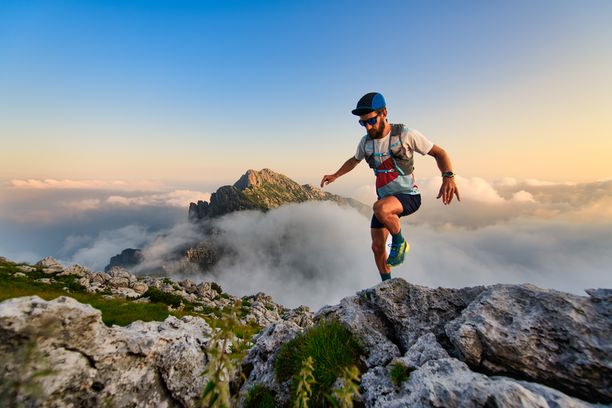
94% of researchers rate our articles as excellent or good
Learn more about the work of our research integrity team to safeguard the quality of each article we publish.
Find out more
MINI REVIEW article
Front. Neurosci., 25 January 2022
Sec. Neurodegeneration
Volume 16 - 2022 | https://doi.org/10.3389/fnins.2022.758182
This article is part of the Research TopicThe Impact of Neurofilament Light Chain (NFL) Quantification in Serum and Cerebrospinal Fluid in Neurodegenerative DiseasesView all 17 articles
Differential diagnosis of neurological disorders and their subtype classification are challenging without specific biomarkers. Genetic forms of these disorders, typified by an autosomal dominant family history, could offer a window to identify potential biomarkers by exploring the presymptomatic stages of the disease. Frontotemporal dementia (FTD) is the second cause of dementia with an age of onset < 65, and its most common mutations are in GRN, C9orf72, and MAPT genes. Several studies have demonstrated that the main proteins involved in FTD pathogenesis can be secreted in exosomes, a specific subtype of extracellular vesicles able to transfer biomolecules between cells avoiding cell-to-cell contact. Neurofilament light chain (NfL) levels in central nervous system have been advocated as biomarkers of axonal injury. NfL concentrations have been found increased in FTD and have been related to disease severity and prognosis. Little information on the relationship between NfL and exosomes in FTD has been collected, deriving mainly from traumatic brain injury. Current review deals with this matter in the attempt to provide an updated discussion of the role of NfL and exosomes as biomarkers of genetic forms of FTD.
Early diagnosis of dementia is challenging, that’s why there is an impelling need for specific biomarkers. Frontotemporal dementia (FTD) encompasses a heterogenous group of neurodegenerative disorders with a wide range of clinical, genetic, and neuropathological features (Bang et al., 2015). About one-third of FTD patients have an autosomal dominant family history (Rohrer et al., 2009), typified by mutations in three genes: granulin (GRN; Baker et al., 2006; Cruts et al., 2006), chromosome 9 open reading frame 72 (C9orf72) (DeJesus-Hernandez et al., 2011; Renton et al., 2011) and microtubule-associated protein tau (MAPT; Hutton et al., 1998). It has been demonstrated that several proteins involved in FTD pathogenesis can be secreted by cells in association with exosomes (Ghidoni et al., 2011; Benussi et al., 2016). Furthermore, mutations in GRN strongly reduce the number of released exosomes also altering their composition (Benussi et al., 2016). Exosomes are a specific subtype of extracellular vesicles (EVs) of 30–150 nm, originating in the endosomal/multivesicular body system and widely distributed in body fluids, including blood. Exosomes can carry a wide variety of DNA, RNA, proteins and lipids, allowing communication between cells avoiding cell-to-cell contact (Raposo and Stoorvogel, 2013; Rajendran et al., 2014). They have been reported as “Trojan horses” of toxic proteins (Ghidoni et al., 2008a), that’s why they may serve as novel biomarkers in neurodegenerative diseases (Rajendran et al., 2014; Longobardi et al., 2021). Exosomes have a lipid bilayer membrane and can cross the blood brain barrier bidirectionally, thus reflecting and tracking neuropathological changes (Chen et al., 2013; Lai et al., 2014). In this context, a number of studies have shown the potential of peripheral blood EVs enriched for neuronal origin (nEVs) to identify biomarkers in several neurological disorders (Mustapic et al., 2017). Moreover, encouraging studies have been published that illustrate how certain biomarkers of AD carried within circulating nEVs, can identify individuals with age-related cognitive decline at an early pre-clinical stage, when symptoms are milder than mild cognitive impairment (Eren et al., 2020). In the same line, significantly lower levels than controls of several excitatory synaptic proteins have been found in plasma nEVs in AD patients (Goetzl et al., 2018). In several neurological diseases, levels of neurofilament light chain (NfL) released from the Central Nervous System (CNS) have been demonstrated to be altered, mainly in the cerebrospinal fluid (CSF; Bridel et al., 2019) but also in serum (Mariotto et al., 2020). Concentrations of NfL, biomarker of axonal damage, are increased in serum of FTD patients and might be related to disease severity and prognosis (Meeter et al., 2016; Rohrer et al., 2016; van der Ende et al., 2019; Benussi et al., 2020). In the present review, the potential role of NfL and exosomes as promising biomarkers for FTD diagnosis are briefly explained in the context of the FTD forms typified by autosomal dominant mutations that allow investigations in the early or even in the presymptomatic stages of the disease.
Frontotemporal dementia is an early-onset form of dementia, with a mean age of symptoms presentation before the age of 65 (Ratnavalli et al., 2002; Knopman et al., 2004). This early dementia is highly hereditary: 30–40% of FTD patients have a positive family history, (Rohrer et al., 2009; Wood et al., 2013; Fostinelli et al., 2018). In FTD families, null mutations in GRN leads to the production of a non-functional or no progranulin protein at all (Baker et al., 2006; Cruts et al., 2006; Ghidoni et al., 2008b; Finch et al., 2009; Sleegers et al., 2009). Mutations in MAPT, encoding for tau protein, typify FTD patients with tau-positive brain inclusions (Hutton et al., 1998; Poorkaj et al., 1998). Furthermore, an intronic expansion of a hexanucleotide repeat in C9orf72 has been found in some families with an autosomal dominant inheritance form of FTD (DeJesus-Hernandez et al., 2011; Renton et al., 2011). Most forms of FTD, encompassing both genetic and sporadic FTD, are characterized by cell inclusion bodies composed of tau or transactive response DNA-binding protein of 43 kDa (TDP-43) (Greaves and Rohrer, 2019). TDP-43 cytoplasmatic inclusion can be found in the CNS of patient with FTD and/or amyotrophic lateral sclerosis (ALS) and could explain neuropathological overlap between these neurodegenerative diseases (Elman et al., 2008).
Neurofilaments are a family of neuronal cytoplasmic proteins divided into three subunits: heavy (NfH), medium (NfM) and light (NfL) chain. They are expressed primarily in neuronal axons where they provide structural support and stabilization of myelinated axons and interact with many proteins and organelles, including mitochondria (Petzold, 2005). NfL is the most abundant and soluble Nf subunit and can be released into blood and CSF in diverse neurological diseases reflecting neuroaxonal injury (Petzold, 2005; Lu et al., 2015; Mattsson et al., 2017; Khalil et al., 2018; Steinacker et al., 2018; Verde et al., 2019). NF gene mutations can cause multiple familial neurodegenerative disorders typified by NF aggregation and transport failure leading to further NF accumulations, including 14 NF-L gene mutations known to cause type 2E and 1F forms of Charcot–Marie–Tooth disease (Yuan et al., 2017). Recently NfL alterations have been also associated with FTD. NfL detection can provide some utility as a biomarker to differentiate specific FTD subtypes and, to support differential diagnosis of FTD from psychiatric disorders. To this regard, it has been recently reported an increase of serum NfL (sNfL) levels in behavioral-FTD but not in psychiatric disorders (Al Shweiki et al., 2019). Furthermore, low CSF level of NfL (cNfL) have been found in presymptomatic carriers of genetic FTD in contrast to high concentration in the symptomatic ones (Scherling et al., 2014; Meeter et al., 2016): in FTD GRN, MAPT or C9orf72 mutation carriers, cNfL levels have reached a 8-fold higher increase in the affected patients than in presymptomatic carriers. On this basis, a role as a biomarker of disease severity and for prediction of the conversion to full dementia has been proposed for cNfL (Scherling et al., 2014; Meeter et al., 2016). Furthermore, it has been shown that sNfL levels were strongly associated with cNfL concentrations in the affected patients (Scherling et al., 2014; Meeter et al., 2016), and that NfL levels associated with disease severity, brain atrophy and patient survival (Meeter et al., 2016). In line with this evidence, van der Ende et al., 2019 showed normal sNfL levels in presymptomatic FTD carriers of GRN, MAPT or C9orf72 mutations and a significant increase of sNfL concentrations after conversion to full dementia. The authors also described higher concentrations of sNfL in presymptomatic converters few years before the disease onset, pointing out to a potential role of sNfL as a prognostic biomarker of genetic FTD (van der Ende et al., 2019).
Exosomes are biologically active entities, facilitating the intercellular communication and the transfer of biomolecules from one cell to another without direct cell-to-cell contact (Raposo and Stoorvogel, 2013; Rajendran et al., 2014). Alteration in intercellular communication in FTD patients with GRN mutation have been previously reported (Benussi et al., 2016). The study (Benussi et al., 2016) showed not only that progranulin was secreted in association with exosomes but also that levels of exosomal progranulin released by fibroblasts as well as the whole release of exosomes were reduced in mutations carrier patients. In brain, Wren et al., 2015, showed a significant alteration in intracellular vesicles trafficking with an accumulation of endosomes and exosomes and a reduction of lysosomes in FTD patients carrying N279K mutation in MAPT. These patients also showed an increase of exosomal proteins in frontal and temporal cortex (Wren et al., 2015). Moreover, it has been shown that both full length TDP-43 and TDP-43 C-terminal fragments were enriched in exosomes isolated from CSF in ALS-FTD patients. On this basis, approaches tackling the transmission of exosomes containing pathological TDP-43 could be a promising therapeutic strategy to halt or delay FTD-ALS progression (Ding et al., 2015). Based on these studies, exosomes and their cargo appear attractive biomarkers that could achieve a high diagnostic efficiency.
The interaction of NfL and exosomes in FTD has been preliminary explored in subjects with traumatic brain injury (TBI). A recent study focused on veterans evidenced that repetitive events of TBI were associated with elevated exosomal and plasma NfL: the years from the first TBI were associated with both plasma and exosomal NfL levels. However, the years since the last TBI positively correlated only with exosomal NfL (Guedes et al., 2020). Similarly, in the study from Peltz et al., 2020 on TBI, NfL in CNS-enriched exosomes isolated from plasma were associated with cognitive impairment, suggesting the utility of exosomal NfL as biomarker of cognitive loss. Conversely, the analysis of plasmatic NfL didn’t show any positive results (Peltz et al., 2020). Alongside a longitudinal study explored exosomal sNfL in patients with moderate-to-severe TBI in association with the free-circulating counterpart (Mondello et al., 2020). The authors found that sNfL levels were higher than their exosomal counterpart and that they positively correlated each other, likely part of a common disease process but pertaining to different pathways. Furthermore, exosomes enriched in sNfL were significantly higher in patients with diffuse TBI rather than in patients with focal lesions, supporting their potential utility in the prediction of neuronal damage (Mondello et al., 2020). In the same line, a study on HIV patients complaining neuropsychological impairment (Sun et al., 2017) showed that neuron-derived exosomes isolated from plasma had increased levels of NfL compared to exosomes from neuropsychologically normal subjects highlighting their usefulness in tracking the worsening of cognitive impairment.
Even though not exhaustive, the present overview summarizes the most relevant evidence collected on the potential role of NfL and exosomes in the genetic form of FTD (Tables 1, 2). The latter can provide information on the presymptomatic stage of the disease, offering a good chance to identify early or prognostic biomarkers and the opportunity to deliver preventive therapeutic strategies in this ideal time to obtain the greatest possibility of success.
Knowledge on the matter discussed is still at the beginning, and further investigation is needed to dissect the potential of this promising field of research and reveal whether the potential that emerged in the TBI study could also apply to genetic FTD. Exosomes represent an important subtype of EVs for the release and transfer of biomolecules among cells, without cells-to-cells contact. The study of the EV content, such as NfL in exosomes, from different tissues and fluids may provide information about the source of origin, reflecting the pathological changes. Moreover, it may predict the course of the disease and the prognosis for the patients, as well as establish a more reliable diagnosis. Since the first EVs description, ultracentrifugation has been the “gold standard” for EVs isolation. Nowadays, additional methodologies have been proposed for a more rapid and efficient EV isolation, such as several commercial kits, based on size exclusion.
Further prospective studies are greatly needed specifically to clarify the performance of exosomal biomarkers in genetic FTD diagnosis and prognosis.
RZ, CS, LB, RS, and RG gave their substantial contribution to conception and design of the manuscript and drafting the manuscript, revising it critically for important intellectual content. All authors have approved the manuscript in its present form for publication and agreed to be accountable for all aspects of the work in ensuring that questions related to the accuracy or integrity of any part of the work are appropriately investigated and resolved.
This work was supported by the Italian Ministry of Health (RF-2016-02361492 and Ricerca Corrente).
The authors declare that the research was conducted in the absence of any commercial or financial relationships that could be construed as a potential conflict of interest.
All claims expressed in this article are solely those of the authors and do not necessarily represent those of their affiliated organizations, or those of the publisher, the editors and the reviewers. Any product that may be evaluated in this article, or claim that may be made by its manufacturer, is not guaranteed or endorsed by the publisher.
Al Shweiki, M. R., Steinacker, P., Oeckl, P., Hengerer, B., Danek, A., Fassbender, K., et al. (2019). Neurofilament light chain as a blood biomarker to differentiate psychiatric disorders from behavioural variant frontotemporal dementia. J. Psychiatr. Res. 113, 137–140. doi: 10.1016/j.jpsychires.2019.03.019
Arrant, A. E., Davis, S. E., Vollmer, R. M., Murchison, C. F., Mobley, J. A., Nana, A. L., et al. (2020). Elevated levels of extracellular vesicles in progranulin-deficient mice and FTD-GRN Patients. Ann. Clin. Transl. Neurol. 7, 2433–2449. doi: 10.1002/acn3.51242
Baker, M., Mackenzie, I. R., Pickering-Brown, S. M., Gass, J., Rademakers, R., Lindholm, C., et al. (2006). Mutations in progranulin cause tau-negative frontotemporal dementia linked to chromosome 17. Nature 442, 916–919. doi: 10.1038/nature05016
Benussi, A., Karikari, T. K., Ashton, N., Gazzina, S., Premi, E., Benussi, L., et al. (2020). Diagnostic and prognostic value of serum NfL and p-Tau(181) in frontotemporal lobar degeneration. J. Neurol. Neurosurg. Psychiatry 91, 960–967. doi: 10.1136/jnnp-2020-323487
Benussi, L., Ciani, M., Tonoli, E., Morbin, M., Palamara, L., Albani, D., et al. (2016). Loss of exosomes in progranulin-associated frontotemporal dementia. Neurobiol. Aging 40, 41–49. doi: 10.1016/j.neurobiolaging.2016.01.001
Bridel, C., van Wieringen, W. N., Zetterberg, H., Tijms, B. M., and Teunissen, C. E., The NFL Group, et al. (2019). Diagnostic value of cerebrospinal fluid neurofilament light protein in neurology: a systematic review and meta-analysis. JAMA Neurol. 76, 1035–1048. doi: 10.1001/jamaneurol.2019.1534
Chen, W. W., Balaj, L., Liau, L. M., Samuels, M. L., Kotsopoulos, S. K., Maguire, C. A., et al. (2013). BEAMing and droplet digital PCR analysis of mutant IDH1 mRNA in glioma patient serum and cerebrospinal fluid extracellular vesicles. Mol. Ther. Nucleic Acids 2:e109. doi: 10.1038/mtna.2013.28
Cruts, M., Gijselinck, I., van der Zee, J., Engelborghs, S., Wils, H., Pirici, D., et al. (2006). Null mutations in progranulin cause ubiquitin-positive frontotemporal dementia linked to chromosome 17q21. Nature 442, 920–924. doi: 10.1038/nature05017
DeJesus-Hernandez, M., Mackenzie, I. R., Boeve, B. F., Boxer, A. L., Baker, M., Rutherford, N. J., et al. (2011). Expanded GGGGCC hexanucleotide repeat in noncoding region of C9ORF72 causes chromosome 9p-linked FTD and ALS. Neuron 72, 245–256. doi: 10.1016/j.neuron.2011.09.011
Ding, X., Ma, M., Teng, J., Teng, R. K., Zhou, S., Yin, J., et al. (2015). Exposure to ALS-FTD-CSF generates TDP-43 aggregates in glioblastoma cells through exosomes and TNTs-like structure. Oncotarget 6, 24178–24191.
Elman, L. B., McCluskey, L., and Grossman, M. (2008). Motor neuron disease and frontotemporal lobar degeneration: a tale of two disorders linked to TDP-43. Neurosignals 16, 85–90. doi: 10.1159/000109762
Eren, E., Hunt, J. F. V., Shardell, M., Chawla, S., Tran, J., Gu, J., et al. (2020). Extracellular vesicle biomarkers of Alzheimer’s disease associated with sub-clinical cognitive decline in late middle age. Alzheimers Dement. 16, 1293–1304. doi: 10.1002/alz.12130
Finch, N., Baker, M., Crook, R., Swanson, K., Kuntz, K., Surtees, R., et al. (2009). Plasma progranulin levels predict progranulin mutation status in frontotemporal dementia patients and asymptomatic family members. Brain 132, 583–591. doi: 10.1093/brain/awn352
Fostinelli, S., Ciani, M., Zanardini, R., Zanetti, O., Binetti, G., Ghidoni, R., et al. (2018). The heritability of frontotemporal lobar degeneration: validation of pedigree classification criteria in a northern Italy cohort. J. Alzheimers Dis. 61, 753–760. doi: 10.3233/JAD-170661
Ghidoni, R., Benussi, L., and Binetti, G. (2008a). Exosomes: the Trojan horses of neurodegeneration. Med. Hypotheses 70, 1226–1227. doi: 10.1016/j.mehy.2007.12.003
Ghidoni, R., Benussi, L., Glionna, M., Franzoni, M., and Binetti, G. (2008b). Low plasma progranulin levels predict progranulin mutations in frontotemporal lobar degeneration. Neurology 71, 1235–1239. doi: 10.1212/01.wnl.0000325058.10218.fc
Ghidoni, R., Paterlini, A., Albertini, V., Glionna, M., Monti, E., Schiaffonati, L., et al. (2011). Cystatin C is released in association with exosomes: a new tool of neuronal communication which is unbalanced in Alzheimer’s disease. Neurobiol. Aging 32, 1435–1442. doi: 10.1016/j.neurobiolaging.2009.08.013
Goetzl, E. J., Abner, E. L., Jicha, G. A., Kapogiannis, D., and Schwartz, J. B. (2018). Declining levels of functionally specialized synaptic proteins in plasma neuronal exosomes with progression of Alzheimer’s disease. FASEB J. 32, 888–893. doi: 10.1096/fj.201700731R
Greaves, C. V., and Rohrer, J. D. (2019). An update on genetic frontotemporal dementia. J. Neurol. 266, 2075–2086. doi: 10.1007/s00415-019-09363-4
Guedes, V. A., Kenney, K., Shahim, P., Qu, B. X., Lai, C., Devoto, C., et al. (2020). Exosomal neurofilament light: a prognostic biomarker for remote symptoms after mild traumatic brain injury? Neurology 94, e2412–e2423. doi: 10.1212/WNL.0000000000009577
Hutton, M., Lendon, C. L., Rizzu, P., Baker, M., Froelich, S., Houlden, H., et al. (1998). Association of missense and 5’-splice-site mutations in tau with the inherited dementia FTDP-17. Nature 393, 702–705. doi: 10.1038/31508
Khalil, M., Teunissen, C. E., Otto, M., Piehl, F., Sormani, M. P., Gattringer, T., et al. (2018). Neurofilaments as biomarkers in neurological disorders. Nat. Rev. Neurol. 14, 577–589. doi: 10.1038/s41582-018-0058-z
Knopman, D. S., Petersen, R. C., Edland, S. D., Cha, R. H., and Rocca, W. A. (2004). The incidence of frontotemporal lobar degeneration in Rochester, Minnesota, 1990 through 1994. Neurology 62, 506–508. doi: 10.1212/01.wnl.0000106827.39764.7e
Lai, C. P., Mardini, O., Ericsson, M., Prabhakar, S., Maguire, C., Chen, J. W., et al. (2014). Dynamic biodistribution of extracellular vesicles in vivo using a multimodal imaging reporter. ACS Nano 28, 483–494. doi: 10.1021/nn404945r
Longobardi, A., Benussi, L., Nicsanu, R., Bellini, S., Ferrari, C., Saraceno, C., et al. (2021). Plasma extracellular vesicle size and concentration are altered in Alzheimer’s disease, dementia with lewy bodies, and frontotemporal dementia. Front. Cell. Dev. Biol. 9:667369. doi: 10.3389/fcell.2021.667369
Lu, C. H., Macdonald-Wallis, C., Gray, E., Pearce, N., Petzold, A., Norgren, N., et al. (2015). Neurofilament light chain: a prognostic biomarker in amyotrophic lateral sclerosis. Neurology 84, 2247–2257. doi: 10.1212/WNL.0000000000001642
Mariotto, S., Sechi, E., and Ferrari, S. (2020). Serum neurofilament light chain studies in neurological disorders, hints for interpretation. J. Neurol. Sci. 416:116986. doi: 10.1016/j.jns.2020.116986
Mattsson, N., Andreasson, U., Zetterberg, H., and Blennow, K., and Alzheimer’s Disease Neuroimaging Initiative (2017). Association of plasma neurofilament light with neurodegeneration in patients with Alzheimer disease. JAMA Neurol. 74, 557–566. doi: 10.1001/jamaneurol.2016.6117
Meeter, L. H., Dopper, E. G., Jiskoot, L. C., Sanchez-Valle, R., Graff, C., Benussi, L., et al. (2016). Neurofilament light chain: a biomarker for genetic frontotemporal dementia. Ann. Clin. Transl. Neurol. 3, 623–636. doi: 10.1002/acn3.325
Mondello, S., Guedes, V. A., Lai, C., Czeiter, E., Amrein, K., Kobeissy, F., et al. (2020). Circulating brain injury exosomal proteins following moderate-to-severe traumatic brain injury: temporal profile, outcome prediction and therapy implications. Cells 9:977. doi: 10.3390/cells9040977
Mustapic, M., Eitan, E., Werner, J. K. Jr., Berkowitz, S. T., Lazaropoulos, M. P., Tran, J., et al. (2017). Plasma extracellular vesicles enriched for neuronal origin: a potential window into brain pathologic processes. Front. Neurosci. 11:278. doi: 10.3389/fnins.2017.00278
Peltz, C. B., Kenney, K., Gill, J., Diaz-Arrastia, R., Gardner, R. C., and Yaffe, K. (2020). Blood biomarkers of traumatic brain injury and cognitive impairment in older veterans. Neurology 95, e1126–e1133. doi: 10.1212/WNL.0000000000010087
Petzold, A. (2005). Neurofilament phosphoforms: surrogate markers for axonal injury, degeneration and loss. J. Neurol. Sci. 233, 183–198. doi: 10.1016/j.jns.2005.03.015
Poorkaj, P., Bird, T. D., Wijsman, E., Nemens, E., Garruto, R. M., Anderson, L., et al. (1998). Tau is a candidate gene for chromosome 17 frontotemporal dementia. Ann. Neurol. 43, 815–825. doi: 10.1002/ana.410430617
Rajendran, L., Bali, J., Barr, M. M., Court, F. A., Krämer-Albers, E. M., Picou, F., et al. (2014). Emerging roles of extracellular vesicles in the nervous system. J. Neurosci. 34, 15482–15489. doi: 10.1523/JNEUROSCI.3258-14.2014
Raposo, G., and Stoorvogel, W. (2013). Extracellular vesicles: exosomes, microvesicles, and friends. J. Cell Biol. 200, 373–383. doi: 10.1083/jcb.201211138
Ratnavalli, E., Brayne, C., Dawson, K., and Hodges, J. R. (2002). The prevalence of frontotemporal dementia. Neurology 58, 1615–1621. doi: 10.1212/wnl.58.11.1615
Renton, A. E., Majounie, E., Waite, A., Simón-Sánchez, J., Rollinson, S., Gibbs, J. R., et al. (2011). A hexanucleotide repeat expansion in C9ORF72 is the cause of chromosome 9p21-linked ALS-FTD. Neuron 72, 257–268. doi: 10.1016/j.neuron.2011.09.010
Rohrer, J. D., Guerreiro, R., Vandrovcova, J., Uphill, J., Reiman, D., Beck, J., et al. (2009). The heritability and genetics of frontotemporal lobar degeneration. Neurology 73, 1451–1456. doi: 10.1212/WNL.0b013e3181bf997a
Rohrer, J. D., Woollacott, I. O., Dick, K. M., Brotherhood, E., Gordon, E., Fellows, A., et al. (2016). Serum neurofilament light chain protein is a measure of disease intensity in frontotemporal dementia. Neurology 87, 1329–1336. doi: 10.1212/WNL.0000000000003154
Scherling, C. S., Hall, T., Berisha, F., Klepac, K., Karydas, A., Coppola, G., et al. (2014). Cerebrospinal fluid neurofilament concentration reflects disease severity in frontotemporal degeneration. Ann. Neurol. 75, 116–126. doi: 10.1002/ana.24052
Sleegers, K., Brouwers, N., Van Damme, P., Engelborghs, S., Gijselinck, I., van der Zee, J., et al. (2009). Serum biomarker for progranulin-associated frontotemporal lobar degeneration. Ann. Neurol. 65, 603–609. doi: 10.1002/ana.21621
Steinacker, P., Anderl-Straub, S., Diehl-Schmid, J., Semler, E., Uttner, I., von Arnim, C. A. F., et al. (2018). Serum neurofilament light chain in behavioral variant frontotemporal dementia. Neurology 91, e1390–e1401. doi: 10.1212/WNL.0000000000006318
Sun, B., Dalvi, P., Abadjian, L., Tang, N., and Pulliam, L. (2017). Blood neuron-derived exosomes as biomarkers of cognitive impairment in HIV. AIDS 31, F9–F17. doi: 10.1097/QAD.0000000000001595
Toft, A., Roos, P., Jääskeläinen, O., Musaeus, C. S., Henriksen, E. E., Johannsen, P., et al. (2020). Serum neurofilament light in patients with frontotemporal dementia caused by CHMP2B mutation. Dement. Geriatr. Cogn. Disord. 49, 533–538. doi: 10.1159/000513877
van der Ende, E. L., Bron, E. E., Poos, J. M., Jiskoot, L. C., Panman, J. L., Papma, J. M., et al. (2021). A data-driven disease progression model of fluid biomarkers in genetic frontotemporal dementia. Brain 11:awab382. doi: 10.1093/brain/awab382
van der Ende, E. L., Meeter, L. H., Poos, J. M., Panman, J. L., Jiskoot, L. C., Dopper, E. G. P., et al. (2019). Serum neurofilament light chain in genetic frontotemporal dementia: a longitudinal, multicentre cohort study. Lancet Neurol. 18, 1103–1111. doi: 10.1016/S1474-4422(19)30354-0
Verde, F., Steinacker, P., Weishaupt, J. H., Kassubek, J., Oeckl, P., Halbgebauer, S., et al. (2019). Neurofilament light chain in serum for the diagnosis of amyotrophic lateral sclerosis. J. Neurol. Neurosurg. Psychiatry 90, 157–164. doi: 10.1136/jnnp-2018-318704
Wilke, C., Reich, S., van Swieten, J. C., Borroni, B., Sanchez-Valle, R., Moreno, F., et al. (2021). Stratifying the presymptomatic phase of genetic frontotemporal dementia by serum NfL and pNfH: a longitudinal multicentre study. Ann. Neurol. 9, 33–47. doi: 10.1002/ana.26265
Wood, E. M., Falcone, D., Suh, E., Irwin, D. J., Chen-Plotkin, A. S., Lee, E. B., et al. (2013). Development and validation of pedigree classification criteria for frontotemporal lobar degeneration. JAMA Neurol. 70, 1411–1417. doi: 10.1001/jamaneurol.2013.3956
Wren, M. C., Zhao, J., Liu, C. C., Murray, M. E., Atagi, Y., Davis, M. D., et al. (2015). Frontotemporal dementia-associated N279K tau mutant disrupts subcellular vesicle trafficking and induces cellular stress in iPSC-derived neural stem cells. Mol. Neurodegener. 10:46. doi: 10.1186/s13024-015-0042-7
Keywords: neurofilament, NfL, exosomes, neurodegeneration, genetic frontotemporal dementia, presymptomatic carriers
Citation: Zanardini R, Saraceno C, Benussi L, Squitti R and Ghidoni R (2022) Exploring Neurofilament Light Chain and Exosomes in the Genetic Forms of Frontotemporal Dementia. Front. Neurosci. 16:758182. doi: 10.3389/fnins.2022.758182
Received: 13 August 2021; Accepted: 03 January 2022;
Published: 25 January 2022.
Edited by:
Massimiliano Filosto, University of Brescia, ItalyReviewed by:
Andrea Vergallo, Sorbonne Université, FranceCopyright © 2022 Zanardini, Saraceno, Benussi, Squitti and Ghidoni. This is an open-access article distributed under the terms of the Creative Commons Attribution License (CC BY). The use, distribution or reproduction in other forums is permitted, provided the original author(s) and the copyright owner(s) are credited and that the original publication in this journal is cited, in accordance with accepted academic practice. No use, distribution or reproduction is permitted which does not comply with these terms.
*Correspondence: Roberta Ghidoni, cmdoaWRvbmlAZmF0ZWJlbmVmcmF0ZWxsaS5ldQ==
Disclaimer: All claims expressed in this article are solely those of the authors and do not necessarily represent those of their affiliated organizations, or those of the publisher, the editors and the reviewers. Any product that may be evaluated in this article or claim that may be made by its manufacturer is not guaranteed or endorsed by the publisher.
Research integrity at Frontiers
Learn more about the work of our research integrity team to safeguard the quality of each article we publish.