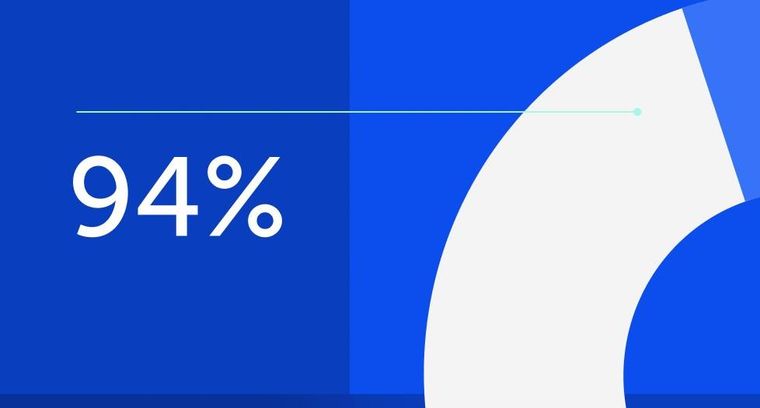
94% of researchers rate our articles as excellent or good
Learn more about the work of our research integrity team to safeguard the quality of each article we publish.
Find out more
ORIGINAL RESEARCH article
Front. Neurosci., 20 October 2022
Sec. Neural Technology
Volume 16 - 2022 | https://doi.org/10.3389/fnins.2022.1029683
This article is part of the Research TopicAdvances in Bioelectronics and Stimulation Strategies for Next Generation NeuroprostheticsView all 11 articles
Electroconvulsive therapy (ECT) is the most effective treatment for severe treatment-resistant depression but concern about cognitive side-effects, particularly memory loss, limits its use. Recent observational studies on large groups of patients who have received ECT report that cognitive side-effects were associated with electric field (EF) induced increases in hippocampal volume, whereas therapeutic efficacy was associated with EF induced increases in sagittal brain structures. The aim in the present study was to determine whether a novel fronto-medial (FM) ECT electrode placement would minimize electric fields in bilateral hippocampi (HIP) whilst maximizing electric fields in dorsal sagittal cortical regions. An anatomically detailed computational head model was used with finite element analysis, to calculate ECT-induced electric fields in specific brain regions identified by translational neuroimaging studies of treatment-resistant depressive illness, for a range of electrode placements. As hypothesized, compared to traditional bitemporal (BT) electrode placement, a specific FM electrode placement reduced bilateral hippocampal electric fields two-to-three-fold, whilst the electric fields in the dorsal anterior cingulate (dAC) were increased by approximately the same amount. We highlight the clinical relevance of this specific FM electrode placement for ECT, which may significantly reduce cognitive and non-cognitive side-effects and suggest a clinical trial is indicated.
Psychiatric illnesses are the leading cause of long term disability world-wide with depression the commonest cause (Whiteford et al., 2013; WHO, 2018). Better treatments, with improved efficacy and reduced side-effects, are needed. Electroconvulsive therapy (ECT) is the most effective treatment for severe treatment-resistant depression, typically used when patients have not responded to multiple treatments or need a rapid response with faster action than other treatments (Pagnin et al., 2004; Wade et al., 2020).
A recent report from the Scottish ECT Accreditation Network (SEAN) national audit (SEAN, 2021), covering a decade of treatments from 2009 to 2018, with ∼4000 treatments per year, found the majority of patients receiving ECT were in the 50–79 years old age range, the most common diagnosis was depression with or without psychotic symptoms, ∼15% of treatment episodes were recorded as emergency lifesaving treatments, and 75% of patients were “much improved” or “very much improved” after a course of ECT. The commonest reported side-effects in the SEAN reports were memory problems, headache and muscle aches and brief disorientation.
Side-effects on memory are among the most important factors limiting acceptance, prescription and use of ECT and may contribute to premature discontinuation of treatment, or a lack of use of ECT when it could be effective (Finnegan and McLoughlin, 2019). Whilst there is little evidence for anterograde memory impairment persisting for longer than a few weeks after the last treatment, the duration of retrograde amnesia is more controversial. In practice, minimization of cognitive side-effects is by identification of patients at higher risk and modifying treatment delivery: e.g., stimulus dose, frequency of treatments, pulse-width, and electrode placement. Bitemporal (BT) placement of electrodes has the greatest therapeutic efficacy but most cognitive side-effects; high dose right unilateral (RUL) electrode placement has fewer side-effects (Finnegan and McLoughlin, 2019) but is generally regarded as less effective.
In health, the hippocampus is crucial for human episodic memory encoding (Squire, 1986; Lisman et al., 2017) with the anterior region also implicated in emotional experience (Gray and McNaughton, 2000; Phelps, 2004). Adverse memory side-effects of ECT are thought to be predominately related to stimulation of the hippocampus, although other regions including the inferior and middle frontal gyri are important for memory retrieval, with all regions stimulated to a variable extent by a wide-variety of ECT electrode placements (Bai et al., 2019). However, for patients who have not received ECT, depressive illness is itself associated with cognitive, including memory impairment (Anderson et al., 2021), and there is robust evidence for hippocampal volume reduction in unipolar and bipolar illnesses (Hibar et al., 2016; Schmaal et al., 2016).
Recently, a series of publications from the Global ECT-MRI Research Collaboration (GEMRIC) have reported important findings. First, a longitudinal neuroimaging study of brain structure in a large number of patients reported that the number of ECT sessions and electrode placement impacts the extent and laterality of ECT-induced hippocampal enlargement, but there was no relationship between hippocampal volume enlargement and clinical outcome (Oltedal et al., 2018). A second study calculated the electric field (EF) induced by ECT in different brain regions, reporting a strong relationship between EF intensity and brain volume increase, but that neither structural volume increase nor EF intensity were associated with antidepressant response (Argyelan et al., 2019) although a relationship has also been reported (Deng et al., 2021). These were univariate studies and an alternative multivariate approach can be helpful (Steele and Paulus, 2019). A third GEMRIC study tested for associations between 80 different brain regions and clinical outcome using a multivariate approach, reporting that structural brain increases in cortical mid-line structures, such as the dorsal anterior cingulate (dAC) and precuneus posterior cingulate (PPC), predicted individual patient response but not medial temporal regions, such as the amygdala and hippocampus, which have been the focus of ECT research thus far (Mulders et al., 2020). Its long been established that induction of a generalized seizure is required for efficacy, and these recent studies suggest that an ECT electrode configuration specifically designed to maximize the induced EF in dorsal sagittal structures, simultaneously minimizing the induced EF in bilateral HIP, could be optimally therapeutic and minimize cognitive side-effects.
Electric field is typically maximal in brain regions immediately deep to the ECT electrodes. This means an electrode placement rotated through 90 degrees from the ubiquitous (in the UK) BT orientation, with the anterior electrode near the dAC and posterior electrode near the PPC in a fronto-medial (FM) configuration (Lee et al., 2016), should maximize the induced EF in these structures, also maximizing the distance from the electrodes to bilateral HIP. However, the precise EF in specific brain sub-regions identified as important in clinical neuroimaging studies of treatment-resistant depression is unclear. Also, different FM electrode placements are possible. Therefore, we used a computational model (Bai et al., 2019) to test the hypothesis that an optimal FM placement would maximize the EF in specific sagittal brain regions whilst minimizing the EF in subregions of bilateral HIP, identified by neuroimaging studies of patients with treatment-resistant depression who had not received ECT.
A finite element head model was constructed from a 3T MRI T1-weighted 1 mm isotropic resolution brain scan provided by the NIH Human Connectome Project (MGH1010, gender: female, age: 25–29) (Fan et al., 2016). Briefly, head tissue compartments were analyzed using Brainsuite1 to segment skin and subcutaneous tissue (fat and muscle), skull, cerebrospinal fluid, gray matter, and white matter. Segmented tissue compartments were then imported into 3D Slicer2 for manual correction of voxels as required (Bai et al., 2019; Bakir et al., 2019). The tissue compartments were assumed to be homogeneous with conductivities assigned from the mean values from multiple studies (Bai et al., 2019). Modeling was done using COMSOL Multiphysics (v5.0) finite element analysis software (COMSOL AB, Stockholm, Sweden) with a direct linear solver. Mean and standard deviation of the EFs were calculated from 100 points selected randomly within anatomically defined regions of interest (ROI).
We calculated EFs in specific sagittal brain regions and bilateral HIP for different electrode configurations. A priori defined spherical ROI were defined using Montreal Neurological Institute (MNI) standard brain anatomy selected from the translational neuroimaging studies discussed later: dAC (0,19,37) 15 mm (Steele et al., 2008; Tolomeo et al., 2016), subgenual AC (sAC) (0,25,−9) 15 mm (Drevets et al., 1997), precuneus posterior cingulate (PPC) (0,−58,46) 15 mm (Johnston et al., 2015) hippocampi (HIP) (−32,−28,−10) 10 mm, (34,−26,−10) 10 mm (Johnston et al., 2015), where the coordinate is the location of the center of the sphere and diameter is in mm. These ROIs are shown in Figure 1. The head model segmentation, manual editing, and configuration of COMSOL for electrode placements and solving was done using the scan in its original non-MNI patient anatomical space. To determine MNI equivalent locations, the T1 scan was spatially normalized using SPM123, then the resultant deformation field used to calculate the inverse transformation (Ashburner, 2016), from MNI back to original patient scan anatomical space.
Figure 1. Regions of Interest (ROI) used for sampling the electric field (EF) distributions; left hippocampus (L-HIP), right hippocampus (R-HIP) and for other abbreviations see main text.
A total of 7 bipolar ECT electrode placements, including 4 alternative FM placements, were investigated: (i) BT–center of each electrode was 3 cm superior to the midpoint of a line from the lateral canthus of the eye to the external ear canal, (ii) bifrontal (BF)–center of each electrode was 5 cm superior to the lateral canthus of the eyes, (iii) RUL–anterior electrode 5 cm superior to the lateral canthus of the right eye with posterior electrode just right of the vertex, (iv) mid-frontal-occipital (MFO)–anterior electrode just above nasion and the posterior electrode over the occiput (15), (v) mid-frontal-vertex (MFV1)–anterior electrode just above nasion (as MFO) but with posterior electrode at the vertex, (vi) MFV2–anterior electrode higher at 2.5 cm above nasion and the posterior electrode at the vertex, and (vii) MFV3–anterior electrode highest at 5 cm above the nasion and the posterior electrode at the vertex. MFV3 is the most dorsal of the 4 sagittal FM electrode configurations. These electrode placements are shown in Figure 2 and modeling for each assumed an ECT current of 800 mA delivered to the scalp from a pair of circular electrodes with 5 cm diameter (Bai et al., 2019).
Figure 2. Electric field (EF) distributions and electroconvulsive therapy (ECT) electrode placements; for the latter anterior is to the left except for the right unilateral (RUL) placement, see main text for abbreviations. High EF (red) and low EF (blue) with color bar showing EF in V/m.
Figure 2 shows sagittal and coronal views of the EF magnitudes for the 7 electrode configurations with the coronal views chosen to intersect the hippocampi ROIs. As expected, the EFs were highest in brain regions under the electrodes. Mean and standard deviation EFs for selected ROI are shown in Table 1.
Table 1. Electroconvulsive therapy (ECT)-induced electric fields in V/m (standard deviation) for different electrode placements sampled within specific regions of interest (ROI).
The BT placement resulted in the highest bilateral hippocampal ROI EFs, with high EFs throughout both temporal lobes and particularly high EFs in bilateral temporal lobe white matter. The right hippocampal ROI EF for RUL placement was comparable to that obtained for the BT placement. With a longer distance between the electrodes and hippocampi, BF resulted in lower hippocampal ROI EFs than BT, and significant EFs only in the most anterior medial frontal brain regions. BT resulted in the lowest EF in the dAC ROI, compared to RUL and BF configurations. In contrast, RUL resulted in a high dAC ROI EF because with RUL, the posterior electrode is close to the vertex. Table 1 also shows the ratio of EFs for dorsal AC ROI to each hippocampal ROI: BT had the lowest and RUL the highest ratios.
Mid-frontal-occipital had the electrodes placed lowest in the sagittal plane and resulted in the highest bilateral hippocampal EFs for all sagittal placements with significant EFs throughout most of the brain. Moving the posterior electrode from the occipital region to the vertex (MFV1) resulted in a reduction in hippocampal ROI EFs and the EFs throughout both temporal lobes. Then, moving the anterior electrode more superior (MFV2) resulted in a further reduction in hippocampal ROI EFs due to increased distance from the electrodes to both HIP. Hippocampal EFs were minimized by moving the anterior electrode still more superior (MFV3) but this resulted in only a small reduction in the dAC ROI EF, compared with the MFV2 placement. The highest dAC ROI to hippocampal EF ROI ratios were obtained using the most superior MFV3 placement but the increase was marginal in comparison to the MFV2 placement.
It is important that the minimum distance between the two electrodes is not extremely low, to avoid superficial shunting of current between the electrodes due to the electrically resistant skull. BF electrode placement is a long-established method for delivering ECT. Traditional BF electrode placement is 5 cm superior to the outer canthus of the eye, making the distance between the centers of the electrodes about 18 cm or less depending on head size. Deducting 2–3 cm from each of the electrodes due to their size makes the closest edge to edge electrode distance 12–14 cm for BF.
With regard to MFV2 placement and assuming standard EEG positions, then nasion to inion is measured for each patient and percentages are used. By definition Fp1 is 10% from nasion to inion, and the vertex is 50% from nasion to inion. With most patients the nasion to Fp1 distance is about 5 cm, so as Fp1 to vertex is 40% of the distance, the Fp1 to vertex distance is about 20 cm. If we assume the center of the anterior MFV2 electrode is at Fp1 or no higher than 1 cm above this, with the posterior electrode at the vertex, then allowing for 5 cm diameter electrodes, this makes the minimum MFV2 electrode edge distance 14–15 cm, which compares favorably with BF placement.
Therefore, from the perspective of maximizing the dAC/hippocampus ratios and ensuring an inter-electrode edge distance no less than BF, the optimal FM electrode placement is MFV2.
Electroconvulsive therapy is the most effective treatment for severe and treatment-resistant depression but use is limited because of concern about side-effects (Finnegan and McLoughlin, 2019). ECT causes increases in the volume of brain structures (Argyelan et al., 2019) and ECT-induced increases in the volume of the dentate gyri of the HIP are associated with increased memory impairment (Gbyl et al., 2021). Volume increases in the HIP are not associated with clinical response (Oltedal et al., 2018; Mulders et al., 2020), but increases in the volume of sagittal cortical regions, such as the dorsal anterior and posterior cingulate are associated with clinical response (Mulders et al., 2020). We therefore tested the hypothesis that one of the FM electrode configurations, but not alternative electrode placements such as BT, would minimize the induced EF in the bilateral HIP and maximize the EFs in the dAC and PPC. Detailed computational modeling confirmed this hypothesis.
A large systematic review and meta-analysis of studies of healthy subjects concluded that a sub-region of the dorsal medial cortex, the dAC, integrates subjective experience of negative affect and pain and is important for cognitive control (Shackman et al., 2011). Clinically, anterior cingulotomy, which comprises small bilateral lesions in the dAC, has long been used as a treatment of last resort for severe treatment-resistant depressive illness, when all other treatments including ECT have been tried and failed, the patient wants the operation and can provide sustained informed consent (Steele et al., 2008). In an observational study of patients who had received cingulotomy, we reported the dAC has a causal role in negative affect so lesions in this region could be therapeutic for patients with otherwise intractable mood, anxiety and pain syndromes (Tolomeo et al., 2016). These findings for cingulotomy may be consistent with a study on different patients who had received ECT and not surgery, where we reported ECT decreases the connectivity between the dAC and other brain regions (Perrin et al., 2012). An unexpected result was that smaller dAC lesions were associated with better clinical response (Steele et al., 2008), suggesting the therapeutic effect may be due to smaller lesions inducing connectivity and plasticity change. Consistent with this, ECT increases plasticity (Dukart et al., 2014) and changes in the dAC predicted clinical response (Mulders et al., 2020).
Depressive illness has been associated with abnormal function of other cingulate regions. We and others reported that the sAC and rostral AC have a blunted reward response using fMRI (Johnston et al., 2015; Rupprechter et al., 2020) with reduced connectivity from the sAC-rostral AC to the basal ganglia (Rupprechter et al., 2020). Others have reported sAC functional abnormalities using PET (Drevets et al., 1997; Mayberg et al., 1999) with a small deep brain stimulation trial reporting beneficial effect (Mayberg et al., 2005) but a larger blinded study did not report efficacy (Holtzheimer et al., 2017). Using fMRI, we also reported blunting of the reward response of the PPC of patients with treatment resistant depression (Johnston et al., 2015), and abnormally increased connectivity between the PPC and the lateral orbitofrontal cortex has been reported (Cheng et al., 2018). Notably, the MF electrode configuration also increases ECT-induced EFs in the sAC and PPC regions compared to alternative configurations such as BT, implying it could induce gray matter increase and plasticity also in these regions.
There is robust evidence from two large ENIGMA consortium studies for hippocampal volume reductions in unipolar (Schmaal et al., 2016) and bipolar (Hibar et al., 2016) illnesses. An influential review of preclinical studies predicted that depression is associated with hippocampal abnormalities linked to ruminations (Deakin and Graeff, 1991; Deakin, 2013) and our fMRI study on patients with treatment-resistant depression reported consistent hippocampal abnormalities (Johnston et al., 2015). Cognitive abnormalities in patients with depression who have not received ECT may be linked to abnormal hippocampal function (Johnston et al., 2015). However, whilst ECT is associated with hippocampal volume increases, reversing depression-associated hippocampal decreases, there is clinical evidence that this change does not represent a causal therapeutic effect (Oltedal et al., 2018; Argyelan et al., 2019) although a relationship has also been reported (Deng et al., 2021). Antidepressants also cause neurogenesis and hippocampal volume increase (Malberg et al., 2021) but without adverse effects on memory (BNF, 2016) and some effective treatments (Steele et al., 2008) have no direct effect on the hippocampus. In contrast to uncertainty about ECT therapeutic mechanisms, evidence for ECT-induced memory problems is relatively robust, particularly for BT (Finnegan and McLoughlin, 2019) which we show is associated with the highest bilateral hippocampal ECT-induced EFs. Alternative electrode placements such as RUL have been proposed to minimize effects on one hippocampus, with RUL treatment efficacy comparable to BT by administering RUL ECT at six times the threshold dose (Finnegan and McLoughlin, 2019). Notably though, we found the induced EF in the stimulated hemisphere with RUL is as high as BT assuming the same stimulation parameters. This implies that in clinical practice the right temporal lobe and hippocampus receives a higher EF with RUL placement than with BT, but the left hemisphere a low EF presumably accounting for reduced cognitive side-effects overall.
Previous studies have used EF modeling to investigate different electrode placements, including BT, BF, RUL, and other configurations including MFO, which all are associated with significant ECT-induced hippocampal EFs (Bai et al., 2019). EF calculations for different electrode placements which included a version of FM have also been reported, which are consistent with our findings. However, the aims of these earlier studies were different: determination of minimum current to induce a seizure in rhesus macaque monkeys using ECT and magnetic seizure therapy using a variety of electrode placements (Peterchev et al., 2015), determination of the spatial extent of brain stimulation necessary to produce a seizure in macaque monkeys using a variety of electrode placements (Lee et al., 2017), and a study that concluded that differences in EF strength from different electrode placements may be linked to cognitive side-effects (Lee et al., 2016). Regarding patients who have actually received a version of FM ECT, to our knowledge there is only a conference abstract published a decade ago reporting a single patient (Rosa et al., 2012); a 40 years old woman with manic phase treatment-resistant schizoaffective disorder, who received as an open label trial, 10 sessions of low current amplitude ECT. The authors reported motor and EEG seizures were qualitatively like conventional ECT, the patient had no significant memory complaints although formal testing was not performed, she had resolution of psychotic and euphoric symptoms, and the authors concluded further investigation of FM placement was indicated.
Our work is novel because (i) it additionally builds on recent GEMRIC (Oltedal et al., 2018; Argyelan et al., 2019; Mulders et al., 2020) studies of patients who have received ECT, (ii) it uses for quantification MNI defined regions reported from neuroimaging studies of patients with severe treatment-resistant depression who have not received ECT (Steele et al., 2008; Johnston et al., 2015; Tolomeo et al., 2016), (iii) we compared four alternative FM electrode placements, and (iv) we highlight the particular clinical relevance of the MFV2 FM electrode placement for actual treatment. This FM electrode placement may have additional clinical advantages over BT electrode placement, by reducing the likelihood of non-cognitive side-effects caused by direct electrical stimulation over temporalis muscle with supraphysiological bite induction, which can cause damage of the tongue, teeth and dental implants, jaw pain and headache (Haghighi et al., 2016; Mulder and Grootens, 2020). Consequently, we suggest a randomized blinded clinical trial is indicated, to compare the therapeutic efficacy and side-effects of MFV2 vs. other placements. Limitations of EF modeling for ECT research have been discussed (Sartorius, 2022), which imply that EFs to brain structures distant from electrodes may be less than calculated, supporting the rationale for the FM orientation avoiding bilateral hippocampal EF effects.
In conclusion, ECT is the most effective treatment for severe treatment-resistant depression yet concern about cognitive side-effects, particularly memory loss, limits prescription and use of ECT (Finnegan and McLoughlin, 2019). We have modeled the induced EF from a range of electrode placements in specific brain regions informed by a range of translational neuroimaging studies, including four alternative FM placements, and highlight the MFV2 placement as being particularly important from a clinical perspective. We predict this electrode placement will minimize cognitive side-effects such as memory loss and may reduce non-cognitive side-effects such as oral cavity damage and headache, whilst maintaining or increasing therapeutic efficacy.
Publicly available datasets were analyzed in this study. This data can be found here: NIH Human Connectome Project (MGH1010).
Ethical review and approval were not required for the study on human participants in accordance with the local legislation and institutional requirements. Written informed consent was not required for the study on human participants in accordance with the local legislation and institutional requirements.
JS, TF, and SB conceived the project. SB and TF did the computational modeling. JS wrote the initial draft. All authors discussed the results and contributed to the final manuscript.
We thank our institutions for non-specific support.
The authors declare that the research was conducted in the absence of any commercial or financial relationships that could be construed as a potential conflict of interest.
All claims expressed in this article are solely those of the authors and do not necessarily represent those of their affiliated organizations, or those of the publisher, the editors and the reviewers. Any product that may be evaluated in this article, or claim that may be made by its manufacturer, is not guaranteed or endorsed by the publisher.
Anderson, I. M., McAllister-Williams, R. H., Downey, D., Elliott, R., and Loo, C. (2021). Cognitive function after electroconvulsive therapy for depression: Relationship to clinical response. Psychol. Med. 51, 1647–1656. doi: 10.1017/S0033291720000379
Argyelan, M., Oltedal, L., Deng, Z. D., Wade, B., Bikson, M., Joanlanne, A., et al. (2019). Electric field causes volumetric changes in the human brain. Elife 8:e49115. doi: 10.7554/eLife.49115
Ashburner, J. (2016). How. to get the individual coordinate from MNI space. Available online at: https://www.jiscmail.ac.uk/cgi-bin/webadmin?A2=spm;8005badf.1606 (accessed March 2, 2022).
Bai, S., Martin, D., Guo, T., Dokos, S., and Loo, C. (2019). Computational comparison of conventional and novel electroconvulsive therapy electrode placements for the treatment of depression. Eur. Psychiatry 60, 71–78. doi: 10.1016/j.eurpsy.2019.05.006
Bakir, A., Bai, S., Lovell, N., Martin, D., Loo, C., and Dokos, S. (2019). “Finite element modelling framework for electroconvulsive therapy and other transcranial stimulations,” in Brain and human body modeling, eds S. Makarov, M. Horner, and G. Noetscher (Cham: Springer).
Cheng, W., Rolls, E. T., Qiu, J., Yang, D., Ruan, H., Wei, D., et al. (2018). Functional connectivity of the precuneus in unmedicated patients with depression. Biol. Psychiatry Cogn. Neurosci. Neuroimaging 3, 1040–1049. doi: 10.1016/j.bpsc.2018.07.008
Deakin, J. (2013). The origins of ‘5-HT and mechanisms of defence’ by Deakin and Graeff: A personal perspective. J. Psychopharmacol. 27, 1084–1089. doi: 10.1177/0269881113503508
Deakin, J. F. W., and Graeff, F. G. (1991). 5-HT and the mechanisms of defence. J. Psychopharmacol. 5, 305–315. doi: 10.1177/026988119100500414
Deng, Z. D., Argyelan, M., Miller, J., Quinn, D. K., Lloyd, M., Jones, T. R., et al. (2021). Electroconvulsive therapy, electric field, neuroplasticity, and clinical outcomes. Mol. Psychiatry 27, 1676–1682. doi: 10.1038/s41380-021-01380-y
Drevets, W. C., Price, J. L., Simpson, J. R. Jr., Todd, R. D., Reich, T., Vannier, M., et al. (1997). Subgenual prefrontal cortex abnormalities in mood disorders. Nature 386, 824–827. doi: 10.1038/386824a0
Dukart, J., Regen, F., Kherif, F., Colla, M., Bajbouj, M., Heuser, I., et al. (2014). Electroconvulsive therapy-induced brain plasticity determines therapeutic outcome in mood disorders. Proc. Natl. Acad. Sci. U.S.A. 111, 1156–1161. doi: 10.1073/pnas.1321399111
Fan, Q., Witzel, T., Nummenmaa, A., Van Dijk, K. R. A., Van Horn, J. D., Drews, M. K., et al. (2016). MGH-USC human connectome project datasets with ultra-high b-value diffusion MRI. Neuroimage 124(Pt B), 1108–1114. doi: 10.1016/j.neuroimage.2015.08.075
Finnegan, M., and McLoughlin, D. M. (2019). “Cognitive side-effects of ECT,” in The ECT handbook, 4th Edn, eds I. N. Ferrier and J. Waite (Cambridge: Cambridge University Press), 109–120. doi: 10.1017/9781911623175.013
Gbyl, K., Stottrup, M. M., Mitta Raghava, J., Xue Jie, S., and Videbech, P. (2021). Hippocampal volume and memory impairment after electroconvulsive therapy in patients with depression. Acta Psychiatr. Scand. 143, 238–252. doi: 10.1111/acps.13259
Gray, J. A., and McNaughton, N. (2000). The neuropsychology of anxiety: An enquiry into the functions of the septo-hippocampal system. Oxford: Oxford University Press.
Haghighi, M., Sedighinejad, A., Naderi Nabi, B., Emiralavi, C., Biazar, G., Mirmozaffari, K., et al. (2016). The incidence and predictors of headache and myalgia in patients after electroconvulsive therapy (ECT). Anesthesiol. Pain Med. 6:e33724. doi: 10.5812/aapm.33724
Hibar, D. P., Westlye, L. T., van Erp, T. G., Rasmussen, J., Leonardo, C. D., Faskowitz, J., et al. (2016). Subcortical volumetric abnormalities in bipolar disorder. Mol. Psychiatry 21, 1710–1716. doi: 10.1038/mp.2015.227
Holtzheimer, P. E., Husain, M. M., Lisanby, S. H., Taylor, S. F., Whitworth, L. A., McClintock, S., et al. (2017). Subcallosal cingulate deep brain stimulation for treatment-resistant depression: A multisite, randomised, sham-controlled trial. Lancet Psychiatry 4, 839–849. doi: 10.1016/S2215-0366(17)30371-1
Johnston, B. A., Tolomeo, S., Gradin, V., Christmas, D., Matthews, K., and Steele, J. D. (2015). Failure of hippocampal deactivation during loss events in treatment-resistant depression. Brain 138(Pt 9), 2766–2776. doi: 10.1093/brain/awv177
Lee, W. H., Lisanby, S. H., Laine, A. F., and Peterchev, A. V. (2016). Comparison of electric field strength and spatial distribution of electroconvulsive therapy and magnetic seizure therapy in a realistic human head model. Eur. Psychiatry 36, 55–64. doi: 10.1016/j.eurpsy.2016.03.003
Lee, W. H., Lisanby, S. H., Laine, A. F., and Peterchev, A. V. (2017). Minimum electric field exposure for seizure induction with electroconvulsive therapy and magnetic seizure therapy. Neuropsychopharmacology 42, 1192–1200. doi: 10.1038/npp.2016.276
Lisman, J., Buzsaki, G., Eichenbaum, H., Nadel, L., Ranganath, C., and Redish, A. D. (2017). Viewpoints: How the hippocampus contributes to memory, navigation and cognition. Nat. Neurosci. 20, 1434–1447. doi: 10.1038/nn.4661
Malberg, J. E., Hen, R., and Madsen, T. M. (2021). Adult neurogenesis and antidepressant treatment: The surprise finding by Ron Duman and the field 20 years later. Biol. Psychiatry 90, 96–101. doi: 10.1016/j.biopsych.2021.01.010
Mayberg, H. S., Liotti, M., Brannan, S. K., McGinnis, S., Mahurin, R. K., Jerabek, P. A., et al. (1999). Reciprocal limbic-cortical function and negative mood: Converging PET findings in depression and normal sadness. Am. J. Psychiatry 156, 675–682. doi: 10.1176/ajp.156.5.675
Mayberg, H. S., Lozano, A. M., Voon, V., McNeely, H. E., Seminowicz, D., Hamani, C., et al. (2005). Deep brain stimulation for treatment-resistant depression. Neuron 45, 651–660. doi: 10.1016/j.neuron.2005.02.014
Mulder, L. A. C., and Grootens, K. P. (2020). The incidence of post-electroconvulsive therapy headache: A systematic review. J. ECT 36, e22–e28. doi: 10.1097/YCT.0000000000000677
Mulders, P. C. R., Llera, A., Beckmann, C. F., Vandenbulcke, M., Stek, M., Sienaert, P., et al. (2020). Structural changes induced by electroconvulsive therapy are associated with clinical outcome. Brain Stimul. 13, 696–704. doi: 10.1016/j.brs.2020.02.020
Oltedal, L., Narr, K. L., Abbott, C., Anand, A., Argyelan, M., Bartsch, H., et al. (2018). Volume of the human hippocampus and clinical response following electroconvulsive therapy. Biol. Psychiatry 84, 574–581. doi: 10.1016/j.biopsych.2018.05.017
Pagnin, D., de Queiroz, V., Pini, S., and Cassano, G. B. (2004). Efficacy of ECT in depression: A meta-analytic review. J. ECT 20, 13–20. doi: 10.1097/00124509-200403000-00004
Perrin, J. S., Merz, S., Bennett, D. M., Currie, J., Steele, D. J., Reid, I. C., et al. (2012). Electroconvulsive therapy reduces frontal cortical connectivity in severe depressive disorder. Proc. Natl. Acad. Sci. U.S.A. 109, 5464–5468. doi: 10.1073/pnas.1117206109
Peterchev, A. V., Krystal, A. D., Rosa, M. A., and Lisanby, S. H. (2015). Individualized low-amplitude seizure therapy: Minimizing current for electroconvulsive therapy and magnetic seizure therapy. Neuropsychopharmacology 40, 2076–2084. doi: 10.1038/npp.2015.122
Phelps, E. A. (2004). Human emotion and memory: Interactions of the amygdala and hippocampal complex. Curr. Opin. Neurobiol. 14, 198–202. doi: 10.1016/j.conb.2004.03.015
Rosa, M. A., Abdo, G. L., Rosa, M. O., LLisanby, S. H., and Peterchev, A. V. (2012). Frontomedial electrode placement with low current amplitude: a case report (abstract from the 22nd annual meeting of the international society of electroconvulsive therapy and neurostimulation). J. ECT 28:146.
Rupprechter, S., Romaniuk, L., Series, P., Hirose, Y., Hawkins, E., Sandu, A. L., et al. (2020). Blunted medial prefrontal cortico-limbic reward-related effective connectivity and depression. Brain 143, 1946–1956. doi: 10.1093/brain/awaa106
Sartorius, A. (2022). Electric field distribution models in ECT research. Mol. Psychiatry. doi: 10.1038/s41380-022-01516-8
Schmaal, L., Veltman, D. J., van Erp, T. G., Samann, P. G., Frodl, T., Jahanshad, N., et al. (2016). Subcortical brain alterations in major depressive disorder: findings from the ENIGMA major depressive disorder working group. Mol. Psychiatry 21, 806–812. doi: 10.1038/mp.2015.69
SEAN (2021). SEAN Annual Report 2019: A summary of ECT in Scotland for 2009-2018. Available online at: https://www.sean.org.uk/AuditReport/Main.html (accessed March 2, 2022).
Shackman, A. J., Salomons, T. V., Slagter, H. A., Fox, A. S., Winter, J. J., and Davidson, R. J. (2011). The integration of negative affect, pain and cognitive control in the cingulate cortex. Nat. Rev. Neurosci. 12, 154–167. doi: 10.1038/nrn2994
Steele, J. D., and Paulus, M. P. (2019). Pragmatic neuroscience for clinical psychiatry. Br. J. Psychiatry 215, 404–408. doi: 10.1192/bjp.2019.88
Steele, J. D., Christmas, D., Eljamel, M. S., and Matthews, K. (2008). Anterior cingulotomy for major depression: Clinical outcome and relationship to lesion characteristics. Biol. Psychiatry. 63, 670–677. doi: 10.1016/j.biopsych.2007.07.019
Tolomeo, S., Christmas, D., Jentzsch, I., Johnston, B. A., Sprenglemeyer, R., Matthews, K., et al. (2016). A causal role for the anterior mid-cingulate cortex in negative affect and cognitive control. Brain 139, 1844–1854.
Wade, B. S. C., Hellemann, G., Espinoza, R. T., Woods, R. P., Joshi, S. H., Redlich, R., et al. (2020). Depressive symptom dimensions in treatment-resistant major depression and their modulation with electroconvulsive therapy. J. ECT 36, 123–129. doi: 10.1097/YCT.0000000000000623
Whiteford, H. A., Degenhardt, L., Rehm, J., Baxter, A. J., Ferrari, A. J., Erskine, H. E., et al. (2013). Global burden of disease attributable to mental and substance use disorders: findings from the Global Burden of Disease Study 2010. Lancet 382, 1575–1586. doi: 10.1016/S0140-6736(13)61611-6
Keywords: ECT, side-effects, electric fields, depression, computational modeling
Citation: Steele JD, Farnan T, Semple DM and Bai S (2022) Fronto-medial electrode placement for electroconvulsive treatment of depression. Front. Neurosci. 16:1029683. doi: 10.3389/fnins.2022.1029683
Received: 27 August 2022; Accepted: 03 October 2022;
Published: 20 October 2022.
Edited by:
Liming Li, Shanghai Jiao Tong University, ChinaReviewed by:
Yao-Chuan Chang, Feinstein Institute for Medical Research, United StatesCopyright © 2022 Steele, Farnan, Semple and Bai. This is an open-access article distributed under the terms of the Creative Commons Attribution License (CC BY). The use, distribution or reproduction in other forums is permitted, provided the original author(s) and the copyright owner(s) are credited and that the original publication in this journal is cited, in accordance with accepted academic practice. No use, distribution or reproduction is permitted which does not comply with these terms.
*Correspondence: J. Douglas Steele, ZHN0ZWVsZUBkdW5kZWUuYWMudWs=
Disclaimer: All claims expressed in this article are solely those of the authors and do not necessarily represent those of their affiliated organizations, or those of the publisher, the editors and the reviewers. Any product that may be evaluated in this article or claim that may be made by its manufacturer is not guaranteed or endorsed by the publisher.
Research integrity at Frontiers
Learn more about the work of our research integrity team to safeguard the quality of each article we publish.