- 1School of Physical Education and Sports Exercise, South China Normal University, Guangzhou, China
- 2National Demonstration Center for Experimental Sports Science Education, South China Normal University, Guangzhou, China
- 3Department of Radiology, The Second People’s Hospital of Yibin, Yibin, China
- 4Department of Radiology, Huaxi MR Research Center (HMRRC), West China Hospital of Sichuan University, Chengdu, China
- 5Key Laboratory for Neuroinformation of Ministry of Education, School of Life Sciences and Technology, University of Electronic Science and Technology of China, Chengdu, China
- 6Research Unit of Psychoradiology, Chinese Academy of Medical Sciences, Chengdu, China
Background: Our previous reports reflected some aspects of neuroplastic changes from long-term Chinese chess training but were mainly based on large-scale intrinsic connectivity. In contrast to functional connectivity among remote brain areas, synchronization of local intrinsic activity demonstrates functional connectivity among regional areas. Until now, local connectivity changes in professional Chinese chess players (PCCPs) have been reported only at specific hubs; whole-brain-based local connectivity and its relation to training profiles has not been revealed.
Objectives: To investigate whole-brain local connectivity changes and their relation to training profiles in PCCPs.
Methods: Regional homogeneity (ReHo) analysis of rs-fMRI data from 22 PCCPs versus 21 novices was performed to determine local connectivity changes and their relation to training profiles.
Results: Compared to novices, PCCPs showed increased regional spontaneous activity in the posterior lobe of the left cerebellum, the left temporal pole, the right amygdala, and the brainstem but decreased ReHo in the right precentral gyrus. From a whole-brain perspective, local activity in areas such as the posterior lobe of the right cerebellum and the caudate correlated with training profiles.
Conclusion: Regional homogeneity changes in PCCPs were consistent with the classical view of automaticity in motor control and learning. Related areas in the pattern indicated an enhanced capacity for emotion regulation, supporting cool and focused attention during gameplay. The possible participation of the basal ganglia-cerebellar-cerebral networks, as suggested by these correlation results, expands our present knowledge of the neural substrates of professional chess players. Meanwhile, ReHo change occurred in an area responsible for the pronunciation and reading of Chinese characters. Additionally, professional Chinese chess training was associated with change in a region that is affected by Alzheimer’s disease (AD).
Introduction
Chess serves studies in cognitive science as Drosophila serves studies in biological science. Many processes, such as perception (Sheridan and Reingold, 2017), memory (Gong et al., 2015), problem solving (Pereira et al., 2020), and empathy (Powell et al., 2017), become more apparent in the classical research paradigm of chess. With the application of non-invasive imaging techniques (Nichelli et al., 1994; Onofrj et al., 1995; Amidzic et al., 2001; Silva et al., 2018; Fuentes-García et al., 2019; Pereira et al., 2020) and especially functional magnetic resonance imaging (fMRI) (Atherton et al., 2003; Bilalić et al., 2011; Wan et al., 2011; Duan et al., 2014; Hänggi et al., 2014; Premi et al., 2020; Wang et al., 2020) in the human brain, neural substrates of cognitive processes have gradually been revealed. In studies investigating these substrates, cognitive research on expertise was a main research domain. Based on the theory of chunks in chess experts (Chase and Simon, 1973), the medial temporal lobe was initially revealed as the basis of long-term memory (LTM) in chess experts (Amidzic et al., 2001), and then the caudate region was comprehensively shown to be responsible for automatically producing the best next move in board games (Wan et al., 2011). Specific regions have long been believed to form the basis of cognitive expertise in board games. However, from the investigation of functional connectivity between the caudate and the default mode network (DMN) (Duan et al., 2012a), step-by-step exploration of specific region-based functional connections was applied (Duan et al., 2014; Sohn et al., 2017; Song et al., 2020; Wang et al., 2020). Recently, whole-brain-based detection of brain functional connectivity was reported as a dynamic functional network characteristic of Chinese chess experts (Premi et al., 2020).
Since the 1st China National Mind Sports Games in 2010 in Chengdu, China, the brain characteristics associated with cognitive expertise in Chinese chess have been discussed throughout the scientific world (e.g., Duan et al., 2012a,b, 2014; Premi et al., 2020; Song et al., 2020; Wang et al., 2020). Chinese chess (Xiangqi in Chinese) is a traditional board game originating from military strategies in ancient China. To the best of our knowledge, this game was first introduced in an English publication in 1895 (Platt, 1895). As in chess, the records of professional players in each competition are compiled to assign ranking points to each player, reflecting the person’s skill level. Xiangqi is remarkable among board games in that its famous endgame problems [such as “wild horses run on the farm” (Hung et al., 2017)], moving rules (such as “the horse moves in the shape of the character “RI” and “the elephant moves in the shape of the character “TIAN”)1 and combat strategies (such as “dāng tóu pào, m ǎ lái tiào”; See text footnote) are described and taught in vivid sentences (“rhymes” or “sayings”) or descriptive battle stories, which may explain the special relationship of this game to the cognitive processes of Chinese language cognition, sematic memory (SM) and episodic memory (EM). Changes in SM and EM are both early markers of Alzheimer’s disease (AD) (Marra et al., 2016; Gagliardi et al., 2019; Venneri et al., 2019). Recently, some reports have discussed the AD-preventive effects of board games (Nakao, 2019; Qureshi, 2019). For example, a previous randomized clinical trial of 147 AD patients reported that AD symptoms were reduced in patients who played the game of Go (Lin et al., 2015).
Our previous studies (Duan et al., 2012a,b, 2014) reported morphological changes in the caudate, the enhanced connectivity of the caudate to the DMN, and remote functional connectivity alterations including different global topological properties of the whole-brain functional networks and intrinsic brain networks. These reports reflected some aspects of neuroplastic changes from long-term Chinese chess training but were mainly based on large-scale intrinsic connectivity. In contrast to functional connectivity among remote brain areas, synchronization of local intrinsic activity demonstrates functional connectivity among regional areas (Jia et al., 2017). Until now, local connectivity changes in professional Chinese chess players (PCCPs) have been reported only at specific hubs (Song et al., 2020); whole-brain-based local connectivity and its relation to training profiles has not been revealed.
Regional homogeneity (ReHo), revealing the homogeneous characteristics of local brain activity, is one kind of postprocessing method of local spontaneous activity. ReHo is based on Kendall’s coefficient concordance (KCC) to measure the similarity of the time series of a given voxel to those of its nearest neighbors in a voxel-wise way in rs-fMRI analysis (Zang et al., 2004). Recently, some reports (e.g., Jiang et al., 2015; Jiang and Zuo, 2016) revealed the neurobiological relevance underlying ReHo, including anatomical morphology, brain development, and neurocognitive factors. Therefore, ReHo was confirmed to be a useful neuroimaging tool to understand human brain function (He et al., 2007; Wang et al., 2011; Wu et al., 2011; Dai et al., 2012; Tian et al., 2012; Dong et al., 2014; Liu et al., 2015; Lv et al., 2019). As one morphological change typical of PCCPs was detected in the caudate (Duan et al., 2012a), which subserves the associative phase of cognitive procedural learning (Chiu et al., 2017), we might expect ReHo changes to be mostly similar to their structural and functional equivalents in neuroimaging studies that have revealed training-specific areas.
In this study, ReHo analysis was performed based on rs-fMRI data to explore the whole-brain local functional connectivity changes in PCCPs and the relation of these changes to training profiles.
Materials and Methods
Participants
A total of 43 subjects were included in the present study. One group included 22 PCCPs (14 males and 8 females; age, 27.32 ± 8.31 years; years of education, 13.45 ± 2.37; rating points, 2410 ± 116; professional training years, 10 ± 9.32; and professional training hours per day, 4.25 ± 1.82). Another group included 21 novices (13 males and 8 females; age, 26.20 ± 8.17 years; years of education, 13.38 ± 3.37) who knew the rules of the game and simple strategies but with no game experience (Campitelli et al., 2005). PCCPs and novices were sex-, education- and age-matched. To further examine the difference between PCCPs and novices, both groups were tested by Raven’s Standard Progressive Matrices, and two groups did not differ on general intelligence (P = 0.63, two tailed t-test). All participants had normal or corrected-to-normal vision. Written informed consent was obtained from all subjects. The proposal was approved by the local Ethics Committee of Huaxi Hospital, Sichuan University.
Data Acquisition
Images were acquired using a 3.0T Siemens Magnetom Trio scanner in the Huaxi MR Research Center. Functional images were acquired using a single-shot, gradient-recalled echo-planar imaging sequence [repetition time (TR) = 2,000 ms, echo time (TE) = 30 ms and flip angle = 90^°]. Thirty transverse slices [field of view (FOV) = 24 cm, in-plane matrix = 64 × 64, slice thickness = 5 mm, without gap, voxel size = 3.8 × 3.8 × 5] and 205 volumes were obtained from each subject. The first five volumes were discarded to ensure steady-state longitudinal magnetization. During the scanning procedure, a standard head coil with foam padding was used to restrict head motion. Subjects were instructed simply to rest with their eyes closed, not to think of anything in particular and not to fall asleep.
Data Analysis
Image pre-processing was performed using SPM8 software.2 The first five volumes were discarded to ensure steady-state longitudinal magnetization. The remaining 200 volumes were first corrected for the temporal difference and head motion. In this study, the threshold for head motion was lower than ±1.5 mm or ±1.5^°. We calculated frame-wise displacement (FD) which reflected the head movement at every different time point by employing 6 displacements from the rigid body motion correction procedure (Power et al., 2012), and found no significant differences (P = 0.82) in FD between PCCPs (0.159 ± 0.16 mm) and novices (0.150 ± 0.07 mm) using two-sample t-tests. The resulting images were then normalized to the standard SPM8 echo-planar imaging template and resampled to a standard stereotaxic space at a resolution of 3 mm × 3 mm × 3 mm. Finally, the normalized images were temporally band-pass filtered (0.01 < f < 0.08 Hz) to reduce the effects of low-frequency drift and high-frequency physiology noise (Biswal et al., 1995); additionally, the linear trend was removed.
Regional Homogeneity Analysis
The KCC (Kendall and Gibbons, 1990) was calculated to measure the similarity of the time series of a defined cluster. In the present study, 27 nearest neighbor voxels were defined as a cluster. The KCC was given to the center voxel (Zang et al., 2004) as follows:
where W is the KCC among given voxels, ranging from 0 to 1; Ri is the sum rank of the ith time point; is the mean of the Ri values; K is the number of time series points within a measured cluster; and n is the number of ranks (here, n = 200 time points). REST software (Resting-state fMRI data analysis toolkit)3 was used to calculate individual ReHo values in a voxel-wise way. Each individual ReHo map was divided by that subject’s global mean KCC value within the brain mask. The ReHo maps were then spatially smoothed with a Gaussian filter of 8 mm of full width at half maximum (FWHM).
Second-Level Analysis
Group statistical analysis was performed in SPM8. The one-sample t-tests results from the two groups were combined to get a new map. By binarizing the map, a combined explicit mask was obtained. The significance threshold was set at P < 0.01, corrected by the false discovery rate (FDR) criterion (Genovese et al., 2002).
Then, two-sample t-tests were performed to show the between-group difference in ReHo. The t-map was created with a combined threshold of P < 0.005 and a minimum cluster size of 46 voxels using the AlphaSim program in REST software. This approach applied Monte Carlo simulation to calculate the probability of false positive detection by taking into consideration both the individual voxel probability threshold and cluster size.
Additionally, to explore whether ReHo correlates with the rating points, years of professional training and training intensity of PCCPs, a correlation analysis of ReHo versus these training profiles was performed in this group for each voxel of the whole brain. For the correlation analyses, we set the significance threshold at P < 0.05 (combined threshold of P < 0.001 and a minimum cluster size of 20 voxels, corrected by AlphaSim).
Results
Within-Group Results
In order to intuitively display ReHo results for Chinese chess novices and PCCPs, a ReHo map was calculated within each group and shown in Figure 1 (one-sample t-test; P < 0.01, corrected by FDR). For visual observation, areas in the DMN (Raichle et al., 2001) including the posterior cingulate cortex/precuneus (PCC/Pcu), medial prefrontal cortex (MPFC) and bilateral inferior parietal lobe (IPL) displayed significantly greater ReHo than other regions.
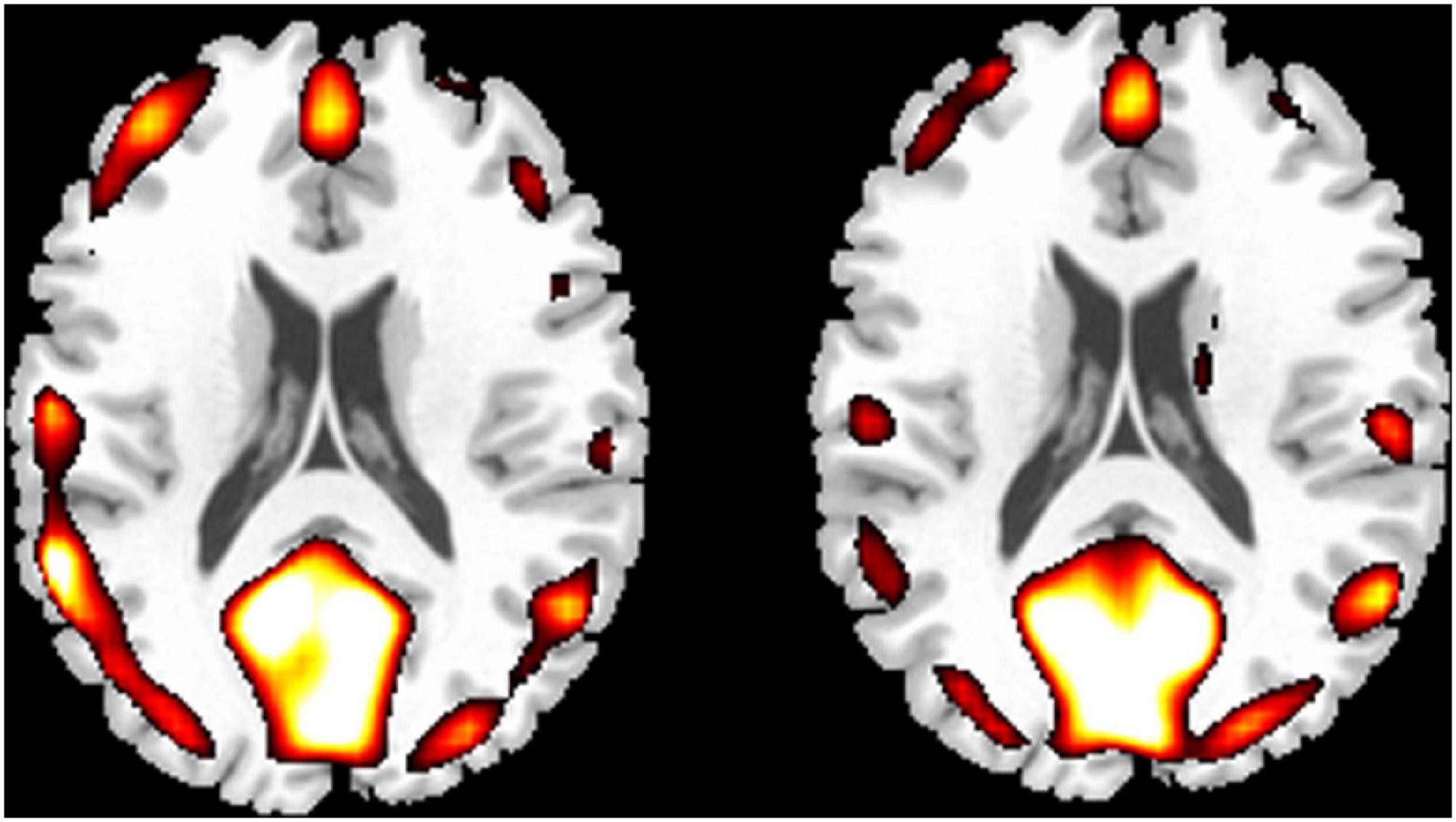
Figure 1. One-sample results for novices (left column) and professional Chinese chess players (PCCPs) (right column). The regions indicated by a warm color showed greater regional homogeneity (ReHo). The threshold was P < 0.01, FDR corrected. The left side of the image corresponds to the left side of the brain.
Between-Group Results
Compared with the novices, PCCPs revealed increased ReHo in the left cerebellum posterior lobe, left temporal pole, right amygdala, and brainstem and decreased ReHo in the right precentral gyrus (two-sample t-test, P < 0.05, corrected by AlphaSim; Figure 2 and Table 1).
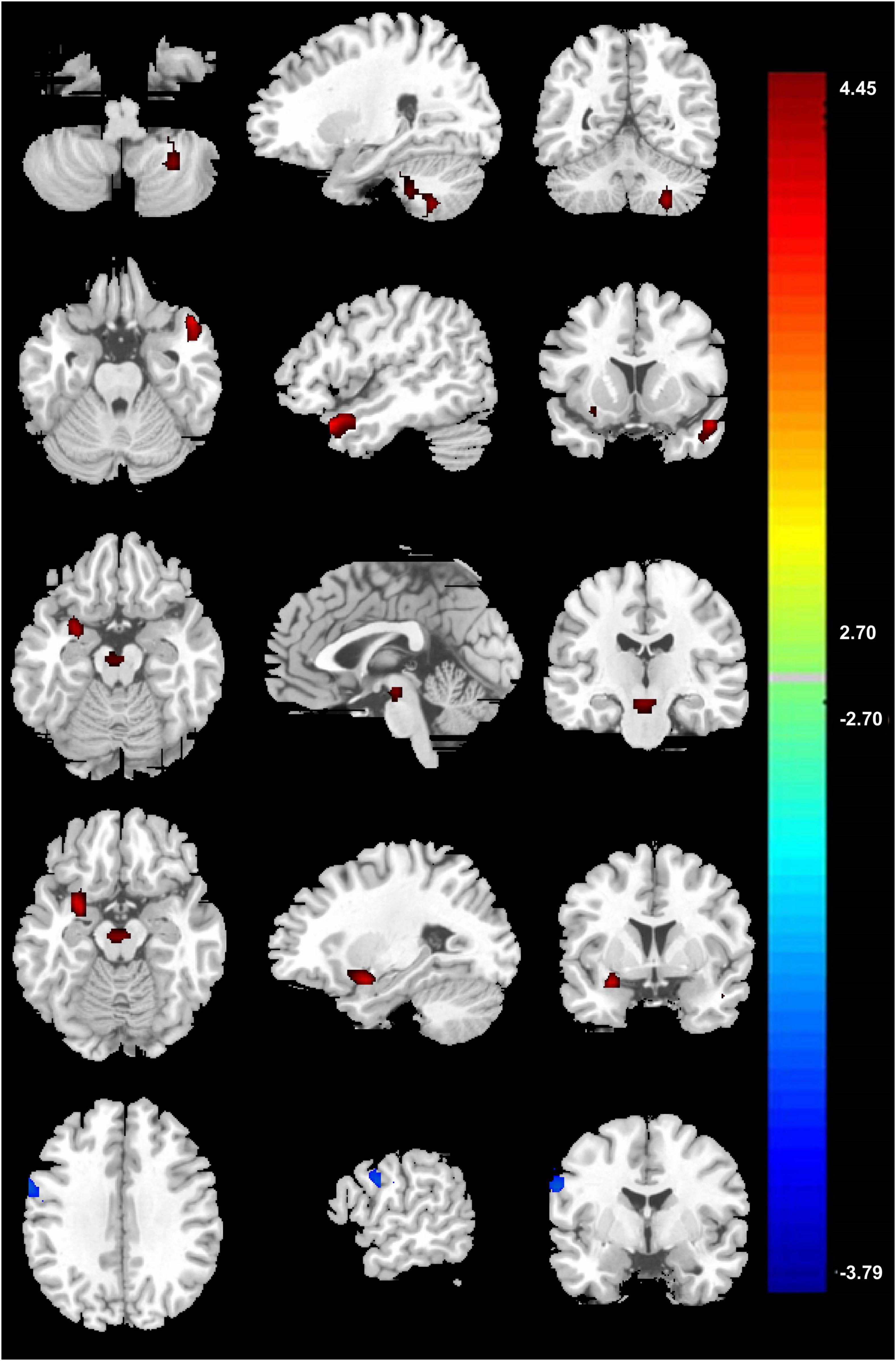
Figure 2. Two-sample result between PCCPs and novices (P < 0.05, AlphaSim corrected, a combined threshold of P < 0.005, and a minimum cluster size of 46 voxels). Hot and cold colors indicate ReHo increases and decreases in PCCPs, respectively, and the number indicates the t-value. The left and right sides in this figure correspond to the right and left sides of the brain, respectively. Further details of these regions are presented in Table 1.
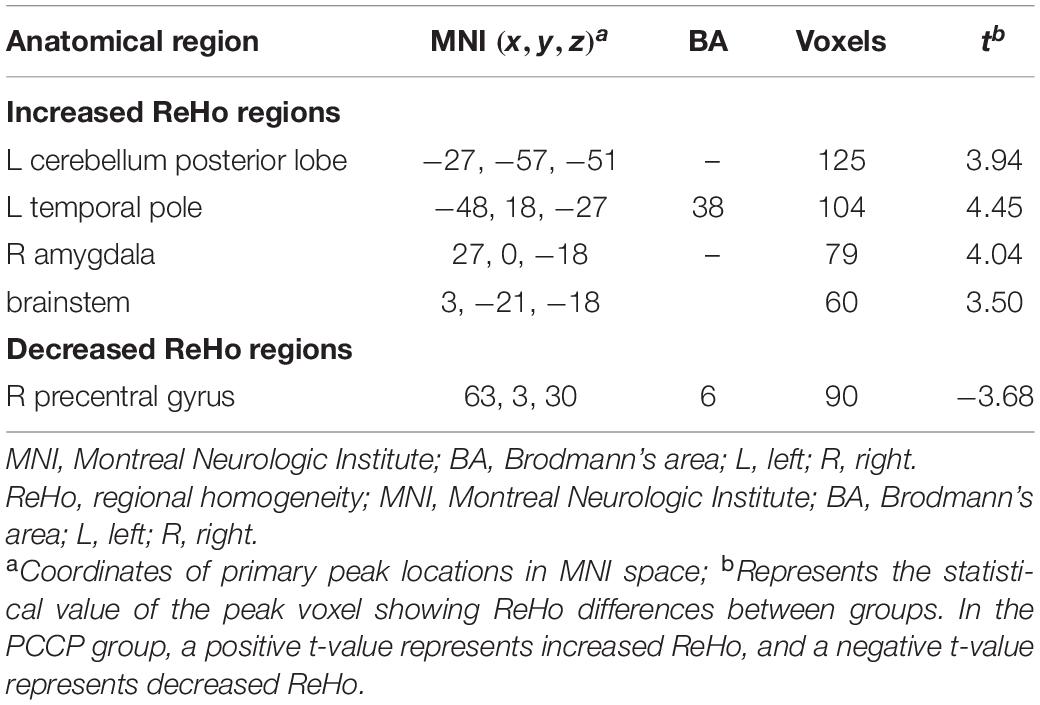
Table 1. Comparison of regions with increased/decreased regional homogeneity (ReHo) in professional Chinese chess players (PCCPs) compared to novices.
Correlational Results
In the voxel-based group comparisons of ReHo maps, we chose a statistical threshold at voxel level P < 0.05 (AlphaSim corrected) with a minimum cluster size of 20 voxels to reduce Type I errors, resulting in a combined threshold of P < 0.001. The correlation of ReHo for each whole-brain voxel against rating points of PCCPs showed a significantly positive correlation in the right precentral gyrus and a significantly negative correlation in the left PCC/Pcu and right middle temporal gyrus (MTG). The professional training years of PCCPs were negatively correlated with the left SMA and right cerebellum posterior lobe. The ReHo of the right caudate was negatively correlated with training hours. Please see Figure 3 and Table 2 for more details.

Figure 3. Significant correlations between ReHo and training profiles in PCCPs (P < 0.05, AlphaSim corrected, a combined threshold of P < 0.001, and a minimum cluster size of 20 voxels). Hot and cold colors indicate positive and negative correlations, respectively. The numbers on the right color-bar refer to the t-value. The left and right sides in this figure correspond to the right and left sides of the brain, respectively. Further details of these regions are presented in Table 2.
Discussion
Neuroscience studies have investigated the neural substrates of motor control and learning, which has shed light on the neural mechanism of expertise. From cognitive processes to associative processes and to automatic processes, changing patterns of skill performance are theoretically described; the main features of the automatic process are “rapid, smooth, effortless, demand little intentional capacity and difficult to consciously disrupt” (Yarrow et al., 2009). In the classical view of automaticity, it is believed that as long-term practice makes skills reflexive, subcortical structures primarily activate, whereas novel behaviors require attention and flexible thinking that depend on the cortex (Ashby et al., 2010). Pertinently, in reports on the brains of chess experts, this behavior was also described as follows: “the pattern of activation moves from frontal parts at the beginning of the process to posterior parts responsible for retrieval of domain specific knowledge around the final expertise stage” (Debarnot et al., 2014). In between-group analyses of this study, the ReHo of PCCPs decreased in the precentral gyrus but increased in the cerebellum, temporal pole, amygdala and brainstem; this pattern of activity was similar to the above-described changes.
A consensus has been reached on the relation between the cerebellum and emotion (Adamaszek et al., 2017). In addition to the cerebellum posterior lobe, we located areas of increased spontaneous brain activity in the temporal pole, amygdala and brainstem. The amygdala reflects emotion (Weymar and Schwabe, 2016), especially emotional regulation (Li et al., 2016; Morawetz et al., 2017) and cognitive reappraisal (d’Arbeloff et al., 2018). Notably, there is also a relation between the brainstem and emotion (Venkatraman et al., 2017). Also, the temporal pole is reported in the emotion of aggression (Breitschuh et al., 2018). As one dimension of personality, emotional expression control was once reported to incrementally contribute to the prediction of chess playing strength (Grabner et al., 2007). A randomized controlled trial protocol described a Go intervention programme designed to enhance elementary school students’ cognitive function and their capacity for emotional and behavioral control (Tachibana et al., 2012). In the current study, we deduce the possibility of enhanced emotion regulation function that results from long-term Chinese chess training, indicating superior emotion regulation ability that supports cool and focused attention in PCCPs during gameplay.
An ordinal consensus supports functional interactions between the basal ganglia and cortex and between the cerebellum and cortex. In the consensus view, the basal ganglia and the cerebellum are reported to form a densely interconnected network, namely, the basal ganglia-cerebellar-cerebral cortical networks, in which the caudate, different parts of the cerebellum and cortex form different networks supplying a neural basis for cognition (such as executive function) as well as for neuropsychiatric disorders (such as AD and anxiety) (Bostan and Strick, 2018). In addition to the negative correlation between the right caudate and training hours each day, we found a negative correlation between the right posterior lobe of the cerebellum and length of professional training and a positive correlation between the right precentral gyrus and rating points. All these findings demonstrate a similar pattern of concordant changes in some brain regions, such as the basal ganglia-cerebellar-cerebral networks in the right hemisphere, among PCCPs. These results add to our present knowledge of the neural substrates of professional chess players. Prior to this study, the caudate had been revealed initially as a neural substrate (Wan et al., 2011; Duan et al., 2012a,b), and the thalamus was recently reported as a neural substrate (as its mediation between the caudate and frontal cortex) (Wang et al., 2020). The present study reflects the participation of the basal ganglia-cerebellar-cerebral networks.
Previous studies reported that as motor skills became automatic, the activation of the SMA decreased (Poldrack et al., 2005; Puttemans et al., 2005). Reports from sequence training, task training and practice processes demonstrate a similar changing trend in the SMA (Nyberg et al., 2006; Hatanaka et al., 2009; Ma et al., 2010). Our result of a negative correlation between the SMA and training years is parallel to these conclusions. In addition to its role in training-specific plasticity, the left SMA plays an important role in phonological processing in Chinese language cognition (Kuo et al., 2004; Veroude et al., 2010); the left SMA also takes part in Chinese character reading (Tan et al., 2000; Kuo et al., 2003). Although the correlation between this training-specific area and language cognition is a novel finding in the study of the neural basis of board game experts, a previous report has already shown such a relationship in brain region mainly contributing to corresponding action to language understanding. For example, Beilock et al. (2008) reported that the left BA6 (dorsal lateral premotor cortex), as a region normally devoted to higher-level action selection and implementation, also supported specialized motor (sports) experience enhancing action-related language understanding even when there was no intention to perform a real action.
Chunking theory-induced neuroscience investigations have indicated that LTM chunks of domain-specific information are stored in the ventral areas of the temporal lobe, including the parahippocampal gyrus (PHG) and fusiform gyrus (Campitelli et al., 2007). The PHG plays an important role in EM (Di Paola et al., 2007; Gallagher and Koh, 2011); changes in EM serve as the earliest and “marker” cognitive function alterations in AD (Bäckman et al., 2001; Aretouli and Brandt, 2010; Gallagher and Koh, 2011; Marra et al., 2016; Gagliardi et al., 2019). Moreover, the connection of the PHG with the PCC/Pcu and MTG was positively correlated with the Mini-Mental State Examination (MMSE) score, indicating functional connectivity that reflects the progression of cognitive degeneration disease. Pertinently, the PHG is important in mediating the connectivity between the hippocampus and hubs of the DMN as well as the connection between the MTG memory system and the DMN (Liu et al., 2016). Both neuropathological (Van Hoesen et al., 2000) and structural MRI (Pantel et al., 2003) evidence have demonstrated that selective morphological brain changes and atrophy in the PHG represent a preclinical stage of AD. Recently, Jia et al. (2017) reported a link between local synchronization alterations in the PHG and APOE-related cerebral physiological heterogeneity. All related findings demonstrated the possibility that plastic ReHo changes in the PHG by professional Chinese chess training may indicate its role in AD prevention. A 5.1-year study of 469 elderly individuals, among whom dementia developed in 124 subjects (of which more than fifty percent developed AD), found that playing board games was associated with a reduced risk of dementia (Verghese et al., 2003). This protection was later explained by the cognitive reserve theory that the number and strength of neuronal connections in the brain could be increased by training; the more connections were built up, the larger reserves to counteract the rate at which neurons were disappearing from the brain as these neurons were destroyed by AD pathology (Marx, 2005). In this study, a negative correlation was found between ReHo of the right MTG and rating points, which demonstrates the relationship between the combined function of the PHG and fusiform gyrus and training-specific experiences among PCCPs.
Conclusion
Through the comparison of ReHo analysis of rs-fMRI data between PCCPs and novices, training-specific whole-brain local connectivity changes in PCCPs were revealed. Brain ReHo of PCCPs demonstrated a similar changing pattern described in the classical view of automaticity in motor control and learning. Besides, some ReHo changes occurred in an area responsible for Chinese character pronouncing and reading. Moreover, professional Chinese chess training induced ReHo changes located in AD-related areas, which suggested the possibility of AD preventive effects from Chinese chess training. Findings from this study demonstrate the feasibility of using ReHo as a research tool to monitor board game-induced brain plastic changes and shed light on the neural substrates underlying cognition in Chinese chess playing.
Data Availability Statement
Data are available in a public, open access repository at the International Neuroimaging Data-Sharing Initiative or INDI (http://fcon_1000.projects.nitrc.org/index.html).
Ethics Statement
The studies involving human participants were reviewed and approved by the Local Ethics Committee of Huaxi Hospital, Sichuan University. The participants provided their written informed consent to participate in this study.
Author Contributions
All authors listed have made a substantial, direct, and intellectual contribution to the work, and approved it for publication.
Funding
This study was supported by the National Key Research and Development Project (2017YFB0403800), Natural Science Foundation of China (31560286), and China Scholarship Council (201906755035).
Conflict of Interest
The authors declare that the research was conducted in the absence of any commercial or financial relationships that could be construed as a potential conflict of interest.
Publisher’s Note
All claims expressed in this article are solely those of the authors and do not necessarily represent those of their affiliated organizations, or those of the publisher, the editors and the reviewers. Any product that may be evaluated in this article, or claim that may be made by its manufacturer, is not guaranteed or endorsed by the publisher.
Acknowledgments
We are grateful to all the professional Chinese chess players and control volunteers who took part in the experiment. We especially thank Chinese Chess International Grandmaster Dahua Liu for his kindly support and insightful suggestions.
Footnotes
- ^ https://en.wikipedia.org/wiki/Xiangqi
- ^ http://www.fil.ion.ucl.ac.uk/spm
- ^ http://sourceforge.net/projects/resting-fmri
References
Adamaszek, M., D’Agata, F., Ferrucci, R., Habas, C., Keulen, S., Kirkby, K. C., et al. (2017). Consensus paper: cerebellum and emotion. Cerebellum 16, 552–576. doi: 10.1007/s12311-016-0815-8
Amidzic, O., Riehle, H. J., Fehr, T., Wienbruch, C., and Elbert, T. (2001). Pattern of focal gamma-bursts in chess players. Nature 412:603. doi: 10.1038/35088119
Aretouli, E., and Brandt, J. (2010). Episodic memory in dementia: characteristics of new learning that differentiate Alzheimer’s, Huntington’s, and Parkinson’s diseases. Arch. Clin. Neuropsychol. 25, 396–409. doi: 10.1093/arclin/acq038
Ashby, F. G., Turner, B. O., and Horvitz, J. C. (2010). Cortical and basal ganglia contributions to habit learning and automaticity. Trends Cogn. Sci. 14, 208–215. doi: 10.1016/j.tics.2010.02.001
Atherton, M., Zhuang, J., Bart, W. M., Hu, X., and He, S. (2003). A functional MRI study of high-level cognition. I. The game of chess. Brain Res. Cogn. Brain Res. 16, 26–31. doi: 10.1016/s0926-6410(02)00207-0
Bäckman, L., Small, B. J., and Fratiglioni, L. (2001). Stability of the preclinical episodic memory deficit in Alzheimer’s disease. Brain 124, 96–102. doi: 10.1093/brain/124.1.96
Beilock, S. L., Lyons, I. M., Mattarella-Micke, A., Nusbaum, H. C., and Small, S. L. (2008). Sports experience changes the neural processing of action language. Proc. Natl. Acad. Sci. U.S.A. 105, 13269–13273. doi: 10.1073/pnas.0803424105
Bilalić, M., Langner, R., Ulrich, R., and Grodd, W. (2011). Many faces of expertise: fusiform face area in chess experts and novices. J. Neurosci. 31, 10206–10214. doi: 10.1523/JNEUROSCI.5727-10.2011
Biswal, B., Yetkin, F. Z., Haughton, V. M., and Hyde, J. S. (1995). Functional connectivity in the motor cortex of resting human brain using echo-planar MRI. Magn. Reson. Med. 34, 537–541. doi: 10.1002/mrm.1910340409
Bostan, A. C., and Strick, P. L. (2018). The basal ganglia and the cerebellum: nodes in an integrated network. Nat. Rev. Neurosci. 19, 338–350. doi: 10.1038/s41583-018-0002-7
Breitschuh, S., Schöne, M., Tozzi, L., Kaufmann, J., Strumpf, H., Fenker, D., et al. (2018). Aggressiveness of martial artists correlates with reduced temporal pole grey matter concentration. Psychiatry Res. Neuroimaging 281, 24–30. doi: 10.1016/j.pscychresns.2018.08.001
Campitelli, G., Gobet, F., Head, K., Buckley, M., and Parker, A. (2007). Brain localization of memory chunks in chessplayers. Int. J. Neurosci. 117, 1641–1659. doi: 10.1080/00207450601041955
Campitelli, G., Gobet, F., and Parker, A. (2005). Structure and stimulus familiarity: a study of memory in chess-players with functional magnetic resonance imaging. Span. J. Psychol. 8, 238–245. doi: 10.1017/s1138741600005126
Chase, W. G., and Simon, H. A. (1973). Perception in chess. Cogn. Psychol. 4, 55–81. doi: 10.1016/0010-0285(73)90004-2
Chiu, Y. C., Jiang, J., and Egner, T. (2017). The caudate nucleus mediates learning of stimulus-control state associations. J. Neurosci. 37, 1028–1038. doi: 10.1523/JNEUROSCI.0778-16.2016
Dai, X. J., Gong, H. H., Wang, Y. X., Zhou, F. Q., Min, Y. J., Zhao, F., et al. (2012). Gender differences in brain regional homogeneity of healthy subjects after normal sleep and after sleep deprivation: a resting-state fMRI study. Sleep Med. 13, 720–727. doi: 10.1016/j.sleep.2011.09.019
d’Arbeloff, T. C., Kim, M. J., Knodt, A. R., Radtke, S. R., Brigidi, B. D., and Hariri, A. R. (2018). Microstructural integrity of a pathway connecting the prefrontal cortex and amygdala moderates the association between cognitive reappraisal and negative emotions. Emotion 18, 912–915. doi: 10.1037/emo0000447
Debarnot, U., Sperduti, M., Di Rienzo, F., and Guillot, A. (2014). Experts bodies, experts minds: how physical and mental training shape the brain. Front. Hum. Neurosci. 8:280. doi: 10.3389/fnhum.2014.00280
Di Paola, M., Macaluso, E., Carlesimo, G. A., Tomaiuolo, F., Worsley, K. J., Fadda, L., et al. (2007). Episodic memory impairment in patients with Alzheimer’s disease is correlated with entorhinal cortex atrophy. A voxel-based morphometry study. J. Neurol. 254, 774–781. doi: 10.1007/s00415-006-0435-1
Dong, M., Qin, W., Zhao, L., Yang, X., Yuan, K., Zeng, F., et al. (2014). Expertise modulates local regional homogeneity of spontaneous brain activity in the resting brain: an fMRI study using the model of skilled acupuncturists. Hum. Brain Mapp. 35, 1074–1084. doi: 10.1002/hbm.22235
Duan, X., He, S., Liao, W., Liang, D., Qiu, L., Wei, L., et al. (2012a). Reduced caudate volume and enhanced striatal-DMN integration in chess experts. Neuroimage 60, 1280–1286. doi: 10.1016/j.neuroimage.2012.01.047
Duan, X., Liao, W., Liang, D., Qiu, L., Gao, Q., Liu, C., et al. (2012b). Large-scale brain networks in board game experts: insights from a domain-related task and task-free resting state. PLoS One 7:e32532. doi: 10.1371/journal.pone.0032532
Duan, X., Long, Z., Chen, H., Liang, D., Qiu, L., Huang, X., et al. (2014). Functional organization of intrinsic connectivity networks in Chinese-chess experts. Brain Res. 1558, 33–43. doi: 10.1016/j.brainres.2014.02.033
Fuentes-García, J. P., Pereira, T., Castro, M. A., Carvalho Santos, A., and Villafaina, S. (2019). Heart and brain responses to real versus simulated chess games in trained chess players: a quantitative EEG and HRV study. Int. J. Environ. Res Public Health 16:5012. doi: 10.3390/ijerph16245021
Gagliardi, G., Epelbaum, S., Houot, M., Bakardjian, H., Boukadida, L., Revillon, M., et al. (2019). Which episodic memory performance is associated with Alzheimer’s disease biomarkers in elderly cognitive complainers? Evidence from a longitudinal observational study with four episodic memory tests (Insight-PreAD). J. Alzheimers Dis. 70, 811–824. doi: 10.3233/JAD-180966
Gallagher, M., and Koh, M. T. (2011). Episodic memory on the path to Alzheimer’s disease. Curr. Opin. Neurobiol. 21, 929–934. doi: 10.1016/j.conb.2011.10.021
Genovese, C. R., Lazar, N. A., and Nichols, T. (2002). Thresholding of statistical maps in functional neuroimaging using the false discovery rate. Neuroimage 15, 870–878. doi: 10.1006/nimg.2001.1037
Gong, Y., Ericsson, K. A., and Moxley, J. H. (2015). Recall of briefly presented chess positions and its relation to chess skill. PLoS One 10:e0118756. doi: 10.1371/journal.pone.0118756
Grabner, R. H., Stern, E., and Neubauer, A. C. (2007). Individual differences in chess expertise: a psychometric investigation. Acta Psychol. 124, 398–420. doi: 10.1016/j.actpsy.2006.07.008
Hänggi, J., Brütsch, K., Siegel, A. M., and Jäncke, L. (2014). The architecture of the chess player’s brain. Neuropsychologia 62, 152–162. doi: 10.1016/j.neuropsychologia.2014.07.019
Hatanaka, N., Tokuno, H., Nambu, A., and Takada, M. (2009). Transdural doppler ultrasonography monitors cerebral blood flow changes in relation to motor tasks. Cereb. Cortex 19, 820–831. doi: 10.1093/cercor/bhn129
He, Y., Wang, L., Zang, Y., Tian, L., Zhang, X., Li, K., et al. (2007). Regional coherence changes in the early stages of Alzheimer’s disease: a combined structural and resting-state functional MRI study. Neuroimage 35, 488–500. doi: 10.1016/j.neuroimage.2006.11.042
Hung, C., Guo, J., and Su, K. (2017). Based on short motion paths and artificial intelligence method for Chinese chess game. J. Robot. Netw. Artif. Life 4, 154–157. doi: 10.2991/jrnal.2017.4.2.11
Jia, X., Zhang, Y., Zang, Y., and Li, H. (2017). Frequency-dependent alterations in regional homogeneity in young carriers of the apolipoprotein E genotype. Sci. Bull. 62, 654–655. doi: 10.1016/j.scib.2017.03.019
Jiang, L., Xu, T., He, Y., Hou, X. H., Wang, J., Cao, X. Y., et al. (2015). Toward neurobiological characterization of functional homogeneity in the human cortex: regional variation, morphological association and functional covariance network organization. Brain Struct. Funct. 220, 2485–2507. doi: 10.1007/s00429-014-0795-8
Jiang, L., and Zuo, X. N. (2016). Regional homogeneity: a multimodal, multiscale neuroimaging marker of the human connectome. Neuroscientist 22, 486–505. doi: 10.1177/1073858415595004
Kuo, W. J., Yeh, T. C., Lee, C. Y., Yu, W., Chou, C. C., Ho, L. T., et al. (2003). Frequency effects of Chinese character processing in the brain: an event-related fMRI study. Neuroimage 18, 720–730. doi: 10.1016/s1053-8119(03)00015-6
Kuo, W. J., Yeh, T. C., Lee, J. R., Chen, L. F., Lee, P. L., Chen, S. S., et al. (2004). Orthographic and phonological processing of Chinese characters: an fMRI study. Neuroimage 21, 1721–1731. doi: 10.1016/j.neuroimage.2003.12.007
Li, Z., Tong, L., Guan, M., He, W., Wang, L., Bu, H., et al. (2016). Altered resting-state amygdala functional connectivity after real-time fMRI emotion self-regulation training. Biomed. Res. Int. 2016:2719895. doi: 10.1155/2016/2719895
Lin, Q., Cao, Y., and Gao, J. (2015). The impacts of a GO-game (Chinese chess) intervention on Alzheimer disease in a Northeast Chinese population. Front. Aging Neurosci. 7:163. doi: 10.3389/fnagi.2015.00163
Liu, C., Chen, Z., Wang, T., Tang, D., Hitchman, G., Sun, J., et al. (2015). Predicting stroop effect from spontaneous neuronal activity: a study of regional homogeneity. PLoS One 10:e0124405. doi: 10.1371/journal.pone.0124405
Liu, J., Zhang, X., Yu, C., Duan, Y., Zhuo, J., Cui, Y., et al. (2016). Impaired parahippocampus connectivity in mild cognitive impairment and Alzheimer’s disease. J. Alzheimers Dis. 49, 1051–1064. doi: 10.3233/JAD-150727
Lv, C., Wang, Q., Chen, C., Qiu, J., Xue, G., and He, Q. (2019). The regional homogeneity patterns of the dorsal medial prefrontal cortex predict individual differences in decision impulsivity. Neuroimage 200, 556–561. doi: 10.1016/j.neuroimage.2019.07.015
Ma, L., Wang, B., Narayana, S., Hazeltine, E., Chen, X., Robin, D. A., et al. (2010). Changes in regional activity are accompanied with changes in inter-regional connectivity during 4 weeks motor learning. Brain Res. 1318, 64–76. doi: 10.1016/j.brainres.2009.12.073
Marra, C., Gainotti, G., Fadda, L., Perri, R., Lacidogna, G., Scaricamazza, E., et al. (2016). Usefulness of an integrated analysis of different memory tasks to predict the progression from mild cognitive impairment to Alzheimer’s disease: the episodic memory score (EMS). J. Alzheimers Dis. 50, 61–70. doi: 10.3233/JAD-150613
Marx, J. (2005). Neuroscience. Preventing Alzheimer’s: a lifelong commitment. Science 309, 864–866. doi: 10.1126/science.309.5736.864
Morawetz, C., Bode, S., Baudewig, J., and Heekeren, H. R. (2017). Effective amygdala-prefrontal connectivity predicts individual differences in successful emotion regulation. Soc. Cogn. Affect. Neurosci. 12, 569–585. doi: 10.1093/scan/nsw169
Nakao, M. (2019). Special series on “effects of board games on health education and promotion” board games as a promising tool for health promotion: a review of recent literature. Biopsychosoc. Med. 13:5. doi: 10.1186/s13030-019-0146-3
Nichelli, P., Grafman, J., Pietrini, P., Alway, D., Carton, J. C., and Miletich, R. (1994). Brain activity in chess playing. Nature 369:191. doi: 10.1038/369191a0
Nyberg, L., Eriksson, J., Larsson, A., and Marklund, P. (2006). Learning by doing versus learning by thinking: an fMRI study of motor and mental training. Neuropsychologia 44, 711–717. doi: 10.1016/j.neuropsychologia.2005.08.006
Onofrj, M., Curatola, L., Valentini, G., Antonelli, M., Thomas, A., and Fulgente, T. (1995). Non-dominant dorsal-prefrontal activation during chess problem solution evidenced by single photon emission computerized tomography (SPECT). Neurosci. Lett. 198, 169–172. doi: 10.1016/0304-3940(95)11985-6
Pantel, J., Kratz, B., Essig, M., and Schröder, J. (2003). Parahippocampal volume deficits in subjects with aging-associated cognitive decline. Am. J. Psychiatry 160, 379–382. doi: 10.1176/appi.ajp.160.2.379
Pereira, T., Castro, M. A., Villafaina, S., Carvalho Santos, A., and Fuentes-García, J. P. (2020). Dynamics of the prefrontal cortex during chess-based problem-solving tasks in competition-experienced chess players: an fNIR study. Sensors 20:3917. doi: 10.3390/s20143917
Poldrack, R. A., Sabb, F. W., Foerde, K., Tom, S. M., Asarnow, R. F., Bookheimer, S. Y., et al. (2005). The neural correlates of motor skill automaticity. J. Neurosci. 25, 5356–5364. doi: 10.1523/JNEUROSCI.3880-04.2005
Powell, J. L., Grossi, D., Corcoran, R., Gobet, F., and García-Fiñana, M. (2017). The neural correlates of theory of mind and their role during empathy and the game of chess: a functional magnetic resonance imaging study. Neuroscience 355, 149–160. doi: 10.1016/j.neuroscience.2017.04.042
Power, J. D., Barnes, K. A., Snyder, A. Z., Schlaggar, B. L., and Petersen, S. E. (2012). Spurious but systematic correlations in functional connectivity MRI networks arise from subject motion. Neuroimage 59, 2142–2154. doi: 10.1016/j.neuroimage.2011.10.018
Premi, E., Gazzina, S., Diano, M., Girelli, A., Calhoun, V. D., Iraji, A., et al. (2020). Enhanced dynamic functional connectivity (whole-brain chronnectome) in chess experts. Sci. Rep. 10:7051. doi: 10.1038/s41598-020-63984-8
Puttemans, V., Wenderoth, N., and Swinnen, S. P. (2005). Changes in brain activation during the acquisition of a multifrequency bimanual coordination task: from the cognitive stage to advanced levels of automaticity. J. Neurosci. 25, 4270–4278. doi: 10.1523/JNEUROSCI.3866-04.2005
Qureshi, H. J. M. (2019). Phathophysiology of Alzheimer’s disease. J. Akhtar Saeed Med. Dent. College 1, 29–34.
Raichle, M. E., MacLeod, A. M., Snyder, A. Z., Powers, W. J., Gusnard, D. A., and Shulman, G. L. (2001). A default mode of brain function. Proc. Natl. Acad. Sci. U.S.A. 98, 676–682. doi: 10.1073/pnas.98.2.676
Sheridan, H., and Reingold, E. M. (2017). Chess players’ eye movements reveal rapid recognition of complex visual patterns: evidence from a chess-related visual search task. J. Vis. 17:4. doi: 10.1167/17.3.4
Silva, L. J., Cesar, F. H. G., Rocha, F. T., and Thomaz, C. E. (2018). A combined eye-tracking and EEG analysis on chess moves. IEEE Latin Am. Trans. Latin Am. Trans. 16, 1288–1297. doi: 10.1109/TLA.2018.8407099
Sohn, W. S., Lee, T. Y., Kwak, S., Yoon, Y. B., and Kwon, J. S. (2017). Higher extrinsic and lower intrinsic connectivity in resting state networks for professional Baduk (Go) players. Brain Behav. 7:e00853. doi: 10.1002/brb3.853
Song, L., Peng, Q., Liu, S., and Wang, J. (2020). Changed hub and functional connectivity patterns of the posterior fusiform gyrus in chess experts. Brain Imaging Behav. 14, 797–805. doi: 10.1007/s11682-018-0020-0
Tachibana, Y., Yoshida, J., Ichinomiya, M., Nouchi, R., Miyauchi, C., Takeuchi, H., et al. (2012). A GO intervention program for enhancing elementary school children’s cognitive functions and control abilities of emotion and behavior: study protocol for a randomized controlled trial. Trials 13:8. doi: 10.1186/1745-6215-13-8
Tan, L. H., Spinks, J. A., Gao, J. H., Liu, H. L., Perfetti, C. A., Xiong, J., et al. (2000). Brain activation in the processing of Chinese characters and words: a functional MRI study. Hum. Brain Mapp. 10, 16–27. doi: 10.1002/(SICI)1097-0193(200005)10:1<16::AID-HBM30>3.0.CO;2-M
Tian, L., Ren, J., and Zang, Y. (2012). Regional homogeneity of resting state fMRI signals predicts Stop signal task performance. Neuroimage 60, 539–544. doi: 10.1016/j.neuroimage.2011.11.098
Van Hoesen, G. W., Augustinack, J. C., Dierking, J., Redman, S. J., and Thangavel, R. (2000). The parahippocampal gyrus in Alzheimer’s disease. Clinical and preclinical neuroanatomical correlates. Ann. N. Y. Acad. Sci. 911, 254–274. doi: 10.1111/j.1749-6632.2000.tb06731.x
Venkatraman, A., Edlow, B. L., and Immordino-Yang, M. H. (2017). The brainstem in emotion: a review. Front. Neuroanat. 11:15. doi: 10.3389/fnana.2017.00015
Venneri, A., Mitolo, M., Beltrachini, L., Varma, S., Della Pietà, C., Jahn-Carta, C., et al. (2019). Beyond episodic memory: semantic processing as independent predictor of hippocampal/perirhinal volume in aging and mild cognitive impairment due to Alzheimer’s disease. Neuropsychology 33, 523–533. doi: 10.1037/neu0000534
Verghese, J., Lipton, R. B., Katz, M. J., Hall, C. B., Derby, C. A., Kuslansky, G., et al. (2003). Leisure activities and the risk of dementia in the elderly. N. Engl. J. Med. 348, 2508–2516. doi: 10.1056/NEJMoa022252
Veroude, K., Norris, D. G., Shumskaya, E., Gullberg, M., and Indefrey, P. (2010). Functional connectivity between brain regions involved in learning words of a new language. Brain Lang. 113, 21–27. doi: 10.1016/j.bandl.2009.12.005
Wan, X., Nakatani, H., Ueno, K., Asamizuya, T., Cheng, K., and Tanaka, K. (2011). The neural basis of intuitive best next-move generation in board game experts. Science 331, 341–346. doi: 10.1126/science.1194732
Wang, K. L., Zhang, H. G., Ping, Z. L., and Hai, Y. (2011). “Chinese chess character recognition with radial harmonic fourier moments,” in Proceedings of the 2011 International Conference on Document Analysis and Recognition, (Beijing: IEEE), 1369–1373.
Wang, Y., Zuo, C., Wang, D., Tao, S., and Hao, L. (2020). Reduced thalamus volume and enhanced thalamus and fronto-parietal network integration in the chess experts. Cereb. Cortex 30, 5560–5569. doi: 10.1093/cercor/bhaa140
Weymar, M., and Schwabe, L. (2016). Amygdala and emotion: the bright side of it. Front. Neurosci. 10:224. doi: 10.3389/fnins.2016.00224
Wu, Q. Z., Li, D. M., Kuang, W. H., Zhang, T. J., Lui, S., Huang, X. Q., et al. (2011). Abnormal regional spontaneous neural activity in treatment-refractory depression revealed by resting-state fMRI. Hum. Brain Mapp. 32, 1290–1299. doi: 10.1002/hbm.21108
Yarrow, K., Brown, P., and Krakauer, J. W. (2009). Inside the brain of an elite athlete: the neural processes that support high achievement in sports. Nat. Rev. Neurosci. 10, 585–596. doi: 10.1038/nrn2672
Keywords: board games, Chinese chess, regional homogeneity, automaticity, Chinese language cognition, AD prevention
Citation: Liang D, Qiu L, Duan X, Chen H, Liu C and Gong Q (2022) Training-Specific Changes in Regional Spontaneous Neural Activity Among Professional Chinese Chess Players. Front. Neurosci. 16:877103. doi: 10.3389/fnins.2022.877103
Received: 16 February 2022; Accepted: 29 April 2022;
Published: 27 May 2022.
Edited by:
Stephen J. Gotts, National Institute of Mental Health (NIH), United StatesCopyright © 2022 Liang, Qiu, Duan, Chen, Liu and Gong. This is an open-access article distributed under the terms of the Creative Commons Attribution License (CC BY). The use, distribution or reproduction in other forums is permitted, provided the original author(s) and the copyright owner(s) are credited and that the original publication in this journal is cited, in accordance with accepted academic practice. No use, distribution or reproduction is permitted which does not comply with these terms.
*Correspondence: Chengyi Liu, bGl1dGN5QHNjbnUuZWR1LmNu; Qiyong Gong, cWl5b25nZ29uZ0BobXJyYy5vcmcuY24=
†These authors have contributed equally to this work