- 1Department of Functional Neurosurgery, The Affiliated Brain Hospital of Nanjing Medical University, Nanjing, China
- 2Department of Anesthesiology, The Affiliated Brain Hospital of Nanjing Medical University, Nanjing, China
Background: Subthalamic nucleus deep brain stimulation (STN–DBS) surgery for Parkinson’s disease (PD) is routinely performed at medical centers worldwide. However, it is debated whether general anesthesia (GA) or traditional local anesthetic (LA) is superior.
Purpose: This study aims to compare the effects of LA and GA operation methods on clinical improvement in patients with PD, such as motor and non-motor symptoms, after STN–DBS surgery at our center.
Method: A total of 157 patients with PD were retrospectively identified as having undergone surgery under LA (n = 81) or GA (n = 76) states. In this study, the Unified Parkinson’s Disease Rating Scale Motor Score (UPDRS-III) in three states, levodopa-equivalent-daily-dose (LEDD), surgical duration, intraoperative microelectrode recording (iMER) signal length, postoperative intracranial volume, electrode implantation error, neuropsychological function, quality of life scores, and complication rates were collected and compared. All patients with PD were routinely followed up at 6, 12, 18, and 24 months postoperatively.
Result: Overall improvement in UPDRS-III was demonstrated at postoperative follow-up, and there was no significant difference between the two groups in medication-off, stimulation-off state and medication-off, stimulation-on state. However, UPDRS-III scores in medication-on, stimulation-on state under GA was significantly lower than that in the LA group. During postoperative follow-up, LEDD in the LA group (6, 12, 18, and 24 months, postoperatively) was significantly lower than in the GA group. However, there were no significant differences at baseline or 1-month between the two groups. The GA group had a shorter surgical duration, lower intracranial volume, and longer iMER signal length than the LA group. However, there was no significant group difference in electrode implantation accuracy and complication rates. Additionally, the Hamilton Anxiety Scale (HAMA) was significantly lower in the GA group than the LA group at 1-month follow-up, but this difference disappeared at longer follow-up. Besides, there was no significant group difference in the 39-item Parkinson’s Disease Questionnaire (PDQ-39) scale scores.
Conclusion: Although both groups showed overall motor function improvement without a significant postoperative difference, the GA group seemed superior in surgical duration, intracranial volume, and iMER signal length. As the accuracy of electrode implantation can be ensured by iMER monitoring, DBS with GA will become more widely accepted.
Introduction
Parkinson’s disease (PD), the main pathological mechanism of loss of dopaminergic neurons in the substantia nigra, is one of the most common neurodegenerative diseases affecting the older adults, second only to Alzheimer’s disease (Tysnes and Storstein, 2017; Simon et al., 2020). Dopaminergic treatment can initially control the motor symptoms of PD; however, as the disease progresses, this treatment is likely to cause medication-related side effects, such as motor fluctuations, dyskinesia, and impulse control disorders (Heumann et al., 2014; Wang et al., 2021). Deep brain stimulation (DBS) surgery targeting the subthalamic nucleus (STN), which has become a mainstream therapy for advanced PD (Herrington et al., 2016), can effectively resolve the above side effects and reduce the required levodopa dosage after surgery (Krack et al., 2019). Furthermore, considering the effects of general anesthesia (GA) on intraoperative microelectrode recording (iMER), an awake technique is the standard approach for DBS electrode insertion at most centers (Tempelhoff, 2017; Bos et al., 2019; Vesper et al., 2022). During this process, intraoperative temporary stimulation is performed to verify the patient’s contralateral limb muscle tension, exercise ability, and any side effects and to determine the optimal electrode implantation target in the target nucleus (Kraus and Pohle, 2000; Chen et al., 2018a; Walker et al., 2019). However, some PD patients with severe medication-off symptoms and excessive anxiety cannot tolerate DBS placement in an awake state (Chen et al., 2018b; Asriyants et al., 2021). Consequently, it is preferable to perform DBS surgery while such patients with PD are asleep (Ko and Burchiel, 2018; Ko et al., 2018).
Although many centers have successfully performed DBS insertion under GA, few reports compare the outcomes of DBS surgery performed under GA or in an awake state with local anesthesia (LA). Here, we present postoperative clinical differences from multiple dimensions for patients with PD undergoing awake or asleep bilateral STN-DBS lead placement at a single center.
Materials and Methods
Patients and Data
A total of 157 patients with PD who underwent STN-DBS surgery at our center between January 2018 and January 2020. A multidisciplinary team performed the diagnosis and surgical indications of the patients based on the United Kingdom PD Brain Bank criteria and relevant expert consensus (Poewe et al., 2017). In addition to routine examinations to rule out surgical contraindications, each patient with PD underwent medication-on and medication-off Unified Parkinson’s Disease Rating Scale part III (UPDRS-III) testing preoperatively and showed an improvement rate of medication-on/off > 30%. Patients who demonstrated dementia or severe psychiatric disorders, a history of radiation-based therapy or DBS therapy for PD, or any other movement disorder were excluded. Patients with PD were not randomly assigned to groups; instead, awake or asleep DBS surgery was determined based on the inclination of the patients and evaluation by neurologists specializing in movement disorders. After surgery, all patients routinely visited the center for assessment of changes in motor function in the medication-off, stimulation-off state (OFF-OFF), the medication-off, stimulation-on state (OFF-ON), and the medication-on, stimulation-on state (ON-ON), and non-motor function.
Neurosurgical Procedure
For the LA group, all dopaminergic medications except levodopa were discontinued at least 3 days before surgery, and levodopa was withdrawn at least 12 h preoperatively due to the intraoperative temporary stimulation test, which was not necessary for the GA group. The detailed surgical procedures were as follows: First, a stereotactic frame was installed under local infiltration anesthesia of the scalp. The two sides of the frame were placed parallel to the anterior commissure–posterior commissure (AC-PC) projection line between 2 cm above the lateral canthus and 3.5 cm above the outer ear hole. Next, a thin slice (1.0 mm) stereotactic computed tomography (CT) scan fused with a 3.0 T magnetic resonance imaging (MRI) scan, including sagittal T1 and axial T2/SWI sequences, was used to guide electrode implantation using the SurgiPlan system. Eventually, bilateral quadripolar electrodes were implanted into the STN under multimodal fusion imaging and intraoperative electrophysiology guidance. In the LA group, patients remained awake during electrode implantation, such as iMER and intraoperative test stimulation, which co-determined the final location of electrode implantation. In contrast, the GA group accepted endotracheal intubation after induction of anesthesia (fentanyl citrate 0.002–0.004 mg/kg, cis-atra library ammonium 0.15 mg/kg, and propofol 1.50–2.50 mg/kg) and the depth of anesthesia was monitored by the bispectral index (BIS) during maintenance of anesthesia (propofol 3–5 mg/kg⋅h and remifentanil 0.03–0.06 mg/kg⋅h). Propofol was completely stopped approximately 15–20 min before iMER, and remifentanil was reduced to 0.007 mg/kg⋅h to ensure a BIS value was 60 ± 5. After obtaining a satisfactory bilateral electrodeposition, the pulse generator was usually implanted into the subclavian subcutaneous fat layer on the patient’s left side under GA in both groups.
Statistical Analysis
Statistical analysis was performed using SPSS Statistics v. 25 (IBM, United States). Continuous data are presented as the mean ± standard deviation (SD), while dichotomous data are expressed as percentages. A value of p < 0.05 was considered statistically significant. The independent samples t-test or the Mann–Whitney U-test depended on whether the data satisfied the normality criterion. A one-way repeated analysis of variance (ANOVA) was performed to compare UPDRS-III and LEDD after testing normality and homogeneity of variance. A Bonferroni correction was applied for multiple comparisons.
Results
Patient Characteristics
As shown in Table 1, the baseline characteristics were similar among patients with PD in the LA and GA groups.
Motor Function Assessment
For all patients (LA = 81 and GA = 76), UPDRS-III scores in three states (OFF-OFF, OFF-ON, and ON-ON) were analyzed at 6, 12, 18, and 24 months postoperatively. Both groups showed improvement in motor function with stimulation-on, although motor function tended to decline with longer follow-up.
OFF-OFF
Significant differences were observed at the different time points within both group by one-way repeated measures ANOVA [LA: F(2.84, 227.11) = 66.91, p < 0.001, GA: F(2.41, 181.03) = 73.19, p < 0.001]. The Post hoc analysis with Bonferroni correction showed that the UPDRS-III scores increased gradually over time postoperatively for both groups. However, in the LA group, there were no significant differences between baseline and 6 months follow-up (p = 1.000), baseline and 12 months follow-up (p = 0.244), and 6 and 12 months follow-up (p = 0.568). In the GA group, there were no significant differences between 6 and 12 months follow-up or 18 and 24 months follow-up (p = 0.080 and p = 0.282, respectively). Two-way repeated measures ANOVA showed significant differences for follow-up time [F(1.40, 104.78) = 15.04, p < 0.001] but not group [F(1.00, 75.00) = 2.39, p = 0.126]. In addition, there was a significant interaction between follow-up time and group [F(2.09, 156.85) = 42.40, p < 0.001]. When the LA and GA groups were analyzed, there were no significant differences at baseline [F(1.00, 75.00) = 0.71, p = 0.404)] 6 months [F(1.00, 75.00) = 0.01, p = 0.930], 12 months [F(1.00, 75.00) = 0.05, p = 0.819], 18 months [F(1.00, 75.00) < 0.001, p = 1.000], and 24 months [F(1.00, 75.00) = 1.38, p = 0.244] (Figure 1A).
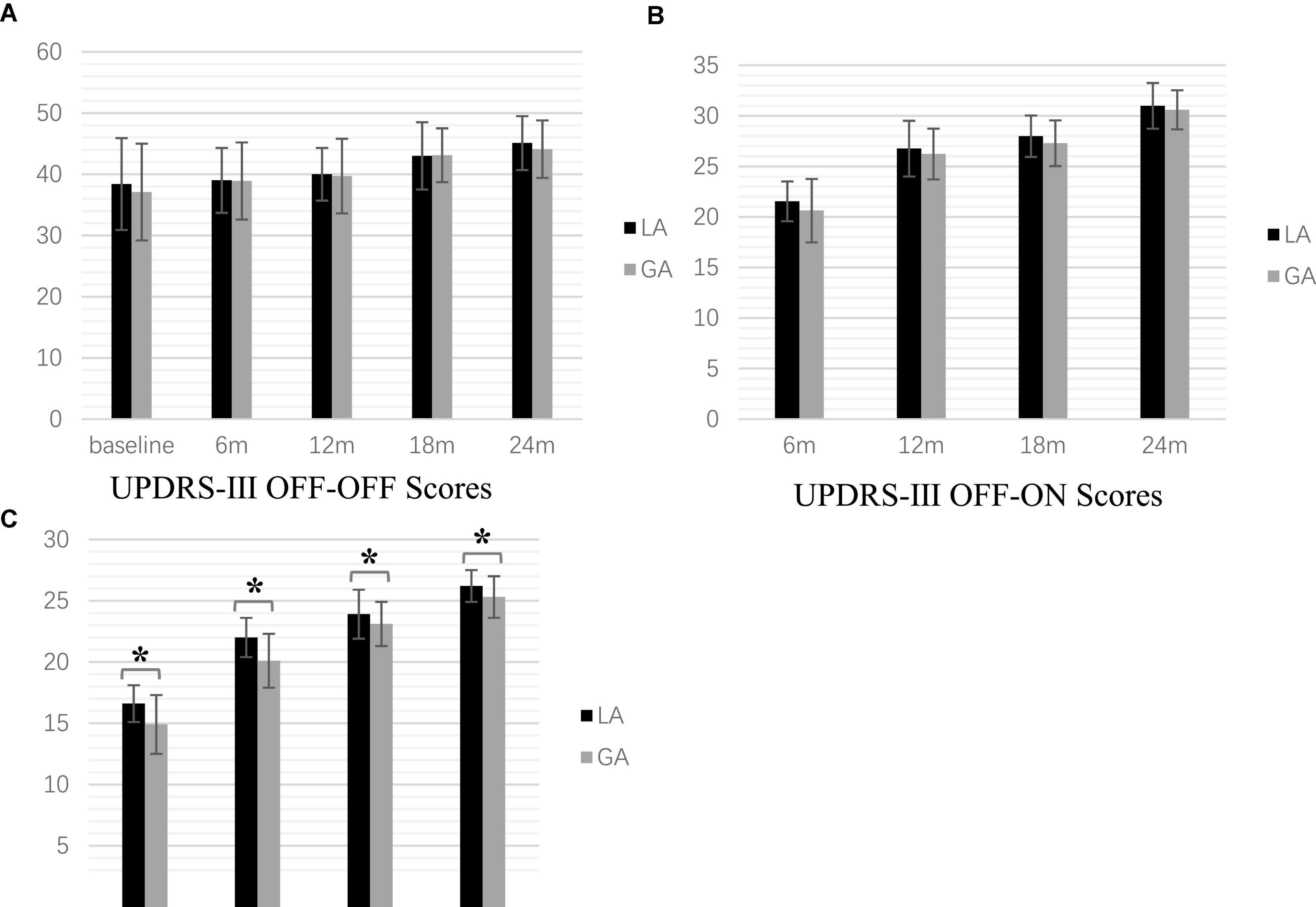
Figure 1. Postoperative motor symptom scale scores at the time point of follow-up (*P < 0.001). (A) UPDRS-β OFF-OFF Scores (B) UPDRS-β OFF-ON Scores (C). UPDRS-β ON-ON Scores.
OFF-ON
One-way repeated measures ANOVA revealed significant differences at different time points in both the LA and GA groups [LA: F(1.44, 115.28) = 269.18, p < 0.001, GA: F(1.41, 106.00) = 228.27, p < 0.001]. The post hoc analysis with Bonferroni correction indicated statistically significant increases at all postoperative follow-up times (all p < 0.001). Two-way repeated measures ANOVA showed significant differences for the follow-up time [F(2.13, 159.50) = 124.11, p < 0.001], but no significant group difference [F(1.00, 75.00) = 0.46, p = 0.499]. Furthermore, there was a significant association between follow-up time and group interaction [F(2.14, 160.48) = 260.95, p < 0.001]. However, there was no significant difference between the GA and LA groups at baseline [F(1.00, 75.00) = 0.71, p = 0.404], 6 months [F(1.00, 75.00) = 3.92, p = 0.051], 12 months [F(1.00, 75.00) = 3.14, p = 0.081], 18 months [F(1.00, 75.00) = 3.22, p = 0.077], and 24 months [F(1.00, 75.00) = 2.61, p = 0.110] (Figure 1B).
ON-ON
[Frame6]Two-way repeated measures ANOVA showed significant differences for follow-up time [F(1.00, 75.00) = 12.66, p = 0.001] and group [F(1.45, 108.60) = 916.36, P < 0.001], but not the interaction [F(1.30, 97.38) = 0.79, p = 0.407]. One-way repeated measures ANOVA revealed significant differences at 6 months [F(1.00, 75.00) = 31.89, p < 0.001], 12 months [F(1.00, 75.00) = 41.93, p < 0.001], 18 months [F(1.00, 75.00) = 5.03, p = 0.028], 24 months [F(1.00, 75.00) = 14.50, p < 0.001], but not the baseline [F(1.00, 75.00) = 0.71, p = 0.404], among the two groups (Figure 1C).
Levodopa Equivalent of Daily Dose Reduction
One-way repeated measures ANOVA showed a significant difference in LEDD with follow-up time for both groups [LA: F(3.71, 296.38) = 3,975.92, p < 0.001, GA: F(3.29, 246.59) = 4,064.22, p < 0.001]. The post hoc analysis with Bonferroni correction showed a statistically significant reduction in both groups compared with the preoperative baseline (both p < 0.001). Mann–Whitney U-tests revealed that LEDD in the LA group (6, 12, 18, and 24 months postoperatively) were significantly lower than those in the GA group (all p < 0.001); however, there were no significant differences at baseline or 1 month between the two groups (p = 0.898 and p = 0.058, respectively) (Figure 2).
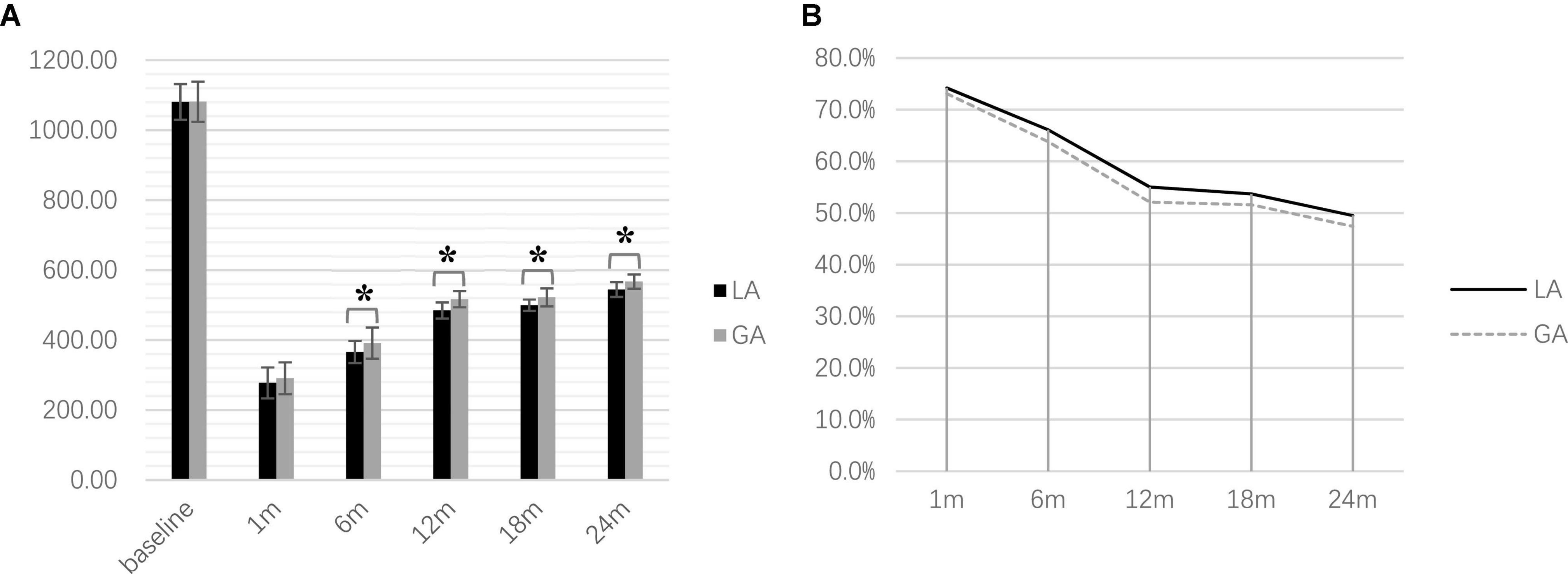
Figure 2. Postoperative levodopa-equivalent-daily-dose at the time point of follow-up (*P < 0.001). (A) Comparison of LEDD between two groups (B) LEDD reduction during postoperative follow-up.
Surgical Duration and Intraoperative Microelectrode Recording Signal Length
The two-sample t-test showed that the surgical duration (lead placement only) in the GA group was significantly shorter than that in the LA group (p < 0.001; LA: 179.7 ± 6.3 min; GA: 109.8 ± 7.3 min). In contrast, bilateral iMER signal length in the GA group was significantly longer than in the LA group (Table 2).
Postoperative Intracranial Volume and Electrode Implantation Error
On the first postoperative day, head CT showed a significant difference between the LA and GA groups in terms of intracranial gas accumulation (6.04 ± 1.33 vs. 3.48 ± 1.22 mL, p < 0.001) (Figure 3A). However, there was no significant difference in the accuracy of electrode implantation between the two groups (LA: 0.78 ± 0.08 mm; GA: 0.77 ± 0.08 mm, p = 0.606), as shown by the fused CT 1 week after surgery and preoperative imaging plans (Figure 3B).
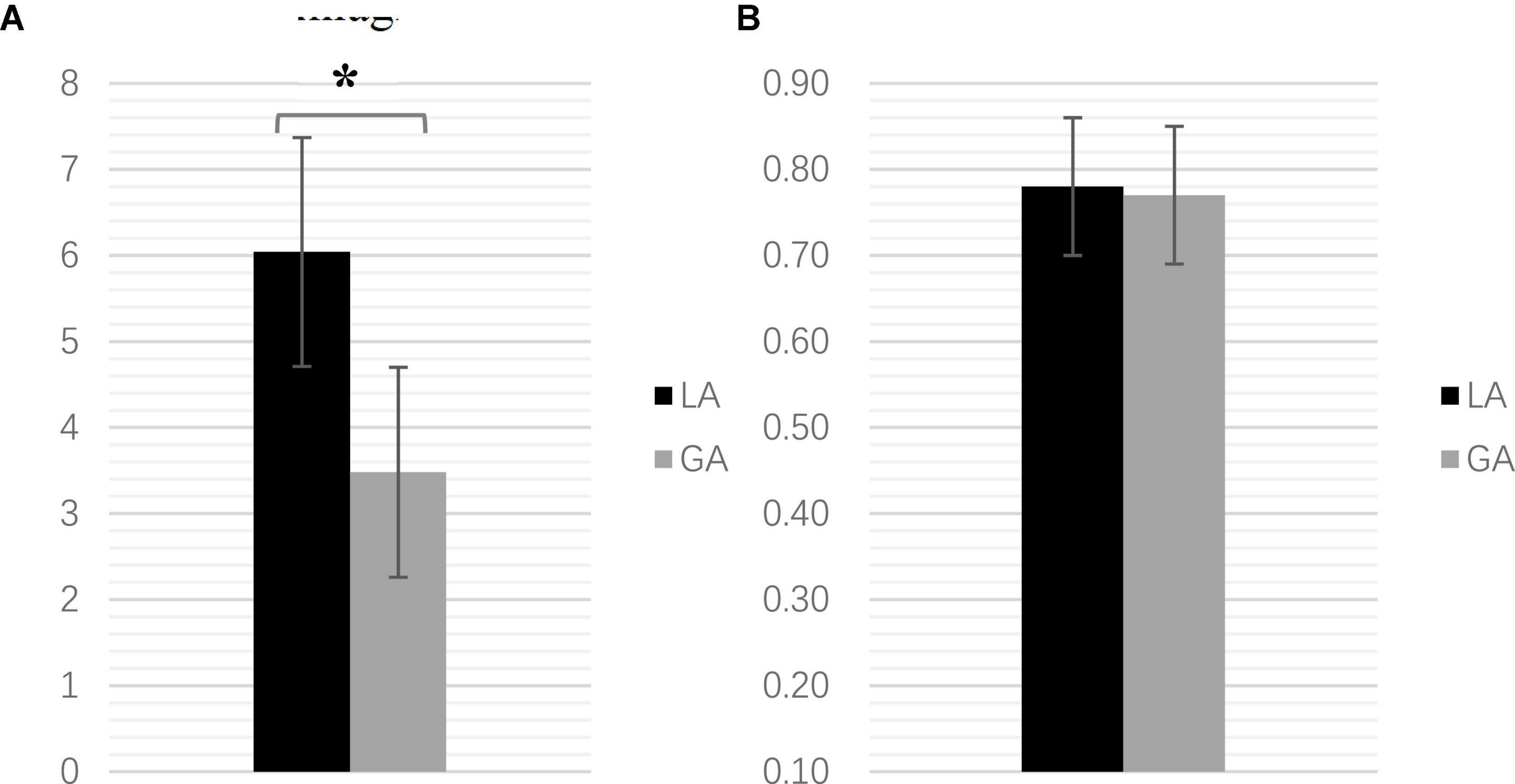
Figure 3. (A) The intracranial volume comparison (mL) (*P < 0.001). (B) The electrode implantation error comparison (mm).
Hamilton Anxiety Scale and 39-Item Parkinson’s Disease Questionnaire
The Mann–Whitney U-tests showed that the postoperative Hamilton Anxiety (HAMA) (1 month) scores in the GA group were significantly lower than those in the LA group (p < 0.001). However, there were no significant group differences in HAMA scores at baseline or the other follow-up time points. Additionally, there were no significant group differences at baseline or postoperative follow-up in the 39-item Parkinson’s Disease Questionnaire (PDQ-39) scores (Table 3).
Complications
No intracranial hematoma, ischemic infarction, or intracranial infection occurred in either group after DBS lead placement. Delayed skin incision infection occurred in two cases in each group (one case each of retroauricular incision infection and frontal incision infection in the LA group; two cases of frontal skin incision infection in the GA group).
Discussion
Since the advent of DBS surgery, lead placement in an awake state has become the standard procedure. The location and depth of electrode implantation are determined by iMER and temporary stimulation tests to minimize the adverse effects of stimulation (Blume et al., 2017; Mehanna et al., 2017; Walker et al., 2019; Frequin et al., 2020). However, lead placement under GA is a better option for some PD patients with severe off-medication symptoms and anxiety. In 2006, Hertel et al. (2006) first reported STN-DBS surgery under GA with intraoperative MER guidance. Many neurosurgery centers are starting to perform asleep electrode insertion using intraoperative imaging (e.g., CT and MRI) or MER guidance to ensure electrode implantation accuracy.
Our study showed that motor function improved postoperatively in the GA and LA groups, as assessed by UPDRS-III motor scores. Notably, UPDRS-III (ON-ON) improvement in the GA group was significantly greater than that in the LA group at postoperative follow-up. However, the group differences in UPDRS-III (OFF-OFF and OFF-ON) disappeared over long-term follow-up. Given these findings, there are two primary considerations. On the one hand, the surgery itself did not stop symptoms from worsening over time, and disease progression was generally similar between both groups. There was no significant difference between the groups in terms of just the postoperative efficacy of DBS stimulation-on in improving motor symptoms. On the other hand, it is possible that disease progression post-GA operation was faster compared with the post-LA operation, increasing the demand for drugs in the GA group. Due to greater PD drug use in the GA group postoperatively, the UPDRS-III (ON-ON) scores were significantly lower than those of the LA group due to the significantly lower LEDD after surgery in the LA group.
In terms of non-motor function, this study demonstrated significant differences in emotion during the short postoperative period, but the differences disappeared over long-term follow-up. However, the psychological status of the patients after DBS surgery was susceptible to various factors, such as the influence of the postoperative programming state. So the difference in the psychological scores could not be attributed to the impact of different anesthesia methods. Nevertheless, given that patients in the LA group remained awake during electrode implantation and had a longer surgical duration, this highlights the advantages of GA surgery, which can provide a more comfortable experience and broaden the patient population.
Our study found no significant differences in the incidence of intracerebral hemorrhages or infections between the LA and GA group. However, the awake group had a larger intracranial air volume (Blasberg et al., 2018; Ko et al., 2018; Jin et al., 2020). There are two possible explanations. First, the surgical duration in the LA group was longer. Second, the intraoperative uncontrollable cough and quiescent tremor in patients with PD increased cerebrospinal fluid loss, further conducive to increased intracranial volume.
The clinical prognosis of patients with PD after DBS implantation is closely related to electrode implantation accuracy (Chen et al., 2015; Li et al., 2016). Different anesthesia protocols involve different methods of ensuring that electrodes are implanted at the intended target (Li et al., 2021). Intraoperative verification of the actual targets depended on MER and the stimulation test in the LA group and MER or intraoperative imaging in the GA group. In our study, the electrophysiological signal length is longer under GA than under LA. The main reason for this result is that every patient in the GA group received microelectrode recording during microelectrode insertion. Most typical STN discharge waveforms can be recorded by selecting the ideal anesthesia protocol and monitoring the anesthesia depth (60 ± 5) through BIS (Jain et al., 2007; Kinfe and Vesper, 2013; Zeiler et al., 2013; Chakrabarti et al., 2014; Bezchlibnyk et al., 2020). However, during local anesthesia surgery, some patients suffer excessive nervousness, coughing, or severe tremors, which would interfere with electrophysiological signals. Since intraoperative images are not used at our center, mainly due to brain shifts caused by intraoperative cerebrospinal fluid loss, there are inevitable errors in the fusion of intraoperative images and preoperative planned images to determine the location of electrode implantation (Larson et al., 2018). However, iMER can monitor whether the microelectrode has entered the target nucleus and the depth of insertion, which has become the gold standard for accurate DBS surgery, requiring no intraoperative imaging. Finally, it should be noted that this study is subjected to limitations in terms of selection bias since patients with PD were not randomized to the GA or LA groups.
Conclusion
Although the implantation of electrodes under LA is the standard DBS procedure, GA is becoming increasingly common due to improvements in anesthesia protocols and technology. Our study found no significant differences between GA and LA in improving motor symptoms or postoperative complication incidence in patients with PD undergoing DBS surgery. However, GA might be superior to LA in terms of short-term postoperative changes in non-motor symptoms. The possible advantages of DBS surgery under GA are that it is more acceptable and suitable for more patients with PD, especially those with obvious motor symptoms and anxiety (Venkatraghavan and Sheshadri, 2017). However, due to the limitations of this study, we cannot conclude that DBS surgery under GA is better than LA. More randomized controlled trials are required to determine which method is ideal.
Data Availability Statement
The original contributions presented in the study are included in the article/supplementary material, further inquiries can be directed to the corresponding author/s.
Ethics Statement
The studies involving human participants were reviewed and approved by the Ethics Committee of the Brain Hospital affiliated with Nanjing Medical University. The patients/participants provided their written informed consent to participate in this study. Written informed consent was obtained from the individual(s) for the publication of any potentially identifiable images or data included in this article.
Author Contributions
YL and LC designed and wrote the manuscript. CQ and BL were responsible for data collection. JL, WD, and YL were responsible for data processing and analysis. WZ and YR contributed to the design of the study. WZ edited and revised the manuscript. All authors contributed to and approved the final manuscript.
Funding
This study was supported by a grant from the subtopic of the 13th Five-Year National Key Research and Development Plan (No. 2016YFC0105901NNZ) and the Nanjing Health Science and Technology Development Special Fund Project (No. ZKX20031).
Conflict of Interest
The authors declare that the research was conducted in the absence of any commercial or financial relationships that could be construed as a potential conflict of interest.
Publisher’s Note
All claims expressed in this article are solely those of the authors and do not necessarily represent those of their affiliated organizations, or those of the publisher, the editors and the reviewers. Any product that may be evaluated in this article, or claim that may be made by its manufacturer, is not guaranteed or endorsed by the publisher.
References
Asriyants, S. V., Tomskiy, A. A., Gamaleya, A. A., and Pronin, I. N. (2021). [Deep brain stimulation of the subthalamic nucleus for parkinson’s disease: awake vs asleep]. Zh Vopr Neirokhir Im N N Burdenko 85, 117–121. doi: 10.17116/neiro202185051117
Bezchlibnyk, Y. B., Sharma, V. D., Naik, K. B., Isbaine, F., Gale, J. T., Cheng, J., et al. (2020). Clinical outcomes of globus pallidus deep brain stimulation for Parkinson disease: a comparison of intraoperative MRI- and MER-guided lead placement. J. Neurosurg. 134, 1072–1082. doi: 10.3171/2019.12.JNS192010
Blasberg, F., Wojtecki, L., Elben, S., Slotty, P. J., Vesper, J., Schnitzler, A., et al. (2018). Comparison of Awake vs. Asleep Surgery for Subthalamic Deep Brain Stimulation in Parkinson’s Disease. Neuromodulation 21, 541–547. doi: 10.1111/ner.12766
Blume, J., Schlaier, J., Rothenfusser, E., Anthofer, J., Zeman, F., Brawanski, A., et al. (2017). Intraoperative clinical testing overestimates the therapeutic window of the permanent DBS electrode in the subthalamic nucleus. Acta Neurochir. 159, 1721–1726. doi: 10.1007/s00701-017-3255-4
Bos, M. J., Alzate Sanchez, A. M., Smeets, A., Bancone, R., Ackermans, L., Absalom, A. R., et al. (2019). Effect of Anesthesia on Microelectrode Recordings during Deep Brain Stimulation Surgery in Tourette Syndrome Patients. Stereotact. Funct. Neurosurg. 97, 225–231. doi: 10.1159/000503691
Chakrabarti, R., Ghazanwy, M., and Tewari, A. (2014). Anesthetic challenges for deep brain stimulation: a systematic approach. N. Am. J. Med. Sci. 6, 359–369. doi: 10.4103/1947-2714.139281
Chen, L., Li, N., Gao, L., Yang, C., Fang, W., Wang, X. L., et al. (2015). Improved stereotactic procedure enhances the accuracy of deep brain stimulation electrode implantation in non-human primates. Int. J. Neurosci. 125, 380–389. doi: 10.3109/00207454.2014.940524
Chen, T., Mirzadeh, Z., Chapple, K. M., Lambert, M., Evidente, V. G. H., Moguel-Cobos, G., et al. (2018a). Intraoperative test stimulation versus stereotactic accuracy as a surgical end point: a comparison of essential tremor outcomes after ventral intermediate nucleus deep brain stimulation. J. Neurosurg. 129, 290–298. doi: 10.3171/2017.3.JNS162487
Chen, T., Mirzadeh, Z., Chapple, K. M., Lambert, M., Shill, H. A., Moguel-Cobos, G., et al. (2018b). Clinical outcomes following awake and asleep deep brain stimulation for Parkinson disease. J. Neurosurg. 130, 109–120. doi: 10.3171/2017.8.JNS17883
Frequin, H. L., Bot, M., Dilai, J., Scholten, M. N., Postma, M., Bour, L. J., et al. (2020). Relative Contribution of Magnetic Resonance Imaging, Microelectrode Recordings, and Awake Test Stimulation in Final Lead Placement during Deep Brain Stimulation Surgery of the Subthalamic Nucleus in Parkinson’s Disease. Stereotact. Funct. Neurosurg. 98, 118–128. doi: 10.1159/000505710
Herrington, T. M., Cheng, J. J., and Eskandar, E. N. (2016). Mechanisms of deep brain stimulation. J. Neurophysiol. 115, 19–38. doi: 10.1152/jn.00281.2015
Hertel, F., Zuchner, M., Weimar, I., Gemmar, P., Noll, B., Bettag, M., et al. (2006). Implantation of electrodes for deep brain stimulation of the subthalamic nucleus in advanced Parkinson’s disease with the aid of intraoperative microrecording under general anesthesia. Neurosurgery 59:E1138. discussion E1138. doi: 10.1227/01.NEU.0000245603.77075.55
Heumann, R., Moratalla, R., Herrero, M. T., Chakrabarty, K., Drucker-Colin, R., Garcia-Montes, J. R., et al. (2014). Dyskinesia in Parkinson’s disease: mechanisms and current non-pharmacological interventions. J. Neurochem. 130, 472–489. doi: 10.1111/jnc.12751
Jain, V., Prabhakar, H., Rath, G. P., and Sharma, D. (2007). Tension pneumocephalus following deep brain stimulation surgery with bispectral index monitoring. Eur. J. Anaesthesiol. 24, 203–204. doi: 10.1017/S0265021506001736
Jin, H., Gong, S., Tao, Y., Huo, H., Sun, X., Song, D., et al. (2020). A comparative study of asleep and awake deep brain stimulation robot-assisted surgery for Parkinson’s disease. NPJ Parkinsons Dis. 6:27. doi: 10.1038/s41531-020-00130-1
Kinfe, T. M., and Vesper, J. (2013). The impact of multichannel microelectrode recording (MER) in deep brain stimulation of the basal ganglia. Acta Neurochir. Suppl. 117, 27–33. doi: 10.1007/978-3-7091-1482-7_5
Ko, A. L., and Burchiel, K. J. (2018). Image-Guided, Asleep Deep Brain Stimulation. Prog. Neurol. Surg. 33, 94–106. doi: 10.1159/000480984
Ko, A. L., Magown, P., Ozpinar, A., Hamzaoglu, V., and Burchiel, K. J. (2018). Asleep Deep Brain Stimulation Reduces Incidence of Intracranial Air during Electrode Implantation. Stereotact. Funct. Neurosurg. 96, 83–90. doi: 10.1159/000488150
Krack, P., Volkmann, J., Tinkhauser, G., and Deuschl, G. (2019). Deep Brain Stimulation in Movement Disorders: From Experimental Surgery to Evidence-Based Therapy. Mov. Dis. 34, 1795–1810. doi: 10.1002/mds.27860
Kraus, J. K., and Pohle, T. (2000). Intraoperative test stimulation with a modified implantable pulse generator in deep brain stimulation. Acta Neurochir. 142, 587–589. doi: 10.1007/s007010050474
Larson, P. S., Starr, P. A., and Martin, A. J. (2018). Deep Brain Stimulation: Interventional and Intraoperative MRI Approaches. Prog. Neurol. Surg. 33, 187–197. doi: 10.1159/000481103
Li, H., Wang, T., Zhang, C., Su, D., Lai, Y., Sun, B., et al. (2021). Asleep Deep Brain Stimulation in Patients With Isolated Dystonia: Stereotactic Accuracy. Efficacy, and Safety. Neuromodulation 24, 272–278. doi: 10.1111/ner.13341
Li, Z., Zhang, J. G., Ye, Y., and Li, X. (2016). Review on Factors Affecting Targeting Accuracy of Deep Brain Stimulation Electrode Implantation between 2001 and 2015. Stereotact. Funct. Neurosurg. 94, 351–362. doi: 10.1159/000449206
Mehanna, R., Machado, A. G., Connett, J. E., Alsaloum, F., and Cooper, S. E. (2017). Intraoperative Microstimulation Predicts Outcome of Postoperative Macrostimulation in Subthalamic Nucleus Deep Brain Stimulation for Parkinson’s Disease. Neuromodulation 20, 456–463. doi: 10.1111/ner.12553
Poewe, W., Seppi, K., Tanner, C. M., Halliday, G. M., Brundin, P., Volkmann, J., et al. (2017). Parkinson disease. Nat. Rev. Dis. Primers 3:17013. doi: 10.1038/nrdp.2017.13
Simon, D. K., Tanner, C. M., and Brundin, P. (2020). Parkinson Disease Epidemiology, Pathology, Genetics, and Pathophysiology. Clin. Geriatr. Med. 36, 1–12. doi: 10.1016/j.cger.2019.08.002
Tempelhoff, R. (2017). General Versus Local Anesthesia for Deep Brain Stimulator Insertion. J. Neurosurg. Anesthesiol. 29:347. doi: 10.1097/ANA.0000000000000394
Tysnes, O. B., and Storstein, A. (2017). Epidemiology of Parkinson’s disease. J. Neural. Transm. 124, 901–905. doi: 10.1007/s00702-017-1686-y
Venkatraghavan, L., and Sheshadri, V. (2017). PRO: General Anesthesia for Deep Brain Stimulator Insertion in Patients With Parkinson Disease. J. Neurosurg. Anesthesiol. 29, 348–349. doi: 10.1097/01.ana.0000520879.70965.73
Vesper, J., Mainzer, B., Senemmar, F., Schnitzler, A., Groiss, S. J., and Slotty, P. J. (2022). Anesthesia for deep brain stimulation system implantation: adapted protocol for awake and asleep surgery using microelectrode recordings. Acta Neurochir. 164, 1175–1182. doi: 10.1007/s00701-021-05108-3
Walker, H. C., Faulk, J., Rahman, A. F., Gonzalez, C. L., Roush, P., Nakhmani, A., et al. (2019). Awake Testing during Deep Brain Stimulation Surgery Predicts Postoperative Stimulation Side Effect Thresholds. Brain Sci. 9, 1–12. doi: 10.3390/brainsci9020044
Wang, M., Wang, W., Gao, Z., Yin, X., Chen, T., Jiang, Z., et al. (2021). Dyskinesia-hyperpyrexia syndrome in Parkinson’s disease: a systematic review. Clin. Auton. Res. 31, 529–542. doi: 10.1007/s10286-021-00801-w
Keywords: deep brain stimulation, general anesthesia, local anesthesia, subthalamic nucleus, Parkinson’s disease, intraoperative microelectrode recording
Citation: Lu Y, Chang L, Li J, Luo B, Dong W, Qiu C, Zhang W and Ruan Y (2022) The Effects of Different Anesthesia Methods on the Treatment of Parkinson’s Disease by Bilateral Deep Brain Stimulation of the Subthalamic Nucleus. Front. Neurosci. 16:917752. doi: 10.3389/fnins.2022.917752
Received: 11 April 2022; Accepted: 26 April 2022;
Published: 26 May 2022.
Edited by:
Nizhuan Wang, ShanghaiTech University, ChinaReviewed by:
Zenglin Cai, Affiliated Suzhou Science and Technology Town Hospital of Nanjing Medical University, ChinaJun Xu, Capital Medical University, China
Copyright © 2022 Lu, Chang, Li, Luo, Dong, Qiu, Zhang and Ruan. This is an open-access article distributed under the terms of the Creative Commons Attribution License (CC BY). The use, distribution or reproduction in other forums is permitted, provided the original author(s) and the copyright owner(s) are credited and that the original publication in this journal is cited, in accordance with accepted academic practice. No use, distribution or reproduction is permitted which does not comply with these terms.
*Correspondence: Wenbin Zhang, wenbinzhang@njmu.edu.cn; Yifeng Ruan, 15422401@qq.com
†These authors have contributed equally to this work and share first authorship