been shown to result in severe brain infarction and thromboembolism (Kumar et al., 2021), but very few information is currently available and further investigations are needed to deeper study the correlation.
Altogether, gut microbiota dysbiosis results in unbalanced microflora composition and plays a role in influencing brain functions and a number of brain pathologies (Deidda and Biazzo, 2021). In particular, C. difficile infection populates brain pathologies and the remarkable amelioration of clinical brain-related symptoms following C. difficile eradication in the gut could open up as a future perspective, the possibility of therapeutic interventions based on the exploitation of gut microbiota-targeted therapy for brain diseases to be used in the first place rather than being used as parallel therapy when also GIT dysfunctions are involved.
GD and MB conceptualized and wrote the manuscript. MA contributed to writing the manuscript. All authors read and revised the manuscript.
Author MB is CEO of the BioArte Ltd.
The remaining authors declare that the research was conducted in the absence of any commercial or financial relationships that could be construed as a potential conflict of interest.
All claims expressed in this article are solely those of the authors and do not necessarily represent those of their affiliated organizations, or those of the publisher, the editors and the reviewers. Any product that may be evaluated in this article, or claim that may be made by its manufacturer, is not guaranteed or endorsed by the publisher.
Abbott, R. D., Petrovitch, H., White, L. R., Masaki, K. H., Tanner, C. M., Curb, J. D., et al. (2001). Frequency of bowel movements and the future risk of Parkinson's disease. Neurology 57, 456–462. doi: 10.1212/WNL.57.3.456
Akbari, E., Asemi, Z., Daneshvar Kakhaki, R., Bahmani, F., Kouchaki, E., Tamtaji, O. R., et al. (2016). Effect of probiotic supplementation on cognitive function and metabolic status in alzheimer's disease: a randomized, double-blind and controlled trial. Front. Aging Neurosci. 8, 256. doi: 10.3389/fnagi.2016.00256
Antony, P. M., Diederich, N. J., Kruger, R., and Balling, R. (2013). The hallmarks of Parkinson's disease. FEBS J. 280, 5981–5993. doi: 10.1111/febs.12335
Barberio, B., Zamani, M., Black, C. J., Savarino, E. V., and Ford, A. C. (2021). Prevalence of symptoms of anxiety and depression in patients with inflammatory bowel disease: a systematic review and meta-analysis. Lancet Gastroenterol. Hepatol. 6, 359–370. doi: 10.1016/S2468-1253(21)00014-5
Bedarf, J. R., Hildebrand, F., Coelho, L. P., Sunagawa, S., Bahram, M., Goeser, F., et al. (2017). Functional implications of microbial and viral gut metagenome changes in early stage L-DOPA-naive Parkinson's disease patients. Genome Med. 9, 39. doi: 10.1186/s13073-017-0428-y
Biagi, E., Candela, M., Centanni, M., Consolandi, C., Rampelli, S., Turroni, S., et al. (2014). Gut microbiome in Down syndrome. PLoS ONE 9, e112023. doi: 10.1371/journal.pone.0112023
Biazzo, M., and Deidda, G. (2022). Fecal microbiota transplantation as new therapeutic avenue for human diseases. J. Clin. Med. 11, 4119. doi: 10.3390/jcm11144119
Bittles, A. H., Bower, C., Hussain, R., and Glasson, E. J. (2007). The four ages of Down syndrome. Eur. J. Public Health 17, 221–225. doi: 10.1093/eurpub/ckl103
Bonfili, L., Cecarini, V., Berardi, S., Scarpona, S., Suchodolski, J. S., Nasuti, C., et al. (2017). Microbiota modulation counteracts Alzheimer's disease progression influencing neuronal proteolysis and gut hormones plasma levels. Sci. Rep. 7, 2426. doi: 10.1038/s41598-017-02587-2
Borghi, E., Borgo, F., Severgnini, M., Savini, M. N., Casiraghi, M. C., and Vignoli, A. (2017). Rett syndrome: a focus on gut microbiota. Int. J. Mol. Sci. 1, 344. doi: 10.3390/ijms18020344
Borody, T. L. S., Campbell, J., Torres, M., and Nowak, A. (2011). Fecal microbiota transplantation (FMT) in multiple sclerosis (MS). Am. J. Gastroenterol. 106, S352. doi: 10.14309/00000434-201110002-00942
Braniste, V., Al-Asmakh, M., Kowal, C., Anuar, F., Abbaspour, A., Toth, M., et al. (2014). The gut microbiota influences blood-brain barrier permeability in mice. Sci. Transl. Med. 6, 263ra158. doi: 10.1126/scitranslmed.3009759
Breen, D. P., Halliday, G. M., and Lang, A. E. (2019). Gut-brain axis and the spread of alpha-synuclein pathology: vagal highway or dead end? Mov. Disord. 34, 307–316. doi: 10.1002/mds.27556
Brown, G. C. (2019). The endotoxin hypothesis of neurodegeneration. J. Neuroinflamm. 16, 180. doi: 10.1186/s12974-019-1564-7
Cao, X., Lin, P., Jiang, P., and Li, C. (2013). Characteristics of the gastrointestinal microbiome in children with autism spectrum disorder: a systematic review. Shanghai Arch Psychiatry 25, 342–353. doi: 10.3969/j.issn.1002-0829.2013.06.003
Carlucci, C., Petrof, E. O., and Allen-Vercoe, E. (2016). Fecal microbiota-based therapeutics for recurrent Clostridium difficile infection, ulcerative colitis and obesity. EBioMedicine 13, 37–45. doi: 10.1016/j.ebiom.2016.09.029
Carr, E. G., and Owen-Deschryver, J. S. (2007). Physical illness, pain, and problem behavior in minimally verbal people with developmental disabilities. J. Autism Dev. Disord. 37, 413–424. doi: 10.1007/s10803-006-0176-0
Cassani, E., Privitera, G., Pezzoli, G., Pusani, C., Madio, C., Iorio, L., et al. (2011). Use of probiotics for the treatment of constipation in Parkinson's disease patients. Minerva Gastroenterol. Dietol. 57, 117–121.
Clairembault, T., Leclair-Visonneau, L., Neunlist, M., and Derkinderen, P. (2015). Enteric glial cells: new players in Parkinson's disease? Mov. Disord. 30, 494–498. doi: 10.1002/mds.25979
Contestabile, A., Magara, S., and Cancedda, L. (2017). The GABAergic hypothesis for cognitive disabilities in Down syndrome. Front. Cell. Neurosci. 11, 54. doi: 10.3389/fncel.2017.00054
Critchfield, J. W., Van Hemert, S., Ash, M., Mulder, L., and Ashwood, P. (2011). The potential role of probiotics in the management of childhood autism spectrum disorders. Gastroenterol. Res. Pract. 2011, 161358. doi: 10.1155/2011/161358
Cryan, J. F., and Dinan, T. G. (2012). Mind-altering microorganisms: the impact of the gut microbiota on brain and behaviour. Nat. Rev. Neurosci. 13, 701–712. doi: 10.1038/nrn3346
Deidda, G., and Biazzo, M. (2021). Gut and brain: investigating physiological and pathological interactions between microbiota and brain to gain new therapeutic avenues for brain diseases. Front. Neurosci. 15, 753915. doi: 10.3389/fnins.2021.753915
Deidda, G., Bozarth, I. F., and Cancedda, L. (2014). Modulation of GABAergic transmission in development and neurodevelopmental disorders: investigating physiology and pathology to gain therapeutic perspectives. Front. Cell. Neurosci. 8, 119. doi: 10.3389/fncel.2014.00119
Deidda, G., Parrini, M., Naskar, S., Bozarth, I. F., Contestabile, A., and Cancedda, L. (2015). Reversing excitatory GABAAR signaling restores synaptic plasticity and memory in a mouse model of Down syndrome. Nat. Med. 21, 318–326. doi: 10.1038/nm.3827
Deidda, G., Pierucci, M., Crunelli, V., and Di Giovanni, G. (2021). 5-HT/GABA interaction in neurodevelopment and plasticity. Prog. Brain Res. 259, 287–317. doi: 10.1016/bs.pbr.2021.01.009
Den, H., Dong, X., Chen, M., and Zou, Z. (2020). Efficacy of probiotics on cognition, and biomarkers of inflammation and oxidative stress in adults with Alzheimer's disease or mild cognitive impairment - a meta-analysis of randomized controlled trials. Aging 12, 4010–4039. doi: 10.18632/aging.102810
Desbonnet, L., Garrett, L., Clarke, G., Kiely, B., Cryan, J. F., and Dinan, T. G. (2010). Effects of the probiotic Bifidobacterium infantis in the maternal separation model of depression. Neuroscience 170, 1179–1188. doi: 10.1016/j.neuroscience.2010.08.005
Di Bella, S., Ascenzi, P., Siarakas, S., Petrosillo, N., and Di Masi, A. (2016). Clostridium difficile toxins A and B: insights into pathogenic properties and extraintestinal effects. Toxins 8, 134. doi: 10.3390/toxins8050134
Di Cristo, G. (2007). Development of cortical GABAergic circuits and its implications for neurodevelopmental disorders. Clin. Genet. 72, 1–8. doi: 10.1111/j.1399-0004.2007.00822.x
Dierssen, M. (2012). Down syndrome: the brain in trisomic mode. Nat. Rev. Neurosci. 13, 844–858. doi: 10.1038/nrn3314
Disease, G. B. D., Injury, I., and Prevalence, C. (2016). Global, regional, and national incidence, prevalence, and years lived with disability for 310 diseases and injuries, 1990-2015: a systematic analysis for the Global Burden of Disease Study 2015. Lancet 388, 1545–1602. doi: 10.1016/S0140-6736(16)31678-6
Dubinsky, M. C., Dotan, I., Rubin, D. T., Bernauer, M., Patel, D., Cheung, R., et al. (2021). Burden of comorbid anxiety and depression in patients with inflammatory bowel disease: a systematic literature review. Expert Rev. Gastroenterol. Hepatol. 15, 985–997. doi: 10.1080/17474124.2021.1911644
Evrensel, A., and Ceylan, M. E. (2016). Fecal microbiota transplantation and its usage in neuropsychiatric disorders. Clin. Psychopharmacol. Neurosci. 14, 231–237. doi: 10.9758/cpn.2016.14.3.231
Eyre, D. W., Cule, M. L., Wilson, D. J., Griffiths, D., Vaughan, A., O'connor, L., et al. (2013). Diverse sources of C. difficile infection identified on whole-genome sequencing. N. Engl. J. Med. 369, 1195–1205. doi: 10.1056/NEJMoa1216064
Fasano, A., Visanji, N. P., Liu, L. W., Lang, A. E., and Pfeiffer, R. F. (2015). Gastrointestinal dysfunction in Parkinson's disease. Lancet Neurol. 14, 625–639. doi: 10.1016/S1474-4422(15)00007-1
Finegold, S. M., Dowd, S. E., Gontcharova, V., Liu, C., Henley, K. E., Wolcott, R. D., et al. (2010). Pyrosequencing study of fecal microflora of autistic and control children. Anaerobe 16, 444–453. doi: 10.1016/j.anaerobe.2010.06.008
Finegold, S. M., Molitoris, D., Song, Y., Liu, C., Vaisanen, M. L., Bolte, E., et al. (2002). Gastrointestinal microflora studies in late-onset autism. Clin. Infect. Dis. 35, S6–S16. doi: 10.1086/341914
Georgescu, D., Ancusa, O. E., Georgescu, L. A., Ionita, I., and Reisz, D. (2016). Nonmotor gastrointestinal disorders in older patients with Parkinson's disease: is there hope? Clin. Interv. Aging 11, 1601–1608. doi: 10.2147/CIA.S106284
Goehler, L. E., Gaykema, R. P., Opitz, N., Reddaway, R., Badr, N., and Lyte, M. (2005). Activation in vagal afferents and central autonomic pathways: early responses to intestinal infection with Campylobacter jejuni. Brain Behav. Immun. 19, 334–344. doi: 10.1016/j.bbi.2004.09.002
Gomez De Aguero, M., Ganal-Vonarburg, S. C., Fuhrer, T., Rupp, S., Uchimura, Y., Li, H., et al. (2016). The maternal microbiota drives early postnatal innate immune development. Science 351, 1296–1302. doi: 10.1126/science.aad2571
Grenham, S., Clarke, G., Cryan, J. F., and Dinan, T. G. (2011). Brain-gut-microbe communication in health and disease. Front. Physiol. 2, 94. doi: 10.3389/fphys.2011.00094
Hamano, D. M., Helm, K. S., and Papin, P. J. (1993). Computer-assisted management of liquid radioactive waste at the University of California, San Diego. Health Phys. 64, 192–194. doi: 10.1097/00004032-199302000-00011
Hasegawa, S., Goto, S., Tsuji, H., Okuno, T., Asahara, T., Nomoto, K., et al. (2015). Intestinal dysbiosis and lowered serum lipopolysaccharide-binding protein in Parkinson's disease. PLoS ONE 10, e0142164. doi: 10.1371/journal.pone.0142164
Hazan, S. (2020). Rapid improvement in Alzheimer's disease symptoms following fecal microbiota transplantation: a case report. J. Int. Med. Res. 48, 300060520925930. doi: 10.1177/0300060520925930
Hill-Burns, E. M., Debelius, J. W., Morton, J. T., Wissemann, W. T., Lewis, M. R., Wallen, Z. D., et al. (2017). Parkinson's disease and Parkinson's disease medications have distinct signatures of the gut microbiome. Mov. Disord. 32, 739–749. doi: 10.1002/mds.26942
Kaluzna-Czaplinska, J., and Blaszczyk, S. (2012). The level of arabinitol in autistic children after probiotic therapy. Nutrition 28, 124–126. doi: 10.1016/j.nut.2011.08.002
Kang, D. W., Adams, J. B., Coleman, D. M., Pollard, E. L., Maldonado, J., Mcdonough-Means, S., et al. (2019). Long-term benefit of microbiota transfer therapy on autism symptoms and gut microbiota. Sci. Rep. 9, 5821. doi: 10.1038/s41598-019-42183-0
Kang, D. W., Adams, J. B., Gregory, A. C., Borody, T., Chittick, L., Fasano, A., et al. (2017). Microbiota Transfer Therapy alters gut ecosystem and improves gastrointestinal and autism symptoms: an open-label study. Microbiome 5, 10. doi: 10.1186/s40168-016-0225-7
Kang, X., Ploner, A., Ludvigsson, J. F., Williams, D. M., Larsson, H., Pedersen, N. L., et al. (2020). Clostridium difficile infection and risk of Parkinson's disease: a Swedish population-based cohort study. Eur. J. Neurol. 27, 2134–2141. doi: 10.1111/ene.14400
Keshavarzian, A., Green, S. J., Engen, P. A., Voigt, R. M., Naqib, A., Forsyth, C. B., et al. (2015). Colonic bacterial composition in Parkinson's disease. Mov. Disord. 30, 1351–1360. doi: 10.1002/mds.26307
Kho, Z. Y., and Lal, S. K. (2018). The human gut microbiome - a potential controller of wellness and disease. Front. Microbiol. 9, 1835. doi: 10.3389/fmicb.2018.01835
Klingelhoefer, L., and Reichmann, H. (2015). Pathogenesis of Parkinson disease–the gut-brain axis and environmental factors. Nat. Rev. Neurol. 11, 625–636. doi: 10.1038/nrneurol.2015.197
Kobayashi, Y., Sugahara, H., Shimada, K., Mitsuyama, E., Kuhara, T., Yasuoka, A., et al. (2017). Therapeutic potential of Bifidobacterium breve strain A1 for preventing cognitive impairment in Alzheimer's disease. Sci. Rep. 7, 13510. doi: 10.1038/s41598-017-13368-2
Kumar, A., Ghazanfar, H., and Davidson, J. M. (2021). A rare case of arterial and venous thromboembolism in a patient with severe Clostridium difficile infection. Cureus 13, e16103. doi: 10.7759/cureus.16103
Lalani, F., Young, E. H., Panchal, R. M., and Reveles, K. R. (2020). GABAergic but not antidepressant medications increase risk for Clostridioides difficile infection in a national cohort of veterans. Open Forum Infect. Dis. 7, ofaa353. doi: 10.1093/ofid/ofaa353
Li, B., He, Y., Ma, J., Huang, P., Du, J., Cao, L., et al. (2019). Mild cognitive impairment has similar alterations as Alzheimer's disease in gut microbiota. Alzheimers. Dement. 15, 1357–1366. doi: 10.1016/j.jalz.2019.07.002
Li, Y., Toothaker, J. M., Ben-Simon, S., Ozeri, L., Schweitzer, R., Mccourt, B. T., et al. (2020). In utero human intestine harbors unique metabolome, including bacterial metabolites. JCI Insight 5, e138751. doi: 10.1172/jci.insight.138751
Liang, D., Leung, R. K., Guan, W., and Au, W. W. (2018). Involvement of gut microbiome in human health and disease: brief overview, knowledge gaps and research opportunities. Gut Pathog. 10, 3. doi: 10.1186/s13099-018-0230-4
Liu, R., Hong, J., Xu, X., Feng, Q., Zhang, D., Gu, Y., et al. (2017). Gut microbiome and serum metabolome alterations in obesity and after weight-loss intervention. Nat. Med. 23, 859–868. doi: 10.1038/nm.4358
Luder, C. G., Giraldo-Velasquez, M., Sendtner, M., and Gross, U. (1999). Toxoplasma gondii in primary rat CNS cells: differential contribution of neurons, astrocytes, and microglial cells for the intracerebral development and stage differentiation. Exp. Parasitol. 93, 23–32. doi: 10.1006/expr.1999.4421
Ma, Q., Xing, C., Long, W., Wang, H. Y., Liu, Q., and Wang, R. F. (2019). Impact of microbiota on central nervous system and neurological diseases: the gut-brain axis. J. Neuroinflamm. 16, 53. doi: 10.1186/s12974-019-1434-3
Makkawi, S., Camara-Lemarroy, C., and Metz, L. (2018). Fecal microbiota transplantation associated with 10 years of stability in a patient with SPMS. Neurol. Neuroimmunol. Neuroinflamm. 5, e459. doi: 10.1212/NXI.0000000000000459
Markowiak, P., and Slizewska, K. (2017). Effects of probiotics, prebiotics, and synbiotics on human health. Nutrients 9, 1021. doi: 10.3390/nu9091021
Mihaila, D., Donegan, J., Barns, S., Larocca, D., Du, Q., Zheng, D., et al. (2019). The oral microbiome of early stage Parkinson's disease and its relationship with functional measures of motor and non-motor function. PLoS ONE 14, e0218252. doi: 10.1371/journal.pone.0218252
Molloy, C. A., and Manning-Courtney, P. (2003). Prevalence of chronic gastrointestinal symptoms in children with autism and autistic spectrum disorders. Autism 7, 165–171. doi: 10.1177/1362361303007002004
Moore, D. J., West, A. B., Dawson, V. L., and Dawson, T. M. (2005). Molecular pathophysiology of Parkinson's disease. Annu. Rev. Neurosci. 28, 57–87. doi: 10.1146/annurev.neuro.28.061604.135718
Motil, K. J., Caeg, E., Barrish, J. O., Geerts, S., Lane, J. B., Percy, A. K., et al. (2012). Gastrointestinal and nutritional problems occur frequently throughout life in girls and women with Rett syndrome. J. Pediatr. Gastroenterol. Nutr. 55, 292–298. doi: 10.1097/MPG.0b013e31824b6159
Mulak, A., and Bonaz, B. (2015). Brain-gut-microbiota axis in Parkinson's disease. World J. Gastroenterol. 21, 10609–10620. doi: 10.3748/wjg.v21.i37.10609
Navarro, F., Liu, Y., and Rhoads, J. M. (2016). Can probiotics benefit children with autism spectrum disorders? World J. Gastroenterol. 22, 10093–10102. doi: 10.3748/wjg.v22.i46.10093
O'grady, K., Knight, D. R., and Riley, T. V. (2021). Antimicrobial resistance in Clostridioides difficile. Eur. J. Clin. Microbiol. Infect. Dis. 40, 2459–2478. doi: 10.1007/s10096-021-04311-5
Padhi, P., Worth, C., Zenitsky, G., Jin, H., Sambamurti, K., Anantharam, V., et al. (2022). Mechanistic insights into gut microbiome dysbiosis-mediated neuroimmune dysregulation and protein misfolding and clearance in the pathogenesis of chronic neurodegenerative disorders. Front. Neurosci. 16, 836605. doi: 10.3389/fnins.2022.836605
Parashar, A., and Udayabanu, M. (2017). Gut microbiota: implications in Parkinson's disease. Parkinsonism Relat. Disord. 38, 1–7. doi: 10.1016/j.parkreldis.2017.02.002
Park, S. H., Lee, J. H., Shin, J., Kim, J. S., Cha, B., Lee, S., et al. (2021). Cognitive function improvement after fecal microbiota transplantation in Alzheimer's dementia patient: a case report. Curr. Med. Res. Opin. 37:10. doi: 10.1080/03007995.2021.1957807
Pasquaretta, C., Gomez-Moracho, T., Heeb, P., and Lihoreau, M. (2018). Exploring interactions between the gut microbiota and social behavior through nutrition. Genes 9, 534. doi: 10.3390/genes9110534
Pequegnat, B., Sagermann, M., Valliani, M., Toh, M., Chow, H., Allen-Vercoe, E., et al. (2013). A vaccine and diagnostic target for Clostridium bolteae, an autism-associated bacterium. Vaccine 31, 2787–2790. doi: 10.1016/j.vaccine.2013.04.018
Petrov, V. A., Saltykova, I. V., Zhukova, I. A., Alifirova, V. M., Zhukova, N. G., Dorofeeva, Y. B., et al. (2017). Analysis of gut microbiota in patients with Parkinson's disease. Bull. Exp. Biol. Med. 162, 734–737. doi: 10.1007/s10517-017-3700-7
Rhee, S. H., Pothoulakis, C., and Mayer, E. A. (2009). Principles and clinical implications of the brain-gut-enteric microbiota axis. Nat. Rev. Gastroenterol. Hepatol. 6, 306–314. doi: 10.1038/nrgastro.2009.35
Rossen, N. G., Macdonald, J. K., De Vries, E. M., D'haens, G. R., De Vos, W. M., Zoetendal, E. G., et al. (2015). Fecal microbiota transplantation as novel therapy in gastroenterology: a systematic review. World J. Gastroenterol. 21, 5359–5371. doi: 10.3748/wjg.v21.i17.5359
Santocchi, E., Guiducci, L., Prosperi, M., Calderoni, S., Gaggini, M., Apicella, F., et al. (2020). Effects of probiotic supplementation on gastrointestinal, sensory and core symptoms in autism spectrum disorders: a randomized controlled trial. Front Psychiatry 11, 550593. doi: 10.3389/fpsyt.2020.550593
Santos, S. F., De Oliveira, H. L., Yamada, E. S., Neves, B. C., and Pereira, A. Jr. (2019). The gut and Parkinson's disease-a bidirectional pathway. Front. Neurol. 10, 574. doi: 10.3389/fneur.2019.00574
Savage, D. C. (1977). Microbial ecology of the gastrointestinal tract. Annu. Rev. Microbiol. 31, 107–133. doi: 10.1146/annurev.mi.31.100177.000543
Scheperjans, F., Aho, V., Pereira, P. A., Koskinen, K., Paulin, L., Pekkonen, E., et al. (2015). Gut microbiota are related to Parkinson's disease and clinical phenotype. Mov. Disord. 30, 350–358. doi: 10.1002/mds.26069
Scheperjans, F., Derkinderen, P., and Borghammer, P. (2018). The gut and Parkinson's disease: hype or hope? J. Parkinsons. Dis. 8, S31–S39. doi: 10.3233/JPD-181477
Schwarz, E., Maukonen, J., Hyytiainen, T., Kieseppa, T., Oresic, M., Sabunciyan, S., et al. (2018). Analysis of microbiota in first episode psychosis identifies preliminary associations with symptom severity and treatment response. Schizophr. Res. 192, 398–403. doi: 10.1016/j.schres.2017.04.017
Severance, E. G., Gressitt, K. L., Stallings, C. R., Katsafanas, E., Schweinfurth, L. A., Savage, C. L., et al. (2016). Candida albicans exposures, sex specificity and cognitive deficits in schizophrenia and bipolar disorder. NPJ Schizophr. 2, 16018. doi: 10.1038/npjschz.2016.18
Shaaban, S. Y., El Gendy, Y. G., Mehanna, N. S., El-Senousy, W. M., El-Feki, H. S. A., Saad, K., et al. (2018). The role of probiotics in children with autism spectrum disorder: a prospective, open-label study. Nutr. Neurosci. 21, 676–681. doi: 10.1080/1028415X.2017.1347746
Shultz, S. R., Aziz, N. A., Yang, L., Sun, M., Macfabe, D. F., and O'brien, T. J. (2015). Intracerebroventricular injection of propionic acid, an enteric metabolite implicated in autism, induces social abnormalities that do not differ between seizure-prone (FAST) and seizure-resistant (SLOW) rats. Behav. Brain Res. 278, 542–548. doi: 10.1016/j.bbr.2014.10.050
Song, Y., Liu, C., Finegold, S., and Real-Time, P. (2004). Quantitation of clostridia in feces of autistic children. Appl. Environ. Microbiol. 70, 6459–6465. doi: 10.1128/AEM.70.11.6459-6465.2004
Strandwitz, P., Kim, K. H., Terekhova, D., Liu, J. K., Sharma, A., Levering, J., et al. (2019). GABA-modulating bacteria of the human gut microbiota. Nat. Microbiol. 4, 396–403. doi: 10.1038/s41564-018-0307-3
Strati, F., Cavalieri, D., Albanese, D., De Felice, C., Donati, C., Hayek, J., et al. (2016). Altered gut microbiota in Rett syndrome. Microbiome 4, 41. doi: 10.1186/s40168-016-0185-y
Sun, J., Xu, J., Ling, Y., Wang, F., Gong, T., Yang, C., et al. (2019). Fecal microbiota transplantation alleviated Alzheimer's disease-like pathogenesis in APP/PS1 transgenic mice. Transl. Psychiatry 9, 189. doi: 10.1038/s41398-019-0525-3
Sun, M. F., and Shen, Y. Q. (2018). Dysbiosis of gut microbiota and microbial metabolites in Parkinson's disease. Ageing Res. Rev. 45, 53–61. doi: 10.1016/j.arr.2018.04.004
Tamtaji, O. R., Taghizadeh, M., Daneshvar Kakhaki, R., Kouchaki, E., Bahmani, F., Borzabadi, S., et al. (2019). Clinical and metabolic response to probiotic administration in people with Parkinson's disease: a randomized, double-blind, placebo-controlled trial. Clin. Nutr. 38, 1031–1035. doi: 10.1016/j.clnu.2018.05.018
Thion, M. S., Low, D., Silvin, A., Chen, J., Grisel, P., Schulte-Schrepping, J., et al. (2018). Microbiome influences prenatal and adult microglia in a sex-specific manner. Cell 172, 500–516.e516. doi: 10.1016/j.cell.2017.11.042
Unger, M. M., Spiegel, J., Dillmann, K. U., Grundmann, D., Philippeit, H., Burmann, J., et al. (2016). Short chain fatty acids and gut microbiota differ between patients with Parkinson's disease and age-matched controls. Parkinsonism Relat. Disord. 32, 66–72. doi: 10.1016/j.parkreldis.2016.08.019
Vendrik, K. E. W., Ooijevaar, R. E., De Jong, P. R. C., Laman, J. D., Van Oosten, B. W., Van Hilten, J. J., et al. (2020). Fecal microbiota transplantation in neurological disorders. Front. Cell. Infect. Microbiol. 10, 98. doi: 10.3389/fcimb.2020.00098
Vinithakumari, A. A., Padhi, P., Hernandez, B., Lin, S. J., Dunkerson-Kurzhumov, A., Showman, L., et al. (2022). Clostridioides difficile infection dysregulates brain dopamine metabolism. Microbiol. Spectr. 10, e0007322. doi: 10.1128/spectrum.00073-22
Vogt, N. M., Kerby, R. L., Dill-Mcfarland, K. A., Harding, S. J., Merluzzi, A. P., Johnson, S. C., et al. (2017). Gut microbiome alterations in Alzheimer's disease. Sci. Rep. 7, 13537. doi: 10.1038/s41598-017-13601-y
Vuong, H. E., Pronovost, G. N., Williams, D. W., Coley, E. J. L., Siegler, E. L., Qiu, A., et al. (2020). The maternal microbiome modulates fetal neurodevelopment in mice. Nature 586, 281–286. doi: 10.1038/s41586-020-2745-3
Wang, H., Lee, I. S., Braun, C., and Enck, P. (2016). Effect of probiotics on central nervous system functions in animals and humans: a systematic review. J. Neurogastroenterol. Motil. 22, 589–605. doi: 10.5056/jnm16018
Wang, L., Christophersen, C. T., Sorich, M. J., Gerber, J. P., Angley, M. T., and Conlon, M. A. (2012). Elevated fecal short chain fatty acid and ammonia concentrations in children with autism spectrum disorder. Dig. Dis. Sci. 57, 2096–2102. doi: 10.1007/s10620-012-2167-7
Wang, L. W., Tancredi, D. J., and Thomas, D. W. (2011). The prevalence of gastrointestinal problems in children across the United States with autism spectrum disorders from families with multiple affected members. J. Dev. Behav. Pediatr. 32, 351–360. doi: 10.1097/DBP.0b013e31821bd06a
Wickramage, I., Spigaglia, P., and Sun, X. (2021). Mechanisms of antibiotic resistance of Clostridioides difficile. J. Antimicrob. Chemother. 76, 3077–3090. doi: 10.1093/jac/dkab231
Wimo, A., Guerchet, M., Ali, G. C., Wu, Y. T., Prina, A. M., Winblad, B., et al. (2017). The worldwide costs of dementia 2015 and comparisons with 2010. Alzheimers. Dement. 13, 1–7. doi: 10.1016/j.jalz.2016.07.150
Winter, G., Hart, R. A., Charlesworth, R. P. G., and Sharpley, C. F. (2018). Gut microbiome and depression: what we know and what we need to know. Rev. Neurosci. 29, 629–643. doi: 10.1515/revneuro-2017-0072
Wortelboer, K., Nieuwdorp, M., and Herrema, H. (2019). Fecal microbiota transplantation beyond Clostridioides difficile infections. EBioMedicine 44, 716–729. doi: 10.1016/j.ebiom.2019.05.066
Xie, C., and Prasad, A. A. (2020). Probiotics treatment improves hippocampal dependent cognition in a rodent model of Parkinson's disease. Microorganisms 8, 1661. doi: 10.3390/microorganisms8111661
Xu, H. M., Huang, H. L., Zhou, Y. L., Zhao, H. L., Xu, J., Shou, D. W., et al. (2021). Fecal microbiota transplantation: a new therapeutic attempt from the gut to the brain. Gastroenterol. Res. Pract. 2021, 6699268. doi: 10.1155/2021/6699268
Yano, J. M., Yu, K., Donaldson, G. P., Shastri, G. G., Ann, P., Ma, L., et al. (2015). Indigenous bacteria from the gut microbiota regulate host serotonin biosynthesis. Cell 161, 264–276. doi: 10.1016/j.cell.2015.02.047
Keywords: C. difficile, brain diseases, microbiota, gut-brain, fecal microbiota transplantation
Citation: Biazzo M, Allegra M and Deidda G (2022) Clostridioides difficile and neurological disorders: New perspectives. Front. Neurosci. 16:946601. doi: 10.3389/fnins.2022.946601
Received: 17 May 2022; Accepted: 24 August 2022;
Published: 20 September 2022.
Edited by:
Mohammad Bashashati, Texas Tech University Health Sciences Center, United StatesReviewed by:
Shankumar Mooyottu, Iowa State University, United StatesCopyright © 2022 Biazzo, Allegra and Deidda. This is an open-access article distributed under the terms of the Creative Commons Attribution License (CC BY). The use, distribution or reproduction in other forums is permitted, provided the original author(s) and the copyright owner(s) are credited and that the original publication in this journal is cited, in accordance with accepted academic practice. No use, distribution or reproduction is permitted which does not comply with these terms.
*Correspondence: Gabriele Deidda, Z2FicmllbGUuZGVpZGRhQHVuaXBkLml0
Disclaimer: All claims expressed in this article are solely those of the authors and do not necessarily represent those of their affiliated organizations, or those of the publisher, the editors and the reviewers. Any product that may be evaluated in this article or claim that may be made by its manufacturer is not guaranteed or endorsed by the publisher.
Research integrity at Frontiers
Learn more about the work of our research integrity team to safeguard the quality of each article we publish.