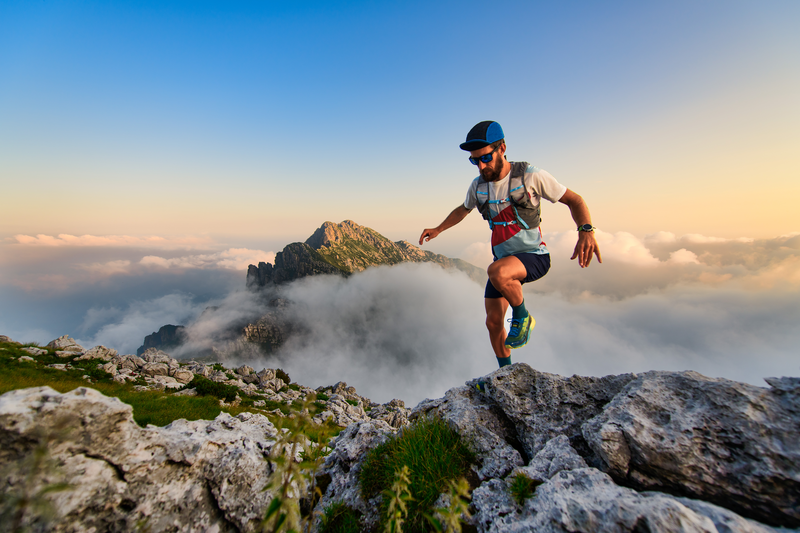
94% of researchers rate our articles as excellent or good
Learn more about the work of our research integrity team to safeguard the quality of each article we publish.
Find out more
MINI REVIEW article
Front. Neurosci. , 22 June 2022
Sec. Neuroenergetics and Brain Health
Volume 16 - 2022 | https://doi.org/10.3389/fnins.2022.898735
This article is part of the Research Topic Obesity and Diabetes: Implications for Brain-Immunometabolism - Volume II View all 7 articles
Carnosine is a dipeptide expressed in both the central nervous system and periphery. Several biological functions have been attributed to carnosine, including as an anti-inflammatory and antioxidant agent, and as a modulator of mitochondrial metabolism. Some of these mechanisms have been implicated in the pathophysiology of coronavirus disease-2019 (COVID-19). COVID-19 is caused by severe acute respiratory syndrome-coronavirus 2 (SARS-CoV-2). The clinical manifestation and recovery time for COVID-19 are variable. Some patients are severely affected by SARS-CoV-2 infection and may experience respiratory failure, thromboembolic disease, neurological symptoms, kidney damage, acute pancreatitis, and even death. COVID-19 patients with comorbidities, including diabetes, are at higher risk of death. Mechanisms underlying the dysfunction of the afflicted organs in COVID-19 patients have been discussed, the most common being the so-called cytokine storm. Given the biological effects attributed to carnosine, adjuvant therapy with this dipeptide could be considered as supportive treatment in patients with either COVID-19 or long COVID.
In 2019 the coronavirus disease-2019 (COVID-19) emerged in China (Huang et al., 2020). COVID-19 is caused by severe acute respiratory syndrome-coronavirus 2 (SARS-CoV-2; Al-Kuraishy and Al-Gareeb, 2020). SARS-CoV-2 has quickly spread around the world with devastating consequences (Bedford et al., 2020). The clinical manifestation and recovery time for COVID-19 are variable (Wen et al., 2020; Wiersinga et al., 2020). Most infected patients remain asymptomatic or present mild symptoms, including a flu-like condition consisting of nasal congestion, loss of taste and smell, fatigue, and fever (Wiersinga et al., 2020; Zhou et al., 2020). However, some patients are more severely affected by SARS-CoV-2 infection and may experience respiratory failure, thromboembolic disease, neurological symptoms, kidney damage, acute pancreatitis, and even death (Piroth et al., 2020; Wiersinga et al., 2020; Ye et al., 2020). Mechanisms underlying the dysfunction of the afflicted organs in COVID-19 patients have been discussed, the most common being the so-called cytokine storm include elevated levels of tumor necrosis factor-alpha (TNF-α) and interleukin 6 (Al-Kuraishy and Al-Gareeb, 2020). At the time of discharge from intensive care units, cytokines levels of COVID-19 patients have normally returned to the physiological range (Rodriguez et al., 2020; Wen et al., 2020). Researchers and health professionals are under pressure to reuse, identify and develop new drugs for this global emergency (Sahebnasagh et al., 2020). Based on the biological effects attributed to carnosine, it is suggested this dipeptide can be considered a palliative therapeutic with respect to COVID-19 and long COVID.
The number of patients recovered from SARS-CoV-2 infection with diseases associated with long COVID is unprecedented and unpredictable (Rando et al., 2021). Long COVID symptoms include physical pain, fatigue, dyspnea, gastrointestinal symptoms, headaches, and memory and psychological disturbances (Mahase, 2020; Mandal et al., 2020; Yelin et al., 2020). These symptoms can evolve over weeks/months following SARS-CoV-2 infection (Mahase, 2020). Whilst some patients report residual COVID-19 symptoms, others may develop new symptoms or new diseases (such as diabetes) long after the initial infection (Cirulli et al., 2020; Korompoki et al., 2021).
Carnosine is a dipeptide composed of β-alanine and L-histidine (Boldyrev et al., 2013). It is expressed in both the central nervous system (CNS) and periphery including skeletal muscle (Nagai and Suda, 1998; Bonfanti et al., 1999; Boldyrev et al., 2013). Carnosine is synthesized by carnosine synthase [EC 6.3.2.11], and its hydrolysis is catalyzed by serum carnosinase (CN1) [EC 3.4.13.20] and/or cytosolic carnosinase (CN2) [EC 3.4.13.3] (Lenney et al., 1982; Lenney, 1990; Teufel et al., 2003). Effective transport of this dipeptide in different cell types occurs via peptide transporter 2 (PepT 2; Xiang et al., 2006; Lopachev et al., 2021).
Recent studies have revealed that carnosine is present in human erythrocytes and that acetyl-carnosine (resistant to serum carnosine attack) is present in human serum, the concentration of each decrease with age (Chaleckis et al., 2016). Other studies have revealed that very low levels of acetyl-carnosine are strongly associated with human frailty (Kameda et al., 2020), and the blood of patient suffering from age-related macular degeneration contained very low amounts of carnosine (Chao de la Barca et al., 2020). So far, several biological functions have been attributed to carnosine, including as an anti-inflammatory (Fresta et al., 2020) and antioxidant (Jain et al., 2020) agent, and as a modulator of mitochondrial metabolism (Macarini et al., 2014; Shen et al., 2014; Macedo et al., 2016). Supported by the myriad of effects reported, carnosine has been suggested to decelerate aging symptoms (Hipkiss and Brownson, 2000), as well as for the treatment of other diseases, including cardiovascular disease (Menon et al., 2021), neurodegenerative diseases (Caruso et al., 2019), and diabetes (Houjeghani et al., 2018). The latter is mainly supported by its hypoglycemic (Barca et al., 2018) and anti-glycation effects (Pepper et al., 2010; Chilukuri et al., 2018). Additionally, carnosine’s ability to partially suppress glycolysis in a variety of cell types from yeast to tumor cells (Renner et al., 2010; Horii et al., 2012; Hipkiss and Gaunitz, 2014) [perhaps by altering mRNA translation (Son et al., 2008)] may also help to explain the dipeptide’s beneficial effects toward SARS-Cov-2 viral infectivity (Hipkiss, 2020). Indeed, such infection usually induces an upregulation of glycolysis in the infected tissue (Bojkova et al., 2020). While carnosine might exert therapeutic activity toward COVID-19 virus infection (Lopachev et al., 2020; Saadah et al., 2020; Feehan et al., 2021), the possibility that the dipeptide could be protective toward long COVID has not been thoroughly examined.
The possible application of carnosine for the treatment of diabetes mellitus has been previously discussed (Hipkiss, 2017). Carnosine supplementation mitigates the elevation of glucose, triglycerides, and TNF-α levels in patients with type-2 diabetes (Houjeghani et al., 2018), and/or in overweight or obese pre-diabetic patients (Liu et al., 2015; de Courten et al., 2016). In this scenario, carnosine was shown to suppress glycolysis in different cell types (Hipkiss, 2011). Carnosine also enhances the clearance of a variety of deleterious aldehydes, such as formaldehyde, methylglyoxal and the glycolytic intermediates dihydroxyacetone phosphate and glyceraldehyde-3-phosphate. All of these reactive aldehydes can modify (glycate) proteins, including mitochondrial proteins (Colzani et al., 2016; Hipkiss et al., 2016; Hipkiss, 2017). Methylglyoxal is responsible for many macromolecular modifications associated with secondary complications of type-2 diabetes, for instance enhanced protein glycation (Hipkiss et al., 2016).
Coronavirus disease-2019 patients with comorbidities are at high risk of death (Piroth et al., 2020). The main complicating conditions include hypertension, cardiovascular disease, obesity, chronic obstructive pulmonary disease, and diabetes (Hendren et al., 2020; Wu and McGoogan, 2020). Diabetes is linked to metabolic and macro/microvascular complications that increase morbidity and mortality in different viral infections (Rasheed et al., 2019). SARS-CoV-2 entry into the target cell is facilitated by the connection from the spike protein to a cellular receptor, attaching the virus to the surface of infected cells (Hoffmann et al., 2020). Cellular receptors known to be involved in SARS-CoV-2 infection are the angiotensin-converting enzyme 2 (ACE2) and the transmembrane serine protease (TMPRSS2 and TMPRSS4; Zhang et al., 2003; Hoffmann et al., 2020). Multiple organs are susceptible to SARS-CoV-2 infection, such as the lungs, digestive tract, kidneys, heart, brain, and pancreas (Gavriatopoulou et al., 2020; Puelles et al., 2020). ACE2 is upregulated in patients with cardiovascular disease, hypertension, and diabetes (Pollard et al., 2020). Diabetic patients are at higher risk for the cytokine storm secondary to the pro-inflammatory state triggered by COVID-19 (Apicella et al., 2020), as well as of diabetic ketoacidosis and mortality (Lim et al., 2020). The cytokine storm also leads to peripheral insulin resistance (Kameda et al., 2020) and disrupts pancreatic β-cells functioning, inhibiting insulin secretion (Mehta et al., 2020). The pancreatic damage and hyperglycemia are further stimulated by the direct invasion of SARS-CoV-2 to the pancreas (Zhang et al., 2003). Taken together, these effects contribute to the development of hyperglycemia in COVID-19 patients (Mehta et al., 2020) and potentially induce type 1 diabetes during the long COVID period (Lim et al., 2020). While we strive to understand how COVID-19 induces diabetes or aggravates the existing disease, it is mandatory to maintain long-term follow-up of these patients.
The term “long COVID” refers to patients with a post-acute COVID-19 (defined as the presence of symptoms 3+ weeks from the onset of symptoms) or chronic COVID-19 (symptoms 12+ weeks; Rubin, 2020; Korompoki et al., 2021). Long-term hyperglycemia in COVID-19 induces oxidative stress, contributing to the development of insulin resistance and dysregulation of pancreatic β-cells (Abdul-Hadi et al., 2020). Many cases of pancreatitis have been reported in COVID-19 patients (Anand et al., 2020; Ghosh et al., 2020; Wang et al., 2020). Pancreatic damage and hyperglycemia may evolve over weeks or months following SARS-CoV-2 infection (Mahase, 2020). In this scenario, reports of late diabetes (and also other complications) in patients recovered from SARS-CoV-2 infection have recently emerged (Mahase, 2020; Morieri et al., 2020; Korompoki et al., 2021).
Carnosine was shown to lower the affinity between ACE2 and the spike protein from SARS-CoV-2 (Saadah et al., 2020). So far, no further actions of carnosine on this mechanism have been reported, nor on the interaction of the virus with host cells, neither on viral lifespan. However, given its antioxidant and anti-inflammatory properties (Fresta et al., 2020; Ooi et al., 2020; Scuto et al., 2020), carnosine may attenuate the cytokine storm in COVID-19 patients. Carnosine could also restrain other COVID-19 complications.
A hypercoagulatory state may contribute to an increased mortality in COVID-19 (Mucha et al., 2020). This dysregulated coagulation can be induced by methylglyoxal, which causes post-synthetic protein glycation in diabetics, including the glycation of the anticoagulants anti-thrombin III (Jacobson et al., 2014) and plasminogen (Gugliucci, 2003). Carnosine can inhibit protein glycation and possibly eliminate methylglyoxal, thereby suppressing the anticoagulant modification induced by this reactive carbonyl (Hipkiss, 2020).
The elderly individuals over 50 years old are particularly susceptible to worse complications (e.g., severe pneumonia) and death following coronavirus infections (Jartti et al., 2011), which is also true for COVID-19 (Daoust, 2020). The immune system is impaired during the aging process, rendering the elderly more susceptible to SARS-CoV-2 infection (Wu and McGoogan, 2020). In this context, carnosine’s putative anti-aging properties delayed senescence, lifespan extension, and rejuvenation of cultured human and rodent cells (McFarland and Holliday, 1994; Gallant et al., 2000; Yuneva et al., 2002; Stvolinsky et al., 2010; Boldyrev et al., 2013; Hipkiss et al., 2016) suggest that the dipeptide could be explored. Cellular aging and cell senescence are associated with telomere shortening (Ishikawa et al., 2016) and increases of transforming growth factor-β (TGF-β) signaling and Smad3 expression (Doyle et al., 2010; Baugé et al., 2013). In cultured human cells, carnosine slows telomeres shortening (Shao et al., 2004) and suppresses TGF-β production and signaling, possibly involving inhibition of the Smad2/3 pathway (Köppel et al., 2011). Carnosine can up-regulate coenzyme Q10 synthesis (Schwank-Xu et al., 2021), thereby stimulating mitochondrial activity and contributing to a less aged cellular physiological state. Since CN1 expression increases throughout development (Lenney et al., 1982), a progressive decline in serum carnosine concentrations is observed in elderly humans (Stuerenburg and Kunze, 1999; Tallon et al., 2007) and a correlation between increased CN1 expression and decreased carnosine levels in specific rat brain regions has been detected (Balion et al., 2007). To date, there is no report indicating alterations on carnosine metabolism enzymes elicited by SARS-CoV-2. The brain is an important organ in the clinical presentation of COVID-19. Long COVID patients may also present long lasting neurological symptoms, including the brain fog, hallucinations, double vision, numbness in their limbs or face, disorientation and difficulty concentrating. These symptoms may persist even after 5 months of SARS-CoV-2 infection (Karuppan et al., 2021). Over the last decades, carnosine has been widely suggested as a contributor to brain health (Hipkiss, 2007; Boldyrev et al., 2013; Hipkiss et al., 2016; Kawahara et al., 2018, 2020; Caruso et al., 2019; Schön et al., 2019).
Dietary administration of carnosine is hampered by the presence of serum carnosinase in humans. Previous suggestions have included the nasal route especially as this could raise carnosine levels in the olfactory bulb and so perhaps alleviate cognitive impairment, anxiety and long COVID-associated brain fog (Ma and Vervoort, 2020). The presence of the carnosine in the airways may also locally suppress infection. There are no reported studies on the possible efficacy of dietary acetyl-carnosine toward almost any age-related condition, with the exception of lenticular cataracts where the direct application of acetyl-carnosine in solution has been proposed as a therapeutic agent (Boldyrev et al., 2013).
The COVID-19 pandemics is a challenging scenario for the global population, researchers, and front-line health professionals (Sahebnasagh et al., 2020). The molecular and cellular bases of COVID-19 are heterogeneous and there is an urge for its complete elucidation. Due to the lack of complete SARS-CoV-2 immunity and of comprehensive vaccination strategy, the unpredictable clinical results of COVID-19 are concerning (Bong et al., 2020; Ma et al., 2020; Ma and Vervoort, 2020). Long COVID is a condition that affects a wide range of patients (Rubin, 2020), which is severely challenging the entire healthcare system. Nevertheless, immunized patients are less likely to develop long COVID compared to the un-immunized (Antonelli et al., 2021). The long-lasting symptomatology can result in chronic morbidity (Shah et al., 2021), including the development of diabetes (Korompoki et al., 2021). The emergence of new COVID-19 variants threatens us with an increase in the proportion of patients suffering from long COVID. Here, we have summarized the available evidence indicating the potential role of carnosine/acetyl-carnosine in ameliorating long-term complications of COVID-19 and diabetes (Figure 1). Whenever possible, the presence of serum acetyl-carnosine and erythrocyte carnosine levels should be measured to determine whether the levels of these peptides could be predictive of morbidity or mortality, and whether raising their levels has any beneficial effects on clinical course and survival. Given the biological effects attributed to carnosine, intranasal adjuvant therapy with this dipeptide could be considered as supportive treatment in patients with either COVID-19 or long COVID.
Figure 1. Potential effects of carnosine/acetyl-carnosine on different organs susceptible to COVID and long COVID complications (created with BioRender.com).
FD and GF contributed to the design, overall collection of information, and writing and final revision of the manuscript. AH was involved in the data collection for diabetes and other long COVID complications, writing and final revision of the manuscript. All authors contributed to the article and approved the submitted version.
Grants from Fundação de Amparo à Pesquisa do Estado do Rio de Janeiro (FAPERJ) and Conselho Nacional de Desenvolvimento Científico e Tecnológico (CNPq) supported this work. FD is recipient of a CNPq Ph.D. fellowship. GF has research fellowships from CNPq and FAPERJ.
The authors declare that the research was conducted in the absence of any commercial or financial relationships that could be construed as a potential conflict of interest.
All claims expressed in this article are solely those of the authors and do not necessarily represent those of their affiliated organizations, or those of the publisher, the editors and the reviewers. Any product that may be evaluated in this article, or claim that may be made by its manufacturer, is not guaranteed or endorsed by the publisher.
Abdul-Hadi, M. H., Naji, M. T., Shams, H. A., Sami, O. M., Al-Harchan, N. A., Al-, Kuraishy, H. M., et al. (2020). Oxidative stress injury and glucolipotoxicity in type 2 diabetes mellitus: the potential role of metformin and sitagliptin. Biomed. Biotechnol. Res. J. 4:166. doi: 10.4103/bbrj.bbrj_7_20
Al-Kuraishy, H. M., and Al-Gareeb, A. I. (2020). From SARS-CoV to nCoV-2019: ruction and argument. Arch. Clin. Infect. Dis. 15:e102624. doi: 10.5812/archcid.10262
Anand, E. R., Major, C., Pickering, O., and Nelson, M. (2020). Acute pancreatitis in a COVID-19 patient. Br. J. Surg. 107;e182. doi: 10.1002/bjs.11657
Antonelli, M., Penfold, R. S., Merino, J., Sudre, C. H., Molteni, E., Berry, S., et al. (2021). Risk factors and disease profile of post-vaccination SARS-CoV-2 infection in UK users of the COVID Symptom Study app: a prospective, community-based, nested, case-control study. Lancet Infect. Dis. 22, 43–55. doi: 10.1016/S1473-3099(21)00460-6
Apicella, M., Campopiano, M. C., Mantuano, M., Mazoni, L., Coppelli, A., and Del Prato, S. (2020). COVID-19 in people with diabetes: understanding the reasons for worse outcomes. Lancet Diabetes Endocrinol. 8, 782–792. doi: 10.1016/S2213-8587(20)30238-2
Balion, C. M., Benson, C., Raina, P. S., Papaioannou, A., Patterson, C., and Ismaila, A. S. (2007). Brain type carnosinase in dementia: a pilot study. BMC Neurol. 7:38. doi: 10.1186/1471-2377-7-38
Barca, A., Gatti, F., Spagnolo, D., Ippati, S., Vetrugno, C., and Verri, T. (2018). Responsiveness of carnosine homeostasis genes in the pancreas and brain of streptozotocin-treated mice exposed to dietary carnosine. Int. J. Mol. Sci. 19:1713. doi: 10.3390/ijms19061713
Baugé, C., Duval, E., Ollitrault, D., Girard, N., Leclercq, S., Galéra, P., et al. (2013). Type II TGFβ receptor modulates chondrocyte phenotype. Age 35, 1105–1116. doi: 10.1007/s11357-012-9433-7
Bedford, J., Enria, D., Giesecke, J., Heymann, D. L., Ihekweazu, C., Kobinger, G., et al. (2020). COVID-19: towards controlling of a pandemic. Lancet 395, 1015–1018.
Bojkova, D., Klann, K., Koch, B., Widera, M., Krause, D., Ciesek, S., et al. (2020). Proteomics of SARS-CoV-2-infected host cells reveals therapy targets. Nature 583, 469–472. doi: 10.1038/s41586-020-2332-7
Boldyrev, A. A., Aldini, G., and Derave, W. (2013). Physiology and pathophysiology of carnosine. Physiol. Rev. 93, 1803–1845. doi: 10.1152/physrev.00039.2012
Bonfanti, L., Peretto, P., De Marchis, S., and Fasolo, A. (1999). Carnosine-related dipeptides in the mammalian brain. Prog. Neurobiol. 59, 333–353. doi: 10.1016/S0301-0082(99)00010-6
Bong, C. L., Brasher, C., Chikumba, E., McDougall, R., Mellin-Olsen, J., and Enright, A. (2020). The COVID-19 pandemic: effects on low- and middle-income countries. Anesth. Analg 131, 86–92. doi: 10.1213/ANE.0000000000004846
Caruso, G., Fresta, C. G., Musso, N., Giambirtone, M., Grasso, M., Spampinato, S. F., et al. (2019). Carnosine prevents Aβ-induced oxidative stress and inflammation in microglial cells: a key role of TGF-β1. Cells 8:64. doi: 10.3390/cells8010064
Chaleckis, R., Murakami, I., Takada, J., Kondoh, H., and Yanagida, M. (2016). Individual variability in human blood metabolites identifies age-related differences. Proc. Natl. Acad. Sci.U.S.A. 113, 4252–4259. doi: 10.1073/pnas.1603023113
Chao de la Barca, J. M., Rondet-Courbis, B., Ferré, M., Muller, J., Buisset, A., Leruez, S., et al. (2020). A plasma metabolomic profiling of exudative age-related macular degeneration showing carnosine and mitochondrial deficiencies. J. Clin. Med. 9:631. doi: 10.3390/jcm9030631
Chilukuri, H., Kulkarni, M. J., and Fernandes, M. (2018). Revisiting amino acids and peptides as anti-glycation agents. Medchemcomm 9, 614–624. doi: 10.1039/c7md00514h
Cirulli, E. T., Barrett, K. M. S., Riffle, S., Bolze, A., Neveux, I., Dabe, S., et al. (2020). Long-term COVID-19 symptoms in a large unselected population. medRxiv [Preprint] doi: 10.1101/2020.10.07.20208702
Colzani, M., De Maddis, D., Casali, G., Carini, M., Vistoli, G., Aldini, G., et al. (2016). Reactivity, selectivity, and reaction mechanisms of aminoguanidine, hydralazine, pyridoxamine, and carnosine as sequestering agents of reactive carbonyl species: a comparative study. Chem. Med. Chem. 11, 1778–1789. doi: 10.1002/cmdc.201500552
Daoust, J. F. (2020). Elderly people and responses to COVID-19 in 27 Countries. PLoS One 15:e0235590. doi: 10.1371/journal.pone.0235590
de Courten, B., Jakubova, M., de Courten, M. P., Kukurova, I. J., Vallova, S., Krumpolec, P., et al. (2016). Effects of carnosine supplementation on glucose metabolism: pilot clinical trial. Obesity 24, 1027–1034. doi: 10.1002/oby.21434
Doyle, K. P., Cekanaviciute, E., Mamer, L. E., and Buckwalter, M. S. T. G. F. (2010). β signaling in the brain increases with aging and signals to astrocytes and innate immune cells in the weeks after stroke. J. Neuroinflammation 7:62. doi: 10.1186/1742-2094-7-62
Feehan, J., de Courten, M., Apostolopoulos, V., and de Courten, B. (2021). Nutritional interventions for COVID-19: a role for carnosine? Nutrients 13:1463. doi: 10.3390/nu13051463
Fresta, C. G., Fidilio, A., Lazzarino, G., Musso, N., Grasso, M., Merlo, S., et al. (2020). Modulation of pro-oxidant and pro-inflammatory activities of M1 macrophages by the natural dipeptide carnosine. Int. J. Mol. Sci. 21:776. doi: 10.3390/ijms21030776
Gallant, S., Semyonova, M., and Yuneva, M. (2000). Carnosine as a potential anti-senescence drug. Biochemistry 65, 866–868.
Gavriatopoulou, M., Korompoki, E., Fotiou, D., Ntanasis-Stathopoulos, I., Psaltopoulou, T., Kastritis, E., et al. (2020). Organ-specific manifestations of COVID-19 infection. Clin. Exp. Med. 20, 493–506. doi: 10.1007/s10238-020-00648-x
Ghosh, A., Gupta, V., and Misra, A. (2020). COVID19 induced acute pancreatitis and pancreatic necrosis in a patient with type 2 diabetes. Diabetes Metab. Syndr. Clin. Res. Rev. 14, 2097–2098. doi: 10.1016/j.dsx.2020.10.008
Gugliucci, A. (2003). A practical method to study functional impairment of proteins by glycation and effects of inhibitors using current coagulation/fibrinolysis reagent kits. Clin. Biochem. 36, 155–158. doi: 10.1016/s0009-9120(02)00442-3
Hendren, N. S., Drazner, M. H., Bozkurt, B., and Cooper, L. (2020). Description and proposed management of the acute COVID-19 cardiovascular syndrome. Circulation 141, 1903–1914. doi: 10.1161/CIRCULATIONAHA.120.047349
Hipkiss, A. R. (2007). Could carnosine or related structures suppress Alzheimer’s disease? J. Alzheimers Dis. 11, 229–240. doi: 10.3233/jad-2007-11210
Hipkiss, A. R. (2011). Energy metabolism, proteotoxic stress and age-related dysfunction – protection by carnosine. Mol. Aspects Med. 32, 267–278. doi: 10.1016/j.mam.2011.10.004
Hipkiss, A. R. (2017). Depression, diabetes and dementia: formaldehyde may be a common causal agent; could carnosine, a pluripotent peptide, be protective? Aging Dis. 8, 128–130. doi: 10.14336/AD.2017.0120
Hipkiss, A. R. (2020). COVID-19 and senotherapeutics: any role for the naturally occurring dipeptide carnosine? Aging Dis. 11, 737–741. doi: 10.14336/AD.2020.0518
Hipkiss, A. R., and Brownson, C. (2000). A possible new role for the anti-ageing peptide carnosine. Cell Mol. Life Sci. 57, 747–753. doi: 10.1007/s000180050039
Hipkiss, A. R., and Gaunitz, F. (2014). Inhibition of tumour cell growth by carnosine: some possible mechanisms. Amino Acids 46, 327–337. doi: 10.1007/s00726-013-1627-5
Hipkiss, A. R., Baye, E., and de Courten, B. (2016). Carnosine and the processes of ageing. Maturitas 93, 28–33. doi: 10.1016/j.maturitas.2016.06.002
Hoffmann, M., Kleine-Weber, H., Schroeder, S., Krüger, N., Herrler, T., Erichsen, S., et al. (2020). SARS-CoV-2 cell entry depends on ACE2 and TMPRSS2 and is blocked by a clinically proven protease inhibitor. Cell 181, 271–280. doi: 10.1016/j.cell.2020.02.052
Horii, Y., Shen, J., Fujisaki, Y., Yoshida, K., and Nagai, K. (2012). Effects of L-carnosine on splenic sympathetic nerve activity and tumor proliferation. Neurosci. Lett. 510, 1–5. doi: 10.1016/j.neulet.2011.12.058
Houjeghani, S., Kheirouri, S., Faraji, E., and Jafarabadi, M. A. L. - (2018). Carnosine supplementation attenuated fasting glucose, triglycerides, advanced glycation end products, and tumor necrosis factor-α levels in patients with type 2 diabetes: a double-blind placebo-controlled randomized clinical trial. Nutr. Res. 49, 96–106. doi: 10.1016/j.nutres.2017.11.003
Huang, C., Wang, Y., Li, X., Ren, L., Zhao, J., Hu, Y., et al. (2020). Clinical features of patients infected with 2019 novel coronavirus in Wuhan, China. Lancet 395, 497–506. doi: 10.1016/S0140-6736(20)30183-5
Ishikawa, N., Nakamura, K., Izumiyama-Shimomura, N., Aida, J., Matsuda, Y., Arai, T., et al. (2016). Changes of telomere status with aging: an update. Geriatr. Gerontol. Int. 1, 30–42. doi: 10.1111/ggi.12772
Jacobson, R., Mignemi, N., Rose, K., O’Rear, L., Sarilla, S., Hamm, H. E., et al. (2014). The hyperglycemic byproduct methylglyoxal impairs anticoagulant activity through covalent adduction of antithrombin III. Thromb Res. 134, 1350–1357. doi: 10.1016/j.thromres.2014.09.038
Jain, S., Kim, E. S., Kim, D., Burrows, D., Felice, M., Kim, M., et al. (2020). Comparative cerebroprotective potential of d- and l-carnosine following ischemic stroke in mice. Int. J. Mol. Sci. 21:3053. doi: 10.3390/ijms21093053
Jartti, L., Langen, H., Söderlund-Venermo, M., Vuorinen, T., Ruuskanen, O., and Jartti, T. (2011). New respiratory viruses and the elderly. Open Respir. Med. J. 5, 61–69. doi: 10.2174/1874306401105010061
Kameda, M., Teruya, T., Yanagida, M., and Kondoh, H. (2020). Frailty markers comprise blood metabolites involved in antioxidation, cognition, and mobility. Proc. Natl. Acad. Sci. 117, 9483–9489. doi: 10.1073/pnas.1920795117
Karuppan, M. K. M., Devadoss, D., Nair, M., Cand, H. S., and Lakshmana, M. K. (2021). SARS-CoV-2 infection in the central and peripheral nervous system-associated morbidities and their potential mechanism. Mol. Neurobiol. 58, 2465–2480. doi: 10.1007/s12035-020-02245-1
Kawahara, M., Sadakane, Y., Mizuno, K., Kato-Negishi, M., and Tanaka, K. I. (2020). Carnosine as a possible drug for zinc-induced neurotoxicity and vascular dementia. Int. J. Mol. Sci. 21:2570. doi: 10.3390/ijms21072570
Kawahara, M., Tanaka, K. I., and Kato-Negishi, M. (2018). Zinc, carnosine, and neurodegenerative diseases. Nutrients 10:147. doi: 10.3390/nu10020147
Köppel, H., Riedl, E., Braunagel, M., Sauerhoefer, S., Ehnert, S., Godoy, P., et al. (2011). L-carnosine inhibits high-glucose-mediated matrix accumulation in human mesangial cells by interfering with TGF-β production and signalling. Nephrol. Dial Transplant. 26, 3852–3858. doi: 10.1093/ndt/gfr324
Korompoki, E., Gavriatopoulou, M., Hicklen, R. S., Ntanasis-Stathopoulos, I., Kastritis, E., Fotiou, D., et al. (2021). Epidemiology and organ specific sequelae of post-acute COVID19: a narrative review. J. Infect. 83, 1–16. doi: 10.1016/j.jinf.2021.05.004
Lenney, J. F. (1990). Separation and characterization of two carnosine-splitting cytosolic dipeptidases from hog kidney (carnosinase and non-specific dipeptidase). Biol. Chem. Hoppe Seyler. 371, 433–440. doi: 10.1515/bchm3.1990.371.1.433
Lenney, J. F., George, R. P., Weiss, A. M., Kucera, C. M., Chan, P. W., and Rinzler, G. S. (1982). Human ando carnosinase: characterization, distinction from cellular carnosinase, and activation by cadmium. Clin. Chim. Acta 123, 221–231. doi: 10.1016/0009-8981(82)90166-8
Lim, S., Bae, J. H., Kwon, H. S., and Nauck, M. A. (2020). COVID-19 and diabetes mellitus: from pathophysiology to clinical management. Nat. Rev. Endocrinol. 13, 1–20. doi: 10.1038/s41574-020-00435-4
Liu, Y., Cotillard, A., Vatier, C., Bastard, J. P., Fellahi, S., Stévant, M., et al. (2015). A dietary supplement containing cinnamon, chromium and carnosine decreases fasting plasma glucose and increases lean mass in overweight or obese pre-diabetic subjects: a randomized, placebo-controlled trial. PLoS One 10:e0138646. doi: 10.1371/journal.pone.0138646
Lopachev, A. V., Abaimov, D. A., Filimonov, I. S., Kulichenkova, K. N., and Fedorova, T. N. (2021). An assessment of the transport mechanism and intraneuronal stability of L-carnosine. Amino Acids 51:5. doi: 10.1007/s00726-021-03094-5
Lopachev, A. V., Kazanskaya, R. B., Khutorova, A. V., and Fedorova, T. N. (2020). An overview of the pathogenic mechanisms involved in severe cases of COVID-19 infection, and the proposal of salicyl-carnosine as a potential drug for its treatment. Eur. J. Pharmacol. 886:173457. doi: 10.1016/j.ejphar.2020.173457
Ma, X., and Vervoort, D. (2020). Critical care capacity during the COVID-19 pandemic: global availability of intensive care beds. J. Crit. Care 58, 96–97. doi: 10.1016/j.jcrc.2020.04.012
Ma, X., Vervoort, D., Reddy, C. L., Park, K. B., and Makasa, E. (2020). Emergency and essential surgical healthcare services during COVID-19 in low- and middle-income countries: a perspective. Int. J. Surg. 79, 43–46. doi: 10.1016/j.ijsu.2020.05.037
Macarini, J. R., Maravai, S. G., Cararo, J. H., Dimer, N. W., Gonçalves, C. L., Kist, L. W., et al. (2014). Impairment of electron transfer chain induced by acute carnosine administration in skeletal muscle of young rats. Biomed. Res. Int. 2014:632986. doi: 10.1155/2014/632986
Macedo, L. W., Cararo, J. H., Maravai, S. G., Gonçalves, C. L., Oliveira, G. M., Kist, L. W., et al. (2016). Carnosine administration increases respiratory chain complexes and citric acid cycle enzyme activities in cerebral cortex of young rats. Mol. Neurobiol. 53, 5582–5590. doi: 10.1007/s12035-015-9475-9
Mahase, E. (2020). Covid-19: what do we know about “long Covid”? BMJ 14:m2815. doi: 10.1136/bmj.m2815
Mandal, S., Barnett, J., Brill, S. E., Brown, J. S., Denneny, E. K., Hare, S. S., et al. (2020). ‘Long-COVID’: a cross-sectional study of persisting symptoms, biomarker and imaging abnormalities following andomized tion for COVID-19. Thorax 76, 396–398. doi: 10.1136/thoraxjnl-2020-215818
McFarland, G. A., and Holliday, R. (1994). Retardation of the senescence of cultured human diploid fibroblasts by carnosine. Exp. Cell Res. 212, 167–175. doi: 10.1006/excr.1994.1132
Mehta, P., McAuley, D. F., Brown, M., Sanchez, E., Tattersall, R. S., and Manson, J. J. (2020). COVID-19: consider cytokine storm syndromes and immunosuppression. Lancet 395, 1033–1034. doi: 10.1016/S0140-6736(20)30628-0
Menon, K., Cameron, J. D., Courten, M., and Courten, B. (2021). Use of carnosine in the prevention of cardiometabolic risk factors in overweight and obese individuals: study protocol for a andomized, double-blind placebo-controlled trial. BMJ 11:e043680. doi: 10.1136/bmjopen-2020-043680
Morieri, M. L., Fadini, G. P., Boscari, F., Fioretto, P., Maran, A., Busetto, L., et al. (2020). Hyperglycemia, glucocorticoid therapy, and outcome of COVID-19. Diabetes Res. Clin. Pract. 168:108449. doi: 10.1016/j.diabres.2020.108449
Mucha, S. R., Dugar, S., McCrae, K., Joseph, D. E., Bartholomew, J., Sacha, G., et al. (2020). Coagulopathy in COVID-19. Cleve Clin. J. Med. 87, 461–468. doi: 10.3949/ccjm.87a.ccc024
Nagai, K., and Suda, T. (1998). Realization of spontaneous healing function by carnosina. Methods Find Exp. Clin. Pharmacol. 10, 497–507.
Ooi, T. C., Chan, K. M., and Sharif, R. (2020). Zinc L-Carnosine Protects CCD-18co Cells from L-Buthionine Sulfoximine-Induced Oxidative Stress via the Induction of Metallothionein and Superoxide Dismutase 1 Expression. Biol. Trace Elem. Res. 198, 464–471. doi: 10.1007/s12011-020-02108-9
Pepper, E. D., Farrell, M. J., Nord, G., and Finkel, S. E. (2010). Antiglycation effects of carnosine and other compounds on the long-term survival of Escherichia coli. Appl. Environ. Microbiol. 76, 7925–7930. doi: 10.1128/AEM.01369-10
Piroth, L., Cottenet, J., Mariet, A. S., Bonniaud, P., Blot, M., Tubert-Bitter, P., et al. (2020). Comparison of the characteristics, morbidity, and mortality of COVID-19 and seasonal influenza: a nationwide, population-based retrospective cohort study. Lancet Respir. Med. 9, 251–259. doi: 10.1016/S2213-2600(20)30527-0
Pollard, C. A., Morran, M. P., and Nestor-Kalinoski, A. L. (2020). The COVID-19 pandemic: a global health crisis. Physiol. Genom. 52, 549–557. doi: 10.1152/physiolgenomics.00089.2020
Puelles, V. G., Lütgehetmann, M., Lindenmeyer, M. T., Sperhake, J. P., Wong, M. N., Allweiss, L., et al. (2020). Multiorgan and renal tropism of SARSCoV-2. N. Engl. J. Med. 383, 590–592. doi: 10.1056/NEJMc2011400
Rando, H. M., Bennett, T. D., Byrd, J. B., Bramante, C., Callahan, T. J., Chute, C. G., et al. (2021). Challenges in defining Long COVID: striking differences across literature, electronic health records, and patient-reported information. medRxiv [Preprint] 26:2021. doi: 10.1101/2021.03.20.21253896
Rasheed, H. A., Al-Kuraishy, H. M., Al-Gareeb, A. I., Hussien, N. R., and Al-Nami, M. S. (2019). Effects of diabetic pharmacotherapy on prolactin hormone in patients with type 2 diabetes mellitus: bane or Boon. J. Adv. Pharmaceut. Technol. Res. 10, 163–168. doi: 10.4103/japtr.JAPTR_65_19
Renner, C., Asperger, A., Seyffarth, A., Meixensberger, J., Gebhardt, R., and Gaunitz, F. (2010). Carnosine inhibits ATP production in cells from malignant glioma. Neurol. Res. 32, 101–105. doi: 10.1179/016164109X12518779082237
Rodriguez, L., Pekkarinen, P. T., Lakshmikanth, T., Tan, Z., Consiglio, C. R., Pou, C., et al. (2020). Systems-level immunomonitoring from acute to recovery phase of severe COVID-19. Cell Rep. Med. 1:100078. doi: 10.1016/j.xcrm.2020.100078
Rubin, R. (2020). As their numbers grow, COVID-19 “Long Haulers” stump experts. JAMA 324, 1381–1383. doi: 10.1001/jama.2020.17709
Saadah, L. M., Deiab, G. I. A., Al-Balas, Q., and Basheti, I. A. (2020). Carnosine to Combat Novel Coronavirus (nCoV): molecular Docking and Modeling to Cocrystallized Host Angiotensin-Converting Enzyme 2 (ACE2) and Viral Spike Protein. Molecules 25:5605. doi: 10.3390/molecules25235605
Sahebnasagh, A., Avan, R., Saghafi, F., Mojtahedzadeh, M., Sadremomtaz, A., Arasteh, O., et al. (2020). Pharmacological treatments of COVID-19. Pharmacol. Rep. 20, 1–33. doi: 10.1007/s43440-020-00152-9
Schön, M., Mousa, A., Berk, M., Chia, W. L., Ukropec, J., Majid, A., et al. (2019). The potential of carnosine in brain-related disorders: a comprehensive review of current evidence. Nutrients 11:1196. doi: 10.3390/nu11061196
Schwank-Xu, C., Forsberg, E., Bentinger, M., Zhao, A., Ansurudeen, I., Dallner, G., et al. (2021). L-carnosine stimulation of coenzyme Q10 biosynthesis promotes improved mitochondrial function and decreases hepatic steatosis in diabetic conditions. Antioxidants 10:793. doi: 10.3390/antiox10050793
Scuto, M., Trovato Salinaro, A., Modafferi, S., Polimeni, A., Pfeffer, T., Weigand, T., et al. (2020). Carnosine activates cellular stress response in podocytes and reduces glycative and lipoperoxidative stress. Biomedicines 8:177. doi: 10.3390/biomedicines8060177
Shah, W., Hillman, T., Playford, E. D., and Hishmeh, L. (2021). Managing the long term effects of covid-19: summary of NICE, SIGN, and RCGP rapid guideline. BMJ 372:n136. doi: 10.1136/bmj.n136
Shao, L., Li, Q. H., and Tan, Z. (2004). L-carnosine. Biochem. Biophys. Res. Commun. 324, 931–936. doi: 10.1016/j.bbrc.2004.09.136
Shen, Y., Tian, Y., Yang, J., Shi, X., Ouyang, L., Gao, J., et al. (2014). Dual effects of carnosine on energy metabolism of cultured cortical astrocytes under normal and ischemic conditions. Regul. Peptides 193, 45–52. doi: 10.1016/j.regpep.2014.08.005
Son, D. O., Satsu, H., Kiso, Y., Totsuka, M., and Shimizu, M. (2008). Inhibitory effect of carnosine on interleukin-8 production in intestinal epithelial cells through translational regulation. Cytokine 42, 265–276. doi: 10.1016/j.cyto.2008.02.011
Stuerenburg, H. J., and Kunze, K. (1999). Concentrations of free carnosine (a putative membrane-protective antioxidant) in human muscle biopsies and rat muscles. Arch. Gerontol. Geriatr. 29, 107–113. doi: 10.1016/s0167-4943(99)00020-5
Stvolinsky, S., Antipin, M., Meguro, K., Sato, T., Abe, H., and Boldyrev, A. A. (2010). Effect of carnosine and its Trolox-modified derivatives on life span of Drosophila melanogaster. Rejuvenation Res. 13, 453–457. doi: 10.1089/rej.2009.1010
Tallon, M. J., Harris, R. C., Maffulli, N., and Tarnopolsky, M. A. (2007). Carnosine, taurine and enzyme activities of human skeletal muscle fibres from elderly subjects with osteoarthritis and young moderately active subjects. Biogerontology 8, 129–137. doi: 10.1007/s10522-006-9038-6
Teufel, M., Saudek, V., Ledig, J. P., Bernhardt, A., Boularand, S., Carreau, A., et al. (2003). Sequence identification and characterization of human carnosinase and a closely related non-specific dipeptidase. J. Biol. Chem. 278, 6521–6531. doi: 10.1074/jbc.M209764200
Wang, F., Wang, H., Fan, J., Zhang, Y., Wang, H., and Zhao, Q. (2020). Pancreatic injury patterns in patients with coronavirus disease 19 pneumonia. Gastroenterology 159, 367–370. doi: 10.1053/j.gastro.2020.03.055
Wen, W., Su, W., Tang, H., Le, W., Zhang, X., Liu, X., et al. (2020). Immune cell profling of COVID-19 patients in the recovery stage by single-cell sequencing. Cell Discov. 6:31. doi: 10.1038/s41421-020-0168-9
Wiersinga, W. J., Rhodes, A., Cheng, A. C., Peacock, S. J., and Prescott, H. C. (2020). Pathophysiology, transmission, diagnosis, and treatment of coronavirus disease 2019 (COVID-19): a review. JAMA 324, 782–793. doi: 10.1001/jama.2020.12839
Wu, Z., and McGoogan, J. M. (2020). Characteristics of and important lessons from the coronavirus disease 2019 (COVID-19) outbreak in China: summary of a report of 72314 cases from the Chinese Center for Disease Control and Prevention. JAMA 323, 1239–1242. doi: 10.1001/jama.2020.2648
Xiang, J., Hu, Y., Smith, D. E., and Keep, R. F. (2006). PEPT2-mediated transport of 5-aminolevulinic acid and carnosine in astrocytes. Brain Res. 1122, 18–23. doi: 10.1016/j.brainres.2006.09.013
Ye, Y., Lagniton, P. N. P., Ye, S., Li, E., and Xu, R. H. (2020). COVID-19: what has been learned and to be learned about the novel coronavirus disease. Int. J. Biol. Sci. 16, 1753–1766. doi: 10.7150/ijbs.45134
Yelin, D., Margalit, I., Yahav, D., Runold, M., and Bruchfeld, J. (2020). Long COVID-19-it’s not over until? Clin. Microbiol. Infect. 27, 506–508. doi: 10.1016/j.cmi.2020.12.001
Yuneva, A. O., Kramarenko, G. G., Vetreshchak, T. V., Gallant, S., and Boldyrev, A. A. (2002). Effect of carnosine on Drosophila melanogaster lifespan. Bull. Exp. Biol. Med. 133, 559–561. doi: 10.1023/a:1020273506970
Zhang, Q. L., Ding, Y. Q., Hou, J. L., He, L., Huang, Z. X., Wang, H. J., et al. (2003). Detection of severe acute respiratory syndrome (SARS)-associated coronavirus RNA in autopsy tissues with in situ hybridization. Acad. J. First Med. Coll. PLA 23, 1125–1127.
Keywords: carnosine, diabetes, long COVID, SARS-CoV-2 infection, anti-glycating agent
Citation: Diniz FC, Hipkiss AR and Ferreira GC (2022) The Potential Use of Carnosine in Diabetes and Other Afflictions Reported in Long COVID Patients. Front. Neurosci. 16:898735. doi: 10.3389/fnins.2022.898735
Received: 17 March 2022; Accepted: 18 May 2022;
Published: 22 June 2022.
Edited by:
Alexandra Latini, Federal University of Santa Catarina, BrazilReviewed by:
Carlos Henrique Xavier, Federal University of Goiás, BrazilCopyright © 2022 Diniz, Hipkiss and Ferreira. This is an open-access article distributed under the terms of the Creative Commons Attribution License (CC BY). The use, distribution or reproduction in other forums is permitted, provided the original author(s) and the copyright owner(s) are credited and that the original publication in this journal is cited, in accordance with accepted academic practice. No use, distribution or reproduction is permitted which does not comply with these terms.
*Correspondence: Gustavo Costa Ferreira, Z3VzdGF2by5mZXJyZWlyYUBiaW9xbWVkLnVmcmouYnI=
Disclaimer: All claims expressed in this article are solely those of the authors and do not necessarily represent those of their affiliated organizations, or those of the publisher, the editors and the reviewers. Any product that may be evaluated in this article or claim that may be made by its manufacturer is not guaranteed or endorsed by the publisher.
Research integrity at Frontiers
Learn more about the work of our research integrity team to safeguard the quality of each article we publish.