- 1Guangdong Key Laboratory of Age-Related Cardiac and Cerebral Diseases, Affiliated Hospital of Guangdong Medical University, Zhanjiang, China
- 2Department of Psychiatry, Affiliated Hospital of Guangdong Medical University, Zhanjiang, China
- 3Department of Rehabilitation Medicine Guangzhou Red Cross Hospital Affiliated to Jinan University, Guangzhou, China
- 4School of Humanities and Management, Guangdong Medical University, Dongguan, China
Circular RNAs (circRNAs) are highly expressed in the central nervous system and have been reported to be associated with neuropsychiatric diseases, but their potential role in major depressive disorder (MDD) remains unclear. Here, we demonstrated that there was a disorder of circRNAs in the blood of MDD patients. It has been preliminarily proved that hsa_circ_0002473, hsa_circ_0079651, hsa_circ_0137187, hsa_circ_0006010, and hsa_circ_0113010 were highly expressed in MDD patients and can be used as diagnostic markers for MDD. Bioinformatics analysis revealed that hsa_circ_0079651, hsa_circ_0137187, hsa_circ_0006010, and hsa_circ_0113010 may affect the neuroplasticity of MDD through the ceRNA mechanism.
Introduction
Depressive disorder is a set of diseases characterized by low spirit and there are approximately 264 million people worldwide suffer from it (GBD 2017 Disease and Injury Incidence and Prevalence Collaborators, 2018). According to DSM-5, depressive disorder includes major depressive disorder (MDD), persistent depressive disorder (PDD), premenstrual dysphoric disorder, disruptive mood dysregulation disorder, depressive disorder due to another medical condition, substance/medication-induced depressive disorder, etc. MDD is the most common type of depressive disorder, characterized by low spirits, loss of interest, inability to feel pleasure, poor concentration, etc. Relapse is also common, and to make matters worse, the patient may act or think suicidally. In comparison to men, women are more likely to suffer from major depression. Data from the China Mental Health Survey (CMHS), from 2013 to 2015, showed a lifetime prevalence of 3.4%, 12-month prevalence of 2.1%, and the 12-month prevalence is higher in women than in men (2.5 vs. 1.7%, p = 0.0061) (Huang et al., 2019). The etiology and pathogenesis of MDD remain unclear. Personality traits and environmental stress, as well as genetics, are thought to play a role in depression (Gonda et al., 2019). Some researchers believe that epigenetic gene modification and immune inflammation are linked to the development of MDD (Park et al., 2019; Beurel et al., 2020). The neurogenic theory posits that MDD is associated with neurogenesis defects, and postmortem investigations indicated that the size and density of neurons in the dorsolateral prefrontal cortex (dlPFC) and dentate gyrus (DG) of MDD patients were lower (Rajkowska, 2000; Boldrini et al., 2013; Malhi and Mann, 2018). However, there is currently no objective method to diagnose MDD. The diagnosis of MDD mainly depends on the patient’s subjective expression, which may be affected by the ability of both the patients and the clinicians to interpret symptoms. Further research into the pathogenesis of MDD will help to identify objective and reliable biomarkers of the disease, which can then be used in order to diagnose MDD, identify specific depressions, and select appropriate treatment.
CircRNAs are a class of covalently closed non-coding RNAs without 3′ poly-A tails or 5′ caps, characterized by high stability, wide expression, and tissue/developmental stage-specific expression, and are highly expressed in blood and brain (Rybak-Wolf et al., 2015). Circular RNAs are more stable and have a longer half-life than linear RNAs, and they are resistant to Ribonuclease R (RNase R) degradation, making them suitable as biomarkers for illness (Wen et al., 2020). CircRNAs have been found to have effects on neurological systems, innate immunity, microRNAs, and various disease-related pathways (Mei et al., 2019), and they are also linked to several neurological illnesses, including Alzheimer’s, Parkinson’s, multiple sclerosis, and schizophrenia (Gokool et al., 2020; Mehta et al., 2020). The field of circRNAs research in depression, however, is still in its infancy, with a few studies looking into the association between MDD and circRNAs (Cui et al., 2016; Zhang et al., 2018, 2019; Huang et al., 2020; Shi et al., 2021). Further studies are needed to fully understand the connection.
In this study, we aimed to identify and validate the differentially expressed circular RNAs in MDD patients, assess their utility as biomarkers, and investigate the possible processes of circRNAs in MDD.
Materials and Methods
Subjects
We recruited 29 MDD patients and 19 healthy controls from June to September 2020. MDD patients were recruited through the Department of Psychology at the Affiliated Hospital of Guangdong Medical University. Participants or their legal guardians were informed of the trial’s benefits and risks before providing written informed permission. All MDD patients met the following inclusion criteria: (1) met the MDD diagnostic criteria of Manual of Mental Disorders (5th Edition) (DSM-V); (2) the 24-item Hamilton Depression Scale (HAMD-24) score ≥ 8, Self-rating depression scale (SDS) score ≥ 53; (3) age 14 to 59 years, and the age of MDD onset under 55 years; (4) Han people; (5) depression patients without any medications or not taking any psychiatric medication for at least three months; (6) no infectious history, no significant physical disorder; (7) no history of smoking or alcohol abuse; (8) no history of head trauma. And the following exclusion criteria were applied for the MDD patients: (1) with other mental disorders, e.g., substance abuse, schizophrenia, obsessive-compulsive disorder, etc.; (2) pregnant or lactating female; (3) long-term use of drugs or health care products. The healthy controls were recruited in the community and the inclusion criteria were as follows: (1) the 24-item Hamilton Depression Scale (HAMD-24) score < 8, Self-rating depression scale (SDS) score < 53; (2) age 18 to 59 years; (3) Han people; (4) not taking any medication for at least three months; (5) no history of mental disorder and no family history of major mental disorder, no significant physical disorder; (6) no history of smoking or alcohol abuse. The following exclusion criteria were applied for the healthy controls: (1) pregnant or lactating female; (2) long-term use of drugs or health care products. All participants were jointly diagnosed by two or more psychiatrists. The demographic data of the patients and controls are shown in Table 1. The differences between MDD patients and healthy controls were not significant in age, gender ratio, or ethnicity, but the HAMD scores were significantly different.
Whole Transcriptome Sequencing
Following an overnight fast, venous blood was taken from each participant. EDTA anticoagulant tubes were used to collect whole blood samples, and whole transcriptome sequencing was done on blood samples from 4 MDD patients and 4 healthy controls. CircRNAs exhibiting | log2(fold change) | > 1 and p-value < 0.05 were identified as significant. Use the FPKM value to identify the mRNA of each coding gene. Differentially expressed mRNAs were identified based on | log2(fold change) | > 0.58 with a p-value < 0.05, and at least one sample of the gene FPKM value ≥ 1.
RNA Extraction and Real-Time Quantitative Reverse Transcription PCR
Total RNA was extracted by Trizol (Invitrogen, United States). According to the manufacturer’s protocol affiliated with Evo M-MLV RT Kit with gDNA Clean for qPCR II (Accurate Biology, China), circRNA was reverse transcribed to complementary cDNA and then quantified by SYBR Green Real-time PCR Master Mix (Accurate Biology, China). β-actin was used as the endogenous control, detailed information about these primers was provided in Supplementary Table 1. The expression levels of circRNAs were normalized to β-actin, and the relative expression levels of circRNAs were shown by the value of the 2–ΔΔCt.
Enzyme-Linked Immunosorbent Assay (ELISA) Analyses
The plasma was extracted by centrifugation at 3,000 rpm, 27°C for 10 min. By the manufacturer’s protocols, ELISA kits (Multi Sciences, China) were used to detect the plasma concentration of BDNF, GDNF, and β-NGF. The maximum absorption wavelength at 450 nm and the reference wavelength at 570 nm was measured using a microplate reader (BioTek Epoch, United States), then subtracting readings at 570 nm from the readings at 450 nm as calibration optical density (OD) value.
Bioinformatics Analysis
Based on miRanda1, we predicted potential miRNA targets for the target circRNAs. The potential mRNA targets of the miRNAs were predicted by TargetScan2). Cytoscape3 was used to delineate the circRNA–miRNA–mRNA network. Using clusterProfiler package to perform gene ontology (GO) analysis to analyze the potential function of the target gene. Kyoko Encyclopedia of Genes and Genomes (KEGG) enrichment analysis analyzed the related pathways of target genes through KOBAS 3.04.
Statistical Analysis
The difference of candidate circRNAs expression between MDD patients and healthy controls was evaluated by t-test. Demographic variables were compared between MDD patients and healthy controls with unpaired t-test with Welch’s correction, Fisher’s exact test, or Mann Whitney test. All tests were two-sided and P-value < 0.05 was considered statistically significant. Statistical analysis was performed with GraphPad Prism (GraphPad Software, United States) version 8.42.
Results
CircRNAs Expression Profile in Major Depressive Disorder Patients and Healthy Controls
We used whole transcriptome sequencing to analyze blood samples from four MDD patients and four healthy controls and identified 445 circRNAs expressing differentially (378 downregulated and 67 upregulated) (Figure 1A). The expression profiles of circRNAs in MDD patients and healthy controls exhibited good consistency, as shown in the heat map analysis of the top 100-dysregulated circRNAs (Figure 1B).
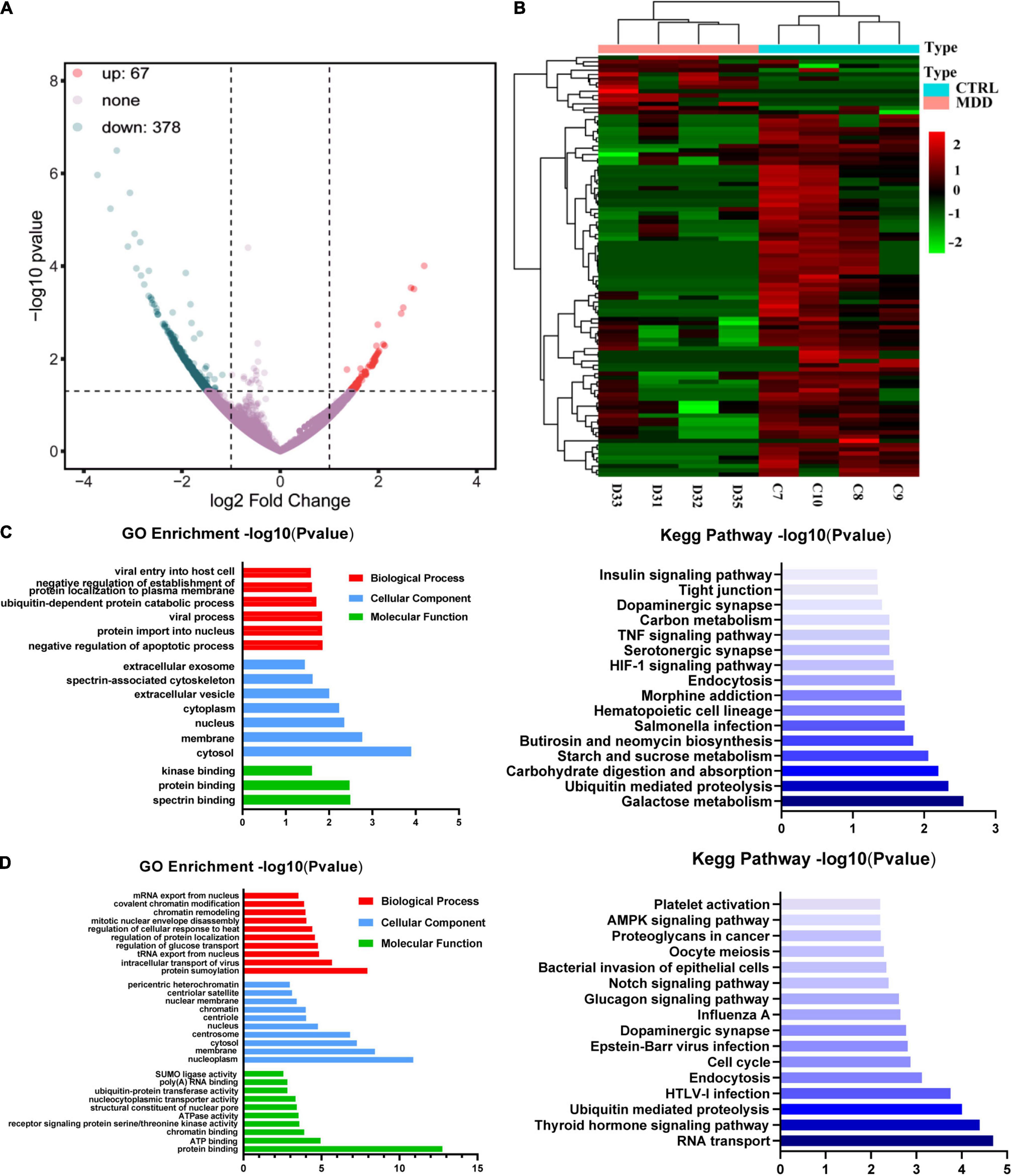
Figure 1. CircRNA expression profiles. (A) Volcano plot of dysregulated circRNAs between 4 MDD patients and 4 healthy controls. (B) Heatmap of top 100 dysregulated circRNAs between 4 MDD patients and 4 healthy. (C) GO and KEGG enrichment analyses based on upregulated circRNAs in MDD patients. (D) GO and KEGG enrichment analyses based on downregulated circRNAs in MDD patients.
Enrichment Analyses of Dysregulated CircRNAs
Gene ontology enrichment analysis and KEGG enrichment analysis based on upregulated circRNAs were shown in Figure 1C. In the GO enrichment results of upregulated circRNAs, negative regulation of apoptotic process (GO: 0043066), protein import into nucleus (GO: 0006606), viral process (GO: 0016032), ubiquitin-dependent protein catabolic process (GO: 0006511), negative regulation of establishment of protein localization to plasma membrane (GO: 0090005) and viral entry into host cell (GO: 0046718) were the mainly enriched biological process terms. In addition, KEGG enrichment analysis (Figure 1C) showed that upregulated circRNAs were enriched in neuron-related pathways: serotonergic synapses (hsa04726) and dopaminergic synapses (hsa04728). GO enrichment analysis and KEGG enrichment analysis based on downregulated circRNAs were shown in Figure 1D. The top five biological process terms of downregulated circRNAs were as follows: protein sumoylation (GO: 0016925), intracellular transport of virus (GO: 0075733), tRNA export from nucleus (GO: 0006409), regulation of glucose transport (GO: 0010827) and regulation of protein localization (GO: 0032880). The first 5 pathways of KEGG enrichment analysis showed that downregulated circRNAs were enriched in RNA transport (hsa03013), thyroid hormone signaling pathway (hsa04919), ubiquitin mediated proteolysis (hsa04120), HTLV-I infection (hsa05166), and endocytosis (hsa04144).
Validation of 20 Dysregulated Candidate CircRNAs
We selected 20 dysregulated circRNAs (the top 10 upregulated and the top 10 downregulated) for further study (Table 2). Heat map analysis of the 10 upregulated circRNAs (Figure 2A) and the 10 downregulated circRNAs (Figure 2B) showed that both could distinguish MDD patients from healthy controls. The 20 dysregulated circular RNAs were further verified by RT-qPCR in 29 MDD patients and 19 healthy controls, subsequently, 5 upregulated circRNAs were consistent with circRNAs sequencing among the 20 dysregulated circRNAs: hsa_circ_0002473, hsa_circ_0079651, hsa_circ_0137187, hsa_circ_0006010 and hsa_circ_0113010 (Figures 2C,D). The receiver operating characteristic curve (ROC) analysis was performed on these five circRNAs to assess their diagnostic capability for MDD (Figure 2E). All of them were able to discriminate between MDD patients and healthy controls, and hsa_circ_0002473 and hsa_circ_0006010 exhibited better predictive capacity of biomarkers (AUC = 0.8619 and AUC = 0.8367, respectively).
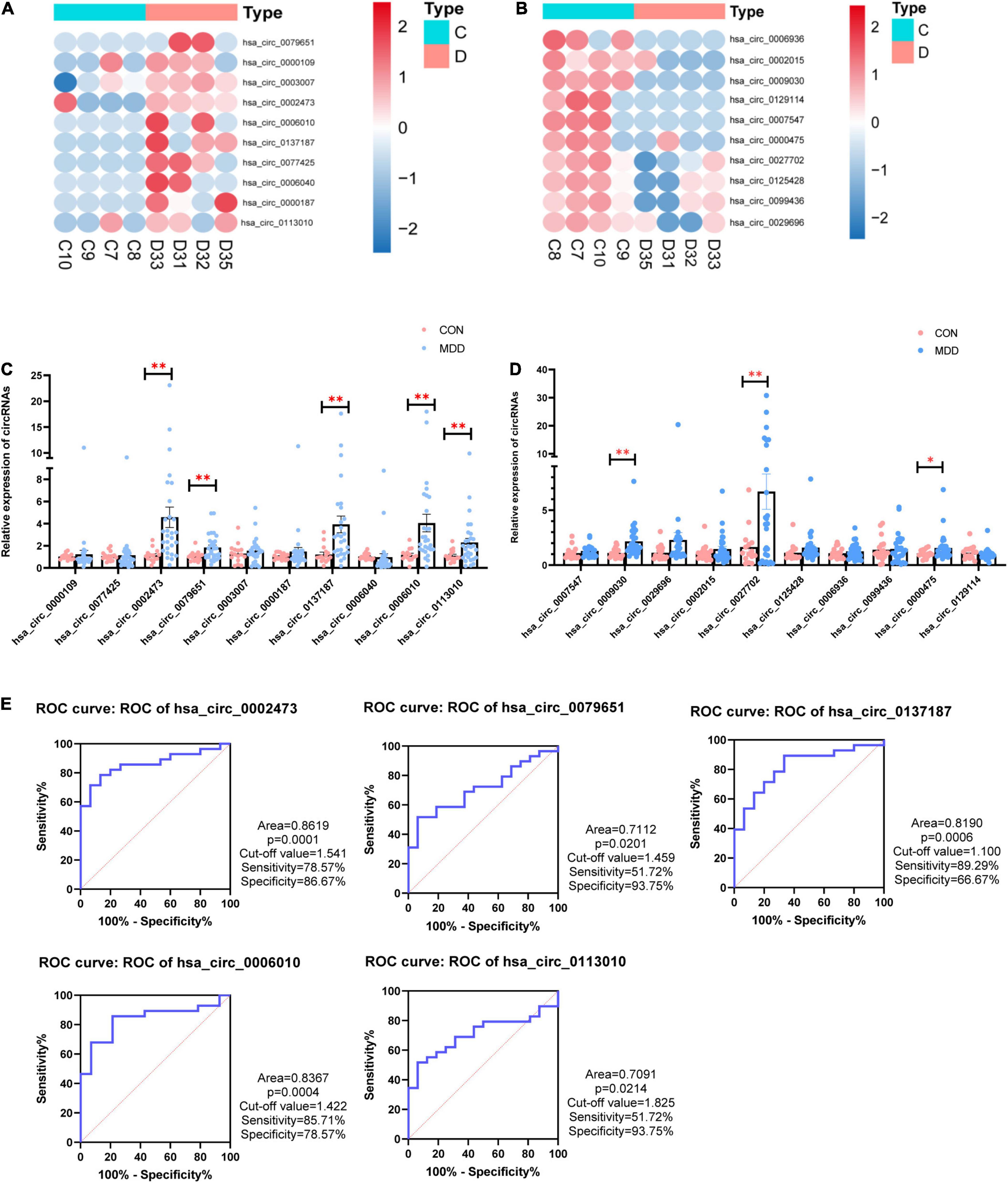
Figure 2. 20 candidate CircRNAs (top 10 upregulated and top 10 downregulated) in MDD patients and healthy controls. (A) Heatmaps of top 10 upregulated circRNAs. (B) Heatmaps of top 10 downregulated circRNAs. (C) Comparison of top 10 upregulated circRNAs relative expressions between 29 MDD patients and 19 healthy controls. (D) Comparison of top 10 downregulated circRNAs relative expressions between 29 MDD patients and 19 healthy controls. (E) ROC curve analyses of 5 verified candidate circRNAs for predicting MDD risk. Data are presented as mean ± standard error of the mean (SEM). *p < 0.05, **p < 0.01.
Functional Prediction of the ceRNA Network of Verified Candidate CircRNAs
All the sequence information of human miRNA was downloaded from the miRBase database5, and then miRanda software was used to predict the binding relationship between circRNAs and miRNAs. The binding relationship between miRNAs and mRNAs was predicted using the binding relationship between miRNA and mRNA in the TargetScan database. For the mRNAs we used 150 genes that had been detected to be upregulated in whole transcriptome sequencing (Supplementary Table 2). Based on these five circRNAs, we obtained a ceRNA network, including 11 miRNA and 36 mRNA (Figure 3A). Among them, we found hsa_circ_0002473 had no ceRNA relationship pair. The probable roles of these 36 mRNAs connected to the ceRNA network were predicted using GO and KEGG enrichment analysis. These mRNAs were linked to the growth and differentiation of neurons, according to biological process items (Figure 3B): hypothalamus cell differentiation (GO: 0021979), regulation of dopaminergic neuron differentiation (GO: 1904338), midbrain dopaminergic neuron differentiation (GO: 1904948), central nervous system neuron differentiation (GO: 0021953), hypothalamus development (GO: 0021854), neurotransmitter receptor transport (GO: 0099637) and forebrain neuron development (GO: 0021884). In terms of cellular components, these mRNAs were enriched in voltage-gated potassium channel complex (GO: 0008076), potassium channel complex (GO: 0034705), extrinsic component of postsynaptic membrane (GO: 0098890), and so on. In addition, these mRNAs were enriched in molecular function such as G protein-coupled peptide receptor activity (GO: 0008528), peptide receptor activity (GO: 0001653), potassium ion transmembrane transporter activity (GO: 0015079), and G-quadruplex DNA binding (GO: 0051880), etc. Regarding KEGG pathways based on target mRNAs (Figure 3C), it was disclosed that 36 mRNAs were enriched in nitrogen metabolism (hsa00910) and fatty acid biosynthesis (hsa00061).
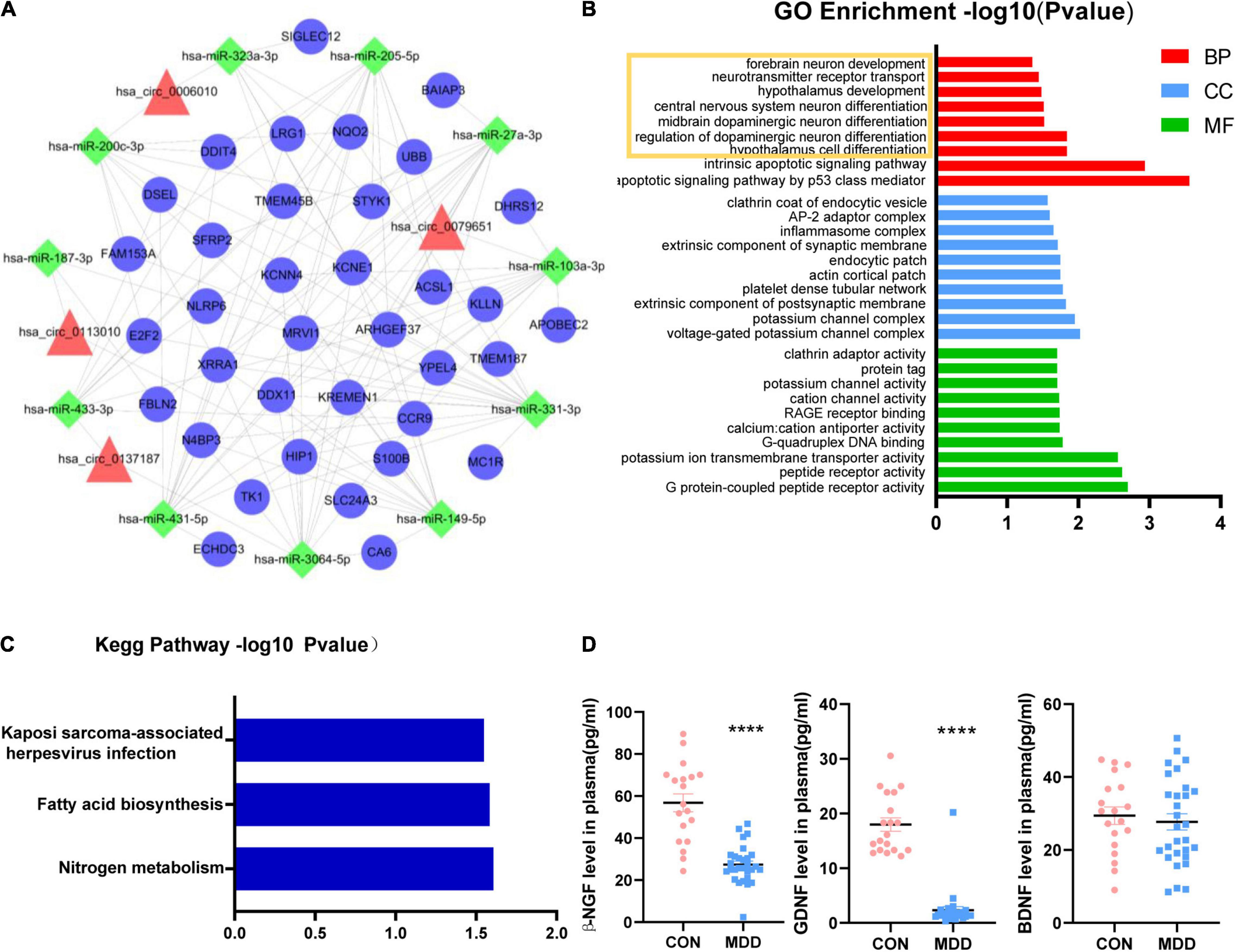
Figure 3. Functional prediction of circHNRNPA2B1, circZFAND1, circDPY19L1P1, and circEPB41. (A): CircRNA-miRNA-mRNA network. Four candidate circRNAs with their potential target 11 miRNAs and 36 mRNAs. Triangles represent circRNAs, squares represent miRNAs and circles represent mRNAs. (B) Part of GO enrichment analysis items based on 36 mRNAs that related to ceRNA network. (C) KEGG enrichment analysis based on 36 mRNAs that related to ceRNA network. (D) The plasma concentration of β-NGF, GDNF, and BDNF between 29 MDD patients and 19 healthy controls. Data are presented as mean ± standard error of the mean (SEM). ****p < 0.0001.
Neurotrophic Factors β-NGF, GDNF Were Decreased in the Plasma of Major Depressive Disorder Patients
The volume and density of neurons in the dorsolateral prefrontal cortex (dlPFC) and dentate gyrus (DG) were observed to be lower in MDD patients (Rajkowska, 2000; Boldrini et al., 2013) which was consistent with our KEGG enrichment analysis (Figure 1C) and biological process prediction (Figure 3B). Neurotrophic factors (NF) such as Glial cell-derived neurotrophic factor (GDNF), beta-nerve growth factor (β-NGF), and brain-derived neurotrophic factor (BDNF), play key factors in promoting neuron growth, maturing, and repairing (Allen et al., 2013; Xiao and Le, 2016) and MDD patients have neurotrophic factor disorders (Shi et al., 2020). We wondered if there are similar changes in the samples we collected. Therefore, we indirectly evaluate the changes in brain neurons by detecting the plasma neurotrophic factors. The results of plasma ELISA (Figure 3D) showed that β-NGF, GDNF was decreased in MDD patients and BDNF was unchanged in MDD patients. In addition, we found that the concentration of β-NGF and GDNF were not associated with the relative expression of these 5 circRNAs.
Discussion
In this study, we performed whole transcriptome sequencing on the whole blood RNA of MDD patients and healthy controls: 67 upregulated circRNAs and 378 downregulated circRNAs (Figure 1A). In addition, upregulated circRNAs were enriched in neuron-related pathways: serotonergic synapses and dopaminergic synapses (Figure 1C). Downregulated circRNAs were enriched in RNA transport, thyroid hormone signaling pathway, ubiquitin mediated proteolysis, HTLV-I infection, and so on (Figure 1D). Then, we selected 20 dysregulated circRNAs (the top 10 upregulated and the top 10 downregulated) (Table 2) for further study. Five upregulated circRNAs: hsa_circ_0002473, hsa_circ_0079651, hsa_circ_0137187, hsa_circ_0006010, and hsa_circ_0113010 were specifically expressed in MDD patients (Figure 2C), and could distinguish MDD patients from healthy controls (Figure 2E). We did not validate circRNAs with the same expression trend in the down-regulated circRNAs (Figure 2D). According to the present research, hsa_circ_0002473, hsa_circ_0079651, hsa_circ_0137187, hsa_circ_0006010, and hsa_circ_0113010 might be novel biomarkers for MDD.
CircRNAs can act as miRNA sponges, preventing miRNAs from binding and suppressing target mRNA (Chen, 2020). In this study, these five circRNAs were discovered for the first time. For these five circRNAs, we constructed a ceRNA network, and this ceRNA network revealed that 36 mRNA may reflect the function of these circRNAs in MDD (Figure 3A). We discovered that hsa_circ_0002473 has no ceRNA relationship, suggesting it does not function as a ceRNA. Biological process items showed that these 36 mRNAs were related to the growth and differentiation of neurons (Figure 3B): regulation of dopaminergic neuron differentiation, midbrain dopaminergic neuron differentiation, central nervous system neuron differentiation, hypothalamus development, neurotransmitter receptor transport, and forebrain neuron development, which was consistent with the KEGG enrichment analysis of the total upregulated circRNAs (Figure 1C). It implies that there may be neuronal changes in MDD patients. Moreover, the size and density of the dorsolateral prefrontal cortex (dlPFC) and dentate gyrus (DG) neurons in MDD patients were reduced (Rajkowska, 2000; Boldrini et al., 2013). There was evidence that neurotrophic factors regulate neurogenesis, and MDD patients often present with neurotrophic factor disorders (Shi et al., 2020). We would like to find out whether neurotrophic factors are affected in this study and if circRNAs are related to neurotrophic factors.
It is known that neurotrophic factors are able to promote the growth, proliferation, differentiation, and survival of neurons; neurotrophic factors such as BDNF, GDNF, and NGF are dysregulated in MDD patients and are important in antidepressant drug mechanisms (Saavedra et al., 2008; Allen et al., 2013; Song et al., 2017; Sun et al., 2019; Castrén and Monteggia, 2021). In this study, β-NGF and GDNF levels decreased in MDD patients, while BDNF levels were unaffected (Figure 3D). We also found that β-NGF and GDNF concentrations do not affect the relative expression of these five circRNAs. There is still no consensus on how these three neurotrophic factors differ in MDD individuals. The majority of research showed that BDNF expression in MDD decreased (de Azevedo Cardoso et al., 2014; Phillips, 2017; Kojima et al., 2019; Teng et al., 2021). The results of our experiment contradicted this. This could be due to different populations in our experiment, or it could be due to a lack of volunteers, resulting in a false negative. Regarding GDNF, one study found the level of GDNF was decreased in young MDD patients (age 13–24 years) but not in old MDD patients (age 25–45 years) (Sun et al., 2019). According to two meta-analyses, NGF levels were significantly lower in MDD than in healthy controls (Chen et al., 2015; Wiener et al., 2015; Çakici et al., 2020), which was consistent with our finding. Another study of depression in adolescent patients discovered increased BDNF but no changes in NGF or GDNF (Bilgic et al., 2020). Taken together, these findings indicate the existence of aberrant neurotrophic factors in patients with MDD, which may be a significant etiology of major depression or simply a “consequence” of the stress response to depression, and restoring neurotrophic factor homeostasis may help relieve depression symptoms.
The above information suggested that hsa_circ_0079651, hsa_circ_0137187, hsa_circ_0006010, and hsa_circ_0113010 are suspected of being involved in the neuroplasticity of MDD, although the specific mechanism requires additional investigation. In the ceRNA network, 11 related miRNAs were discovered (Figure 3A); among them, hsa-miR-103a-3p (Devoto et al., 2020), hsa-miR-27a-3p (Sala Frigerio et al., 2013), and hsa-miR-200c-3p (Lee et al., 2018) were abnormal in neuropsychiatric diseases in previous investigations. Among the 36 mRNAs associated with the ceRNA network (Figure 3A), 3 mRNAs had been reported to be related to depression (DDIT4, S100B, Fbln2). DDIT4 levels were elevated in MDD patients and had been verified to be related to neuronal atrophy and depressive behavior caused by chronic stress (Malagelada et al., 2011; Ota et al., 2014; Wang et al., 2018; Perez-Sisques et al., 2021); S100B levels were also elevated in MDD patients, and associated with glial cell dysregulation (Gos et al., 2013; Güleş et al., 2020; O’Leary and Mechawar, 2021; Zhang et al., 2021). Reduced Fbln2 alleviates the state of cognitive impairment and neural function in mice with chronic unpredictable mild stress (CUMS) through inhibiting the TGF-β1 signaling pathway (Tang et al., 2018). Additionally, 15 mRNAs had been linked to neurogenesis or neuropsychiatric disorders (Table 3). These investigations back up the current findings that hsa_circ_0079651, hsa_circ_0137187, hsa_circ_0006010, and hsa_circ_0113010 are linked to neuroplasticity in MDD. However, just a few studies have delved into the mechanisms of circRNAs in depression. One study found circDYM improved depressive-like behavior in mice through inhibition of miR-9 activity and leading to increased expression of the downstream target HECTD1 and increase of HSP90 ubiquitination then reducing microglia activation (Zhang et al., 2018). The gut microbiota of NLRP3 KO mice transplanted into chronic unpredictable stress (CUS) mice modulated astrocyte dysfunction and improved depression-like behavior in CUS mice via circHIPK2 (Zhang et al., 2019). CircSTAG1 was also found to alleviate depressive-like behavior in CUS mice: circSTAG1 trapped ALKBH5 and reduced its translocation to the nucleus, leading to increased m6A methylation of FAAH mRNA and degradation of FAAH in astrocytes, followed by alleviation of depressive-like behavior in mice (Huang et al., 2020). Likewise, our study provides clues to the role of circular RNAs in MDD: circRNAs may affect neuroplasticity through ceRNA mechanisms in MDD patients, and their specific pathway in MDD merit more study.
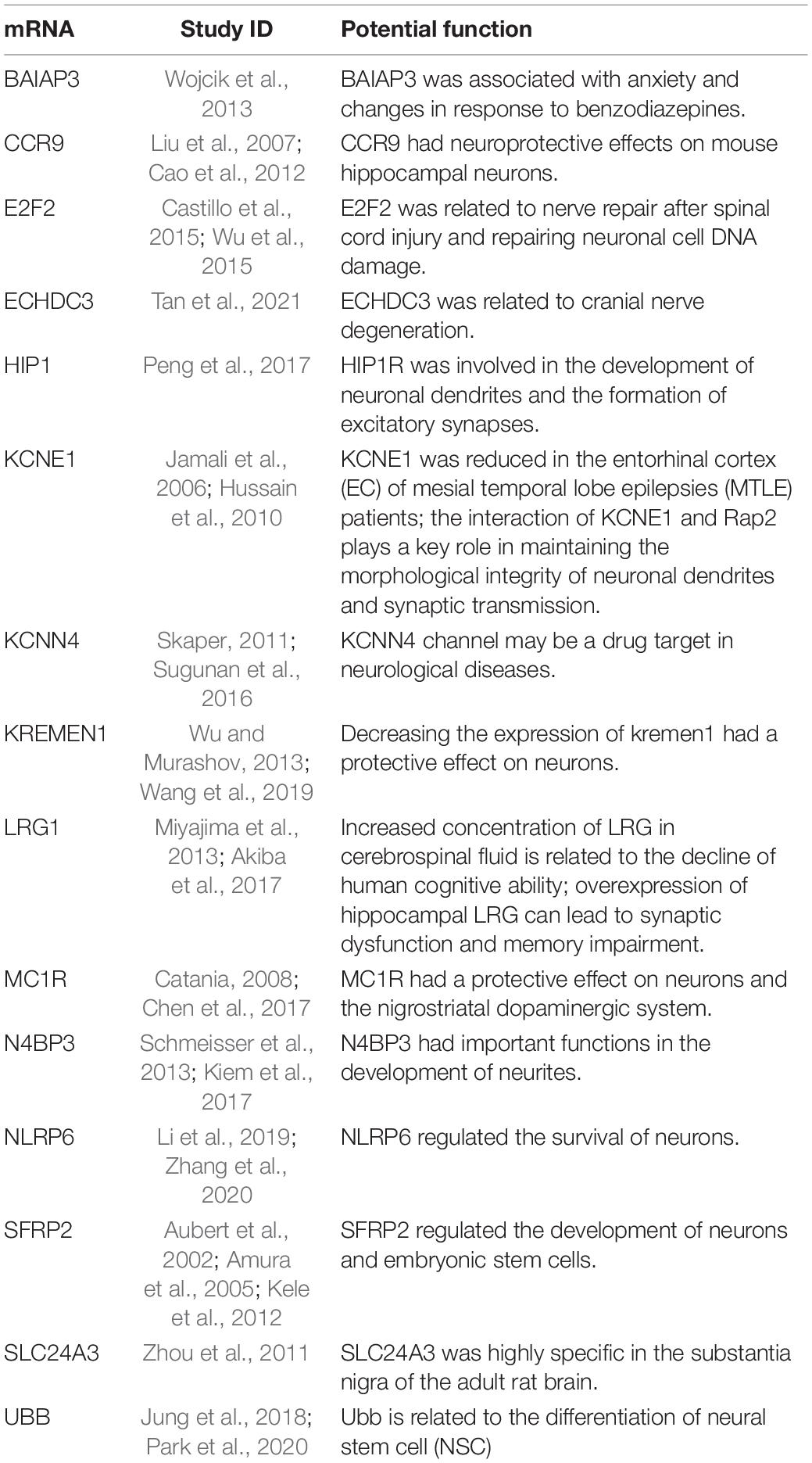
Table 3. The potential function of 15 mRNAs in neurogenesis or neuropsychiatric disorders based on previous studies.
To sum up the above, we found the disorder of circRNAs in the blood of MDD patients and preliminarily demonstrated that hsa_circ_0002473, hsa_circ_0079651, hsa_circ_0137187, hsa_circ_0006010, and hsa_circ_0113010 were highly expressed in MDD patients and could be used as diagnostic markers for MDD. Among them, hsa_circ_0079651, hsa_circ_0137187, hsa_circ_0006010, and hsa_circ_0113010 that may affect the neuroplasticity of MDD through the ceRNA mechanism, and their exact pathway in MDD deserve further study.
Data Availability Statement
The datasets presented in this study can be found in online repositories. The names of the repository/repositories and accession number(s) can be found below: https://www.ncbi.nlm.nih.gov/geo/query/acc.cgi?acc=GSE190518.
Ethics Statement
The studies involving human participants were reviewed and approved by Ethics Committee of Guangdong Medical University. Written informed consent to participate in this study was provided by the participants’ legal guardian/next of kin.
Author Contributions
YC and LC designed the study and conceptualization. YC, LC, DZ, and YJ finished the original draft. ZL, DZ, YW, QP, JL, JY, and HL diagnosed and collected samples. DZ, YJ, RC, and XC did the investigation and statistical analysis. YC and LC revised the investigation and methodology. All authors contributed to the article and approved the submitted version.
Funding
This work was supported by the Natural Science Foundation of Guangdong Province (2022A1515012275).
Conflict of Interest
The authors declare that the research was conducted in the absence of any commercial or financial relationships that could be construed as a potential conflict of interest.
Publisher’s Note
All claims expressed in this article are solely those of the authors and do not necessarily represent those of their affiliated organizations, or those of the publisher, the editors and the reviewers. Any product that may be evaluated in this article, or claim that may be made by its manufacturer, is not guaranteed or endorsed by the publisher.
Acknowledgments
We thank Jiwei Li for providing technical assistance in bioinformatics analysis.
Supplementary Material
The Supplementary Material for this article can be found online at: https://www.frontiersin.org/articles/10.3389/fnins.2022.844422/full#supplementary-material
Footnotes
- ^ http://www.microrna.org/microrna/home.do
- ^ http://www.targetscan.org/
- ^ http://cytoscape.github.io/
- ^ http://kobas.cbi.pku.edu.cn/
- ^ https://mirbase.org/
References
Akiba, C., Nakajima, M., Miyajima, M., Ogino, I., Miura, M., Inoue, R., et al. (2017). Leucine-rich α2-glycoprotein overexpression in the brain contributes to memory impairment. Neurobiol. Aging 60, 11–19. doi: 10.1016/j.neurobiolaging.2017.08.014
Allen, S. J., Watson, J. J., Shoemark, D. K., Barua, N. U., and Patel, N. K. (2013). GDNF, NGF and BDNF as therapeutic options for neurodegeneration. Pharmacol. Ther. 138, 155–175. doi: 10.1016/j.pharmthera.2013.01.004
Amura, C. R., Marek, L., Winn, R. A., and Heasley, L. E. (2005). Inhibited neurogenesis in JNK1-deficient embryonic stem cells. Mol. Cell. Biol. 24, 10791–10802. doi: 10.1128/MCB.25.24.10791
Aubert, J., Dunstan, H., Chambers, I., and Smith, A. (2002). Functional gene screening in embryonic stem cells implicates Wnt antagonism in neural differentiation. Nat. Biotechnol. 20, 1240–1245. doi: 10.1038/nbt763
Beurel, E., Toups, M., and Nemeroff, C. B. (2020). The bidirectional relationship of depression and inflammation: double trouble. Neuron 107, 234–256. doi: 10.1016/j.neuron.2020.06.002
Bilgic, A., Celikkol, S. C., Kilinc, I., and Akca, O. F. (2020). Exploring the association between depression, suicidality and serum neurotrophin levels in adolescents. Int. J. Psychiatry Clin. Pract. 24, 143–150. doi: 10.1080/13651501.2020.1723643
Boldrini, M., Santiago, A. N., Hen, R., Dwork, A. J., Rosoklija, G. B., Tamir, H., et al. (2013). Hippocampal granule neuron number and dentate gyrus volume in antidepressant-treated and untreated major depression. Neuropsychopharmacol. 38, 1068–1077. doi: 10.1038/npp.2013.5
Çakici, N., Sutterland, A. L., Penninx, B. W. J. H., Dalm, V. A., de Haan, L., and van Beveren, N. J. M. (2020). Altered peripheral blood compounds in drug-naïve first-episode patients with either schizophrenia or major depressive disorder: a meta-analysis. Brain Behav. Immun. 88, 547–558. doi: 10.1016/j.bbi.2020.04.039
Cao, X., Ma, J., Wu, G., Zhang, C., Wang, L., Dai, S., et al. (2012). Thymus-expressed chemokine promotes survival of PC12 cells via PI3K pathway. Neurochem. Int. 60, 163–169. doi: 10.1016/j.neuint.2011.05.005
Castillo, D. S., Campalans, A., Belluscio, L. M., Carcagno, A. L., Radicella, J. P., Canepa, E. T., et al. (2015). E2F1 and E2F2 induction in response to DNA damage preserves genomic stability in neuronal cells. Cell Cycle 14, 1300–1314. doi: 10.4161/15384101.2014.985031
Castrén, E., and Monteggia, L. M. (2021). Brain-Derived neurotrophic Factor signaling in depression and antidepressant action. Biological. Psychiatry 90, 128–136. doi: 10.1016/j.biopsych.2021.05.008
Catania, A. (2008). Neuroprotective actions of melanocortins: a therapeutic opportunity. Trends Neurosci. 31, 353–360. doi: 10.1016/j.tins.2008.04.002
Chen, L. (2020). The expanding regulatory mechanisms and cellular functions of circular RNAs. Nat. Rev. Mol. Cell Biol. 21, 475–490. doi: 10.1038/s41580-020-0243-y
Chen, X., Chen, H., Cai, W., Maguire, M., Ya, B., Zuo, F., et al. (2017). The melanoma-linked “redhead” MC1R influences dopaminergic neuron survival. Ann. Neurol. 81, 395–406. doi: 10.1002/ana.24852
Chen, Y. W., Lin, P. Y., Tu, K. Y., Cheng, Y. S., Wu, C. K., and Tseng, P. T. (2015). Significantly lower nerve growth factor levels in patients with major depressive disorder than in healthy subjects: a meta-analysis and systematic review. Neuropsychiatr. Dis. Treat 11, 925–933. doi: 10.2147/NDT.S81432
GBD 2017 Disease and Injury Incidence and Prevalence Collaborators (2018). Global, regional, and national incidence, prevalence, and years lived with disability for 354 diseases and injuries for 195 countries and territories, 1990–2017: a systematic analysis for the Global Burden of Disease Study 2017. Global Health Metr. 392, 1789–1858. doi: 10.1016/S0140-6736(18)32279-7
Cui, X., Niu, W., Kong, L., He, M., Jiang, K., Chen, S., et al. (2016). hsa_circRNA_103636: potential novel diagnostic and therapeutic biomarker in Major depressive disorder. Biomark. Med. 10, 943–952. doi: 10.2217/bmm-2016-0130
de Azevedo Cardoso, T., Mondin, T. C., Wiener, C. D., Marques, M. B., Fucolo, B. D. Á, Pinheiro, R. T., et al. (2014). Neurotrophic factors, clinical features and gender differences in depression. Neurochem. Res. 39, 1571–1578. doi: 10.1007/s11064-014-1349-4
Devoto, C., Lai, C., Qu, B., Guedes, V. A., Leete, J., Wilde, E., et al. (2020). Exosomal micrornas in military personnel with mild traumatic brain injury: preliminary results from the chronic effects of neurotrauma consortium biomarker discovery project. J. Neurotraum. 37, 2482–2492. doi: 10.1089/neu.2019.6933
Gokool, A., Loy, C. T., Halliday, G. M., and Voineagu, I. (2020). Circular RNAs the brain transcriptome comes full circle. Trends Neurosci. 43, 752–766. doi: 10.1016/j.tins.2020.07.007
Gonda, X., Petschner, P., Eszlari, N., Baksa, D., Edes, A., Antal, P., et al. (2019). Genetic variants in major depressive disorder: from pathophysiology to therapy. Pharmacol. Therapeut. 194, 22–43. doi: 10.1016/j.pharmthera.2018.09.002
Gos, T., Schroeter, M. L., Lessel, W., Bernstein, H., Dobrowolny, H., Schiltz, K., et al. (2013). S100B-immunopositive astrocytes and oligodendrocytes in the hippocampus are differentially afflicted in unipolar and bipolar depression: a postmortem study. J. Psychiatr. Res. 47, 1694–1699. doi: 10.1016/j.jpsychires.2013.07.005
Güleş, E., Iosifescu, D. V., and Tural, Ü (2020). Plasma neuronal and glial markers and anterior cingulate metabolite levels in major depressive disorder: a pilot study. Neuropsychobiology 79, 214–221. doi: 10.1159/000505782
Huang, R., Zhang, Y., Bai, Y., Han, B., Ju, M., Chen, B., et al. (2020). N6-methyladenosine modification of fatty acid amide hydrolase messenger RNA in circular RNA STAG1–regulated astrocyte dysfunction and depressive-like behaviors. Biological. psychiatry 88, 392–404. doi: 10.1016/j.biopsych.2020.02.018
Huang, Y., Wang, Y., Wang, H., Liu, Z., and Yu, X. (2019). Prevalence of mental disorders in China: a cross-sectional epidemiological study. Lancet Psychiatry 6, 211–224. doi: 10.1016/S2215-0366(18)30511-X
Hussain, N. K., Hsin, H., Huganir, R. L., and Sheng, M. (2010). MINK and TNIK differentially act on rap2-mediated signal transduction to regulate neuronal structure and AMPA receptor function. J. Neurosci. 30, 14786–14794. doi: 10.1523/JNEUROSCI.4124-10.2010
Jamali, S., Bartolomei, F., Robaglia-Schlupp, A., Massacrier, A., Peragut, J. C., Regis, J., et al. (2006). Large-scale expression study of human mesial temporal lobe epilepsy: evidence for dysregulation of the neurotransmission and complement systems in the entorhinal cortex. Brain 129, 625–641. doi: 10.1093/brain/awl001
Jung, B., Park, C., and Ryu, K. (2018). Temporal downregulation of the polyubiquitin gene Ubb affects neuronal differentiation, but not maturation, in cells cultured in vitro. Sci. Rep. 8:1. doi: 10.1038/s41598-018-21032-6
Kele, J., Andersson, E. R., Villaescusa, J. C., Cajanek, L., Parish, C. L., Bonilla, S., et al. (2012). SFRP1 and SFRP2 Dose-dependently regulate midbrain dopamine neuron development in vivo and in embryonic stem cells. Stem. Cells 30, 865–875. doi: 10.1002/stem.1049
Kiem, L., Dietmann, P., Linnemann, A., Schmeisser, M. J., and Kühl, S. J. (2017). The Nedd4 binding protein 3 is required for anterior neural development in Xenopus laevis. Dev. Biol. 423, 66–76. doi: 10.1016/j.ydbio.2017.01.009
Kojima, M., Matsui, K., and Mizui, T. (2019). BDNF pro-peptide: physiological mechanisms and implications for depression. Cell Tissue Res. 377, 73–79. doi: 10.1007/s00441-019-03034-6
Lee, M., Cho, H., Jung, S. H., Yim, S., Cho, S., Chun, J., et al. (2018). Circulating MicroRNA Expression Levels Associated With Internet Gaming Disorder. Front. Psychiatry 9:81. doi: 10.3389/fpsyt.2018.00081
Li, J., Yu, R., Zhang, L., Wen, S., Wang, S., Zhang, X., et al. (2019). Dietary fructose-induced gut dysbiosis promotes mouse hippocampal neuroinflammation: a benefit of short-chain fatty acids. Microbiome 7:98. doi: 10.1186/s40168-019-0713-7
Liu, J. X., Cao, X., Tang, Y. C., Liu, Y., and Tang, F. R. (2007). CCR7, CCR8, CCR9 and CCR10 in the mouse hippocampal CA1 area and the dentate gyrus during and after pilocarpine-induced status epilepticus. J. Neurochem. 100, 1072–1088. doi: 10.1111/j.1471-4159.2006.04272.x
Malagelada, C., Lopez-Toledano, M. A., Willett, R. T., Jin, Z. H., Shelanski, M. L., and Greene, L. A. (2011). RTP801/REDD1 Regulates the timing of cortical neurogenesis and neuron migration. J. Neurosci. 31, 3186–3196. doi: 10.1523/JNEUROSCI.4011-10.2011
Malhi, G. S., and Mann, J. J. (2018). Depression. Lancet 392, 2299–2312. doi: 10.1016/S0140-6736(18)31948-2
Mehta, S. L., Dempsey, R. J., and Vemuganti, R. (2020). Role of circular RNAs in brain development and CNS diseases. Prog. Neurobiol. 186:101746. doi: 10.1016/j.pneurobio.2020.101746
Mei, S. X., Ai, Y., and Jeremy, E. W. (2019). Biogenesis and functions of circular RNAs come into focus. Trends Cell Biol. 30, 226–240. doi: 10.1016/j.tcb.2019.12.004
Miyajima, M., Nakajima, M., Motoi, Y., Moriya, M., Sugano, H., Ogino, I., et al. (2013). Leucine-rich α 2-glycoprotein is a novel biomarker of neurodegenerative disease in human cerebrospinal fluid and causes neurodegeneration in mouse cerebral cortex. PLoS One 8:e74453. doi: 10.1371/journal.pone.0074453
O’Leary, L. A., and Mechawar, N. (2021). Implication of cerebral astrocytes in major depression: a review of fine neuroanatomical evidence in humans. Glia 69, 2077–2099. doi: 10.1002/glia.23994
Ota, K. T., Liu, R., Voleti, B., Maldonado-Aviles, J. G., Duric, V., Iwata, M., et al. (2014). REDD1 is essential for stress-induced synaptic loss and depressive behavior. Nat. Med. 20, 531–535. doi: 10.1038/nm.3513
Park, C., Rosenblat, J. D., Brietzke, E., Pan, Z., Lee, Y., Cao, B., et al. (2019). Stress, epigenetics and depression: a systematic review. Neurosci. Biobehav. Rev. 102, 139–152. doi: 10.1016/j.neubiorev.2019.04.010
Park, C. W., Jung, B. K., and Ryu, K. Y. (2020). Disruption of the polyubiquitin gene Ubb reduces the self-renewal capacity of neural stem cells. Biochem. Biophys. Res. Commun. 527, 372–378. doi: 10.1016/j.bbrc.2020.04.042
Peng, L., Yang, Q., Xu, X., Du, Y., Wu, Y., Shi, X., et al. (2017). Huntingtin-interacting protein 1-related protein plays a critical role in dendritic development and excitatory synapse formation in hippocampal neurons. Front. Mol. Neurosci. 10:186. doi: 10.3389/fnmol.2017.00186
Perez-Sisques, L., Sancho-Balsells, A., Solana-Balaguer, J., Campoy-Campos, G., Vives-Isern, M., Soler-Palazon, F., et al. (2021). RTP801/REDD1 contributes to neuroinflammation severity and memory impairments in Alzheimer’s disease. Cell Death Dis. 12:616. doi: 10.1038/s41419-021-03899-y
Phillips, C. (2017). Brain-derived neurotrophic factor, depression, and physical activity: making the neuroplastic connection. Neural Plast. 2017:7260130. doi: 10.1155/2017/7260130
Rajkowska, G. (2000). Postmortem studies in mood disorders indicate altered numbers of neurons and glial cells. Biological. Psychiatry 48, 766–777. doi: 10.1016/S0006-3223(00)00950-1
Rybak-Wolf, A., Stottmeister, C., Glažar, P., Jens, M., Pino, N., Giusti, S., et al. (2015). Circular RNAs in the mammalian brain are highly abundant. Conserved Dynamically Expressed. Mol. Cell 58, 870–885. doi: 10.1016/j.molcel.2015.03.027
Saavedra, A., Baltazar, G., and Duarte, E. P. (2008). Driving GDNF expression: the green and the red traffic lights. Prog. Neurobiol. 86, 186–215. doi: 10.1016/j.pneurobio.2008.09.006
Sala Frigerio, C., Lau, P., Salta, E., Tournoy, J., Bossers, K., Vandenberghe, R., et al. (2013). Reduced expression of hsa-miR-27a-3p in CSF of patients with Alzheimer disease. Neurology 81, 2103–2106. doi: 10.1212/01.wnl.0000437306.37850.22
Schmeisser, M. J., Kuhl, S. J., Schoen, M., Beth, N. H., Weis, T. M., Grabrucker, A. M., et al. (2013). The Nedd4-binding protein 3 (N4BP3) is crucial for axonal and dendritic branching in developing neurons. Neural Dev. 8:18. doi: 10.1186/1749-8104-8-18
Shi, Y., Luan, D., Song, R., and Zhang, Z. (2020). Value of peripheral neurotrophin levels for the diagnosis of depression and response to treatment: a systematic review and meta-analysis. Eur. Neuropsychopharm. 41, 40–51. doi: 10.1016/j.euroneuro.2020.09.633
Shi, Y., Song, R., Wang, Z., Zhang, H., Zhu, J., Yue, Y., et al. (2021). Potential clinical value of circular RNAs as peripheral biomarkers for the diagnosis and treatment of major depressive disorder. EBioMedicine 66:103337. doi: 10.1016/j.ebiom.2021.103337
Skaper, S. D. (2011). Ion channels on microglia: therapeutic targets for neuroprotection. CNS Neurol. Disord. Drug Targets 10, 44–56. doi: 10.2174/187152711794488638
Song, M., Martinowich, K., and Lee, F. S. (2017). BDNF at the synapse: why location matters. Mol. Psychiatr. 22, 1370–1375. doi: 10.1038/mp.2017.144
Sugunan, S., Nampoothiri, S. S., Garg, T., and Krishnamurthy, R. G. (2016). Role of KCa3.1 channels in CNS diseases: a concise review. CNS Neurol. Disord. Drug Targets 15, 1299–1305. doi: 10.2174/1871527315666160822111913
Sun, J., Kong, L., Wu, F., Wei, Y., Zhu, Y., Yin, Z., et al. (2019). Decreased plasma glial cell line-derived neurotrophic factor level in major depressive disorder is associated with age and clinical severity. J. Affect. Disord. 245, 602–607. doi: 10.1016/j.jad.2018.11.068
Tan, M. S., Yang, Y. X., Xu, W., Wang, H. F., Tan, L., Zuo, C. T., et al. (2021). Associations of Alzheimer’s disease risk variants with gene expression, amyloidosis, tauopathy, and neurodegeneration. Alzheimers Res. Ther. 13:15. doi: 10.1186/s13195-020-00755-7
Tang, C., Yang, J. T., Liu, Q., Wang, Y., and Wang, W. (2018). Up-regulated miR-192-5p expression rescues cognitive impairment and restores neural function in mice with depression via the Fbln2-mediated TGF-β1 signaling pathway. FASEB J. 33, 606–618. doi: 10.1096/fj.201800210RR
Teng, Z., Wang, L., Li, S., Tan, Y., Qiu, Y., Wu, C., et al. (2021). Low BDNF levels in serum are associated with cognitive impairments in medication-naïve patients with current depressive episode in BD II and MDD. J. Affect. Disord. 293, 90–96. doi: 10.1016/j.jad.2021.06.018
Wang, H., Lu, B., and Chen, J. (2019). Knockdown of lncRNA SNHG1 attenuated Aβ25-35-inudced neuronal injury via regulating KREMEN1 by acting as a ceRNA of miR-137 in neuronal cells. Biochem. Bioph. Res. Commun. 518, 438–444. doi: 10.1016/j.bbrc.2019.08.033
Wang, Q., Zhao, G., Yang, Z., Liu, X., and Xie, P. (2018). Downregulation of microRNA1243p suppresses the mTOR signaling pathway by targeting DDIT4 in males with major depressive disorder. Int. J. Mol. Med. 41, 493–500. doi: 10.3892/ijmm.2017.3235
Wen, G., Zhou, T., and Gu, W. (2020). The potential of using blood circular RNA as liquid biopsy biomarker for human diseases. Protein Cell 12, 911–946. doi: 10.1007/s13238-020-00799-3
Wiener, C. D., de Mello Ferreira, S., Moreira, F. P., Bittencourt, G., de Oliveira, J. F., Lopez Molina, M., et al. (2015). Serum levels of nerve growth factor (NGF) in patients with major depression disorder and. suicide risk. J. Affect. Disord. 184, 245–248. doi: 10.1016/j.jad.2015.05.067
Wojcik, S. M., Tantra, M., Stepniak, B., Man, K. N., Muller-Ribbe, K., Begemann, M., et al. (2013). Genetic markers of a Munc13 protein family member, BAIAP3, are gender specifically associated with anxiety and benzodiazepine abuse in mice and humans. Mol. Med. 19, 135–148. doi: 10.2119/molmed.2013.00033
Wu, D., and Murashov, A. K. (2013). MicroRNA-431 regulates axon regeneration in mature sensory neurons by targeting the Wnt antagonist Kremen1. Front. Mol. Neurosci. 6:35. doi: 10.3389/fnmol.2013.00035
Wu, J., Sabirzhanov, B., Stoica, B. A., Lipinski, M. M., Zhao, Z., Zhao, S., et al. (2015). Ablation of the transcription factors E2F1-2 limits neuroinflammation and associated neurological deficits after contusive spinal cord injury. Cell Cycle 14, 3698–3712. doi: 10.1080/15384101.2015.1104436
Xiao, N., and Le, Q. (2016). Neurotrophic factors and their potential applications in tissue regeneration. Arch. Immunol. Ther. Exp. 64, 89–99.
Zhang, J., Jiang, N., Zhang, L., Meng, C., Zhao, J., and Wu, J. (2020). NLRP6 expressed in astrocytes aggravates neurons injury after OGD/R through activating the inflammasome and inducing pyroptosis. Int. Immunopharmacol. 80:106183. doi: 10.1016/j.intimp.2019.106183
Zhang, L., Verwer, R. W. H., Zhao, J., Huitinga, I., Lucassen, P. J., and Swaab, D. F. (2021). Changes in glial gene expression in the prefrontal cortex in relation to major depressive disorder, suicide and psychotic features. J. Affect. Disorders 295, 893–903. doi: 10.1016/j.jad.2021.08.098
Zhang, Y., Du, L., Bai, Y., Han, B., He, C., Gong, L., et al. (2018). CircDYM ameliorates depressive-like behavior by targeting miR-9 to regulate microglial activation via HSP90 ubiquitination. Mol. Psychiatr. 25, 1175–1190. doi: 10.1038/s41380-018-0285-0
Zhang, Y., Huang, R., Cheng, M., Wang, L., Chao, J., Li, J., et al. (2019). Gut microbiota from NLRP3-deficient mice ameliorates depressive-like behaviors by regulating astrocyte dysfunction via circHIPK2. Microbiome 7:116. doi: 10.1186/s40168-019-0733-3
Keywords: major depressive disorder, circular RNA, biomarker, neuroplasticity, bioinformatics analysis
Citation: Zhang D, Ji Y, Chen X, Chen R, Wei Y, Peng Q, Lin J, Yin J, Li H, Cui L, Lin Z and Cai Y (2022) Peripheral Blood Circular RNAs as a Biomarker for Major Depressive Disorder and Prediction of Possible Pathways. Front. Neurosci. 16:844422. doi: 10.3389/fnins.2022.844422
Received: 28 December 2021; Accepted: 10 March 2022;
Published: 31 March 2022.
Edited by:
Jee-Yeon Hwang, Creighton University, United StatesReviewed by:
Lili Zeng, Shanghai Jiao Tong University, ChinaKeun Woo Park, Burke Neurological Institute (BNI), United States
Copyright © 2022 Zhang, Ji, Chen, Chen, Wei, Peng, Lin, Yin, Li, Cui, Lin and Cai. This is an open-access article distributed under the terms of the Creative Commons Attribution License (CC BY). The use, distribution or reproduction in other forums is permitted, provided the original author(s) and the copyright owner(s) are credited and that the original publication in this journal is cited, in accordance with accepted academic practice. No use, distribution or reproduction is permitted which does not comply with these terms.
*Correspondence: Zhixiong Lin, emhpeGlvbmdsaW56akAxNjMuY29t; Yujie Cai, Y2FpeXVqaWVAZ2RtdS5lZHUuY24=
†These authors have contributed equally to this work