- 1Department of Drug Design and Pharmacology, University of Copenhagen, Copenhagen, Denmark
- 2Department of Veterinary and Animal Sciences, University of Copenhagen, Copenhagen, Denmark
- 3Department of Ophthalmology, Copenhagen University Hospital, Rigshospitalet, Glostrup, Denmark
- 4Department of Ophthalmology, University Medical Centre Ljubljana, Ljubljana, Slovenia
- 5Medical Faculty, University of Ljubljana, Ljubljana, Slovenia
- 6Division of Eye and Vision, Department of Clinical Neuroscience, St. Erik Eye Hospital, Karolinska Institutet, Stockholm, Sweden
- 7Centre for Public Health, Queen’s University Belfast, Belfast, United Kingdom
Glaucoma is a common ocular neurodegenerative disease characterized by the progressive loss of retinal ganglion cells and their axons. It is the most common cause of irreversible blindness. With an increasing number of glaucoma patients and disease progression despite treatment, it is paramount to develop new and effective therapeutics. Emerging new candidates are the receptor agonists of the incretin hormone glucagon-like-peptide-1 (GLP-1), originally used for the treatment of diabetes. GLP-1 receptor (GLP-1R) agonists have shown neuroprotective effects in preclinical and clinical studies on neurodegenerative diseases in both the brain (e.g., Alzheimer’s disease, Parkinson’s disease, stroke and diabetic neuropathy) and the eye (e.g., diabetic retinopathy and AMD). However, there are currently very few studies investigating the protective effects of GLP-1R agonists in the treatment of specifically glaucoma. Based on a literature search on PubMed, the Cochrane Library, and ClinicalTrials.gov, this review aims to summarize current clinical literature on GLP-1 receptor agonists in the treatment of neurodegenerative diseases to elucidate their potential in future anti-glaucomatous treatment strategies.
Introduction
Glaucoma is one of the most common neurodegenerative eye diseases and the leading cause of irreversible blindness. It is estimated to affect ∼112 million people worldwide by 2040 (Tham et al., 2014). Glaucoma is characterized by the progressive and irreversible loss of retinal ganglion cells, the output neurons of the retina. There are three primary risk factors for glaucoma; increasing age, genetic risk and elevated intraocular pressure (IOP). Elevated IOP is strongly associated with disease progression in ∼60% of patients, but all available anti-glaucomatous therapies (pharmacological and surgical) exclusively target IOP (Kolko, 2015, 2017; Kolko et al., 2015; Kalouda et al., 2017; Quigley, 2019). The number of glaucoma cases associated with an actually elevated IOP varies significantly across glaucoma subtypes, and many glaucoma patients progress to blindness despite low IOPs and/or are refractory to IOP-lowering treatments (Dielemans et al., 1994; Heijl et al., 2002; Topouzis and Anastasopoulos, 2007; Cedrone et al., 2008; Peters et al., 2013; Ho and Wong, 2019). Therefore, there is an urgent unmet need to explore new therapies targeting mechanisms of glaucomatous neurodegeneration in addition to IOP reduction. One such important new route of study in glaucoma, and the focus of this review, may be the glucagon-like peptide 1 (GLP-1).
Glucagon-like peptide 1 (GLP-1) is an incretin hormone that, like its fellow incretin hormone GIP (glucose-dependent insulinotropic polypeptide), stimulates a decrease in blood glucose levels after nutrient intake. GLP-1 achieves the decrease in blood glucose levels by potentiating glucose-induced insulin secretion (the “incretin”-effect), improving insulin sensitivity and inhibiting glucagon release. Furthermore, GLP-1 regulates weight and satiety and delays gastric emptying (Gutniak et al., 1992; Drucker, 2018; Yildirim Simsir et al., 2018). The first agents targeting GLP-1 receptor (GLP-1R) signaling were developed to optimize the treatment of type 2 diabetes mellitus (T2D) as an adjunct to metformin when therapeutic goals where not met (Yildirim Simsir et al., 2018). The first GLP-1R agonists received FDA-approval in 2005 and included exenatide. Subsequently, many different GLP-1R agonists were developed and approved, including: extended release exenatide (exenatide XR), liraglutide, lixisenatide, dulaglutide, and semaglutide (Table 1). Although all GLP-1R agonists are effective in stabilizing blood glucose levels, glycated hemoglobin (HbA1c), cholesterol levels, insulin sensitivity, and several other beneficial aspects of metabolism in T2D patients (Courrèges et al., 2008; Patel et al., 2014; Jinnouchi et al., 2015; Rizvi et al., 2015; Rizzo et al., 2015, 2016), drug administration has been challenging. Until recently, the treatments had to be administered subcutaneously (Table 1). In September 2019, the first oral GLP-1R agonist for T2D was approved by FDA (Davies et al., 2017; Aroda et al., 2019; Husain et al., 2019; Mosenzon et al., 2019; Pieber et al., 2019; Pratley et al., 2019; Rodbard et al., 2019; Rosenstock et al., 2019; The U.S. Food and Drug administration, 2019; Zinman et al., 2019a; Yabe et al., 2020; Yamada et al., 2020; Khoo and Lin, 2021). With its great beneficial systemic effects and promising results from previous randomized clinical trials with injectable semaglutide, the oral GLP-1R agonist, Rybelsus®, also seem to be a promising and convenient anti-obesity treatment option (Khoo and Lin, 2021).
Glucagon-like peptide 1 (GLP-1) agonists not only exert a beneficial systemic effect, but also cross the blood-brain barrier (Kastin et al., 2002; Secher et al., 2014; Yildirim Simsir et al., 2018). In this context, GLP-1R agonists have been shown to affect the central nervous system and exhibit neuroprotective properties in animal models of several neurodegenerative diseases, including Alzheimer’s and Parkinson’s disease, stroke, diabetic retinopathy, and ocular hypertension (McClean et al., 2011; Hao et al., 2012; Fan et al., 2014; Gonçalves et al., 2016; Zhang et al., 2018, 2019; Basalay et al., 2019; Yang et al., 2019; Chang et al., 2020; Ramos et al., 2020; Ren et al., 2020; Sterling et al., 2020; Zhai et al., 2020). The potential neuroprotective properties of GLP-1R agonists have also been demonstrated in clinical trials, with Novo Nordisk currently running a phase III clinical trial of oral semaglutide as a potential treatment option for Alzheimer’s disease in the announced EVOKE trial program (NCT04777409, NCT04777396).
In addition to GLP-1R agonists, orally administrated DPP4-inhibitors, e.g., sitagliptin and saxagliptin, which are known to potentiate the effect of endogenous GLP-1 by inhibiting its degradation, have also been clinically studied as protective agents against retinopathy (Chung et al., 2016), Alzheimer’s disease (Isik et al., 2017), and Parkinson’s disease (Svenningsson et al., 2016) in T2D patients, although DPP4-inhibitors cannot cross the blood-brain barrier (Mousa and Ayoub, 2019).
Recently, a registry-based case-control study of 1,961 patients also associated the use of GLP-1R agonists with a reduced risk of glaucoma (Sterling et al., 2021), strongly supporting further research into the use of agents that increase GLP-1R signaling as anti-glaucomatous treatment strategies. Accordingly, previous studies of oral antidiabetics and insulin have also been proposed for the treatment of glaucoma as well as other ocular conditions such as age-related macular degeneration (AMD) (Ott et al., 2014; Lin et al., 2015, 2017; Chung et al., 2016, 2019; Maleškić et al., 2017; Douros et al., 2018; Kim et al., 2018; Li et al., 2018; Vilsbøll et al., 2018; Wang et al., 2018; Brown et al., 2019; Chen et al., 2019; Fan et al., 2020; Gaborit et al., 2020; Blitzer et al., 2021; Sterling et al., 2021). The present review aims to summarize current literature on antidiabetics in the treatment of neurodegenerative diseases with a special focus on GLP-1R agonists and glaucoma.
Method of Literature Search
PubMed and the Cochrane Library were searched in April 2021 (Figure 1). The search was not limited to a specific language. However, mainly clinical studies and clinically inclined reviews were included as core sources. In addition, the Cochrane Library was screened for relevant Cochrane reviews only. MeSH-controlled vocabulary terms and related keywords were combined using appropriate Boolean operators to find literature either investigating or discussing incretin-based drugs (GLP-1R agonists and DPP4-inhibitors) for the treatment of neurodegenerative diseases, particularly glaucoma, diabetic retinopathy, AMD, Alzheimer’s disease, Parkinson’s disease, stroke and neuropathy. Search words included: GLP-1, Glucagon-Like Peptide 1, semaglutide, oral semaglutide, Rybelsus, Ozempic, liraglutide, lixisenatide, dulaglutide, albiglutide, exenatide, DPP4 inhibitor, dipeptidyl phosphatase 4 inhibitor, sitagliptin, antidiabetics, metformin, insulin, type 2 diabetes, diabetes, diabetes mellitus, neurodegenerative diseases, glaucoma, AMD, age-related macular degeneration, diabetic retinopathy, elderly, Alzheimer, dementia, cognitive impairment, Alzheimer’s Disease, Parkinson, Parkinson’s Disease, stroke, neuropathy. The reference lists of all core sources were searched for additional references. Completed and ongoing trials examining the role of GLP-1R agonists, especially semaglutide, in the treatment of glaucoma, AMD, Alzheimer’s, and Parkinson’s disease, were searched on the public clinical trial registry clinicaltrials.gov (NCT04777409, NCT04777396, NCT03811561, NCT01843075, NCT04232969, NCT03659682, NCT02953665, NCT04305002, NCT03439943, NCT04269642, NCT04154072, NCT02673931, NCT02829502, NCT02838589, NCT00418288, NCT00256256, NCT03948347, NCT03287076, NCT02684578, NCT05035095). Completed clinical trials with unknown status, no recent updates, no public or published results, and no relevance to the subject of the current review were excluded.
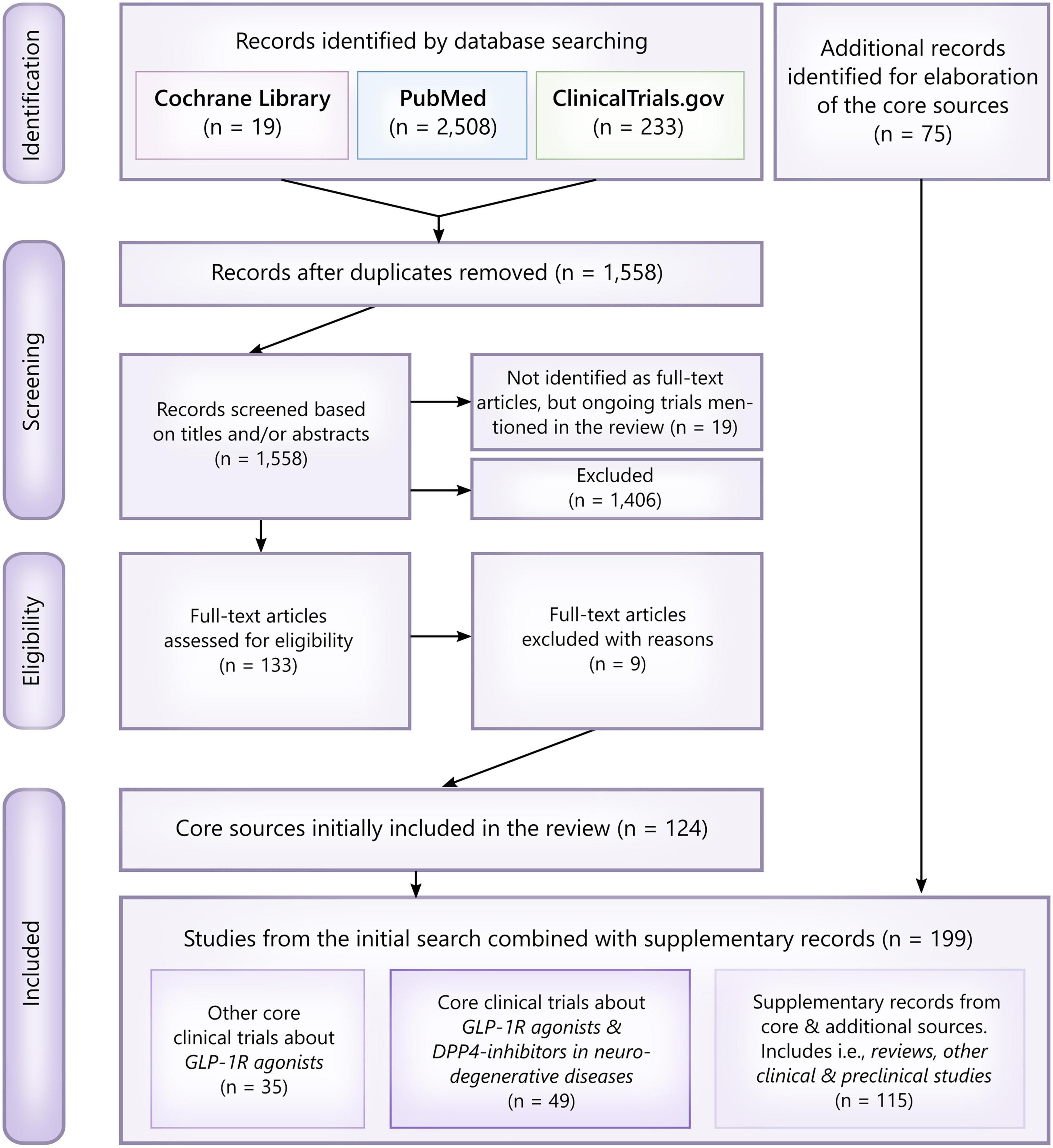
Figure 1. PRISMA flowchart and overview of the literature search. Studies were primarily excluded based on their clinical relevance and whether or not they assessed a neuroprotective effect of GLP-1R agonists/DPP4-inhibitors or not. Thus, included core studies were assessed as eligible if identified as randomized clinical trials, pilot studies, other interventional studies, epidemiological studies (cohort studies, case-control studies, etc.) and studies analyzing samples from clinical trials. For elaboration of the core sources, i.e., reviews, preclinical studies investigating the neuroprotective mechanisms behind GLP-1R agonists, relevant abstracts and post hoc analyses were included as additional records. In total, 199 records were included.
Results and Discussion
Oral Semaglutide Has Great Potential for the Prevention of Retinal Neurodegeneration
There is a demonstrated neuroprotective effect of GLP-1R agonists in both the retina and brain in preclinical studies of ocular hypertension, diabetic retinopathy, Alzheimer’s disease, Parkinson’s disease, stroke, neuropathy, and several other neurodegenerative diseases (Kastin et al., 2002; McClean et al., 2011; Hao et al., 2012; Fan et al., 2014; Secher et al., 2014; Gonçalves et al., 2016; Zhang et al., 2018, 2019; Basalay et al., 2019; Yang et al., 2019; Chang et al., 2020; Ramos et al., 2020; Ren et al., 2020; Sterling et al., 2020; Zhai et al., 2020). In the retina, GLP-1R agonists have specifically protected retinal ganglion and glia cells, such as the Müller glia, from various stresses (Hao et al., 2012; Fan et al., 2014; Gonçalves et al., 2016; Yang et al., 2019; Ramos et al., 2020; Ren et al., 2020; Sterling et al., 2020) (Figure 2).
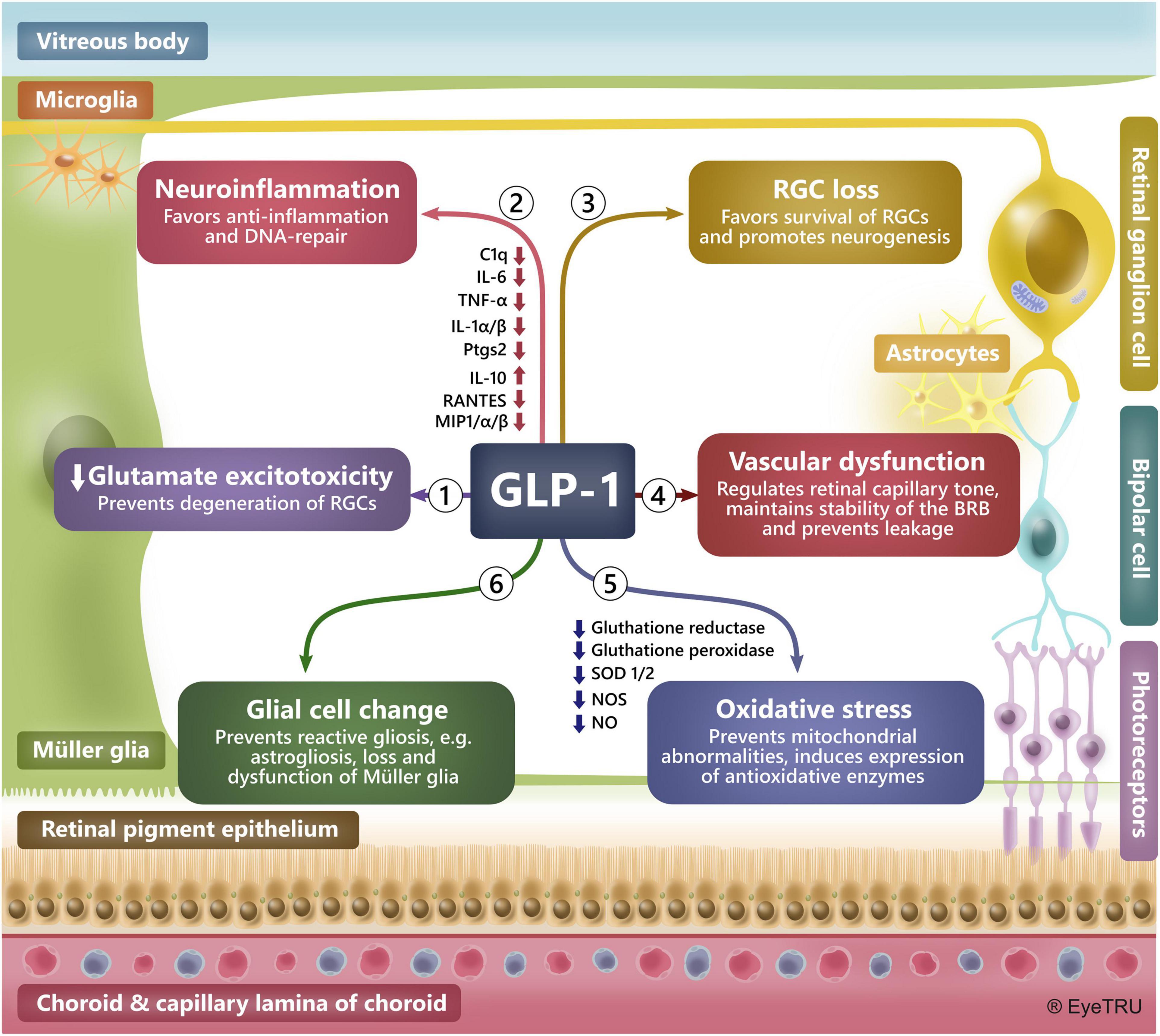
Figure 2. Mechanisms behind a neuroprotective effect of GLP-1R agonists in the retina. Preclinical studies have demonstrated a glia- and neuroprotective effect of GLP-1R agonists in the brain and retina. In the retina GLP-1R agonists have shown to protect against neurodegeneration by preventing: (1) Glutamate excitotoxicity by, e.g., upregulating glutamate transporters, (2) Neuroinflammation by, e.g., inducing anti-inflammatory cytokines, reducing levels of pro-inflammatory factors and favoring DNA-repair, (3) Loss of retinal ganglion cells (RGCs), (4) Vascular dysfunction by, e.g., maintaining the blood-retinal barrier and regulating the tone of retinal capillaries, (5) Oxidative stress by, e.g., inducing an anti-oxidative environment and maintaining mitochondrial integrity, (6) Glial cell change by, e.g., reducing ocular hypertension-induced astrocyte reactivity, dysfunction and loss of Müller glia. BRB: Blood-retina-barrier.
One of the most promising and effective GLP-1R agonists is semaglutide (Tables 1, 2) (Trujillo et al., 2021), which until September 2019 was only available as an injectable formulation. Semaglutide is also being tested in trials against diabetic eye diseases expected to end in 2027 (NCT03811561; the FOCUS trial). Now, with its ability to be administered orally, semaglutide offers great opportunities in the treatment of T2D as well as other potential disease groups. Semaglutide was designed from the backbone of another GLP-1R agonist, liraglutide, which has been tested as a prospective neuroprotective treatment option against dementia (Femminella et al., 2019; Evaluate, 2020) (NCT01843075, the ELAD study). In addition, semaglutide; like dulaglutide and exenatide XR, were developed to act as a long-acting GLP-1 analog, replacing the previous subcutaneous injections once daily with once weekly administration, potentially improving patient adherence and convenience (Lau et al., 2015).
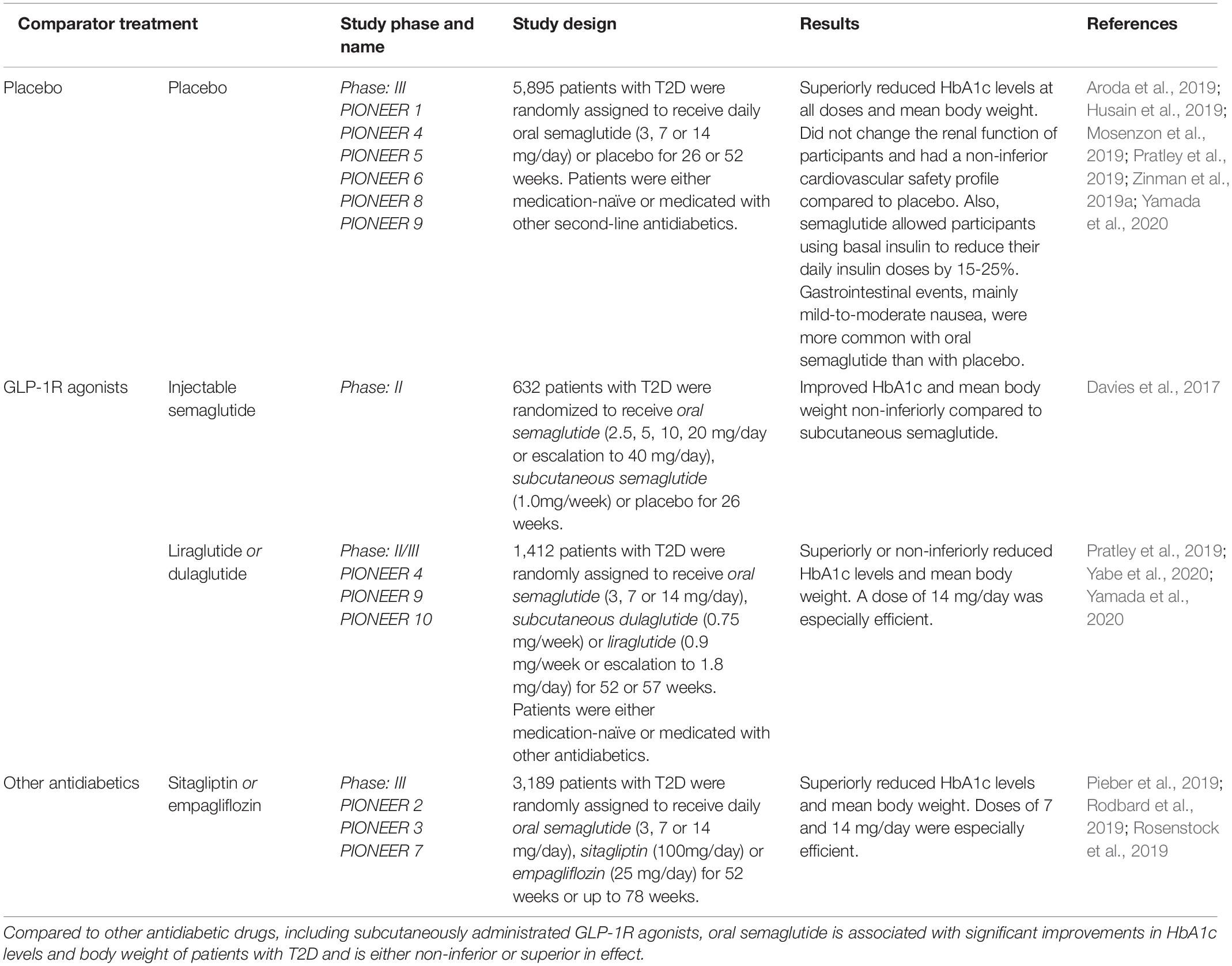
Table 2. Clinical interventional studies elucidating the efficacy and safety-profile of oral semaglutide in comparison to placebo or other antidiabetics in patients with T2D.
The safety and efficacy of semaglutide have been assessed in several phase II and III clinical trials (Marso et al., 2016a; Nauck et al., 2016; Ahrén et al., 2017; Aroda et al., 2017, 2019; Davies et al., 2017; Sorli et al., 2017; Ahmann et al., 2018; Kaku et al., 2018; Pratley et al., 2018, 2019; Rodbard et al., 2018, 2019; Seino et al., 2018; Husain et al., 2019; Lingvay et al., 2019; Mosenzon et al., 2019; Pieber et al., 2019; Rosenstock et al., 2019; Zinman et al., 2019a,b; Capehorn et al., 2020; Ji et al., 2020; Yabe et al., 2020; Yamada et al., 2020). Among these, there are 15 studies assessing the injectable semaglutide as part of the SUSTAIN trial series and 10 evaluating the newly approved oral semaglutide as part of the PIONEER trial program (Table 2). Through the SUSTAIN 1-10 (Marso et al., 2016a; Ahrén et al., 2017; Aroda et al., 2017; Sorli et al., 2017; Ahmann et al., 2018; Pratley et al., 2018; Rodbard et al., 2018; Lingvay et al., 2019; Zinman et al., 2019b; Capehorn et al., 2020) trials, the effect of injectable semaglutide, measured as reductions in glycated hemoglobin and bodyweight, was generally significantly better than treatment with DPP4-inhibitors (sitagliptin) (Ahrén et al., 2017; Seino et al., 2018; Ji et al., 2020), SGLT-2-inhibitors (canagliflozin) (Lingvay et al., 2019), long-acting insulin (insulin glargine) (Aroda et al., 2017), and other GLP-1R agonists [exenatide XR (Ahmann et al., 2018), dulaglutide (Pratley et al., 2018) and liraglutide (Capehorn et al., 2020)]. In the PIONEER 3 (Rosenstock et al., 2019) and 7 (Pieber et al., 2019) trials, oral semaglutide was also found to be superior to the DPP4-inhibitor sitagliptin. In addition, a retrospective database study estimated that patients’ persistence of injectable semaglutide treatment was significantly higher than treatment with dulaglutide, liraglutide and exenatide XR (Table 1) (Uzoigwe et al., 2021). Semaglutide has also shown promise as an effective anti-obesity treatment option. In a randomized clinical trial, a dose of 2.4 mg injectable semaglutide weekly in subjects with or without weight-related complication, semaglutide, along with lifestyle interventions, was associated with sustained as well as clinically relevant reduction in body weight (Khoo and Lin, 2021). In 2021, Novo Nordisk launched the OASIS trial expected to end in 2023 assessing the beneficial effects and safety-profile of 50 mg daily semaglutide compared to placebo (NCT05035095).
When comparing oral semaglutide with injectable GLP-1R agonists as in the PIONEER 4 trial (Pratley et al., 2019), oral semaglutide appears to be an even better option than injectable alternatives. In addition, daily oral semaglutide was found to be ranked as the best GLP-1R agonist to reduce cardiovascular death and death from any cause when indirectly compared with injectable semaglutide, liraglutide, lixisenatide, dulaglutide, albiglutide, exenatide and placebo (Alfayez et al., 2020). Compared to subcutaneously injected semaglutide, a phase II trial showed that oral semaglutide was equally effective in improving HbA1c and body weight for the T2D patients included in the trial (Davies et al., 2017).
Oral semaglutide is also likely to be more cost-effective than other existing GLP-1R agonists in the treatment of T2D patients (Hansen et al., 2020). Across the PIONEER trials, the most common adverse effects of oral semaglutide were related to the gastrointestinal tract, similar to the injectable GLP-1R agonists, and manifested as: vomiting, diarrhea and nausea (Seidu et al., 2021). In addition, oral semaglutide was not associated with severe cardiovascular outcomes or further kidney impairment in patients with renal dysfunction (Mosenzon et al., 2019). This further supports oral semaglutide as effective despite long-standing T2D and comorbidities. Thus, oral semaglutide appears to be the most effective and convenient option compared to other GLP-1R agonists, DPP4-inhibitors, and second-line antidiabetic agents. Novo Nordisk also recently started recruiting participants to the EVOKE trial program (phase III) expected to end in 2024, in which the effectiveness of oral semaglutide in the treatment of early Alzheimer’s disease will be assessed by measuring changes in clinical dementia rating, daily activity, mild cognitive impairment and mini-mental state examinations and other secondary outcome measures (NCT04777409, NCT04777396). Furthermore, as semaglutide is the only GLP-1R agonist that can be administrated as both an oral and injectable formulation, the selection of the most convenient mode of administration can be made on an individual level to best suit the patients’ preferences and needs. Given the potential neuroprotective and insulin sensitivity-regulating effects of compounds that increase GLP-1R signaling, semaglutide appears to be a promising candidate for further investigation as a novel add-on compound in the treatment of neurodegenerative diseases, such as glaucoma.
Glucagon-Like Peptide 1 Agonists and Other Antidiabetic Agents as a Potential Treatment for Glaucoma
Several antidiabetic compounds have shown potential for glaucoma treatment (Lin et al., 2015; Maleškić et al., 2017; Sterling et al., 2021) (Table 3). In support of this, several studies have suggested that diabetes mellitus is a risk factor for glaucoma (Oshitari et al., 2007; Chopra et al., 2008; Welinder et al., 2009; Zhou et al., 2014; Horwitz et al., 2016; Jung et al., 2018; Hanyuda et al., 2020). Thus, it raises the question of whether glaucoma may be associated with retinal insulin desensitization (Agostinone et al., 2018) as well as insulin desensitization of the brain as associated with Alzheimer’s and Parkinson’s disease (Faiq et al., 2014; Dada, 2017; Faiq and Dada, 2017). However, the mechanistic link and relationship between glaucoma and T2D still needs further analysis and requires further elucidation (Tielsch et al., 1995; Ellis et al., 2000; Quigley, 2009).
Metformin
Studies conducted by Lin et al. (2015) have shown that patients with T2D had a reduced risk of developing glaucoma when exposed to antihyperglycemic agents, in particular metformin, which was associated with a reduced risk of developing open-angle glaucoma. A later observational study also associated the use of metformin with a reduced risk of both glaucoma and diabetic retinopathy (Maleškić et al., 2017). The use of metformin has also been linked with a lower risk of developing other neurodegenerative eye conditions, such as AMD (Brown et al., 2019; Chen et al., 2019; Blitzer et al., 2021), and diabetic retinopathy (Maleškić et al., 2017; Li et al., 2018; Fan et al., 2020) (Table 4). The effects of metformin on AMD progression have not yet been directly investigated. However, there is currently an ongoing trial (scheduled for completion in 2023) that assesses the use of metformin to minimize geographic atrophy progression in AMD patients (NCT02684578).
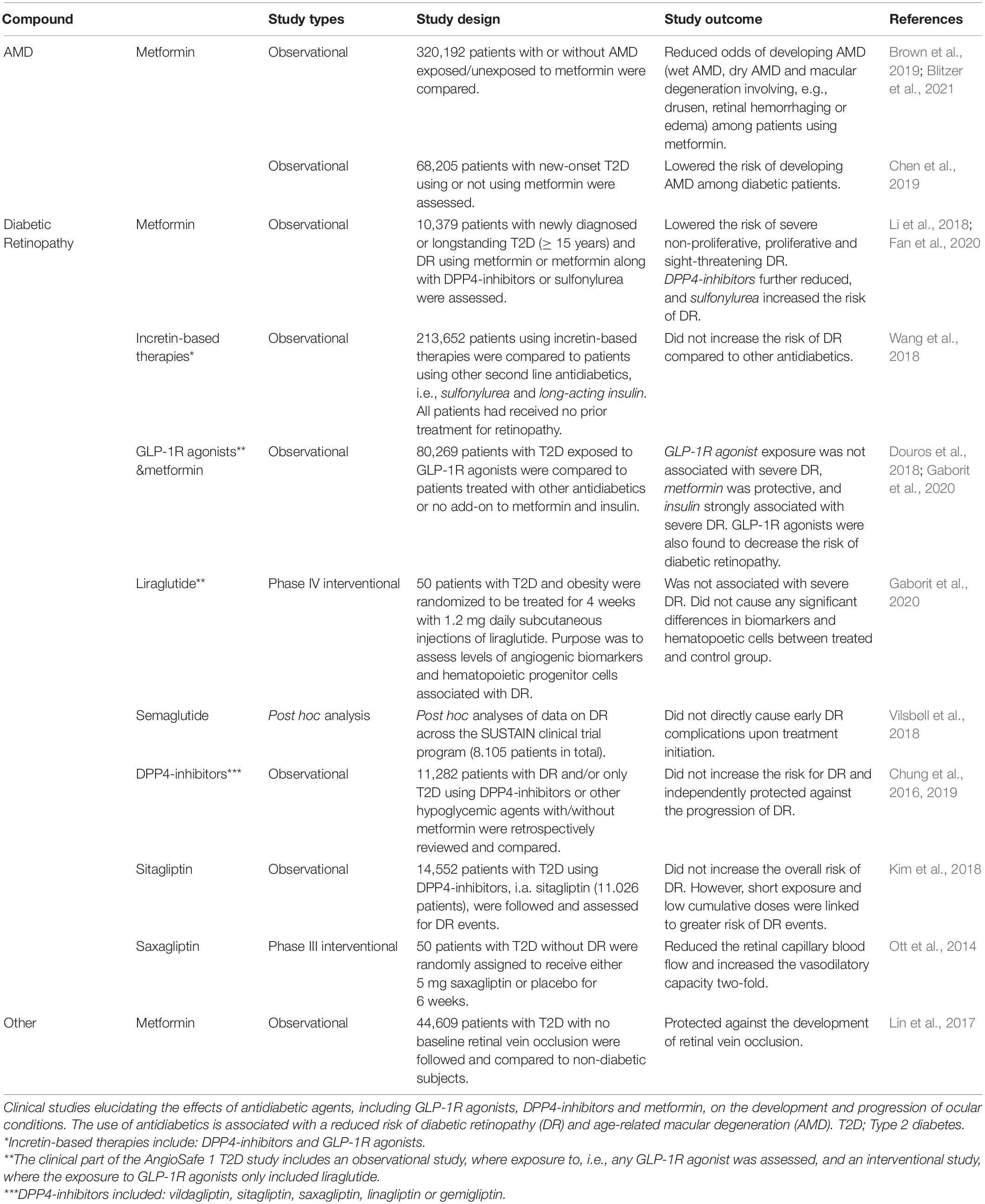
Table 4. Clinical studies elucidating the use of antidiabetics against ocular conditions other than glaucoma.
DPP4-Inhibitors
In addition, with respect to other ocular conditions and the use of antidiabetic drugs, DPP4-inhibitors have been suggested to exert a protective role in the progression of diabetic retinopathy (Ott et al., 2014; Chung et al., 2016, 2019; Kim et al., 2018). In a cohort study, DPP4-inhibitors appeared to protect against diabetic retinopathy progression, independent of their glucose-lowering effect (Chung et al., 2016). Another population-based study in South Korea comparing the use of DPP4-inhibitors with other oral glucose lowering agents (sulfonylurea, thiazolidinedione, and metformin) showed that the overall risk of diabetic retinopathy events was not increased (Kim et al., 2018). These findings are supported by a small double-blinded, placebo-controlled trial in 50 T2D patients, where 6 weeks of treatment with the DPP4-inhibitor, saxagliptin, increased vasodilation capacity and decreased retinal capillary blood flow (Ott et al., 2014). Another recent cohort study showed that the combination of metformin with DPP4-inhibitors had a strong beneficial effect against non-proliferative diabetic retinopathy (NPDR) (Fan et al., 2020). Moreover, the same study showed that the use of sulfonylurea instead of DPP4-inhibitors in combination with metformin increased the risk of NPDR (Fan et al., 2020). Thus, several clinical studies have linked the use of DPP4-inhibitors to either a reduced odds of diabetic retinopathy or a reduced risk of diabetic retinopathy progression (Ott et al., 2014; Kim et al., 2018; Wang et al., 2018; Chung et al., 2019; Fan et al., 2020).
Glucagon-Like Peptide 1 Agonists
Sterling et al. (2021) linked the exposure to GLP-1R agonists (i.e., dulaglutide, liraglutide, lixisenatide, exenatide, and semaglutide) to a low risk of developing open-angle glaucoma (Sterling et al., 2021). However, previous studies have raised concern that treatment with GLP-1R agonists may increase the risk of diabetic retinopathy, making them less favorable to be used against diabetic eye complications (Diabetes Control and Complications Trial Research Group, Nathan et al., 1993; No authors listed, 1998, 1999; Aiello and Dcct/Edic Research Group, 2014; Green et al., 2015; Marso et al., 2016b; Jingi et al., 2017; Tang et al., 2018; Bain et al., 2019; Lim et al., 2019; Bethel et al., 2021). Nevertheless, this effect of GLP-1R agonists is most likely caused by a rapid decrease in patients’ blood glucose levels as discussed later (Vilsbøll et al., 2018; Bain et al., 2019; Lim et al., 2019). The AngioSafe 1 study, which is also designed to clarify the association between exposure to GLP-1R agonists and diabetic retinopathy through clinical and preclinical study designs found no risk for severe diabetic retinopathy when patients were treated with GLP-1R agonists, and no effect of GLP-1R agonists on angiogenesis (Gaborit et al., 2020). In prospect, the effect of GLP-1R agonist exposure on severe diabetic retinopathy should be further elucidated in the ongoing AngioSafe 2 study (NCT02671864). Another phase III interventional study by Novo Nordisk, the FOCUS trial, also investigates the long-term effects of injectable semaglutide in diabetic eye diseases (NCT03811561).
Diabetic Retinopathy and Glucagon-Like Peptide 1 Agonists
Previous studies, including the DCCT, SUSTAIN-6, LEADER and TECOS trials, have linked exposure to incretin-based therapies and other hypoglycemic agents (i.e., insulin, injectable semaglutide, liraglutide, and oral sitagliptin) to the exacerbation of pre-existing retinopathy (Diabetes Control and Complications Trial Research Group, Nathan et al., 1993; No authors listed, 1998, 1999; Aiello and Dcct/Edic Research Group, 2014; Green et al., 2015; Marso et al., 2016a,b; Jingi et al., 2017; Tang et al., 2018; Bain et al., 2019; Lim et al., 2019; Bethel et al., 2021). For example, the SUSTAIN-6 trial showed that the risk of retinopathy events was increased during the first 2 months of treatment with injectable semaglutide. Nevertheless, a post hoc analysis across the SUSTAIN trials (Marso et al., 2016a; Ahrén et al., 2017; Aroda et al., 2017; Sorli et al., 2017; Ahmann et al., 2018; Kaku et al., 2018; Pratley et al., 2018; Rodbard et al., 2018; Seino et al., 2018; Lingvay et al., 2019; Zinman et al., 2019b; Capehorn et al., 2020; Ji et al., 2020) associated the increased risk of diabetic retinopathy with being limited to the early stages of treatment and mainly caused by rapid improvements in glycemic control, i.e., not a direct adverse effect of semaglutide (Vilsbøll et al., 2018). As part of the AngioSafe 1 study, both an observational and interventional phase IV study of patients with T2D showed that GLP-1R agonists did not increase the risk for severe diabetic retinopathy (Gaborit et al., 2020). This was further supported by another study comparing GLP-1R agonists to thiazolidinedione and long-acting insulin where GLP-1R agonists did not increase the risk of diabetic retinopathy over an average treatment time of less than 1 year (Wang et al., 2018). Accordingly, a cohort study found no association between the exposure to GLP-1R agonists and diabetic retinopathy, and in fact GLP-1R agonists were found to even decrease the risk of diabetic retinopathy by 33% when compared to new-users of insulin (Douros et al., 2018). Over a longer period of time, the progression of diabetic retinopathy after intensified glycemic control has also been demonstrated to be transient and reversible (Aiello and Dcct/Edic Research Group, 2014). The intensive glycemic control of diabetic patients appears to reduce both the risk of progression and the onset of diabetic retinopathy (Aiello and Dcct/Edic Research Group, 2014 with conventional antidiabetic treatments. Thus, the association between hypoglycemic agents and the exacerbation of diabetic retinopathy remains uncertain, and the early worsening is likely due to rapid lowering of blood glucose and intensified glycemic control, which, in the long run, also appears to be beneficial (Aiello and Dcct/Edic Research Group, 2014; Jingi et al., 2017; Tang et al., 2018; Vilsbøll et al., 2018; Wang et al., 2018; Bethel et al., 2021). A recent meta-regression analysis of GLP-1R agonist cardiovascular outcome trials (LEADER, SUSTAIN-6, EXSCEL, HARMONY, REWIND, and PIONEER-6 trials) has suggested that clinicians should consider the status of diabetic retinopathy in patients before initiating treatment with GLP-1R agonists (Bethel et al., 2021). In summary, the risk of diabetic retinopathy does not seem to be increased with the use of GLP-1R agonists and is being further investigated in ongoing trials (NCT02671864, NCT03811561). Thus, the patient’s retinopathy status must be determined before initiating treatment with incretin-based therapies as the GLP-1R agonists.
What Are the Possible Concerns About Glucagon-Like Peptide 1 Agonists as Potential Treatments for Glaucoma?
If GLP-1R agonists are to be used in the treatment of glaucoma patients, their retinopathy status must be determined before start of treatment. The most common type of glaucoma is primary open-angle glaucoma, with the typical patients being elderly and often underweight (Klein et al., 1992; Leske et al., 1994; Wensor et al., 1998; Jonasson et al., 2003; Iwase et al., 2004; de Voogd et al., 2005; Na et al., 2020). Accordingly, the appetite suppressant and weight loss effects of GLP-1R stimulation (Khoo and Lin, 2021) should be considered in potential trials with GLP-1R agonists as therapeutic agents for the treatment of glaucoma. Consideration should also be given as oral semaglutide has been associated with greater discontinuation of treatment in patient groups aged 65 years or older (Aroda et al., 2020). The most common reason stated for patients discontinuing oral semaglutide has been its mild to moderate gastrointestinal adverse effects, especially nausea, which is experienced by up to 50% of patients (Filippatos et al., 2014; Pratley et al., 2019; Zinman et al., 2019a; Aroda et al., 2020; Evaluate, 2020). Therefore, premature discontinuation of oral semaglutide due to adverse effects turn out to be only a few percent higher than its injectable alternatives. In the PIONEER-4 trial, 11% of the patients using oral semaglutide discontinued treatment because of adverse effects compared to 9% of the patients using subcutaneous liraglutide (Pratley et al., 2019). Another phase II interventional study found that premature cessation of treatment due to adverse effects was also higher for oral semaglutide than injectable semaglutide (Warren et al., 2018). However, this study as well as other studies (Davies et al., 2017; Warren et al., 2018; Aroda et al., 2020; Wright and Aroda, 2020) reported that both oral and injectable semaglutide were mostly discontinued due to gastrointestinal adverse effects. This together with the fact that patients showed treatment satisfaction in favor of injectable semaglutide rather than, e.g., injectable liraglutide in the SUSTAIN-10 trial (Capehorn et al., 2020), suggests that the higher proportion of patients discontinuing oral semaglutide treatment may be due to patients having to administer oral semaglutide once every day versus once a week with injectable semaglutide, making compliance for oral semaglutide more difficult and more expensive. Therefore, premature discontinuation of oral semaglutide may be due to the fact that elderly patients may be weaker, prefer treatment once a week, and are more susceptible to gastrointestinal intolerance (Newton, 2005) combined with baseline comorbidities that complicate continuation of treatment. Premature discontinuation of treatment due to adverse effects may also be simply due to the dosage of oral semaglutide and the rate of dose escalation. For instance, a dose of 7 mg oral semaglutide in the PIONEER 8 trial caused a lower percentage of patients discontinuing treatment compared to 14 mg oral semaglutide (7 mg: 9% and 14 mg: 13%, respectively) (Zinman et al., 2019a). Similar to the conventional subcutaneously administrated GLP-1R agonists, another study found that slow dose escalation of oral semaglutide resulted in a lower proportion of patients discontinuing treatment (Davies et al., 2017). Furthermore, the adverse effects of oral semaglutide tend to exacerbate as the dose is increased (Wright and Aroda, 2020). This suggests that the premature discontinuation of oral semaglutide in trials may also be due to patients’ expectations at the start of treatment and lack of counseling that effects such as nausea are frequent experiences that typically disappear over time. However, a recent Japanese health-related quality of life assessment of the PIONEER-10 trial showed that patients tended to be more satisfied when treated with oral semaglutide than with injectable dulaglutide (Ishii et al., 2021). Patients treated with oral semaglutide were also more adherent to the treatment (Ishii et al., 2021). This suggests that oral GLP-1 receptor agonists, compared to injectable GLP-1 receptor agonists, result in greater patient satisfaction and treatment persistence.
In summary, current knowledge about the potential disadvantages of GLP-1R agonist drugs is few compared to the potential beneficial neuroprotective properties. Semaglutide, in both injectable and oral formulations, appears to be a promising potential GLP-1R agonist in combination with conventional IOP-lowering agents to decrease the rate of glaucoma worsening. However, it would be wise to assess retinopathy status, patients’ BMI, age and preference for weekly subcutaneous injections or daily oral administrations as well as the dose before starting treatment.
Glucagon-Like Peptide 1 Agonists Show Neuroprotective Properties in the Treatment of Other Neurodegenerative Diseases
Glucagon-like peptide 1 (GLP-1) and GLP1R agonists have been associated not only with neuroprotection in the retina but also in the brain in various animal models of neurodegenerative diseases (Zhang et al., 2018, 2019; Basalay et al., 2019; Yang et al., 2019; Chang et al., 2020; Zhai et al., 2020). The promising preclinical results have now paved the way for evaluating the use of GLP-1R agonists in clinical studies with neurodegenerative diseases such as Alzheimer’s disease, Parkinson’s disease, stroke, and diabetic neuropathy. The neuroprotective effect of GLP-1R agonists is that it can either directly/locally cause neuroprotection by acting on neuronal tissue or indirectly/systemically cause neuroprotection by improving glycemic control and treating existing insulin resistance (Figure 3) (Fiory et al., 2019; Femminella et al., 2021).
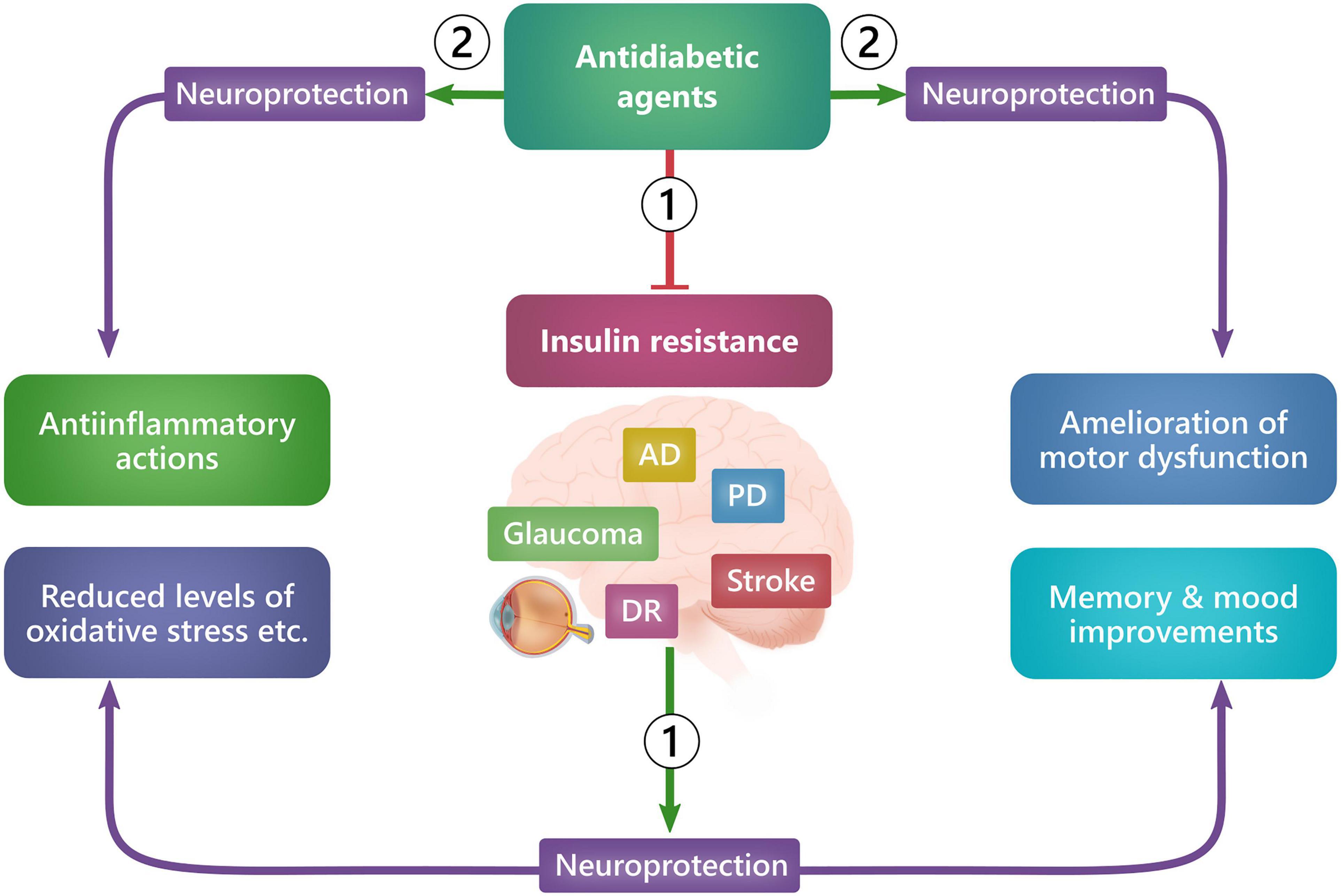
Figure 3. Ways in which GLP-1R agonists exert neuroprotection. GLP-1R agonists can exert neuroprotection either systemically (1), by improving glycemic control and reducing insulin resistance, or locally (2), by acting on receptors in neuronal tissue cells and thus causing, e.g., antiinflammation, preservation of memory, dopaminergic neurons, motor function and mood improvements. AD: Alzheimer’s disease, DR: Diabetic retinopathy, PD: Parkinson’s disease.
Alzheimer’s Disease
Several early studies have identified the presence of cognitive impairment in patients with T2D (Perlmuter et al., 1984; Helkala et al., 1995; Vanhanen et al., 1999), and T2D has been recognized as a risk factor for the development of Alzheimer’s disease (Janson et al., 2004). In addition, Alzheimer’s disease has also been directly linked to insulin resistance (Neth and Craft, 2017; Arnold et al., 2018; Hölscher, 2019; Kellar and Craft, 2020), even in the absence of concomitant diabetes (Schrijvers et al., 2010). In this case, peripheral insulin resistance was associated with decreased hippocampal glucose metabolism and lower volume of gray matter in a population of non-diabetic patients with Alzheimer’s disease (Femminella et al., 2021). Similarly, patients with cognitive impairment and Alzheimer’s disease have been shown to improve in memory, attention, mental tasks, cognition, and brain activation following insulin treatment, both intravenously and intranasally (Craft et al., 1996, 1999, 2003, 2012, 2017; Kern et al., 2001; Reger et al., 2006, 2008a,b; Claxton et al., 2015; Femminella et al., 2021; Hallschmid, 2021). Although these are promising results, evidence for a protective role for insulin remains low, and a recent Cochrane review from 2017 found no protective or preventive association between cognitive impairment and the use of antidiabetic T2D treatment strategies (Areosa Sastre et al., 2017) (i.e., metformin, insulin, repaglinide, glibenclamide, glimepiride, rosiglitazone, gliclazide, perindopril-indapamide). An aspiring novel take on insulin regulation is the administration of GLP-1R agonists, as these both regulate insulin sensitivity in the brain (Sandoval and Sisley, 2015) and possibly exert direct neuroprotective effects (Figure 3).
Besides preclinical studies pointing toward a potential use of GLP-1R agonists in the treatment of Alzheimer’s (McClean et al., 2011; Chang et al., 2020), a recent post hoc analysis led by Novo Nordisk comprising 15,820 patients with T2D, the use of the GLP-1R agonists liraglutide and semaglutide was also associated with a 53% significantly lower risk of developing dementia (Ballard et al., 2020). These findings have led to the initiation of the EVOKE trial program in 2021, which will enlighten a potential neuroprotective effect of oral semaglutide in the future. Previously, a neuroprotective effect of GLP-1R agonists has also been supported by long-term treatment with another GLP-1R agonist, dulaglutide, which reduced cognitive impairment in patients with T2D compared to placebo (NCT01394952; the REWIND trial) (Cukierman-Yaffe et al., 2020). 6-months of treatment with the GLP-1R agonist liraglutide (Victoza®) in Alzheimer’s disease patients was found to prevent declines in cerebral glucose metabolism, which is associated with synaptic dysfunction, cognitive impairment, and progression of the disease (Gejl et al., 2016) (Table 5). 12 weeks liraglutide-treatment of patients at high risk for dementia was also shown to increase connectivity in the default mode network supporting the fact that GLP-1R agonists may reduce or hinder the progression of Alzheimer’s disease (Watson et al., 2019). Supporting the hypothesis that increased GLP-1R signaling potentially promotes neuroprotection, DPP4-inhibitors are also associated with improved cognitive function (Isik et al., 2017; Xue et al., 2020). The use of GLP-1R agonists in Alzheimer’s disease also appears to be beneficial in an as yet unpublished multicenter, 12-month, phase II UK trial (NCT01843075, the ELAD-study), which evaluates liraglutide as a potential therapeutic agent in Alzheimer’s patients with mild dementia (Femminella et al., 2019). Results from the ELAD-study, presented at the Clinical Trials on Alzheimer’s Disease Conference (CTAD) 2020, showed that daily injections of liraglutide improved secondary outcomes of the study, i.e., the volume of gray matter and the cognitive function measured as improvements in Alzheimer’s Disease Assessment Scale (ADAS) scores (Evaluate, 2020). Thus, the use of GLP-1R agonists and DPP4-inhibitors has been reported to prevent cognitive impairment in several clinical studies (Gejl et al., 2016; Isik et al., 2017; Mansur et al., 2017; Watson et al., 2019; Ballard et al., 2020; Cukierman-Yaffe et al., 2020; Xue et al., 2020).
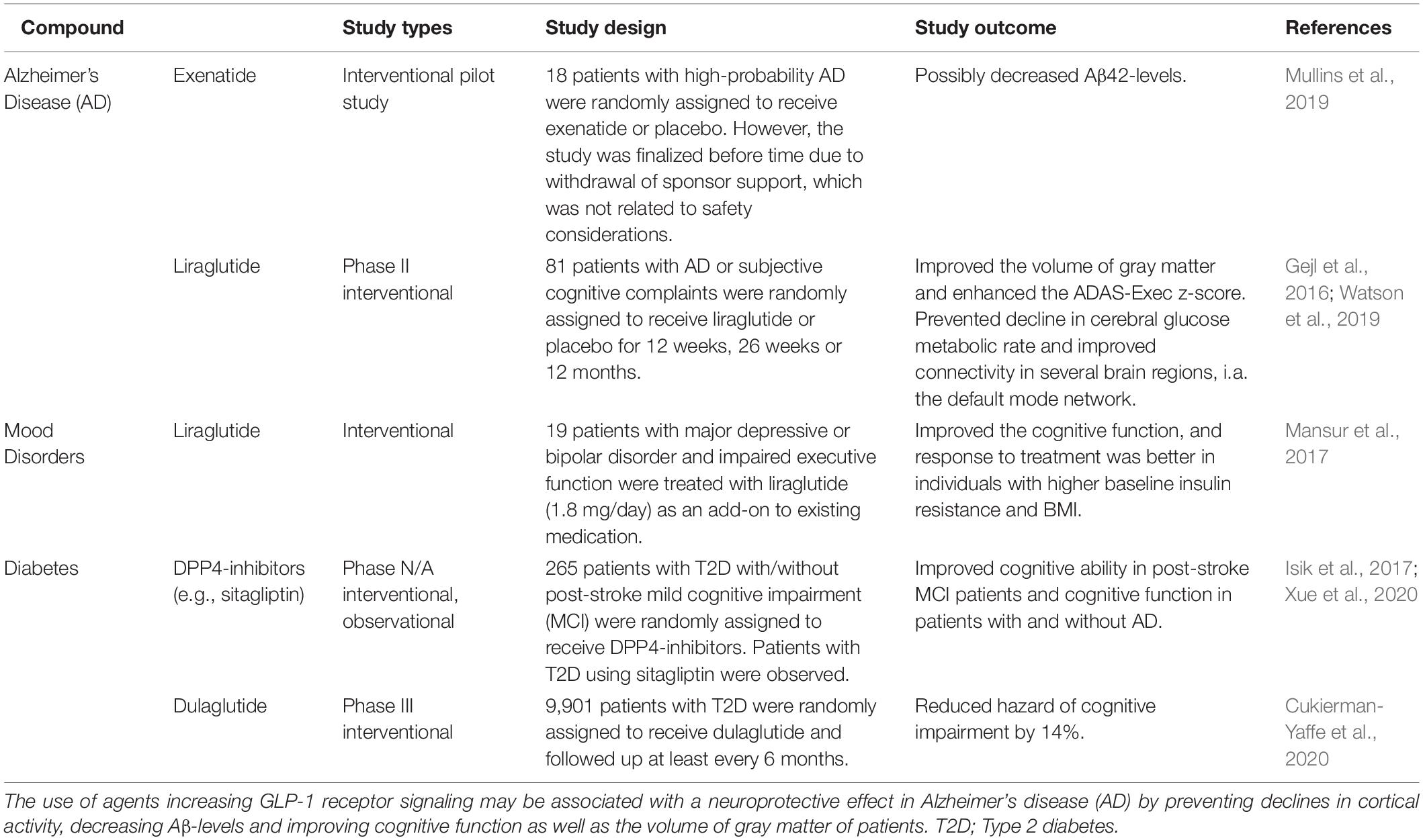
Table 5. Clinical studies elucidating the use of GLP-1R agonists in Alzheimer’s disease and cognitive dysfunction in other conditions.
In contrast, a recent pilot study could not conclude any neuroprotective property of the GLP-1R agonist exenatide, other than a reduced level of Aβ (NCT01255163) (Mullins et al., 2019). However, the lack of significant results favoring a neuroprotective effect of GLP-1R agonists may be due to early business-related withdrawal of sponsor support (Mullins et al., 2019). Thus, there is evidence in favor of a neuroprotective effect of GLP-1R agonists in Alzheimer’s disease, which will hopefully be better elucidated in ongoing clinical trials such as the EVOKE trial program.
Parkinson’s Disease
Clinical studies on GLP-1R agonists in Parkinson’s disease have focused on the GLP-1R agonist exenatide, which has yielded many promising results (Aviles-Olmos et al., 2013, 2014; Athauda et al., 2017, 2018, 2019a,b; Brauer et al., 2020) (Table 6). These notable developments have even led to the initiation of a phase III clinical trial with exenatide (Bydureon®) as an antiparkinsonian agent, launched in the beginning of 2020 (NCT04232969). The observed neuroprotective effects of GLP-1R agonists can be attributed to a possible mechanistic link between Parkinson’s disease and diabetes. Low insulin sensitivity has, similar to Alzheimer’s disease, been associated with an increased risk of developing Parkinson’s disease (Athauda and Foltynie, 2016). In addition, insulin is expected to have a decisive influence on the dopaminergic system (Fiory et al., 2019). Death of dopaminergic neurons in the substantia nigra pars compacta and changes in striatal nuclei in patients with Parkinson’s disease have been correlated with remarkable changes in structures involved with insulin signaling (Fiory et al., 2019). A possible mechanism behind the properties of exenatide in the study by Athauda et al. (2019a) was suggested to be a normalization of brain insulin signaling and an involvement of Akt signaling pathways. This was supported through electrochemiluminescence assay quantifications of proteins related to insulin signaling (i.e., phosphorylated forms of Akt) from neuronal-derived exosomes harvested from peripheral blood samples of Parkinson’s patients who had participated in the study (NCT01971242) (Athauda et al., 2019a).
A recent population-based cohort study by Brauer et al. (2020) also found evidence of a lower incidence of Parkinson’s disease in diabetic short-term and long-term users of GLP-1R agonists and DPP4-inhibitors (Brauer et al., 2020), suggesting beneficial assets of anti-diabetic drugs in the fight against Parkinson’s disease. This finding has been confirmed by other studies in which subcutaneous administration of exenatide to randomly assigned Parkinson’s patients was associated with greatly improved outcome measures that assessed the severity of motor symptom (Aviles-Olmos et al., 2013, 2014; Athauda et al., 2017, 2018). Motor function of Parkinson’s patients who have previously been exposed to exenatide has been shown to improve even 12 months after terminated treatment (Aviles-Olmos et al., 2014). Furthermore, exenatide appears to both suppress motor impairments (i.e., dyskinesia) and improve cognitive function of patients with Parkinson’s disease (Aviles-Olmos et al., 2013; Athauda et al., 2017). In addition, a post hoc analysis further revealed that exenatide improved a wide range of non-motor symptoms (i.e., mood, depression) in patients with Parkinson’s disease compared to placebo (Athauda et al., 2018). Concerning the treatment of Parkinson’s patients with GLP-1R agonists, there seems to be one compelling aspect regarding effectiveness of the treatment, and that is that the agonists should be administered as early as possible after diagnosis, as increasing age and disease duration over 10 years is associated with less effective response to exenatide treatment (Athauda et al., 2019b).
The effects of other GLP-1R agonists in the treatment of patients with Parkinson’s disease are also in line to be elucidated. A phase II study is currently investigating the neuroprotective effects of injectable semaglutide in 270 Parkinson’s patients in a double-blinded, placebo-controlled design (NCT03659682). Also, liraglutide (NCT02953665), lixisenatide (NCT03439943), exenatide (NCT04305002), sustained release exenatide – PT320 (NCT04269642) and a pegylated form of exenatide, NLY01 (NCT04154072), are all undergoing clinical investigation in Parkinson’s disease.
While GLP-1R agonists show promising results, there is a need to further investigate the neuroprotective effects of DPP4-inhibitors in Parkinson’s patients (Svenningsson et al., 2016; Brauer et al., 2020; Ece Bayram and Irene Litvan, 2020). Nevertheless, the numerous previous studies of exenatide combined with the initiation of a phase III clinical trial of exenatide, combined with ongoing trials, undeniably provide a promising basis for the prospective neuroprotective use of GLP-1R agonists in Parkinson’s disease.
Stroke
Stroke is associated with cognitive dysfunction (O’Brien et al., 2003), glaucoma (Lee et al., 2018; Rim et al., 2018), and secodary neurodegeneration (Ong et al., 2017). Several preclinical studies have observed GLP-1R agonists as neuroprotective compounds in rodent stroke models (Basalay et al., 2019; Yang et al., 2019), and GLP1-R agonists have been suggested for the use in patients with ischemic stroke (Maskery et al., 2021). In general, studies on the potential of GLP-1R agonists in stroke have focused on its effectiveness in either treating acute stroke or preventing the onset of stroke. In acute stroke, GLP-1R agonists have been suggested to treat stroke-induced hyperglycemia (post-stroke hyperglycemia) that is associated with, e.g., disruption of the blood-brain-barrier, intensified inflammatory reactions, edema, increased infarct size and worsening of functional outcomes (Li et al., 2013). Compared to insulin, GLP-1R agonists also have the advantage of preventing incidences of hypoglycemia. In a pilot study, 11 patients with ischemic stroke were treated with exenatide within app. 4-12 h after onset appeared to reduce hyperglycemia and hypoglycemic incidences (Daly et al., 2013). Another ongoing study (TEXAIS-study) also assesses the potential of exenatide in acute stroke (NCT03287076) (Muller et al., 2018). GLP-1R agonists have also shown effect in preventing stroke. Meta-analyses of large cardiovascular outcome trials have demonstrated that GLP-1R agonists, i.e., dulaglutide, liraglutide, injectable and oral semaglutide, have a potential stroke-protective effect (Pfeffer et al., 2015; Marso et al., 2016a,b; Holman et al., 2017; Hernandez et al., 2018; Kristensen et al., 2019; Alfayez et al., 2020; Gerstein et al., 2020; Strain et al., 2020). Another meta-analysis of large cardiovascular outcome trials such as SUSTAIN (injectable semaglutide), LEADER (liraglutide), HARMONY (albiglutide) (Hernandez et al., 2018), ELIXA (lixisenatide) (Pfeffer et al., 2015) and EXSCEL (exenatide) (Holman et al., 2017) appeared to reduce the risk of total stroke (by, i.e., 13% or 16%) (Bellastella et al., 2020; Gerstein et al., 2020). Semaglutide and dulaglutide were also associated with significantly reduced risks for stroke, where semaglutide appear to be better (Alfayez et al., 2020; Evans et al., 2021). However, in line with the potential neuroprotective effect of GLP-1R agonists in ischemic stroke, Rigshospitalet Denmark is running a randomized clinical trial of exenatide as an organ protecting agent, e.g., a brain-protecting agent, where stroke is one of the primary outcome measures (NCT02673931). Another Danish study is also investigating the effect of GLP-1R agonists on cerebral blood flow (NCT02829502). In fact, there are many other recruiting or completed studies investigating the protective effects of GLP-1R agonists (NCT02838589, NCT00418288, NCT00256256, NCT03948347) and also DPP4-inhibitors (NCT01107886, NCT00968708) in stroke. Thus, GLP-1R agonists show encouraging potential as stroke-protective agents, but there is still a need to investigate their effects in further clinical therapeutic trials.
Diabetic Neuropathy
Several preclinical studies have suggested that GLP-1 receptor activation is a promising mechanism that prevents neurodegeneration and improves neuroprotection in peripheral nerves (Yamamoto et al., 2002; Perry et al., 2007; Luciani et al., 2010; Griffioen et al., 2011; Himeno et al., 2011; Kan et al., 2012). A prospective, open-label pilot study of the DPP4-inhibitor teneligliptin in patients with T2D treatment has been shown to improve the patient’s peripheral and autonomic neuropathy status (Syngle et al., 2021). An unpublished clinical study has also associated a lacking responsiveness to GLP-1R agonist treatment (liraglutide and exenatide) with a higher presence of cardiovascular autonomic neuropathy (Clinical Trial Registration Number: 7459) (Duvnajak et al., 2017). However, DPP4-inhibitors, e.g., saxagliptin, have shown to have a neutral impact on the incidence of diabetic neuropathy (Taylor and Lam, 2020). A proof-of concept open-label randomized clinical trial has also assessed the effect of GLP-1R agonists on 46 patients with T2D and mild to moderate diabetic peripheral neuropathy (Jaiswal et al., 2015). Patients were randomized to be treated with either exenatide or insulin glargine for 18 months. The trial found no statistically significant effect on measures of neuropathy, i.e., no significant differences in confirmed clinical neuropathy, measures of cardiovascular autonomic neuropathy, nerve conduction tests nor intra-epidermal nerve fiber densities (Jaiswal et al., 2015). Although, the lack of significant differences in patients’ neuropathic statuses might be the result of: (1) the comparison to insulin, which also promotes glycemic control, and (2) that GLP-1 receptor agonists mainly prevent the development of neuropathy. The latter is also supported by the TODINELI-trial (Brock et al., 2019), where treatment of type 1 diabetic patients with liraglutide reduced levels of proinflammatory cytokines (IL-6) but did not improve the status of patients that had already established autonomic or diabetic polyneuropathy. Thus, clinical trials related to the neuroprotective role of GLP-1R agonists and DPP4-inhibitors in neuropathy are sparse (Mehta et al., 2021). Nevertheless, promising results from preclinical trials and the TODINELI-trial promote the idea of investigating the protective effects of GLP-1R agonists on neuropathy in further clinical trials.
Conclusion
The present review has summarized the current clinical evidence for a potential use of GLP-1R agonists in prospective neuroprotective treatment strategies against glaucoma and other neurodegenerative diseases. GLP-1R agonists have exerted a neuroprotective effect in several preclinical studies in both the brain and the retina. The most promising GLP-1R agonist in terms of efficacy, adverse effects, convenience for patients, cost-effectiveness and risk of cardiovascular complications is semaglutide, which is the only GLP-1R agonist that can be administrated as both an oral and injectable formulation. A recent association between exposure to GLP-1R agonists and a reduced risk of glaucoma makes it even more justified to consider the neuroprotective potential of GLP-1R agonists, particularly semaglutide, in anti-glaucomatous treatment strategies. Agents that increase GLP-1R signaling also appear to reduce the likelihood of developing diabetic retinopathy, AMD, cognitive dysfunction, motor dysfunction, stroke-induced neurodegenerative impairments, and neuropathy, supporting a potential neuroprotective effect of semaglutide in glaucoma. Furthermore, as oral semaglutide has recently entered phase III trials against Alzheimer’s disease, and injectable semaglutide is in the phase II trial of Parkinson’s disease as well as in the phase III trial against diabetic eye diseases, the potential of specifically semaglutide in glaucoma is additionally endorsed. However, there are currently no clinical nor any preclinical studies assessing the neuroprotective effects of semaglutide in glaucoma. Therefore, future studies are strongly needed to further investigate the potential of semaglutide as a repurposed novel neuroprotective agent in the treatment of glaucoma.
Author Contributions
All authors listed have made a substantial, direct, and intellectual contribution to the work, and approved it for publication.
Funding
This work was supported by Mauritzen La Fontaine Familiefond, the BRIDGE – Translational Excellence Programme funded by the Novo Nordisk Foundation (Grant agreement no. NNF18SA0034956). PW was supported by Karolinska Institutet in the form of a Board of Research Faculty Funded Career Position and by St. Erik Eye Hospital philanthropic donations, Vetenskapsrådet 2018-02124.
Conflict of Interest
The authors declare that the research was conducted in the absence of any commercial or financial relationships that could be construed as a potential conflict of interest.
Publisher’s Note
All claims expressed in this article are solely those of the authors and do not necessarily represent those of their affiliated organizations, or those of the publisher, the editors and the reviewers. Any product that may be evaluated in this article, or claim that may be made by its manufacturer, is not guaranteed or endorsed by the publisher.
References
Agostinone, J., Alarcon-Martinez, L., Gamlin, C., Yu, W. Q., Wong, R. O. L., and Di Polo, A. (2018). Insulin signalling promotes dendrite and synapse regeneration and restores circuit function after axonal injury. Brain 141, 1963–1980. doi: 10.1093/brain/awy142
Ahmann, A. J., Capehorn, M., Charpentier, G., Dotta, F., Henkel, E., Lingvay, I., et al. (2018). Efficacy and Safety of Once-Weekly Semaglutide Versus Exenatide ER in Subjects With Type 2 Diabetes (SUSTAIN 3): a 56-Week, Open-Label, Randomized Clinical Trial. Diabetes Care 41, 258–266. doi: 10.2337/dc17-0417
Ahrén, B., Masmiquel, L., Kumar, H., Sargin, M., Karsbøl, J. D., Jacobsen, S. H., et al. (2017). Efficacy and safety of once-weekly semaglutide versus once-daily sitagliptin as an add-on to metformin, thiazolidinediones, or both, in patients with type 2 diabetes (SUSTAIN 2): a 56-week, double-blind, phase 3a, randomised trial. Lancet Diabetes Endocrinol. 5, 341–354. doi: 10.1016/S2213-8587(17)30092-X
Aiello, L. P., and Dcct/Edic Research Group. (2014). Diabetic retinopathy and other ocular findings in the diabetes control and complications trial/epidemiology of diabetes interventions and complications study. Diabetes Care 37, 17–23. doi: 10.2337/dc13-2251
Alfayez, O. M., Almohammed, O. A., Alkhezi, O. S., Almutairi, A. R., and Al Yami, M. S. (2020). Indirect comparison of glucagon like peptide-1 receptor agonists regarding cardiovascular safety and mortality in patients with type 2 diabetes mellitus: network meta-analysis. Cardiovasc. Diabetol. 19:96. doi: 10.1186/s12933-020-01070-z
Areosa Sastre, A., Vernooij, R. W., González-Colaço Harmand, M., and Martínez, G. (2017). Effect of the treatment of Type 2 diabetes mellitus on the development of cognitive impairment and dementia. Cochrane Database Syst Rev. 6:CD003804. doi: 10.1002/14651858.CD003804.pub2
Arnold, S. E., Arvanitakis, Z., Macauley-Rambach, S. L., Koenig, A. M., Wang, H. Y., Ahima, R. S., et al. (2018). Brain insulin resistance in type 2 diabetes and Alzheimer disease: concepts and conundrums. Nat. Rev. Neurol. 14, 168–181. doi: 10.1038/nrneurol.2017.185
Aroda, R. V., Bauer, R., Hertz, C. L., Montanya, E., Sørrig, R., Warren, M. L., et al. (2020). Efficacy and safety of oral semaglutide by baseline age in the PIONEER clinical trial program. Poster Present. Am. Diabetes Assoc. 44, S24–S25. doi: 10.2337/db20-932-P
Aroda, V. R., Bain, S. C., Cariou, B., Piletič, M., Rose, L., Axelsen, M., et al. (2017). Efficacy and safety of once-weekly semaglutide versus once-daily insulin glargine as add-on to metformin (with or without sulfonylureas) in insulin-naive patients with type 2 diabetes (SUSTAIN 4): a randomised, open-label, parallel-group, multicentre, multinational, phase 3a trial. Lancet Diabetes Endocrinol. 5, 355–366. doi: 10.1016/S2213-8587(17)30085-2
Aroda, V. R., Rosenstock, J., Terauchi, Y., Altuntas, Y., Lalic, N. M., Morales Villegas, E. C., et al. (2019). PIONEER 1: randomized Clinical Trial of the Efficacy and Safety of Oral Semaglutide Monotherapy in Comparison With Placebo in Patients With Type 2 Diabetes. Diabetes Care. 42, 1724–1732. doi: 10.2337/dc19-0749
Athauda, D., and Foltynie, T. (2016). Insulin resistance and Parkinson’s disease: a new target for disease modification? Prog. Neurobiol. 14, 98–120. doi: 10.1016/j.pneurobio.2016.10.001
Athauda, D., Gulyani, S., Karnati, H. K., Li, Y., Tweedie, D., Mustapic, M., et al. (2019a). Utility of Neuronal-Derived Exosomes to Examine Molecular Mechanisms That Affect Motor Function in Patients With Parkinson Disease: a Secondary Analysis of the Exenatide-PD Trial. JAMA Neurol. 76, 420–429. doi: 10.1001/jamaneurol.2018.4304
Athauda, D., Maclagan, K., Budnik, N., Zampedri, L., Hibbert, S., Skene, S. S., et al. (2018). What Effects Might Exenatide have on Non-Motor Symptoms in Parkinson’s Disease: a Post Hoc Analysis. J. Parkinsons Dis. 8, 247–258. doi: 10.3233/JPD-181329
Athauda, D., Maclagan, K., Budnik, N., Zampedri, L., Hibbert, S., Aviles-Olmos, I., et al. (2019b). Post hoc analysis of the Exenatide-PD trial-Factors that predict response. Eur. J. Neurosci. 49, 410–421. doi: 10.1111/ejn.14096
Athauda, D., Maclagan, K., Skene, S. S., Bajwa-Joseph, M., Letchford, D., Chowdhury, K., et al. (2017). Exenatide once weekly versus placebo in Parkinson’s disease: a randomised, double-blind, placebo-controlled trial. Lancet 390, 1664–1675. doi: 10.1016/S0140-6736(17)31585-4
Aviles-Olmos, I., Dickson, J., Kefalopoulou, Z., Djamshidian, A., Ell, P., Soderlund, T., et al. (2013). Exenatide and the treatment of patients with Parkinson’s disease. J. Clin. Invest. 123, 2730–2736. doi: 10.1172/JCI68295
Aviles-Olmos, I., Dickson, J., Kefalopoulou, Z., Djamshidian, A., Kahan, J., Ell, P., et al. (2014). Motor and cognitive advantages persist 12 months after exenatide exposure in Parkinson’s disease. J. Parkinsons Dis. 4, 337–344. doi: 10.3233/JPD-140364
Bain, S. C., Klufas, M. A., Ho, A., and Matthews, D. R. (2019). Worsening of diabetic retinopathy with rapid improvement in systemic glucose control: a review. Diabetes Obes. Metab. 21, 454–466. doi: 10.1111/dom.13538
Ballard, C., Nørgaard, C. H., Friedrich, S., Mørch, L. S., Gerds, T., Møller, D. V., et al. (2020). Liraglutide and semaglutide: pooled post hoc analysis to evaluate risk of dementia in patients with type 2 diabetes. Alzheimers Dement. 16:S9. doi: 10.1002/alz.042909
Basalay, M. V., Davidson, S. M., and Yellon, D. M. (2019). Neuroprotection in Rats Following Ischaemia-Reperfusion Injury by GLP-1 Analogues-Liraglutide and Semaglutide. Cardiovasc. Drugs Ther. 33, 661–667. doi: 10.1007/s10557-019-06915-8
Bellastella, G., Maiorino, M. I., Longo, M., Scappaticcio, L., Chiodini, P., Esposito, K., et al. (2020). Glucagon-Like Peptide-1 Receptor Agonists and Prevention of Stroke Systematic Review of Cardiovascular Outcome Trials With Meta-Analysis. Stroke 51, 666–669. doi: 10.1161/STROKEAHA.119.027557
Bethel, M. A., Diaz, R., Castellana, N., Bhattacharya, I., Gerstein, H. C., and Lakshmanan, M. C. (2021). HbA1c Change and Diabetic Retinopathy During GLP-1 Receptor Agonist Cardiovascular Outcome Trials: a Meta-analysis and Meta-regression. Diabetes Care 44, 290–296. doi: 10.2337/dc20-1815
Blitzer, A. L., Ham, S. A., Colby, K. A., and Skondra, D. (2021). Association of Metformin Use With Age-Related Macular Degeneration: a Case-Control Study. JAMA Ophthalmol. 139, 302–309. doi: 10.1001/jamaophthalmol.2020.6331
Brauer, R., Wei, L., Ma, T., Athauda, D., Girges, C., Vijiaratnam, N., et al. (2020). Diabetes medications and risk of Parkinson’s disease: a cohort study of patients with diabetes. Brain 143, 3067–3076. doi: 10.1093/brain/awaa262
Brown, E. E., Ball, J. D., Chen, Z., Khurshid, G. S., Prosperi, M., and Ash, J. D. (2019). The Common Antidiabetic Drug Metformin Reduces Odds of Developing Age-Related Macular Degeneration. Invest. Ophthalmol. Vis. Sci. 60, 1470–1477. doi: 10.1167/iovs.18-26422
Brock, C., Hansen, C. S., Karmisholt, J., Møller, H. J., Juhl, A., Farmer, A. D., et al. (2019). Liraglutide treatment reduced interleukin-6 in adults with type 1 diabetes but did not improve established autonomic or polyneuropathy. Br. J. Clin. Pharmacol. 85, 2512–2523. doi: 10.1111/bcp.14063
Capehorn, M. S., Catarig, A. M., Furberg, J. K., Janez, A., Price, H. C., Tadayon, S., et al. (2020). Efficacy and safety of once-weekly semaglutide 1.0mg vs once-daily liraglutide 1.2mg as add-on to 1-3 oral antidiabetic drugs in subjects with type 2 diabetes (SUSTAIN 10). Diabetes Metab. 46, 100–109. doi: 10.1016/j.diabet.2019.101117
Cedrone, C., Mancino, R., Cerulli, A., Cesareo, M., and Nucci, C. (2008). Epidemiology of primary glaucoma: prevalence, incidence, and blinding effects. Prog. Brain Res. 173, 3–14. doi: 10.1016/S0079-6123(08)01101-1
Chang, Y. F., Zhang, D., Hu, W. M., Liu, D. X., and Li, L. (2020). Semaglutide-mediated protection against Aβ correlated with enhancement of autophagy and inhibition of apoptosis. J. Clin. Neurosci. 81, 234–239. doi: 10.1016/j.jocn.2020.09.054
Chen, Y. Y., Shen, Y. C., Lai, Y. J., Wang, C. Y., Lin, K. H., Feng, S. C., et al. (2019). Association between Metformin and a Lower Risk of Age-Related Macular Degeneration in Patients with Type 2 Diabetes. J. Ophthalmol. 2019:1649156. doi: 10.1155/2019/1649156
Chopra, V., Varma, R., Francis, B. A., Wu, J., Torres, M., Azen, S. P., et al. (2008). Type 2 diabetes mellitus and the risk of open-angle glaucoma the Los Angeles Latino Eye Study. Ophthalmology 115, 227–232.e1. doi: 10.1016/j.ophtha.2007.04.049
Chung, Y. R., Ha, K. H., Kim, H. C., Park, S. J., Lee, K., and Kim, D. J. (2019). Dipeptidyl Peptidase-4 Inhibitors versus Other Antidiabetic Drugs Added to Metformin Monotherapy in Diabetic Retinopathy Progression: a Real World-Based Cohort Study. Diabetes Metab. J. 43, 640–648. doi: 10.4093/dmj.2018.0137
Chung, Y. R., Park, S. W., Kim, J. W., Kim, J. H., and Lee, K. (2016). Protective effects of dipeptidyl peptidase-4 inhibitors on progression of diabetic retinopathy in patients with type 2 diabetes. Retina 36, 2357–2363. doi: 10.1097/IAE.0000000000001098
Claxton, A., Baker, L. D., Hanson, A., Trittschuh, E. H., Cholerton, B., Morgan, A., et al. (2015). Long Acting Intranasal Insulin Detemir Improves Cognition for Adults with Mild Cognitive Impairment or Early-Stage Alzheimer’s Disease Dementia. J. Alzheimers. Dis. 45, 1269–1270. doi: 10.3233/JAD-159002
Courrèges, J. P., Vilsbøll, T., Zdravkovic, M., Le-Thi, T., Krarup, T., Schmitz, O., et al. (2008). Beneficial effects of once-daily liraglutide, a human glucagon-like peptide-1 analogue, on cardiovascular risk biomarkers in patients with Type 2 diabetes. Diabetes Med. 25, 1129–1131. doi: 10.1111/j.1464-5491.2008.02484.x
Craft, S., Asthana, S., Cook, D. G., Baker, L. D., Cherrier, M., Purganan, K., et al. (2003). Insulin dose-response effects on memory and plasma amyloid precursor protein in Alzheimer’s disease: interactions with apolipoprotein E genotype. Psychoneuroendocrinology 28, 809–822. doi: 10.1016/s0306-4530(02)00087-2
Craft, S., Asthana, S., Newcomer, J. W., Wilkinson, C. W., Matos, I. T., Baker, L. D., et al. (1999). Enhancement of memory in Alzheimer disease with insulin and somatostatin, but not glucose. Arch. Gen. Psychiatry. 56, 1135–1140. doi: 10.1001/archpsyc.56.12.1135
Craft, S., Baker, L. D., Montine, T. J., Minoshima, S., Watson, G. S., Claxton, A., et al. (2012). Intranasal insulin therapy for Alzheimer disease and amnestic mild cognitive impairment: a pilot clinical trial. Arch. Neurol. 69, 29–38. doi: 10.1001/archneurol.2011.233
Craft, S., Claxton, A., Baker, L. D., Hanson, A. J., Cholerton, B., Trittschuh, E. H., et al. (2017). Effects of regular and long-acting insulin on cognition and Alzheimer’s disease biomarkers: a pilot clinical trial. J. Alzheimers. Dis. 57, 1325–1334. doi: 10.3233/JAD-161256
Craft, S., Newcomer, J., Kanne, S., Dagogo-Jack, S., Cryer, P., Sheline, Y., et al. (1996). Memory improvement following induced hyperinsulinemia in Alzheimer’s disease. Neurobiol. Aging 7, 123–130. doi: 10.1016/0197-4580(95)02002-0
Cukierman-Yaffe, T., Gerstein, H. C., Colhoun, H. M., Diaz, R., García-Pérez, L. E., Lakshmanan, M., et al. (2020). Effect of dulaglutide on cognitive impairment in type 2 diabetes: an exploratory analysis of the REWIND trial. Lancet Neurol. 19, 582–590. doi: 10.1016/S1474-4422(20)30173-3
Dada, T. (2017). Is Glaucoma a Neurodegeneration caused by Central Insulin Resistance: diabetes Type 4? J. Curr. Glaucoma Pract. 11, 77–79. doi: 10.5005/jp-journals-10028-1228
Daly, S. C., Chemmanam, T., Loh, P. S., Gilligan, A., Dear, A. E., Simpson, R. W., et al. (2013). Exenatide in acute ischemic stroke. Int. J. Stroke 8, E44. doi: 10.1111/ijs.12073
Davies, M., Pieber, T. R., Hartoft-Nielsen, M. L., Hansen, O., Jabbour, S., and Rosenstock, J. (2017). Effect of Oral Semaglutide Compared With Placebo and Subcutaneous Semaglutide on Glycemic Control in Patients With Type 2 Diabetes: a Randomized Clinical Trial. JAMA 318, 1460–1470. doi: 10.1001/jama.2017.14752
de Voogd, S., Ikram, M. K., Wolfs, R. C., Jansonius, N. M., Hofman, A., and de Jong, P. T. (2005). Incidence of open-angle glaucoma in a general elderly population: the Rotterdam Study. Ophthalmology 112, 1487–1493. doi: 10.1016/j.ophtha.2005.04.018
Diabetes Control and Complications Trial Research Group, Nathan, D. M., Genuth, S., Lachin, J., Cleary, P., Crofford, O., et al. (1993). The effect of intensive treatment of diabetes on the development and progression of long-term complications in insulin-dependent diabetes mellitus. N. Engl. J. Med. 329, 977–986. doi: 10.1056/NEJM199309303291401
Dielemans, I., Vingerling, J. R., Wolfs, R. C., Hofman, A., Grobbee, D. E., and de Jong, P. T. (1994). The prevalence of primary open-angle glaucoma in a population-based study in The Netherlands. The Rotterdam Study. Ophthalmology 101, 1851–1855. doi: 10.1016/s0161-6420(94)31090-6
Douros, A., Filion, K. B., Yin, H., Yu, O. H., Etminan, M., Udell, J. A., et al. (2018). Glucagon-Like Peptide 1 Receptor Agonists and the Risk of Incident Diabetic Retinopathy. Diabetes Care 41, 2330–2338. doi: 10.2337/dc17-2280
Drucker, D. J. (2018). Mechanisms of Action and Therapeutic Application of Glucagon-like Peptide-1. Cell. Metab. 27, 740–756. doi: 10.1016/j.cmet.2018.03.001
Duvnajak, I., Bulum, T., and Rebrina, S. V. (2017). Autonomic Neuropathy is Associated with the Lack of Glucagon-like Peptide-1 Receptor Agonists Treatment Efficacy in Patients with Type 2 Diabetes. Available online at: https://www.easd.org/virtualmeeting/home.html#!resources/autonomic-neuropathy-is-associated-with-the-lack-of-glucagon-like-peptide-1-receptor-agonists-treatment-efficacy-in-patients-with-type-2-diabetes (accessed August 10, 2021).
Ece Bayram, and Irene Litvan. (2020). Lowering the risk of Parkinson’s disease with GLP-1 agonists and DPP4 inhibitors in type 2 diabetes. Brain 143, 2868–2871. doi: 10.1093/brain/awaa287
Ellis, J. D., Evans, J. M., Ruta, D. A., Baines, P. S., Leese, G., MacDonald, T. M., et al. (2000). Glaucoma incidence in an unselected cohort of diabetic patients: is diabetes mellitus a risk factor for glaucoma? DARTS/MEMO collaboration. Diabetes Audit and Research in Tayside Study. Medicines Monitoring Unit. Br. J. Ophthalmol. 84, 1218–1224. doi: 10.1136/bjo.84.11.1218
Evaluate (2020). CTAD 2020 – ELAD fails, but GLP-1s could still have a future in Alzheimer’s. Available online at: https://www.evaluate.com/vantage/articles/news/snippets/ctad-2020-elad-fails-glp-1s-could-still-have-future-alzheimers (accessed March 31, 2021).
Evans, L. M., Mellbin, L., Johansen, P., Lawson, J., Paine, A., and Sandberg, A. (2021). A population-adjusted indirect comparison of cardiovascular benefits of once-weekly subcutaneous semaglutide and dulaglutide in the treatment of patients with type 2 diabetes, with or without established cardiovascular disease. Endocrinol. Diabetes Metab. 4:e00259. doi: 10.1002/edm2.259
Faiq, M. A., and Dada, T. (2017). Diabetes Type 4: a Paradigm Shift in the Understanding of Glaucoma, the Brain Specific Diabetes and the Candidature of Insulin as a Therapeutic Agent. Curr. Mol. Med. 17, 46–59. doi: 10.2174/1566524017666170206153415
Faiq, M. A., Dada, R., Saluja, D., and Dada, T. (2014). Glaucoma–diabetes of the brain: a radical hypothesis about its nature and pathogenesis. Med. Hypotheses 82, 535–546. doi: 10.1016/j.mehy.2014.02.005
Fan, Y. P., Wu, C. T., Lin, J. L., Hsiung, C. A., Liu, H. Y., Lai, J. N., et al. (2020). Metformin Treatment Is Associated with a Decreased Risk of Nonproliferative Diabetic Retinopathy in Patients with Type 2 Diabetes Mellitus: a Population-Based Cohort Study. J. Diabetes Res. 2020:9161039. doi: 10.1155/2020/9161039
Fan, Y., Liu, K., Wang, Q., Ruan, Y., Zhang, Y., and Ye, W. (2014). Exendin-4 protects retinal cells from early diabetes in Goto-Kakizaki rats by increasing the Bcl-2/Bax and Bcl-xL/Bax ratios and reducing reactive gliosis. Mol. Vis. 2014, 1557–1568.
Femminella, G. D., Frangou, E., Love, S. B., Busza, G., Holmes, C., Ritchie, C., et al. (2019). Evaluating the effects of the novel GLP-1 analogue liraglutide in Alzheimer’s disease: study protocol for a randomised controlled trial (ELAD study). Trials 20:191. doi: 10.1186/s13063-019-3259-x
Femminella, G. D., Livingston, N. R., Raza, S., van der Doef, T., Frangou, E., Love, S., et al. (2021). Does insulin resistance influence neurodegeneration in non-diabetic Alzheimer’s subjects? Alzheimers. Res. Ther. 13:47. doi: 10.1186/s13195-021-00784-w
Filippatos, T. D., Panagiotopoulou, T. V., and Elisaf, M. S. (2014). Adverse Effects of GLP-1 Receptor Agonists. Rev. Diabet. Stud. 11, 202–230. doi: 10.1900/RDS.2014.11.202
Fiory, F., Perruolo, G., Cimmino, I., Cabaro, S., Pignalosa, F. C., Miele, C., et al. (2019). The Relevance of Insulin Action in the Dopaminergic System. Front. Neurosci. 2019:868. doi: 10.3389/fnins.2019.00868
Gaborit, B., Julla, J. B., Besbes, S., Proust, M., Vincentelli, C., Alos, B., et al. (2020). Glucagon-like Peptide 1 Receptor Agonists, Diabetic Retinopathy and Angiogenesis: The AngioSafe Type 2 Diabetes Study. J. Clin. Endocrinol. Metab. 105:dgz069. doi: 10.1210/clinem/dgz069
Gejl, M., Gjedde, A., Egefjord, L., Møller, A., Hansen, S. B., Vang, K., et al. (2016). In Alzheimer’s Disease, 6-Month Treatment with GLP-1 Analog Prevents Decline of Brain Glucose Metabolism: randomized, Placebo-Controlled, Double-Blind Clinical Trial. Front. Aging Neurosci. 8:108. doi: 10.3389/fnagi.2016.00108
Gerstein, H. C., Hart, R., Colhoun, H. M., Diaz, R., Lakshmanan, M., Botros, F. T., et al. (2020). The effect of dulaglutide on stroke: an exploratory analysis of the REWIND trial. Lancet Diabetes Endocrinol. 8, 106–114. doi: 10.1016/S2213-8587(19)30423-1
Gonçalves, A., Lin, C. M., Muthusamy, A., Fontes-Ribeiro, C., Ambrósio, A. F., Abcouwer, S. F., et al. (2016). Protective Effect of a GLP-1 Analog on Ischemia-Reperfusion Induced Blood-Retinal Barrier Breakdown and Inflammation. Invest. Ophthalmol. Vis. Sci. 57, 2584–92. doi: 10.1167/iovs.15-19006
Green, J. B., Bethel, M. A., Armstrong, P. W., Buse, J. B., Engel, S. S., Garg, J., et al. (2015). Effect of Sitagliptin on Cardiovascular Outcomes in Type 2 Diabetes. N. Engl. J. Med. 373, 232–242. doi: 10.1056/NEJMoa1501352
Griffioen, K. J., Wan, R., Okun, E., Wang, X., Lovett-Barr, M. R., Li, Y., et al. (2011). GLP-1 receptor stimulation depresses heart rate variability and inhibits neurotransmission to cardiac vagal neurons. Cardiovasc. Res. 89, 72–78. doi: 10.1093/cvr/cvq271
Gutniak, M., Orskov, C., Holst, J. J., Ahrén, B., and Efendic, S. (1992). Antidiabetogenic effect of glucagon-like peptide-1 (7-36)amide in normal subjects and patients with diabetes mellitus. N. Engl. J. Med. 326, 1316–1322. doi: 10.1056/NEJM199205143262003
Hallschmid, M. (2021). Intranasal Insulin for Alzheimer’s Disease. CNS Drugs 35, 21–37. doi: 10.1007/s40263-020-00781-x
Hansen, B. B., Nuhoho, S., Ali, S. N., Dang-Tan, T., Valentine, W. J., Malkin, S. J. P., et al. (2020). Oral semaglutide versus injectable glucagon-like peptide-1 receptor agonists: a cost of control analysis. J. Med. Econ. 23, 650–658. doi: 10.1080/13696998.2020.1722678
Hanyuda, A., Sawada, N., Yuki, K., Uchino, M., Ozawa, Y., Sasaki, M., et al. (2020). Relationships of diabetes and hyperglycaemia with intraocular pressure in a Japanese population: the JPHC-NEXT Eye Study. Sci. Rep. 10:5355. doi: 10.1038/s41598-020-62135-3
Hao, M., Kuang, H. Y., Fu, Z., Gao, X. Y., Liu, Y., and Deng, W. (2012). Exenatide prevents high-glucose-induced damage of retinal ganglion cells through a mitochondrial mechanism. Neurochem. Int. 61, 1–6. doi: 10.1016/j.neuint.2012.04.009
Heijl, A., Leske, M. C., Bengtsson, B., Hyman, L., Bengtsson, B., and Hussein, M. (2002). Reduction of intraocular pressure and glaucoma progression: results from the Early Manifest Glaucoma Trial. Arch. Ophthalmol. 120, 1268–1279. doi: 10.1001/archopht.120.10.1268
Helkala, E. L., Niskanen, L., Viinamaki, H., Partanen, J., and Uusitupa, M. (1995). Short-term and long-term memory in elderly patients with NIDDM. Diabet. Care 18, 681–685. doi: 10.2337/diacare.18.5.681
Hernandez, A. F., Green, J. B., Janmohamed, S., D’Agostino, R. B. Sr., Granger, C. B., Jones, N. P., et al. (2018). Albiglutide and cardiovascular outcomes in patients with type 2 diabetes and cardiovascular disease (Harmony Outcomes): a double-blind, randomised placebo-controlled trial. Lancet 392, 1519–1529. doi: 10.1016/S0140-6736(18)32261-X
Himeno, T., Kamiya, H., Naruse, K., Harada, N., Ozaki, N., Seino, Y., et al. (2011). Beneficial effects of exendin-4 on experimental polyneuropathy in diabetic mice. Diabetes 60, 2397–2406. doi: 10.2337/db10-1462
Ho, C. H., and Wong, J. K. W. (2019). Role of 24-Hour Intraocular Pressure Monitoring in Glaucoma Management. J. Ophthalmol. 2019:3632197. doi: 10.1155/2019/3632197
Holman, R. R., Bethel, M. A., Mentz, R. J., Thompson, V. P., Lokhnygina, Y., Buse, J. B., et al. (2017). Effects of Once-Weekly Exenatide on Cardiovascular Outcomes in Type 2 Diabetes. N. Engl. J. Med. 377, 1228–1239. doi: 10.1056/NEJMoa1612917
Hölscher, C. (2019). Insulin Signaling Impairment in the Brain as a Risk Factor in Alzheimer’s Disease. Front. Aging Neurosci. 11:88. doi: 10.3389/fnagi.2019.00088
Horwitz, A., Petrovski, B. É, Torp-Pedersen, C., and Kolko, M. (2016). Danish Nationwide Data Reveal a Link between Diabetes Mellitus. Diabetic Retinopathy, and Glaucoma. J. Diabetes Res. 2016:2684674. doi: 10.1155/2016/2684674
Husain, M., Birkenfeld, A. L., Donsmark, M., Dungan, K., Eliaschewitz, F. G., Franco, D. R., et al. (2019). Oral Semaglutide and Cardiovascular Outcomes in Patients with Type 2 Diabetes. N. Engl. J. Med. 381, 841–851. doi: 10.1056/NEJMoa1901118
Ishii, H., Hansen, B. B., Langer, J., and Horio, H. (2021). Effect of Orally Administered Semaglutide Versus Dulaglutide on Diabetes-Related Quality of Life in Japanese Patients with Type 2 Diabetes: the PIONEER 10 Randomized. Active-Controlled Trial. Diabetes Ther. 12, 613–623. doi: 10.1007/s13300-020-00985-w
Isik, A. T., Soysal, P., Yay, A., and Usarel, C. (2017). The effects of sitagliptin, a DPP-4 inhibitor, on cognitive functions in elderly diabetic patients with or without Alzheimer’s disease. Diabetes Res. Clin. Pract. 123, 192–198. doi: 10.1016/j.diabres.2016.12.010
Iwase, A., Suzuki, Y., Araie, M., Yamamoto, T., Abe, H., Shirato, S., et al. (2004). The prevalence of primary open-angle glaucoma in Japanese: the Tajimi Study. Ophthalmology 111, 1641–1648. doi: 10.1016/j.ophtha.2004.03.029
Jaiswal, M., Martin, C. L., Brown, M. B., Callaghan, B., Albers, J. W., Feldman, E. L., et al. (2015). Effects of exenatide on measures of diabetic neuropathy in subjects with type 2 diabetes: results from an 18-month proof-of-concept open-label randomized study. J. Diabetes Compl. 29, 1287–1294. doi: 10.1016/j.jdiacomp.2015.07.013
Janson, J., Laedtke, T., Parisi, J. E., O’Brien, P., Petersen, R. C., and Butler, P. C. (2004). Increased risk of type 2 diabetes in Alzheimer disease. Diabetes 53, 474–481.
Ji, L., Dong, X., Li, Y., Li, Y., Lim, S., Liu, M., et al. (2020). Efficacy and safety of once-weekly semaglutide versus once-daily sitagliptin as add-on to metformin in patients with type 2 diabetes in SUSTAIN China: a 30-week, double-blind, phase 3a, randomized trial. Diabetes Obes. Metab. 23, 404–414. doi: 10.1111/dom.14232
Jingi, A. M., Tankeu, A. T., Ateba, N. A., and Noubiap, J. J. (2017). Mechanism of worsening diabetic retinopathy with rapid lowering of blood glucose: the synergistic hypothesis. BMC Endocr. Disord. 17:63. doi: 10.1186/s12902-017-0213-3
Jinnouchi, H., Sugiyama, S., Yoshida, A., Hieshima, K., Kurinami, N., Suzuki, T., et al. (2015). Liraglutide, a glucagon-like peptide-1 analog, increased insulin sensitivity assessed by hyperinsulinemic-euglycemic clamp examination in patients with uncontrolled type 2 diabetes mellitus. J. Diabetes Res. 2015:706416. doi: 10.1155/2015/706416
Jonasson, F., Damji, K. F., Arnarsson, A., Sverrisson, T., Wang, L., Sasaki, H., et al. (2003). Prevalence of open-angle glaucoma in Iceland: reykjavik Eye Study. Eye 17, 747–753. doi: 10.1038/sj.eye.6700374
Jung, Y., Han, K., Park, H. L., and Park, C. K. (2018). Type 2 diabetes mellitus and risk of open-angle glaucoma development in Koreans: an 11-year nationwide propensity-score-matched study. Diabetes Metab. 44, 328–332. doi: 10.1016/j.diabet.2017.09.007
Kaku, K., Yamada, Y., Watada, H., Abiko, A., Nishida, T., Zacho, J., et al. (2018). Safety and efficacy of once-weekly semaglutide vs additional oral antidiabetic drugs in Japanese people with inadequately controlled type 2 diabetes: a randomized trial. Diabetes Obes. Metab. 20, 1202–1212. doi: 10.1111/dom.13218
Kalouda, P., Keskini, C., Anastasopoulos, E., and Topouzis, F. (2017). Achievements and Limits of Current Medical Therapy of Glaucoma. Dev. Ophthalmol. 59, 1–14. doi: 10.1159/000458482
Kan, M., Guo, G., Singh, B., Singh, V., and Zochodne, D. W. (2012). Glucagon-like peptide 1, insulin, sensory neurons, and diabetic neuropathy. J. Neuropathol. Exp. Neurol. 71, 494–510. doi: 10.1097/NEN.0b013e3182580673
Kastin, A. J., Akerstrom, V., and Pan, W. (2002). Interactions of glucagon-like peptide-1 (GLP-1) with the blood-brain barrier. J. Mol. Neurosci. 18, 7–14. doi: 10.1385/JMN:18:1-2:07
Kellar, D., and Craft, S. (2020). Brain insulin resistance in Alzheimer’s disease and related disorders: mechanisms and therapeutic approaches. Lancet Neurol. 19, 758–766. doi: 10.1016/S1474-4422(20)30231-3
Kern, W., Peters, A., Fruehwald-Schultes, B., Deininger, E., Born, J., and Fehm, H. L. (2001). Improving influence of insulin on cognitive functions in humans. Neuroendocrinology 74, 270–280. doi: 10.1159/000054694
Khoo, T. K., and Lin, J. (2021). Once-Weekly Semaglutide in Adults with Overweight or Obesity. N. Engl. J. Med. 385:e4. doi: 10.1056/NEJMc2106918
Kim, N. H., Choi, J., Kim, N. H., Choi, K. M., Baik, S. H., Lee, J., et al. (2018). Dipeptidyl peptidase-4 inhibitor use and risk of diabetic retinopathy: a population-based study. Diabetes Metab. 44, 361–367. doi: 10.1016/j.diabet.2018.03.004
Klein, B. E., Klein, R., Sponsel, W. E., Franke, T., Cantor, L. B., Martone, J., et al. (1992). Prevalence of glaucoma. The Beaver Dam Eye Study. Ophthalmology 99, 1499–1504. doi: 10.1016/s0161-6420(92)31774-9
Kolko, M. (2015). Present and New Treatment Strategies in the Management of Glaucoma. Open Ophthalmol. J. 9, 89–100. doi: 10.2174/1874364101509010089
Kolko, M. (2017). [Detection and prevention of blindness in patients with glaucoma is a socio-economical challenge]. Ugeskr Laeg. 179:V06160444.
Kolko, M., Horwitz, A., Thygesen, J., Jeppesen, J., and Torp-Pedersen, C. (2015). The Prevalence and Incidence of Glaucoma in Denmark in a Fifteen Year Period: a Nationwide Study. PLoS One 10:e0132048. doi: 10.1371/journal.pone.0132048
Kristensen, S. L., Rørth, R., Jhund, P. S., Docherty, K. F., Sattar, N., Preiss, D., et al. (2019). Cardiovascular, mortality, and kidney outcomes with GLP-1 receptor agonists in patients with type 2 diabetes: a systematic review and meta-analysis of cardiovascular outcome trials. Lancet Diabetes Endocrinol. 7, 776–785. doi: 10.1016/S2213-8587(19)30249-9
Lau, J., Bloch, P., Schäffer, L., Pettersson, I., Spetzler, J., Kofoed, J., et al. (2015). Discovery of the Once-Weekly Glucagon-Like Peptide-1 (GLP-1) Analogue Semaglutide. J. Med. Chem. 58, 7370–7380. doi: 10.1021/acs.jmedchem.5b00726
Lee, W. J., Jeoung, J. W., Na, K. I., Kim, Y. K., Kim, C. Y., and Park, K. H. (2018). Relationship Between Open-angle Glaucoma and Stroke: a 2010 to 2012 Korea National Health and Nutrition Examination Survey. J. Glaucoma. 27, 22–27. doi: 10.1097/IJG.0000000000000829
Leske, M. C., Connell, A. M., Schachat, A. P., and Hyman, L. (1994). The Barbados Eye Study. Prevalence of open angle glaucoma. Arch. Ophthalmol. 112, 821–829. doi: 10.1001/archopht.1994.01090180121046
Li, W. A., Moore-Langston, S., Chakraborty, T., Rafols, J. A., Conti, A. C., and Ding, Y. (2013). Hyperglycemia in stroke and possible treatments. Neurol. Res. 35, 479–491. doi: 10.1179/1743132813Y.0000000209
Li, Y., Ryu, C., Munie, M., Noorulla, S., Rana, S., Edwards, P., et al. (2018). Association of Metformin Treatment with Reduced Severity of Diabetic Retinopathy in Type 2 Diabetic Patients. J. Diabetes Res. 2018:2801450. doi: 10.1155/2018/2801450
Lim, S. W., van Wijngaarden, P., Harper, C. A., and Al-Qureshi, S. H. (2019). Early worsening of diabetic retinopathy due to intensive glycaemic control. Clin. Exp. Ophthalmol. 47, 265–273. doi: 10.1111/ceo.13393
Lin, H. C., Stein, J. D., Nan, B., Childers, D., Newman-Casey, P. A., Thompson, D. A., et al. (2015). Association of Geroprotective Effects of Metformin and Risk of Open-Angle Glaucoma in Persons With Diabetes Mellitus. JAMA Ophthalmol. 133, 915–923. doi: 10.1001/jamaophthalmol.2015.1440
Lin, T. C., Hwang, D. K., Hsu, C. C., Peng, C. H., Wang, M. L., Chiou, S. H., et al. (2017). Protective effect of metformin against retinal vein occlusions in diabetes mellitus - A nationwide population-based study. PLoS One 12:e0188136. doi: 10.1371/journal.pone.0188136
Lingvay, I., Catarig, A. M., Frias, J. P., Kumar, H., Lausvig, N. L., le Roux, C. W., et al. (2019). Efficacy and safety of once-weekly semaglutide versus daily canagliflozin as add-on to metformin in patients with type 2 diabetes (SUSTAIN 8): a double-blind, phase 3b, randomised controlled trial. Lancet Diabetes Endocrinol. 7, 834–844. doi: 10.1016/S2213-8587(19)30311-0
Luciani, P., Deledda, C., Benvenuti, S., Cellai, I., Squecco, R., Monici, M., et al. (2010). Differentiating effects of the glucagon-like peptide-1 analogue exendin-4 in a human neuronal cell model. Cell. Mol. Life Sci. 67, 3711–3723. doi: 10.1007/s00018-010-0398-3
Maleškić, S., Kusturica, J., Gušić, E., Rakanović-Todić, M., Šečić, D., Burnazović-Ristić, L., et al. (2017). Metformin use associated with protective effects for ocular complications in patients with type 2 diabetes - observational study. Acta Med. Acad. 46, 116–123. doi: 10.5644/ama2006-124.196
Mansur, R. B., Ahmed, J., Cha, D. S., Woldeyohannes, H. O., Subramaniapillai, M., Lovshin, J., et al. (2017). Liraglutide promotes improvements in objective measures of cognitive dysfunction in individuals with mood disorders: a pilot, open-label study. J. Affect. Disord. 2017, 114–120. doi: 10.1016/j.jad.2016.09.056
Marso, S. P., Bain, S. C., Consoli, A., Eliaschewitz, F. G., Jódar, E., Leiter, L. A., et al. (2016a). SUSTAIN-6 Investigators. Semaglutide and Cardiovascular Outcomes in Patients with Type 2 Diabetes. N. Engl. J. Med. 375, 1834–1844. doi: 10.1056/NEJMoa1607141
Marso, S. P., Daniels, G. H., Brown-Frandsen, K., Kristensen, P., Mann, J. F., Nauck, M. A., et al. (2016b). Liraglutide and Cardiovascular Outcomes in Type 2 Diabetes. N. Engl. J. Med. 375, 311–322. doi: 10.1056/NEJMoa1603827
Maskery, M. P., Holscher, C., Jones, S. P., Price, C. I., Strain, W. D., Watkins, C. L., et al. (2021). Glucagon-like peptide-1 receptor agonists as neuroprotective agents for ischemic stroke: a systematic scoping review. J. Cereb. Blood Flow Metab. 41, 14–30. doi: 10.1177/0271678X20952011
McClean, P. L., Parthsarathy, V., Faivre, E., and Hölscher, C. (2011). The diabetes drug liraglutide prevents degenerative processes in a mouse model of Alzheimer’s disease. J. Neurosci. 31, 6587–6594. doi: 10.1523/JNEUROSCI.0529-11.2011
Mehta, K., Behl, T., Kumar, A., Uddin, M. S., Zengin, G., and Arora, S. (2021). Deciphering the Neuroprotective Role of Glucagon-like Peptide-1 Agonists in Diabetic Neuropathy: current Perspective and Future Directions. Curr. Protein Pept. Sci. 22, 4–18. doi: 10.2174/1389203721999201208195901
Mosenzon, O., Blicher, T. M., Rosenlund, S., Eriksson, J. W., Heller, S., Hels, O. H., et al. (2019). Efficacy and safety of oral semaglutide in patients with type 2 diabetes and moderate renal impairment (PIONEER 5): a placebo-controlled, randomised, phase 3a trial. Lancet Diabetes Endocrinol. 7, 515–527. doi: 10.1016/S2213-8587(19)30192-5
Mousa, S. A., and Ayoub, B. M. (2019). Repositioning of dipeptidyl peptidase-4 inhibitors and glucagon like peptide-1 agonists as potential neuroprotective agents. Neural. Regen. Res. 14, 745–748. doi: 10.4103/1673-5374.249217
Muller, C., Cheung, N. W., Dewey, H., Churilov, L., Middleton, S., Thijs, V., et al. (2018). Treatment with exenatide in acute ischemic stroke trial protocol: a prospective, randomized, open label, blinded end-point study of exenatide vs. standard care in post stroke hyperglycemia. Int. J. Stroke 13, 857–862. doi: 10.1177/1747493018784436
Mullins, R. J., Mustapic, M., Chia, C. W., Carlson, O., Gulyani, S., Tran, J., et al. (2019). A Pilot Study of Exenatide Actions in Alzheimer’s Disease. Curr. Alzheimer. Res. 16, 741–752. doi: 10.2174/1567205016666190913155950
Na, K. S., Kim, J. H., Paik, J. S., Cho, W. K., Ha, M., Park, Y. G., et al. (2020). Underweight increases the risk of primary open-angle glaucoma in diabetes patients: a Korean nationwide cohort study. Medicine 99:e19285. doi: 10.1097/MD.0000000000019285
Nauck, M. A., Petrie, J. R., Sesti, G., Mannucci, E., Courrèges, J. P., Lindegaard, M. L., et al. (2016). A Phase 2, Randomized, Dose-Finding Study of the Novel Once-Weekly Human GLP-1 Analog, Semaglutide, Compared With Placebo and Open-Label Liraglutide in Patients With Type 2 Diabetes. Diabetes Care 39, 231–241. doi: 10.2337/dc15-0165
Neth, B. J., and Craft, S. (2017). Insulin resistance and Alzheimer’s disease: bioenergetic linkages. Front. Aging Neurosci. 9:345. doi: 10.3389/fnagi.2017.00345
Newton, J. L. (2005). Effect of age-related changes in gastric physiology on tolerability of medications for older people. Drugs Aging 22, 655–661. doi: 10.2165/00002512-200522080-00003
No authors listed. (1998). Early worsening of diabetic retinopathy in the Diabetes Control and Complications Trial. Arch. Ophthalmol. 116, 874–886. doi: 10.1001/archopht.116.7.874
No authors listed. (1999). Intensive blood-glucose control with sulphonylureas or insulin compared with conventional treatment and risk of complications in patients with type 2 diabetes (UKPDS 33). UK Prospective Diabetes Study (UKPDS) Group. Lancet 352, 837–853. doi: 10.1016/s0140-6736(98)07019-6
O’Brien, J. T., Erkinjuntti, T., Reisberg, B., Roman, G., Sawada, T., Pantoni, L., et al. (2003). Vascular cognitive impairment. Lancet Neurol. 2, 89–98. doi: 10.1016/s1474-4422(03)00305-3
Ong, L. K., Walker, F. R., and Nilsson, M. (2017). Is Stroke a Neurodegenerative Condition? A Critical Review of Secondary Neurodegeneration and Amyloid-beta Accumulation after Stroke. AIMS Med. Sci. 4, 1–16. doi: 10.3934/medsci.2017.1.1
Oshitari, T., Fujimoto, N., Hanawa, K., Adachi-Usami, E., and Roy, S. (2007). Effect of chronic hyperglycemia on intraocular pressure in patients with diabetes. Am. J. Ophthalmol. 143, 363–365. doi: 10.1016/j.ajo.2006.09.033
Ott, C., Raff, U., Schmidt, S., Kistner, I., Friedrich, S., Bramlage, P., et al. (2014). Effects of saxagliptin on early microvascular changes in patients with type 2 diabetes. Cardiovasc. Diabetol. 13:19. doi: 10.1186/1475-2840-13-19
Patel, V. J., Joharapurkar, A. A., Shah, G. B., and Jain, M. R. (2014). Effect of GLP-1 based therapies on diabetic dyslipidemia. Curr. Diabetes Rev. 10, 238–250. doi: 10.2174/1573399810666140707092506
Perlmuter, L. C., Hakami, M. K., Hodgson-Harrington, C., Ginsberg, J., Katz, J., Singer, D. E., et al. (1984). Decreased cognitive function in aging non-insulin-dependent diabetic patients. Am. J. Med. 77, 1043–1048. doi: 10.1016/0002-9343(84)90186-4
Perry, T., Holloway, H. W., Weerasuriya, A., Mouton, P. R., Duffy, K., Mattison, J. A., et al. (2007). Evidence of GLP-1-mediated neuroprotection in an animal model of pyridoxine-induced peripheral sensory neuropathy. Exp. Neurol. 203, 293–301. doi: 10.1016/j.expneurol.2006.09.028
Peters, D., Bengtsson, B., and Heijl, A. (2013). Lifetime risk of blindness in open-angle glaucoma. Am. J. Ophthalmol. 156, 724–730. doi: 10.1016/j.ajo.2013.05.027
Pfeffer, M. A., Claggett, B., Diaz, R., Dickstein, K., Gerstein, H. C., Køber, L. V., et al. (2015). Lixisenatide in Patients with Type 2 Diabetes and Acute Coronary Syndrome. N. Engl. J. Med. 373, 2247–2257. doi: 10.1056/NEJMoa1509225
Pieber, T. R., Bode, B., Mertens, A., Cho, Y. M., Christiansen, E., Hertz, C. L., et al. (2019). Efficacy and safety of oral semaglutide with flexible dose adjustment versus sitagliptin in type 2 diabetes (PIONEER 7): a multicentre, open-label, randomised, phase 3a trial. Lancet Diabetes Endocrinol. 7, 528–539. doi: 10.1016/S2213-8587(19)30194-9
Pratley, R. E., Aroda, V. R., Lingvay, I., Lüdemann, J., Andreassen, C., Navarria, A., et al. (2018). SUSTAIN 7 investigators. Semaglutide versus dulaglutide once weekly in patients with type 2 diabetes (SUSTAIN 7): a randomised, open-label, phase 3b trial. Lancet Diabetes Endocrinol. 6, 275–286. doi: 10.1016/S2213-8587(18)30024-X
Pratley, R., Amod, A., Hoff, S. T., Kadowaki, T., Lingvay, I., Nauck, M., et al. (2019). Oral semaglutide versus subcutaneous liraglutide and placebo in type 2 diabetes (PIONEER 4): a randomised, double-blind, phase 3a trial. Lancet 394, 39–50. doi: 10.1016/S0140-6736(19)31271-1
Quigley, H. A. (2009). Can diabetes be good for glaucoma? Why can’t we believe our own eyes (or data)? Arch. Ophthalmol. 127, 227–229. doi: 10.1001/archophthalmol.2008.596
Ramos, H., Bogdanov, P., Sampedro, J., Huerta, J., Simó, R., and Hernández, C. (2020). Beneficial Effects of Glucagon-Like Peptide-1 (GLP-1) in Diabetes-Induced Retinal Abnormalities: involvement of Oxidative Stress. Antioxidants 9:846. doi: 10.3390/antiox9090846
Reger, M. A., Watson, G. S., Frey, W. H. II, Cholerton, B., Keeling, M. L., Belongia, D. A., et al. (2006). Effects of intranasal insulin on cognition in memory-impaired older adults: modulation by APOE genotype. Neurobiol. Aging. 27, 451–458. doi: 10.1016/j.neurobiolaging.2005.03.016
Reger, M. A., Watson, G. S., Green, P. S., Baker, L. D., Cholerton, B., Fishel, M. A., et al. (2008a). Intranasal insulin administration dose-dependently modulates verbal memory and plasma amyloid-beta in memory-impaired older adults. J. Alzheimers. Dis. 13, 323–331. doi: 10.3233/jad-2008-13309
Reger, M. A., Watson, G. S., Green, P. S., Wilkinson, C. W., Baker, L. D., Cholerton, B., et al. (2008b). Intranasal insulin improves cognition and modulates beta-amyloid in early AD. Neurology 70, 440–448. doi: 10.1212/01.WNL.0000265401.62434.36
Ren, X., Sun, L., Wei, L., Liu, J., Zhu, J., Yu, Q., et al. (2020). Liraglutide Up-regulation Thioredoxin Attenuated Müller Cells Apoptosis in High Glucose by Regulating Oxidative Stress and Endoplasmic Reticulum Stress. Curr. Eye Res. 45, 1283–1291. doi: 10.1080/02713683.2020.1737137
Rim, T. H., Lee, S. Y., Bae, H. W., Kim, S. S., and Kim, C. Y. (2018). Increased stroke risk among patients with open-angle glaucoma: a 10-year follow-up cohort study. Br. J. Ophthalmol. 102, 338–343. doi: 10.1136/bjophthalmol-2017-310415
Rizvi, A. A., Patti, A. M., Giglio, R. V., Nikolic, D., Amato, A., Al-Busaidi, N., et al. (2015). Liraglutide improves carotid intima-media thickness in patients with type 2 diabetes and non-alcoholic fatty liver disease: an 8-month prospective pilot study. Expert Opin. Biol. Ther. 15, 1391–1397. doi: 10.1517/14712598.2015.1067299
Rizzo, M., Abate, N., Chandalia, M., Rizvi, A. A., Giglio, R. V., Nikolic, D., et al. (2015). Liraglutide reduces oxidative stress and restores heme oxygenase-1 and ghrelin levels in patients with type 2 diabetes: a prospective pilot study. J. Clin. Endocrinol. Metab. 100, 603–606. doi: 10.1210/jc.2014-2291
Rizzo, M., Rizvi, A. A., Patti, A. M., Nikolic, D., Giglio, R. V., Castellino, G., et al. (2016). Liraglutide improves metabolic parameters and carotid intima-media thickness in diabetic patients with the metabolic syndrome: an 18-month prospective study. Cardiovasc. Diabetol. 15:162. doi: 10.1186/s12933-016-0480-8
Rodbard, H. W., Lingvay, I., Reed, J., de la Rosa, R., Rose, L., Sugimoto, D., et al. (2018). Semaglutide Added to Basal Insulin in Type 2 Diabetes (SUSTAIN 5): a Randomized, Controlled Trial. J. Clin. Endocrinol. Metab. 103, 2291–2301. doi: 10.1210/jc.2018-00070
Rodbard, H. W., Rosenstock, J., Canani, L. H., Deerochanawong, C., Gumprecht, J., Lindberg, S. Ø, et al. (2019). Oral Semaglutide Versus Empagliflozin in Patients With Type 2 Diabetes Uncontrolled on Metformin: the PIONEER 2 Trial. Diabetes Care 42, 2272–2281. doi: 10.2337/dc19-0883
Rosenstock, J., Allison, D., Birkenfeld, A. L., Blicher, T. M., Deenadayalan, S., Jacobsen, J. B., et al. (2019). Effect of Additional Oral Semaglutide vs Sitagliptin on Glycated Hemoglobin in Adults With Type 2 Diabetes Uncontrolled With Metformin Alone or With Sulfonylurea: the PIONEER 3 Randomized Clinical Trial. JAMA 321, 1466–1480. doi: 10.1001/jama.2019.2942
Sandoval, D., and Sisley, S. R. (2015). Brain GLP-1 and insulin sensitivity. Mol. Cell. Endocrinol. 2015, 27–32. doi: 10.1016/j.mce.2015.02.017
Schrijvers, E. M., Witteman, J. C., Sijbrands, E. J., Hofman, A., Koudstaal, P. J., and Breteler, M. M. (2010). Insulin metabolism and the risk of Alzheimer disease: the Rotterdam study. Neurology 75, 1982–1987. doi: 10.1212/WNL.0b013e3181ffe4f6
Secher, A., Jelsing, J., Baquero, A. F., Hecksher-Sørensen, J., Cowley, M. A., Dalbøge, L. S., et al. (2014). The arcuate nucleus mediates GLP-1 receptor agonist liraglutide-dependent weight loss. J. Clin. Invest. 124, 4473–4488. doi: 10.1172/JCI75276
Seidu, S., Mellbin, L., Kaiser, M., and Khunti, K. (2021). Will oral semaglutide be a game-changer in the management of type 2 diabetes in primary care? Prim. Care Diabetes 15, 59–68. doi: 10.1016/j.pcd.2020.07.011
Seino, Y., Terauchi, Y., Osonoi, T., Yabe, D., Abe, N., Nishida, T., et al. (2018). Safety and efficacy of semaglutide once weekly vs sitagliptin once daily, both as monotherapy in Japanese people with type 2 diabetes. Diabetes Obes. Metab. 20, 378–388. doi: 10.1111/dom.13082
Sorli, C., Harashima, S. I., Tsoukas, G. M., Unger, J., Karsbøl, J. D., Hansen, T., et al. (2017). Efficacy and safety of once-weekly semaglutide monotherapy versus placebo in patients with type 2 diabetes (SUSTAIN 1): a double-blind, randomised, placebo-controlled, parallel-group, multinational, multicentre phase 3a trial. Lancet Diabetes Endocrinol. 5, 251–260. doi: 10.1016/S2213-8587(17)30013-X
Sterling, J. K., Adetunji, M. O., Guttha, S., Bargoud, A. R., Uyhazi, K. E., Ross, A. G., et al. (2020). GLP-1 Receptor Agonist NLY01 Reduces Retinal Inflammation and Neuron Death Secondary to Ocular Hypertension. Cell Rep. 33:108271. doi: 10.1016/j.celrep.2020.108271
Sterling, J., Hua, P., Dunaief, J. L., Cui, Q. N., and VanderBeek, B. L. (2021). Glucagon-like peptide 1 receptor agonist use is associated with reduced risk for glaucoma [published online ahead of print, 2021 Aug 19]. Br. J. Ophthalmol. Bjophthalmol. 2021:319232. doi: 10.1136/bjophthalmol-2021-319232
Strain, W. D., Holst, A. G., Rasmussen, S., Saevereid, H. A., and James, M. A. (2020). Effects of liraglutide and semaglutide on stroke subtypes in patients with type 2 diabetes: a post hoc analysis of the LEADER, SUSTAIN 6 and PIONEER 6 trials. Euro. Heart J. 41:ehaa946.2424.
Svenningsson, P., Wirdefeldt, K., Yin, L., Fang, F., Markaki, I., Efendic, S., et al. (2016). Reduced incidence of Parkinson’s disease after dipeptidyl peptidase-4 inhibitors-A nationwide case-control study. Mov. Disord. 31, 1422–1423. doi: 10.1002/mds.26734
Syngle, A., Chahal, S., and Vohra, K. (2021). Efficacy and tolerability of DPP4 inhibitor, teneligliptin, on autonomic and peripheral neuropathy in type 2 diabetes: an open label, pilot study. Neurol. Sci. 42, 1429–1436. doi: 10.1007/s10072-020-04681-2
Tang, H., Li, G., Zhao, Y., Wang, F., Gower, E. W., Shi, L., et al. (2018). Comparisons of diabetic retinopathy events associated with glucose-lowering drugs in patients with type 2 diabetes mellitus: a network meta-analysis. Diabetes Obes. Metab. 20, 1262–1279. doi: 10.1111/dom.13232
Taylor, O. M., and Lam, C. (2020). The Effect of Dipeptidyl Peptidase-4 Inhibitors on Macrovascular and Microvascular Complications of Diabetes Mellitus: a Systematic Review. Curr. Ther. Res. Clin. Exp. 93:100596. doi: 10.1016/j.curtheres.2020.100596
Tham, Y.-C., Li, X., Wong, T. Y., Quigley, H. A., Aung, T., and Cheng, C.-Y. (2014). Global prevalence of glaucoma and projections of glaucoma burden through 2040: a systematic review and meta-analysis. Ophthalmology 121, 2081–2090. doi: 10.1016/j.ophtha.2014.05.013
The U.S. Food and Drug administration (2019). FDA Approves First Oral GLP-1 Treatment for Type 2 Diabetes. Available online at: https://www.fda.gov/news-events/press-announcements/fda-approves-first-oral-glp-1-treatment-type-2-diabetes (accessed March 31, 2021).
Tielsch, J. M., Katz, J., Quigley, H. A., Javitt, J. C., and Sommer, A. (1995). Diabetes, intraocular pressure, and primary open-angle glaucoma in the Baltimore Eye Survey. Ophthalmology 102, 48–53. doi: 10.1016/s0161-6420(95)31055-x
Topouzis, F., and Anastasopoulos, E. (2007). Glaucoma—The Importance of Early Detection and Early Treatment. Eur. Ophthalmic. Rev. 13, 13–14. doi: 10.17925/EOR.2007.00.00.13
Trujillo, J. M., Nuffer, W., and Smith, B. A. (2021). GLP-1 receptor agonists: an updated review of head-to-head clinical studies. Ther. Adv. Endocrinol. Metab. 12:2042018821997320. doi: 10.1177/2042018821997320
Uzoigwe, C., Liang, Y., Whitmire, S., and Paprocki, Y. (2021). Semaglutide Once-Weekly Persistence and Adherence Versus Other GLP-1 RAs in Patients with Type 2 Diabetes in a US Real-World Setting. Diabetes Ther. 12, 1475–1489. doi: 10.1007/s13300-021-01053-7
Vanhanen, M., Kuusisto, J., Koivisto, K., Mykkänen, L., Helkala, E. L., Hänninen, T., et al. (1999). Type-2 diabetes and cognitive function in a non-demented population. Acta Neurol. Scand. 100, 97–101. doi: 10.1111/j.1600-0404.1999.tb01045.x
Vilsbøll, T., Bain, S. C., Leiter, L. A., Lingvay, I., Matthews, D., Simó, R., et al. (2018). Semaglutide, reduction in glycated haemoglobin and the risk of diabetic retinopathy. Diabetes Obes. Metab. 20, 889–897. doi: 10.1111/dom.13172
Wang, T., Hong, J. L., Gower, E. W., Pate, V., Garg, S., Buse, J. B., et al. (2018). Incretin-Based Therapies and Diabetic Retinopathy: real-World Evidence in Older U.S. Adults. Diabetes Care. 41, 1998–2009. doi: 10.2337/dc17-2285
Warren, M., Chaykin, L., Trachtenbarg, D., Nayak, G., Wijayasinghe, N., and Cariou, B. (2018). Semaglutide as a therapeutic option for elderly patients with type 2 diabetes: pooled analysis of the SUSTAIN 1-5 trials. Diabetes Obes. Metab. 20, 2291–2297. doi: 10.1111/dom.13331
Watson, K. T., Wroolie, T. E., Tong, G., Foland-Ross, L. C., Frangou, S., Singh, M., et al. (2019). Neural correlates of liraglutide effects in persons at risk for Alzheimer’s disease. Behav. Brain Res. 356, 271–278. doi: 10.1016/j.bbr.2018.08.006
Welinder, L. G., Riis, A. H., Knudsen, L. L., and Thomsen, R. W. (2009). Diabetes, glycemic control and risk of medical glaucoma treatment: a population-based case-control study. Clin. Epidemiol. 1, 125–31. doi: 10.2147/clep.s6831
Wensor, M. D., McCarty, C. A., Stanislavsky, Y. L., Livingston, P. M., and Taylor, H. R. (1998). The prevalence of glaucoma in the Melbourne Visual Impairment Project. Ophthalmology 105, 733–739. doi: 10.1016/S0161-6420(98)94031-3
Wright, E. E. Jr., and Aroda, V. R. (2020). Clinical review of the efficacy and safety of oral semaglutide in patients with type 2 diabetes considered for injectable GLP-1 receptor agonist therapy or currently on insulin therapy. Postgrad. Med. 132, 26–36. doi: 10.1080/00325481.2020.1798127
Xue, J., Wang, C., Pan, C., Xing, H., Xu, L., Chen, X., et al. (2020). Effect of DPP-4 inhibitor on elderly patients with T2DM combined with MCI. Exp Ther Med. 19, 1356–1362. doi: 10.3892/etm.2019.8339
Yabe, D., Nakamura, J., Kaneto, H., Deenadayalan, S., Navarria, A., Gislum, M., et al. (2020). Safety and efficacy of oral semaglutide versus dulaglutide in Japanese patients with type 2 diabetes (PIONEER 10): an open-label, randomised, active-controlled, phase 3a trial. Lancet Diabetes Endocrinol. 8, 392–406. doi: 10.1016/S2213-8587(20)30074-7
Yamada, Y., Katagiri, H., Hamamoto, Y., Deenadayalan, S., Navarria, A., Nishijima, K., et al. (2020). Dose-response, efficacy, and safety of oral semaglutide monotherapy in Japanese patients with type 2 diabetes (PIONEER 9): a 52-week, phase 2/3a, randomised, controlled trial. Lancet Diabetes Endocrinol. 8, 377–391. doi: 10.1016/S2213-8587(20)30075-9
Yamamoto, H., Lee, C. E., Marcus, J. N., Williams, T. D., Overton, J. M., Lopez, M. E., et al. (2002). Glucagon-like peptide-1 receptor stimulation increases blood pressure and heart rate and activates autonomic regulatory neurons. J. Clin. Invest. 110, 43–52. doi: 10.1172/JCI15595
Yang, X., Feng, P., Zhang, X., Li, D., Wang, R., Ji, C., et al. (2019). The diabetes drug semaglutide reduces infarct size, inflammation, and apoptosis, and normalizes neurogenesis in a rat model of stroke. Neuropharmacology 158:107748. doi: 10.1016/j.neuropharm.2019.107748
Yildirim Simsir, I., Soyaltin, U. E., and Cetinkalp, S. (2018). Glucagon like peptide-1 (GLP-1) likes Alzheimer’s disease. Diabetes Metab. Syndr. 12, 469–475. doi: 10.1016/j.dsx.2018.03.002
Zhai, R., Xu, H., Hu, F., Wu, J., Kong, X., and Sun, X. (2020). Exendin-4, a GLP-1 receptor agonist regulates retinal capillary tone and restores microvascular patency after ischaemia-reperfusion injury. Br. J. Pharmacol. 177, 3389–3402. doi: 10.1111/bph.15059
Zhang, L., Zhang, L., Li, L., and Hölscher, C. (2018). Neuroprotective effects of the novel GLP-1 long acting analogue semaglutide in the MPTP Parkinson’s disease mouse model. Neuropeptides 71, 70–80. doi: 10.1016/j.npep.2018.07.003
Zhang, L., Zhang, L., Li, L., and Hölscher, C. (2019). Semaglutide is Neuroprotective and Reduces α-Synuclein Levels in the Chronic MPTP Mouse Model of Parkinson’s Disease. J. Parkinsons. Dis. 9, 157–171. doi: 10.3233/JPD-181503
Zhou, M., Wang, W., Huang, W., and Zhang, X. (2014). Diabetes mellitus as a risk factor for open-angle glaucoma: a systematic review and meta-analysis. PLoS One 9:e102972. doi: 10.1371/journal.pone.0102972
Zinman, B., Aroda, V. R., Buse, J. B., Cariou, B., Harris, S. B., Hoff, S. T., et al. (2019a). Efficacy, Safety, and Tolerability of Oral Semaglutide Versus Placebo Added to Insulin With or Without Metformin in Patients With Type 2 Diabetes: the PIONEER 8 Trial. Diabetes Care 42, 2262–2271. doi: 10.2337/dc19-0898
Keywords: glaucoma, GLP-1 receptor agonists, antidiabtics, ophthalmology, neuroprotection, neurodegenerative diseases
Citation: Mouhammad ZA, Vohra R, Horwitz A, Thein A-S, Rovelt J, Cvenkel B, Williams PA, Azuara-Blanco A and Kolko M (2022) Glucagon-Like Peptide 1 Receptor Agonists – Potential Game Changers in the Treatment of Glaucoma? Front. Neurosci. 16:824054. doi: 10.3389/fnins.2022.824054
Received: 28 November 2021; Accepted: 10 January 2022;
Published: 21 February 2022.
Edited by:
Sabrina Reinehr, Ruhr-University Bochum, GermanyReviewed by:
Venkateswarlu Kanamarlapudi, Swansea University Medical School, United KingdomStephanie C. Joachim, Ruhr University Bochum, Germany
Copyright © 2022 Mouhammad, Vohra, Horwitz, Thein, Rovelt, Cvenkel, Williams, Azuara-Blanco and Kolko. This is an open-access article distributed under the terms of the Creative Commons Attribution License (CC BY). The use, distribution or reproduction in other forums is permitted, provided the original author(s) and the copyright owner(s) are credited and that the original publication in this journal is cited, in accordance with accepted academic practice. No use, distribution or reproduction is permitted which does not comply with these terms.
*Correspondence: Miriam Kolko, miriamk@sund.ku.dk