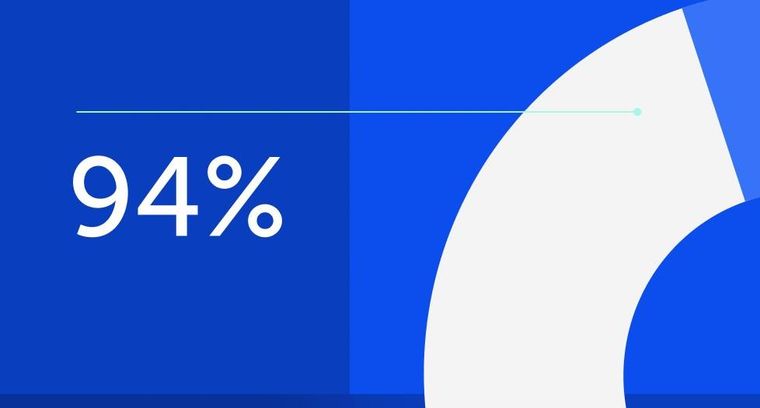
94% of researchers rate our articles as excellent or good
Learn more about the work of our research integrity team to safeguard the quality of each article we publish.
Find out more
REVIEW article
Front. Neurosci., 22 July 2022
Sec. Neuropharmacology
Volume 16 - 2022 | https://doi.org/10.3389/fnins.2022.922331
This article is part of the Research TopicNovel Therapeutic Target and Drug Discovery for Neurological DiseasesView all 21 articles
In addition to its profound implications in the fight against cancer, pyroptosis have important role in the regulation of neuronal injury. Microglia are not only central members of the immune regulation of the central nervous system (CNS), but are also involved in the development and homeostatic maintenance of the nervous system. Under various pathological overstimulation, microglia pyroptosis contributes to the massive release of intracellular inflammatory mediators leading to neuroinflammation and ultimately to neuronal damages. In addition, microglia pyroptosis lead to further neurological damage by decreasing the ability to cleanse harmful substances. The pathogenic roles of microglia in a variety of CNS diseases such as neurodegenerative diseases, stroke, multiple sclerosis and depression, and many other neurological disorders have been gradually unveiled. In the context of different neurological disorders, inhibition of microglia pyroptosis by targeting NOD-like receptor family pyrin domain containing (NLRP) 3, caspase-1 and gasdermins (GSDMs) by various chemical agents as well as natural products significantly improve the symptoms or outcome in animal models. This study will provide new ideas for immunomodulatory treatment of CNS diseases.
The term “pyroptosis” is of ancient Greek origin, meaning fire and falling, it is characterized by early rupture of the plasma membrane and release of proinflammatory intracellular contents (Black et al., 1989). Therefore, pyroptosis is also known as inflammatory cell death in recent years (Van Opdenbosch and Lamkanfi, 2019). External stimuli such as pathogen-associated molecular patterns (PAMPs), damage-associated molecular patterns (DAMPs), dsDNA, multiple bacterial, viral antigenic components initiate cellular pyroptosis by activating different types of inflammasome receptors (Xue et al., 2019). Cell pyroptosis is mainly executed by the gasdermin family of proteins (Shi et al., 2017). The N-terminal end of the GSDM is activated by the shearing action of caspase family members. The activated GSDM-N terminus has membrane pore-forming properties and induces the formation of transmembrane pore channels and pyroptosis, along with the release of intracellularly activated inflammatory mediators (Bergsbaken et al., 2009; Shi et al., 2017). The GSDM plays an important role in the body's anti-infection and anti-cancer immunity and is expected to be a new target for anti-cancer therapy (Man et al., 2017; Loveless et al., 2021; Wu et al., 2021). However, cellular damage and inflammation caused by excessive and abnormal pyroptosis have been found to be important pathogenic factors in a variety of diseases, such as cardiovascular diseases, motor system diseases, autoimmune diseases (McKenzie et al., 2018; Tao et al., 2020; Wang et al., 2020c). Recent studies have shown that neuronal pyroptosis is associated with neurodegenerative diseases, stroke, traumatic brain injury (TBI), infection-induced brain damage, and a variety of central nervous system (CNS) pathologies such as epilepsy (McKenzie et al., 2020a). Pyroptosis has become the focus of research on the pathogenic mechanisms of the CNS.
Microglia are the resident innate immune cells of the CNS with important immunomodulatory functions and play a critical role in the neuronal development, myelin repair, and regeneration of the nervous system (Colonna and Butovsky, 2017; Lloyd and Miron, 2019). They can regulate inflammation and oxidative stress and influence synaptic plasticity and blood-brain barrier (BBB) stability, which are essential for the regulation of CNS homeostasis (Li and Barres, 2018; Zhou et al., 2019; Ronaldson and Davis, 2020). Although the concept of microglia polarization is currently controversial, the binary classification of microglia according to their beneficial or harmful function is still widely used today (Hu et al., 2015; Ransohoff, 2016a; Wan et al., 2022b). Recent studies suggest that its function is not limited to regulating development and simply removing cellular debris in disease, but that its abundance of modifiable genes also provides a large number of targets for regulating neurological diseases (Prinz et al., 2021). Given the multifunctional regulatory properties of microglia in the CNS, their association with epilepsy, neuropathic pain, ischemic stroke, neurodegenerative diseases, and depression are also being preliminarily revealed (Orihuela et al., 2016; Nestle et al., 2020; Yao et al., 2021). The links between the physiopathological functional state of microglia and various neurological diseases have been extensively investigated.
Microglia pyroptosis is an important manifestation of neuroinflammation and is closely associated with the development of several neurological diseases, such as ischemic brain injury, stroke, and neurodegenerative diseases. Microglia pyroptosis-induced inflammatory responses are associated with neurodegeneration and cell death in the brains of Parkinson's patients (Zhang et al., 2016). IL-1β and IL-18 levels were found to be significantly higher in the cerebrospinal fluid of Parkinson's patients with microglia pyroptosis than in the healthy controls (Zhang et al., 2016; Chang et al., 2020; Hu et al., 2020; Xu et al., 2021b). In addition, it was shown that inhibition of microglia pyroptosis by reducing the levels of the NLRP3 complex with CD73 or reduced advanced oxidation protein products (AOPPs) alleviated spinal cord injury (SCI) induced by persistent neuroinflammation (Liu et al., 2020; Xu et al., 2021b). In a mouse model of intracerebral hemorrhage (ICH), inhibiting microglia pyroptosis by suppressing NLRP3 complexes could improve SCI symptoms. In the ICH mouse model, the anti-inflammatory effect of Didymin was partially associated with its anti-microglia pyroptosis effect (Gu et al., 2022). This paper reviews the recent association between microglia pyroptosis and various neurological disorders, as well as the currently available or potential agents that regulate microglia pyroptosis, thus providing new ideas for the treatment of neurological disorders.
Pyroptosis is a type of programmed cell death but is different from apoptosis and necrosis. Both pyroptosis and necrosis are part of the same lytic programmed death and eventually release contents leading to inflammation (Frank and Vince, 2019). However, the mechanisms and cell morphology are different, which may be due to the different biochemical activities of the gasdermin D (GSDMD) and mixed lineage kinase domain-like proteins (MLKL) that mediate pyroptosis (Jorgensen and Miao, 2015). Pyroptosis is one of the defense mechanisms of host cells against pathogens. Usually external pathogens promote cellular pyroptosis activation, while a few pathogens have the ability to inhibit host cell pyroptosis (Bergsbaken et al., 2009). With the rupture of the plasma membrane, exposed extracellular pathogens are further targeted and cleared by recruited immune cells. Classical microglia pyroptosis is divided into two main steps. The initiation step refers mainly to microbial and inflammatory mediator-mediated activation of inflammation-associated genes. This is followed by the activation of inflammasome and downstream pyroptosis-related pathways, culminating in cell death mediated by the pore-forming protein GSDMD (Vande Walle and Lamkanfi, 2016; Fang et al., 2020). In addition, the non-classical pyroptosis pathway GSDMD activation is independent of caspase-1. Caspase-4/5/11 are directly stimulated by LPS and thus activate downstream GSDMD (Shi et al., 2014).
Inflammasomes are multiprotein complexes containing pattern recognition receptors (PRRs) that mediate the innate immune response of the body to infectious microbes and host protein molecules. The PRR family typically contains multiple members, including Toll-like receptors (TLRs), Nod-like receptors (NLRs) (Kanneganti et al., 2007; Sahoo, 2020). PRRs in inflammasomes sense and recognize PAMPs and DAMPs of host or environmental origin (Guo et al., 2015). In mammals, in addition to the most common NLRP3, different types of inflammasome receptors such as absent in melanoma 2 (AIM2), NLRC4, NLRP1b, and NLRP6 recognize different activation signals and activate downstream caspase-1 (Xue et al., 2019; Fang et al., 2020). Upon activation, caspase-1 mediates the shear activation of IL-1β and IL-18, as well as GSDMD, leading to the formation of membrane pores, cell swelling, release of cell contents, and ultimately pyroptosis (Voet et al., 2019; Wang et al., 2019; Xue et al., 2019). Because NLRP3 inflammasomes have a wide recognition range, targeting NLRP3 to regulate pyroptosis has become one of the focal points of current research. Isoflurane general anesthesia induces pyroptosis by activating NLRP3 inflammasomes, while NLRP3 inhibitor MCC950 attenuates pyroptosis-related cognitive dysfunction (Fan et al., 2018). Additionally, isoliquiritin and kanglexin improve depression by downregulating NLRP3 levels and subsequent neuronal pyroptosis (Bian et al., 2020; Li et al., 2021a).
GSDMD is highly expressed in the epithelium and skin of the gastrointestinal tract, yet its function remains largely unknown. As the executioner of pyroptosis, GSDMD is only one member of the GSDM family, with other members including GSDMA, GSDMB, GSDMC, GSDMD, DFNA5, and DFNB59 (Shi et al., 2017). In the GSDM family, the sequence homology is about 45%, with the GSDM-N structural domain being the most conserved region. It was concluded that the GSDM-N domain of GSDMD is thought to possess extremely strong pore-forming toxicity and then induce pyroptosis (Shi et al., 2015; Ding et al., 2016). In studies in mouse models, different species of GSDM activation have similar pore-forming properties. At present, the upstream and downstream signal regulation mechanism of pyroptosis executive protein GSDMD is clearly studied (Shi et al., 2017). Recent studies have found that streptococcal pyrogenic exotoxin B shears GSDMA and thus induce pyroptosis, while the upstream regulatory mechanisms of the remaining GSDM members are still unknown (Deng et al., 2022). The GSDMD proteins are in an autoinhibited state in the normal state (Ding et al., 2016). GSDMD contains about 480 amino acids, and it is connected to two structural domains, GSDM-N terminus and GSDM-C terminus, by a long loop. Activated caspase-1 and caspase-11 efficiently cleave the GSDMD at an aspartate site within the loop. This cleavage is essential for release of pore-forming GSDM-N terminus (Shi et al., 2015). The role of pyroptosis in CNS disease has emerged in multiple animal disease models. VX-765, a small molecule inhibitor of caspase-1, reduces the expression of inflammasomes and pyroptosis-associated proteins in the CNS, thereby inhibiting axonal injury in multiple sclerosis (MS) (McKenzie et al., 2018). Caspase-1 gene ablation in mice model significantly inhibited TBI-induced pyroptosis and neurological damage (Liu et al., 2018).
Studies on the molecular regulation of its non-canonical pathway of pyroptosis, especially caspase-11-related, are currently more limited. LPS-mediated caspase-11-dependent non-classical pyroptosis pathway can be inhibited by ethyl pyruvate (Qiu et al., 2020). Regulation of caspase-11 is thought to be possibly related to phosphodiesterase 8A (PDE8A) mediated cyclic adenosine monophosphate (cAMP) metabolism (Hou et al., 2019). Adenosine diphosphate (ADP) -ribosylation of caspase-11 was found to protect cells from pyroptosis in Shigella-infected mice (Li et al., 2021b). AIM2 is also a target for regulating pyroptosis. In vitro, andrographolide significantly inhibited AIM2 inflammasomes and blocked caspase1/GSDMD-mediated myeloid-derived macrophage pyroptosis (Gao et al., 2019). LncRNA MEG3 induced cellular pyroptosis of middle cerebral artery occlusion (MCAO) mice in ischemic brain by sponging miR-485 targeting AIM2 (Liang et al., 2020a). Thus, non-coding RNAs are also important for regulating pyroptosis. In cellular experiments in diabetic retinopathy, overexpression of miR-590-3p was found to downregulate caspase-1-dependent pyroptosis via downregulation of NLRP1 and downstream NADPH oxidase 4 (NOX4) pathway (Gu et al., 2019). As the study of pyroptosis in human diseases has advanced, chemotherapeutic drugs and miRNAs have now been found to inhibit malignant progression of tumors by inducing tumor pyroptosis (Xia et al., 2019). Targeting pyroptosis has an unignorable role in the treatment of diseases.
There is a link between apoptosis and pyroptosis. After apoptosis, the absence of macrophages to remove the apoptotic cells trigger GSDMD-mediated secondary cell death with a pathological pattern similar to pyroptosis (Kovacs and Miao, 2017; Rogers et al., 2017). When GSDMD expression is too low or in the presence of GSDMD defects, caspase-1 induces apoptosis via the Bid-caspase 9-caspase 3 axis or caspase-7 (Taabazuing et al., 2017; Tsuchiya et al., 2019). However, if the cleavage site of GSDMD is designed as a recognition site for caspase-3, it may convert apoptosis into pyroptosis (Wang et al., 2017). In addition, oxidation of phospholipids may also be associated with GSDMD activation-induced pyroptosis. Glutathione peroxidase 4 (GPX4) and vitamin E inhibit pyroptosis in mouse macrophages by suppressing lipid peroxidation (Imai and Nakagawa, 2003). In contrast, myeloid-specific GPX4 deficiency leads to a significant increase in caspase-1 and caspase-11-mediated GSDMD lysis (Kang et al., 2018). Meanwhile, GPX4 plays an important role in regulating ferroptosis. This suggests the potential connection between pyroptosis and ferroptosis.
Neurodegenerative diseases are a series of disorders caused by progressive loss of neurons in the CNS (Yu et al., 2017). The main pathological changes include amyloid deposition and progressive neurodegenerative changes. Among them, amyloid proteins including Aβ, tau and α-synuclein exhibit similar properties to prion proteins in pathological experiments and are able to self-replicate and spread throughout the nervous system (Vaquer-Alicea and Diamond, 2019; Tian et al., 2020). Microglia play an important role in the course of neurodegenerative diseases (Wan et al., 2022a). In the development of Alzheimer's disease (AD), the caspase activation and recruitment domain (CARD/ASC)-containing bridging proteins released by microglia pyroptosis rapidly bind to Aβ and increase the formation of Aβ oligomers and aggregates (Venegas et al., 2017). The formed ASC-Aβ complex also leads to multiple responses in surrounding cells, such as increased caspase-1 activation, IL-1β maturation and GSDMD cleavage, and promotes NLRP3 inflammasome formation and pyroptosis in neighboring microglia (Heneka et al., 2018; Luciunaite et al., 2020). In addition, microglia pyroptosis lead to more ASC release, thereby exacerbating pyroptosis-induced neuroinflammatory damage (Friker et al., 2020). The ASC-Aβ complex also promotes microglia activation and secretion of inflammatory mediators and neurotoxic cytokines, while the efficiency of Aβ degradation in microglia is reduced (Sarlus and Heneka, 2017). In the early stages of AD, microglia in patients are activated by Aβ, which is removed by receptor-mediated phagocytosis and degradation, inhibiting to some extent the deposition of Aβ in the interstitial (Newcombe et al., 2018). However, as Aβ accumulates, microglia are continuously activated and produce excessive amounts of pro-inflammatory cytokines (Van Zeller et al., 2021). In addition, the autocrine secretion of membrane receptors from microglia that bind and help microglia phagocytose Aβ gradually decreases, and the activity of various degradative enzymes, such as enkephalinase, insulin-degrading enzymes, and angiotensin-converting enzyme I (ACEI) decreases, further leading to reduced clearance of Aβ and continued microglial cell stimulation (Yu and Ye, 2015; Van Zeller et al., 2021). Researchers have speculated that tau and Aβ are functionally similar. Tau protein also activates inflammasomes and induces microglia pyroptosis via the NLRP3-ASC axis (Stancu et al., 2019). This finding is supported by the results of several necropsy analyses (Ransohoff, 2016b; Leyns and Holtzman, 2017).
The pathological marker of Parkinson's disease (PD) differs considerably from that of AD. The pathological marker of PD is fibrillar α-synuclein, which tends to accumulate in neurons and eventually leads to the formation of Lewy bodies (Przedborski, 2017). Similar to Aβ, α-synuclein also induces the activation of microglia NLRP3 inflammasomes and thus promotes the release of ASC from microglia and the formation of extracellular ASC patches (Zhou et al., 2016; de Alba, 2019). Delayed and strong activation of NLRP3 inflammasomes and a significant increase in extracellular ASC release were observed in LPS and α-synuclein-stimulated activated mouse microglia, but microglia did not undergo pyroptosis (Gordon et al., 2018). Furthermore, activation of pyroptosis-related pathways was associated with the metabolism of α-synuclein. It was shown that the activation and aggregation of inflammasomes in neuronal cells are closely related to the cleavage of extracellular α-synuclein (Wang et al., 2016; Hu et al., 2022). In BE (2)-M17 human dopaminergic neuroblastoma cells, caspase-1 activated by inflammasomes was found to cleave α-synuclein in vitro and produce aggregates with neuronal toxicity (Wang et al., 2016). In a rat model of PD induced by LPS and 6-hydroxydopamine (6-OHDA), NLRP3 inflammasomes components were found to be highly expressed in microglia, and caspase-1 inhibitor (Ac-YVAD-CMK) reversed this result (Mao et al., 2017). This suggests that microglia pyroptosis may be associated with pathological cleavage of extracellular α-synuclein and the formation of Lewy bodies. However, whether α-synuclein ultimately induces microglia pyroptosis may depend on the concentration of α-synuclein, and the quantification of this concentration needs to be determined by further studies. Furthermore, in N-methyl-4-phenyl-1,2,3,6-tetrahydropyridine (MPTP)-induced PD mice, baicalein inhibited NLRP3/caspase-1/GSDMD pathway-mediated microglia pyroptosis, thereby reducing PD symptoms (Rui et al., 2020). These studies suggest that inhibition of microglia pyroptosis may alleviate the progression of PD.
Caspase-1 plays a key role in the process of pyroptosis. The activation of Caspase-1 exists in the brain of Huntington's disease (HD) patients and in HD mouse models, and the inhibition of caspase-1 in HD mouse models can slow down the progression of the disease (Ona et al., 1999; Paldino et al., 2020). Huntington's protein (HTT) is the key to the disease and activated caspase-1 hydrolyzes and cleaves HTT to produce an N-terminal mutated fragment (N-htt), leading to neuronal dysfunction and death (Kim et al., 2001; Sanchez Mejia and Friedlander, 2001). This suggests that pyroptosis may be potentially linked to the pathological generation of HTT. The current study shows that HD patients have distinct neuroinflammatory features at the site of brain lesions, while no upregulation of immune cells from the periphery, such as lymphocytes and neutrophils, was found in the brain tissue of HD patients (Bjorkqvist et al., 2008; Palpagama et al., 2019). Significant NLRP3 activation was found in microglia in a mouse model of HD, suggesting a potential link between neuroinflammation triggered by microglia pyroptosis and HD (Siew et al., 2019). However, a different result has been obtained that NLRP3 expression levels were significantly elevated in other cells of the striatum of HD mice, but no significant NLRP3 activation was found in microglia (Paldino et al., 2020). This heterogeneity of results due to the spatial location of the brain needs to be elucidated by more in-depth studies.
Ischemic stroke and secondary cerebral ischemia-reperfusion injury are both very serious cerebrovascular diseases (Graeser et al., 2019). Ischemia and hypoxia trigger a series of neurological damage responses such as oxidative stress and neuroinflammation (Langhauser et al., 2012; Nabavi et al., 2015). Neuroinflammation induced by microglia pyroptosis is thought to be a key factor promoting neuronal damage after ischemia (Ceulemans et al., 2010). On the one hand, neuroinflammation promotes the clearance of dead cellular debris induced by reduced cerebral blood flow and ischemia-reperfusion (Xu et al., 2019a). On the other hand, it may lead to infarct exacerbation and low neuronal plasticity (Kriz, 2006). Microglia are one of the most important phagocytic cells for the removal of necrotic substances, but hyperactivation-induced microglia pyroptosis is an important cause of exacerbation of neuroinflammation-related damage after stroke (Iadecola and Anrather, 2011; Xu et al., 2019a). In the mouse MCAO-induced I/R model, microglia GSDMD expression is elevated in the ischemic region, which mediates microglia pyroptosis and neuroinflammation-related injury (Voet et al., 2019; Zhang et al., 2019; Wang et al., 2020b).
It has been found that NLRP3 expression is increased in microglia of ischemic stroke patients, and increased expression of NLRP3 inflammasomes component proteins and downstream products IL-1β and IL-18 was also observed in the mouse MCAO/R model (Fann et al., 2013). Many studies have revealed that the mechanism of NLRP3 inflammasome in microglia involved in the regulation of cerebral ischemic injury may be related to the NF-κB pathway, mitogen-activated protein kinase (MAPK) signaling pathway, Hypoxia Inducible Factor-1α (HIF-1α), reactive oxygen species (ROS) production (Ma et al., 2014; Fann et al., 2018; Jiang et al., 2020). In oxygen-glucose deprivation and reoxygenation (OGD/R) and MCAO/R-treated rat BV2 microglia, Salidroside (Sal) was found to inhibit microglia NLRP3 inflammasomes activation by suppressing the TLR4/NF-κB signaling pathway, thereby inhibited I/R-induced BV2 cells pyroptosis and further neuronal damage (Liu et al., 2021). A similar phenomenon was observed from the Meisoindigo-treated MCAO mouse model (Ye et al., 2019; Liu et al., 2021). These studies suggest that amelioration of ischemic stroke can be achieved by inhibiting the TLR4/NF-κB signaling pathway. In a human cell assay, ROS levels in OGD/R-treated human BV2 microglia were significantly higher than those in the untreated group, and co-culture with hypoxia-pretreated olfactory mucosa mesenchymal stem cells (OM-MSCs) showed reduced ROS levels and less pyroptosis (Huang et al., 2020). OM-MSCs have immunomodulatory and reparative functions and replace or repair damaged cells, and OM-MSCs upregulate the expression and release of HIF-1α under OGD/R conditions, suppressing the expression of NLRP3 inflammasome and pyroptosis-related proteins in co-cultured BV2 microglia and reducing the pyroptosis of BV2 microglia under OGD/R conditions (Coppin et al., 2019; Dabrowska et al., 2019; Huang et al., 2020). These studies suggest that ischemia-induced production of HIF-1α and ROS play a key role in pyroptosis regulation in BV2 microglia under OGD/R conditions. Another study found that NLRP3 inflammasomes expression levels were not altered in a mouse OGD model, and only NLRC4 inflammasome expression was upregulated and induced BV2 microglia pyroptosis. Knockdown of NLRC4 by siRNA significantly reduced pyroptosis in microglia under ischemic conditions (Poh et al., 2019). The difference in NLRP3 and NLRC4 expression under ischemic stroke conditions remains to be elucidated.
MS is an incurable progressive demyelinating disease of the CNS characterized by multiple demyelinating plaques in the white matter, neurodegeneration, and axonal transection or loss (Kornek and Lassmann, 2003; Dendrou et al., 2015). The etiology of MS is not yet clear. Previous studies have shown increased expression of NLRP3 inflammasomes activation and its downstream products in CNS tissue and peripheral serum in MS patients compared to non-MS patients, leading to BBB damage and neurotoxicity (Huang et al., 2004; Burm et al., 2016; McKenzie et al., 2018). In addition, a study found a large number of GSDMD-immunopositive cell fragments in the frontal white matter of cadavers from MS patients, suggesting a potential link between GSDMD-mediated microglia pyroptosis and MS (McKenzie et al., 2018). Because MS is a human-specific disease, it can only be partially simulated in animal models, such as the animal experimental autoimmune encephalomyelitis (EAE) model, which has approximately the same neuropathological features as MS and assist in determining the factors influencing MS (Ransohoff, 2012; Kipp et al., 2017). It has been found that the expression level of NLRP3/ASC-caspase/GSDMD pathway is significantly increased in mouse EAE models, while the use of Liraglutide significantly downregulates the protein level of caspase-1 and microglia pyroptosis levels (Song et al., 2022). Another study showed that in addition to caspase-1, caspase-3 and caspase-7 also mediated microglia pyroptosis in post-mortem brain tissue from patients with progressive MS and in a mouse EAE model. This study found that caspase-1 activates caspase-3/7 in MS patients and EAE mouse models, and caspase-3/7 and its substrates, such as PARP, DFF45 and ROCK1, after cleavage activation, induce GSDMD-mediated microglial cell pyroptosis by disrupting the cellular protein hydrolysis network and promoting microglial cell nuclear cohesion glial cell pyroptosis (McKenzie et al., 2020b).
Major depressive disorder (MDD) is a serious neuropsychiatric disorder that remains a medical management challenge (Malhi and Mann, 2018). The direct pathogenesis of depression remains unclear, and some studies now suggest a close relationship between MDD and neuroinflammation triggered by NLRP3 inflammasomes (Raedler, 2011; Dey and Hankey Giblin, 2018). In patients with MDD, untreated patients have increased levels of IL-1β and IL-18 in the circulation and increased expression of NLRP3 compared to patients treated with the antidepressant amitriptyline (Raedler, 2011). The expression of NLRP3 was increased. Elevated IL-1β mRNA and protein levels were also found in the prefrontal cortex in a chronic mild stress (CMS)-induced depression model in rats, while no similar phenomenon was observed in blood (Pan et al., 2014). The same phenomenon was not observed in blood. In the chronic unpredictable mild stress (CUMS)-induced depression mouse model, researchers found significantly elevated levels of IL-1β protein in serum and hippocampus, but not in NLRP3 knockout mice, probably because NLRP3 knockout inhibited MAPK pathway and NF-κB pathway activation (Su et al., 2017). Many studies have also revealed other mechanisms involved in the regulation of MDD by NLRP3 inflammasomes in microglia, which may be related to dysregulation of miRNA-27a/SYK/NF-κB pathway, nuclear factor-erythroid 2 -related factor 2 (Nrf2) (Ajami et al., 2011; Eggen et al., 2013; Arioz et al., 2019; Li et al., 2021a). In the LPS-induced mouse Depressive-like behavior (DLB) model, Melatonin pretreatment inhibited Keap-1-mediated Nrf2 proteasomal degradation, suppressed NLRP3 inflammasomes activation, downregulated GSDMD cleavage, and ultimately protected N9 microglia from pyroptosis (Arioz et al., 2019). Similarly, in MDD patients and in LPS or chronic social defeat stress (CSDS)-induced depression models in mice, the use of Isoliquiritin upregulated miRNA-27a expression and downregulated SYK expression, thereby protecting microglia from pyroptosis and alleviating MDD symptoms in mice (Li et al., 2021a). These experiments illustrate that microglia pyroptosis is closely linked to MDD. Interestingly, in a CMS-induced mouse depression model, astrocyte NLRP3 inflammasomes and GSDMD in the hippocampus of mice were found to activate and induce cell pyroptosis, while microglia did not show significant pyroptosis (Catanese et al., 2021) (Figure 1).
Figure 1. Pathologies of neurological diseases associated with microglia pyroptosis. In various neurological diseases, NLRP3 inflammasome in microglia can be activated and then the caspase-1 is activated. Activated caspase-1 on the one hand cleaves GSDMD, which produces N-terminal structural domain and induces cell membrane perforation and subsequent pyroptosis. On the other hand, activated caspase-1 cleaves IL-1β and IL-18 precursors, rising the extracellular level of IL-1β and IL-18 and amplify neuroinflammatory responses. In neurodegenerative diseases, various misfolded aggregated proteins are taken up by microglia, leading to NLRP3 inflammasome activation and microglia pyroptosis. While in ischemia stroke, ischemic necrotic cell debris is taken up by microglia performing immune clearance functions, leading to microglia NLRP3 inflammasome activation. Microglia NLRP3 inflammasome activation is also present in MDD and MS. All these diseases lead to NLRP3-dependent microglia pyroptosis and neuroinflammation. Moreover, worsening neuroinflammation promotes the production of multiple pathological markers of neurological disease and induces microglia pyroptosis, which leads to persistent disease progression.
Targeting NLRP-related proteins to regulate microglia pyroptosis affect the progression of multiple neurological diseases. Targeting NLRP to inhibit pyroptosis plays a neuroprotective role in stroke. The human-specific gene CHRFAM7A inhibits NLRP3 inflammasomes activation and reduces intracellular levels of NLRP3, a pyroptosis-related protein, thereby reducing OGD/R-induced neurological damage (Cao et al., 2021). The triggering receptor expressed on myeloid cells (TREM)-1 antagonist LP17 inhibited microglia pyroptosis and effectively improved neurological function in subarachnoid hemorrhage (SAH) patients (Liang et al., 2020b; Xu et al., 2021a). C-C chemokine receptor 5 (CCR5) activation promotes microglia pyroptosis and neurological deficits after ICH in mice via the protein kinase A (PKA)/cAMP response element binding (CREB)/NLRP1 signaling pathway. Maraviroc inhibition of CCR5 improves neurological function in ICH patients (Yan et al., 2021). Dexmedetomidine (Dex) inhibits microglia pyroptosis by blocking the purinergic 2X7 receptor (P2X7R)/NLRP3 pathway, thereby providing protection against ischemic brain injury (Sun et al., 2021). Andrographolide and Curcumin effectively reduce neurostructural damage and functional impairment caused by stroke by inhibiting NF-κB signaling and NLRP3 inflammasomes-mediated microglia pyroptosis (Li et al., 2018; Ran et al., 2021). Salvianolic acids for injection (SAFI) reduced brain ischemia reperfusion injury (BIRI) by reducing the shift in microglia phenotype from M1 to M2 and inhibiting microglia NLRP3 inflammasomes-induced pyroptosis (Ma et al., 2021). The plasma containing Melatonin has been shown to reduce BIRI. Melatonin-containing plasma exosomes inhibited ischemia-induced inflammatory responses and pyroptosis by modulating the TLR4/NF-κB signaling pathway (Wang et al., 2020a).
Targeting NLRP3 to inhibit microglia pyroptosis inhibition alleviates cognitive impairment. NLRP3 inhibitors MCC950 and the ethyl acetate fraction of Bungeanum improve cognitive function in mice by inhibiting LPS-induced caspase-1 activation and pyroptosis in microglia (Dempsey et al., 2017; Zhao et al., 2021). Curcumin treatment significantly improved diabetes mellitus (DM)/chronic cerebral hypoperfusion (CCH)-induced cognitive impairment by modulating the TREM2/TLR4/NF-κB pathway and reducing NLRP3-dependent pyroptosis (Zheng et al., 2021). Studies have shown that chronic aluminum exposure is associated with the development of AD and cognitive impairment (Klotz et al., 2017). Aluminum exposure causes microglia pyroptosis and neuroinflammation through the DEAD-box helicase 3 X-linked (DDX3X)/NLRP3 inflammasomes signaling pathway, while Resveratrol alleviates aluminum exposure-induced neurological damage by activating sirtuin1 (SIRT1) (Hao et al., 2021). Hypoxic preconditioning of the Ca2+/calcium/calmodulin-dependent protein kinase II (CaMKII)/CREB signaling pathway inhibited microglia pyroptosis and thereby improved Amyloid precursor protein (APP)/CREB signaling in presenilin-1 (PS1) mice with brain damage (Song et al., 2021).
A variety of agents effectively inhibit PD progression by targeting microglia pyroptosis. Baicalein reverses MPTP-induced neuroinflammation in mice by inhibiting the NLRP3/caspase-1/GSDMD pathway and has a role in PD treatment (Rui et al., 2020). Kaemperfol ameliorates behavioral deficits in PD rats by inhibiting p38MAPK/NF-κB pathway, inhibiting microglia activation, and downregulating pyroptosis-related proteins (Cai et al., 2022). In a mouse model of depression, Quercetin (Qu) and Isoliquiritin treatment inhibited microglia pyroptosis-mediated neurotoxicity and thus exerted antidepressant effects (Han et al., 2021; Li et al., 2021a).
Notably, nuclear factor-kappa B (NF-κB) signaling plays a key role in the formation of NLRP3 inflammasomes in the study of the whole range of pyroptosis regulation-related signals (Yuan et al., 2021). Para-aminosalicylic acid (PAS-Na) antagonizes Mn-induced activation of NLRP3 inflammasomes in the basal ganglia of rats by inhibiting activation of the NF-κB pathway and oxidative stress induced by BV2 cell pyroptosis (Peng et al., 2020). Nrf-2 activates heme oxygenase-1 (HO-1), and Dimethyl itaconate (DI) are involved in the Nrf-2/ HO-1 pathway inhibit NLRP3 inflammasomes assembly and GSDMD cleavage and induces cellular autophagy (Yang et al., 2021). Sulforaphane (SFN) and Dimethyl fumarate (DMF) activate nuclear factor erythroid 2-related factor 2 (Nrf2) and inhibit NF-κB, thereby inhibiting NLRP3 inflammasomes formation and subsequent microglial cell pyroptosis in mice (Tastan et al., 2021; Tufekci et al., 2021). In palmitic acid-treated BV2 cells, miR-124 inhibited microglia pro-inflammatory responses by suppressing the TLR4/myeloid differentiation factor 88 (MyD88)/NF-κB signaling pathway (Yang et al., 2022). Microglia pyroptosis plays a crucial role in secondary injury of SCI (Xu et al., 2020). Celastrol inhibits microglia activation and NF-κB/p-p65 expression in vivo and in vitro, and attenuates the inflammatory response in SCI induction (Dai et al., 2019).
Given the important role of caspases and GSDM family-related proteins in cell pyroptosis, numerous drug trials targeting them have been initiated. AC-YVAD-CMK, a selective inhibitor of caspase-1, was found to inhibit microglia pyroptosis and induce an anti-inflammatory phenotype in microglia, thereby improving ICH mice (Lin et al., 2018). The caspase-1 inhibitor VX765 has been found to reduce neurological damage after TBI by inhibiting pyroptosis and the high-mobility cassette-1/TLR4/ NF-κB pathway activity, resulting in a better therapeutic effect on TBI (Sun et al., 2020). Clinical doses of sevoflurane exacerbated AD progression via the NLRP3/caspase-1/GSDMD axis. VX-765 significantly inhibited the activation of microglia pyroptosis-related pathways and attenuated sevoflurane-induced release of IL-1, IL-18 and tau-related kinases and phosphatases (Xu et al., 2019b; Tian et al., 2021a). Paeoniflorin (PF) exerted antidepressant effects by inhibiting caspase-11-dependent pyroptosis signaling induced by hyperactivation of hippocampal microglia in ricin-treated mice, and attenuated neuroinflammatory responses (Tian et al., 2021b). Under neuroinflammatory conditions, caspase-3/7 activation promotes GSDMD-associated microglia pyroptosis, and inhibition of GSDMD by siRNA transduction inhibits microglia pyroptosis, providing a new therapeutic opportunity for neuroinflammatory diseases such as MS (McKenzie et al., 2018, 2020b).
Mafenide (MAF) inhibits GSDMD cleavage through direct binding to the GSDMD-Asp275 site, downregulates p30-GSDMD expression, and suppresses bone marrow-derived macrophages (iBMDM) and BV2 microglia pyroptosis (Han et al., 2020b). In contrast, Sulfa-4 and Sulfa-22 target GSDMD cleavage, inhibit pyroptosis and inflammatory factor release, and have a therapeutic effect on neuroinflammation in AD (Esmaeili-Mahani et al., 2021). MiRNA-22 was negatively correlated with the expression of inflammatory factors in AD patients (Han et al., 2020a). Adipose-derived mesenchymal stem cells miRNA-22 loaded exosomes (Exo-miRNA-22) inhibited microglia pyroptosis and decreased inflammatory factor release by targeting GSDMD, and improved neurological function in AD mice (Zhai et al., 2021). GSDMD-mediated microglia pyroptosis is involved in kainic acid-induced seizures, and DMF, as an inhibitor of GSDMD N-terminal fragments (GSDMD-N), significantly reduce microglia pyroptosis and the expression of inflammatory factors such as IL-1 and IL-18, and play a certain role in the treatment of epilepsy (Xia et al., 2021). In SCI, the immunosuppressive molecule CD73 attenuates GSDMD-mediated microglia pyroptosis by promoting the phosphatidylinositol 3-kinase (PI3K)/AKT/Foxo1 signaling pathway (Xu et al., 2021b). Table 1 graphically covers the mechanisms in which the various drugs mentioned above modulate neurological disorders by targeting microglia pyroptosis.
Table 1. Regulation of microglia pyroptosis by various reagents for treatment of neurological diseases.
Microglia pyroptosis is now a common cause of secondary neuronal injury. However, direct studies on microglia pyroptosis are still lacking. And there are still many questions related to pyroptosis that remain to be addressed. For example, it is true that some bacteria are thought to have evolved mechanisms to resist pyroptosis and thus evade immunity, such as Shigella. However, it is still widely believed that pathogen-associated PAMP and DAMP activate the pyroptosis pathway by activating GSDMD-associated proteins in the downstream pathway. The pyroptosis pathway has been more extensively studied and more activation pathways have been identified. In addition to the classical caspase-1-mediated pyroptosis pathway, the LPS-mediated caspase-4/5/11 non-classical pyroptosis pathway, and pyroptosis via apoptotic transformation.
Currently, inhibition of microglia pyroptosis has been affirmed in various neurological disease models for its associated therapeutic effects. Dexmedetomidine, Andrographolide, Curcumin and Salvianolic acids for injection have shown their relevant therapeutic effects by targeting NLRP3, caspases and GSDMs. This provides new ideas for immunomodulatory therapy of the CNS. However, there are still some questions about the place of NLRP3 in pyroptosis in specific neurological diseases. For example, in a mouse model of OGD studying ischemic stroke, elevated NLRC4 is thought to contribute to microglia pyroptosis without a significant association with NLRP3. Significantly elevated levels of NLRP3 expression in other cells of the striatum of HD mice, but no significant NLRP3 activation was found in microglia. In a CMS-induced depression model in mice, astrocytes in the hippocampus showed cellular pyroptosis, while microglia did not show significant pyroptosis. In contrast, in PD, microglia pyroptosis may be related to α-synuclein concentration. Therefore, the initiation mechanism of microglia pyroptosis in different diseases and its differences in spatial and temporal aspects need to be more elucidated.
XW and TW designed this article. TW, XG, and MF wrote the manuscript and prepared the figures. TW, XS, and YD critically revised the manuscript for important intellectual content. All authors read and approved the final manuscript and agreed to be accountable for all aspects of this work.
The authors declare that the research was conducted in the absence of any commercial or financial relationships that could be construed as a potential conflict of interest.
All claims expressed in this article are solely those of the authors and do not necessarily represent those of their affiliated organizations, or those of the publisher, the editors and the reviewers. Any product that may be evaluated in this article, or claim that may be made by its manufacturer, is not guaranteed or endorsed by the publisher.
We thank Gang Fan for his suggestions on the design and revision of this article.
CNS, central nervous system; NLRP3, NOD-like receptor thermal protein domain associated protein 3; GSDMs, caspase-1 and gasdermins; PAMPs, pathogen-associated molecular patterns; DAMPs, damage-associated molecular patterns; TBI, traumatic brain injury; BBB, blood-brain barrier; LPS, lipopolysaccharide; IFN-γ, interferon-γ; Aβ, amyloid-beta peptides; IL-1β, interleukin-1β; AOPPs, advanced oxidation protein products; SCI, spinal cord injury; ICH, intracerebral hemorrhage; MLKL, mixed lineage kinase domain-like proteins; PRRs, pattern recognition receptors; TLRs, toll-like receptor; AIM2, absent in melanoma 2; MS, multiple sclerosis; PDE8A, phosphodiesterase 8A; cAMP, cyclic adenosine monophosphate; ADP, adenosine diphosphate; MCAO, middle cerebral artery occlusion; NOX4, NADPH oxidase 4; GPX4, glutathione peroxidase 4; AD, Alzheimer's disease; CARD/ASC, caspase activation and recruitment domain; ACEI, angiotensin-converting enzyme I; PD, Parkinson's disease; 6-OHDA, 6-hydroxydopamine; MPTP, 1-Methyl-4-phenyl-1, 2, 3, 6-tetrahydropyridine; HD, Huntington's disease; HTT, Huntington's protein; MAPK, mitogen-activated protein kinase; HIF-1α, hypoxia inducible factor-1α; ROS, reactive oxygen species; OGD/R, oxygen-glucose deprivation and reoxygenation; OM-MSCs, olfactory mucosa mesenchymal stem cells; EAE, experimental autoimmune encephalomyelitis; CUMS, chronic unpredictable mild stress; Nrf2, nuclear factor-erythroid 2 -related factor 2; DLB, depressive-like behavior; CSDS, chronic social defeat stress; TREM, triggering receptor expressed on myeloid cells; CCR5, C-C chemokine receptor 5; SAH, subarachnoid hemorrhage; PKA, protein kinase A; CREB, cAMP response element binding; Dex, Dexmedetomidine; P2X7R, purinergic 2X7 receptor; SAFI, salvianolic acids for injection; BIRI, brain ischemia reperfusion injury; CCH, chronic cerebral hypoperfusion; DM, diabetes mellitus; DDX3X, DEAD-box helicase 3 X-linked; SITR1, sirtuin1, CaMKII, Ca2+/calcium/calmodulin-dependent protein kinase II; APP, amyloid precursor protein; PS1, presenilin-1; NF-?B, nuclear factor-kappa B; PAS-NA, Para-aminosalicylic acid; HO-1, heme oxygenase-1; DI, dimethyl itaconate; SFN, sulforaphane; MyD88, myeloid differentiation factor 88; PF, Paeoniflorin; iBMDM, immortalized bone marrow-derived macrophages; MAF, Mafenide; PI3K, phosphatidylinositol 3-kinase.
Ajami, B., Bennett, J. L., Krieger, C., McNagny, K. M., and Rossi, F. M. (2011). Infiltrating monocytes trigger EAE progression, but do not contribute to the resident microglia pool. Nat. Neurosci. 14, 1142–1149. doi: 10.1038/nn.2887
Arioz, B. I., Tastan, B., Tarakcioglu, E., Tufekci, K. U., Olcum, M., Ersoy, N., et al. (2019). Melatonin attenuates LPS-induced acute depressive-like behaviors and microglial NLRP3 inflammasome activation through the SIRT1/Nrf2 pathway. Front. Immunol. 10, 1511. doi: 10.3389/fimmu.2019.01511
Bergsbaken, T., Fink, S. L., and Cookson, B. T. (2009). Pyroptosis: host cell death and inflammation. Nat. Rev. Microbiol. 7, 99–109. doi: 10.1038/nrmicro2070
Bian, Y., Li, X., Pang, P., Hu, X. L., Yu, S. T., Liu, Y. N., et al. (2020). Kanglexin, a novel anthraquinone compound, protects against myocardial ischemic injury in mice by suppressing NLRP3 and pyroptosis. Acta. Pharmacol. Sinica. 41, 319–326. doi: 10.1038/s41401-019-0307-8
Bjorkqvist, M., Wild, E. J., Thiele, J., Silvestroni, A., Andre, R., Lahiri, N., et al. (2008). A novel pathogenic pathway of immune activation detectable before clinical onset in Huntington's disease. J. Exp. Med. 205, 1869–1877. doi: 10.1084/jem.20080178
Black, R. A., Kronheim, S. R., Merriam, J. E., March, C. J., and Hopp, T. P. (1989). A. pre-aspartate-specific protease from human leukocytes that cleaves pro-interleukin-1 beta. J. Biol. Chem. 264, 5323–6. doi: 10.1016/S0021-9258(18)83546-3
Burm, S. M., Peferoen, L. A., Zuiderwijk-Sick, E. A., Haanstra, K. G. T, Hart, B. A., van der Valk, P., et al. (2016). Expression of IL-1beta in rhesus EAE and MS lesions is mainly induced in the CNS itself. J. Neuroinflam. 13, 138. doi: 10.1186/s12974-016-0605-8
Cai, M., Zhuang, W., Lv, E., Liu, Z., Wang, Y., Zhang, W., et al. (2022). Kaemperfol alleviates pyroptosis and microglia-mediated neuroinflammation in Parkinson's disease via inhibiting p38MAPK/NF-κB signaling pathway. Neurochem. Int. 152, 105221. doi: 10.1016/j.neuint.2021.105221
Cao, X., Wang, Y., and Gao, L. (2021). CHRFAM7A overexpression attenuates cerebral ischemia-reperfusion injury via inhibiting microglia pyroptosis mediated by the NLRP3/Caspase-1 pathway. Inflammation. 44, 1023–1034. doi: 10.1007/s10753-020-01398-4
Catanese, S., Aringhieri, G., Vivaldi, C., Salani, F., Vitali, S., Pecora, I., et al. (2021). Role of baseline computed-tomography-evaluated body composition in predicting outcome and toxicity from first-line therapy in advanced gastric cancer patients. J. Clin. Med. 10, 1079. doi: 10.3390/jcm10051079
Ceulemans, A. G., Zgavc, T., Kooijman, R., Hachimi-Idrissi, S., Sarre, S., Michotte, Y., et al. (2010). The dual role of the neuroinflammatory response after ischemic stroke: modulatory effects of hypothermia. J. Neuroinflam. 7, 74. doi: 10.1186/1742-2094-7-74
Chang, Y., Zhu, J., Wang, D., Li, H., He, Y., Liu, K., et al. (2020). NLRP3 inflammasome-mediated microglial pyroptosis is critically involved in the development of post-cardiac arrest brain injury. J. Neuroinflam. 17, 219. doi: 10.1186/s12974-020-01879-1
Colonna, M., and Butovsky, O. (2017). Microglia function in the central nervous system during health and neurodegeneration. Ann. Rev. Immunol. 35, 441–468. doi: 10.1146/annurev-immunol-051116-052358
Coppin, L., Sokal, E., and Stephenne, X. (2019). Thrombogenic risk induced by intravascular mesenchymal stem cell therapy: current status and future perspectives. Cells. 8, 1160. doi: 10.3390/cells8101160
Dabrowska, S., Andrzejewska, A., Lukomska, B., and Janowski, M. (2019). Neuroinflammation as a target for treatment of stroke using mesenchymal stem cells and extracellular vesicles. J. Neuroinflam. 16, 178. doi: 10.1186/s12974-019-1571-8
Dai, W., Wang, X., Teng, H., Li, C., Wang, B., Wang, J., et al. (2019). Celastrol inhibits microglial pyroptosis and attenuates inflammatory reaction in acute spinal cord injury rats. Int. Immunopharmacol. 66, 215–223. doi: 10.1016/j.intimp.2018.11.029
de Alba, E. (2019). Structure, interactions and self-assembly of ASC-dependent inflammasomes. Arch. Biochem. Biophys. 670, 15–31. doi: 10.1016/j.abb.2019.05.023
Dempsey, C., Rubio Araiz, A., Bryson, K. J., Finucane, O., Larkin, C., Mills, E. L., et al. (2017). Inhibiting the NLRP3 inflammasome with MCC950 promotes non-phlogistic clearance of amyloid-β and cognitive function in APP/PS1 mice. Brain Behav. Immun. 61, 306–316. doi: 10.1016/j.bbi.2016.12.014
Dendrou, C. A., Fugger, L., and Friese, M. A. (2015). Immunopathology of multiple sclerosis. Nat. Rev. Immunol. 15, 545–558. doi: 10.1038/nri3871
Deng, W., Bai, Y., Deng, F., Pan, Y., Mei, S., Zheng, Z., et al. (2022). Streptococcal pyrogenic exotoxin B cleaves GSDMA and triggers pyroptosis. Nature. 602, 496–502. doi: 10.1038/s41586-021-04384-4
Dey, A., and Hankey Giblin, P. A. (2018). Insights into macrophage heterogeneity and cytokine-induced neuroinflammation in major depressive disorder. Pharmaceuticals. 11, 64. doi: 10.3390/ph11030064
Ding, J., Wang, K., Liu, W., She, Y., Sun, Q., Shi, J., et al. (2016). Pore-forming activity and structural autoinhibition of the gasdermin family. Nature. 535, 111–116. doi: 10.1038/nature18590
Eggen, B. J., Raj, D., Hanisch, U. K., and Boddeke, H. W. (2013). Microglial phenotype and adaptation. J. Neuroimmune Pharmacol. 8, 807–823. doi: 10.1007/s11481-013-9490-4
Esmaeili-Mahani, S., Haghparast, E., Nezhadi, A., Abbasnejad, M., and Sheibani, V. (2021). Apelin-13 prevents hippocampal synaptic plasticity impairment in Parkinsonism rats. J. Chem. Neuroanat. 111, 101884. doi: 10.1016/j.jchemneu.2020.101884
Fan, Y., Du, L., Fu, Q., Zhou, Z., Zhang, J., Li, G., et al. (2018). Inhibiting the NLRP3 inflammasome with MCC950 ameliorates isoflurane-induced pyroptosis and cognitive impairment in aged mice. Front. Cell. Neurosci. 12, 426. doi: 10.3389/fncel.2018.00426
Fang, Y., Tian, S., Pan, Y., Li, W., Wang, Q., Tang, Y., et al. (2020). Pyroptosis: a new frontier in cancer. Biomed. Pharmacother. 121, 109595. doi: 10.1016/j.biopha.2019.109595
Fann, D. Y., Lee, S. Y., Manzanero, S., Tang, S. C., Gelderblom, M., Chunduri, P., et al. (2013). Intravenous immunoglobulin suppresses NLRP1 and NLRP3 inflammasome-mediated neuronal death in ischemic stroke. Cell Death Dis. 4, e790. doi: 10.1038/cddis.2013.326
Fann, D. Y., Lim, Y. A., Cheng, Y. L., Lok, K. Z., Chunduri, P., Baik, S. H., et al. (2018). Evidence that NF-kappaB and MAPK signaling promotes NLRP inflammasome activation in neurons following ischemic stroke. Mol. Neurobiol. 55, 1082–1096. doi: 10.1007/s12035-017-0394-9
Frank, D., and Vince, J. E. (2019). Pyroptosis versus necroptosis: similarities, differences, and crosstalk. Cell Death Diff. 26, 99–114. doi: 10.1038/s41418-018-0212-6
Friker, L. L., Scheiblich, H., Hochheiser, I. V., Brinkschulte, R., Riedel, D., Latz, E., et al. (2020). Beta-amyloid clustering around ASC fibrils boosts its toxicity in microglia. Cell Rep. 30, 3743–54.e6. doi: 10.1016/j.celrep.2020.02.025
Gao, J., Peng, S., Shan, X., Deng, G., Shen, L., Sun, J., et al. (2019). Inhibition of AIM2 inflammasome-mediated pyroptosis by Andrographolide contributes to amelioration of radiation-induced lung inflammation and fibrosis. Cell Death Dis. 10, 957. doi: 10.1038/s41419-019-2195-8
Gordon, R., Albornoz, E. A., Christie, D. C., Langley, M. R., Kumar, V., Mantovani, S., et al. (2018). Inflammasome inhibition prevents alpha-synuclein pathology and dopaminergic neurodegeneration in mice. Sci. Transl. Med. 10, eaah4066. doi: 10.1126/scitranslmed.aah4066
Graeser, M., Thieben, F., Szwargulski, P., Werner, F., Gdaniec, N., Boberg, M., et al. (2019). Human-sized magnetic particle imaging for brain applications. Nat. Commun. 10, 1936. doi: 10.1038/s41467-019-09704-x
Gu, C., Draga, D., Zhou, C., Su, T., Zou, C., Gu, Q., et al. (2019). miR-590-3p inhibits pyroptosis in diabetic retinopathy by targeting NLRP1 and inactivating the NOX4 signaling pathway. Investig. Ophthalmol. Visual Sci. 60, 4215–4223. doi: 10.1167/iovs.19-27825
Gu, L., Sun, M., Li, R., Zhang, X., Tao, Y., Yuan, Y., et al. (2022). Didymin suppresses microglia pyroptosis and neuroinflammation through the Asc/Caspase-1/GSDMD pathway following experimental intracerebral hemorrhage. Front. Immunol. 13, 810582. doi: 10.3389/fimmu.2022.810582
Guo, H., Callaway, J. B., and Ting, J. P. (2015). Inflammasomes: mechanism of action, role in disease, and therapeutics. Nat. Med. 21, 677–687. doi: 10.1038/nm.3893
Han, C., Guo, L., Yang, Y., Guan, Q., Shen, H., Sheng, Y., et al. (2020a). Mechanism of microRNA-22 in regulating neuroinflammation in Alzheimer's disease. Brain Behav. 10, e01627. doi: 10.1002/brb3.1627
Han, C., Yang, Y., Yu, A., Guo, L., Guan, Q., Shen, H., et al. (2020b). Investigation on the mechanism of mafenide in inhibiting pyroptosis and the release of inflammatory factors. Eur. J. Pharm. Sci. 147, 105303. doi: 10.1016/j.ejps.2020.105303
Han, X., Xu, T., Fang, Q., Zhang, H., Yue, L., Hu, G., et al. (2021). Quercetin hinders microglial activation to alleviate neurotoxicity via the interplay between NLRP3 inflammasome and mitophagy. Redox Biol. 44, 102010. doi: 10.1016/j.redox.2021.102010
Hao, W., Hao, C., Wu, C., Xu, Y., Wu, S., Lu, X., et al. (2021). Aluminum impairs cognitive function by activating DDX3X-NLRP3-mediated pyroptosis signaling pathway. Food Chem. Toxicol. 157, 112591. doi: 10.1016/j.fct.2021.112591
Heneka, M. T., McManus, R. M., and Latz, E. (2018). Inflammasome signalling in brain function and neurodegenerative disease. Nat. Rev. Neurosci. 19, 610–621. doi: 10.1038/s41583-018-0055-7
Hou, C., Jiang, F., Ma, H., Zhu, Q., Wang, Z., Zhao, B., et al. (2019). Prognostic role of preoperative platelet, fibrinogen, and D-dimer levels in patients with non-small cell lung cancer: a multicenter prospective study. Thoracic Cancer. 10, 304–311. doi: 10.1111/1759-7714.12956
Hu, J., Zeng, C., Wei, J., Duan, F., Liu, S., Zhao, Y., et al. (2020). The combination of Panax ginseng and Angelica sinensis alleviates ischemia brain injury by suppressing NLRP3 inflammasome activation and microglial pyroptosis. Phytomed. Int. J. Phytother. Phytopharmacol. 76, 153251. doi: 10.1016/j.phymed.2020.153251
Hu, Q., Hong, M., Huang, M., Gong, Q., Zhang, X., Uversky, V. N., et al. (2022). Age-dependent aggregation of alpha-synuclein in the nervous system of gut-brain axis is associated with caspase-1 activation. Metab. Brain Dis. 37, 1669–1681. doi: 10.1007/s11011-022-00917-6
Hu, X., Leak, R. K., Shi, Y., Suenaga, J., Gao, Y., Zheng, P., et al. (2015). Microglial and macrophage polarization—new prospects for brain repair. Nature Rev. Neurol. 11, 56–64. doi: 10.1038/nrneurol.2014.207
Huang, W. X., Huang, P., and Hillert, J. (2004). Increased expression of caspase-1 and interleukin-18 in peripheral blood mononuclear cells in patients with multiple sclerosis. Mult. Scler. 10, 482–487. doi: 10.1191/1352458504ms1071oa
Huang, Y., Tan, F., Zhuo, Y., Liu, J., He, J., Duan, D., et al. (2020). Hypoxia-preconditioned olfactory mucosa mesenchymal stem cells abolish cerebral ischemia/reperfusion-induced pyroptosis and apoptotic death of microglial cells by activating HIF-1alpha. Aging. 12, 10931–10950. doi: 10.18632/aging.103307
Iadecola, C., and Anrather, J. (2011). The immunology of stroke: from mechanisms to translation. Nat. Med. 17, 796–808. doi: 10.1038/nm.2399
Imai, H., and Nakagawa, Y. (2003). Biological significance of phospholipid hydroperoxide glutathione peroxidase (PHGPx, GPx4) in mammalian cells. Free Rad. Biol. Med. 34, 145–169. doi: 10.1016/S0891-5849(02)01197-8
Jiang, Q., Geng, X., Warren, J., Eugene Paul Cosky, E., Kaura, S., Stone, C., et al. (2020). Hypoxia inducible factor-1alpha (HIF-1alpha) mediates NLRP3 inflammasome-dependent-pyroptotic and apoptotic cell death following ischemic stroke. Neuroscience. 448, 126–139. doi: 10.1016/j.neuroscience.2020.09.036
Jorgensen, I., and Miao, E. A. (2015). Pyroptotic cell death defends against intracellular pathogens. Immunol. Rev. 265, 130–142. doi: 10.1111/imr.12287
Kang, R., Zeng, L., Zhu, S., Xie, Y., Liu, J., Wen, Q., et al. (2018). Lipid peroxidation drives gasdermin D-mediated pyroptosis in lethal polymicrobial sepsis. Cell Host Microbe. 24, 97–108.e4. doi: 10.1016/j.chom.2018.05.009
Kanneganti, T. D., Lamkanfi, M., and Núñez, G. (2007). Intracellular NOD-like receptors in host defense and disease. Immunity. 27, 549–559. doi: 10.1016/j.immuni.2007.10.002
Kim, Y. J., Yi, Y., Sapp, E., Wang, Y., Cuiffo, B., Kegel, K. B., et al. (2001). Caspase 3-cleaved N-terminal fragments of wild-type and mutant huntingtin are present in normal and Huntington's disease brains, associate with membranes, and undergo calpain-dependent proteolysis. Proc. Natl. Acad. Sci. U. S. A. 98, 12784–12789. doi: 10.1073/pnas.221451398
Kipp, M., Nyamoya, S., Hochstrasser, T., and Amor, S. (2017). Multiple sclerosis animal models: a clinical and histopathological perspective. Brain Pathol. 27, 123–137. doi: 10.1111/bpa.12454
Klotz, K., Weistenhöfer, W., Neff, F., Hartwig, A., van Thriel, C., Drexler, H., et al. (2017). The health effects of aluminum exposure. Deutsches Arzteblatt Int. 114, 653–659. doi: 10.3238/arztebl.2017.0653
Kornek, B., and Lassmann, H. (2003). Neuropathology of multiple sclerosis-new concepts. Brain Res. Bull. 61, 321–326. doi: 10.1016/S0361-9230(03)00095-9
Kovacs, S. B., and Miao, E. A. (2017). Gasdermins: effectors of pyroptosis. Trends Cell Biol. 27, 673–684. doi: 10.1016/j.tcb.2017.05.005
Kriz, J. (2006). Inflammation in ischemic brain injury: timing is important. Crit. Rev. Neurobiol. 18, 145–157. doi: 10.1615/CritRevNeurobiol.v18.i1-2.150
Langhauser, F., Gob, E., Kraft, P., Geis, C., Schmitt, J., Brede, M., et al. (2012). Kininogen deficiency protects from ischemic neurodegeneration in mice by reducing thrombosis, blood-brain barrier damage, and inflammation. Blood. 120, 4082–4092. doi: 10.1182/blood-2012-06-440057
Leyns, C. E. G., and Holtzman, D. M. (2017). Glial contributions to neurodegeneration in tauopathies. Mol. Neurodegener. 12, 50. doi: 10.1186/s13024-017-0192-x
Li, Q., and Barres, B. A. (2018). Microglia and macrophages in brain homeostasis and disease. Nat. Rev. Immunol. 18, 225–242. doi: 10.1038/nri.2017.125
Li, X., Wang, T., Zhang, D., Li, H., Shen, H., Ding, X., et al. (2018). Andrographolide ameliorates intracerebral hemorrhage induced secondary brain injury by inhibiting neuroinflammation induction. Neuropharmacology. 141, 305–315. doi: 10.1016/j.neuropharm.2018.09.015
Li, Y., Song, W., Tong, Y., Zhang, X., Zhao, J., Gao, X., et al. (2021a). Isoliquiritin ameliorates depression by suppressing NLRP3-mediated pyroptosis via miRNA-27a/SYK/NF-κB axis. J. Neuroinflam. 18, 1. doi: 10.1186/s12974-020-02040-8
Li, Z., Liu, W., Fu, J., Cheng, S., Xu, Y., Wang, Z., et al. (2021b). Shigella evades pyroptosis by arginine ADP-riboxanation of caspase-11. Nature. 599, 290–295. doi: 10.1038/s41586-021-04020-1
Liang, J., Wang, Q., Li, J. Q., Guo, T., and Yu, D. (2020a). Long non-coding RNA MEG3 promotes cerebral ischemia-reperfusion injury through increasing pyroptosis by targeting miR-485/AIM2 axis. Exp. Neurol. 325, 113139. doi: 10.1016/j.expneurol.2019.113139
Liang, Y. B., Song, P. P., Zhu, Y. H., Xu, J. M., Zhu, P. Z., Liu, R. R., et al. (2020b). TREM-1-targeting LP17 attenuates cerebral ischemia-induced neuronal injury by inhibiting oxidative stress and pyroptosis. Biochem. Biophys. Res. Commun. 529, 554–561. doi: 10.1016/j.bbrc.2020.05.056
Lin, X., Ye, H., Siaw-Debrah, F., Pan, S., He, Z., Ni, H., et al. (2018). AC-YVAD-CMK inhibits pyroptosis and improves functional outcome after intracerebral hemorrhage. Biomed. Res. Int. 2018, 3706047. doi: 10.1155/2018/3706047
Liu, J., Ma, W., Zang, C. H., Wang, G. D., Zhang, S. J., Wu, H. J., et al. (2021). Salidroside inhibits NLRP3 inflammasome activation and apoptosis in microglia induced by cerebral ischemia/reperfusion injury by inhibiting the TLR4/NF-kappaB signaling pathway. Ann. Transl. Med. 9, 1694. doi: 10.21037/atm-21-5752
Liu, W., Chen, Y., Meng, J., Wu, M., Bi, F., Chang, C., et al. (2018). Ablation of caspase-1 protects against TBI-induced pyroptosis in vitro and in vivo. J. Neuroinflam. 15, 48. doi: 10.1186/s12974-018-1083-y
Liu, Z., Yao, X., Jiang, W., Li, W., Zhu, S., Liao, C., et al. (2020). Advanced oxidation protein products induce microglia-mediated neuroinflammation via MAPKs-NF-κB signaling pathway and pyroptosis after secondary spinal cord injury. J Neuroinflam. 17, 90. doi: 10.1186/s12974-020-01751-2
Lloyd, A. F., and Miron, V. E. (2019). The pro-remyelination properties of microglia in the central nervous system. Nat. Rev. Neurol. 15, 447–458. doi: 10.1038/s41582-019-0184-2
Loveless, R., Bloomquist, R., and Teng, Y. (2021). Pyroptosis at the forefront of anticancer immunity. J. Exp. Clin. Cancer Res. CR. 40, 264. doi: 10.1186/s13046-021-02065-8
Luciunaite, A., McManus, R. M., Jankunec, M., Racz, I., Dansokho, C., Dalgediene, I., et al. (2020). Soluble Abeta oligomers and protofibrils induce NLRP3 inflammasome activation in microglia. J. Neurochem. 155, 650–661. doi: 10.1111/jnc.14945
Ma, D. C., Zhang, N. N., Zhang, Y. N., and Chen, H. S. (2021). Salvianolic Acids for Injection alleviates cerebral ischemia/reperfusion injury by switching M1/M2 phenotypes and inhibiting NLRP3 inflammasome/pyroptosis axis in microglia in vivo and in vitro. J. Ethnopharmacol. 270, 113776. doi: 10.1016/j.jep.2021.113776
Ma, Q., Chen, S., Hu, Q., Feng, H., Zhang, J. H., Tang, J., et al. (2014). NLRP3 inflammasome contributes to inflammation after intracerebral hemorrhage. Ann. Neurol. 75, 209–219. doi: 10.1002/ana.24070
Malhi, G. S., and Mann, J. J. (2018). Depression. Lancet. 392, 2299–2312. doi: 10.1016/S0140-6736(18)31948-2
Man, S. M., Karki, R., and Kanneganti, T. D. (2017). Molecular mechanisms and functions of pyroptosis, inflammatory caspases and inflammasomes in infectious diseases. Immunol. Rev. 277, 61–75. doi: 10.1111/imr.12534
Mao, Z., Liu, C., Ji, S., Yang, Q., Ye, H., Han, H., et al. (2017). The NLRP3 inflammasome is involved in the pathogenesis of Parkinson's disease in rats. Neurochem. Res. 42, 1104–1115. doi: 10.1007/s11064-017-2185-0
McKenzie, B. A., Dixit, V. M., and Power, C. (2020a). Fiery cell death: pyroptosis in the central nervous system. Trends Neurosci. 43, 55–73. doi: 10.1016/j.tins.2019.11.005
McKenzie, B. A., Fernandes, J. P., Doan, M. A. L., Schmitt, L. M., Branton, W. G., Power, C., et al. (2020b). Activation of the executioner caspases-3 and−7 promotes microglial pyroptosis in models of multiple sclerosis. J. Neuroinflam. 17, 253. doi: 10.1186/s12974-020-01902-5
McKenzie, B. A., Mamik, M. K., Saito, L. B., Boghozian, R., Monaco, M. C., Major, E. O., et al. (2018). Caspase-1 inhibition prevents glial inflammasome activation and pyroptosis in models of multiple sclerosis. Proc. Natl. Acad. Sci. U. S. A. 115, E6065–e74. doi: 10.1073/pnas.1722041115
Nabavi, S. F., Sureda, A., Habtemariam, S., and Nabavi, S. M. (2015). Ginsenoside Rd and ischemic stroke; a short review of literatures. J. Ginseng. Res. 39, 299–303. doi: 10.1016/j.jgr.2015.02.002
Nestle, U., Adebahr, S., Kaier, K., Gkika, E., Schimek-Jasch, T., Hechtner, M., et al. (2020). Quality of life after pulmonary stereotactic fractionated radiotherapy (SBRT): Results of the phase II STRIPE trial. Radiother. Oncol. J. Eur. Soc. Therap. Radiol. Oncol. 148, 82–88. doi: 10.1016/j.radonc.2020.03.018
Newcombe, E. A., Camats-Perna, J., Silva, M. L., Valmas, N., Huat, T. J., Medeiros, R., et al. (2018). Inflammation: the link between comorbidities, genetics, and Alzheimer's disease. J. Neuroinflam. 15, 276. doi: 10.1186/s12974-018-1313-3
Ona, V. O., Li, M., Vonsattel, J. P., Andrews, L. J., Khan, S. Q., Chung, W. M., et al. (1999). Inhibition of caspase-1 slows disease progression in a mouse model of Huntington's disease. Nature. 399, 263–267. doi: 10.1038/20446
Orihuela, R., McPherson, C. A., and Harry, G. J. (2016). Microglial M1/M2 polarization and metabolic states. Br. J. Pharmacol. 173, 649–665. doi: 10.1111/bph.13139
Paldino, E., D'Angelo, V., Sancesario, G., and Fusco, F. R. (2020). Pyroptotic cell death in the R6/2 mouse model of Huntington's disease: new insight on the inflammasome. Cell Death Discov. 6, 69. doi: 10.1038/s41420-020-00293-z
Palpagama, T. H., Waldvogel, H. J., Faull, R. L. M., and Kwakowsky, A. (2019). The role of microglia and astrocytes in Huntington's disease. Front. Mol. Neurosci. 12, 258. doi: 10.3389/fnmol.2019.00258
Pan, Y., Chen, X. Y., Zhang, Q. Y., and Kong, L. D. (2014). Microglial NLRP3 inflammasome activation mediates IL-1beta-related inflammation in prefrontal cortex of depressive rats. Brain Behav. Immun. 41, 90–100. doi: 10.1016/j.bbi.2014.04.007
Peng, D., Li, J., Deng, Y., Zhu, X., Zhao, L., Zhang, Y., et al. (2020). Sodium para-aminosalicylic acid inhibits manganese-induced NLRP3 inflammasome-dependent pyroptosis by inhibiting NF-κB pathway activation and oxidative stress. J. Neuroinflam. 17, 343. doi: 10.1186/s12974-020-02018-6
Poh, L., Kang, S. W., Baik, S. H., Ng, G. Y. Q., She, D. T., Balaganapathy, P., et al. (2019). Evidence that NLRC4 inflammasome mediates apoptotic and pyroptotic microglial death following ischemic stroke. Brain Behav. Immun. 75, 34–47. doi: 10.1016/j.bbi.2018.09.001
Prinz, M., Masuda, T., Wheeler, M. A., and Quintana, F. J. (2021). Microglia and central nervous system-associated macrophages-from origin to disease modulation. Ann. Rev. Immunol. 39, 251–277. doi: 10.1146/annurev-immunol-093019-110159
Przedborski, S. (2017). The two-century journey of Parkinson disease research. Nat. Rev. Neurosci. 18, 251–259. doi: 10.1038/nrn.2017.25
Qiu, X., Cheng, X., Zhang, J., Yuan, C., Zhao, M., Yang, X., et al. (2020). Ethyl pyruvate confers protection against endotoxemia and sepsis by inhibiting caspase-11-dependent cell pyroptosis. Int. Immunopharmacol. 78, 106016. doi: 10.1016/j.intimp.2019.106016
Raedler, T. J. (2011). Inflammatory mechanisms in major depressive disorder. Curr. Opin. Psychiatry. 24, 519–525. doi: 10.1097/YCO.0b013e32834b9db6
Ran, Y., Su, W., Gao, F., Ding, Z., Yang, S., Ye, L., et al. (2021). Curcumin ameliorates white matter injury after ischemic stroke by inhibiting microglia/macrophage pyroptosis through NF-kappaB suppression and NLRP3 inflammasome inhibition. Oxid. Med. Cell Longev. 2021, 1552127. doi: 10.1155/2021/1552127
Ransohoff, R. M. (2012). Animal models of multiple sclerosis: the good, the bad and the bottom line. Nat. Neurosci. 15, 1074–1077. doi: 10.1038/nn.3168
Ransohoff, R. M. (2016a). A polarizing question: do M1 and M2 microglia exist? Nat. Neurosci. 19, 987–991. doi: 10.1038/nn.4338
Ransohoff, R. M. (2016b). How neuroinflammation contributes to neurodegeneration. Science. 353, 777–783. doi: 10.1126/science.aag2590
Rogers, C., Fernandes-Alnemri, T., Mayes, L., Alnemri, D., Cingolani, G., Alnemri, E. S., et al. (2017). Cleavage of DFNA5 by caspase-3 during apoptosis mediates progression to secondary necrotic/pyroptotic cell death. Nat. Commun. 8, 14128. doi: 10.1038/ncomms14128
Ronaldson, P. T., and Davis, T. P. (2020). Regulation of blood-brain barrier integrity by microglia in health and disease: a therapeutic opportunity. J. Cerebral Blood Flow Metabol. 40:S6–s24. doi: 10.1177/0271678X20951995
Rui, W., Li, S., Xiao, H., Xiao, M., and Shi, J. (2020). Baicalein attenuates neuroinflammation by inhibiting NLRP3/caspase-1/GSDMD pathway in MPTP induced mice model of Parkinson's disease. Int. J. Neuropsychopharmacol. 23, 762–773. doi: 10.1093/ijnp/pyaa060
Sahoo, B. R. (2020). Structure of fish Toll-like receptors (TLR) and NOD-like receptors (NLR). Int. J. Biol. Macromol. 161, 1602–1617. doi: 10.1016/j.ijbiomac.2020.07.293
Sanchez Mejia, R. O., and Friedlander, R. M. (2001). Caspases in Huntington's disease. Neuroscientist. 7, 480–489. doi: 10.1177/107385840100700604
Sarlus, H., and Heneka, M. T. (2017). Microglia in Alzheimer's disease. J. Clin. Invest. 127, 3240–3249. doi: 10.1172/JCI90606
Shi, J., Gao, W., and Shao, F. (2017). Pyroptosis: gasdermin-mediated programmed necrotic cell death. Trends Biochem. Sci. 42, 245–254. doi: 10.1016/j.tibs.2016.10.004
Shi, J., Zhao, Y., Wang, K., Shi, X., Wang, Y., Huang, H., et al. (2015). Cleavage of GSDMD by inflammatory caspases determines pyroptotic cell death. Nature. 526, 660–665. doi: 10.1038/nature15514
Shi, J., Zhao, Y., Wang, Y., Gao, W., Ding, J., Li, P., et al. (2014). Inflammatory caspases are innate immune receptors for intracellular LPS. Nature. 514, 187–192. doi: 10.1038/nature13683
Siew, J. J., Chen, H. M., Chen, H. Y., Chen, H. L., Chen, C. M., Soong, B. W., et al. (2019). Galectin-3 is required for the microglia-mediated brain inflammation in a model of Huntington's disease. Nat. Commun. 10, 3473. doi: 10.1038/s41467-019-11441-0
Song, S., Guo, R., Mehmood, A., Zhang, L., Yin, B., Yuan, C., et al. (2022). Liraglutide attenuate central nervous inflammation and demyelination through AMPK and pyroptosis-related NLRP3 pathway. CNS Neurosci. Ther. 28, 422–434. doi: 10.1111/cns.13791
Song, X., Cui, Z., He, J., Yang, T., and Sun, X. (2021). κ-opioid receptor agonist, U50488H, inhibits pyroptosis through NLRP3 via the Ca(2+)/CaMKII/CREB signaling pathway and improves synaptic plasticity in APP/PS1 mice. Mol. Med. Rep. 24, 12168. doi: 10.3892/mmr.2021.12168
Stancu, I. C., Cremers, N., Vanrusselt, H., Couturier, J., Vanoosthuyse, A., Kessels, S., et al. (2019). Aggregated Tau activates NLRP3-ASC inflammasome exacerbating exogenously seeded and non-exogenously seeded Tau pathology in vivo. Acta Neuropathol. 137, 599–617. doi: 10.1007/s00401-018-01957-y
Su, W. J., Zhang, Y., Chen, Y., Gong, H., Lian, Y. J., Peng, W., et al. (2017). NLRP3 gene knockout blocks NF-kappaB and MAPK signaling pathway in CUMS-induced depression mouse model. Behav. Brain Res. 322, 1–8. doi: 10.1016/j.bbr.2017.01.018
Sun, K., Zhang, J., Yang, Q., Zhu, J., Zhang, X., Wu, K., et al. (2021). Dexmedetomidine exerts a protective effect on ischemic brain injury by inhibiting the P2X7R/NLRP3/Caspase-1 signaling pathway. Brain Res. Bull. 174, 11–21. doi: 10.1016/j.brainresbull.2021.05.006
Sun, Z., Nyanzu, M., Yang, S., Zhu, X., Wang, K., Ru, J., et al. (2020). VX765 attenuates pyroptosis and HMGB1/TLR4/NF-κB pathways to improve functional outcomes in TBI mice. Oxid. Med. Cell Longev. 2020, 7879629. doi: 10.1155/2020/7879629
Taabazuing, C. Y., Okondo, M. C., and Bachovchin, D. A. (2017). Pyroptosis and apoptosis pathways engage in bidirectional crosstalk in monocytes and macrophages. Cell Chem. Biol. 24, 507–14.e4. doi: 10.1016/j.chembiol.2017.03.009
Tao, Z., Wang, J., Wen, K., Yao, R., Da, W., Zhou, S., et al. (2020). Pyroptosis in Osteoblasts: a novel hypothesis underlying the pathogenesis of osteoporosis. Front. Endocrinol. 11, 548812. doi: 10.3389/fendo.2020.548812
Tastan, B., Arioz, B. I., Tufekci, K. U., Tarakcioglu, E., Gonul, C. P., Genc, K., et al. (2021). Dimethyl fumarate alleviates NLRP3 inflammasome activation in microglia and sickness behavior in LPS-challenged mice. Front. Immunol. 12, 737065. doi: 10.3389/fimmu.2021.737065
Tian, D., Xing, Y., Gao, W., Zhang, H., Song, Y., Tian, Y., et al. (2021a). Sevoflurane aggravates the progress of Alzheimer's disease through NLRP3/Caspase-1/Gasdermin D pathway. Front. Cell Dev. Biol. 9, 801422. doi: 10.3389/fcell.2021.801422
Tian, D. D., Wang, M., Liu, A., Gao, M. R., Qiu, C., Yu, W., et al. (2021b). Antidepressant effect of paeoniflorin is through inhibiting pyroptosis CASP-11/GSDMD pathway. Mol. Neurobiol. 58, 761–776. doi: 10.1007/s12035-020-02144-5
Tian, Y., Meng, L., and Zhang, Z. (2020). What is strain in neurodegenerative diseases? Cell Mol. Life Sci. 77, 665–676. doi: 10.1007/s00018-019-03298-9
Tsuchiya, K., Nakajima, S., Hosojima, S., Thi Nguyen, D., Hattori, T., Manh Le, T., et al. (2019). Caspase-1 initiates apoptosis in the absence of gasdermin D. Nat. Commun. 10, 2091. doi: 10.1038/s41467-019-09753-2
Tufekci, K. U., Ercan, I., Isci, K. B., Olcum, M., Tastan, B., Gonul, C. P., et al. (2021). Sulforaphane inhibits NLRP3 inflammasome activation in microglia through Nrf2-mediated miRNA alteration. Immunol. Lett. 233, 20–30. doi: 10.1016/j.imlet.2021.03.004
Van Opdenbosch, N., and Lamkanfi, M. (2019). Caspases in cell death, inflammation, and disease. Immunity. 50, 1352–1364. doi: 10.1016/j.immuni.2019.05.020
Van Zeller, M., Dias, D., Sebastiao, A. M., and Valente, C. A. (2021). NLRP3 Inflammasome: a starring role in amyloid-beta- and tau-driven pathological events in Alzheimer's disease. J. Alzheimers Dis. 83, 939–961. doi: 10.3233/JAD-210268
Vande Walle, L., and Lamkanfi, M. (2016). Pyroptosis. Curr. Biol. 26, R568–R72. doi: 10.1016/j.cub.2016.02.019
Vaquer-Alicea, J., and Diamond, M. I. (2019). Propagation of protein aggregation in neurodegenerative diseases. Annu. Rev. Biochem. 88, 785–810. doi: 10.1146/annurev-biochem-061516-045049
Venegas, C., Kumar, S., Franklin, B. S., Dierkes, T., Brinkschulte, R., Tejera, D., et al. (2017). Microglia-derived ASC specks cross-seed amyloid-beta in Alzheimer's disease. Nature. 552, 355–361. doi: 10.1038/nature25158
Voet, S., Srinivasan, S., Lamkanfi, M., and van Loo, G. (2019). Inflammasomes in neuroinflammatory and neurodegenerative diseases. EMBO Mol. Med. 11, 248. doi: 10.15252/emmm.201810248
Wan, T., Fu, M., Jiang, Y., Jiang, W., Li, P., Zhou, S., et al. (2022a). Research progress on mechanism of neuroprotective roles of apelin-13 in prevention and treatment of Alzheimer's disease. Neurochem. Res. 47, 205–217. doi: 10.1007/s11064-021-03448-1
Wan, T., Huang, Y., Gao, X., Wu, W., and Guo, W. (2022b). Microglia polarization: a novel target of exosome for stroke treatment. Front. Cell Dev. Biol. 10, 842320. doi: 10.3389/fcell.2022.842320
Wang, K., Ru, J., Zhang, H., Chen, J., Lin, X., Lin, Z., et al. (2020a). Melatonin enhances the therapeutic effect of plasma exosomes against cerebral ischemia-induced pyroptosis through the TLR4/NF-κB PATHWAY. Front Neurosci. 14, 848. doi: 10.3389/fnins.2020.00848
Wang, K., Sun, Z., Ru, J., Wang, S., Huang, L., Ruan, L., et al. (2020b). Ablation of GSDMD improves outcome of ischemic stroke through blocking canonical and non-canonical inflammasomes dependent pyroptosis in microglia. Front. Neurol. 11, 577927. doi: 10.3389/fneur.2020.577927
Wang, Q., Wu, J., Zeng, Y., Chen, K., Wang, C., Yang, S., et al. (2020c). Pyroptosis: A pro-inflammatory type of cell death in cardiovascular disease. Clin. Chim. Acta Int. J. Clin. Chem. 510, 62–72. doi: 10.1016/j.cca.2020.06.044
Wang, S., Yuan, Y. H., Chen, N. H., and Wang, H. B. (2019). The mechanisms of NLRP3 inflammasome/pyroptosis activation and their role in Parkinson's disease. Int. Immunopharmacol. 67, 458–464. doi: 10.1016/j.intimp.2018.12.019
Wang, W., Nguyen, L. T., Burlak, C., Chegini, F., Guo, F., Chataway, T., et al. (2016). Caspase-1 causes truncation and aggregation of the Parkinson's disease-associated protein alpha-synuclein. Proc. Natl. Acad. Sci. U. S. A. 113, 9587–9592. doi: 10.1073/pnas.1610099113
Wang, Y., Gao, W., Shi, X., Ding, J., Liu, W., He, H., et al. (2017). Chemotherapy drugs induce pyroptosis through caspase-3 cleavage of a gasdermin. Nature. 547, 99–103. doi: 10.1038/nature22393
Wu, D., Wang, S., Yu, G., and Chen, X. (2021). Cell death mediated by the pyroptosis pathway with the aid of nanotechnology: prospects for cancer therapy. Angewandte Chem.. 60, 8018–8034. doi: 10.1002/anie.202010281
Xia, L., Liu, L., Cai, Y., Zhang, Y., Tong, F., Wang, Q., et al. (2021). Inhibition of gasdermin D-mediated pyroptosis attenuates the severity of seizures and astroglial damage in kainic acid-induced epileptic mice. Front. Pharmacol. 12, 751644. doi: 10.3389/fphar.2021.751644
Xia, X., Wang, X., Cheng, Z., Qin, W., Lei, L., Jiang, J., et al. (2019). The role of pyroptosis in cancer: pro-cancer or pro-“host”? Cell Death Dis. 10, 650. doi: 10.1038/s41419-019-1883-8
Xu, P., Hong, Y., Xie, Y., Yuan, K., Li, J., Sun, R., et al. (2021a). TREM-1 exacerbates neuroinflammatory injury via NLRP3 inflammasome-mediated pyroptosis in experimental subarachnoid hemorrhage. Transl. Stroke Res. 12, 643–659. doi: 10.1007/s12975-020-00840-x
Xu, P., Zhang, X., Liu, Q., Xie, Y., Shi, X., Chen, J., et al. (2019a). Microglial TREM-1 receptor mediates neuroinflammatory injury via interaction with SYK in experimental ischemic stroke. Cell Death Dis. 10, 555. doi: 10.1038/s41419-019-1777-9
Xu, S., Wang, J., Jiang, J., Song, J., Zhu, W., Zhang, F., et al. (2020). TLR4 promotes microglial pyroptosis via lncRNA-F630028O10Rik by activating PI3K/AKT pathway after spinal cord injury. Cell Death Dis. 11, 693. doi: 10.1038/s41419-020-02824-z
Xu, S., Wang, J., Zhong, J., Shao, M., Jiang, J., Song, J., et al. (2021b). CD73 alleviates GSDMD-mediated microglia pyroptosis in spinal cord injury through PI3K/AKT/Foxo1 signaling. Clin. Transl. Med. 11, e269. doi: 10.1002/ctm2.269
Xu, X. E., Liu, L., Wang, Y. C., Wang, C. T., Zheng, Q., Liu, Q. X., et al. (2019b). Caspase-1 inhibitor exerts brain-protective effects against sepsis-associated encephalopathy and cognitive impairments in a mouse model of sepsis. Brain Behav. Immun. 80, 859–870. doi: 10.1016/j.bbi.2019.05.038
Xue, Y., Enosi Tuipulotu, D., Tan, W. H., Kay, C., and Man, S. M. (2019). Emerging activators and regulators of inflammasomes and pyroptosis. Trends Immunol. 40, 1035–1052. doi: 10.1016/j.it.2019.09.005
Yan, J., Xu, W., Lenahan, C., Huang, L., Wen, J., Li, G., et al. (2021). CCR5 Activation promotes NLRP1-dependent neuronal pyroptosis via CCR5/PKA/CREB pathway after intracerebral hemorrhage. Stroke. 52, 4021–4032. doi: 10.1161/STROKEAHA.120.033285
Yang, C., Sui, G., Wang, L., Chen, Z., and Wang, F. (2022). MiR-124 prevents the microglial proinflammatory response by inhibiting the activities of TLR4 and downstream NLRP3 in palmitic acid-treated BV2 cells. J. Mol. Neurosci. 72, 496–506. doi: 10.1007/s12031-021-01921-8
Yang, S., Zhang, X., Zhang, H., Lin, X., Chen, X., Zhang, Y., et al. (2021). Dimethyl itaconate inhibits LPSinduced microglia inflammation and inflammasomemediated pyroptosis via inducing autophagy and regulating the Nrf2/HO1 signaling pathway. Mol. Med. Rep. 24, 12311. doi: 10.3892/mmr.2021.12311
Yao, Y., Li, C., Qian, F., Zhao, Y., Shi, X., Hong, D., et al. (2021). Ginsenoside Rg1 inhibits microglia pyroptosis induced by lipopolysaccharide through regulating STAT3 signaling. J. Inflam. Res. 14, 6619–6632. doi: 10.2147/JIR.S326888
Ye, Y., Jin, T., Zhang, X., Zeng, Z., Ye, B., Wang, J., et al. (2019). Meisoindigo protects against focal cerebral ischemia-reperfusion injury by inhibiting NLRP3 inflammasome activation and regulating microglia/macrophage polarization via TLR4/NF-kappaB signaling pathway. Front. Cell Neurosci. 13, 553. doi: 10.3389/fncel.2019.00553
Yu, M., Fu, Y., Liang, Y., Song, H., Yao, Y., Wu, P., et al. (2017). Suppression of MAPK11 or HIPK3 reduces mutant Huntingtin levels in Huntington's disease models. Cell Res. 27, 1441–1465. doi: 10.1038/cr.2017.113
Yu, Y., and Ye, R. D. (2015). Microglial abeta receptors in Alzheimer's disease. Cell Mol. Neurobiol. 35, 71–83. doi: 10.1007/s10571-014-0101-6
Yuan, L., Zhu, Y., Huang, S., Lin, L., Jiang, X., Chen, S., et al. (2021). NF-κB/ROS and ERK pathways regulate NLRP3 inflammasome activation in Listeria monocytogenes infected BV2 microglia cells. J. Microbiol. 59, 771–781. doi: 10.1007/s12275-021-0692-9
Zhai, L., Shen, H., Sheng, Y., and Guan, Q. A. D. M. S. C. (2021). Exo-MicroRNA-22 improve neurological function and neuroinflammation in mice with Alzheimer's disease. J. Cell. Mol. Med. 25, 7513–7523. doi: 10.1111/jcmm.16787
Zhang, D., Qian, J., Zhang, P., Li, H., Shen, H., Li, X., et al. (2019). Gasdermin D serves as a key executioner of pyroptosis in experimental cerebral ischemia and reperfusion model both in vivo and in vitro. J. Neurosci. Res. 97, 645–660. doi: 10.1002/jnr.24385
Zhang, P., Shao, X. Y., Qi, G. J., Chen, Q., Bu, L. L., Chen, L. J., et al. (2016). Cdk5-dependent activation of neuronal inflammasomes in Parkinson's disease. Mov. Disord. 31, 366–376. doi: 10.1002/mds.26488
Zhao, M., Dai, Y., Li, P., Wang, J., Ma, T., Xu, S., et al. (2021). Inhibition of NLRP3 inflammasome activation and pyroptosis with the ethyl acetate fraction of Bungeanum ameliorated cognitive dysfunction in aged mice. Food Funct. 12, 10443–10458. doi: 10.1039/D1FO00876E
Zheng, Y., Zhang, J., Zhao, Y., Zhang, Y., Zhang, X., Guan, J., et al. (2021). Curcumin protects against cognitive impairments in a rat model of chronic cerebral hypoperfusion combined with diabetes mellitus by suppressing neuroinflammation, apoptosis, and pyroptosis. Int. Immunopharmacol. 93, 107422. doi: 10.1016/j.intimp.2021.107422
Zhou, L. J., Peng, J., Xu, Y. N., Zeng, W. J., Zhang, J., Wei, X., et al. (2019). Microglia are indispensable for synaptic plasticity in the spinal dorsal horn and chronic pain. Cell Rep. 27, 3844–59.e6. doi: 10.1016/j.celrep.2019.05.087
Keywords: microglia, pyroptosis, GSDMs, neuroinflammation, neurological diseases
Citation: Wu X, Wan T, Gao X, Fu M, Duan Y, Shen X and Guo W (2022) Microglia Pyroptosis: A Candidate Target for Neurological Diseases Treatment. Front. Neurosci. 16:922331. doi: 10.3389/fnins.2022.922331
Received: 17 April 2022; Accepted: 23 June 2022;
Published: 22 July 2022.
Edited by:
Asma Perveen, Glocal University, IndiaReviewed by:
Xiangping Chu, University of Missouri–Kansas City, United StatesCopyright © 2022 Wu, Wan, Gao, Fu, Duan, Shen and Guo. This is an open-access article distributed under the terms of the Creative Commons Attribution License (CC BY). The use, distribution or reproduction in other forums is permitted, provided the original author(s) and the copyright owner(s) are credited and that the original publication in this journal is cited, in accordance with accepted academic practice. No use, distribution or reproduction is permitted which does not comply with these terms.
*Correspondence: Xiangru Shen, MzIyNjYxODQyN0BxcS5jb20=; Weiming Guo, MjUzNzc5MjExQHFxLmNvbQ==
†These authors have contributed equally to this work and share first authorship
‡These authors have contributed equally to this work
Disclaimer: All claims expressed in this article are solely those of the authors and do not necessarily represent those of their affiliated organizations, or those of the publisher, the editors and the reviewers. Any product that may be evaluated in this article or claim that may be made by its manufacturer is not guaranteed or endorsed by the publisher.
Research integrity at Frontiers
Learn more about the work of our research integrity team to safeguard the quality of each article we publish.