- Physiology Research Center, Institute for Basic Sciences, Kashan University of Medical Sciences, Kashan, Iran
Introduction: It is well known that the intestinal bacteria substantially affect physiological processes in many body organs. Especially, through a bidirectional communication called as gut-microbiota-brain axis, the gut microbiota deeply influences development and function of the nervous system. Hippocampus, as a part of medial temporal lobe, is known to be involved in cognition, emotion, and anxiety. Growing evidence indicates that the hippocampus is a target of the gut microbiota. We used a broad search linking the hippocampus with the gut microbiota and probiotics.
Methods: All experimental studies and clinical trials published until end of 2021 were reviewed. Influence of the gut microbiota on the behavioral, electrophysiological, biochemical and histological aspects of the hippocampus were evaluated in this review.
Results: The effect of disrupted gut microbiota and probiotic supplements on the microbiota-hippocampus link is also considered. Studies show that a healthy gut microbiota is necessary for normal hippocampus dependent learning and memory and synaptic plasticity. The known current mechanisms are production and modulation of neurotrophins, neurotransmitters and receptors, regulation of intracellular molecular processes, normalizing the inflammatory/anti-inflammatory and oxidative/antioxidant factors, and histological stability of the hippocampus. Activity of the hippocampal neuronal circuits as well as behavioral functions of the hippocampus positively respond to different mixtures of probiotic bacteria.
Discussion: Growing evidence from animal researches indicate a close association between the hippocampus with the gut microbiota and probiotic bacteria as well. However, human studies and clinical trials verifying such a link are scant. Since the most of papers on this topic have been published over the past 3 years, intensive future research awaits.
1 Introduction
The gut microbiota refers to all the species of commensal cells comprising approximately 100 trillion microbes that inhabits the entire gastrointestinal tract. With over 1,000 species and 7,000 strains the microbiota is known as a dynamic ecosystem dominated by anaerobic bacteria, but also includes viruses and bacteriophages, protozoa, archaea, and fungi (Lankelma et al., 2015). The two most prominent phyla of the gut microbiota present in human are Firmicutes and Bacteroidetes (Eckburg et al., 2005; Qin et al., 2010; Bäckhed, 2011). It encompasses almost 1–2 kg in adult human (Forsythe and Kunze, 2013; Toda et al., 2019) that equals weight (1.5 kg) of a normal adult brain (Parent and Carpenter, 1996). Although in primary studies the usefulness of the gut microbes was mostly considered in the gastrointestinal tract, however, it was found that, through production of a variety of bioactive substances, this intestinal flora impacts various body organs. Especially, the gut microbiota has a bidirectional communication with the nervous system called as the “microbiota-gut-brain axis” (Cryan and O’Mahony, 2011). This mutual connection occurs through several routs including neural, immune, metabolic and endocrine pathways (Novotny et al., 2019), and an intact gut microbiota is required for proper brain function (Grenham et al., 2011).
The brain is an organ that is more susceptible to both internal and external environmental factors in adolescence and early adulthood. During this period, the neuronal architecture and function undergo rapid modulation to cope with environmental challenges (Grenham et al., 2011). The gut microbiota plays a role in developmental programming of the brain, specifically synapse maturation, and synaptogenesis (Diaz Heijtz et al., 2011).
Hippocampus is the well-known area of brain involved in behavioral function. Particularly, it is a critical brain region associated with learning and memory and, in turn, is closely related to dementia and many other mental disorders such as depression and anxiety (Toda et al., 2019). For these, it is not surprising that the hippocampus has long term been under focus of behavioral and electrophysiological investigations. Histologically regular neuronal circuits of hippocampus have been subject of the experimental forms of synaptic plasticity, long term potentiation (LTP) and long term depression (LTD); both are known as candidate mechanisms of learning and memory.
Favorable effect of the gut microbiota on, at least, some cognitive brain function undoubtedly must be preceded through affecting the hippocampus. Although still scarce, however, evidence suggesting a direct link between the gut microbiota and hippocampal formation is increasing. In line, numerous studies have shown that the intestinal microbiota may affect hippocampal-related behaviors including learning and memory (Tang et al., 2021). The hippocampus appears to be particularly (Moloney et al., 2017) subject to the microbiota-gut-brain axis signaling related to neuronal morphology, neurogenesis, and neurotransmission (Clarke et al., 2013; Ogbonnaya et al., 2015; Luczynski et al., 2016).
Normal function of the brain strongly depends on natural composition of the gut flora, known as “Eubiosis” (Zhang et al., 2015). “Dysbiosis,” on the other hand, is reflected in decreased intestinal biodiversity or increased pathogenic bacteria. Under dysbiosis, the messages sent to the brain propagate unhealthy signals leading to inflammation, oxidative stress, unbalanced homeostasis of energy and increased cellular degeneration (Noble et al., 2017). Oxidative stress and neuroinflammation, in turn, are two major systemic conditions that aggravate neurodegeneration. The alterations occurred in the gut microbiota affect many aspects of the hippocampus through the vagus nerve pathway, the systemic pathway to increase the permeability of mucosa-intestinal barrier and blood-brain barrier and finally regulate hippocampus-dependent cognition and behaviors (Tang et al., 2021).
The composition and diversity of intestinal bacteria, the neuroactive substances, proinflammatory and inflammatory factors, and the levels of some gut microbiota metabolites such as lipopolysaccharide (LPS), trimethylamine N-oxide (TMAO) and amyloid beta (Aβ) in the gut microbiota could be affected by many treatments including antibiotics, germ free administration, diet, probiotics, prebiotics, and fecal microbiota transplantation (Tang et al., 2021). Probiotics, defined as live microorganisms with capability of promoting health to human and animal hosts when administered adequately and continuously (Sherman et al., 2009). The prominent commonly used probiotics to target the central nervous system (CNS) functions are mostly composed of two lactic acid producing bacteria genera including Lactobacilli and Bifidobacteria which are also normally found in healthy gut (Bodera and Chcialowski, 2009; Azad et al., 2018). Hence, it is not surprising that probiotics are considered to be used to repair damaged gut microbiota (Kwok et al., 2014). Probiotics influence dysfunction of the CNS in neurological disorders by increasing both diversity and count of the gut bacteria (Kwok et al., 2014). Evidence from animal research indicates that probiotic administration affects behavior, neurobiochemistry, and histology of hippocampus (Liu et al., 2015; Ho et al., 2019; Rahmati et al., 2019). This systematic review was mainly aimed to explore a link between the gut microbiota with behavioral, electrophysiological, biochemical and histological aspects of the hippocampus. The favorable role of probiotics on the above-mentioned aspects of hippocampus is also considered.
2 Methods
2.1 Search strategy
We used a broad search string including “hippocampus AND gut microbiota” or “hippocampus AND probiotics.” All papers published until end of 2021 were considered in Web of Science and PubMed. Additional papers were obtained from references of identified papers. Registries for clinical trials were checked.
2.2 Study selection
Preferred Reporting Items for Systematic Reviews and Meta-Analyses (PRISMA) course of action were used in this study (Figure 1). The main criteria for selecting papers were experimental studies that correlate the gut microbiota and/or probiotics to one of the following aspects: learning and memory, synaptic plasticity, LTP, neurotransmitter and neuromodulator alterations in the hippocampus, histological changes in the hippocampus, response of the hippocampus to changes in the plasma and cerebrospinal fluid levels of biochemical factors such as proinflammatory or inflammatory factors, cytokines, oxidants and antioxidants. Our database search yielded articles. All articles pertaining to the comprehensive, systematic and minireviews, non-original studies such as book reviews, letters to editor and editorials were excluded in this review; except the contents for preliminary studies and discussion, when necessary. The titles and abstracts of all articles were screened and selected further review if met the inclusion criteria. In total, full-text articles were assessed for eligibility and 139 met the above-mentioned criteria for review.
2.3 Quality assessment
First-grade studies were those that directly link hippocampus to the gut microbiota and probiotics. The second-grade studies were those working on the factors from the others organs (e.g., gastrointestinal tract) or other parts of the CNS that affect the hippocampus.
3 Results
The reviewed studies were those focused on relationship of the gut microbiota and probiotic with the hippocampus. The findings consider hippocampus-dependent cognitive function, level of brain derived nerve factor (BDNF) in the hippocampus, the hippocampus synaptic plasticity, the histological changes in the hippocampus and the balance of anti-oxidant/oxidant and anti-inflammatory/inflammatory factors. Table 1 briefly describe characteristics of the studies included in the systematic review. Also, details of the reviewed papers are given in the Supplementary Table 1.
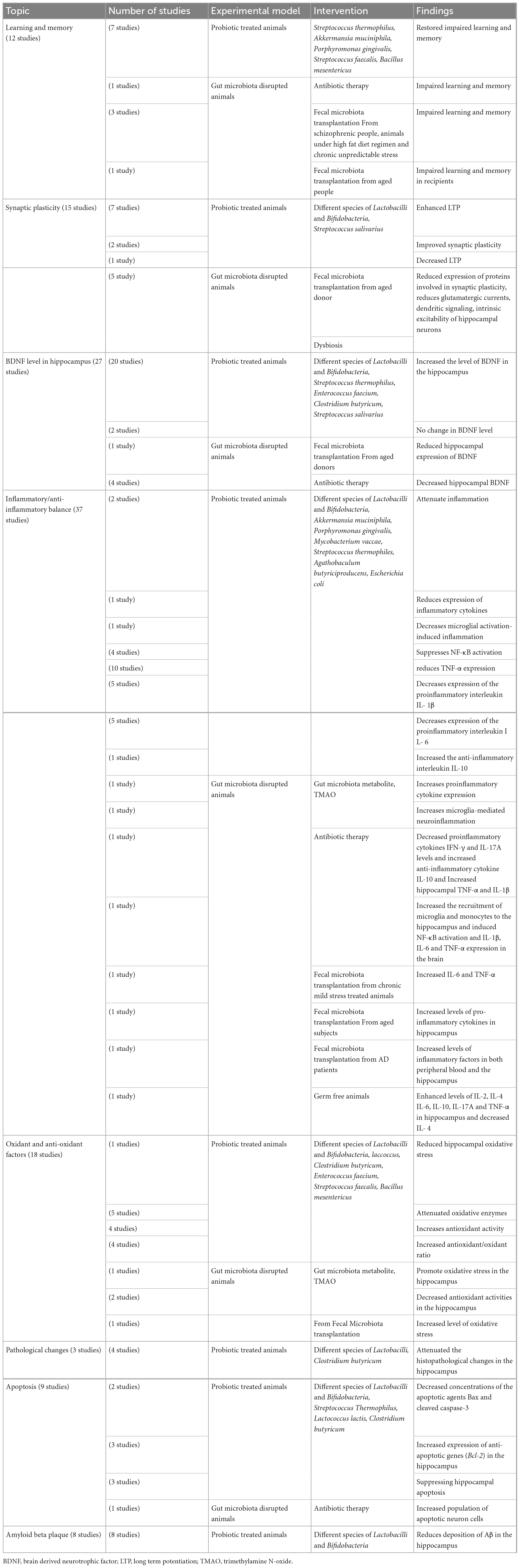
Table 1. Brief description of studies indicating a link between the gut microbiota and the hippocampus.
3.1 Learning and memory
Twelve studies have explored a link between hippocampus-dependent learning and memory with the disrupted gut microbiota and the helpful role of probiotic supplements. Results of one study showed that damage to the gut microbiota leads to impaired learning and memory (Zeraati et al., 2019). Seven studies have considered the effect of probiotics on hippocampus-dependent learning and memory in the animal models. Lactobacilli and Bifidobacteria were the most probiotic bacteria used in these studies. Streptococcus thermophilus, Akkermansia muciniphila, Porphyromonas gingivalis, Streptococcus faecalis, and Bacillus mesentericus were the other administered probiotics. In all cases the probiotic therapy improved the impaired learning and memory (Zhang et al., 2018; Ho et al., 2019; McVey Neufeld et al., 2019; Rahmati et al., 2019; Liu et al., 2020; Roy Sarkar et al., 2020; Cui et al., 2021; Zolfaghari et al., 2021). Three studies reported that fecal microbiota transplantation from schizophrenic people (Zhu et al., 2020), high fat diet (Yang et al., 2019) and chronic unpredictable stress (Shan et al., 2020) gave rise to impaired learning and memory. One study indicated that TMAO may affect social behaviors by regulating metabolites in the hippocampus (Luo et al., 2021). One study confirmed positive role of gut microbial metabolite short-chain fatty acids (SCFAs) in cognitive deficits (Xu et al., 2021). In one clinical trial, fecal microbiota transplantation from aged donors weaken spatial learning and memory in young adult recipients (D’Amato et al., 2020).
3.2 Synaptic plasticity
Hippocampal synaptic plasticity following probiotic therapy and fecal microbiota transplantation was evaluated in 15 studies. Different species of Lactobacilli and Bifidobacteria, and Streptococcus salivarius were used as probiotic bacteria. The probiotic administration enhanced LTP in 6 studies (Davari et al., 2013; Distrutti et al., 2014; Asl et al., 2019; Rezaei Asl et al., 2019; Rezaeiasl et al., 2019; Talani et al., 2020). Two studies reported improving effect of probiotics on the synaptic plasticity (Luck et al., 2020; Wang et al., 2020a). One study showed striking alteration of hippocampal LTP in male germ-free slices (Darch et al., 2021). On the other hand, one study reported decreased hippocampal LTP following probiotic administration (Tahmasebi et al., 2020). Fecal microbiota transplantation from aged donor mice reduced expression of proteins involved in synaptic plasticity (D’Amato et al., 2020).
Evidence indicates that while dysbiosis alters neuronal functions, reduces spontaneous postsynaptic glutamatergic currents (Cordella et al., 2021), alters integration of dendritic signaling (Darch et al., 2021) and decreases intrinsic excitability in hippocampal neurons (Kim et al., 2022) probiotic treatment selectively increases the intrinsic excitability of hippocampal CA1 pyramidal neurons (Kim et al., 2022).
3.3 BDNF level in the hippocampus
Twenty-seven studies investigated relevancy of the BDNF concentration in the hippocampus with the altered gut flora and the probiotic treatments. The main administered probiotics were Lactobacilli and Bifidobacteria; however, other probiotics such as Streptococcus thermophilus, Enterococcus faecium, Clostridium butyricum, Streptococcus salivarius, and Prevotella histicola were also used. In most of the studies the probiotic treatment increased the level of BDNF in the hippocampus (Distrutti et al., 2014; Liang et al., 2015; Liu et al., 2015; Corpuz et al., 2018; Jang et al., 2018a,2019a,2019b; Romo-Araiza et al., 2018; Lee et al., 2019; Sovijit et al., 2019; Tsukahara et al., 2019; Han et al., 2020a,b; Kim et al., 2020; Mohammed et al., 2020; Talani et al., 2020; Yang et al., 2020; Yun et al., 2020; Zhang et al., 2020). However, in two studies the probiotic treatments could not change in the level of BDNF (O’Sullivan et al., 2011; Rahmati et al., 2019). The probiotic treatment also elevated the BDNF expression in cultured hippocampal neurons (Choi et al., 2019). Fecal microbiota transplantation from the aged donors reduced the hippocampal expression of BDNF in one study (Li et al., 2020). Using a mixture of antibiotics, four studies induced gut microbiota depletion. The results showed that hippocampal BDNF was significantly decreased in these animals (Olson et al., 2018; Bistoletti et al., 2019; Zeraati et al., 2019; Lee et al., 2020).
3.4 Histopathological considerations
The effect of probiotic supplementations on histopathological changes of the hippocampus was assessed in animal models. Lactobacilli species and Clostridium butyricum were used as probiotics. Results showed that the probiotic treatments attenuated the histopathological changes in the hippocampus (Liu et al., 2015; Sun et al., 2016; Mohammed et al., 2020).
3.5 Apoptosis
Nine studies have focused on apoptosis. Beside the main bacteria Lactobacilli and Bifidobacteria, the other administered probiotic bacteria were Streptococcus thermophilus, Lactococcus lactis, and Clostridium butyricum. All studies reported positive effect of the probiotics in prevention of apoptosis in the hippocampus. Two studies reported decreased concentrations of the apoptotic agents Bax and cleaved caspase-3 (Sun et al., 2016; Xu et al., 2018). Also, 3 studies showed increased expression of anti-apoptotic genes (Bcl-2) in the hippocampus (Liu et al., 2015; Sun et al., 2016; Xin et al., 2020). The results of two studies showed prevention of the hippocampal apoptosis (Mohammadi et al., 2019; Rahmati et al., 2019). One study reported the protective effects of probiotics on mitochondrion-mediated apoptosis in the hippocampus (Wang et al., 2020a). Conversely, fecal microbiota transplantation from the antibiotic-induced microbiota disrupted mice resulted resulted in increased population of apoptotic neuron cells (Jang et al., 2018a,b).
3.6 Amyloid beta plaques
Eight studies assessed if probiotic supplementation underlies Aβ deposition in the hippocampus. Different species of Lactobacilli and Bifidobacteria were used as probiotics. All studies found that the probiotic treatment reduces deposition of Aβ in the hippocampus (Athari Nik Azm et al., 2018; Abraham et al., 2019; Lee et al., 2019; Liu et al., 2020; Mehrabadi and Sadr, 2020; Wang et al., 2020b; Wu et al., 2020; Go et al., 2021).
3.7 Oxidant and anti-oxidant factors
Eighteen studies have searched the effect of probiotic therapy on the oxidants/antioxidants factors in the hippocampus. The administered probiotics were Lactobacilli, Bifidobacteria, Lactococcus lactis, Clostridium butyricum, Enterococcus faecium, Streptococcus faecalis, and Bacillus mesentericus. One study showed that probiotic treatment reduced the hippocampal oxidative stress (Chunchai et al., 2018). Five studies reported that probiotic supplementations attenuated oxidative enzymes (Sun et al., 2016; Alchujyan et al., 2018; Xu et al., 2018; Ramalho et al., 2019; Salami et al., 2019). Consistently, 4 studies showed that probiotic administration increases antioxidant activity (Divyashri et al., 2015; Huang et al., 2018; Ho et al., 2019; Roy Sarkar et al., 2020). Four studies reported an increased antioxidant/oxidant ratio in the probiotic treated animals (Sun et al., 2016; Xu et al., 2018; Rezaeiasl et al., 2019; Mehrabadi and Sadr, 2020). Gut microbiota-derived metabolite TMAO promotes oxidative stress and downregulates the antioxidant methionine sulfoxide reductase A (MsrA) enzyme in the hippocampus, which may sensitize the hippocampus to oxidative stress. In two studies TMAO decreased antioxidant activities in the hippocampus (Meng et al., 2019; Zhao et al., 2019). In one study age-related fecal microbiota transplantation increased level of oxidative stress in young rats (Li et al., 2020). In a study fecal microbiota transplantation elevated SOD and CAT activities and GSH/GSSG ratio and diminished ROS, GSSG, and MDA levels in the hippocampus after traumatic brain injury (Du et al., 2021).
3.8 Inflammatory and anti-inflammatory factors
Fifty-three researches found a relation between gut microbiota and probiotics with Inflammatory and anti-inflammatory factors in the hippocampus. Of them, thirty-seven studies tested inflammatory responses of hippocampus to probiotic administration. Again, Lactobacilli and Bifidobacteria were the main administered probiotics and the other bacteria were Akkermansia muciniphila, Porphyromonas gingivalis, Mycobacterium vaccae, Streptococcus thermophiles, Agathobaculum butyriciproducens, Escherichia coli, and Prevotella histicola.
Probiotic administrations are reported to attenuate inflammation (Ni et al., 2019; Wang et al., 2020b), reduce expression of inflammatory cytokines (Guo et al., 2019) and decreases microglial activation-induced inflammation (Chunchai et al., 2018). Evidence indicates that probiotics increase anti-inflammatory cytokines and reduce inflammatory cytokines. Probiotic administration suppresses NF-κB activation (Jeong et al., 2016; Olson et al., 2018; Lee et al., 2019; Han et al., 2020a). Also, probiotic therapy reduces the expression of proinflammatory cytokine TNF-α (Sousa et al., 2008; Jeong et al., 2016; Lee et al., 2019; Lin et al., 2019; Yang et al., 2019; Han et al., 2020a,b; Mehrabadi and Sadr, 2020; Wu et al., 2020; Zolfaghari et al., 2021). Probiotics also decrease expression of the proinflammatory IL-1β (Jeong et al., 2016; Lin et al., 2019; Yang et al., 2019; Mehrabadi and Sadr, 2020; Wu et al., 2020), IL-6 (Abildgaard et al., 2017; Yang et al., 2019; Han et al., 2020a,b; Wu et al., 2020; Xiao et al., 2020) and IFN-γ (Ma et al., 2021). On the other hand, it is reported that probiotic supplementation increased the anti-inflammatory IL-10 (Wang et al., 2020a).
Two studies proved that TMAO increases expression of proinflammatory cytokines (Zhao et al., 2019) and reactive oxygen species (ROS) microglia-mediated neuroinflammation (Meng et al., 2019). One study showed that antibiotic-induced gut microbial dysbiosis after traumatic brain injury increased neuroinflammation (Celorrio et al., 2021). In another study it was found that antibiotic-induced microbiota depletion decreases the proinflammatory cytokines IFN-γ and IL-17A levels and increases the anti-inflammatory cytokine IL-10. It also increased hippocampal TNF-α and IL-1β in EAE-induced mice (Zeraati et al., 2019). Four studies applied the fecal microbiota transplantation technique. In one study fecal microbiota transplantation of mice treated with ampicillin increased the recruitment of microglia and monocytes to the hippocampus. It also induced NF-κB activation and expression of IL-1β, IL-6, and TNF-α in the brain (Jang et al., 2018a). Another study showed that fecal microbiota transplantation from chronic mild stress treated animals increased IL-6 and TNF-α (Marcondes Ávila et al., 2020). Age-related fecal microbiota transplantation increased levels of pro-inflammatory cytokines in hippocampus (Li et al., 2020). Fecal microbiota transplantation from the patients with Alzheimer’s disease increased levels of inflammatory factors in both peripheral blood and the hippocampus (Shen et al., 2020). Studies on the germ free animals, the animals deprived from natural gut flora, showed enhanced levels of IL-2, IL-4, IL-6, IL-10, IL-17A, and TNF-α (Olson et al., 2018; de Miranda et al., 2020) and decreased level of IL-4 (Wu et al., 2020) in the hippocampus. Figure 2 illustrate the relationship of the gut microbiota, under eubiosis and dysbiosis conditions, and probiotic bacteria with the hippocampal related cognitive functions and synaptic plasticity.
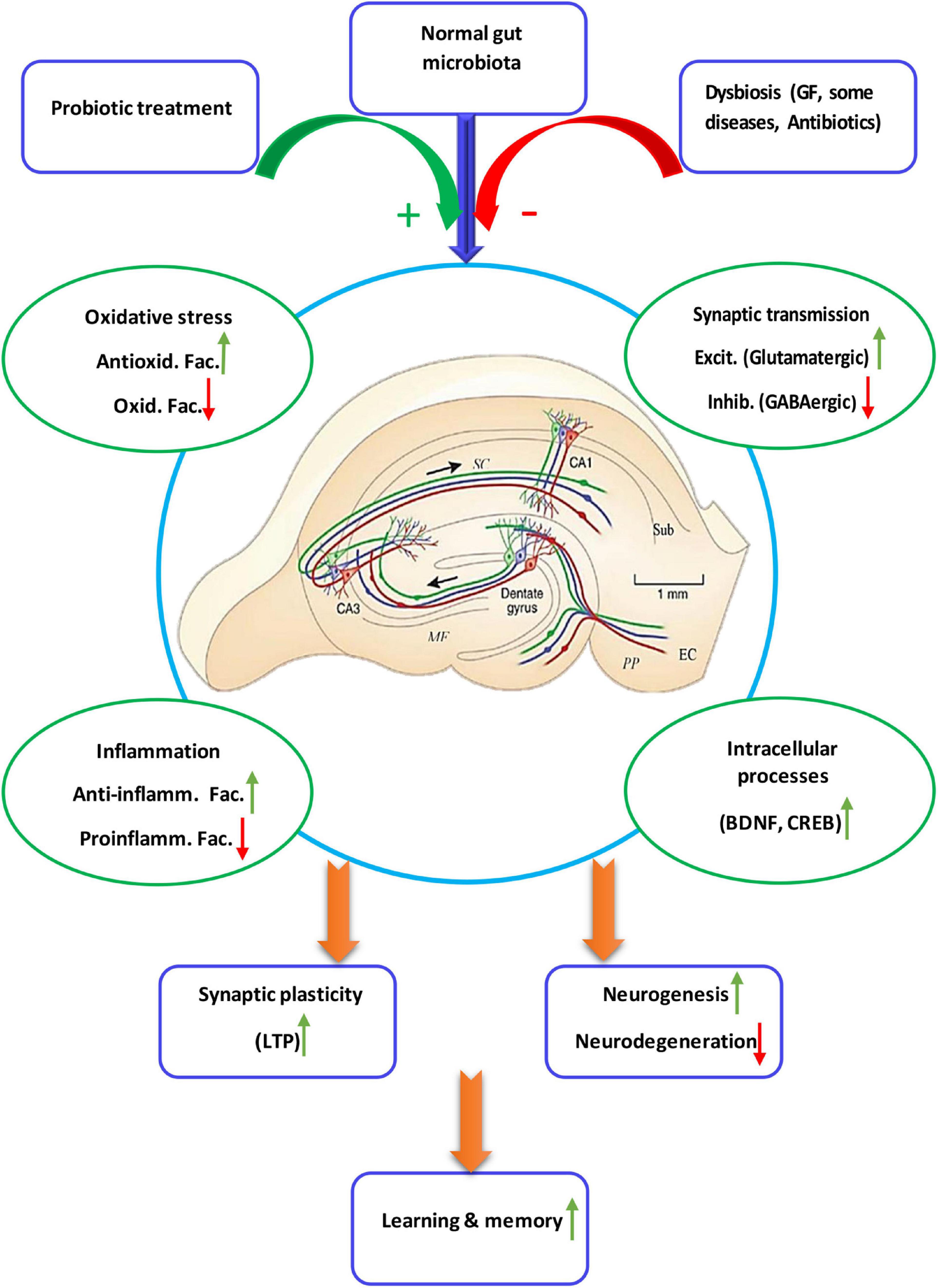
Figure 2. Schematic diagram showing details of the microbiota-gut-hippocampus axis at different levels. The normal gut microbiota enhances synaptic plasticity and, thus, hippocampal-dependent learning and memory, through a balance of anti-inflammatory/inflammatory and antioxidant/oxidant agents, stimulatory/inhibitory synaptic transmission, as well as enhancement of intracellular devices. While intestinal microbiota negatively affect biochemistry, immunology, neurochemistry and intracellular cascades of synaptic plasticity and cognition, probiotic treatment restores dysbiotic hippocampal disorders. Anti-inflamm. Fac., anti-inflammatory factors; Proinflamm. Fac., proinflammatory factors; Antioxid. Fac., antioxidant factors, Oxid. Fac., oxidant factors; Excit, excitatory, Inhib., inhibitory.
4 Discussion
Majority of the reviewed papers studying either the normal or disrupted gut microbiota indicates a strong link between the hippocampus-dependent learning and memory and synaptic plasticity with the intestinal flora. Growing proofs indicating considerable role of beneficial bacteria from either the gut microbiota or probiotics in the axis mainly comes from the studies carried out in the disrupted gut microbiota and germ free subjects. It has been shown that the hippocampus-related behaviors such as anxiety, depression, and cognitive function are abnormal in germ free animals. Diaz Heijtz et al. (2011), found that 50 genes in the hippocampus of germ free animals are significantly different (higher or lower) expressed in compared with those with normal gut microbiota. On the other hand, supporting the damaged gut microbiota by probiotic supplements restores its normal function. Also, fecal microbiota transplantation technique confirms necessity of a healthy gut microbiota for normal hippocampal dependent phenomena.
The hippocampal dependent cognitive function is mechanistically contributed to occurrence of an important form of synaptic plasticity, LTP. The hippocampal LTP is essential for the coding and storage of long term spatial memories so that prevention of LTP induction in the hippocampus affects the spatial learning (Bannerman et al., 2014). Therefore, having a cross talk between the LTP and the learning and memory, all factors affecting the LTP deeply influence the cognitive behaviors. A key regulator of the LTP itself is the neurotrophin BDNF (Monteggia et al., 2004). The BDNF is known to be associated with generating and preserving LTP in the CA1 region of the hippocampus (Diógenes et al., 2011; Katche et al., 2013) and therefore it plays an important role in the cognitive function (Pang and Lu, 2004). The cognitive impairments are reported to be partly mediated by altered BDNF expression in the hippocampus (Frohlich et al., 2016). Except a few, most of the reviewed studies demonstrated that the gut microbiota disruption reduced the hippocampal expression of BDNF. Literature reviewed here shows that probiotic treatment strengthens the suppressed LTP in the CA3-CA1 pathway of the hippocampus. Therefore, modulation of the BDNF by the gut microbiota and probiotic bacteria partly explains its role in regulation of synaptic transmission and, thus, cognitive function of the hippocampus.
The LTP in the CA3-CA1 pathway of the hippocampus is NMDA receptor-dependent. Altered expression of some neurotransmitters or their receptors is observed in the animals with absent or disrupted gut microbiota. The NMDA receptor level in the hippocampus is decreased in the damaged gut microbiota (Wang et al., 2015) and the germ free animals (Neufeld et al., 2011). Microbiome fecal transplants from patients with schizophrenia heads to lower glutamate and higher glutamine and GABA in the hippocampus (Zheng et al., 2019). Bravo et al. observed enhanced memory consolidation, with decreased hippocampal GABAB1b mRNA; suggesting a potential mechanism by which cognitive behavior may be modulated (Athari Nik Azm et al., 2018). Also, absence of gut microbiota significantly upregulates the glucocorticoid receptor pathway genes in hippocampus (Cristiano et al., 2018). From these considerations it can be concluded that a mechanism by which the helpful bacteria may affect the hippocampus-dependent cognitive function is influencing neurotrophins, neurotransmitters, and receptors.
Unexpected to many other areas of brain, neurogenesis occurs in the adult hippocampus and it is believed that this process importantly influences learning and memory (Marín-Burgin and Schinder, 2012). It is reported that there is a connection between the gut microbiota composition and their metabolic function to neurogenesis in the adult hippocampus (Wei et al., 2021) so that antibiotic-induced gut microbial dysbiosis can increase hippocampal neuronal loss (Celorrio et al., 2021).
It is reported that a path by which microbiota is able to affect the CNS functions is the change of hippocampal neurogenesis in adults (Kempermann et al., 2015). Consistently, numerous findings suggest that some brain processes which are regulated by neurogenesis in adult hippocampus are affected in germ free animals (Gareau et al., 2011). These evidences indicate that, through histological support, the gut microbiota can underlie the hippocampal function.
Another mechanism by which the gut microbiota impacts the hippocampus is its important role in modulating microRNAs (miRNAs); the small, non-coding RNAs which emerge as key post-transcriptional modulators of gene expression (Cui et al., 2014). They influence hippocampal gene expression (Moloney et al., 2017) and, thus, considerably affect the processes critical to hippocampal development (Moloney et al., 2017). Inhibition of miRNAs in the hippocampus results in changes in cognitive behavior in rodents (Malmevik et al., 2016) and it is suggested that many of the behavioral effects mediated by the gut microbiota are controlled by miRNAs (Moloney et al., 2017). Studies also show that the cyclic-adenosine-monophosphate response element binding (CREB) in hippocampal neurons is regulated by the gut microbiota. It is shown that absence of the gut microbiota from birth is associated with decreased hippocampal CREB (Zeng et al., 2016). The CREB regulates the genes related to neuronal differentiation, synaptic plasticity, and learning and memory (Murray et al., 2019). Therefore, gene expression could be an intracellular path through which the gut microbiota regulates hippocampus-related synaptic plasticity and cognition.
This review demonstrates that the intestinal bacteria has a powerful influence on the neuroinflammation so as to the gut microbiota has been proved to be an important neuroimmunomodulator (Foster, 2016; Rea et al., 2016). Evidence indicate that any damage to the gut microbiota increases the inflammatory factors in the hippocampus. It is demonstrated that there is a specific inflammatory pattern, in dorsal and ventral hippocampus, that is significantly correlated with gut microbiota composition (Lopizzo et al., 2021). Gut dysbiosis is shown to contribute to both peripheral and central inflammatory processes and cognitive deficits (Wang et al., 2021).
Neuroinflammation is associated with the cognitive impairment and memory decline, as the hippocampus is prone to develop alterations in synaptic transmission and plasticity during inflammation (Di Filippo et al., 2013). Whereas secretion of pro-inflammatory cytokines may be inhibited by BDNF, neuroinflammation, itself, is shown to negatively affect the hippocampal expression of BDNF (Mora, 2013; Moreno-Fernández et al., 2013; Ryan and Nolan, 2016). One of the biological changes related to inflammation is the activity of cytokines; the proteins that regulate inflammation (Scheller et al., 2011). Cytokines can modulate the neurogenesis, the synapse formation and plasticity (Hodes et al., 2015), influence the activity of neurons, astrocytes and microglia (see below) in the CNS (Kohman and Rhodes, 2013) and, thus, impact the cognition and mood (Valkanova et al., 2013; Khandaker et al., 2014). Especially, the microglia are the resident immune cells in the brain and has been causally linked to developmental neuronal cell death (Marín-Teva et al., 2011). These immune cells respond to perturbations by initiating an immune response involving the release of proinflammatory cytokines and actively promote developmental cell death in the hippocampus and cerebellum (Wakselman et al., 2008; Marín-Teva et al., 2011). On the other hand, the papers reviewed here revealed that probiotic administrations reduce inflammation through attenuating expression of the inflammatory cytokines and the microglial induced inflammation. Further, the probiotics positively affect production of the anti-inflammatory factors. Also, evidence from oxidative and antioxidative activities proves that the probiotic bacteria increases the antioxidative activities in cost of suppressing the oxidative activities. Conversely, some gut microbiota-derived metabolites (discussed below) as well as fecal microbiota transplantation from aged people proceed the oxidative stress in the hippocampus of recipient subjects. Overall, the gut and probiotic bacteria auspiciously balance inflammation and oxidation; in favor of anti-inflammatory and antioxidant agents.
The intestinal microbiota is responsible for a significant production of SCFA (Romo-Araiza et al., 2018) and the probiotics are known to highly influence production of the SCFAs. Production of butyrate, the most important SCFA, by healthy gut microbiota can facilitate LTP and the formation of long term memory (Levenson et al., 2004). It is reported that the probiotics can improve learning and memory through an increase in the butyrate that, in turn, increases BDNF and decreases the proinflammatory cytokine concentrations in the hippocampus (Romo-Araiza et al., 2018). In addition, the expression of enzymes involved in the production of antioxidant enzymes can be triggered by butyrate production (Hamer et al., 2009; Aguilar et al., 2016; Bourassa et al., 2016; Slattery et al., 2016). The other SCFA propionate is known to up-regulate the protein CREB that, in turn, regulates synaptic plasticity, memory formation, and the reward system (Slattery et al., 2016).
Despite aforementioned valuable roles of the gut microbiota, it may play injurious role under adverse conditions of the gut flora. TMAO, a gut microbiota metabolite, increases proinflammatory cytokine expression and microglia-mediated neuroinflammation. Also, structural bacterial components such as LPS provide low grade tonic stimulation of the innate immune system. Higher abundance of LPS is detectable in hippocampus of the people with Alzheimer’s disease (Zhao et al., 2017). Dysfunctional responses of the adaptive immune system to some bacterial proteins and production of some neurotoxic metabolites such as D-lactic acid and ammonia are the other examples. Even helpful metabolites such as SCFAs may have neurotoxic effects (Galland, 2014).
5 Future implications and limitations
Current research shows a close relationship between several brain disorders and changes in the gut microbiota (Salami, 2021); promising favorable role of bacteria in diagnosis and/or treatment of the brain diseases. In particular, from a therapeutic point of view, it can be hypothesized whether fecal microbiota transplantation or the administration of probiotics can improve hippocampal-dependent learning and memory.
Because this systematic review was devoted to the studies directly related to the hippocampus, very little data was available from human research and clinical trials, and so, at least in terms of cognitive function, we are just getting started.
Therefore, human studies are encouraged to investigate the potential effects of the gut microbiota and probiotics on the hippocampus-related behaviors, particularly in brain diseases associated with cognitive disorders. Since majority of the papers considering relevancy of the hippocampus with the gut and probiotic bacteria have been published in the last 3 years, it promises a future with intensive research in this area.
6 Conclusion
Overall, the production and modulation of neurotrophins, neurotransmitters and receptors, regulation of intracellular molecular processes, balance of inflammatory/anti-inflammatory and oxidative/antioxidant factors, and preservation of structural aspects of the hippocampus can be considered as important ways through them the gut microbiota affect the synaptic activity in the hippocampal neural circuits and hippocampus related behaviors. The supportive role of the probiotic bacteria, whether as supplementation for health or as therapeutic tool, is appreciated. However, the degree of efficacy of probiotics, optimal application paths, sex specificity, ages with the best most vulnerability to probiotics and the duration of treatment should be considered.
Data availability statement
The original contributions presented in this study are included in the article/Supplementary material, further inquiries can be directed to the corresponding author.
Author contributions
MSa was the project leader of the review, designed the review, tabulated the review results, interpreted and discussed review findings, and did the screening, data abstraction, and quality appraisal. MSo conceived the review and prepared the tables and drawings. Both authors managed the review processes, summarized and described the review findings, and prepared the final version.
Conflict of interest
The authors declare that the research was conducted in the absence of any commercial or financial relationships that could be construed as a potential conflict of interest.
Publisher’s note
All claims expressed in this article are solely those of the authors and do not necessarily represent those of their affiliated organizations, or those of the publisher, the editors and the reviewers. Any product that may be evaluated in this article, or claim that may be made by its manufacturer, is not guaranteed or endorsed by the publisher.
Supplementary material
The Supplementary Material for this article can be found online at: https://www.frontiersin.org/articles/10.3389/fnins.2022.1065995/full#supplementary-material
References
Abildgaard, A., Elfving, B., Hokland, M., Wegener, G., and Lund, S. (2017). Probiotic treatment reduces depressive-like behaviour in rats independently of diet. Psychoneuroendocrinology 79, 40–48.x doi: 10.1016/j.psyneuen.2017.02.014
Abraham, D., Feher, J., Scuderi, G. L., Szabo, D., Dobolyi, A., Cservenak, M., et al. (2019). Exercise and probiotics attenuate the development of Alzheimer’s disease in transgenic mice: Role of microbiome. Exp. Gerontol. 115, 122–131. doi: 10.1016/j.exger.2018.12.005
Aguilar, E. C., Santos, L. C., Leonel, A. J., de Oliveira, J. S., Santos, E. A., Navia-Pelaez, J. M., et al. (2016). Oral butyrate reduces oxidative stress in atherosclerotic lesion sites by a mechanism involving NADPH oxidase down-regulation in endothelial cells. J. Nutr. Biochem. 34, 99–105. doi: 10.1016/j.jnutbio.2016.05.002
Alchujyan, N., Hovhannisyan, M., Movsesyan, N., Madoyan, R., Sargsyan, H., Aghababova, A., et al. (2018). Free and zeolite-immobilized probiotic mixture versus sodium valproate in prevention of oxidative stress and modulation of the L-Arginine intracellular metabolic pathways in the rat brain and blood following dexamphetamine-induced bipolar D. Eur. Chem. Bull. 7:42. doi: 10.17628/ecb.2018.7.42-51
Asl, Z. R., Sepehri, G., and Salami, M. (2019). Probiotic treatment improves the impaired spatial cognitive performance and restores synaptic plasticity in an animal model of Alzheimer’s disease. Behav. Brain Res. 376:112183.
Athari Nik Azm, S., Djazayeri, A., Safa, M., Azami, K., Ahmadvand, B., Sabbaghziarani, F., et al. (2018). Lactobacilli and bifidobacteria ameliorate memory and learning deficits and oxidative stress in beta-amyloid (1-42) injected rats. Appl. Physiol. Nutr. Metab. 43, 718–726. doi: 10.1139/apnm-2017-0648
Azad, M. A. K., Sarker, M., and Li, T. (2018). Probiotic species in the modulation of gut microbiota: An overview. Biomed. Res. Int. 2018:9478630. doi: 10.1155/2018/9478630
Bäckhed, F. (2011). Programming of host metabolism by the gut microbiota. Ann. Nutr. Metab. 58(Suppl. 2), 44–52. doi: 10.1159/000328042
Bannerman, D. M., Sprengel, R., Sanderson, D. J., McHugh, S. B., Rawlins, J. N., Monyer, H., et al. (2014). Hippocampal synaptic plasticity, spatial memory and anxiety. Nat. Rev. Neurosci. 15, 181–192. doi: 10.1038/nrn3677
Bistoletti, M., Caputi, V., Baranzini, N., Marchesi, N., Filpa, V., Marsilio, I., et al. (2019). Antibiotic treatment-induced dysbiosis differently affects BDNF and TrkB expression in the brain and in the gut of juvenile mice. PLoS One 14:e0212856. doi: 10.1371/journal.pone.0212856
Bodera, P., and Chcialowski, A. (2009). Immunomodulatory effect of probiotic bacteria. Recent Pat. Inflamm. Allergy Drug Discov. 3, 58–64.
Bourassa, M. W., Alim, I., Bultman, S. J., and Ratan, R. R. (2016). Butyrate, neuroepigenetics and the gut microbiome: Can a high fiber diet improve brain health? Neurosci. Lett. 625, 56–63. doi: 10.1016/j.neulet.2016.02.009
Celorrio, M., Abellanas, M. A., Rhodes, J., Goodwin, V., Moritz, J., Vadivelu, S., et al. (2021). Gut microbial dysbiosis after traumatic brain injury modulates the immune response and impairs neurogenesis. Acta Neuropathol. Commun. 9:40. doi: 10.1186/s40478-021-01137-2
Choi, J., Kim, Y. K., and Han, P. L. (2019). Extracellular vesicles derived from lactobacillus plantarum increase BDNF expression in cultured hippocampal neurons and produce antidepressant-like effects in mice. Exp. Neurobiol. 28, 158–171. doi: 10.5607/en.2019.28.2.158
Chunchai, T., Thunapong, W., Yasom, S., Wanchai, K., Eaimworawuthikul, S., Metzler, G., et al. (2018). Decreased microglial activation through gut-brain axis by prebiotics, probiotics, or synbiotics effectively restored cognitive function in obese-insulin resistant rats. J. Neuroinflammation 15:11. doi: 10.1186/s12974-018-1055-2
Clarke, G., Grenham, S., Scully, P., Fitzgerald, P., Moloney, R. D., Shanahan, F., et al. (2013). The microbiome-gut-brain axis during early life regulates the hippocampal serotonergic system in a sex-dependent manner. Mol. Psychiatry 18, 666–673. doi: 10.1038/mp.2012.77
Cordella, F., Sanchini, C., Rosito, M., Ferrucci, L., Pediconi, N., Cortese, B., et al. (2021). Antibiotics treatment modulates microglia-synapses interaction. Cells 10:2648. doi: 10.3390/cells10102648
Corpuz, H. M., Ichikawa, S., Arimura, M., Mihara, T., Kumagai, T., Mitani, T., et al. (2018). Long-term diet supplementation with lactobacillus paracasei K71 prevents age-related cognitive decline in senescence-accelerated mouse prone 8. Nutrients 10:762. doi: 10.3390/nu10060762
Cristiano, C., Pirozzi, C., Coretti, L., Cavaliere, G., Lama, A., Russo, R., et al. (2018). Palmitoylethanolamide counteracts autistic-like behaviours in BTBR T+tf/J mice: Contribution of central and peripheral mechanisms. Brain Behav. Immun. 74, 166–175. doi: 10.1016/j.bbi.2018.09.003
Cryan, J. F., and O’Mahony, S. M. (2011). The microbiome-gut-brain axis: From bowel to behavior. Neurogastroenterol. Motil. 23, 187–192. doi: 10.1111/j.1365-2982.2010.01664.x
Cui, M., Wang, Y., Sun, B., Xiao, Z., Ye, L., and Zhang, X. (2014). MiR-205 modulates abnormal lipid metabolism of hepatoma cells via targeting acyl-CoA synthetase long-chain family member 1 (ACSL1) mRNA. Biochem. Biophys. Res. Commun. 444, 270–275. doi: 10.1016/j.bbrc.2014.01.051
Cui, X., Zhou, S., Xia, G., Chen, J., Jiang, L., Huang, J., et al. (2021). A multispecies probiotic accelerates fear extinction and inhibits relapse in mice: Role of microglia. Neuropharmacology 193:108613. doi: 10.1016/j.neuropharm.2021.108613
D’Amato, A., Di Cesare Mannelli, L., Lucarini, E., Man, A. L., Le Gall, G., Branca, J. J. V., et al. (2020). Faecal microbiota transplant from aged donor mice affects spatial learning and memory via modulating hippocampal synaptic plasticity- and neurotransmission-related proteins in young recipients. Microbiome 8:140. doi: 10.1186/s40168-020-00914-w
Darch, H. T., Collins, M. K., O’Riordan, K. J., and Cryan, J. F. (2021). Microbial memories: Sex-dependent impact of the gut microbiome on hippocampal plasticity. Eur. J. Neurosci. 54, 5235–5244. doi: 10.1111/ejn.15119
Davari, S., Talaei, S. A., Alaei, H., and Salami, M. (2013). Probiotics treatment improves diabetes-induced impairment of synaptic activity and cognitive function: behavioral and electrophysiological proofs for microbiome-gut-brain axis. Neuroscience 240, 287–296. doi: 10.1016/j.neuroscience.2013.02.055
de Miranda, A. S., Vieira, ÉL. M., dos Reis Bastos, J., Ferreira, R. N., Nicoli, J. R., Teixeira, M. M., et al. (2020). Role of gut microbiota in the GBR12909 model of mania-like behavior in mice. J. Neuroimmunol. 346:577292. doi: 10.1016/j.jneuroim.2020.577292
Di Filippo, M., Chiasserini, D., Gardoni, F., Viviani, B., Tozzi, A., Giampà, C., et al. (2013). Effects of central and peripheral inflammation on hippocampal synaptic plasticity. Neurobiol. Dis. 52, 229–236. doi: 10.1016/j.nbd.2012.12.009
Diaz Heijtz, R., Wang, S., Anuar, F., Qian, Y., Björkholm, B., Samuelsson, A., et al. (2011). Normal gut microbiota modulates brain development and behavior. Proc. Natl. Acad. Sci. U.S.A. 108, 3047–3052. doi: 10.1073/pnas.1010529108
Diógenes, M. J., Costenla, A. R., Lopes, L. V., Jerónimo-Santos, A., Sousa, V. C., Fontinha, B. M., et al. (2011). Enhancement of LTP in aged rats is dependent on endogenous BDNF. Neuropsychopharmacology 36, 1823–1836. doi: 10.1038/npp.2011.64
Distrutti, E., O’Reilly, J. A., McDonald, C., Cipriani, S., Renga, B., Lynch, M. A., et al. (2014). Modulation of intestinal microbiota by the probiotic VSL#3 resets brain gene expression and ameliorates the age-related deficit in LTP. PLoS One 9:e106503. doi: 10.1371/journal.pone.0106503
Divyashri, G., Krishna, G., and Muralidhara Prapulla, S. G. (2015). Probiotic attributes, antioxidant, anti-inflammatory and neuromodulatory effects of Enterococcus faecium CFR 3003: In vitro and in vivo evidence. J. Med. Microbiol. 64, 1527–1540. doi: 10.1099/jmm.0.000184
Du, D., Tang, W., Zhou, C., Sun, X., Wei, Z., Zhong, J., et al. (2021). Fecal microbiota transplantation is a promising method to restore gut microbiota dysbiosis and relieve neurological deficits after traumatic brain injury. Oxid Med. Cell Longev. 2021:5816837. doi: 10.1155/2021/5816837
Eckburg, P. B., Bik, E. M., Bernstein, C. N., Purdom, E., Dethlefsen, L., Sargent, M., et al. (2005). Diversity of the human intestinal microbial flora. Science 308, 1635–1638. doi: 10.1126/science.1110591
Forsythe, P., and Kunze, W. A. (2013). Voices from within: gut microbes and the CNS. Cell. Mol. Life Sci. 70, 55–69. doi: 10.1007/s00018-012-1028-z
Foster, J. A. (2016). Gut microbiome and behavior: Focus on neuroimmune interactions. Int. Rev. Neurobiol. 131, 49–65. doi: 10.1016/bs.irn.2016.07.005
Frohlich, E. E., Farzi, A., Mayerhofer, R., Reichmann, F., Jacan, A., Wagner, B., et al. (2016). Cognitive impairment by antibiotic-induced gut dysbiosis: Analysis of gut microbiota-brain communication. Brain Behav. Immun. 56, 140–155. doi: 10.1016/j.bbi.2016.02.020
Galland, L. (2014). The gut microbiome and the brain. J. Med. Food 17, 1261–1272. doi: 10.1089/jmf.2014.7000
Gareau, M. G., Wine, E., Rodrigues, D. M., Cho, J. H., Whary, M. T., Philpott, D. J., et al. (2011). Bacterial infection causes stress-induced memory dysfunction in mice. Gut 60, 307–317. doi: 10.1136/gut.2009.202515
Go, J., Chang, D. H., Ryu, Y. K., Park, H. Y., Lee, I. B., Noh, J. R., et al. (2021). Human gut microbiota Agathobaculum butyriciproducens improves cognitive impairment in LPS-induced and APP/PS1 mouse models of Alzheimer’s disease. Nutr. Res. 86, 96–108. doi: 10.1016/j.nutres.2020.12.010
Grenham, S., Clarke, G., Cryan, J. F., and Dinan, T. G. (2011). Brain-gut-microbe communication in health and disease. Front. Physiol. 2:94. doi: 10.3389/fphys.2011.00094
Guo, Y., Xie, J. P., Deng, K., Li, X., Yuan, Y., Xuan, Q., et al. (2019). Prophylactic effects of bifidobacterium adolescentis on anxiety and depression-like phenotypes after chronic stress: A role of the gut microbiota-inflammation axis. Front. Behav. Neurosci. 13:126. doi: 10.3389/fnbeh.2019.00126
Hamer, H. M., Jonkers, D. M., Bast, A., Vanhoutvin, S. A., Fischer, M. A., Kodde, A., et al. (2009). Butyrate modulates oxidative stress in the colonic mucosa of healthy humans. Clin. Nutr. 28, 88–93. doi: 10.1016/j.clnu.2008.11.002
Han, S. K., Joo, M. K., Kim, J. K., Jeung, W., Kang, H., and Kim, D. H. (2020a). Bifidobacteria-fermented red ginseng and its constituents ginsenoside rd and protopanaxatriol alleviate anxiety/depression in mice by the amelioration of gut dysbiosis. Nutrients 12:901. doi: 10.3390/nu12040901
Han, S. K., Kim, J. K., Joo, M. K., Lee, K. E., Han, S. W., and Kim, D. H. (2020b). Lactobacillus reuteri NK33 and bifidobacterium adolescentis NK98 alleviate Escherichia coli-induced depression and gut dysbiosis in mice. J. Microbiol. Biotechnol. 30, 1222–1226. doi: 10.4014/jmb.2002.02058
Ho, S. T., Hsieh, Y. T., Wang, S. Y., and Chen, M. J. (2019). Improving effect of a probiotic mixture on memory and learning abilities in d-galactose-treated aging mice. J. Dairy Sci. 102, 1901–1909. doi: 10.3168/jds.2018-15811
Hodes, G. E., Kana, V., and Menard, C. (2015). Neuroimmune mechanisms of depression. Nat. Neurosci. 18, 1386–1393. doi: 10.1038/nn.4113
Huang, S. Y., Chen, L. H., Wang, M. F., Hsu, C. C., Chan, C. H., Li, J. X., et al. (2018). Lactobacillus paracasei PS23 delays progression of age-related cognitive decline in senescence accelerated mouse prone 8 (SAMP8) mice. Nutrients 10:894. doi: 10.3390/nu10070894
Jang, H. M., Han, S. K., Kim, J. K., Oh, S. J., Jang, H. B., and Kim, D. H. (2019a). Lactobacillus sakei alleviates high-fat-diet-induced obesity and anxiety in mice by inducing AMPK activation and SIRT1 expression and inhibiting gut microbiota-mediated NF-κB activation. Mol. Nutr. Food Res. 63:e1800978. doi: 10.1002/mnfr.201800978
Jang, H. M., Lee, K. E., and Kim, D. H. (2019b). The preventive and curative effects of Lactobacillus reuteri NK33 and Bifidobacterium adolescentis NK98 on immobilization stress-induced anxiety/depression and colitis in mice. Nutrients 11:819. doi: 10.3390/nu11040819
Jang, H. M., Lee, H. J., Jang, S. E., Han, M. J., and Kim, D. H. (2018a). Evidence for interplay among antibacterial-induced gut microbiota disturbance, neuro-inflammation, and anxiety in mice. Mucosal. Immunol. 11, 1386–1397. doi: 10.1038/s41385-018-0042-3
Jang, H. M., Lee, K. E., Lee, H. J., and Kim, D. H. (2018b). Immobilization stress- induced Escherichia coli causes anxiety by inducing NF-kappa B activation through gut microbiota disturbance. Sci. Rep. 8:13897. doi: 10.1038/s41598-018-31764-0
Jeong, J. J., Kim, K. A., Hwang, Y. J., Han, M. J., and Kim, D. H. (2016). Anti-inflammaging effects of Lactobacillus brevis OW38 in aged mice. Benef. Microbes. 7, 707–718. doi: 10.3920/bm2016.0016
Katche, C., Cammarota, M., and Medina, J. H. (2013). Molecular signatures and mechanisms of long-lasting memory consolidation and storage. Neurobiol. Learn. Mem. 106, 40–47. doi: 10.1016/j.nlm.2013.06.018
Kempermann, G., Song, H., and Gage, F. H. (2015). Neurogenesis in the adult hippocampus. Cold Spring Harb. Perspect. Biol. 7:a018812. doi: 10.1101/cshperspect.a018812
Khandaker, G. M., Pearson, R. M., Zammit, S., Lewis, G., and Jones, P. B. (2014). Association of serum interleukin 6 and C-reactive protein in childhood with depression and psychosis in young adult life: A population-based longitudinal study. JAMA Psychiatry 71, 1121–1128. doi: 10.1001/jamapsychiatry.2014.1332
Kim, J. K., Lee, K. E., Lee, S. A., Jang, H. M., and Kim, D. H. (2020). Interplay between human gut bacteria Escherichia coli and Lactobacillus mucosae in the occurrence of neuropsychiatric disorders in mice. Front. Immunol. 11:273. doi: 10.3389/fimmu.2020.00273
Kim, J., Kim, D. W., Lee, A., Mason, M., Jouroukhin, Y., Woo, H., et al. (2022). Homeostatic regulation of neuronal excitability by probiotics in male germ-free mice. J. Neurosci. Res. 100, 444–460. doi: 10.1002/jnr.24999
Kohman, R. A., and Rhodes, J. S. (2013). Neurogenesis, inflammation and behavior. Brain Behav. Immun. 27, 22–32. doi: 10.1016/j.bbi.2012.09.003
Kwok, L. Y., Wang, L., Zhang, J., Guo, Z., and Zhang, H. (2014). A pilot study on the effect of Lactobacillus casei Zhang on intestinal microbiota parameters in Chinese subjects of different age. Benef. Microb. 5, 295–304. doi: 10.3920/bm2013.0047
Lankelma, J. M., Nieuwdorp, M., de Vos, W. M., and Wiersinga, W. J. (2015). The gut microbiota in internal medicine: Implications for health and disease. Neth. J. Med. 73, 61–68.
Lee, H. J., Lee, K. E., Kim, J. K., and Kim, D. H. (2019). Suppression of gut dysbiosis by Bifidobacterium longum alleviates cognitive decline in 5XFAD transgenic and aged mice. Sci. Rep. 9:11814. doi: 10.1038/s41598-019-48342-7
Lee, K. E., Kim, J. K., Han, S. K., Lee, D. Y., Lee, H. J., Yim, S. V., et al. (2020). The extracellular vesicle of gut microbial Paenalcaligenes hominis is a risk factor for vagus nerve-mediated cognitive impairment. Microbiome 8:107. doi: 10.1186/s40168-020-00881-2
Levenson, J. M., O’Riordan, K. J., Brown, K. D., Trinh, M. A., Molfese, D. L., and Sweatt, J. D. (2004). Regulation of histone acetylation during memory formation in the hippocampus. J. Biol. Chem. 279, 40545–40559. doi: 10.1074/jbc.M402229200
Li, Y. L., Ning, L., Yin, Y. R., Wang, R., Zhang, Z. Y., Hao, L. J., et al. (2020). Age-related shifts in gut microbiota contribute to cognitive decline in aged rats. Aging US 12, 7801–7817. doi: 10.18632/aging.103093
Liang, S., Wang, T., Hu, X., Luo, J., Li, W., Wu, X., et al. (2015). Administration of Lactobacillus helveticus NS8 improves behavioral, cognitive, and biochemical aberrations caused by chronic restraint stress. Neuroscience 310, 561–577. doi: 10.1016/j.neuroscience.2015.09.033
Lin, T., Liu, X., Xiao, D., Zhang, D., Cai, Y., and Zhu, X. (2019). Lactobacillus spp. as probiotics for prevention and treatment of enteritis in the lined seahorse (Hippocampus erectus) juveniles. Aquaculture 503, 16–25. doi: 10.1016/j.aquaculture.2018.12.083
Liu, J. L., Yu, C. Y., Li, R. L., Liu, K. M., Jin, G. E., Ge, R. L., et al. (2020). High-altitude Tibetan fermented milk ameliorated cognitive dysfunction by modified gut microbiota in Alzheimer’s disease transgenic mice. Food Funct. 11, 5308–5319. doi: 10.1039/c9fo03007g
Liu, J., Sun, J., Wang, F., Yu, X., Ling, Z., Li, H., et al. (2015). Neuroprotective effects of clostridium butyricum against vascular dementia in mice via metabolic butyrate. Biomed. Res. Int. 2015:412946. doi: 10.1155/2015/412946
Lopizzo, N., Marizzoni, M., Begni, V., Mazzelli, M., Provasi, S., Borruso, L., et al. (2021). Social isolation in adolescence and long-term changes in the gut microbiota composition and in the hippocampal inflammation: Implications for psychiatric disorders - Dirk Hellhammer award paper 2021. Psychoneuroendocrinology 133:105416. doi: 10.1016/j.psyneuen.2021.105416
Luck, B., Engevik, M. A., Ganesh, B. P., Lackey, E. P., Lin, T., Balderas, M., et al. (2020). Bifidobacteria shape host neural circuits during postnatal development by promoting synapse formation and microglial function. Sci. Rep. 10:7737. doi: 10.1038/s41598-020-64173-3
Luczynski, P., Whelan, S. O., O’Sullivan, C., Clarke, G., Shanahan, F., Dinan, T. G., et al. (2016). Adult microbiota-deficient mice have distinct dendritic morphological changes: differential effects in the amygdala and hippocampus. Eur. J. Neurosci. 44, 2654–2666. doi: 10.1111/ejn.13291
Luo, Y., Zhao, P., Dou, M., Mao, J., Zhang, G., Su, Y., et al. (2021). Exogenous microbiota-derived metabolite trimethylamine N-oxide treatment alters social behaviors: Involvement of hippocampal metabolic adaptation. Neuropharmacology 191:108563. doi: 10.1016/j.neuropharm.2021.108563
Ma, X., Shin, Y. J., Jang, H. M., Joo, M. K., Yoo, J. W., and Kim, D. H. (2021). Lactobacillus rhamnosus and Bifidobacterium longum alleviate colitis and cognitive impairment in mice by regulating IFN-γ to IL-10 and TNF-α to IL-10 expression ratios. Sci. Rep. 11:20659. doi: 10.1038/s41598-021-00096-x
Malmevik, J., Petri, R., Knauff, P., Brattås, P. L., Åkerblom, M., and Jakobsson, J. (2016). Distinct cognitive effects and underlying transcriptome changes upon inhibition of individual miRNAs in hippocampal neurons. Sci. Rep. 6:19879. doi: 10.1038/srep19879
Marcondes Ávila, P. R., Fiorot, M., Michels, M., Dominguini, D., Abatti, M., Vieira, A., et al. (2020). Effects of microbiota transplantation and the role of the vagus nerve in gut-brain axis in animals subjected to chronic mild stress. J. Affect. Disord. 277, 410–416. doi: 10.1016/j.jad.2020.08.013
Marín-Burgin, A., and Schinder, A. F. (2012). Requirement of adult-born neurons for hippocampus-dependent learning. Behav. Brain Res. 227, 391–399. doi: 10.1016/j.bbr.2011.07.001
Marín-Teva, J. L., Cuadros, M. A., Martín-Oliva, D., and Navascués, J. (2011). Microglia and neuronal cell death. Neuron. Glia Biol. 7, 25–40. doi: 10.1017/s1740925x12000014
McVey Neufeld, K. A., O’Mahony, S. M., Hoban, A. E., Waworuntu, R. V., Berg, B. M., Dinan, T. G., et al. (2019). Neurobehavioural effects of Lactobacillus rhamnosus GG alone and in combination with prebiotics polydextrose and galactooligosaccharide in male rats exposed to early-life stress. Nutr. Neurosci. 22, 425–434. doi: 10.1080/1028415x.2017.1397875
Mehrabadi, S., and Sadr, S. S. (2020). Assessment of probiotics mixture on memory function, inflammation markers, and oxidative stress in an Alzheimer’s disease model of rats. Iran. Biomed. J. 24, 220–228. doi: 10.29252/ibj.24.4.220
Meng, F. Q., Li, N., Li, D. L., Song, B. F., and Li, L. (2019). The presence of elevated circulating trimethylamine N-oxide exaggerates postoperative cognitive dysfunction in aged rats. Behav. Brain Res. 368, 94–100. doi: 10.1016/j.bbr.2019.111902
Mohammadi, G., Dargahi, L., Naserpour, T., Mirzanejad, Y., Alizadeh, S. A., Peymani, A., et al. (2019). Probiotic mixture of Lactobacillus helveticus R0052 and Bifidobacterium longum R0175 attenuates hippocampal apoptosis induced by lipopolysaccharide in rats. Int. Microbiol. 22, 317–323. doi: 10.1007/s10123-018-00051-3
Mohammed, S. K., Magdy, Y. M., El-Waseef, D. A. A., Nabih, E. S., Hamouda, M. A., and El-kharashi, O. A. (2020). Modulation of hippocampal TLR4/BDNF signal pathway using probiotics is a step closer towards treating cognitive impairment in NASH model. Physiol. Behav. 214:112762. doi: 10.1016/j.physbeh.2019.112762
Moloney, G. M., O’Leary, O. F., Salvo-Romero, E., Desbonnet, L., Shanahan, F., Dinan, T. G., et al. (2017). Microbial regulation of hippocampal miRNA expression: Implications for transcription of kynurenine pathway enzymes. Behav. Brain Res. 334, 50–54. doi: 10.1016/j.bbr.2017.07.026
Monteggia, L. M., Barrot, M., Powell, C. M., Berton, O., Galanis, V., Gemelli, T., et al. (2004). Essential role of brain-derived neurotrophic factor in adult hippocampal function. Proc. Natl. Acad. Sci. U.S.A. 101, 10827–10832. doi: 10.1073/pnas.0402141101
Mora, F. (2013). Successful brain aging: plasticity, environmental enrichment, and lifestyle. Dialogues Clin. Neurosci. 15, 45–52. doi: 10.31887/DCNS.2013.15.1/fmora
Moreno-Fernández, R., Pedraza, C., and Gallo, M. (2013). Neurogénesis hipocampal adulta y envejecimiento cognitivo. Escr. Psicol. 6, 14–24. doi: 10.5231/psy.writ.2013.2510
Murray, E., Sharma, R., Smith, K. B., Mar, K. D., Barve, R., Lukasik, M., et al. (2019). Probiotic consumption during puberty mitigates LPS-induced immune responses and protects against stress-induced depression- and anxiety-like behaviors in adulthood in a sex-specific manner. Brain Behav. Immun. 81, 198–212. doi: 10.1016/j.bbi.2019.06.016
Neufeld, K. M., Kang, N., Bienenstock, J., and Foster, J. A. (2011). Reduced anxiety-like behavior and central neurochemical change in germ-free mice. Neurogastroenterol. Motil. 25:e119. doi: 10.1111/j.1365-2982.2010.01620.x
Ni, Y. H., Yang, X., Zheng, L. J., Wang, Z., Wu, L. X., Jiang, J. L., et al. (2019). Lactobacillus and Bifidobacterium improves physiological function and cognitive ability in aged mice by the regulation of gut microbiota. Mol. Nutr. Food Res. 63:e1900603. doi: 10.1002/mnfr.201900603
Noble, E. E., Hsu, T. M., and Kanoski, S. E. (2017). Gut to brain dysbiosis: Mechanisms linking western diet consumption, the microbiome, and cognitive impairment. Front. Behav. Neurosci. 11:9. doi: 10.3389/fnbeh.2017.00009
Novotny, M., Klimova, B., and Valis, M. (2019). Microbiome and cognitive impairment: Can any diets influence learning processes in a positive way? Front. Aging. Neurosci. 11:170. doi: 10.3389/fnagi.2019.00170
Ogbonnaya, E. S., Clarke, G., Shanahan, F., Dinan, T. G., Cryan, J. F., and O’Leary, O. F. (2015). Adult hippocampal neurogenesis is regulated by the microbiome. Biol. Psychiatry 78, e7–e9. doi: 10.1016/j.biopsych.2014.12.023
Olson, C. A., Vuong, H. E., Yano, J. M., Liang, Q. Y., Nusbaum, D. J., and Hsiao, E. Y. (2018). The gut microbiota mediates the anti-seizure effects of the ketogenic diet. Cell 173, 1728.e–1741.e. doi: 10.1016/j.cell.2018.04.027
O’Sullivan, E., Barrett, E., Grenham, S., Fitzgerald, P., Stanton, C., Ross, R. P., et al. (2011). BDNF expression in the hippocampus of maternally separated rats: does Bifidobacterium breve 6330 alter BDNF levels? Benef. Microb. 2, 199–207. doi: 10.3920/bm2011.0015
Pang, P. T., and Lu, B. (2004). Regulation of late-phase LTP and long-term memory in normal and aging hippocampus: role of secreted proteins tPA and BDNF. Ageing. Res. Rev. 3, 407–430. doi: 10.1016/j.arr.2004.07.002
Parent, A., and Carpenter, M. B. (1996). Carpenter’s human neuroanatomy [Online]. Baltimore: Williams & Wilkins.
Qin, J., Li, R., Raes, J., Arumugam, M., Burgdorf, K. S., Manichanh, C., et al. (2010). A human gut microbial gene catalogue established by metagenomic sequencing. Nature 464, 59–65. doi: 10.1038/nature08821
Rahmati, H., Momenabadi, S., Vafaei, A. A., Bandegi, A. R., Mazaheri, Z., and Vakili, A. (2019). Probiotic supplementation attenuates hippocampus injury and spatial learning and memory impairments in a cerebral hypoperfusion mouse model. Mol. Biol. Rep. 46, 4985–4995. doi: 10.1007/s11033-019-04949-7
Ramalho, J. B., Soares, M. B., Spiazzi, C. C., Bicca, D. F., Soares, V. M., Pereira, J. G., et al. (2019). In vitro probiotic and antioxidant potential of Lactococcus lactis subsp. cremoris LL95 and its effect in mice behaviour. Nutrients 11:901. doi: 10.3390/nu11040901
Rea, K., Dinan, T. G., and Cryan, J. F. (2016). The microbiome: A key regulator of stress and neuroinflammation. Neurobiol. Stress 4, 23–33. doi: 10.1016/j.ynstr.2016.03.001
Rezaei Asl, Z., Sepehri, G., and Salami, M. (2019). Probiotic treatment improves the impaired spatial cognitive performance and restores synaptic plasticity in an animal model of Alzheimer’s disease. Behav. Brain Res. 376:112183.
Rezaeiasl, Z., Salami, M., and Sepehri, G. (2019). The effects of probiotic Lactobacillus and Bifidobacterium strains on memory and learning behavior, long-term potentiation (LTP), and some biochemical parameters in beta-amyloid-induced rat’s model of Alzheimer’s Disease. Prev. Nutr. Food Sci. 24, 265–273. doi: 10.3746/pnf.2019.24.3.265
Romo-Araiza, A., Gutierrez-Salmean, G., Galvan, E. J., Hernandez-Frausto, M., Herrera-Lopez, G., Romo-Parra, H., et al. (2018). Probiotics and prebiotics as a therapeutic strategy to improve memory in a model of middle-aged rats. Front. Aging. Neurosci. 10:416. doi: 10.3389/fnagi.2018.00416
Roy Sarkar, S., Mitra Mazumder, P., and Banerjee, S. (2020). Probiotics protect against gut dysbiosis associated decline in learning and memory. J. Neuroimmunol. 348:577390. doi: 10.1016/j.jneuroim.2020.577390
Ryan, S. M., and Nolan, Y. M. (2016). Neuroinflammation negatively affects adult hippocampal neurogenesis and cognition: Can exercise compensate? Neurosci. Biobehav. Rev. 61, 121–131. doi: 10.1016/j.neubiorev.2015.12.004
Salami, M. (2021). Interplay of good bacteria and central nervous system: Cognitive aspects and mechanistic considerations. Front. Neurosci. 15:613120. doi: 10.3389/fnins.2021.613120
Salami, M., Kouchaki, E., Asemi, Z., and Tamtaji, O. R. (2019). How probiotic bacteria influence the motor and mental behaviors as well as immunological and oxidative biomarkers in multiple sclerosis? A double blind clinical trial. J. Funct. Foods 52, 8–13. doi: 10.1016/j.jff.2018.10.023
Scheller, J., Chalaris, A., Schmidt-Arras, D., and Rose-John, S. (2011). The pro- and anti-inflammatory properties of the cytokine interleukin-6. Biochim. Biophys. Acta 1813, 878–888. doi: 10.1016/j.bbamcr.2011.01.034
Shan, B., Ai, Z., Zeng, S., Song, Y., Jiagui, S., Zeng, Q., et al. (2020). Gut microbiome-derived lactate promotes to anxiety-like behaviors through GPR81 receptor-mediated lipid metabolism pathway. Psychoneuroendocrinology 117:104699. doi: 10.1016/j.psyneuen.2020.104699
Shen, H. P., Guan, Q. B., Zhang, X. L., Yuan, C., Tan, Z. Y., Zhai, L. P., et al. (2020). New mechanism of neuroinflammation in Alzheimer’s disease: The activation of NLRP3 inflammasome mediated by gut microbiota. Prog. Neuro Psychopharmacol. Biol. Psychiatry 100:109884. doi: 10.1016/j.pnpbp.2020.109884
Sherman, P. M., Ossa, J. C., and Johnson-Henry, K. (2009). Unraveling mechanisms of action of probiotics. Nutr. Clin. Pract. 24, 10–14. doi: 10.1177/0884533608329231
Slattery, J., MacFabe, D. F., Kahler, S. G., and Frye, R. E. (2016). Enteric ecosystem disruption in autism spectrum disorder: Can the microbiota and macrobiota be restored? Curr. Pharm. Des. 22, 6107–6121. doi: 10.2174/1381612822666160905123953
Sousa, R., Halper, J., Zhang, J., Lewis, S. J., and Li, W. I. (2008). Effect of Lactobacillus acidophilus supernatants on body weight and leptin expression in rats. BMC Comple. Altern. Med. 8:5. doi: 10.1186/1472-6882-8-5
Sovijit, W. N., Sovijit, W. E., Pu, S., Usuda, K., Inoue, R., Watanabe, G., et al. (2019). Ovarian progesterone suppresses depression and anxiety-like behaviors by increasing the Lactobacillus population of gut microbiota in ovariectomized mice. Neurosci. Res. 68, 76–82. doi: 10.1016/j.neures.2019.04.005
Sun, J., Wang, F., Ling, Z., Yu, X., Chen, W., Li, H., et al. (2016). Clostridium butyricum attenuates cerebral ischemia/reperfusion injury in diabetic mice via modulation of gut microbiota. Brain Res. 1642, 180–188. doi: 10.1016/j.brainres.2016.03.042
Tahmasebi, S., Oryan, S., Mohajerani, H. R., Akbari, N., and Palizvan, M. R. (2020). Probiotics and Nigella sativa extract supplementation improved behavioral and electrophysiological effects of PTZ-induced chemical kindling in rats. Epilepsy Behav. 104:106897. doi: 10.1016/j.yebeh.2019.106897
Talani, G., Biggio, F., Mostallino, M. C., Locci, V., Porcedda, C., Boi, L., et al. (2020). Treatment with gut bifidobacteria improves hippocampal plasticity and cognitive behavior in adult healthy rats. Neuropharmacology 165:107909. doi: 10.1016/j.neuropharm.2019.107909
Tang, W., Meng, Z. Y., Li, N., Liu, Y. Y., Li, L., Chen, D. F., et al. (2021). Roles of gut microbiota in the regulation of hippocampal plasticity, inflammation, and hippocampus-dependent behaviors. Front. Cell. Infect. Microbiol. 10:611014. doi: 10.3389/fcimb.2020.611014
Toda, T., Parylak, S. L., Linker, S. B., and Gage, F. H. (2019). The role of adult hippocampal neurogenesis in brain health and disease. Mol. Psychiatry 24, 67–87. doi: 10.1038/s41380-018-0036-2
Tsukahara, T., Kawase, T., Yoshida, H., Bukawa, W., Kan, T., and Toyoda, A. (2019). Preliminary investigation of the effect of oral supplementation of Lactobacillus plantarum strain SNK12 on mRNA levels of neurotrophic factors and GABA receptors in the hippocampus of mice under stress-free and sub-chronic mild social defeat-stressing conditions. Biosci. Biotechnol. Biochem. 83, 2345–2354. doi: 10.1080/09168451.2019.1659717
Valkanova, V., Ebmeier, K. P., and Allan, C. L. (2013). CRP, IL-6 and depression: A systematic review and meta-analysis of longitudinal studies. J. Affect. Disord. 150, 736–744. doi: 10.1016/j.jad.2013.06.004
Wakselman, S., Béchade, C., Roumier, A., Bernard, D., Triller, A., and Bessis, A. (2008). Developmental neuronal death in hippocampus requires the microglial CD11b integrin and DAP12 immunoreceptor. J. Neurosci. 28:8138. doi: 10.1523/JNEUROSCI.1006-08.2008
Wang, H., Sun, Y., Xin, J., Zhang, T., Sun, N., Ni, X., et al. (2020a). Lactobacillus johnsonii BS15 prevents psychological stress-induced memory dysfunction in mice by modulating the gut-brain axis. Front. Microbiol. 11:1941. doi: 10.3389/fmicb.2020.01941
Wang, Q. J., Shen, Y. E., Wang, X., Fu, S., Zhang, X., Zhang, Y. N., et al. (2020b). Concomitant memantine and Lactobacillus plantarum treatment attenuates cognitive impairments in APP/PS1 mice. Aging 12, 628–649. doi: 10.18632/aging.102645
Wang, T., Hu, X., Liang, S., Li, W., Wu, X., Wang, L., et al. (2015). Lactobacillus fermentum NS9 restores the antibiotic induced physiological and psychological abnormalities in rats. Benef. Microb. 6, 707–717. doi: 10.3920/bm2014.0177
Wang, Z., Chen, W. H., Li, S. X., He, Z. M., Zhu, W. L., Ji, Y. B., et al. (2021). Gut microbiota modulates the inflammatory response and cognitive impairment induced by sleep deprivation. Mol. Psychiatry 26, 6277–6292.
Wei, G. Z., Martin, K. A., Xing, P. Y., Agrawal, R., Whiley, L., Wood, T. K., et al. (2021). Tryptophan-metabolizing gut microbes regulate adult neurogenesis via the aryl hydrocarbon receptor. Proc. Natl. Acad. Sci. U.S.A. 118:e2021091118. doi: 10.1073/pnas.2021091118
Wu, Q., Li, Q. F., Zhang, X., Ntim, M., Wu, X. F., Li, M., et al. (2020). Treatment with Bifidobacteria can suppress A beta accumulation and neuroinflammation in APP/PS1 mice. Peerj 8:e10262. doi: 10.7717/peerj.10262
Xiao, J., Wang, T., Xu, Y., Gu, X. Z., Li, D. Y., Niu, K., et al. (2020). Long-term probiotic intervention mitigates memory dysfunction through a novel H3K27me3-based mechanism in lead-exposed rats. Transl. Psychiatry 10:25. doi: 10.1038/s41398-020-0719-8
Xin, J. G., Zene, D., Wang, H. S., Sun, N., Khalique, A., Zhao, Y., et al. (2020). Lactobacillus johnsonii BS15 improves intestinal environment against fluoride-induced memory impairment in mice-a study based on the gut-brain axis hypothesis. Peerj 8:e10125. doi: 10.7717/peerj.10125
Xu, N. N., Fan, W. T., Zhou, X. Y., Liu, Y. P., Ma, P., Qi, S. H., et al. (2018). Probiotics decrease depressive behaviors induced by constipation via activating the AKT signaling pathway. Metab. Brain Dis. 33, 1625–1633. doi: 10.1007/s11011-018-0269-4
Xu, X., Wang, K., Cao, X., Li, Z., Zhou, Y., Ren, J., et al. (2021). Gut microbial metabolite short-chain fatt acids partially reverse surgery and anesthesia-induced behavior deficits in C57BL/6J mice. Front. Neurosci. 15:664641. doi: 10.3389/fnins.2021.664641
Yang, X. Y., Zheng, M. X., Hao, S. S., Shi, H. L., Lin, D. H., Chen, X., et al. (2020). Curdlan prevents the cognitive deficits induced by a high-fat diet in mice via the gut-brain axis. Front. Neurosci. 14:384. doi: 10.3389/fnins.2020.00384
Yang, Y. J., Zhong, Z. Q., Wang, B. J., Xia, X. W., Yao, W. Y., Huang, L., et al. (2019). Early-life high-fat diet-induced obesity programs hippocampal development and cognitive functions via regulation of gut commensal Akkermansia muciniphila. Neuropsychopharmacology 44, 2054–2064. doi: 10.1038/s41386-019-0437-1
Yun, S. W., Kim, J. K., Lee, K. E., Oh, Y. J., Choi, H. J., Han, M. J., et al. (2020). A probiotic Lactobacillus gasseri alleviates Escherichia coli-induced cognitive impairment and depression in mice by regulating IL-1β expression and gut microbiota. Nutrients 12:3441. doi: 10.3390/nu12113441
Zeng, L., Zeng, B. H., Wang, H. Y., Li, B., Huo, R., Zheng, P., et al. (2016). Microbiota modulates behavior and protein kinase C mediated cAMP response element-binding protein signaling. Sci. Rep. 6:29998. doi: 10.1038/srep29998
Zeraati, M., Enayati, M., Kafami, L., Shahidi, S. H., and Salari, A. A. (2019). Gut microbiota depletion from early adolescence alters adult immunological and neurobehavioral responses in a mouse model of multiple sclerosis. Neuropharmacology 157:107685. doi: 10.1016/j.neuropharm.2019.107685
Zhang, J., Yu, C., Zhang, X., Chen, H., Dong, J., Lu, W., et al. (2018). Porphyromonas gingivalis lipopolysaccharide induces cognitive dysfunction, mediated by neuronal inflammation via activation of the TLR4 signaling pathway in C57BL/6 mice. J. Neuroinflammation 15:37. doi: 10.1186/s12974-017-1052-x
Zhang, Y. J., Li, S., Gan, R. Y., Zhou, T., Xu, D. P., and Li, H. B. (2015). Impacts of gut bacteria on human health and diseases. Int. J. Mol. Sci. 16, 7493–7519.
Zhang, Y., Pu, F., Cheng, R., Guo, J., Shen, X., Wang, S., et al. (2020). Effect of heat-inactivated Lactobacillus paracasei N1115 on microbiota and gut-brain axis related molecules. Biosci. Microbiota. Food Health 39, 89–99. doi: 10.12938/bmfh.2019-025
Zhao, L., Zhang, C. Y., Cao, G. L., Dong, X. Y., Li, D. L., and Jiang, L. (2019). Higher circulating trimethylamine N-oxide sensitizes sevoflurane-induced cognitive dysfunction in aged rats probably by downregulating hippocampal methionine sulfoxide reductase A. Neurochem. Res. 44, 2506–2516.
Zhao, Y., Jaber, V., and Lukiw, W. J. (2017). Secretory products of the human GI tract microbiome and their potential impact on Alzheimer’s disease (AD): Detection of lipopolysaccharide (LPS) in AD hippocampus. Front. Cell. Infect. Microbiol. 7:318. doi: 10.3389/fcimb.2017.00318
Zheng, P., Zeng, B. H., Liu, M. L., Chen, J. J., Pan, J. X., Han, Y., et al. (2019). The gut microbiome from patients with schizophrenia modulates the glutamate-glutamine-GABA cycle and schizophrenia-relevant behaviors in mice. Sci. Adv. 5:eaau8317. doi: 10.1126/sciadv.aau8317
Zhu, F., Guo, R. J., Wang, W., Ju, Y. M., Wang, Q., Ma, Q. Y., et al. (2020). Transplantation of microbiota from drug-free patients with schizophrenia causes schizophrenia-like abnormal behaviors and dysregulated kynurenine metabolism in mice. Mol. Psychiatry 25, 2905–2918. doi: 10.1038/s41380-019-0475-4
Keywords: hippocampus, gut microbiota, learning and memory, synaptic plasticity, probiotics
Citation: Salami M and Soheili M (2022) The microbiota-gut- hippocampus axis. Front. Neurosci. 16:1065995. doi: 10.3389/fnins.2022.1065995
Received: 15 October 2022; Accepted: 07 December 2022;
Published: 23 December 2022.
Edited by:
Yi Xu, Hefei University of Technology, ChinaReviewed by:
Sadia Sana, Government College University Faisalabad, PakistanAhmad Ali, University of Mumbai, India
Copyright © 2022 Salami and Soheili. This is an open-access article distributed under the terms of the Creative Commons Attribution License (CC BY). The use, distribution or reproduction in other forums is permitted, provided the original author(s) and the copyright owner(s) are credited and that the original publication in this journal is cited, in accordance with accepted academic practice. No use, distribution or reproduction is permitted which does not comply with these terms.
*Correspondence: Mahmoud Salami, ✉ c2FsYW1pLW1Aa2F1bXMuYWMuaXI=