- 1Department of Aerospace Medicine, Fourth Military Medical University, Xi’an, China
- 2Department of Biomedical Engineering, Fourth Military Medical University, Xi’an, China
- 3Institute of Technical Medicine, Furtwangen University, Villingen-Schwenningen, Germany
- 4Air Force Medical Center, Fourth Military Medical University, Beijing, China
Low back pain (LBP) is known to pose a serious threat to helicopter pilots. This study aimed to explore the potential of electrical bio-impedance (EBI) technique with the advantages of no radiation, non-invasiveness and low cost, which is intended to be used as a daily detection tool to assess LBP in primary aviation medical units. The LBP scales (severity) in 72 helicopter pilots were assessed using a pain questionnaire, while the bilateral impedance measurements of the lumbar muscle were carried out with a high precision EBI measurement system. Results showed that the modulus of lumbar muscle impedance increased with LBP scale whereas the phase angle decreased. For different LBP scales, significant differences were found in the modulus of lumbar muscle impedance sum on both sides (Zsum), as well as in the modulus and phase angle of lumbar muscle impedance difference between both sides (Zdiff and ϕdiff), respectively (P < 0.05). Moreover, Spearman’s correlation analysis manifested a strong correlation between Zsum and LBP scale (R = 0.692, P < 0.01), an excellent correlation between Zdiff and LBP scale (R = 0.86, P < 0.01), and a desirable correlation between ϕdiff and LBP scale (R = −0.858, P < 0.01). In addition, receiver operator characteristic analysis showed that for LBP prediction, the area under receiver operator characteristic curve of Zsum, Zdiff, and ϕdiff were 0.931, 0.992, and 0.965, respectively. These findings demonstrated that EBI could sensitively and accurately detect the state of lumbar muscle associated with LBP, which might be the potential tool for daily detection of LBP in primary aviation medical units.
Introduction
Low back pain (LBP) is a recurrent or sometimes persistent disorder defined as the pain localized between the 12th rib and the inferior gluteal folds, which has been one of the most common musculoskeletal diseases worldwide (Krismer and van Tulder, 2007; Wu et al., 2020). For helicopter pilots, due to prolonged exposure to whole-body vibration of high-intensity and confined sitting posture, they complain about LBP frequently (Posch et al., 2019; da Silva, 2020). LBP poses a serious threat to helicopter pilots because it can impair the operational capabilities closely related to flight safety, such as attention, motion control and postural stability (Harrison et al., 2009; Gaydos, 2012). To prevent LBP and alleviate its adverse effects, a comprehensive set of solutions, including flight-specific exercises, the use of lumbar support as well ergonomic cockpit and seat, have been applied for helicopter pilots (Gaydos, 2012; Murray et al., 2015; Andersen et al., 2017). Unfortunately, the incidence of LBP among helicopter pilots has remained high as a result of inevitable vibration and poor sitting posture. A recent epidemiological survey reported that the 3-month and 12-month prevalence of LBP for military helicopter pilots was as high as 42.3 and 48.1% (Posch et al., 2019), respectively. Meanwhile, aviation medicine research showed that early detection of LBP and timely implementation of medical intervention prior to the observation of apparent symptoms, such as physiotherapy and neuromuscular electrical stimulation, could particularly be beneficial to the health and well-being of pilots (Palmer and Bovenzi, 2015; Alrwaily, 2017; Marins et al., 2020). Additionally, objective assessment of LBP severity is helpful for implementing appropriate and specific treatment. Therefore, objective detection and evaluation of LBF is of critical importance to minimize the influences of LBP on helicopter pilots’ health and their flight performance.
Numerous studies have shown that the lumbar muscle dysfunction including the loss of strength and endurance of muscle is considered predictive for the development of LBP. The existing evaluation methods in clinical practice involves surface electromyogram (sEMG) and functional imaging. The parameters in frequency and time domain of sEMG signal can reflect the lumbar muscle function (Villafane et al., 2016; Rose-Dulcina et al., 2020; Arvanitidis et al., 2021), but the sEMG characteristics of the lumbar muscle associated with LBP have not been systematically clarified (Wang et al., 2019). Although functional imaging such as X-ray, CT and MRI can accurately diagnose the obvious changes in muscle structure and state, these imaging modalities might be not suitable as the daily detection tool in primary medical units to evaluate LBF for consideration of radiation and cost of equipment (Ching et al., 2013). Additionally, some novel methods such as sonomyography and acoustic myography can reflect the muscle pain by describing the muscle structure and morphology, but their clinical effectiveness needs to be further verified (Huang et al., 2007; Harrison, 2018; Zhang et al., 2022). Moreover, although LBP can be assessed by subjective evaluation, this practice may not reflect the true state of lumbar muscle because there exist differences in pain tolerance among people. Thus, it is still necessary to explore a simple and effective method for daily LBP detection in primary medical units.
Electrical bio-impedance (EBI) is a relatively new technique that can provide information on physiological and pathological states of human tissues (Abe et al., 2021; Al-harosh et al., 2022). In EBI, a small amount of electrical current is injected into the targeted area of the body through surface electrodes and simultaneously the resulting voltage across the internal tissues are measured to calculate the electrical impedance of the tissue. With the unique advantages of no radiation, non-invasiveness, easy operation and portability, EBI has been widely employed in biomedical applications, especially in the assessment of the state of human tissues, such as brain edema and inflammation (Lingwood et al., 2003; Mortreux et al., 2019; Dai et al., 2020; Al-harosh et al., 2022). Moreover, previous studies have shown that the impedance characteristics of muscle is highly related to muscle state, and thus in theory, EBI has potential to detect LBP of helicopter pilots by assessing the state of lumbar muscle (Ching et al., 2013; Wang et al., 2019).
To date, several studies have explored the ability of EBI to detect LBP. Wang et al. (2019) found that impedance phase (at 100 kHz) of lumbosacral paraspinal muscle in LBP patients was lower than that in healthy individuals. They also observed significantly increased difference in impedance between both sides of the spine in LBP patients. Ching et al. (2013) found significant differences in impedance properties of lumbar paraspinal muscles between acute LBF patients and healthy subjects. Fujimoto et al. (2019) compared the measurements of dual-energy X-ray absorptiometry (DXA) and bio-impedance analysis among LBP patients and concluded that EBI and DXA were significantly correlated in both female and male patients. Other studies also reported the difference in electrical impedance between LBP patients and healthy individuals (Fujimoto et al., 2018; Rutkove et al., 2019). These previous studies demonstrated the high sensitivity of EBI to lumbar muscle dysfunction caused by LBP; however, these results seemed insufficient to support direct use of EBI to detect LBP in helicopter pilots. First, the subjects studied in previous literature were patients, rather than particular group of helicopter pilots. Second, previous studies did not perform any quantitative pain rating for LBP so that no relationship between the severity of LBP and muscle impedance could be established.
In this study, the severity (scale) of LBP in 72 helicopter pilots was determined and impedance measurements of the lumbar muscle were carried out. Next, the impedance characteristics of lumbar muscle with different LBP scales were compared. Finally, the correlation between muscle impedance and LBP scales was established to explore the feasibility of using EBI to evaluate the lumbar muscle dysfunction caused by LBP.
Materials and Methods
Participants and Ethics Statement
A total of 92 Chinese male helicopter pilots were invited to participate in the present study. All invited participants were informed of the study protocol. Finally, 72 pilots accepted the invitation and were enrolled in this study. Inclusion criteria were: (1) maintaining operational flight status at enrollment, (2) having the flying experience of more than one year, and (3) having no history of spinal fractures or surgery. Exclusion criterion was: receiving the treatment or intervention for LBP prior to the study.
All participants signed the written consent form prior to the study. This study was approved by the Ethics Committee of Fourth Military Medical University (No.KY20163064-1) and carried out in accordance with relevant regulations and guidelines.
Questionnaire and Implementation
The Chinese version of Oswestry Disability Index (ODI), one of the most commonly used patient-reported outcome measures for individuals with LBP in clinical setting (Chow and Chan, 2005), was adopted to evaluate the severity of LBP for each pilot. ODI consists of ten sections to assess the extent of LBP according to various activities of daily life, including personal care, lifting, walking, sitting, standing, sleeping, sex (if applicable), social and travel. Each section has 6 scales, corresponding to 0–5 points. The higher the score, the more severe the dysfunction. Total score summarizes the scores in all sections and is normalized according to Eq. 1.
Total score = [(total score)/(number of items completed by subjects × 5)] × 100 (Krismer and van Tulder, 2007).
Scores are stratified into severity: 0–20%, minimal disability; 21–40%, moderate disability; 41–60%, severe disability; 61–80%, crippling back pain; 81–100%, these patients are either bed-bound or have an exaggeration of their symptoms.
In this study, we defined an LBP scale, ranging from 1 to 5, as no pain, mild dysfunction, moderate dysfunction, severe dysfunction and complete dysfunction, respectively. The LBP score is a linearly discretized variation of the total score. Table 1 shows the corresponding relationship between total scores, ODI scales and LBP scales. It should be stated that the minimal disability in the ODI scales was divided into no pain and mild pain in the LBP scales, so that the differences could be compared in lumbar muscle impedances between the cases of no pain and pain. Additionally, among all pilots, none scored more than 60, which meant that no one had crippling back pain or was bed-bound; therefore, these two ODI scales were integrated as complete dysfunction in the LBP scales.
Bioimpedance Measurement
Impedance measurement of lumbar muscle on both sides of the spine was performed. The bioimpedance measurement system consists of a Solartron 1260 impedance analyzer and a Solartron 1294 interface (Solartron Analytical, Farnborough, United Kingdom), which can operate at the frequency range from 10 Hz to 1 MHz with the accuracy of better than 0.01%. In this study, the current of 1 mA at 50 kHz was applied because the characteristic frequency of impedance property of muscle tissue was around 50 kHz (Bartels et al., 2015; Pandeya et al., 2021). In order to reduce the effect of electrode-skin contact impedance, the four electrode strategy was adopted (Yang et al., 2016, 2017b). To facilitate the implementation of in vivo measurements, we developed a measurement module that can conveniently convert the four electrodes of measurement system to four probes made of silver, two of which were used for excitation and the other two for measurement, as shown in Figure 1. The four probes (1 mm in diameter) are distributed at four corners of a square with a side length of 8 mm. This electrode configuration has been widely used for impedance measurement of biological tissues (Smallwood et al., 2002; Keshtkar, 2007; Laufer et al., 2010; Keshtkar et al., 2012; Bharati et al., 2013).
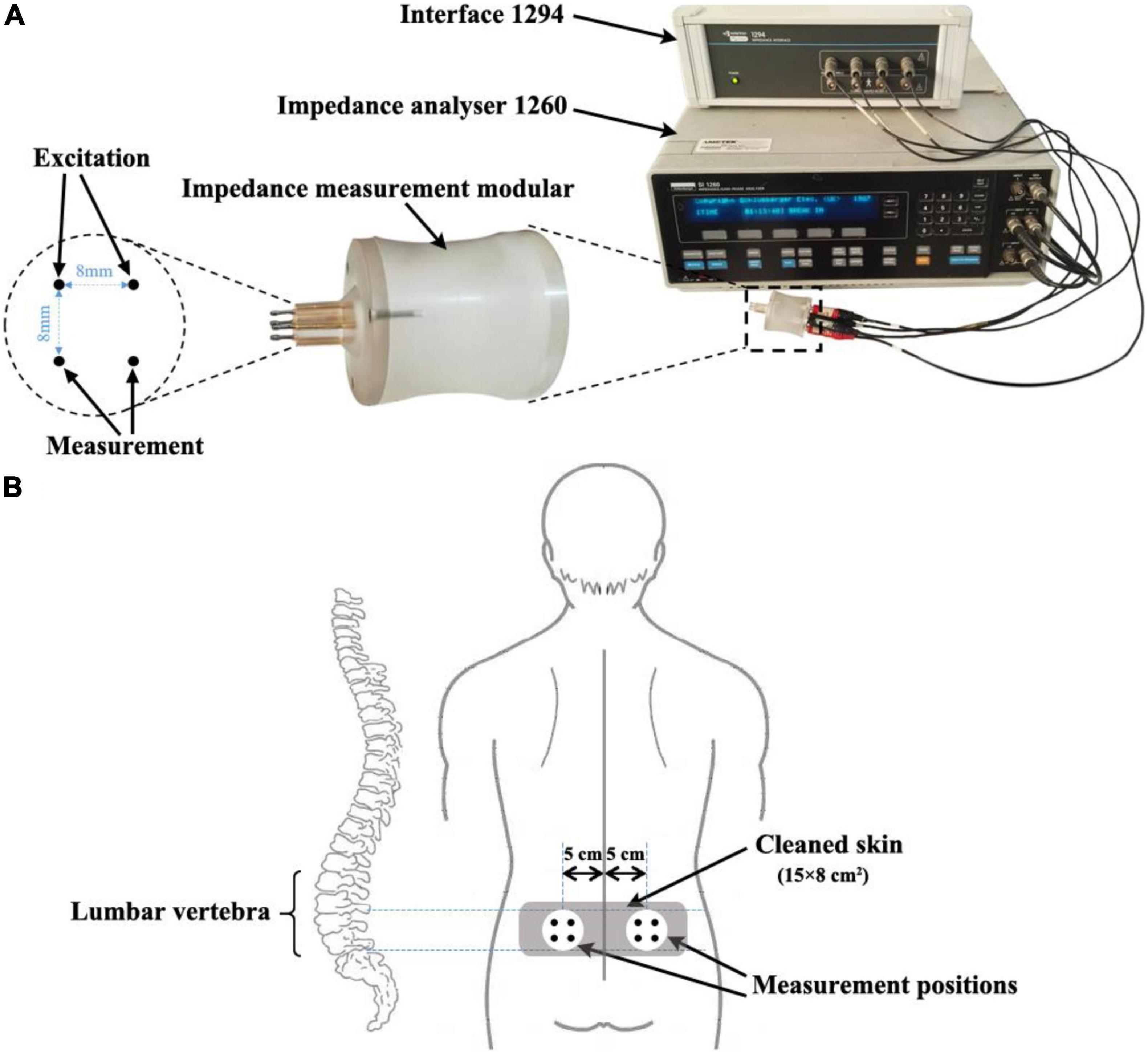
Figure 1. Impedance measurement system and schematic diagram of measurement positions. (A) Impedance measurement system consists of an impedance analyzer (Solartron 1260), a measurement interface (Solartron 1294) and a measurement module developed by our group. (B) Schematic diagram of measurement positions: the impedance measurement module was placed between L4-5 with its center 5 cm from each side of the spine.
The impedance measurement process of lumbar paraspinal muscle was as follows. First, the participant was asked to maintain an upright sitting position. An experienced doctor (specialized in aerospace medicine) determined the region over the L4-5 paraspinal skin (approximately 15 × 8 cm2) and cleaned the skin with medical alcohol. Second, the impedance module was placed in the cleaned area with its center 5 cm from the right side of the spine; and the connecting line of the two excitation probes was parallel to the spine. Then impedance measurement was performed. Third, the impedance measurement on the left side was carried out subsequently at the symmetrical position of the impedance module. To minimize random error in the measurement, the impedance measurements were conducted three times on each side within 2 min. The average of the three measurements was taken as the final result.
Statistical Analysis
The data were summarized in mean ± standard deviation. The coefficient of variation of impedance measurement was calculated for each measurement position to evaluate the measurement reliability.
The impedance modulus and phase of the lumbar muscle on left and right sides of the spine for each participant were obtained, which were denoted as ZL,ϕL,ZR and ϕR, respectively. To evaluate the sensitivity of lumbar muscle impedance to LBP, the sum of impedance on both sides (i.e., Zsum = ZL + ZR and ϕsum = ϕL + ϕR) was compared between individuals with LBP (Scale 2–5) and those without LBP (Scale 1). The reason for this is that different severities of LBP affected the microstate of lumbar muscle to varying degrees. Also, the sum of impedance on both sides (Zsum and ϕsum) for different LBP scales was, respectively, compared. Similarly, in order to further analyze the effect of LBP on the impedance characteristic of the lumbar muscle, the impedance differences between the two sides (i.e., Zdiff = |ZL−ZR| and ϕdiff = |ϕL−ϕR|) were compared between individuals with LBP (Scale 2–5) and those without LBP (Scale 1). This can be justified by the fact that the extent to which LBP affects the microstate of the lumbar muscle on the left and right side of the spine is not exactly the same. Moreover, Zdiff and ϕdiff for different LBP scales were, respectively, compared. The statistical comparisons were carried out with the post hoc test after one-way analysis of variance (ANOVA).
To establish the relationship between lumbar muscle impedance and LBP, the Spearman’s correlation was utilized to assess the correlation between LBP scale and impedance characteristics (Zsum,ϕsum,Zdiff, and ϕdiff), in which all impedance characteristics and the corresponding LBP scales (Scale 1–4) were involved. To evaluate the ability of lumbar muscle impedance for detection of LBP (namely differentiation of pain from no pain), the receiver operator characteristics (ROC) analysis was employed to calculate the sensitivity, specificity, and area under the ROC curve (AUC) of impedance for LBP prediction. In the ROC analysis, all impedances and the corresponding LBP scales (Scale 1–4) were used to predict pain (Scale 2–4), i.e., the impedance corresponding to Scale 1 (no pain) was marked as 0 and the impedance corresponding to Scale 2–4 (pain) was marked as 1. SPSS 22.0 (IBM Software, Armonk, NY, United States) was utilized for statistical analysis and a P-value < 0.05 was considered statistically significant.
Results
Basic Information
Participant Characteristics
Participants were aged from 22 to 48 (32.49 ± 6.56) years, with a weight range from 62 to 91 (73.23 ± 6.78) kg and a height range from 167 to 185 (174.41 ± 3.90) cm. Figure 2 shows the symptoms of LBP and the ODI questionnaire results. Fifty-one participants reported the presence of LBP, 14 individuals already had moderate and severe dysfunction, but no one had complete dysfunction.
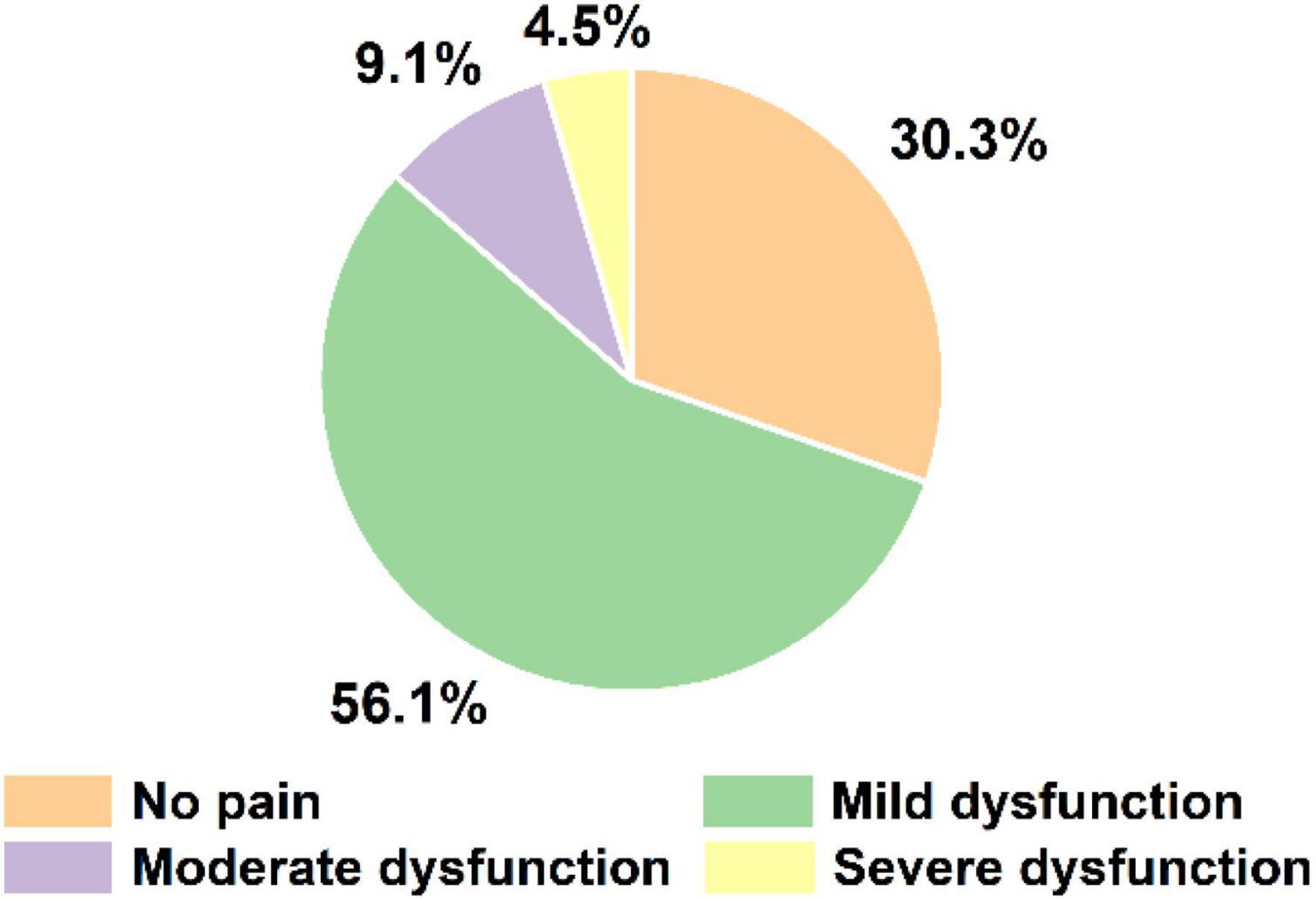
Figure 2. The distribution of subjects with corresponding LBP scale. Complete dysfunction was not applicable to any participants.
Variability of Impedance Measurement
A total of 144 sets of impedance data were obtained from the lumbar muscle on both sides of the spine in 72 subjects. Figure 3 shows that the coefficient of variation for the impedance modulus and for the phase angle at all measurement positions were all less than 0.25.
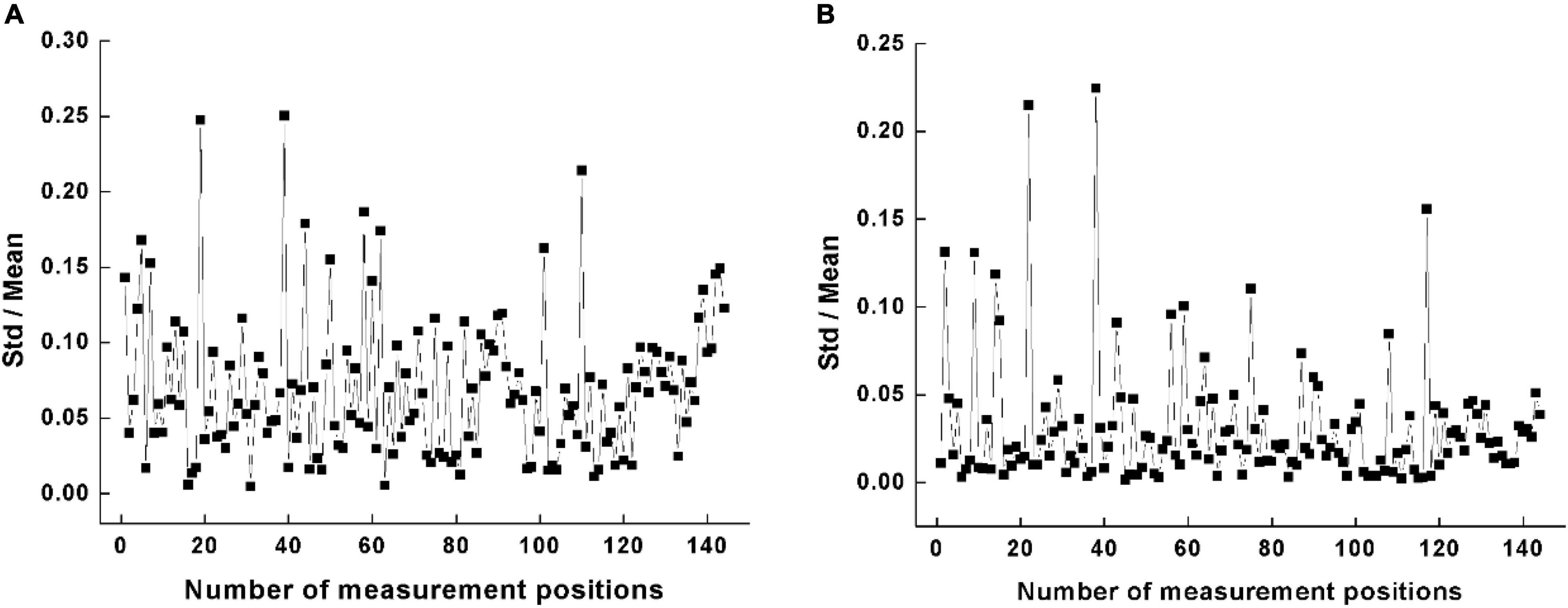
Figure 3. Variability of impedance measurement at all measurement positions. (A) Coefficient of variation for the impedance modulus. (B) R coefficient of variation for the impedance phase angle.
Analysis of Sensitivity of Lumbar Muscle Impedance to Low Back Pain
Comparison of Zsum and ϕsum for Different Low Back Pain Scales
Figure 4 shows the comparison of the Zsum and ϕsum between participants without LBP (Scale 1) and those with LBP (Scale 2–4). There was a significant difference in impedance modulus Zsum(pain: 6.09 ± 1.70; no pain: 3.48 ± 0.72; P < 0.05), whereas no significant difference was found in impedance phase angle ϕsum (pain: 1.01 ± 0.20; no pain: 1.06 ± 0.19; P = 0.736).
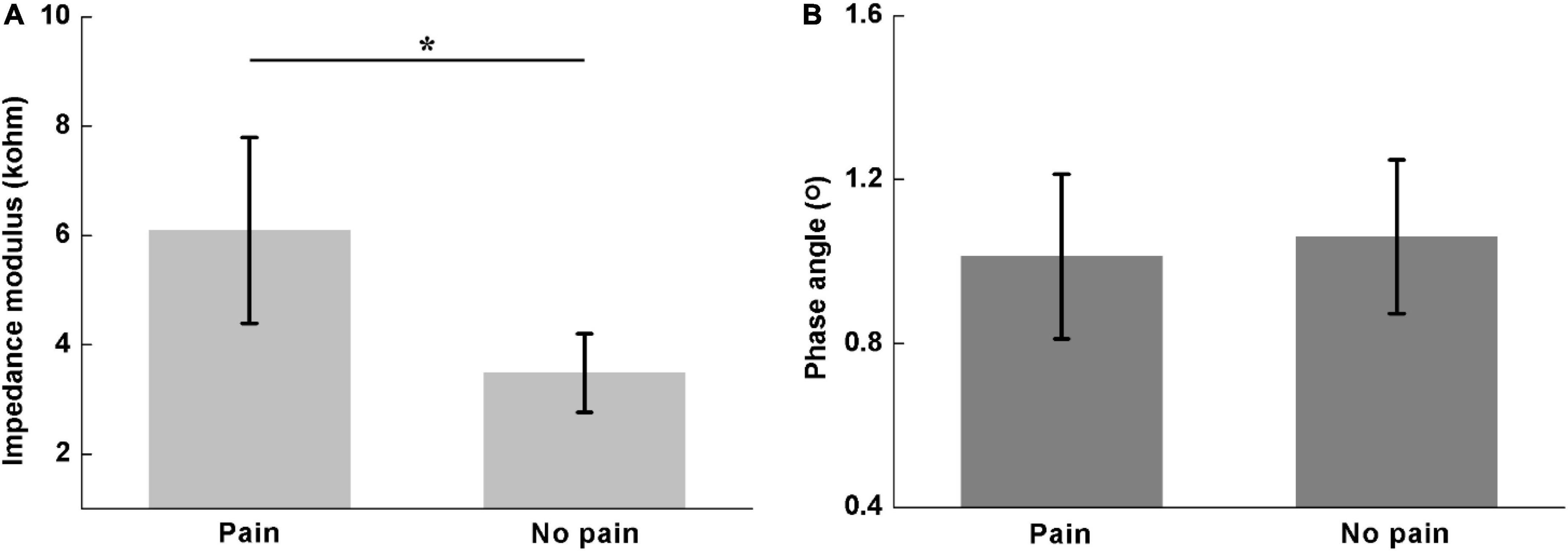
Figure 4. Comparisons of the sum of lumbar muscle impedance on both sides between participants with and without LBP, including (A) impedance modulus (pain: 6.09 ± 1.70; no pain: 3.48 ± 0.72) and (B) phase angle (pain: 1.01 ± 0.20; no pain: 1.06 ± 0.19). *denotes P < 0.05.
Figure 5 shows the comparisons of Zsum and ϕsum for different LBP scales. The Zsum increased whereas the ϕsum decreased along with the LBP severity. Significant differences in Zsum were found between Scale 1 and 3 (Scale 1: 3.48 ± 0.72; Scale 3: 6.48 ± 1.46; P < 0.01), Scale 1 and 4 (Scale 1: 3.48 ± 0.72; Scale 4: 7.45 ± 0.98; P < 0.01), and Scale 2 and 4 (Scale 2: 5.22 ± 1.67; Scale 4: 7.45 ± 0.98; P < 0.05), respectively. However, for ϕsum, significant difference was only found between Scale 1 and 4 (Scale 1: 1.06 ± 0.19; Scale 4: 0.76 ± 0.23; P < 0.05).
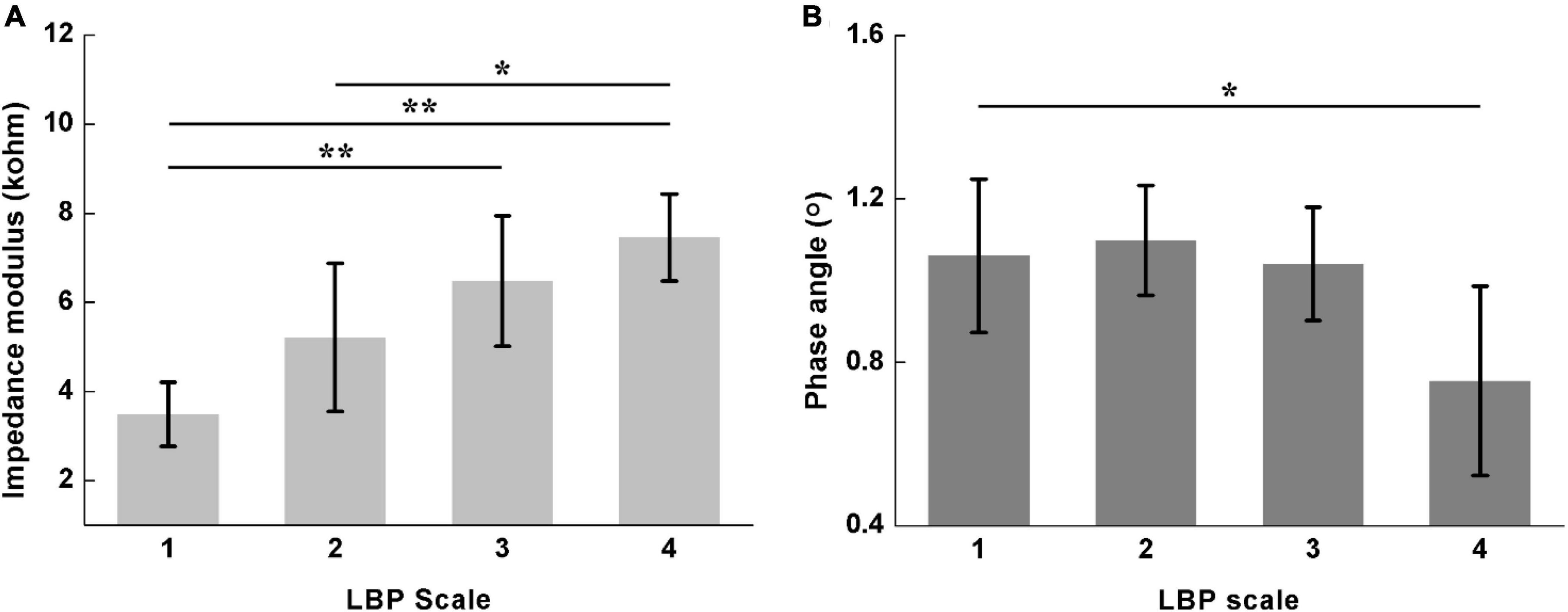
Figure 5. Comparisons of the sum of lumbar muscle impedance on both sides for different LBP scales, including (A) impedance modulus (Scale 1: 3.48 ± 0.72; Scale 2: 5.22 ± 1.67; Scale 3: 6.48 ± 1.46; Scale 4: 7.45 ± 0.98) and (B) phase angle (Scale 1: 1.06 ± 0.19; Scale 2: 1.10 ± 0.13; Scale 3: 1.04 ± 0.14; Scale 4: 0.76 ± 0.23). Scale 1–4 of LBP represent no pain, mild dysfunction, moderate dysfunction and severe dysfunction, respectively. *denotes P < 0.05 and **denotes P < 0.01.
Comparison of Zdiff and ϕdiff for Different Low Back Pain Scales
Figure 6 shows the comparison of Zdiff and ϕdiff between participants with and without LBP. There were significant differences in both Zdiff(pain: 2.59 ± 1.42; no pain: 0.42 ± 0.24; P < 0.01) and ϕdiff(pain: 0.16 ± 0.08; no pain: 0.06 ± 0.03; P < 0.01).
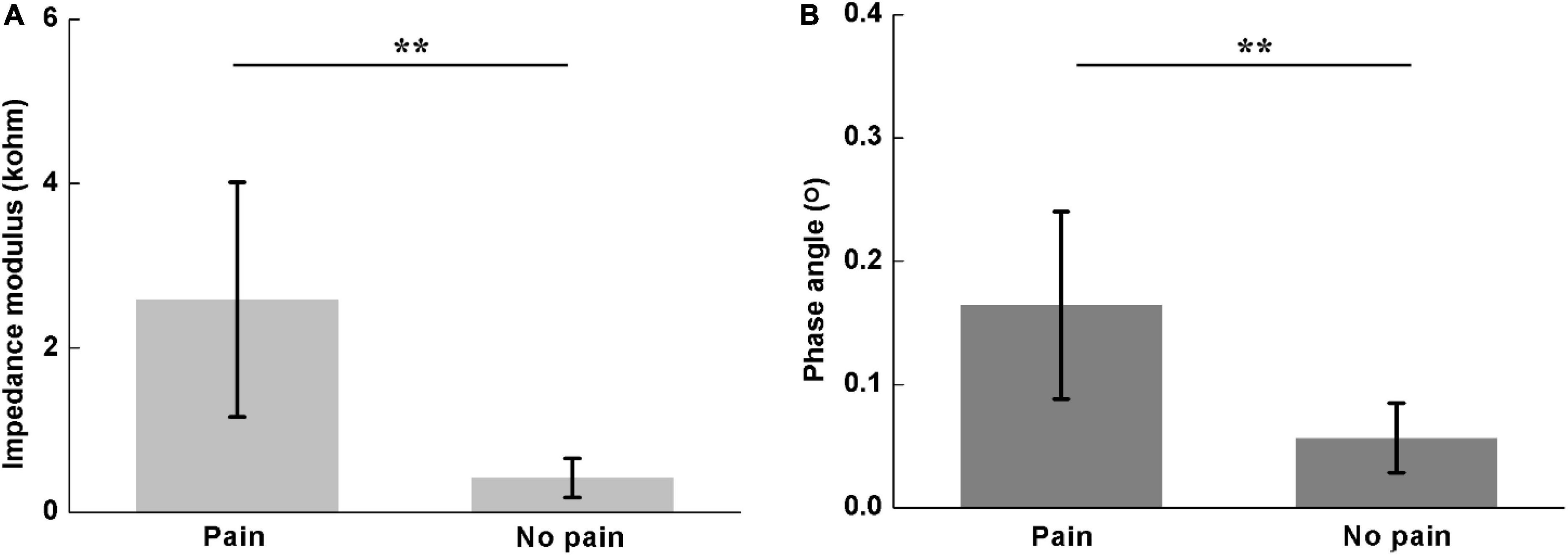
Figure 6. Comparisons of difference of lumbar muscle impedance on both sides between participants with and without LBP, including (A) impedance modulus (pain: 2.59 ± 1.42; no pain: 0.42 ± 0.24) and (B) phase angle (pain: 0.10 ± 0.05; no pain: 0.11 ± 0.03). **denotes P < 0.01.
Figure 7 shows the comparison of Zdiff and ϕdiff for different LBP scales. Significant differences in Zdiff were found among four pain scales (Scale 1: 0.42 ± 0.24; Scale 2: 1.85 ± 0.87; Scale 3: 3.08 ± 1.05; Scale 4: 3.99 ± 1.23; P < 0.05), except between Scale 3 and 4 (P = 0.465). Similarly, significant differences were also found in ϕdiff among four pain scales (Scale 1: 0.06 ± 0.03; Scale 2: 0.10 ± 0.03; Scale 3: 0.20 ± 0.05; Scale 4: 0.25 ± 0.08; P < 0.05), except between Scale 3 and 4 (P = 0.402).
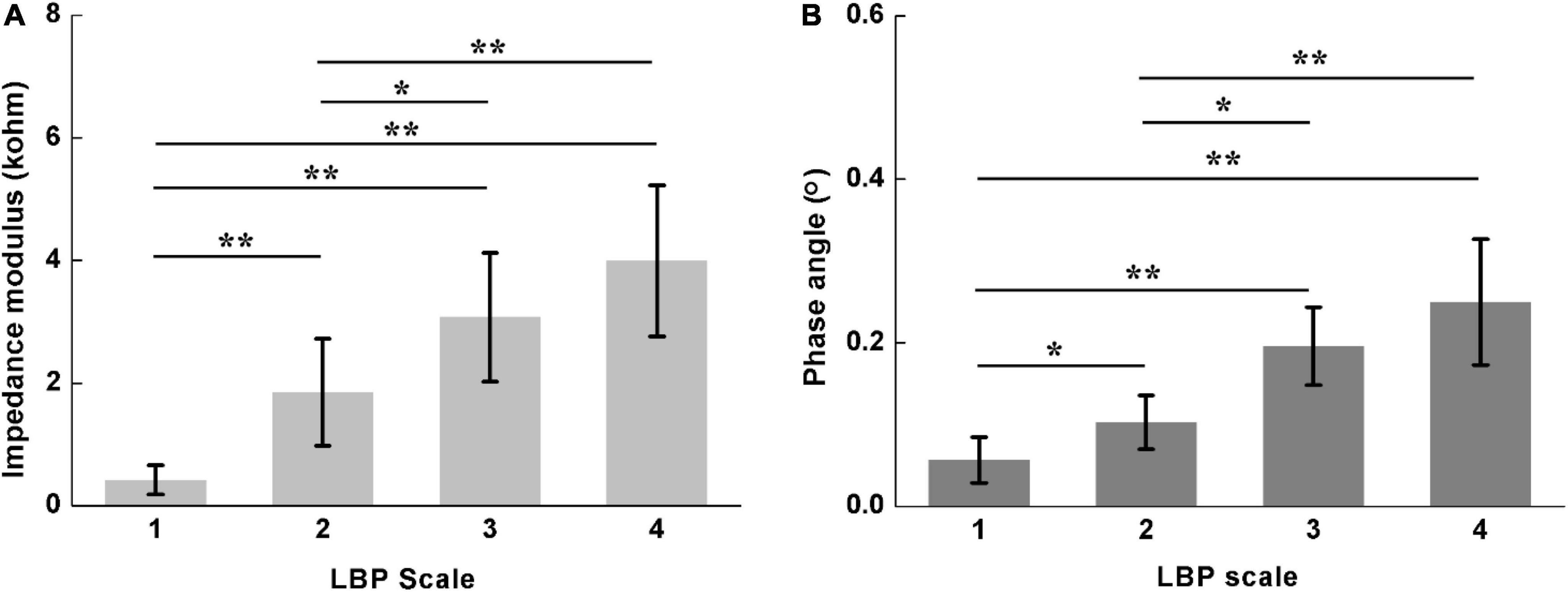
Figure 7. Comparison of difference of lumbar muscle impedance on both sides for different LBP scales, including (A) impedance modulus (Scale 1: 0.42 ± 0.24; Scale 2: 1.85 ± 0.87; Scale 3: 3.08 ± 1.05; Scale 4: 3.99 ± 1.23) and (B) phase angle (Scale 1: 0.06 ± 0.03; Scale 2: 0.10 ± 0.03; Scale 3: 0.20 ± 0.05; Scale 4: 0.25 ± 0.08). LBP Scale 1–4 represents no pain, mild dysfunction, moderate dysfunction and severe dysfunction, respectively. *denotes P < 0.05; **denotes P < 0.01.
Assessment of the Relationship Between Lumbar Muscle Impedance and Low Back Pain
Correlation Between Lumbar Muscle Impedance and Low Back Pain Scale
The correlations between lumbar muscle impedance and LBP are presented in Table 2, in which the following significant results could be observed: a positive association between Zsum and LBP scales (R = 0.692, P < 0.01), and a weak negative association between ϕsum and LBP scales (R = −0.281, P > 0.05). Also, the higher association was found between Zdiff and LBP scales (R = 0.860, P < 0.01), and also between ϕdiff and pain scales (R = −0.858, P < 0.01).
Identification Evaluation
Receiver operator characteristics analysis showed that AUC of Zsum,ϕsum,Zdiff and ϕdiff were 0.931, 0.548, 0.992, and 0.965 for pain prediction (LBP Scale 2, 3, and 4), respectively. The sensitivity and specificity ranged from 0.501 to 0.976 and from 0.667 to 1, respectively (Figure 8 and Table 3).
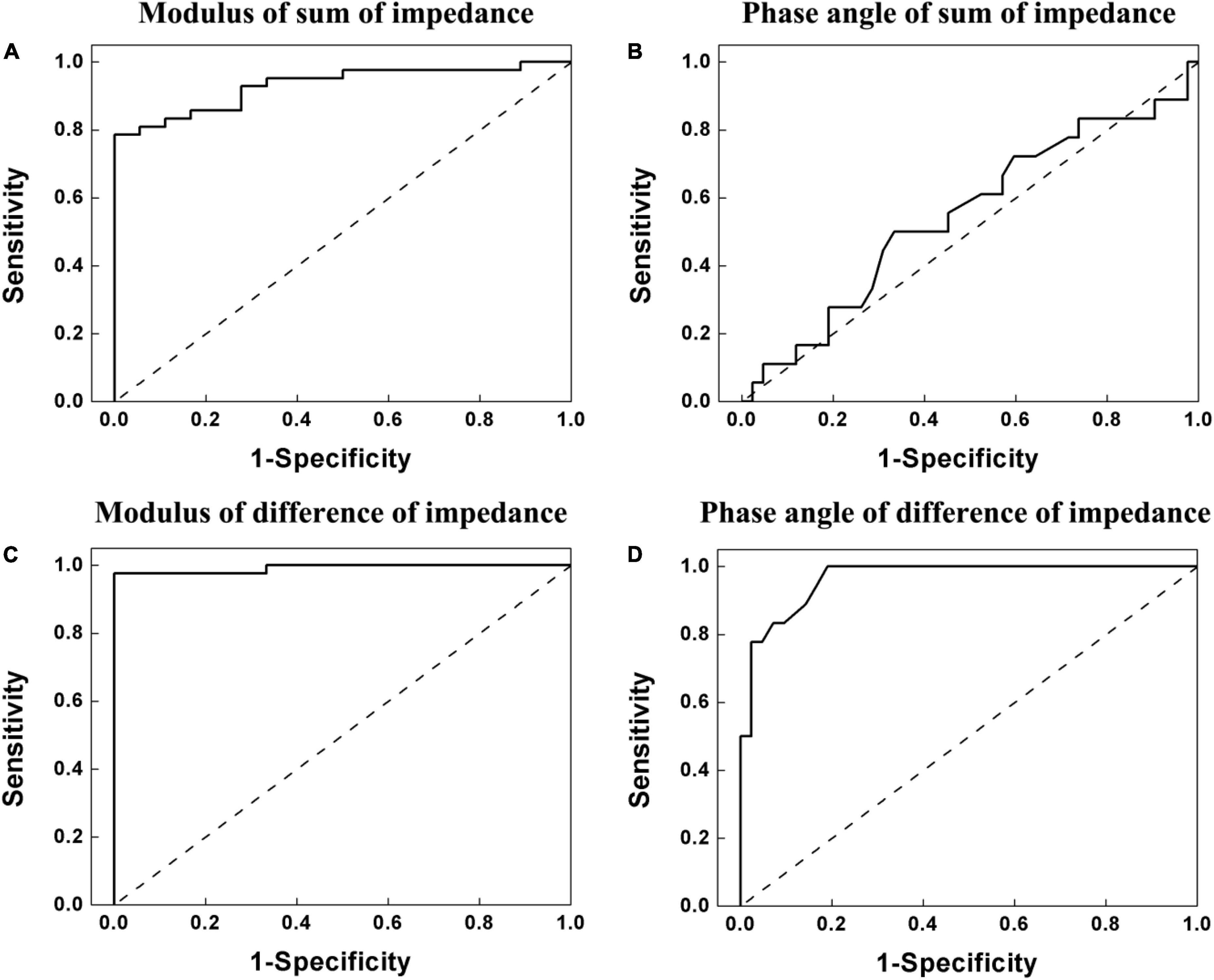
Figure 8. Receiver operating characteristic (ROC) curve of the lumbar muscle impedance for ability to predict pain (Scale 2, 3, and 4 in ODI). (A) Modulus of sum of lumbar muscle impedance on both sides. (B) Phase angle of sum of lumbar muscle impedance on both sides. (C) Modulus of difference of lumbar muscle impedance on both sides. (D) Phase angle of difference of lumbar muscle impedance on both sides. For modulus (Zsum) and phase angle (ϕsum) of sum of lumbar muscle impedance on both sides, as well as modulus (Zdiff) and phase angle (ϕdiff) of difference of lumbar muscle impedance on both sides, the areas under the ROC curve (AUC) of the, the were 0.931, 0.548, 0.992, and 0.965, respectively, for pain prediction (LBP Scale 2, 3, and 4). The sensitivity and specificity range from 0.501 to 0.976 and from 0.667 to 1, respectively.
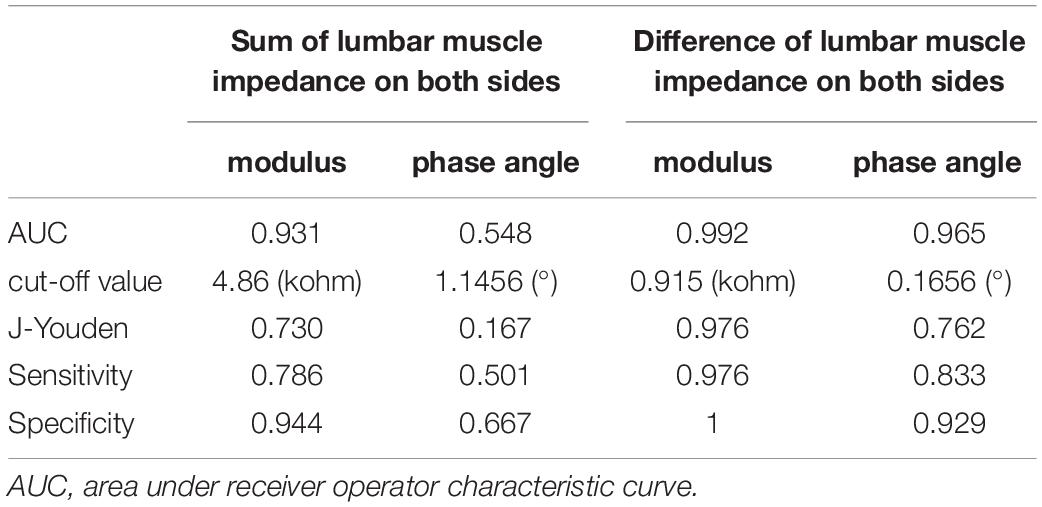
Table 3. Area under the receiver operator characteristic curves for ability to predict pain for lumbar muscle impedance of helicopter pilots.
Discussion
In this study, we explored the feasibility of using EBI to assess LBP by means of measuring lumbar muscle impedance and LBP scales in 72 helicopter pilots. The correlation between impedance characteristics and LBP scales were significant.
Summary and Explanations of Experimental Results
Analysis of Sensitivity of Lumbar Muscle Impedance to Low Back Pain
In this study, the modulus of lumbar muscle impedance increased with the LBP severity whereas the phase angle decreased. Also for different LBP scales, significant differences were found, respectively, in Zsum, as well as in Zdiff and ϕdiff. These results indicated the capability of lumbar muscle impedance to accurately reflect LBP scales, suggesting that lumbar muscle impedance should be sensitive to the state of lumbar muscle associated with LBP in helicopter pilots. Overall, our results largely agree with the previous studies. For example, Fujita et al. (2001) and Ching et al. (2013) observed that individuals with LBP had larger impedance than healthy volunteers. Dibai-Filho et al. (2018) concluded that individuals with lower pressure pain thresholds had higher impedance in the torso. However, these previous studies aimed at clinical patients, rather than the particular group of helicopter pilots. Additionally, contrary to the present study, Yamamoto et al. (2006) found a significant decrease in electrical impedance after pain provocation in patients with shoulder pain. This difference may be attributed to some practical factors, such as impedance measurement strategy. In the study of Yamamoto et al. (2006) the impedance measurement was carried out using three-electrode method with a very low frequency current (10 Hz). Considering that the electrode-skin contact impedance was much larger than the tissues impedance at low frequencies (below 1 kHz) (Rosell et al., 1988; Yang et al., 2017a), their measurement results included not only the tissue impedance between measuring electrodes, but also the electrode-skin contact impedance. To effectively eliminate the influence of electrode-skin contact impedance on the measurement results, we adopted the four-electrode approach to perform impedance measurement in this study (Bartels et al., 2015; Pandeya et al., 2021).
The difference of lumbar muscle impedance for different LBP scales could be associated with the physiological structure of muscle tissue. Due to the peculiarities of the occupation, helicopter pilots were often exposed to the vibration and fixed sitting posture for a long time, which readily caused muscle fatigue. The continuous accumulation of muscle fatigue could lead to chronic inflammation or even microvascular damage in the internal muscle tissue. Inflammation could cause two changes, which have opposite effects on the lumbar muscle impedance. On one hand, the muscle inflammation commonly resulted in local swelling because more interstitial fluid and blood at the local area were required for the facilitation of muscle repair. This increased the extracellular gaps and thus would be expected to decrease resistance (Song et al., 2018). On the other hand, the inflamed muscles were under repair and thus many proteins needed in the repairmen process were synthesized inside the muscle cells. This could cause a significant increase of intracellular resistance. However, according to the equivalent circuit of the impedance of the lumbar paraspinal muscles suggested by Yamamoto and Yamamoto (1976), the increase of intracellular resistance of inflamed muscles would increase the overall impedance of lumbar muscle tissue when the high frequency current (>1 kHz, 50 kHz in this study) was employed because it could pass through the cell membrane (Ching et al., 2013). Therefore, the modulus of lumbar muscle impedance of pilots with LBP was higher than those without, as shown in Figure 3. Moreover, as the LBP severity increased, more severe inflammation further increased the impedance modulus, as shown in Figure 4.
As for the change in the phase angle caused by LBP, the possible explanations were as follows. At the stage of muscle inflammation, the myocyte membranes remained stable or underwent little change, so the reactance of lumbar muscle impedance might not change greatly. However, due to the significant increase in the resistance of lumbar muscle impedance, the phase angle (determined by the ratio of reactance to resistance) of lumbar muscle impedance decreased (Figures 3, 4).
Analysis of Relationship Between Lumbar Muscle Impedance and Low Back Pain
It was found that the trend of modulus of lumbar muscle impedance was the same as that of LBP scale. By contrast, the trend of impedance phase angle was exactly the opposite to that of LBP scale. Besides, Spearman’s correlation analysis manifested that there was a strong correlation between Zsum and LBP scale (R = 0.692, P < 0.01), an excellent correlation between Zdiff and LBP scale (R = 0.86, P < 0.01), and a desirable correlation between ϕdiff and LBP scale (R = −0.858, P < 0.01). These high correlation coefficients suggested that Zsum,Zdiff, and ϕdiff of EBI technique are potentially useful metrics to assess LBP scale. In addition, ROC analysis showed that in pain prediction (LBP Scale 2, 3, and 4), AUC of Zsum,Zdiff, and ϕdiff are all above 0.93, indicating that EBI could determine the state of lumbar muscle associated with LBP.
In the present study, the correlations between Zdiff and LBP scales, ϕdiff and LBP scales were higher than those using Zsum and ϕsum. This might be because the process of subtraction removed some of inter-individual variability. First, despite of strict control in the placement of measurement probes, the probe positions on each subject could not be ensured to be identical owing to the variation in individual low back sizes and shapes. It should be noted that a small error of probe position could lead to a large alteration of impedance (Dai et al., 2020). Second, there were significant differences in physical characteristics of all the subjects. For example, the weight of all the subjects widely ranged from 62 to 91 (73.23 ± 6.78) kg and their height ranged from 167 to 185 (174.41 ± 3.90) cm. These physiological differences could result in considerable variations in the distributions of electric fields for all individuals. Therefore, in the future, a standard strategy of impedance measurement as well as the reliable parameters extracted from lumbar muscle impedance should be explored to alleviate the effects of inter-individual variability, so that the quantitative relationship between lumbar muscle impedance and LBP could be established. Nevertheless, with high correlations between the EBI-based parameters and LBP scores (Table 2) and high AUC (Figure 8), it suggested that EBI technique was a potentially useful tool to assess LBP severity.
Considerations in Assessment of Low Back Pain With Electrical Bio-Impedance in Practical Application
In this study, we performed impedance measurement by using a commercial high-accuracy impedance measurement system. This system ensured the correctness and reliability of our experimental results; however, its large size, heavy weight and high cost make it difficult to be widely used in the primary medical units. Thus, from the perspective of practical application, new impedance measurement system need to be developed. First, in order to minimize the size and weight of the measurement, highly integrated electronic components are preferred, such as the high-precision chip AD5933 specially designed for bioimpedance measurement (Yamamoto and Yamamoto, 1976). Second, for convenient use, it is best to integrate the impedance system into a chip or a circuit board to further reduce its size to embed it into the measurement module. Third, a small excitation current should be explored (1 mA was used in the present study) whether it could also obtain reliable measurements to evaluate LBP, so that a battery of small size can be used as power supply to further minimize the impedance system (Yang et al., 2021).
Limitations and Future Work
In this study, we measured the impedance of lumbar muscle only at the very frequency of 50 kHz for the following reasons. First, in practice, for rapid assessment of LBP of pilots, the impedance at one single frequency was preferred. Second, Bartels et al. (2015) measured the impedance of muscle tissue at frequencies between 4 and 1,000 kHz and they found that the characteristic frequency of impedance property of muscle tissue was around 50 kHz. However, the use of the excitation current at a single frequency may have several limitations. First, the impedance measurement at a fixed frequency could not allow the mathematical modeling of impedance spectra properties to obtain dielectric parameters, such as characteristic frequencies within different frequency dispersions ranges in the Cole-Cole model (Tang et al., 2009; Elwakil et al., 2021). These dielectric parameters may be more useful to evaluate the state of lumbar muscle. Second, impedance information at low frequencies (<1 kHz) was not involved. In several studies it was found that impedance at low frequencies may be more sensitive to muscle state (Kumar et al., 2019a; Mortreux et al., 2019). Therefore, further studies on impedance measurement by sweeping frequency over a wide frequency range will be designed to investigate the specified dielectric parameters to assess LBP on the premise of rapid measurement and safety.
In this study, the impedance of lumbar muscle on each side of the spine was measured in a short time period of 2 min, whereas the time-varying impedance of lumbar muscle was not measured. Considering the possibility of using the time-varying impedance to reflect the development of LBP, we will design a bioimpedance system to monitor/measure time-varying impedance of lumbar muscle. Moreover, the ability of lumbar muscle impedance to detect LBP, namely differentiate pain from no pain, was preliminarily explored by performing ROC analysis. However, the impedances of lumbar muscles in only 72 helicopter pilots were collected in this study, which may not be enough for accurate prediction of pain. In future work, a larger impedance database from helicopter pilots will be established to improve the accuracy of pain prediction.
In this study, we measured the impedance of lumbar muscle and attempted to establish the correlation between impedance properties and LBP. Nevertheless, the intrinsic physiological mechanism between LBP and impedance remained unclear since the microscopic morphology for different LBP scales was not measured. To date, several studies have investigated the influence of microscopic conditions of biological tissues on bioimpedance, such as the cell morphology (Wang et al., 2015; Song et al., 2018) and electrolyte concentrations in biological tissues (Kumar et al., 2019a,b). Therefore, further work on intrinsic physiological mechanism will be performed in order to provide a solid biophysical fundamental to use EBI for early detection of LBP among helicopter pilots.
Conclusion
In this study, we explored the feasibility of using EBI technique to detect LBP by means of measuring the lumbar muscle impedance of 72 helicopter pilots and quantitatively analyzing correlation between muscle impedance characteristics and LBP scale. Results showed that for different LBP scales, there existed the significant differences in the modulus of sum of lumbar muscle impedance on both sides, as well as the modulus and phase angle of difference of lumbar muscle impedance on both sides, respectively. These results suggested that EBI has potential to be the daily detection tool in primary medical units to assess the state of the lumbar muscle associated with LBP, so that timely medical intervention could be carried out prior to the observation of apparent symptoms. Future work focusing on optimization of measurement parameters, extraction of impedance features and development of portable impedance measurement system would be designed to improve the practical application of EBI for early detection of LBP among helicopter pilots.
Data Availability Statement
The original contributions presented in this study are included in the article/supplementary material, further inquiries can be directed to the corresponding authors.
Ethics Statement
The studies involving human participants were reviewed and approved by the Ethics Committee of Fourth Military Medical University (No. KY20163064-1). The patients/participants provided their written informed consent to participate in this study.
Author Contributions
HW, JD, CW, ZG, YL, GT, and LY contributed to this research and subsequent manuscript from conception to final preparation. HW, JD, YL, and ZZ helped design, collect, and analyze data. All authors contributed to the article and approved the submitted version.
Funding
This work was partially supported by the National Natural Science Foundation of China (NSFC 61901478 and 81601636), Medical Program of FMMU (Grant Nos. 2018HKTS10 and 2019ZTC01), and Key R&D Program of Shaanxi Province (2021SF-027).
Conflict of Interest
The authors declare that the research was conducted in the absence of any commercial or financial relationships that could be construed as a potential conflict of interest.
Publisher’s Note
All claims expressed in this article are solely those of the authors and do not necessarily represent those of their affiliated organizations, or those of the publisher, the editors and the reviewers. Any product that may be evaluated in this article, or claim that may be made by its manufacturer, is not guaranteed or endorsed by the publisher.
References
Abe, T., Yoshimura, Y., Imai, R., Yoneoka, Y., Tsubaki, A., and Sato, Y. (2021). Impact of phase angle on physical function in patients with acute stroke. J. Stroke Cerebrovasc. Dis. 30:105941. doi: 10.1016/j.jstrokecerebrovasdis.2021.105941
Al-harosh, M., Chernikov, E., and Shchukin, S. (2022). Patient specific modeling for renal blood monitoring using electrical bio-impedance. Sensors 22:606. doi: 10.3390/s22020606
Alrwaily, M. A. (2017). Comparison Between Stabilization Exercises and Stabilization Exercises Supplemented With Neuromuscular Electrical Stimulation in Patients With Chronic Low Back Pain: A Phase I Randomized Controlled Trial. Ph.D. thesis. Ann Arbor: University of Pittsburgh.
Andersen, K., Baardsen, R., Dalen, I., and Larsen, J. P. (2017). Impact of exercise programs among helicopter pilots with transient LBP. BMC Musculoskelet. Disord. 18:269. doi: 10.1186/s12891-017-1631-0N
Arvanitidis, M., Bikinis, N., Petrakis, S., Gkioka, A., Tsimpolis, D., Falla, D., et al. (2021). Spatial distribution of lumbar erector spinae muscle activity in individuals with and without chronic low back pain during a dynamic isokinetic fatiguing task. Clin. Biomec. 81:105214. doi: 10.1016/j.clinbiomech.2020.105214
Bartels, E. M., Sorensen, E. R., and Harrison, A. P. (2015). Multi-frequency bioimpedance in human muscle assessment. Physiol. Rep. 3:e12354. doi: 10.14814/phy2.12354
Bharati, S., Rishi, P., Tripathi, S. K., and Koul, A. (2013). Changes in the electrical properties at an early stage of mouse liver carcinogenesis. Bioelectromagnetics 34, 429–436. doi: 10.1002/bem.21783
Ching, C. T., Chen, Y. C., Lu, L. H., Hsieh, P. F., Hsiao, C. S., Sun, T. P., et al. (2013). Characterization of the muscle electrical properties in low back pain patients by electrical impedance myography. PLoS One 8:e61639. doi: 10.1371/journal.pone.0061639
Chow, J. H. W., and Chan, C. C. H. (2005). Validation of the Chinese version of the oswestry disability index. Work 25, 307–314.
da Silva, G. V. (2020). Low back pain prevalence in Brazilian helicopter pilots. Ergon. Int. J. 4:e16000240.
Dai, M., Cao, Q., Ding, S., Zhao, Z., Cao, X., Wen, Z., et al. (2020). Real-time monitoring hypoxia at high altitudes using electrical bioimpedance technique: an animal experiment. J. Appl. Physiol. 130, 952–963. doi: 10.1152/japplphysiol.00712.2020
Dibai-Filho, A. V., Barros, M. A., de Oliveira, A. K., and de Jesus Guirro, R. R. (2018). Electrical impedance of the torso is associated with the pressure pain threshold on myofascial trigger points in patients with chronic neck pain: a cross-sectional study. J. Back Musculoskelet. Rehabil. 31, 275–284. doi: 10.3233/BMR-169671
Elwakil, A. S., Al–Ali, A. A., and Maundy, B. J. (2021). Extending the double-dispersion Cole–Cole, Cole–Davidson and Havriliak–Negami electrochemical impedance spectroscopy models. Eur. Biophys. J. 50, 915–926.
Fujimoto, K., Inage, K., Eguchi, Y., Orita, S., Suzuki, M., Kubota, G., et al. (2018). Use of bioelectrical impedance analysis for the measurement of appendicular skeletal muscle mass/whole fat mass and its relevance in assessing osteoporosis among patients with low back pain: a comparative analysis uuing dual X-ray absorptiometry. Asian. Spine J. 12, 839–845. doi: 10.31616/asj.2018.12.5.839
Fujimoto, K., Inage, K., Eguchi, Y., Orita, S., Toyoguchi, T., Yamauchi, K., et al. (2019). Dual-energy X-ray absorptiometry and bioelectrical impedance analysis are beneficial tools for measuring the trunk muscle mass of patients with low back pain. Spine Surg. Relat. Res. 3, 335–341. doi: 10.22603/ssrr.2018-0040
Fujita, T., Fujii, Y., Okada, S. F., Miyauchi, A., and Takagi, Y. (2001). Fall of skin impedance and bone and joint pain. J. Bone Miner. Metab. 19, 175–179. doi: 10.1007/s007740170038
Gaydos, S. J. (2012). Low back pain: considerations for rotary-wing aircrew. Aviat. Space Environ. Med. 83, 879–889. doi: 10.3357/asem.3274.2012
Harrison, A. P. A. (2018). More precise, repeatable and diagnostic alternative to surface electromyography – an appraisal of the clinical utility of acoustic myography. Clin. Physiol. Funct. Imaging 38, 312–325. doi: 10.1111/cpf.12417
Harrison, M. F., Neary, J. P., Albert, W. J., Kuruganti, U., Croll, J. C., Chancey, V. C., et al. (2009). Measuring neuromuscular fatigue in cervical spinal musculature of military helicopter aircrew. Mil. Med. 174, 1183–1189. doi: 10.7205/milmed-d-00-7409
Huang, Q. H., Zheng, Y. P., Chena, X., He, J. F., and Shi, J. A. (2007). System for the synchronized recording of sonomyography, electromyography and joint angle. Open Biomed. Eng. J. 1, 77–84. doi: 10.2174/1874120700701010077
Keshtkar, A. (2007). Virtual bladder biopsy using bio-impedance spectroscopy at 62.500Hz–1.024MHz. Measurement 40, 585–590.
Keshtkar, A., Salehnia, Z., Somi, M. H., and Eftekharsadat, A. T. (2012). Some early results related to electrical impedance of normal and abnormal gastric tissue. Phys. Med. 28, 19–24. doi: 10.1016/j.ejmp.2011.01.002
Krismer, M., and van Tulder, M. (2007). Low back pain (non-specific). Best Pract. Res. Clin. Rheumatol. 21, 77–91. doi: 10.1007/s00108-018-0475-5
Kumar, G., Kasiviswanathan, U., Mukherjee, S., Kumar Mahto, S., Sharma, N., and Patnaik, R. (2019a). Changes in electrolyte concentrations alter the impedance during ischemia-reperfusion injury in rat brain. Physiol. Meas. 40:105004. doi: 10.1088/1361-6579/ab47ee
Kumar, G., Paliwal, P., Mukherjee, S., Patnaik, N., Krishnamurthy, S., and Patnaik, R. (2019b). Pharmacokinetics and brain penetration study of chlorogenic acid in rats. Xenobiotica 49, 339–345. doi: 10.1080/00498254.2018.1445882
Laufer, S., Ivorra, A., Reuter, V. E., Rubinsky, B., and Solomon, S. B. (2010). Electrical impedance characterization of normal and cancerous human hepatic tissue. Physiol. Meas. 31, 995–1009. doi: 10.1088/0967-3334/31/7/009
Lingwood, B. E., Dunster, K. R., Healy, G. N., Ward, L. C., and Colditz, P. B. (2003). Cerebral impedance and neurological outcome following a mild or severe hypoxic/ischemic episode in neonatal piglets. Brain Res. 969, 160–167. doi: 10.1016/s0006-8993(03)02295-9
Marins, E., Caputo, E., da Silva, M., Dawes, J., Orr, R., and Alberton, C. L. (2020). Conservative interventions for non-specific low back pain in tactical populations: a systematic review of randomized controlled trials. Sustain. 12:7922.
Mortreux, M., Semple, C., Riveros, D., Nagy, J. A., and Rutkove, S. B. (2019). Electrical impedance myography for the detection of muscle inflammation induced by lambda-carrageenan. PLoS One 14:e0223265. doi: 10.1371/journal.pone.0223265
Murray, M., Lange, B., Nornberg, B. R., Sogaard, K., and Sjogaard, G. (2015). Specific exercise training for reducing neck and shoulder pain among military helicopter pilots and crew members: a randomized controlled trial protocol. BMC Musculoskelet. Disord. 16:198. doi: 10.1186/s12891-015-0655-6
Palmer, K. T., and Bovenzi, M. (2015). Rheumatic effects of vibration at work. Best Pract. Res. Clin. Rheumatol. 29, 424–439. doi: 10.1016/j.berh.2015.05.001
Pandeya, S. R., Nagy, J. A., Riveros, D., Semple, C., Taylor, R. S., Sanchez, B., et al. (2021). Relationships between in vivo surface and ex vivo electrical impedance myography measurements in three different neuromuscular disorder mouse model. PLoS One 16:e0259071. doi: 10.1371/journal.pone.0259071
Posch, M., Schranz, A., Lener, M., Senn, W., Äng, B. O., Burtscher, M., et al. (2019). Prevalence and potential risk factors of flight-related neck, shoulder and low back pain among helicopter pilots and crewmembers: a questionnaire-based study. BMC Musculoskelet. Disord. 20:44. doi: 10.1186/s12891-019-2421-7
Rose-Dulcina, K., Genevay, S., Dominguez, D., Armand, S., and Vuillerme, N. (2020). Flexion-relaxation ratio asymmetry and its relation with trunk lateral ROM in individuals with and without chronic nonspecific low back pain. Spine 45, E1–E9. doi: 10.1097/BRS.0000000000003196
Rosell, J., Colominas, J., Riu, P., Pallas-Areny, R., and Webster, J. G. (1988). Skin impedance from 1 Hz to 1 MHz. IEEE Trans. Biomed. Eng. 35, 649–651. doi: 10.1109/10.4599
Rutkove, S., Wang, Y., Freedman, L., Lyczmanenko, A., and Keel, J. (2019). Electrical impedance myography for assessing patients with low back pain. Neurology 92, P2.6-069.
Smallwood, R. H., Keshtkar, A., Wilkinson, B. A., Lee, J. A., and Hamdy, F. C. (2002). Electrical impedance spectroscopy (EIS) in the urinary bladder: the effect of inflammation and edema on identification of malignancy. IEEE Trans. Med. Imaging 21, 708–710. doi: 10.1109/TMI.2002.800608
Song, J., Chen, R., Yang, L., Zhang, G., Li, W., Zhao, Z., et al. (2018). Electrical impedance changes at different phases of cerebral edema in rats with ischemic brain injury. BioMed. Res. Int. 2018:9765174. doi: 10.1155/2018/9765174
Tang, C., You, F., Cheng, G., Gao, D., Fu, F., and Dong, X. (2009). Modeling the frequency dependence of the electrical properties of the live human skull. Physiol. Meas. 30, 1293–1301. doi: 10.1088/0967-3334/30/12/001
Villafane, J. H., Gobbo, M., Peranzoni, M., Naik, G., Imperio, G., Cleland, J. A., et al. (2016). Validity and everyday clinical applicability of lumbar muscle fatigue assessment methods in patients with chronic non-specific low back pain: a systematic review. Disabil. Rehabil. 38, 1859–1871. doi: 10.3109/09638288.2015.1107777
Wang, H., He, Y., Yan, Q., You, F., Fu, F., Dong, X., et al. (2015). Correlation between the dielectric properties and biological activities of humanex vivohepatic tissue. Phys. Med. Biol. 60, 2603–2617.
Wang, Y., Freedman, L., Buck, M., Bohorquez, J., Rutkove, S. B., and Keel, J. (2019). Electrical impedance myography for assessing paraspinal muscles of patients with low back pain. J. Electr. Bioimpedance 10, 103–109. doi: 10.2478/joeb-2019-0015
Wu, A., March, L., Zheng, X., Huang, J., Wang, X., Zhao, J., et al. (2020). Global low back pain prevalence and years lived with disability from 1990 to 2017: estimates from the global of disease study 2017. Ann. Transl. Med. 8:299.
Yamamoto, N., Itoi, E., Minagawa, H., Seki, N., Abe, H., Shimada, Y., et al. (2006). Objective evaluation of shoulder pain by measuring skin impedance. Orthopedics 29, 1121–1123. doi: 10.3928/01477447-20061201-14
Yamamoto, T., and Yamamoto, Y. (1976). Electrical properties of the epidermal stratum corneum. Med. Biol. Eng. 14, 151–158.
Yang, L., Dai, M., Wang, H., Cao, X., Li, S., Fu, F., et al. (2021). A wireless, low-power, and miniaturized EIT system for remote and long-term monitoring of lung ventilation in the isolation ward of ICU. IEEE Trans. Instrum. Meas. 70, 1–11.
Yang, L., Liu, W., Chen, R., Zhang, G., Li, W., Fu, F., et al. (2017b). In vivo bioimpedance spectroscopy characterization of healthy, hemorrhagic and ischemic rabbit brain within 10 Hz-1 MHz. Sensors (Basel) 17:791. doi: 10.3390/s17040791
Yang, L., Dai, M., Xu, C., Zhang, G., Li, W., Fu, F., et al. (2017a). The frequency spectral properties of electrode-skin contact impedance on human head and its frequency-dependent effects on frequency-difference EIT in stroke detection from 10Hz to 1MHz. PLoS One 12:e0170563. doi: 10.1371/journal.pone.0170563
Yang, L., Zhang, G., Song, J., Dai, M., Xu, C., Dong, X., et al. (2016). Ex-vivo characterization of bioimpedance spectroscopy of normal, ischemic and hemorrhagic rabbit brain tissue at frequencies from 10 Hz to 1 MHz. Sensors (Basel) 16:1942. doi: 10.3390/s16111942
Keywords: low back pain, pilot, electrical bio-impedance, state of lumbar muscle, assessment
Citation: Wang H, Dai J, Wang C, Gao Z, Liu Y, Dai M, Zhao Z, Yang L and Tan G (2022) Assessment of Low Back Pain in Helicopter Pilots Using Electrical Bio-Impedance Technique: A Feasibility Study. Front. Neurosci. 16:883348. doi: 10.3389/fnins.2022.883348
Received: 11 March 2022; Accepted: 07 June 2022;
Published: 14 July 2022.
Edited by:
Aydin Farajidavar, New York Institute of Technology, United StatesReviewed by:
Shahriar Gharibzadeh, Shahid Beheshti University, IranNikunj Arunkumar Bhagat, University of Texas Health Science Center at Houston, United States
Copyright © 2022 Wang, Dai, Wang, Gao, Liu, Dai, Zhao, Yang and Tan. This is an open-access article distributed under the terms of the Creative Commons Attribution License (CC BY). The use, distribution or reproduction in other forums is permitted, provided the original author(s) and the copyright owner(s) are credited and that the original publication in this journal is cited, in accordance with accepted academic practice. No use, distribution or reproduction is permitted which does not comply with these terms.
*Correspondence: Lin Yang, yanglin.0601@163.com; Guodong Tan, nzt539@163.com
†These authors have contributed equally to this work