- Department Neurology, Bethune First Hospital of Jilin University, Jilin University, Changchun, China
The transcriptional regulator nuclear factor kappa B (NF-κB) modulates cellular biological activity by binding to promoter regions in the nucleus and transcribing various protein-coding genes. The NF-κB pathway plays a major role in the expressing genes related to inflammation, including chemokines, interleukins, and tumor necrosis factor. It also transcribes genes that can promote neuronal survival or apoptosis. Epilepsy is one of the most common brain disorders and it not only causes death worldwide but also affects the day-to-day life of affected individuals. While epilepsy has diverse treatment options, there remain patients who are not sensitive to the existing treatment methods. Recent studies have implicated the critical role of NF-κB in epilepsy. It is upregulated in neurons, glial cells, and endothelial cells, due to neuronal loss, glial cell proliferation, blood-brain barrier dysfunction, and hippocampal sclerosis through the glutamate and γ-aminobutyric acid imbalance, ion concentration changes, and other mechanisms. In this review, we summarize the functional changes caused by the upregulation of NF-κB in the central nervous system during different periods after seizures. This review is the first to deconvolute the complicated functions of NF-κB, and speculate that the regulation of NF-κB can be a safe and effective treatment strategy for epilepsy.
Introduction
Epilepsy is among the most common brain disorders (Scheffer et al., 2017) and is characterized by repeated convulsions due to abnormal excitation of neurons (Wijnen et al., 2017). It is estimated that 50–70 million people (Trinka et al., 2019), accounting for approximately 1–2% of the world’s population, are affected by epilepsy. According to a survey, approximately 70% of people with epilepsy can have normal lives if they are adequately treated with anti-epileptic drugs (World Health Organization, 2005). However, anti-epileptic drugs used by patients block epileptic seizures but do not affect the underlying pathology or disease progression. Recently, some studies have highlighted the important pathophysiological role of inflammation in epilepsy (Paudel et al., 2019) and revealed that excessive activation of inflammatory pathways is a sign of epilepsy (Marcheselli and Bazan, 1996; Russmann et al., 2017). Studies have demonstrated that brain injury-induced inflammation and apoptosis are causative factors of epilepsy (Kwon et al., 2013); therefore, seizures can also cause the expression of inflammatory factors. These mediators can trigger the activation of the nuclear factor kappa B (NF-κB) pathway, and the activated NF-κB can, in return, promote their transcription.
Nuclear factor kappa B encodes various proteins that play a crucial role in immunity, inflammation, cell growth, survival, and apoptosis (Singh and Singh, 2020). Moreover, NF-κB up-regulation can increase the expression of pro-inflammatory cytokines during the proliferation of hippocampal glial cells (Wang et al., 2017), and this plays a crucial role in epilepsy. The NF-κB transcription factor family in mammals consists of five proteins (Oeckinghaus and Ghosh, 2009) and can be subdivided into the Rel subfamily [including c-Rel, Rel B, and Rel A (aka p65)] and the NF-κB subfamily (including p105/p50, p100/p52 and so on), both of which have a Rel homology domain (Mitchell et al., 2016). Rel proteins have a C-terminal transactivation domain that can activate transcription. Some proteins in the NF-κB subfamily that cannot function as transcriptional activators become shorter (p105 to p50 and p100 to p52) through limited proteolysis (Gilmore, 2006). Normally, NF-κB exists in an inactive state as a dimer (usually p50 and p65) bound to an inhibitor (IκB) (Karin, 1999) in the cytoplasm. Theoretically, there are 15 possible combinations of dimers but only 13 dimers are known to exist in cells (Zhang et al., 2017). The IκB protein family consists of IκBα, IκBβ, IκBγ, IκBδ, IκBε, and Drosophila Cactus, among others. When the cell receives a stimulus, there is an intracellular activation of the IκB kinase (IKK) complex comprising catalytic kinase enzymes including IKK-α, IKK-β, and IKK-γ/NF-κB essential modulator, which further integrates the downstream activating signals by phosphorylating the inhibitor of NF-κB (Mitchell et al., 2016).
Two types of NF-κB signal transduction pathways have been identified. In the classical pathway, NF-κB is activated by extracellular stimuli such as Tumor Necrosis Factor-α (TNF-α), which activates cell surface receptors and recruit adaptor proteins through the cytoplasmic domain, triggers a signaling cascade that ultimately activates the IKK complex through phosphorylation of the serine of IKKβ (Ser177 and Ser181). The activated IKK complex phosphorylates IκBα (at Ser32/Ser36 on IκBα) (Kendellen et al., 2014), which is degraded by ubiquitination. This causes NF-κB dimers (mostly p65 and p50), which actively shuttle between the nucleus and cytosol, to stay nuclear and induce gene expression. In contrast with this, the signal-related adaptor protein, in the non-canonical pathway, can only be recruited by some molecules, such as CD40, and ubiquitination is not required. In this pathway, IKKα is phosphorylated, and p100 is recruited leading to the phosphorylation of p100 by IKKα, partial hydrolysis, and conversion to p52 to expose its nuclear localization signal and DNA binding domain. It can form a complex with Rel B in the human nucleus to activate the target gene expression (Verma et al., 2019). Cross-talk exists between activation pathways. Both Rel B and p100 genes contain κB binding sites, and their transcription is dependent on p65 (Bren et al., 2001). p100, also called IκBδ, can inhibit p65 (Shih et al., 2011). When p52 is lacking, Rel B forms a dimer with p50. Similarly, when p50 is lacking, p65 forms a dimer with p52, which has almost the same level of p65 activation and target inflammatory gene expression (Hoffmann et al., 2003). IκBα is a target gene of NF-κB, and re-expressed IκBα inhibits NF-κB activity. Other target genes, such as interleukins (ILs) and TNF-α, can block IKK-dependent phosphorylation of IκBα to inhibit NF-κB activation. Studies have found that the NF-κB pathway can stay active for 30–60 min in most cells (Qin et al., 2007) and be activated under several conditions that can promote the transcription of target genes that affect the function of neurons.
Nuclear factor kappa B overexpresses various genes implicated in oxidative stress and inflammatory diseases and can regulate neurogenesis, neuronal death survival, and synaptic plasticity (Buckmaster and Dudek, 1997; O’Neill and Kaltschmidt, 1997). Brain tissue analysis of epileptic patients and animal models has shown that NF-κB has complex functions related to neuron survival and injury (Mattson, 2005). Therefore, targeting of NF-κB by selective or non-selective inhibitors or agonists in epilepsy can serve as an attractive therapeutic approach.
Changes of Nuclear Factor Kappa B Expression in Status Epilepticus
Increased NF-κB expression has been observed in brain tissue from both animal models (Lerner-Natoli et al., 2000) and patients with epilepsy (Lubin et al., 2007; Table 1).
In animal models, the increase in NF-κB first occurs in neurons after seizures. The first report demonstrating the expression of NF-κB in the rat brain showed that the activity of NF-κB (p50) in hippocampal neurons increases rapidly (within 4 h) in response to epilepsy induced by pentylenetetrazole (Prasad et al., 1994). Another study also showed a significant upregulation of NF-κB 4 h after kainic acid (KA) injection that peaked 8–16 h post-treatment (Rong and Baudry, 1996). Given the different cells in the brain, Lerner-Natoli et al. (2000) found that the changes in NF-κB expression first occurred in the hippocampal neurons 24 h after KA injection, which was earlier than that in glial cells. Thus, the NF-κB upregulation at this timepoint in neurons was independent of glial cells and may be related to calcium influx (Furukawa and Mattson, 1998) caused by the activation of N-methyl-D-aspartic acid (NMDA) and α-amino-3-hydroxy-5-methyl-4-isoxazole-propionic acid receptors.
Nuclear factor kappa B expression increased in glial cells later than its change in neurons. Pérez-Otano et al. (1996) proposed that NF-κB expression increased in astrocytes 2 days after KA injection. Matsuoka et al. (1999) found that NF-κB expression was upregulated in blood vessels and glial cells, but it disappeared in vertebral neurons after 1 day of status epilepticus (SE) induced by KA microinjection Similarly, Lerner-Natoli et al. (2000) demonstrated that overexpression and increased activation of NF-κB occurred in thickened astrocytes 4–8 days after SE. Previous studies have proposed that the activation of inflammatory signals in glial cells is involved in KA-induced neurodegeneration, which suggests that the activated NF-κB in glial cells participates in the delayed and long-term response of glia to injury.
Astrocytes and microglia have been extensively studied in epilepsy. However, the cell types that overexpress NF-κB have not been established. Pérez-Otano et al. (1996) and Lerner-Natoli et al. (2000) both found that the number of microglia increased after SE but NF-κB was expressed in astrocytes. On the other hand, Kim and his group found an increase in phosphorylated NF-κB at the threonine 435 site in microglia after SE (Kim et al., 2019). Similarly, NF-κB serine 276 phosphorylation was found to be increased in microglia in the frontoparietal cortex (Kim et al., 2020) or piriform cortex (Lee et al., 2014). The elevated high mobility group protein B1 after epilepsy must activate microglia through the toll-like receptor 4 (TLR4)/receptor for advanced glycation endproducts for late glycation end products of the NF-κB pathway to disrupt the function of neurons (Shi et al., 2018; Massey et al., 2019; Rosciszewski et al., 2019). Currently, there is no literature on NF-κB expression in oligodendrocytes and NG2 cells (polydendrocytes) in epilepsy.
There has also been controversy about how long NF-κB persists after SE. Voutsinos-Porche et al. (2004) and his group found that the immunohistochemical expression of NF-κB began increasing 12 h post-injection and returned to basal levels by 3 and 6 days. In contrast, Wang et al. (2017) found that the expression of NF-κB in the epileptic hippocampus was highest on the 14th day after SE; on the 60th day the expression of NF-κB in the epilepsy group was higher than that in the controls Despite these inconsistencies, the close relationship between inflammation and epilepsy has been confirmed. Thus, as a major regulatory factor of inflammation, NF-κB plays an important role in the occurrence and development of epilepsy (Figure 1).
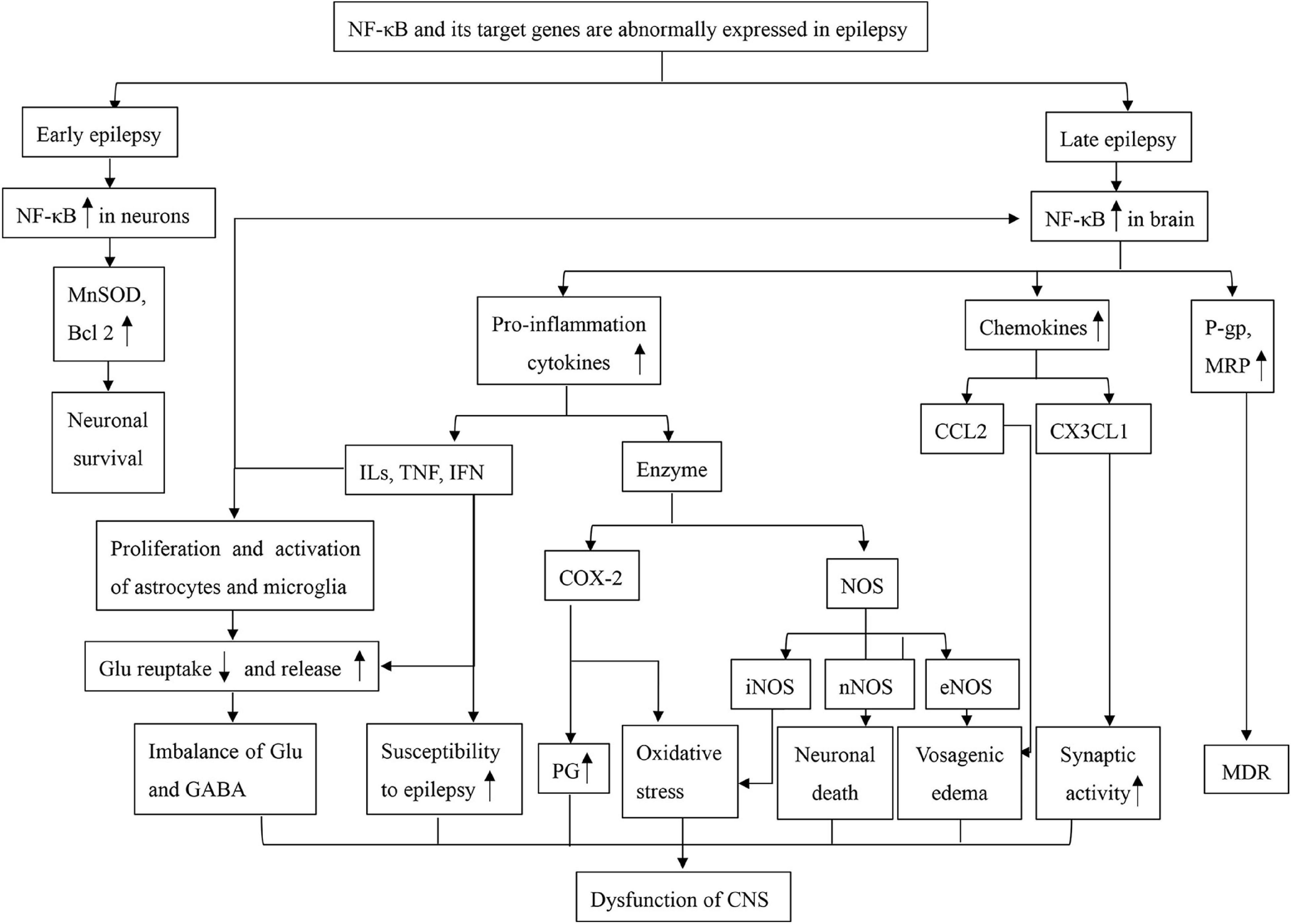
Figure 1. A mind map of NF-κB dysfunction in epilepsy. Bcl2, B-cell lymphoma-2; CCL2, chemokine (C-C motif) ligand 2; COX-2, cyclooxygenase 2; CX3CL1, fractalkine; Glu, glutamate; GABA, γ-aminobutyric acid; ILs, interleukins; IFN, interferons; MnSOD, manganese-superoxide dismutase; MRP, multidrug resistance protein; MDR, multiple drug resistance; NF-κB, nuclear factor kappa B; NOS, nitric oxide synthase; eNOS, endothelial nitric oxide synthase; iNOS, inducible nitric oxide synthase; nNOS, neuronal nitric oxide synthase; PG, prostaglandin; P-gp, P-glycoprotein; TNF, Tumor Necrosis Factor.
Abnormal Expression of Nuclear Factor Kappa B and Its Target Genes Can Affect Neuronal Function and Survival
Nuclear Factor Kappa Band Neuronal Survival
After establishing that NF-κB expression changes in epilepsy, several studies related to NF-κB function in neurons have emerged. When neuronal cells were exposed to external stimuli related to NF-κB, such as TNFα pretreatment (Furukawa and Mattson, 1998) or activation of TNF receptors (Marchetti et al., 2004), their survival rates could increase. Rel A knockout (Carrasco et al., 2000) or IκBα overexpression in cells induced apoptosis by NF-κB elimination (Mattson and Camandola, 2001), which suggests that NF-κB actively inhibits cell death signaling. At the same time, some studies have suggested that the upregulation of NF-κB plays a neuroprotective role in the short-term after seizures in animal models (Rong and Baudry, 1996; Mattson et al., 1997; Carrasco et al., 2000; Lerner-Natoli et al., 2000; Blondeau et al., 2001; Lanzillotta et al., 2010), which may be due to an increase in the expression of proteins associated with neuronal survival. Singh et al. concluded that NF-κB activation in neurons during epilepsy can promote the expression of B-cell lymphoma-2 (Bcl2) (Singh and Singh, 2020), and Mattson et al. showed that manganese-superoxide dismutase and Bcl2, which were necessary for neuronal plasticity and physical activity, were the target genes of NF-κB (Mattson and Camandola, 2001). Since NF-κB has different subunits, studies are now investigating whether the phosphorylation of different subunits has different effects. The phosphorylation mentioned in the above studies has almost always been of the p65 subunit. However, Ryu et al. (2011a) and Kim et al. (2013) showed that the phosphorylation of p52 can promote the expression of Bcl2, the phosphorylation of p65-Ser529 participates in cell growth, while phosphorylation at other sites on p65 may be related to cell death and inflammation. Moreover, c-Rel can induce apoptosis and is strongly activated in apoptotic neurons (Abbadie et al., 1993). We cannot know the exact NF-κB subunit upregulated in neurons during early epileptic onset, but there is no doubt that it is associated with neuron survival.
Dysfunctions of Nuclear Factor Kappa B and Neurons
Nuclear factor kappa B is a major inducer of pro-inflammatory cytokines (Mattson, 2005), which can cause increased spontaneous epileptic recurrence and encode neurotoxic substances. NF-κB acts as a key point of convergence for multiple stress signals, including pro-inflammatory cytokines and oxidative stress (Miller et al., 2014). The target genes of NF-κB associated with epilepsy include cytokines, enzymes, and receptors, among others (Grilli and Memo, 1999).
Nuclear Factor Kappa B Inflammation, Abnormal Neuronal Function, and Apoptosis
The molecules induced by NF-κB are mainly divided into ILs, interferons (IFNs), TNF superfamily, colony-stimulating factors, and some enzymes, which can cause neuronal death by inducing inflammation. Among these, the most widely studied are ILs, TNFs, cyclooxygenase 2 (COX-2), and nitric oxide synthase.
Pro-inflammatory cytokines, such as IL-1α, IL-1β, IL-6, IL-10, TNF-α, and IFN-γ, are usually released by activated microglia and astrocytes (O’Neill and Kaltschmidt, 1997; Vezzani and Baram, 2007) and typically concentrated in low quantities within the brain. Their expression increased after seizures (Scorza et al., 2018), and they can enhance neuronal excitability and form a toxic microenvironment that promotes the progression of epilepsy (Kovács et al., 2011; Huang et al., 2021). The imbalance between glutamate and γ-aminobutyric acid (GABA) in the brain after epileptic seizures depends on the action of these cytokines (Hu et al., 2000; Takeuchi et al., 2006), and they may cause neuronal death through mitochondrial dysfunction or abnormal ion currents (Folbergrová and Kunz, 2012). Particularly, TNF-α has been found to increase microglial glutamate (Takeuchi et al., 2006) and induce GABA receptor endocytosis (Stellwagen et al., 2005). Moreover, IL-1β, TNF-α, and IFN-γ inhibit glutamate reuptake in astrocytes, and this can be blocked by IFN-β (Hu et al., 2000). The activation of TLR4/NF-κB in glial cells increases susceptibility to epilepsy (Iori et al., 2013) and is possibly associated with increased concentrations of IL-1β (Dubé et al., 2005), TNF-α (Balosso et al., 2005), IL-6 (Fukuda et al., 2007), and IL-2 (De Sarro et al., 1994). NF-κB target genes can also, in turn, activate it through corresponding receptors on neurons and glial cells to promote the expression of pro-inflammatory factors (Listwak et al., 2013), which further aggravates brain injury. These pro-inflammatory factors can also have beneficial effects, such as increased survival rates, observed in TNF-α-pretreated neurons in response to harmful stimuli; these effects may be mediated by NF-κB (Furukawa and Mattson, 1998).
The most widely studied enzymes induced by NF-κB in glial cells during epilepsy include COX-2 and nitric oxide synthase. After SE, the expression of COX-2 in glial cells and neurons increases, and it is often considered a marker of inflammation (Drion et al., 2018). COX-2, which is expressed at low to moderate levels in the cell bodies and dendritic spines of hippocampal neurons, is shown to be modulated by NF-κB (Yamagata et al., 1993) in neurons during SE (Yagami et al., 2016; Arena et al., 2019). COX-2 expression is regulated by synaptic activity (Kaufmann et al., 1996) and corresponds to the role of NF-κB in synaptic transmission (Yamagata et al., 1993). COX-2 inhibition prevents long-term potentiation of the perforant-path synapse to the dentate granule cells (Chen et al., 2002). After selective inhibition of COX-2, the excitability of hippocampal CA1 pyramidal neurons and dendritic membranes significantly reduced, which may be due to alterations in potassium currents (Chen and Bazan, 2005). COX-2 can also transform arachidonic acid into prostaglandin and other harmful substances to promote inflammation (Xu et al., 2020). At the same time, antiepileptic treatments can reduce the COX-2 in glial cells (Drion et al., 2018). Neuronal nitric oxide synthase, which is primarily expressed in neurons (Cinelli et al., 2020), can trigger pentylenetetrazole kindling epilepsy-induced endoplasmic reticulum stress and oxidative damage (Zhu et al., 2017). It can oxidize amino acids to produce nitric oxide (NO) and promote inflammation, like other nitric oxide synthases (Dreyer et al., 2004), as well as mediate glutamate-induced neuronal death and cause mitochondrial damage (Brown and Bal-Price, 2003). Activated microglial cells can kill neurons via NO from inducible nitric oxide synthase (iNOS) by inhibiting neuronal respiration, rapid glutamate release from both astrocytes and neurons, and subsequent excitotoxic death of the neurons (Brown and Bal-Price, 2003). Both COX-2 (Mazumder et al., 2017) and iNOS (Tawfik et al., 2018) cause oxidative stress, and they may be related to neuronal apoptosis induced by mitochondria (Wang et al., 2019).
Circulation Between Chemokines and Nuclear Factor Kappa B in Epilepsy
Chemokines are small cytokines or signaling proteins secreted by cells, with a molecular weight of approximately 8–10 kDa, and they can be regulated by NF-κB. They have four conserved cysteine residues to ensure their tertiary structure and can be divided into the following four major subfamilies: CXC, CC, CX3C, and XC (Fu et al., 2010; Huang et al., 2014, 2017; Karimian et al., 2017). Astrocytes, resident microglia, and endothelial cells have been identified as the cellular source of chemokines in the central nervous system under physiological and pathological conditions (Ambrosini and Aloisi, 2004; Fabene et al., 2010; Bozzi and Caleo, 2016). As their receptors are usually expressed on glial cells, chemokines affect epilepsy by regulating glial function. Several chemokine variants are known to alter neuronal physiology by modulating voltage-dependent channels, activating g-protein-gated potassium inflow channels, and increasing the release of certain neurotransmitters (Fabene et al., 2010). Wang et al. (2016) found that the levels of chemokine (C-C motif) ligand 2 (CCL2) and CC chemokine receptor 2 were increased in patients with intractable epilepsy. An increase in CC chemokine receptor 2 was also observed in CD68 + microglia, which can be caused by seizures (Bozzi and Caleo, 2016). Fractalkine (CX3CL1) is a transmembrane chemokine expressed by neurons (Pawelec et al., 2020) and glial cells (Ali et al., 2015). Microglia maintain the neurogenic niche environment through the interaction with neurons via fractalkine signaling under physiological conditions (Araki et al., 2021). However, under pathological conditions, the CX3CL1/chemokine (C-X3-C motif) receptor 1 axis can alter synaptic activity in epilepsy through microglia (Wake et al., 2009), and the upregulation of CX3CL1 can decrease GABA currents through the GABAA receptor (Roseti et al., 2013). Temporal lobe epilepsy patients also exhibit increased chemokine (C-X-C motif) ligand and (CXCL) 4 receptor expression in microglia and astrocytes, which eventually increase the glutamate levels (Lee et al., 2007). The increase in CXCL2 mRNA production has been demonstrated in epilepsy (Hu et al., 2020), and knockout of the CXCL2 receptor gene in astrocytes can affect the functioning of the blood-brain barrier (BBB) (Liu X. X. et al., 2020). Chemokines and their receptor genes are targeted by NF-κB (Fu et al., 2010; Duan et al., 2014; Ni et al., 2019), and chemokine receptors can also activate NF-κB (Ding et al., 2016; Bai et al., 2018; Zhuang et al., 2019), which may serve as a vicious circle in epilepsy.
Functional Crosstalk Between Nrf2 and Nuclear Factor Kappa B Inhibits the Expression of Antioxidant Molecules
Nuclear factor (erythroid-derived 2) -like 2 (Nrf2) is a transcription factor that can enhance the expressions of several antioxidants (Singh et al., 2018). In epilepsy models, Nrf2 expression reduction has been observed (Geng et al., 2018), but the ablation of the Nrf2 gene also enhances the degradation of IκBα, which leads to increased activation of the NF-κB protein and further upregulation of the expression of pro-inflammatory cytokines (Thimmulappa et al., 2006). Another mechanism is the competition between Nrf2 and NF-κB for the transcriptional coactivator creb binding protein complex (Singh et al., 2019). Injury-induced increase in NF-κB expression limits the availability of creb binding protein for Nrf2 complex formation and leads to an increase in the expression of NF-κB-driven inflammatory genes (Wardyn et al., 2015). Moreover, the decreased expression of antioxidant molecules may lead to the aggravation of neuronal damage caused by oxidative stress, which further affects the function of the nervous system.
Other Factors Influencing Neuronal Apoptosis Pathway Associated With Nuclear Factor Kappa B
As a key regulator of apoptosis, increased levels of NF-κB in the hippocampus inevitably lead to increased levels of caspase-3, which is associated with apoptosis (Mohamed et al., 2020). The excitotoxicity of neurons caused by overstimulation of NMDA receptors can directly activate NF-κB and cause cell apoptosis (Engelmann et al., 2014), which may be related to calcium influx (Vezzani et al., 2008).
Abnormal Activation of Nuclear Factor Kappa B Can Affect Glial Cell Activity Through Proliferation and Polarization
The following are the four main types of glial cells: astrocytes, microglia, oligodendrocytes, and nerve/glial antigen 2 cells (polydendrocytes) (Pitkänen and Lukasiuk, 2009). Of these, astrocytes and microglia are the most widely studied in epilepsy. Overactivation of NF-κB in glial cells can cause changes in central nervous system function, promote seizures, and affect cellular activity, proliferation, and polarization of the glial cell.
The pro-inflammatory factors in epileptic models are mainly expressed through glial cells, which, in turn, affect the activity of neurons. Because of the presence of related receptors, the changes in the expressions of these molecules can affect the function of the glial cells. TNF-α stimulation of primary astrocytes in vitro can promote cell proliferation and viability and increase the expressions of NF-κB, P-glycoprotein (P-gp), and multidrug resistance-associated proteins 1 (Wang X. et al., 2018). The latter two are associated with drug resistance in epilepsy. TNF-α and IL-1β can promote the activity and proliferation of astrocytes (Cui et al., 2011) and microglia (Bruttger et al., 2015; Zhao et al., 2018) and inhibit glutamate reuptake into primary astrocytes (Ye and Sontheimer, 1996), thereby aggravating seizures. IL-1 receptor-associated kinase 1 is also regulated by NF-κB (Deng et al., 2019), which can also activate NF-κB to produce pro-inflammatory effects in glial cells (Liu G. J. et al., 2020). Chemokines such as CCL2 can also promote primary cultured microglia proliferation (Zhang et al., 2018).
Microglia activation plays a key role in regulating inflammation and immune response and can have a pro-inflammatory or anti-inflammatory effect depending on the M1/M2 polarization phenotype (Zhao et al., 2019). The phosphorylation of p65-Ser276 can convert microglia from the resting state to the activated state (Kim et al., 2020). The increase of p65 in microglia can promote the transition to the M1 phenotype of microglia (Zhang et al., 2019). Activated M1 microglia can release IL-1α and TNF, which can induce an A1 astrocyte phenotype, leading to neuronal and oligodendrocyte death, as well as synaptic collapse (Liddelow et al., 2017). The increased NF-κB expression in astrocytes also has the same effect (Xu et al., 2018).
Individual studies have demonstrated different points of view. For instance, the p50 subunit can regulate the balance of microglia M1/M2, and its dysfunction can lead to chronic inflammation (Taetzsch et al., 2015). Moreover, the expressions of TNF-α, IL-1β, and colony-stimulating factors can decrease the activation of microglia and astrocytes (Pérez-Otano et al., 1996). SE causes autophagic death of astrocytes through TNF-α, and the phosphorylation of p65/RelA-Ser529 (Ryu et al., 2011b) and IL-1 has an inhibitory effect on pentylenetetrazole (PTZ)-induced (Miller et al., 1991) or kindling-induced (Sayyah et al., 2005) seizures. Andrzejczak et al. suggested that the different functions of pro-inflammatory factors may depend on their concentration and the type of receptors involved in the response (Andrzejczak, 2011).
Nuclear Factor Kappa B, Endothelial Cells, the Blood-Brain Barrier, and Drug-Resistant Epilepsy
Nuclear factor kappa B is expressed in neurons, glial cells, and endothelial cells. The expressions of cytokines (Liu X. X. et al., 2020), receptors (Kamali et al., 2020), enzymes, and P-gp (Ke et al., 2019) are altered in endothelial cells just like in neurons and glial cells, and similar effects are observed.
Under physiological conditions, activating NF-κB can protect the BBB (Ridder et al., 2015). Dysfunctions of NF-κB threonine 435 phosphorylation and endothelial NOS in endothelial cells (Kim et al., 2019) can lead to vasogenic edema with neutrophil infiltration and loss of astrocytes (Kim et al., 2012). After SE, resident microglia are the main source of chemokine (C-C motif) ligand 2. Due to the destruction of the BBB integrity, chemokine (C-C motif) ligand 2 and CC chemokine receptor 2 can recruit monocytes and macrophages, leading to the infiltration of white blood cells and the formation of vasogenic edema (Kim et al., 2020). Excitotoxicity is a central pathological pathway in epilepsy with BBB dysfunction (Barna et al., 2020). Seizures may modulate the BBB function, affect astrocytes and the innate immune system, and ultimately alter neuronal networks (Löscher and Friedman, 2020). This may, in turn, lead to changes in the central nervous system (Marchi et al., 2012).
Several studies have shown that BBB dysfunction is one of the main causes of drug resistance in epilepsy (Ogaki et al., 2020). P-gp is a member of the ATP-binding box superfamily of transmembrane proteins and is targeted by several pharmacological barriers (Ambudkar et al., 2006). Specifically, its expression increases through different signaling pathways by L-glutamate stimulation (Zhu and Liu, 2004). It binds to drugs and ATP, which, in turn, pumps the drugs out of the cell, reducing the intracellular concentration of drugs and making the cell resistant to them. Ke et al. (2019) found that P-gp was overexpressed in bEnd.3 cells and showed that endothelial cells play a role in the development of drug-resistant epilepsy. The p65 subunit can be combined with the Multi-Drug Resistance Gene 1 to promote the expression of P-gp on the cell membrane (Geick et al., 2001; Yu et al., 2011; Deng et al., 2019). The activation of NF-κB enhances the spillover transport of antiepileptic drugs across the BBB (Yu et al., 2011). The NMDA receptor and COX-2 can increase the expressions of P-gp in the BBB after SE (Bauer et al., 2008). P-gp is also expressed in glial cells (Wang X. et al., 2018) and neurons (Deng et al., 2019; Merelli et al., 2019) and may have an abnormal expression after SE. Interestingly, the overexpression of P-gp may be one of the mechanisms for the development of drug-resistant epilepsy that is closely associated with sudden unexpected death observed in epilepsy (Auzmendi et al., 2021). However, specific inhibitors of P-gp are known to produce unpredictable toxicity in clinical trials (Deng et al., 2009).
Effects of Existing Treatments on Nuclear Factor Kappa B
In animal experiments, it has been found that directly inhibiting the activity of NF-κB will have a certain effect on epilepsy. Pyrrolidine dithiocarbamate salt (PDTC) is a drug that can specifically antagonize NF-κB (Soerensen et al., 2009). Yu et al. (2011) found that PDTC pretreatment prolonged the seizure onset time, decreased the P-gp overexpression, and failed to prevent brain cell loss in KA-induced rats. Shin et al. (2004) reported that PDTC prevented hippocampal neuronal loss in the KA-induced seizure model, and the use of a low dose of PDTC can almost completely protect from lesions in the piriform cortex (Soerensen et al., 2009) and attenuate the microglial activation (Lv et al., 2014). Treatment with 150 mg/kg PDTC before and after SE significantly increased the mortality rate to 100% (Soerensen et al., 2009). SN50 peptide can also inhibit the activity of NF-κB. It reduces the vasogenic edema caused by epilepsy (Kim and Kang, 2017) and inhibits the NF-κB-induced expression of P-gp in rat brain capillaries (Yu et al., 2011). The mRNA and protein levels of P-gp were remarkably reduced when NF-κB p65 was knocked down by siRNA transfections (Ke et al., 2019). Furthermore, NF-κB “decoy” inhibited COX-2 expression in an epileptic rat brain (Xu et al., 2020).
Some non-selective drugs can also act through the NF-κB pathway. Aspirin, a member of non-steroidal anti-inflammatory drugs, can reduce the content of NF-κB and protect against corticohippocampal neurodegeneration in an epilepsy model induced by PTZ (Abd-Elghafour et al., 2017). Dimethyl fumarate, an activator of Nrf2, downregulated the expression of inflammatory factors (NF-κB) with the reduction of the seizure score, percentage of kindled rats, and neurological damage score (Singh et al., 2019). Edaravone can protect hippocampal neurons from damage in KA-induced epilepsy rats by upregulating Nrf2 and downregulating NF-κB (Liu et al., 2018). Valproic acid can reduce the death of neural progenitor cells through the activation of NF-κB signaling (Go et al., 2011), which indicates that NF-κB can promote neuronal survival mentioned above. Long-term use of Antiseizure medications, such as phenytoin (Zhou et al., 2015), valproic acid (Rao et al., 2007), carbamazepine (Yu et al., 2017), pregabalin (Nader et al., 2018; Attia et al., 2019), and diazepam (Firdous et al., 2021), can reduce the amount of NF-κB in animal tissues or cells to protect neurons and suppress inflammation. Carbamazepine can also regulate the expression of P-gp, which may be related to drug-resistant epilepsy (Yu et al., 2017). From these, we found that different antiepileptic drugs may play different roles in the NF-κB pathway. This may be related to the fact that phosphorylation at different sites of different NF-κB molecules can cause different effects.
The discovery of new therapy is also important. Extracts from plants, such as curcumin (Karimian et al., 2017), rosaceae (Firdous et al., 2021), hyperforin (Lee et al., 2014), asiatic acid (Wang Z. H. et al., 2018), and gastrodin (Zhou et al., 2015), can downregulate neuroinflammation by preventing NF-κB phosphorylation in animal models, and their mechanisms of action are similar to that of valproate (Chen et al., 2018). Sitagliptin and nilotinib (Nader et al., 2018; Attia et al., 2019), neither of which is related to epilepsy treatment, can be used to treat epilepsy via the NF-κB pathway when used together with anticonvulsants. Anti-inflammatory miR-146a extended the latency of generalized convulsions, reduced the seizure severity, and decreased the expressions of its target mRNAs (IRAK-1 and TRAF-6) and NF-κB (Tao et al., 2017). The above treatment methods are not used in clinical practice, but the ketogenic diet, originally developed for the treatment of epilepsy in non-responder children, exhibits anti-inflammatory effects by inhibiting NF-κB (Pinto et al., 2018). There are no experiments on p65-related gene knockout mice, because mice lacking the p65 gene die within embryonic days 14–15 (Won et al., 1999).
Conclusion
Nuclear factor kappa B is a transcription factor whose upregulation is responsible for increasing the expression of pro-inflammatory cytokines during neuronal excitability and hippocampal gliosis. It can be divided into two subfamilies and both include many factors, but the dimers that have been discovered to play a role in epilepsy do not contain all the molecules. Because the existing studies have shown that phosphorylation of different molecules, or phosphorylation at different sites of the same molecule, may exert different physiological functions, the identification of the effects of different molecules is particularly important. It is undeniable that in epilepsy, the upregulation of NF-κB can play a role in protecting neurons, and there is no doubt that the inflammatory response and antioxidant imbalance caused by NF-κB activation during epilepsy has a harmful effect on the central nervous system. Further research is needed to identify the role of different NF-κB molecules and different dimers in epilepsy, and to find new and more stable compounds that specifically inhibit one or more molecules of NF-κB to treat epilepsy. Hopefully 1 day, these drugs can be applied clinically and can bring good news to patients with epilepsy.
Author Contributions
MC wrote the manuscript. WL provided the ideas and finally revised the manuscript. Both authors contributed to the article and approved the submitted version.
Conflict of Interest
The authors declare that the research was conducted in the absence of any commercial or financial relationships that could be construed as a potential conflict of interest.
Publisher’s Note
All claims expressed in this article are solely those of the authors and do not necessarily represent those of their affiliated organizations, or those of the publisher, the editors and the reviewers. Any product that may be evaluated in this article, or claim that may be made by its manufacturer, is not guaranteed or endorsed by the publisher.
Acknowledgments
We would like to thank Editage (www.editage.cn) for English language editing.
References
Abbadie, C., Kabrun, N., Bouali, F., Smardova, J., Stéhelin, D., Vandenbunder, B., et al. (1993). High levels of c-rel expression are associated with programmed cell death in the developing avian embryo and in bone marrow cells in vitro. Cell 75, 899–912. doi: 10.1016/0092-8674(93)90534-w
Abd-Elghafour, B. A., El-Sayed, N. M., Ahmed, A. A. M., Zaitone, S. A., and Moustafa, Y. M. (2017). Aspirin and (or) omega-3 polyunsaturated fatty acids protect against corticohippocampal neurodegeneration and downregulate lipoxin A production and formyl peptide receptor-like 1 expression in pentylenetetrazole-kindled rats. Can J. Physiol. Pharmacol. 95, 340–348. doi: 10.1139/cjpp-2016-0060
Ali, I., Chugh, D., and Ekdahl, C. T. (2015). Role of fractalkine-CX3CR1 pathway in seizure-induced microglial activation, neurodegeneration, and neuroblast production in the adult rat brain. Neurobiol. Dis. 74, 194–203. doi: 10.1016/j.nbd.2014.11.009
Ambrosini, E., and Aloisi, F. (2004). Chemokines and glial cells: a complex network in the central nervous system. Neurochem. Res. 29, 1017–1038. doi: 10.1023/b:nere.0000021246.96864.89
Ambudkar, S. V., Kim, I.-W., and Sauna, Z. E. (2006). The power of the pump: mechanisms of action of P-glycoprotein (ABCB1). Eur. J. Pharm. Sci. 27, 392–400. doi: 10.1016/j.ejps.2005.10.010
Andrzejczak, D. (2011). [Epilepsy and pro-inflammatory cytokines. Immunomodulating properties of antiepileptic drugs]. Neurol. Neurochir. Pol. 45, 275–285. doi: 10.1016/s0028-3843(14)60080-3
Araki, T., Ikegaya, Y., and Koyama, R. (2021). The effects of microglia- and astrocyte-derived factors on neurogenesis in health and disease. Eur. J. Neurosci. 54, 5880–5901. doi: 10.1111/ejn.14969
Arena, A., Zimmer, T. S., van Scheppingen, J., Korotkov, A., Anink, J. J., Mühlebner, A., et al. (2019). Oxidative stress and inflammation in a spectrum of epileptogenic cortical malformations: molecular insights into their interdependence. Brain Pathol. 29, 351–365. doi: 10.1111/bpa.12661
Attia, G. M., Elmansy, R. A., and Elsaed, W. M. (2019). Neuroprotective effect of nilotinib on pentylenetetrazol-induced epilepsy in adult rat hippocampus: involvement of oxidative stress, autophagy, inflammation, and apoptosis. Folia Neuropathol. 57, 146–160. doi: 10.5114/fn.2019.84423
Auzmendi, J., Akyuz, E., and Lazarowski, A. (2021). The role of P-glycoprotein (P-gp) and inwardly rectifying potassium (Kir) channels in sudden unexpected death in epilepsy (SUDEP). Epilepsy. Behav. 121(Pt B), 106590. doi: 10.1016/j.yebeh.2019.106590
Bai, W., Zhou, J., Zhou, N., Liu, Q., Cui, J., Zou, W., et al. (2018). Hypoxia-increased RAGE expression regulates chemotaxis and pro-inflammatory cytokines release through nuclear translocation of NF-κ B and HIF1α in THP-1 cells. Biochem. Biophys. Res. Commun. 495:2282–2288. doi: 10.1016/j.bbrc.2017.12.084
Balosso, S., Ravizza, T., Perego, C., Peschon, J., Campbell, I. L., De Simoni, M. G., et al. (2005). Tumor necrosis factor-alpha inhibits seizures in mice via p75 receptors. Ann. Neurol. 57, 804–812. doi: 10.1002/ana.20480
Barna, L., Walter, F. R., Harazin, A., Bocsik, A., Kincses, A., Tubak, V., et al. (2020). Simvastatin, edaravone and dexamethasone protect against kainate-induced brain endothelial cell damage. Fluids Barriers CNS 17:5. doi: 10.1186/s12987-019-0166-1
Bauer, B., Hartz, A. M., Pekcec, A., Toellner, K., Miller, D. S., and Potschka, H. (2008). Seizure-induced up-regulation of P-glycoprotein at the blood-brain barrier through glutamate and cyclooxygenase-2 signaling. Mol. Pharmacol. 73, 1444–1453. doi: 10.1124/mol.107.041210
Blondeau, N., Widmann, C., Lazdunski, M., and Heurteaux, C. (2001). Activation of the nuclear factor-kappaB is a key event in brain tolerance. J. Neurosci. 21, 4668–4677. doi: 10.1523/JNEUROSCI.21-13-04668.2001
Bozzi, Y., and Caleo, M. (2016). Epilepsy, Seizures, and Inflammation: Role of the C-C Motif Ligand 2 Chemokine. DNA Cell Biol. 35, 257–260. doi: 10.1089/dna.2016.3345
Bren, G. D., Solan, N. J., Miyoshi, H., Pennington, K. N., Pobst, L. J., and Paya, C. V. (2001). Transcription of the RelB gene is regulated by NF-kappaB. Oncogene 20, 7722–7733. doi: 10.1038/sj.onc.1204868
Brown, G. C., and Bal-Price, A. (2003). Inflammatory neurodegeneration mediated by nitric oxide, glutamate, and mitochondria. Mol. Neurobiol. 27, 325–355. doi: 10.1385/MN:27:3:325
Bruttger, J., Karram, K., Wörtge, S., Regen, T., Marini, F., Hoppmann, N., et al. (2015). Genetic Cell Ablation Reveals Clusters of Local Self-Renewing Microglia in the Mammalian Central Nervous System. Immunity 43:1. doi: 10.1016/j.immuni.2015.06.012
Buckmaster, P. S., and Dudek, F. E. (1997). Network properties of the dentate gyrus in epileptic rats with hilar neuron loss and granule cell axon reorganization. J. Neurophysiol. 77, 2685–2696. doi: 10.1152/jn.1997.77.5.2685
Carrasco, J., Penkowa, M., Hadberg, H., Molinero, A., and Hidalgo, J. (2000). Enhanced seizures and hippocampal neurodegeneration following kainic acid-induced seizures in metallothionein-I + II-deficient mice. Eur. J. Neurosci. 12, 2311–2322. doi: 10.1046/j.1460-9568.2000.00128.x
Chen, C., and Bazan, N. G. (2005). Lipid signaling: sleep, synaptic plasticity, and neuroprotection. Prostaglandins Lipid. Mediat. 77, 65–76. doi: 10.1016/j.prostaglandins.2005.07.001
Chen, C., Magee, J. C., and Bazan, N. G. (2002). Cyclooxygenase-2 regulates prostaglandin E2 signaling in hippocampal long-term synaptic plasticity. J. Neurophysiol. 87, 2851–2857. doi: 10.1152/jn.2002.87.6.2851
Chen, J.-Y., Chu, L.-W., Cheng, K.-I., Hsieh, S.-L., Juan, Y.-S., and Wu, B.-N. (2018). Valproate reduces neuroinflammation and neuronal death in a rat chronic constriction injury model. Sci. Rep. 8:16457. doi: 10.1038/s41598-018-34915-5
Cinelli, M. A., Do, H. T., Miley, G. P., and Silverman, R. B. (2020). Inducible nitric oxide synthase: Regulation, structure, and inhibition. Med. Res. Rev. 40, 158–189. doi: 10.1002/med.21599
Cui, M., Huang, Y., Tian, C., Zhao, Y., and Zheng, J. (2011). FOXO3a inhibits TNF-α- and IL-1β-induced astrocyte proliferation:Implication for reactive astrogliosis. Glia 59, 641–654. doi: 10.1002/glia.21134
De Sarro, G., Rotiroti, D., Audino, M. G., Gratteri, S., and Nisticó, G. (1994). Effects of interleukin-2 on various models of experimental epilepsy in DBA/2 mice. Neuroimmunomodulation 1, 361–369. doi: 10.1159/000097189
Deng, X., Luyendyk, J. P., Ganey, P. E., and Roth, R. A. (2009). Inflammatory stress and idiosyncratic hepatotoxicity: hints from animal models. Pharmacol. Rev. 61, 262–282. doi: 10.1124/pr.109.001727
Deng, X., Shao, Y., Xie, Y., Feng, Y., Wu, M., Wang, M., et al. (2019). MicroRNA-146a-5p Downregulates the Expression of P-Glycoprotein in Rats with Lithium-Pilocarpine-Induced Status Epilepticus. Biol. Pharm. Bull. 42, 744–750. doi: 10.1248/bpb.b18-00937
Ding, X.-M., Pan, L., Wang, Y., and Xu, Q.-Z. (2016). Baicalin exerts protective effects against lipopolysaccharide-induced acute lung injury by regulating the crosstalk between the CX3CL1-CX3CR1 axis and NF-κB pathway in CX3CL1-knockout mice. Int. J. Mol. Med. 37, 703–715. doi: 10.3892/ijmm.2016.2456
Dreyer, J., Schleicher, M., Tappe, A., Schilling, K., Kuner, T., Kusumawidijaja, G., et al. (2004). Nitric oxide synthase (NOS)-interacting protein interacts with neuronal NOS and regulates its distribution and activity. J. Neurosci. 24, 10454–10465. doi: 10.1523/JNEUROSCI.2265-04.2004
Drion, C. M., van Scheppingen, J., Arena, A., Geijtenbeek, K. W., Kooijman, L., van Vliet, E. A., et al. (2018). Effects of rapamycin and curcumin on inflammation and oxidative stress in vitro and in vivo - in search of potential anti-epileptogenic strategies for temporal lobe epilepsy. J. Neuroinflam. 15:212. doi: 10.1186/s12974-018-1247-9
Duan, M., Yao, H., Cai, Y., Liao, K., Seth, P., and Buch, S. H. I. V. - (2014). 1 Tat disrupts CX3CL1-CX3CR1 axis in microglia via the NF-κBYY1 pathway. Curr. HIV Res. 12, 189–200. doi: 10.2174/1570162x12666140526123119
Dubé, C., Vezzani, A., Behrens, M., Bartfai, T., and Baram, T. Z. (2005). Interleukin-1beta contributes to the generation of experimental febrile seizures. Ann. Neurol. 57, 152–155. doi: 10.1002/ana.20358
Engelmann, C., Weih, F., and Haenold, R. (2014). Role of nuclear factor kappa B in central nervous system regeneration. Neural. Regen. Res. 9, 707–711. doi: 10.4103/1673-5374.131572
Fabene, P. F., Bramanti, P., and Constantin, G. (2010). The emerging role for chemokines in epilepsy. J. Neuroimmunol. 224, 22–27. doi: 10.1016/j.jneuroim.2010.05.016
Firdous, A., Sarwar, S., Shah, F. A., Tabasum, S., Zeb, A., Nadeem, H., et al. (2021). Contribution of Attenuation of TNF-α and NF-κB in the Anti-Epileptic, Anti-Apoptotic and Neuroprotective Potential of Fruit and Its Chitosan Encapsulation. Molecules 26:8. doi: 10.3390/molecules26082347
Folbergrová, J., and Kunz, W. S. (2012). Mitochondrial dysfunction in epilepsy. Mitochondrion 12, 35–40. doi: 10.1016/j.mito.2011.04.004
Fu, E. S., Zhang, Y. P., Sagen, J., Candiotti, K. A., Morton, P. D., Liebl, D. J., et al. (2010). Transgenic inhibition of glial NF-kappa B reduces pain behavior and inflammation after peripheral nerve injury. Pain 148, 509–518. doi: 10.1016/j.pain.2010.01.001
Fukuda, M., Morimoto, T., Suzuki, Y., Shinonaga, C., and Ishida, Y. (2007). Interleukin-6 attenuates hyperthermia-induced seizures in developing rats. Brain Dev. 29, 644–648. doi: 10.1016/j.braindev.2007.04.007
Furukawa, K., and Mattson, M. P. (1998). The transcription factor NF-kappaB mediates increases in calcium currents and decreases in NMDA- and AMPA/kainate-induced currents induced by tumor necrosis factor-alpha in hippocampal neurons. J. Neurochem. 70, 1876–1886. doi: 10.1046/j.1471-4159.1998.70051876.x
Geick, A., Eichelbaum, M., and Burk, O. (2001). Nuclear receptor response elements mediate induction of intestinal MDR1 by rifampin. J. Biol. Chem. 276, 14581–14587. doi: 10.1074/jbc.M010173200
Geng, J.-F., Liu, X., Zhao, H.-B., Fan, W.-F., Geng, J.-J., and Liu, X.-Z. (2018). LncRNA UCA1 inhibits epilepsy and seizure-induced brain injury by regulating miR-495/Nrf2-ARE signal pathway. Int. J. Biochem. Cell Biol. 99, 133–139. doi: 10.1016/j.biocel.2018.03.021
Gilmore, T. D. (2006). Introduction to NF-kappaB: players, pathways, perspectives. Oncogene 25, 6680–6684. doi: 10.1038/sj.onc.1209954
Go, H. S., Seo, J. E., Kim, K. C., Han, S. M., Kim, P., Kang, Y. S., et al. (2011). Valproic acid inhibits neural progenitor cell death by activation of NF-κB signaling pathway and up-regulation of Bcl-XL. J. Biomed. Sci. 18:48. doi: 10.1186/1423-0127-18-48
Grilli, M., and Memo, M. (1999). Nuclear factor-kappaB/Rel proteins: a point of convergence of signalling pathways relevant in neuronal function and dysfunction. Biochem. Pharmacol. 57, 1–7. doi: 10.1016/s0006-2952(98)00214-7
Hoffmann, A., Leung, T. H., and Baltimore, D. (2003). Genetic analysis of NF-kappaB/Rel transcription factors defines functional specificities. EMBO J. 22, 5530–5539. doi: 10.1093/emboj/cdg534
Hu, H., Zhu, T., Gong, L., Zhao, Y., Shao, Y., Li, S., et al. (2020). Transient receptor potential melastatin 2 contributes to neuroinflammation and negatively regulates cognitive outcomes in a pilocarpine-induced mouse model of epilepsy. Int. Immunopharmacol. 87:106824. doi: 10.1016/j.intimp.2020.106824
Hu, S., Sheng, W. S., Ehrlich, L. C., Peterson, P. K., and Chao, C. C. (2000). Cytokine effects on glutamate uptake by human astrocytes. Neuroimmunomodulation 7, 153–159. doi: 10.1159/000026433
Huang, X., Zeng, Y., Jiang, Y., Qin, Y., Luo, W., Xiang, S., et al. (2017). Lipopolysaccharide-Binding Protein Downregulates Fractalkine through Activation of p38 MAPK and NF-B. Mediators Inflamm 2017, 9734837. doi: 10.1155/2017/9734837
Huang, X.-Y., Hu, Q.-P., Shi, H.-Y., Zheng, Y.-Y., Hu, R.-R., and Guo, Q. (2021). Everolimus inhibits PI3K/Akt/mTOR and NF-kB/IL-6 signaling and protects seizure-induced brain injury in rats. J. Chem. Neuroanat. 114:101960. doi: 10.1016/j.jchemneu.2021.101960
Huang, Y.-N., Lai, C.-C., Chiu, C.-T., Lin, J.-J., and Wang, J.-Y. L. - (2014). ascorbate attenuates the endotoxin-induced production of inflammatory mediators by inhibiting MAPK activation and NF-κB translocation in cortical neurons/glia Cocultures. PLoS One 9:e97276. doi: 10.1371/journal.pone.0097276
Iori, V., Maroso, M., Rizzi, M., Iyer, A. M., Vertemara, R., Carli, M., et al. (2013). Receptor for Advanced Glycation Endproducts is upregulated in temporal lobe epilepsy and contributes to experimental seizures. Neurobiol. Dis. 58, 102–114. doi: 10.1016/j.nbd.2013.03.006
Kamali, A. N., Zian, Z., Bautista, J. M., Hamedifar, H., Hossein-Khannazer, N., Hosseinzadeh, R., et al. (2020). The Potential Role of Pro-Inflammatory and Anti-Inflammatory Cytokines in Epilepsy Pathogenesis. Endocr. Metab. Immune Disord Drug Targets 2020:940. doi: 10.2174/1871530320999201116200940
Karimian, M. S., Pirro, M., Majeed, M., and Sahebkar, A. (2017). Curcumin as a natural regulator of monocyte chemoattractant protein-1. Cytokine Growth Factor Rev. 33, 55–63. doi: 10.1016/j.cytogfr.2016.10.001
Karin, M. (1999). How NF-kappaB is activated: the role of the IkappaB kinase (IKK) complex. Oncogene 18, 6867–6874. doi: 10.1038/sj.onc.1203219
Kaufmann, W. E., Worley, P. F., Pegg, J., Bremer, M., and Isakson, P. (1996). COX-2, a synaptically induced enzyme, is expressed by excitatory neurons at postsynaptic sites in rat cerebral cortex. Proc. Natl. Acad. Sci. U S A 93, 2317–2321. doi: 10.1073/pnas.93.6.2317
Ke, X.-J., Cheng, Y.-F., Yu, N., and Di, Q. (2019). Effects of carbamazepine on the P-gp and CYP3A expression correlated with PXR or NF-κB activity in the bEnd.3 cells. Neurosci. Lett. 690, 48–55. doi: 10.1016/j.neulet.2018.10.016
Kendellen, M. F., Bradford, J. W., Lawrence, C. L., Clark, K. S., and Baldwin, A. S. (2014). Canonical and non-canonical NF-κB signaling promotes breast cancer tumor-initiating cells. Oncogene 33, 1297–1305. doi: 10.1038/onc.2013.64
Kim, J. E., and Kang, T. C. (2017). TRPC3- and ET(B) receptor-mediated PI3K/AKT activation induces vasogenic edema formation following status epilepticus. Brain Res. 1672, 58–64. doi: 10.1016/j.brainres.2017.07.020
Kim, J.-E., Park, H., Lee, J.-E., and Kang, T.-C. (2020). CDDO-Me Inhibits Microglial Activation and Monocyte Infiltration by Abrogating NFκB- and p38 MAPK-Mediated Signaling Pathways Following Status Epilepticus. Cells 9:5. doi: 10.3390/cells9051123
Kim, J.-E., Ryu, H. J., Choi, S. Y., and Kang, T.-C. (2012). Tumor necrosis factor-α-mediated threonine 435 phosphorylation of p65 nuclear factor-κB subunit in endothelial cells induces vasogenic edema and neutrophil infiltration in the rat piriform cortex following status epilepticus. J. Neuroinflammation. 9:6. doi: 10.1186/1742-2094-9-6
Kim, M.-J., Park, H., Choi, S.-H., Kong, M.-J., Kim, J.-E., and Kang, T.-C. (2019). CDDO-Me Attenuates Vasogenic Edema and Astroglial Death by Regulating NF-κB p65 Phosphorylations and Nrf2 Expression Following Status Epilepticus. Int. J. Mol. Sci. 20:19. doi: 10.3390/ijms20194862
Kim, W. I., Ryu, H. J., Kim, J.-E., Seo, C. H., Lee, B. C., Choi, I.-G., et al. (2013). Differential nuclear factor-kappa B phosphorylation induced by lipopolysaccharide in the hippocampus of P2X7 receptor knockout mouse. Neurol. Res. 35, 369–381. doi: 10.1179/1743132812Y.0000000137
Kovács, Z., Czurkó, A., Kékesi, K. A., and Juhász, G. (2011). Intracerebroventricularly administered lipopolysaccharide enhances spike-wave discharges in freely moving WAG/Rij rats. Brain Res. Bull 85, 410–416. doi: 10.1016/j.brainresbull.2011.05.003
Kwon, Y. S., Pineda, E., Auvin, S., Shin, D., Mazarati, A., and Sankar, R. (2013). Neuroprotective and antiepileptogenic effects of combination of anti-inflammatory drugs in the immature brain. J. Neuroinflam. 10:30. doi: 10.1186/1742-2094-10-30
Lanzillotta, A., Sarnico, I., Ingrassia, R., Boroni, F., Branca, C., Benarese, M., et al. (2010). The acetylation of RelA in Lys310 dictates the NF-κB-dependent response in post-ischemic injury. Cell Death Dis. 1:e96. doi: 10.1038/cddis.2010.76
Lee, S.-K., Kim, J.-E., Kim, Y.-J., Kim, M.-J., and Kang, T.-C. (2014). Hyperforin attenuates microglia activation and inhibits p65-Ser276 NFκB phosphorylation in the rat piriform cortex following status epilepticus. Neurosci. Res. 85, 39–50. doi: 10.1016/j.neures.2014.05.006
Lee, T.-S., Mane, S., Eid, T., Zhao, H., Lin, A., Guan, Z., et al. (2007). Gene expression in temporal lobe epilepsy is consistent with increased release of glutamate by astrocytes. Mol. Med. 13, 1–2. doi: 10.2119/2006-00079.Lee
Lerner-Natoli, M., Montpied, P., Rousset, M. C., Bockaert, J., and Rondouin, G. (2000). Sequential expression of surface antigens and transcription factor NFkappaB by hippocampal cells in excitotoxicity and experimental epilepsy. Epilepsy. Res. 41, 141–154. doi: 10.1016/s0920-1211(00)00132-7
Liddelow, S. A., Guttenplan, K. A., Clarke, L. E., Bennett, F. C., Bohlen, C. J., Schirmer, L., et al. (2017). Neurotoxic reactive astrocytes are induced by activated microglia. Nature 541, 481–487. doi: 10.1038/nature21029
Listwak, S. J., Rathore, P., and Herkenham, M. (2013). Minimal NF-κB activity in neurons. Neuroscience 250, 282–299. doi: 10.1016/j.neuroscience.2013.07.013
Liu, G.-J., Tao, T., Wang, H., Zhou, Y., Gao, X., Gao, Y.-Y., et al. (2020). Functions of resolvin D1-ALX/FPR2 receptor interaction in the hemoglobin-induced microglial inflammatory response and neuronal injury. J. Neuroinflammation. 17:239. doi: 10.1186/s12974-020-01918-x
Liu, X.-X., Yang, L., Shao, L.-X., He, Y., Wu, G., Bao, Y.-H., et al. (2020). Endothelial Cdk5 deficit leads to the development of spontaneous epilepsy through CXCL1/CXCR2-mediated reactive astrogliosis. J. Exp. Med. 217:1. doi: 10.1084/jem.20180992
Liu, Z., Yang, C., Meng, X., Li, Z., Lv, C., and Cao, P. (2018). Neuroprotection of edaravone on the hippocampus of kainate-induced epilepsy rats through Nrf2/HO-1 pathway. Neurochem. Int. 112, 159–165. doi: 10.1016/j.neuint.2017.07.001
Löscher, W., and Friedman, A. (2020). Structural, Molecular, and Functional Alterations of the Blood-Brain Barrier during Epileptogenesis and Epilepsy: a Cause, Consequence, or Both? Int. J. Mol. Sci. 21:2. doi: 10.3390/ijms21020591
Lubin, F. D., Ren, Y., Xu, X., and Anderson, A. E. (2007). Nuclear factor-kappa B regulates seizure threshold and gene transcription following convulsant stimulation. J. Neurochem. 103, 1381–1395. doi: 10.1111/j.1471-4159.2007.04863.x
Lv, R., Xu, X., Luo, Z., Shen, N., Wang, F., and Zhao, Y. (2014). Pyrrolidine dithiocarbamate (PDTC) inhibits the overexpression of MCP-1 and attenuates microglial activation in the hippocampus of a pilocarpine-induced status epilepticus rat model. Exp. Ther. Med. 7, 39–45. doi: 10.3892/etm.2013.1397
Marcheselli, V. L., and Bazan, N. G. (1996). Sustained induction of prostaglandin endoperoxide synthase-2 by seizures in hippocampus. Inhibition by a platelet-activating factor antagonist. J. Biol. Chem. 271, 24794–24799. doi: 10.1074/jbc.271.40.24794
Marchetti, L., Klein, M., Schlett, K., Pfizenmaier, K., and Eisel, U. L. M. (2004). Tumor necrosis factor (TNF)-mediated neuroprotection against glutamate-induced excitotoxicity is enhanced by N-methyl-D-aspartate receptor activation. Essential role of a TNF receptor 2-mediated phosphatidylinositol 3-kinase-dependent NF-kappa B pathway. J. Biol. Chem. 279, 32869–32881. doi: 10.1074/jbc.M311766200
Marchi, N., Granata, T., Ghosh, C., and Janigro, D. (2012). Blood-brain barrier dysfunction and epilepsy: pathophysiologic role and therapeutic approaches. Epilepsia 53, 1877–1886. doi: 10.1111/j.1528-1167.2012.03637.x
Massey, N., Puttachary, S., Bhat, S. M., Kanthasamy, A. G., and Charavaryamath, C. (2019). HMGB1-RAGE Signaling Plays a Role in Organic Dust-Induced Microglial Activation and Neuroinflammation. Toxicol. Sci. 169, 579–592. doi: 10.1093/toxsci/kfz071
Matsuoka, Y., Kitamura, Y., Okazaki, M., Terai, K., and Taniguchi, T. (1999). Kainic acid-induced activation of nuclear factor-kappaB in rat hippocampus. Exp. Brain Res. 124, 215–222. doi: 10.1007/s002210050616
Mattson, M. P., and Camandola, S. (2001). NF-kappaB in neuronal plasticity and neurodegenerative disorders. J. Clin. Invest. 107, 247–254. doi: 10.1172/JCI11916
Mattson, M. P., Goodman, Y., Luo, H., Fu, W., and Furukawa, K. (1997). Activation of NF-kappaB protects hippocampal neurons against oxidative stress-induced apoptosis: evidence for induction of manganese superoxide dismutase and suppression of peroxynitrite production and protein tyrosine nitration. J. Neurosci. Res. 49, 681–697. doi: 10.1002/(SICI)1097-4547(19970915)49:6<681::AID-JNR3>3.0.CO;2-3
Mattson, M. P. N. F. (2005). kappaB in the survival and plasticity of neurons. Neurochem. Res. 30, 883–893. doi: 10.1007/s11064-005-6961-x
Mazumder, A. G., Sharma, P., Patial, V., and Singh, D. (2017). Crocin Attenuates Kindling Development and Associated Cognitive Impairments in Mice via Inhibiting Reactive Oxygen Species-Mediated NF-κB Activation. Basic Clin. Pharmacol. Toxicol. 120, 426–433. doi: 10.1111/bcpt.12694
Merelli, A., Ramos, A. J., Lazarowski, A., and Auzmendi, J. (2019). Convulsive Stress Mimics Brain Hypoxia and Promotes the P-Glycoprotein (P-gp) and Erythropoietin Receptor Overexpression. Recombinant Human Erythropoietin Effect on P-gp Activity. Front. Neurosci. 13:750. doi: 10.3389/fnins.2019.00750
Miller, J. A., Kirkley, K. A., Padmanabhan, R., Liang, L.-P., Raol, Y. H., Patel, M., et al. (2014). Repeated exposure to low doses of kainic acid activates nuclear factor kappa B (NF-κB) prior to seizure in transgenic NF-κB/EGFP reporter mice. Neurotoxicology 44, 39–47. doi: 10.1016/j.neuro.2014.04.010
Miller, L. G., Galpern, W. R., Dunlap, K., Dinarello, C. A., and Turner, T. J. (1991). Interleukin-1 augments gamma-aminobutyric acidA receptor function in brain. Mol. Pharmacol. 39, 105–108.
Mitchell, S., Vargas, J., and Hoffmann, A. (2016). Signaling via the NFκB system. Wiley Interdiscip. Rev. Syst. Biol. Med. 8, 227–241. doi: 10.1002/wsbm.1331
Mohamed, M. A. E., Abdel-Rahman, R. F., Mahmoud, S. S., Khattab, M. M., and Safar, M. M. (2020). Metformin and trimetazidine ameliorate diabetes-induced cognitive impediment in status epileptic rats. Epilepsy Behav. 104, (Pt A):106893. doi: 10.1016/j.yebeh.2019.106893
Nader, M. A., Ateyya, H., El-Shafey, M., and El-Sherbeeny, N. A. (2018). Sitagliptin enhances the neuroprotective effect of pregabalin against pentylenetetrazole-induced acute epileptogenesis in mice: implication of oxidative, inflammatory, apoptotic and autophagy pathways. Neurochem. Int. 115, 11–23. doi: 10.1016/j.neuint.2017.10.006
Ni, H., Wang, Y., An, K., Liu, Q., Xu, L., Zhu, C., et al. (2019). Crosstalk between NFκB-dependent astrocytic CXCL1 and neuron CXCR2 plays a role in descending pain facilitation. J. Neuroinflamm. 16:1. doi: 10.1186/s12974-018-1391-2
Oeckinghaus, A., and Ghosh, S. (2009). The NF-kappaB family of transcription factors and its regulation. Cold Spring Harb. Perspect. Biol. 1:a000034. doi: 10.1101/cshperspect.a000034
Ogaki, A., Ikegaya, Y., and Koyama, R. (2020). Vascular Abnormalities and the Role of Vascular Endothelial Growth Factor in the Epileptic Brain. Front. Pharmacol. 11:20. doi: 10.3389/fphar.2020.00020
Ojo, E. S., Ishola, I. O., Ben-Azu, B., Afolayan, O. O., James, A. B., Ajayi, A. M., et al. (2019). Ameliorative influence of Cnestis ferruginea vahl ex DC (Connaraceae) root extract on kainic acid-induced temporal lobe epilepsy in mice: role of oxidative stress and neuroinflammation. J. Ethnopharmacol. 243:112117. doi: 10.1016/j.jep.2019.112117
O’Neill, L. A., and Kaltschmidt, C. (1997). NF-kappa B: a crucial transcription factor for glial and neuronal cell function. Trends Neurosci. 20, 252–258. doi: 10.1016/s0166-2236(96)01035-1
Paudel, Y. N., Kumari, Y., Abidin, S. A. Z., Othman, I., and Shaikh, M. F. (2020). Pilocarpine Induced Behavioral and Biochemical Alterations in Chronic Seizure-Like Condition in Adult Zebrafish. Int. J. Mol. Sci. 21:7. doi: 10.3390/ijms21072492
Paudel, Y. N., Semple, B. D., Jones, N. C., Othman, I., and Shaikh, M. F. (2019). High mobility group box 1 (HMGB1) as a novel frontier in epileptogenesis: from pathogenesis to therapeutic approaches. J. Neurochem. 151, 542–557. doi: 10.1111/jnc.14663
Pawelec, P., Ziemka-Nalecz, M., Sypecka, J., and Zalewska, T. (2020). The Impact of the CX3CL1/CX3CR1 Axis in Neurological Disorders. Cells 9:10. doi: 10.3390/cells9102277
Pérez-Otano, I., McMillian, M. K., Chen, J., Bing, G., Hong, J. S., and Pennypacker, K. R. (1996). Induction of NF-kB-like transcription factors in brain areas susceptible to kainate toxicity. Glia 16, 306–315. doi: 10.1002/(SICI)1098-1136(199604)16:4<306::AID-GLIA3>3.0.CO;2-Y
Pinto, A., Bonucci, A., Maggi, E., Corsi, M., and Businaro, R. (2018). Anti-Oxidant and Anti-Inflammatory Activity of Ketogenic Diet: new Perspectives for Neuroprotection in Alzheimer’s Disease. Antioxidants 7:5. doi: 10.3390/antiox7050063
Pitkänen, A., and Lukasiuk, K. (2009). Molecular and cellular basis of epileptogenesis in symptomatic epilepsy. Epilepsy Behav. 14(Suppl. 1), 16–25. doi: 10.1016/j.yebeh.2008.09.023
Prasad, A. V., Pilcher, W. H., and Joseph, S. A. (1994). Nuclear factor-kappa B in rat brain: enhanced DNA-binding activity following convulsant-induced seizures. Neurosci. Lett. 170, 145–148. doi: 10.1016/0304-3940(94)90260-7
Qin, Z.-H., Tao, L.-Y., and Chen, X. (2007). Dual roles of NF-kappaB in cell survival and implications of NF-kappaB inhibitors in neuroprotective therapy. Acta Pharmacol. Sin. 28, 1859–1872. doi: 10.1111/j.1745-7254.2007.00741.x
Qu, Z., Jia, L., Xie, T., Zhen, J., Si, P., Cui, Z., et al. (2019). (-)-Epigallocatechin-3-Gallate Protects Against Lithium-Pilocarpine-Induced Epilepsy by Inhibiting the Toll-Like Receptor 4 (TLR4)/Nuclear Factor-κB (NF-κB) Signaling Pathway. Med. Sci. Monit. 25, 1749–1758. doi: 10.12659/MSM.915025
Rao, J. S., Bazinet, R. P., Rapoport, S. I., and Lee, H.-J. (2007). Chronic treatment of rats with sodium valproate downregulates frontal cortex NF-kappaB DNA binding activity and COX-2 mRNA. Bipolar. Disord. 9, 513–520. doi: 10.1111/j.1399-5618.2007.00361.x
Ridder, D. A., Wenzel, J., Müller, K., Töllner, K., Tong, X.-K., Assmann, J. C., et al. (2015). Brain endothelial TAK1 and NEMO safeguard the neurovascular unit. J. Exp. Med. 212, 1529–1549. doi: 10.1084/jem.20150165
Rong, Y., and Baudry, M. (1996). Seizure activity results in a rapid induction of nuclear factor-kappa B in adult but not juvenile rat limbic structures. J. Neurochem. 67, 662–668. doi: 10.1046/j.1471-4159.1996.67020662.x
Rosciszewski, G., Cadena, V., Auzmendi, J., Cieri, M. B., Lukin, J., Rossi, A. R., et al. (2019). Detrimental Effects of HMGB-1 Require Microglial-Astroglial Interaction: Implications for the Status Epilepticus -Induced Neuroinflammation. Front. Cell Neurosci. 13:380. doi: 10.3389/fncel.2019.00380
Roseti, C., Fucile, S., Lauro, C., Martinello, K., Bertollini, C., Esposito, V., et al. (2013). Fractalkine/CX3CL1 modulates GABAA currents in human temporal lobe epilepsy. Epilepsia 54, 1834–1844. doi: 10.1111/epi.12354
Russmann, V., Brendel, M., Mille, E., Helm-Vicidomini, A., Beck, R., Günther, L., et al. (2017). Identification of brain regions predicting epileptogenesis by serial [F]GE-180 positron emission tomography imaging of neuroinflammation in a rat model of temporal lobe epilepsy. Neuroimage Clin. 15, 35–44. doi: 10.1016/j.nicl.2017.04.003
Ryu, H. J., Kim, J. E., Yeo, S. I., Kim, M. J., Jo, S. M., and Kang, T. C. (2011a). ReLA/P65-serine 536 nuclear factor-kappa B phosphorylation is related to vulnerability to status epilepticus in the rat hippocampus. Neuroscience 2011:187. doi: 10.1016/j.neuroscience.2011.04.048
Ryu, H. J., Kim, J.-E., Yeo, S.-I., and Kang, T.-C. (2011b). p65/RelA-Ser529 NF-κB subunit phosphorylation induces autophagic astroglial death (Clasmatodendrosis) following status epilepticus. Cell Mol. Neurobiol. 31, 1071–1078. doi: 10.1007/s10571-011-9706-1
Sayyah, M., Beheshti, S., Shokrgozar, M. A., Eslami-far, A., Deljoo, Z., Khabiri, A. R., et al. (2005). Antiepileptogenic and anticonvulsant activity of interleukin-1 beta in amygdala-kindled rats. Exp. Neurol. 191, 145–153. doi: 10.1016/j.expneurol.2004.08.032
Scheffer, I. E., Berkovic, S., Capovilla, G., Connolly, M. B., French, J., Guilhoto, L., et al. (2017). ILAE classification of the epilepsies: Position paper of the ILAE Commission for Classification and Terminology. Epilepsia 58, 512–521. doi: 10.1111/epi.13709
Scorza, C. A., Marques, M. J. G., Gomes da Silva, S., Naffah-Mazzacoratti, M. D. G., Scorza, F. A., and Cavalheiro, E. A. (2018). Status epilepticus does not induce acute brain inflammatory response in the Amazon rodent Proechimys, an animal model resistant to epileptogenesis. Neurosci. Lett. 668, 169–173. doi: 10.1016/j.neulet.2017.02.049
Shi, Y., Zhang, L., Teng, J., and Miao, W. (2018). HMGB1 mediates microglia activation via the TLR4/NF-κB pathway in coriaria lactone induced epilepsy. Mol. Med. Rep. 17, 5125–5131. doi: 10.3892/mmr.2018.8485
Shih, V. F.-S., Tsui, R., Caldwell, A., and Hoffmann, A. (2011). A single NFκB system for both canonical and non-canonical signaling. Cell Res. 21:1. doi: 10.1038/cr.2010.161
Shin, E.-J., Jhoo, J. H., Kim, W.-K., Jhoo, W. K., Lee, C., Jung, B. D., et al. (2004). Protection against kainate neurotoxicity by pyrrolidine dithiocarbamate. Clin. Exp. Pharmacol. Physiol. 31, 320–326. doi: 10.1111/j.1440-1681.2004.03990.x
Singh, N., Saha, L., Kumari, P., Singh, J., Bhatia, A., Banerjee, D., et al. (2019). Effect of dimethyl fumarate on neuroinflammation and apoptosis in pentylenetetrazol kindling model in rats. Brain Res. Bull. 144, 233–245. doi: 10.1016/j.brainresbull.2018.11.013
Singh, N., Vijayanti, S., and Saha, L. (2018). Targeting crosstalk between Nuclear factor (erythroid-derived 2)-like 2 and Nuclear factor kappa beta pathway by Nrf2 activator dimethyl fumarate in epileptogenesis. Int. J. Neurosci. 128, 987–994. doi: 10.1080/00207454.2018.1441149
Singh, S., and Singh, T. G. (2020). Role of Nuclear Factor Kappa B (NF-κB) Signalling in Neurodegenerative Diseases: an Mechanistic Approach. Curr. Neuropharmacol. 18, 918–935. doi: 10.2174/1570159X18666200207120949
Soerensen, J., Pekcec, A., Fuest, C., Nickel, A., and Potschka, H. (2009). Pyrrolidine dithiocarbamate protects the piriform cortex in the pilocarpine status epilepticus model. Epilepsy. Res. 87, 177–183. doi: 10.1016/j.eplepsyres.2009.08.013
Stellwagen, D., Beattie, E. C., Seo, J. Y., and Malenka, R. C. (2005). Differential regulation of AMPA receptor and GABA receptor trafficking by tumor necrosis factor-alpha. J. Neurosci. 25, 3219–3228. doi: 10.1523/JNEUROSCI.4486-04.2005
Taetzsch, T., Levesque, S., McGraw, C., Brookins, S., Luqa, R., Bonini, M. G., et al. (2015). Redox regulation of NF-κB p50 and M1 polarization in microglia. Glia 63, 423–440. doi: 10.1002/glia.22762
Takeuchi, H., Jin, S., Wang, J., Zhang, G., Kawanokuchi, J., Kuno, R., et al. (2006). Tumor necrosis factor-alpha induces neurotoxicity via glutamate release from hemichannels of activated microglia in an autocrine manner. J. Biol. Chem. 281, 21362–21368. doi: 10.1074/jbc.M600504200
Tao, H., Zhao, J., Liu, T., Cai, Y., Zhou, X., Xing, H., et al. (2017). Intranasal Delivery of miR-146a Mimics Delayed Seizure Onset in the Lithium-Pilocarpine Mouse Model. Mediat. Inflamm. 2017:6512620. doi: 10.1155/2017/6512620
Tawfik, K. M., Moustafa, Y. M., and El-Azab, M. F. (2018). Neuroprotective mechanisms of sildenafil and selenium in PTZ-kindling model: implications in epilepsy. Eur. J. Pharmacol. 833, 131–144. doi: 10.1016/j.ejphar.2018.05.035
Thimmulappa, R. K., Lee, H., Rangasamy, T., Reddy, S. P., Yamamoto, M., Kensler, T. W., et al. (2006). Nrf2 is a critical regulator of the innate immune response and survival during experimental sepsis. J. Clin. Invest. 116, 984–995. doi: 10.1172/JCI25790
Trinka, E., Kwan, P., Lee, B., and Dash, A. (2019). Epilepsy in Asia: Disease burden, management barriers, and challenges. Epilepsia 60(Suppl.):1. doi: 10.1111/epi.14458
Verma, S., De Jesus, P., Chanda, S. K., and Verma, I. M. (2019). SNW1, a Novel Transcriptional Regulator of the NF-κB Pathway. Mol. Cell Biol. 39:3. doi: 10.1128/MCB.00415-18
Vezzani, A., and Baram, T. Z. (2007). New roles for interleukin-1 Beta in the mechanisms of epilepsy. Epilepsy. Curr. 7, 45–50. doi: 10.1111/j.1535-7511.2007.00165.x
Vezzani, A., Ravizza, T., Balosso, S., and Aronica, E. (2008). Glia as a source of cytokines: implications for neuronal excitability and survival. Epilepsia 49(Suppl. 2), 24–32. doi: 10.1111/j.1528-1167.2008.01490.x
Voutsinos-Porche, B., Koning, E., Kaplan, H., Ferrandon, A., Guenounou, M., Nehlig, A., et al. (2004). Temporal patterns of the cerebral inflammatory response in the rat lithium-pilocarpine model of temporal lobe epilepsy. Neurobiol. Dis. 17, 385–402. doi: 10.1016/j.nbd.2004.07.023
Wake, H., Moorhouse, A. J., Jinno, S., Kohsaka, S., and Nabekura, J. (2009). Resting microglia directly monitor the functional state of synapses in vivo and determine the fate of ischemic terminals. J. Neurosci. 29, 3974–3980. doi: 10.1523/JNEUROSCI.4363-08.2009
Wang, A., Si, Z., Xue, P., Li, X., and Liu, J. (2019). Tacrolimus protects hippocampal neurons of rats with status epilepticus through suppressing oxidative stress and inhibiting mitochondrial pathway of apoptosis. Brain Res. 1715, 176–181. doi: 10.1016/j.brainres.2019.02.031
Wang, C., Yang, L., Zhang, J., Lin, Z., Qi, J., and Duan, S. (2016). Higher expression of monocyte chemoattractant protein 1 and its receptor in brain tissue of intractable epilepsy patients. J. Clin. Neurosci. 28, 134–140. doi: 10.1016/j.jocn.2015.07.033
Wang, H. K., Yan, H., Wang, K., and Wang, J. (2017). Dynamic regulation effect of long non-coding RNA-UCA1 on NF-kB in hippocampus of epilepsy rats. Eur. Rev. Med. Pharmacol. Sci. 21, 3113–3119.
Wang, X., Huang, S., Jiang, Y., Liu, Y., Song, T., Li, D., et al. (2018). Reactive astrocytes increase the expression of P-gp and Mrp1 via TNF-α and NF-κB signaling. Mol. Med. Rep. 17, 1198–1204. doi: 10.3892/mmr.2017.7939
Wang, Z.-H., Mong, M.-C., Yang, Y.-C., and Yin, M.-C. (2018). Asiatic acid and maslinic acid attenuated kainic acid-induced seizure through decreasing hippocampal inflammatory and oxidative stress. Epilepsy. Res. 139, 28–34. doi: 10.1016/j.eplepsyres.2017.11.003
Wardyn, J. D., Ponsford, A. H., and Sanderson, C. M. (2015). Dissecting molecular cross-talk between Nrf2 and NF-κB response pathways. Biochem. Soc. Trans. 43, 621–626. doi: 10.1042/BST20150014
Wijnen, B. F. M., van Mastrigt, G. A. P. G., Evers, S. M. A. A., Gershuni, O., Lambrechts, D. A. J. E., Majoie, M. H. J. M., et al. (2017). A systematic review of economic evaluations of treatments for patients with epilepsy. Epilepsia 58, 706–726. doi: 10.1111/epi.13655
Won, S. J., Ko, H. W., Kim, E. Y., Park, E. C., Huh, K., Jung, N. P., et al. (1999). Nuclear factor kappa B-mediated kainate neurotoxicity in the rat and hamster hippocampus. Neuroscience 94, 83–91. doi: 10.1016/s0306-4522(99)00196-7
World Health Organization (2005). Atlas: epilepsy care in the world. Available online at: wwwwhoint/mental_health/publications/atlas_epilepsy_care_2005/en/ (Accessed April 28, 2016).
Xu, J., Sun, Y., Zhao, Y., Li, Q., Ge, B., Dai, S., et al. (2020). NF-κB “decoy” inhibits COX-2 expression in epileptic rat brain. J. Integr. Neurosci. 19, 77–87. doi: 10.31083/j.jin.2020.01.1248
Xu, X., Zhang, A., Zhu, Y., He, W., Di, W., Fang, Y., et al. (2018). MFG-E8 reverses microglial-induced neurotoxic astrocyte (A1) via NF-κB and PI3K-Akt pathways. J. Cell Physiol. 234, 904–914. doi: 10.1002/jcp.26918
Yagami, T., Koma, H., and Yamamoto, Y. (2016). Pathophysiological Roles of Cyclooxygenases and Prostaglandins in the Central Nervous System. Mol. Neurobiol. 53, 4754–4771. doi: 10.1007/s12035-015-9355-3
Yamagata, K., Andreasson, K. I., Kaufmann, W. E., Barnes, C. A., and Worley, P. F. (1993). Expression of a mitogen-inducible cyclooxygenase in brain neurons: regulation by synaptic activity and glucocorticoids. Neuron 11, 371–386. doi: 10.1016/0896-6273(93)90192-t
Ye, Z. C., and Sontheimer, H. (1996). Cytokine modulation of glial glutamate uptake: a possible involvement of nitric oxide. Neuroreport 7, 2181–2185. doi: 10.1097/00001756-199609020-00025
Yu, N., Di, Q., Liu, H., Hu, Y., Jiang, Y., and Yan, Y.-K. (2011). Nuclear factor-kappa B activity regulates brain expression of P-glycoprotein in the kainic acid-induced seizure rats. Mediat. Inflamm. 2011:670613. doi: 10.1155/2011/670613
Yu, N., Zhang, Y.-F., Zhang, K., Cheng, Y.-F., Ma, H.-Y., and Di, Q. (2017). Pregnane X Receptor Not Nuclear Factor-kappa B Up-regulates P-glycoprotein Expression in the Brain of Chronic Epileptic Rats Induced by Kainic Acid. Neurochem. Res. 42, 2167–2177. doi: 10.1007/s11064-017-2224-x
Zhang, J., Zheng, Y., Luo, Y., Du, Y., Zhang, X., and Fu, J. (2019). Curcumin inhibits LPS-induced neuroinflammation by promoting microglial M2 polarization via TREM2/TLR4/NF-κB pathways in BV2 cells. Mol. Immunol. 116, 29–37. doi: 10.1016/j.molimm.2019.09.020
Zhang, L., Ma, P., Guan, Q., Meng, L., Su, L., Wang, L., et al. (2018). Effect of chemokine CC ligand 2 (CCL2) on α-synuclein-induced microglia proliferation and neuronal apoptosis. Mol. Med. Rep. 18, 4213–4218. doi: 10.3892/mmr.2018.9468
Zhang, Q., Lenardo, M. J., and Baltimore, D. (2017). 30 Years of NF-κB: A Blossoming of Relevance to Human Pathobiology. Cell 168, 37–57. doi: 10.1016/j.cell.2016.12.012
Zhao, H., Zhu, C., and Huang, D. (2018). Microglial activation: an important process in the onset of epilepsy. Am. J. Transl. Res. 10, 2877–2889.
Zhao, R., Ying, M., Gu, S., Yin, W., Li, Y., Yuan, H., et al. (2019). Cysteinyl Leukotriene Receptor 2 is Involved in Inflammation and Neuronal Damage by Mediating Microglia M1/M2 Polarization through NF-κB Pathway. Neuroscience 2029:422. doi: 10.1016/j.neuroscience.2019.10.048
Zhou, Z., Lin, Y., Zheng, H., He, Y., Xu, H., Zhang, S., et al. (2015). Anticonvulsive and neuroprotective effects of synergetic combination of phenytoin and gastrodin on the convulsion induced by penicillin in mice. Fundam. Clin. Pharmacol. 29, 371–381. doi: 10.1111/fcp.12127
Zhu, H.-J., and Liu, G.-Q. (2004). Glutamate up-regulates P-glycoprotein expression in rat brain microvessel endothelial cells by an NMDA receptor-mediated mechanism. Life Sci. 75, 1313–1322. doi: 10.1016/j.lfs.2004.02.027
Zhu, X., Dong, J., Han, B., Huang, R., Zhang, A., Xia, Z., et al. (2017). Neuronal Nitric Oxide Synthase Contributes to PTZ Kindling Epilepsy-Induced Hippocampal Endoplasmic Reticulum Stress and Oxidative Damage. Front. Cell Neurosci. 11:377. doi: 10.3389/fncel.2017.00377
Keywords: epilepsy, NF-kappa B, inflammation, chemokines, interleukins, tumor necrosis factor
Citation: Cai M and Lin W (2022) The Function of NF-Kappa B During Epilepsy, a Potential Therapeutic Target. Front. Neurosci. 16:851394. doi: 10.3389/fnins.2022.851394
Received: 09 January 2022; Accepted: 22 February 2022;
Published: 10 March 2022.
Edited by:
Partha Sarathi Sarkar, University of Texas Medical Branch at Galveston, United StatesReviewed by:
Zucai Xu, Affiliated Hospital of Zunyi Medical University, ChinaCarmen Rubio, Manuel Velasco Suárez Instituto Nacional de Neurología y Neurocirugía, Mexico
Copyright © 2022 Cai and Lin. This is an open-access article distributed under the terms of the Creative Commons Attribution License (CC BY). The use, distribution or reproduction in other forums is permitted, provided the original author(s) and the copyright owner(s) are credited and that the original publication in this journal is cited, in accordance with accepted academic practice. No use, distribution or reproduction is permitted which does not comply with these terms.
*Correspondence: Weihong Lin, bGlud2hAamx1LmVkdS5jbg==