- 1Department of Breast Medicine, Liaoning Cancer Hospital, Cancer Hospital of China Medical University, Shenyang, China
- 2Department of Pharmacology, Liaoning Cancer Hospital, Cancer Hospital of China Medical University, Shenyang, China
- 3Liaoning Microhealth Biotechnology Co., Ltd., Shenyang, China
- 4Department of Breast Medicine, Cancer Hospital of Dalian University of Technology, Liaoning Cancer Hospital, Shenyang, China
The relationship between gut microbiota (GM) and mental health is one of the focuses of psychobiology research. In recent years, the microbial-gut-brain axis (MGBA) concept has gradually formed about this bidirectional communication between gut and brain. But how the GM is involved in regulating brain function and how they affect emotional disorders these mechanisms are tenuous and limited to animal research, and often controversial. Therefore, in this review, we attempt to summarize and categorize the latest advances in current research on the mechanisms of GM and depression to provide valid information for future diagnoses and therapy of mental disorders. Finally, we introduced some antidepressant regimens that can help restore gut dysbiosis, including classic antidepressants, Chinese materia medica (CMM), diet, and exogenous strains. These studies provide further insight into GM’s role and potential pathways in emotion-related diseases, which holds essential possible clinical outcomes for people with depression or related psychiatric disorders. Future research should focus on clarifying the causal role of GM in disease and developing microbial targets, applying these findings to the prevention and treatment of depression.
Introduction
As early as 1880, Kraepelin established major depressive disorder (MDD) as an independent mental disorder (Kendler, 2017). In addition to some physiological symptoms like weariness and sleep problems, it primarily distinguishes high neuroticism, low self-esteem, severe negative and repetitive thinking, and impaired cognitive reactivity. In more severe cases, delusions and suicidal conduct may also manifest (Struijs et al., 2021). In 2019, the World Health Organization estimated that the global economic cost of anxiety and depression disorders was up to $1 trillion, and this trend expects to rise (Doran and Kinchin, 2019). Although there has been a revolution regarding psychotropic treatments and psychotherapeutics in the last few decades, one-third of patients still do not achieve sustained remission during an MDD episode (Monroe and Harkness, 2022; Rush et al., 2022). Therefore, there is an urgent need to gain new insights into these psychiatric disorders’ underlying pathological mechanisms to help patients alleviate symptoms and improve their quality of life.
The pathogenic factors of MDD are also very complex, including biological, psychological, and social factors (Rostila, 2020; Dudek et al., 2021). Several hypotheses also exist for the pathogenesis of depression, but most of them have some limitations. For example, the most widely accepted theory is the monoamine hypothesis. It argues that insufficient activity of monoaminergic neurons causes depression (Perez-Caballero et al., 2019). Recent clinical findings have demonstrated that up to 30% of individuals do not respond to the monoamine hypothesis class of medications. Moreover, this hypothesis does not explain the response latency of antidepressants (Boku et al., 2018). Subsequently, receptor theory claimed that adaptive changes in receptors could explain this gradual clinical response (Wang H.-Q. et al., 2021). This theory claims that abnormal signal transmission causes abnormal neurotransmitter receptor coupling or changes in downstream signaling cascade responses (Jesulola et al., 2018). However, the durability and safety of drugs targeting receptor therapy (e.g., ketamine) and differences in gender expression of receptors add to the limitations of current treatment (Ma et al., 2018; Rosenblat et al., 2019). This difference in expression brings attention to the genetic level. For the genetic hypothesis, there is much epidemiological evidence for a genetic contribution to MDD susceptibility (Mitchell et al., 2022). Many genes, such as NEGR1, CTC-467M3.3, TMEM106B, etc., have a strong, colocalized and potentially causal association with depression (Dall’Aglio et al., 2021). But it was found that different definitions of depression have distinct genes, which makes it necessary to be more cautious in our research on the genetic studies (Wu et al., 2022). From our literature review, there is no one-size-fits-all statement about the pathophysiological mechanisms of depression. The most likely explanation is that it involves multiple mechanisms and that these mechanisms are interrelated, ultimately manifesting in the human body in a range of pathological symptoms.
Over the past few decades, with advances in sequencing technology, researchers have developed a more profound understanding of the human microbiome. It has also shown that symbiotic microorganisms in the body, especially GM, are involved in regulating the balance between health and disease (Cani, 2018; Sorbara and Pamer, 2022). The latest hypothesis for depression has also evolved into the concept of the MGBA (Chang et al., 2022). This formulation clarifies the existence of a bidirectional signaling network between the brain and the gut and interconnects neural, metabolic, and immune regulatory mechanisms (Tremblay et al., 2021). MDD is also no longer considered to be a single mental condition, according to contemporary psychological and bioinformatics theories. It has obvious biological manifestations, mainly involving four aspects: the dysfunction of the brain, the immune system, the hypothalamus–pituitary–adrenal (HPA) axis, the immune system, and gut-brain dysfunction (Liang et al., 2018). Brain dysfunction mainly manifests as neurotransmitter imbalance (Dalvi-Garcia et al., 2021), impaired neuroplasticity (Tartt et al., 2022), and abnormal neurogenesis (Kim and Park, 2021). Immune system disorders mainly reflect oxidative stress and chronic inflammation (Czarny et al., 2018; Luca et al., 2019). The primary sign of HPA axis dysfunction is the maladjustment of negative feedback mechanisms (Du et al., 2020; Cheiran Pereira et al., 2021). Gut dysbiosis and accompanying gastrointestinal diseases are the main symptoms of gut-brain dysfunction (Oungpasuk et al., 2020; McGuinness et al., 2022). The existing literature suggests that the GM is directly or indirectly involved in the above processes through the MGBA, which is a good inspiration for us to carry out mechanistic studies or search for microbial biomarkers (Barrio et al., 2022; Suda and Matsuda, 2022). This paper reviews the possible mechanisms involved in the pathogenesis of MDD by GM based on some preclinical and clinical studies and discusses potential therapeutic ideas based on regulation of GM, which may provide some reference for future research on the mechanisms of depression prevention, etiology, and treatment.
Characteristic gut microbiota profile in depressive states
Although significant challenges remain in studying host-microbiota interactions, much metabolites-focused research has identified some microbial targets associated with disease progression, including metabolic disease, cardiovascular disease, psychiatric disorders, and others (Wang and Zhao, 2018; Wu et al., 2021). In terms of depression, there is also substantial evidence supporting the critical regulatory role of GM and its metabolites in disease progression (Table 1). Therefore, targeting the GM profile may be an effective strategy to facilitate depression-related pathological features.
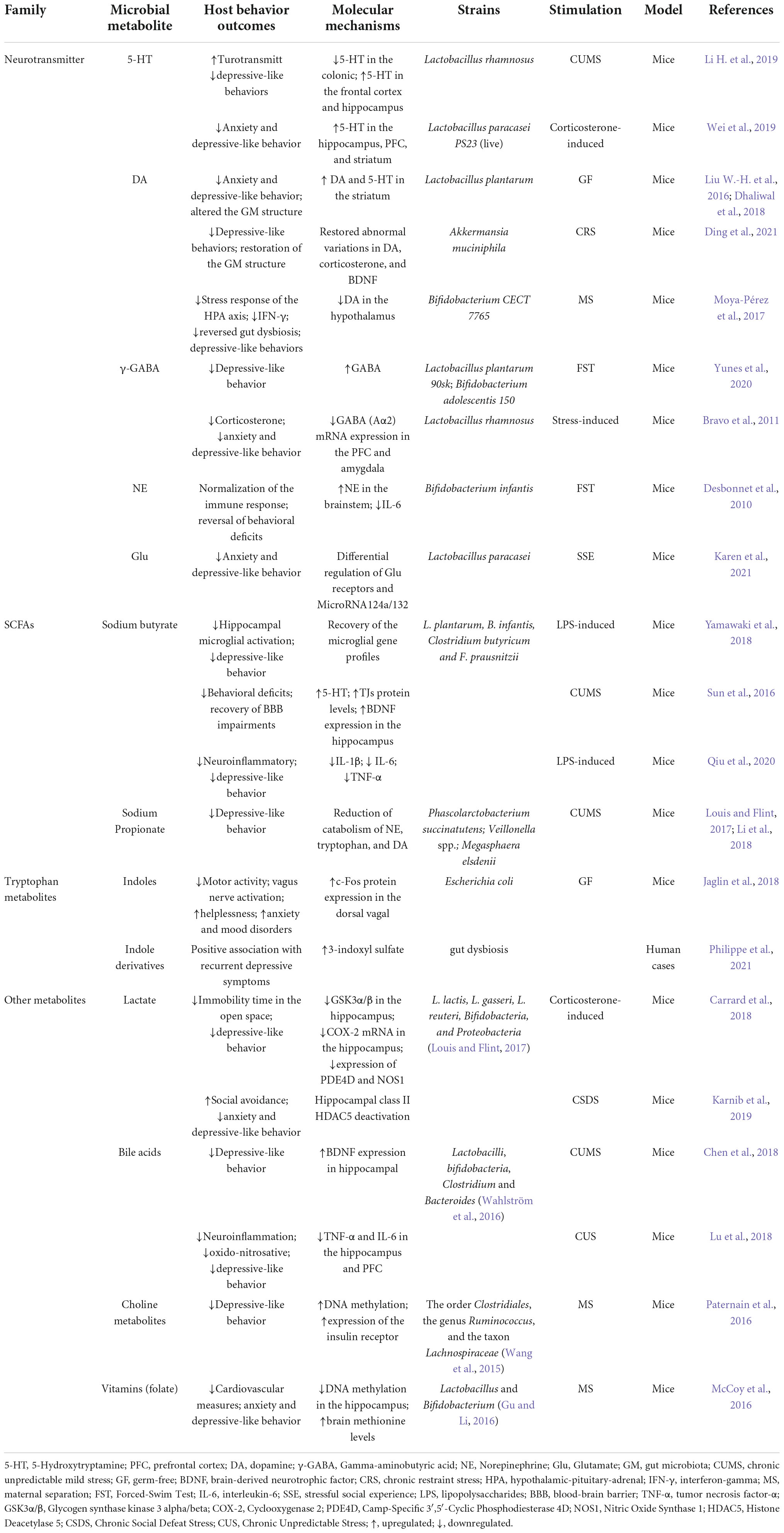
Table 1. Studies investigating the biological link of microbial metabolites on depressive-like behavior.
To explore the characteristic GM composition, many scientists began to take different approaches to establish rodent depression models to observe variations. For example, olfactory bulbectomy (OB), chronic unpredictable mild stress (CUMS), chronic forced swim test, social defeat stress, learned helplessness, etc. (Ramaker and Dulawa, 2017). Correspondingly, we have observed the characteristic changes in these different depressed mouse models (Medina-Rodriguez et al., 2020; Kosuge et al., 2021; Jiang X. et al., 2022; Meng et al., 2022). For example, mice exposed to CUMS exhibited a lower abundance of Lactobacillus and a relatively higher level of Akkermansia (Li N. et al., 2019). Park et al. (2013) found that in addition to gut dysbiosis in OB mice, signaling pathways projecting from the prefrontal cortex (PFC) to the hypothalamus were also impaired. These studies suggest that signals of depression can also be transmitted from top to down.
In addition, some clinical evidence points to the characteristic GM composition of depression (Lai et al., 2021; Zhong et al., 2022). In April 2022, McGuinness et al. (2022) conducted a systematic review of the community composition between 1,038 MDD cases and 1,048 non-MDD controls. In the aspect of species composition, butyrate-producing bacteria significantly reduced in the MDD group, such as Faecalibacterium and Coprococcus (Valles-Colomer et al., 2019). The higher levels of bacteria taxa are mainly associated with the production of lactic acid, glutamate metabolism, and GABA metabolisms, such as Lactobacillus, Enterococcus, and Streptococcus (Lyu et al., 2018; Yogeswara et al., 2020). Although the observations varied considerably between studies at the genus level, the same trends were shown at the phylum and class levels, indicating that there may be some confounding factors in sample selection. In terms of diversity, some studies have come up with various opinions. For example, Bai et al. (2021) suggested that there was no significant difference in alpha diversity (species richness) between MDD patients and healthy controls (Chao, p = 0.62). In contrast, Rong et al. (2019) indicated a significant difference between the two groups, with the MDD group being significantly lower (Chao, p = 0.003). Intriguingly, the populations in both studies were from China, excluding heterogeneity due to region. Recent findings in other psychiatric disorders, such as Parkinson’s disease and autism spectrum disorder, have also reported equivocal alpha diversity findings (Ho et al., 2020; Nuzum et al., 2020). Clearly, alpha diversity is not an appropriate indicator of the mental health status of the host.
Potential mechanism of gut microbiota involved in the regulation of major depressive disorder
Based on the strong correlation between GM and depression, in this section, we describe possible mechanisms by which GM mediates the regulation of MDD. These mechanisms rely mainly on the vagus nerve and peripheral circulation for signaling. Peripheral circulation primarily interferes with intracerebral neurotransmitters, inflammation, HPA axis reactivity, and epigenetic modification processes (Figure 1).
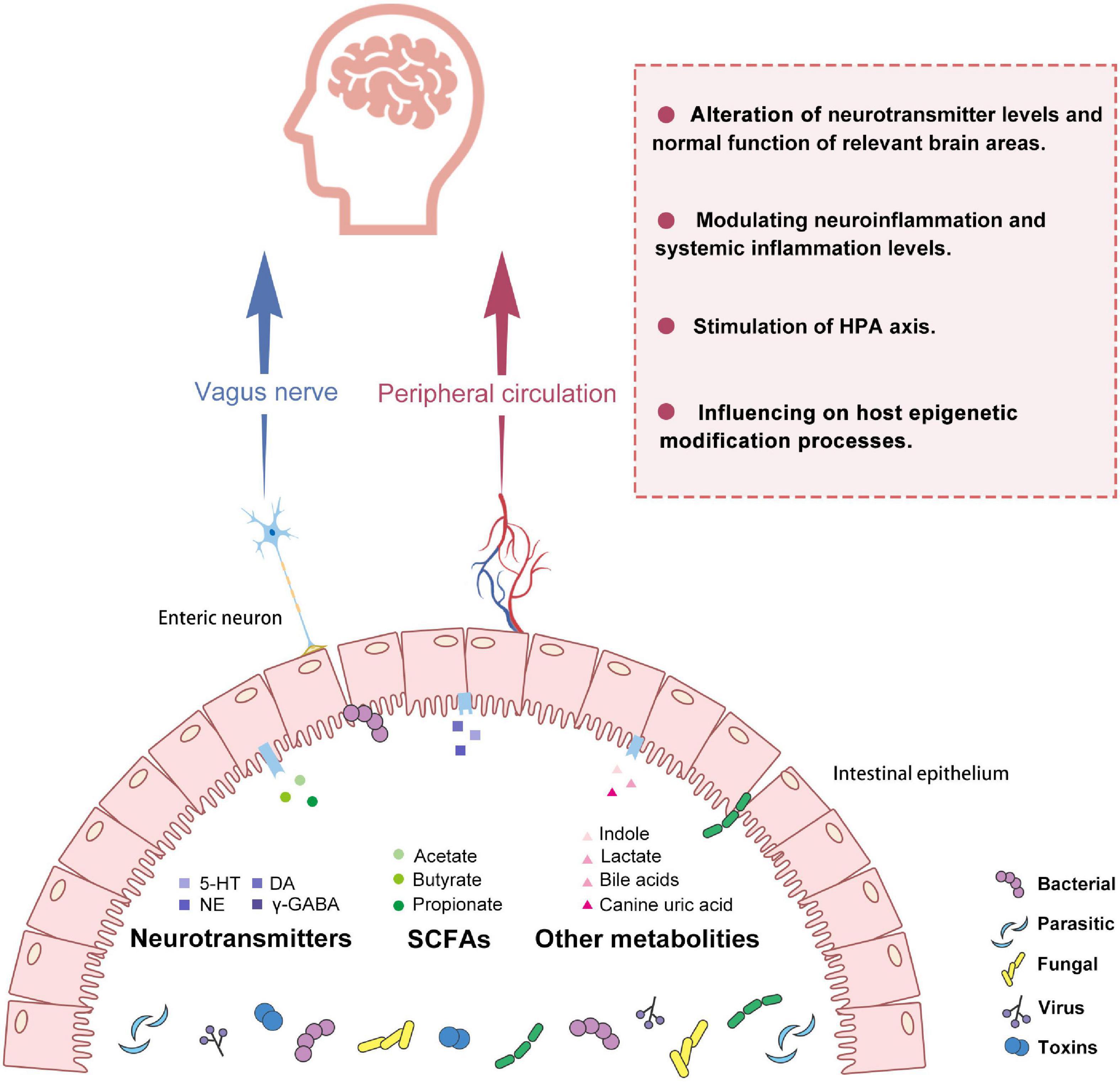
Figure 1. Potential pathways for gut microbiota to make connections with the brain in depression. GM and its metabolites include SCFAs, neurotransmitters, etc., which can act as a bridge to transmit information from the gut to the brain. This communication consists mainly of neural and humoral pathways. On the one hand, it can affect neuroimmune and neuroendocrine mechanisms closely related to the vagus nerve. On the other hand, GM and its metabolites can also cross the intestinal barrier and enter the systemic circulation to modulate host neurotransmitter levels, the immune system, the endocrine system, and epigenetic processes. GM, gut microbiota; 5HT, 5-hydroxytryptamine; DA, Dopamine; NE, Norepinephrine; γ-GABA, Gamma-aminobutyric acid; SCFAs, short-chain fatty acids; HPA, hypothalamic-pituitary-adrenal axis.
Reliance on the vagus nerve for signaling
In the intestine, the enteric nervous system, which consists of numerous enteric neurons, can independently regulate many complex functions (Rao and Gershon, 2016; Li et al., 2020). Enteric neurons locate beneath the intestinal epithelium. They can communicate directly or indirectly with GM, eventually transmitting signals to other body organs (including the CNS) (Cawthon and de La Serre, 2018). Yang et al. (2022) found that severing the subdiaphragmatic vagus nerve could reduce depression-like phenotypes in the depression model mice and had effects on the composition of the GM. Clearly, the subphrenic vagus nerve is one of the pathways of depression signaling. Zhang et al. (2020), Pu et al. (2021), and Wang S. et al. (2021) obtained depressed mouse models by knocking out different genes to perform experiments as above, all of which all confirmed the existence of this pathway. In addition, it has been found that the antidepressant effect of some probiotics (Lactobacillus intestinalis and Lactobacillus reuteri) also depends on the integrity of the vagus nerve (Wang et al., 2020). These studies demonstrate the importance of vagal pathways in depression, but the molecular mechanisms of how GM affects the enteric vagus need to be explored in the future.
Influence on neurotransmitter levels and function of relevant areas
The monoamine hypothesis has focused scientists’ attention on monoamine neurotransmitters such as 5-Hydroxytryptamine (5-HT), dopamine (DA), gamma-aminobutyric acid (γ-GABA), noradrenaline (NE), etc.(Jiang Y. et al., 2022). Some specific genera like Candida, Streptococcus, and Escherichia can directly produce 5-HT to affect peripheral and cerebral 5-HT levels. Under normal physiological conditions, 5-HT cannot pass through the blood-brain barrier (BBB), but under pathological conditions, tryptophan produced by GM can penetrate the impaired BBB through blood circulation. And then, it produces 5-HT precursors in the brain to supplement the 5-HT deficiency in the brain (Makris et al., 2021). Pleasure deficiency is also associated with the dysfunction of the DA system (Rincon-Cortes and Grace, 2020). Tsavkelova et al. (2000) detected the neurotransmitter of microorganisms by high-performance liquid chromatography and found that Bacillus subtilis, Escherichia coli, etc., could produce DA. The administration of Bifidobacterium pseudocatenulatum CECT 7765 has been shown that it can decrease the intestinal hypercatecholaminergic activity of the depressed model and reduce intestinal DA concentrations, ultimately attenuating the excessive stress response of the HPA axis (Moya-Perez et al., 2017; Huang and Wu, 2021). In terms of γ-GABA, it acts as an inhibitory transmitter in regulating multiple functions in the brain, including anxiety, motivation, and the reward system (Petty et al., 1995; Lowes et al., 2021; Zhang et al., 2021). Lactobacillus plantarum and Bifidobacterium adolescentis, ascertained as the most effective GABA-producing bacteria, have antidepressant effects comparable to fluoxetine in mice models (Yunes et al., 2020). In the case of NE, animal studies have shown that Bifidobacterium infantis restores basal NE concentrations in the brainstem and reverses behavioral deficits in depressed mice through the humoral route (Desbonnet et al., 2010). Although many studies have demonstrated the close relationship between GM and these neuroactive metabolites, experimental results have mostly been limited to animal studies. The reproducibility of these mechanisms in clinical studies still needs to be invested in a more in-depth study.
Patients with MDD exhibit dysfunction or hisomorphic disruption in the brain associated with emotions, such as abnormal neurogenesis (Kim and Park, 2021), targeted disruption of the BBB (Dion-Albert et al., 2022), abnormal amygdala and hippocampus function (Price and Duman, 2020; Grogans et al., 2022), impaired structure and function of glial cells (Zhou et al., 2021). Scientists are also investigating the effects between GM and brain-related tissues. The colonization of Bacteroides fragilis, Bacteroides uniformis, has been shown to impair hippocampal neurogenesis and deplete serotonin levels in the brain, exacerbating depressive states (Zhang et al., 2022). GM diversity and composition also affect the release of gut peptides, including cholecystokinin, glucagon-like peptides, and so on. These gut peptides cross the damaged BBB and directly influence the hypothalamic arcuate nucleus, ultimately affecting the normal function of the amygdala and nucleus accumbens, which is associated with mood (Weltens et al., 2018; Merchak and Gaultier, 2020).
Modulating inflammation levels
Clinical studies have shown that MDD patients have a higher serum concentration of IL-1β, IL-4, IL-8, and IL-10. The level of inflammatory markers is also closely related to the severity of depression (Ogłodek, 2022). The close association between inflammation (neuroinflammation and systemic inflammation) and MDD has also been the subject of many in-depth studies (Hayley et al., 2021; Zheng et al., 2021).
In terms of neuroinflammation, the current view focuses more on central microglia (Jia et al., 2021). Some forms of depression are sometimes called microgliopathy (Deng et al., 2020). Erny et al. (2015) observed that microglia in germ-free mice are severely defective and their immune system disorder. Colonization with a complex GM to mice can restore microglia features (Erny et al., 2015). This proves that GM is also involved in shaping the resident immune system in the CNS. In turn, neuroinflammation can affect the normal function of the GM. The inflammatory environment can induce the tryptophan metabolism of the microorganism to be biased toward the kynurenine pathway. And it eventually metabolized to quinolinic acid (Ryan et al., 2020). Neurotoxic quinolinic acid can affect the function of astrocytes and induce depressive symptoms (Öztürk et al., 2021). Significantly, GM can also positively modulate the brain’s inflammation level (Merchak and Gaultier, 2020). The short-chain fatty acids (SCFAs) can be produced by Eubacterium rectale, Roseburia faecis, Eubacterium hallii, etc. It can cross the BBB and act on the aryl hydrocarbon receptors. They are involved in the systemic regulation of cytokine profiles and reduce neuroinflammation (Ortega et al., 2022). Indole derivatives may also play a neuroprotective role by reducing neuroinflammatory markers through activating astrocytes (Rothhammer et al., 2016). For example, Bifidobacterium spp. and Lactobacillus spp. can produce indole-related compounds, Indole-3-lactic acid, and indole-3-aldehyde, which may be related to their antidepressant effects (Roager and Licht, 2018).
How does peripheral inflammation affect the level of intracerebral cytokine levels? There is much evidence that MDD patients have symptoms of increased intestinal permeability (Akdis, 2021). Ohlsson et al. (2019) observed a statistically significant correlation between intestinal permeability markers and inflammatory factors in patients with depression. Alvarez-Mon et al. (2020) continued investigating this correlation and found that bacterial translocation blunted the expansion of regulatory T lymphocytes, thus amplifying the tendency for inflammation. Intestinal symbiont translocation can also drive increased nitrosation to activate immune-inflammatory and oxidative pathways. They negatively affect neurogenesis and synaptic plasticity, ultimately inducing depression (Maes et al., 2019). Increased intestinal permeability further increases the plasma concentration of lipopolysaccharide (LPS). It is also known as endotoxin, cell wall components of Gram-negative bacteria (Kuti et al., 2020). LPS can directly destroy BBB function (Peng et al., 2021). Meanwhile, it can also stimulate the Nuclear factor-k-gene binding pathway and promote cytokine production through binding to Toll-like receptor 4 (Guo et al., 2019). After BBB injury, a large number of cytokines and neurotoxins will enter the brain and further activate the inflammatory response and damage the brain tissue. Moreover, stress can also induce BBB dysfunction (Matsuno et al., 2022). This vicious cycle eventually leads to the onset of depression. Therefore, we believe that some harmful bacteria open channels for immune factors by disrupting the intestinal mucosal barrier and the BBB, which could explain the immune disorders present in the brain of most depressed patients.
Stimulation of hypothalamus–pituitary–adrenal axis
The HPA axis is an essential component of the MGBA and regulates the host stress response (Karaca et al., 2021). MDD patients also exhibit hyperactivity of the HPA axis. It is mainly in the form of increased glandular reactivity, disruption of hormone secretion, and negative feedback dysregulation (Mikulska et al., 2021). These disorders can further lead to intestinal inflammation, neuronal damage, and cortisol overproduction, all of which are associated with depression (Liu et al., 2020). Notably, adrenal glands in the HPA axis secrete cortisol with a circadian rhythm, which develops in parallel with the GM early in life (de Weerth, 2017). It can be seen that there is some complex connection between the GM and the HPA axis.
To date, many mechanisms have been proposed for how GM affects the HPA axis. First, as mentioned in the previous section, there is a close relationship between gut dysbiosis and inflammation. And these cytokines are also very effective activators of the HPA axis (Misiak et al., 2020). Proinflammatory cytokines can interfere with the cortisol cycle’s negative feedback to stimulate the HPA axis’ activity (Zhou et al., 2022). GM can also stimulate the HPA axis through other mediators capable of crossing the BBB, such as microbial antigens and prostaglandins (Mikulska et al., 2021). In addition to relying on these mediators, bacteria can directly participate in the intervention process. For example, LPS and peptidoglycan can activate the HPA axis by activating the innate immune system, which ultimately induces depression (Gonzalez-Santana and Diaz Heijtz, 2020; Smith et al., 2021). There is also evidence that GM can directly modulate steroidogenesis in the gut and adrenal to exaggerate HPA axis response (Vagnerová et al., 2019).
Second, GM-derived metabolites are also involved in regulating the HPA axis through humoral pathways. For instance, chronic indole (Bacteroides spp., Lactobacillus spp., and Clostridium spp.) can induce adrenomedullary Pnmt gene overexpression. And it can increase the catecholamine biosynthesis pathway in the adrenergic system along with noradrenaline. In this way, indole can increase the vulnerability of mice under chronic mild stress, ultimately leading to depressive behavior (Smith and Macfarlane, 1996; Park et al., 2013; Rothhammer et al., 2016; Qu et al., 2019). And beyond that, GM can also play a positive role in depression by modulating the HPA axis. For example, its downstream metabolites, SCFAs, can reduce gene expression of some encoding proteins in the HPA axis, thereby attenuating the stress response of the HPA axis (van de Wouw et al., 2018). Finally, GM can also influence the signal input to the sub-diaphragmatic vagus nerve. The nucleus of the solitary tract activates the HPA axis via noradrenergic neurons (Paton et al., 2000; Herman et al., 2016).
In fact, there is also evidence that the HPA axis also affects the GM in turn. In a study of irritable bowel syndrome, the HPA axis influences GM composition through vagal tone and cortisol homeostasis (Pellissier and Bonaz, 2017). While all of these mechanisms are strongly supported by these animal studies, there is a paucity of population studies. And there is a lack of longitudinal studies, so the causal relationship between the GM and HPA axis needs to be addressed in future studies.
Alteration of epigenetic modifications process in the brain
Because of the heterogeneity of depression, its onset and progression are not well described by a single gene. On the contrary, it is more believed that the clinical manifestations of depression are co-presented by the activation or silencing of many genes caused by environmental factors (gut dysbiosis, diet, infection, etc.), the namely epigenetic mechanism (Alshaya, 2022). The current research mainly involves three pathways: post-translational histone modifications, RNA interference, and DNA methylation (Czarny et al., 2021).
Regarding histone modifications, related studies have pointed out that the acetylation site and the succinylation site on lysine in the hippocampus are close to depression (Liu et al., 2021b; Yu et al., 2021). GM can ease depression through this mechanism. Butyrate, a type of SCFAs, is a typical histone deacetylase inhibitor. It can promote histone acetylation to regulate the expression of proinflammatory factors and BDNF (Couto et al., 2020; Yang et al., 2020). For example, the administration of F. prausnitzii can increase butyrate levels in CUMS mice. The subsequent mechanism suggested that its antidepressant effect is closely related to the protein acetylation and the IL-6/STAT3/IL-17 pathway (Zhou et al., 2018; Hao et al., 2019). In addition, butyrate can also promote BNDF expression levels in the hippocampus. When treated with butyrate-producing bacteria (Bifidobacterium infantis and Clostridium butyricum), recovery of BDNF expression was observed in depressed mouse models (Sun et al., 2018; Tian et al., 2019). A multicenter clinical trial study also provided sufficient evidence (Kim et al., 2021). Moreover, related research shows that Lactobacillus rhamnosus can reduce hippocampal GABAAα 2 mRNA expression in the depressive model (McVey Neufeld et al., 2019). However, whether this mechanism is also dependent on histone modification needs further evidence.
GM can also influence host mental health by affecting specific miRNA levels (Rosa et al., 2022). Liu et al. (2021a) examined relevant RNA levels in mice colonized with fecal from MDD patients. And they found that 200 mRNAs, 358 lncRNAs, and 4 miRNAs were differentially expressed compared to the control group. Functional analysis showed that these differential mRNAs were associated with inflammatory response (Liu et al., 2021a). Some probiotics, such as Lactobacillus paracasei, have also been shown to prevent early life stress-induced depression-like behavior by mediating differential regulation of microRNA124a/132 and glutamate receptors (Karen et al., 2021). Finally, affecting host DNA methylation is a necessary mechanism as well. In the study of Wei et al. (2014), sodium butyrate can up-regulate the expression level of TET1mRNA in the PFC of depressed mice, playing an antidepressant-like role. Subsequent mechanistic studies confirmed that this epigenetic effect is associated with TET1-mediated increased and decreased methylation of BDNF (Wei et al., 2014).
Treatment of depression from the perspective of gut microbiota
Based on the influential role of GM in the occurrence and pathogenesis of depression, we have noted that GM shows a powerful research potential in the study of antidepressant treatment, whether as an independent treatment or an auxiliary measure (Table 2). This section reviews some antidepressant approaches in regulating the GM structure, including classical antidepressants, CMM, dietary, and exogenous strains (Figure 2).
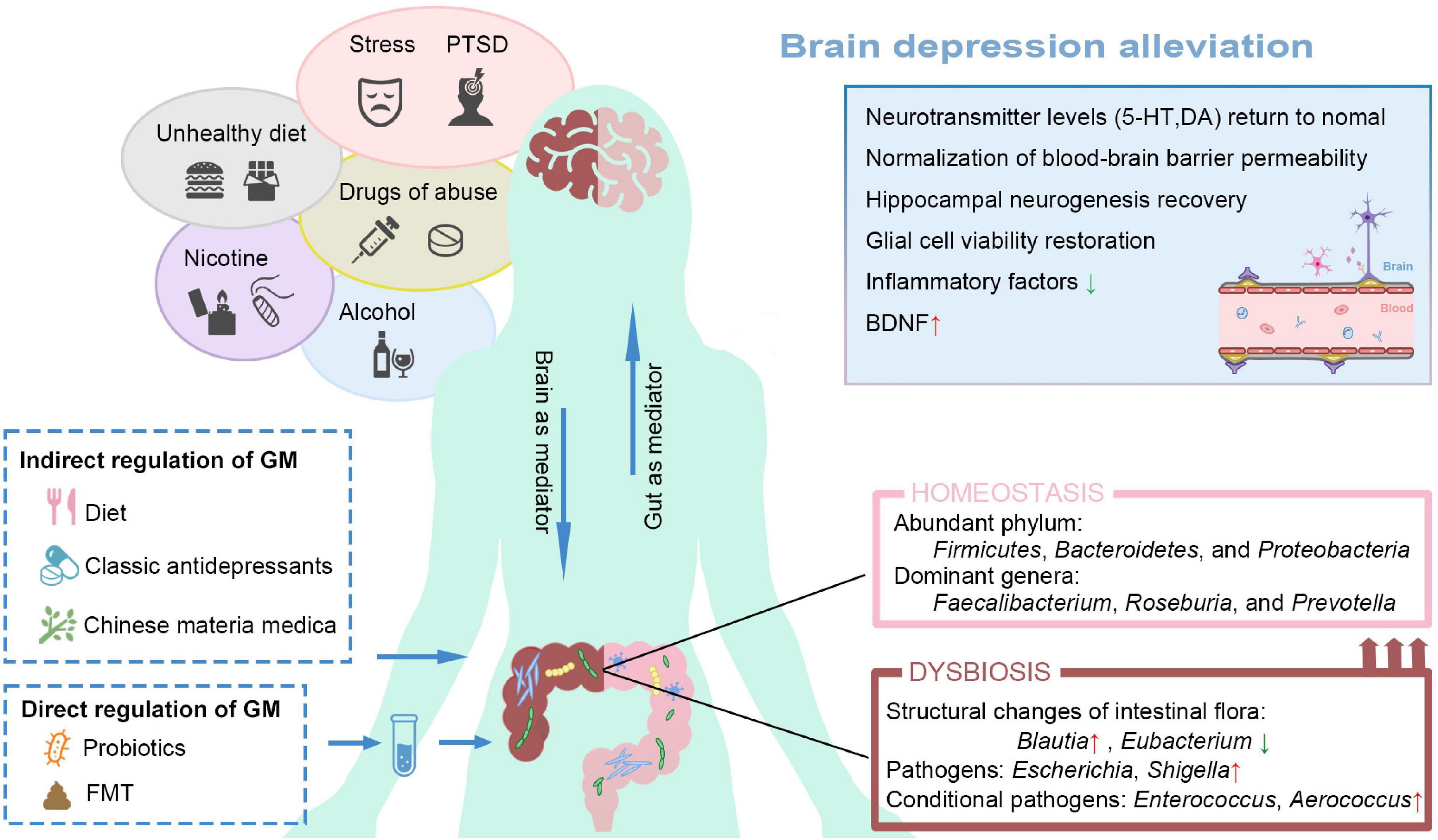
Figure 2. Ameliorates depression from the perspective of restructuring the GM. Poor lifestyle habits and external stressors can increase the risk of depression. MDD patients also exhibit gut dysbiosis. Restore intestinal homeostasis can be restored through direct or indirect regimens, thus alleviating depressive symptoms. GM, gut microbiota; 5HT, 5-hydroxytryptamine; DA, Dopamine; BDNF, brain-derived neurotrophic factor; PTSD, post-traumatic stress disorder; FMT, Fecal Microbiota Transplantation.
Classic antidepressants
Antidepressants have been used for a long time, but the effectiveness of antidepressants varies widely among individuals. To study the biological mechanisms behind this, Lukiæ et al. (2019) used three antidepressants (SSRI, SNRI, NRI) to treat depressed mice. They analyzed variations in GM by 16s rRNA gene sequencing. The results show that it all can alter the composition of the GM; only the specific strains and the degree of impact differ (Lukiæ et al., 2019; Sun et al., 2019). We hypothesized that the strength of antidepressant efficacy might be related to the ability to restore GM homeostasis.
Ketamine, the classic drug for treatment-resistant depression, substantial evidence has indicated that some of its antidepressant effects may be related to the restoration of GM (Hua et al., 2022). Huang et al. (2019) found that ketamine significantly improved the species diversity (α-diversity) of the GM of depressed mice. The β-diversity analysis also indicated a significantly different profile after ketamine administration (Huang et al., 2019). Yang et al. (2017) further investigated the two ketamine enantiomers and found that (R)-ketamine significantly reduced Mollicutes and butyricimonas levels in CSDS mice compared to (S)-ketamine. This may explain why (R)-ketamine has a greater and longer-lasting potency than (S)-ketamine. Other studies have shown that (R)-ketamine-mediated changes in GM are associated with the activation of microglia and bone mineral density, which is also inextricably linked to depression (Wan et al., 2022; Wang et al., 2022). Other first-line nervous systems drugs, such as sertraline, imipramine, and chlorpromazine, have also shown regulatory potential in these pathways in the GM. These drugs can significantly increase the levels of GABA and DA and reduce the glutamic acid metabolism of GM (Ma et al., 2021). The antibiotic rifaximin can also increase the relative abundance of Ruminococci, and Spirillaceae, increasing butyrate concentration in the brain (Li et al., 2021).
In addition to antidepressants modulating the abundance of specific strains, antidepressant efficacy is also influenced by GM. For example, Adlercreutzia equolifaciens can diminish the therapeutic effect of duloxetine (Lukiæ et al., 2019). It is important to note that the antibiotic-like effects of psychotropic drugs on GM are sometimes overlooked. Drugs inhibit the growth of GM, thereby inducing dysbiosis and causing disease progression, which may be an actual reason for the poor outcome of current clinical treatment (Ait Chait et al., 2021).
Chinese materia medica
As early as the Han Dynasty in China, Zhang Zhongjing documented classical herbal formulas for the treatment of depression, but the specific pharmacology mechanism of the recipe is unclear (Chi et al., 2019). In recent studies, the antidepressant-like effects exerted by some CMM have been shown to correlate with GM. For example, Shugan Granule can significantly improve abnormal behavior and inflammation in the hippocampus of chronic restraint stress mice. Follow-up mechanistic studies have shown that its administration enriches Butyricimonas and Candidatus Arthromitus in the gut of mice, reduces the abundance of Bacteroides, and is closely associated with the PI3K/Akt/mTOR pathway (Li et al., 2022). Schisandra has demonstrated that it can attenuate gut dysbiosis in depressive mice by inhibiting TLR4/NF-κB signaling pathway (Sun et al., 2020). In addition to modulating inflammation, CMM also plays a role in regulating neurotransmitter levels. Neferine treatment can reduce the immobility time of depressed mice and increase the neurotransmitters in the hippocampus, such as DA, 5-HT, and NE. Meanwhile, an increase in the relative abundance of Lactobacillus in the mice colon was also observed (Dong et al., 2022). In another study, the targets of specific antidepressant CMM can focus on the regulation of purine metabolism (Ji et al., 2022). Animal studies related to CMM, such as cistanche tuberosa (Fan et al., 2021) and crocetin (Lin et al., 2021), have indicated that their antidepressant-like effects are closely related to the regulation of GM composition.
In addition to animal studies, clinical studies have provided corresponding evidence. Shao et al. (2021) interfered with the herbal formula Xiao-Chai-Hu-Tang for cancer patients with depression. After administration, depressive symptoms were lessened, and gut dysbiosis was partially reversed in subjects (particularly reducing abundances of Parabacteroides, Blautia, and Ruminococcaceae bacterium). Interestingly, this anti-depressive herbal medicine also exhibited some antitumor effects, with potential mechanisms involving the TLR4/MyD88/NF-κB signaling (Shao et al., 2021). Notably, these pathways also play an essential role in the pathogenesis of depression involving microbiota. Recent systematic reviews and meta-analyses have also shown that CMM reduces adverse events compared to antidepressants (Wang et al., 2019). Given the limited number of current studies, it is premature to summarize the benefits and risks of CMM for depression accurately.
Dietary
Nutritional psychiatry supports the notion that dietary therapy can relieve depressive symptoms and promote brain health (Marx et al., 2021). At the same time, long-term dietary patterns also influence human GM (Bolte et al., 2021). Yan et al. (2021) found that maternal consumption of sulforaphane glucosinolate during pregnancy and lactation can affect the GM diversity of the offspring. This effect reduces the likelihood of stress-related psychiatric disorders in the offspring (Wei et al., 2022). In addition, specific types of dietary such as the Mediterranean diet (mainly fiber, fish, and whole grains), have been shown to modulate the GM composition by increasing the abundance of microorganisms that metabolize SCFAs, thereby reducing the onset of depression (Wei et al., 2022). Ketogenic diets (low carbohydrate, moderate protein, high fat) can help improve the efficiency of monoaminergic drugs by increasing GABA levels. And it also influences GM composition, such as an increased abundance of Enterobacteriaceae, Akkermansia, and Slackia, as well as a decreased abundance of Bifidobacteria and Lachnobacterium (Włodarczyk et al., 2021). An interventional randomized clinical trial demonstrated that flavonoid-rich orange juice could alleviate depression by increasing BDNF and the Trichophyton family (Ortega et al., 2022). Polyphenols have also been shown to ameliorate depressive symptoms by inhibiting the mitogen-activated protein kinase pathway involved in oxidative stress and inflammation (Behl et al., 2022). Polyphenols also act as prebiotics to provide nutrients for microorganisms, which complement each other (Zhou et al., 2020). A healthy dietary pattern will enhance the abundance of beneficial genera and thus reduce the risk of depression. We believe that future nutritional intervention studies also need to consider the regulation of GM as an essential part.
Direct intervention in microbiota structure
Psychobiotics for depression, probiotics
Recent meta-analyses and systematic reviews have confirmed the antidepressant efficacy of probiotics in clinical studies (Amirani et al., 2020; Nikolova et al., 2021). Relevant animal studies have also shown that this effect is related to the regulation of the structure of the GM. Marin et al. (2017) used Lactobacillus reuteri to intervene in CUMS mice and found that only restoring Lactobacillus levels was sufficient to improve the metabolic changes and behavioral abnormalities associated with stress. In another study, Heat-sterilized Bifidobacterium shortum can modulate GM composition and thus prevent the depressive symptoms induced by chronic social defeat stress. Such strains with functional food components could be used as novel therapies (Kosuge et al., 2021; Tian et al., 2022). Of note, studies have shown that co-administration of probiotics and prebiotics can achieve a greater antidepressant effect (Moludi et al., 2022). Although some studies have found no significant improvement in depressive symptoms with prebiotics alone, it is possible that the substantial nutritional effect of prebiotics increases the survival rate of probiotics and thus amplifies the antidepressant ability of probiotics (Markowiak and Śliżewska, 2017; Alli et al., 2022; Karbownik et al., 2022). No relevant studies have reported problems such as withdrawal reactions and side effects caused by probiotics, which appear to be a promising intervention in depression. The optimal combination of probiotic strains, doses, and methods is not yet precise. To solve these problems, additional randomized, double-blind, placebo-controlled trials are needed to unravel the puzzle.
Fecal microbiota transplantation
Another way to directly interfere with GM is fecal microbiota transplantation (FMT). Its excellent performance in depression and microbial structure disorders has led us to see new treatment orientations (Bakken et al., 2011; Fond et al., 2020). In an animal study by Zhang et al. (2019), transplantation from NLRP3 KO flora significantly ameliorated depression-like behavior in recipient mice. In their inquiry, the FMT mechanism primarily relies on the inhibition of circHIPK2 expression in depressive mice. In human subjects, FMT also showed similar effects. In a case report of FMT as an adjunctive treatment for depression, the patients enrolled showed an improvement in depressive symptoms, an increase in GM diversity, and a relief in gastrointestinal symptoms. However, the effect was not long-lasting, and they speculated that the efficacy of FMT was related to the microbial similarity of the donor and the recipient (Doll et al., 2022). The main challenge for FMT is enhancing the success rate, the optimal delivery route, donor selection, and other issues that need to be explored. Our perceptions and insights are still growing.
Recently, a novel type of transplant that is also an alternative to FMT, Microbial Ecosystem Therapeutic (MET)-2, demonstrated good antidepressant results in phase 2, a double-blind, placebo-controlled study. An increase in the abundance and diversity of beneficial bacteria was observed in the MET-2 group gut, suggesting that the use of MET-2 may promote the repopulation of the GM and modulate the healthy genus associated with MGBA (Chinna Meyyappan et al., 2021). However, the study’s sample size was too small, and a larger multicenter trial is needed to validate the feasibility of MET-2. In addition, Liu S. et al. (2016) showed that fecal microRNA could regulate inter-species genes to facilitate the host GM. We speculate that transplantation of depression-related miRNA (has-miR-139-3p, miRNAs-let-7e-5p, has-let-7f-5p, etc.) restored to their normal levels may also have a therapeutic effect, which requires further animal and clinical studies to verify (Gecys et al., 2022).
Summary and perspective
In summary, depression can alter the composition of the GM, and gut dysbiosis can further exacerbate depression’s severity. This tight inter-regulatory link has increased the possibility of alleviating depression by regulating GM. Therefore, we have summarized and integrated current studies on possible pathophysiological mechanisms of GM involved in depression and exemplified existing alleviating regimens related to GM. We hope to provide some reference for future exploration of microbiota targeting measures.
In future research, we need to standardize assessing depression degree, which is related to the accuracy and reproducibility of experimental results of depression-related mechanisms. Notably, more longitudinal and human studies are required to fill in the gaps in current knowledge. In addition, understanding the dynamics of microbial metabolites in vivo remains a significant challenge. As more studies are conducted, it is expected that the pathogenesis of depression will be further elucidated in the future, laying the foundation for developing microbiome-based therapies.
Author contributions
JX and TS conceived and designed the review. WH and JX wrote the draft of manuscript. MH and NW collected the information. MB worked on the electronic manuscript of all the figures in this review. All authors read and approved the final manuscript and submission of this manuscript.
Funding
This work was supported by the CSCO Project (Y-2019Genecast-019, JX), the Liaoning Cancer Hospital Yangtse River Scholars Project (JX), the Medical Engineering Cross Research Fund between Liaoning Cancer Hospital and Dalian University of Technology (LD202022, TS), the LiaoNing Revitalization Talents Program (XLYC1907160, JX), and the Fundamental Research Funds for the Central Universities (2022029, TS; 2022030, JX).
Conflict of interest
MB was employed by the company Liaoning Microhealth Biotechnology Co., Ltd.
The remaining authors declare that the research was conducted in the absence of any commercial or financial relationships that could be construed as a potential conflict of interest.
Publisher’s note
All claims expressed in this article are solely those of the authors and do not necessarily represent those of their affiliated organizations, or those of the publisher, the editors and the reviewers. Any product that may be evaluated in this article, or claim that may be made by its manufacturer, is not guaranteed or endorsed by the publisher.
References
Ait Chait, Y., Mottawea, W., Tompkins, T. A., and Hammami, R. (2021). Nutritional and therapeutic approaches for protecting human gut microbiota from psychotropic treatments. Prog. Neuropsychopharmacol. Biol. Psychiatry 108:110182. doi: 10.1016/j.pnpbp.2020.110182
Akdis, C. A. (2021). Does the epithelial barrier hypothesis explain the increase in allergy, autoimmunity and other chronic conditions? Nat. Rev. Immunol. 21, 739–751. doi: 10.1038/s41577-021-00538-7
Alli, S. R., Gorbovskaya, I., Liu, J. C. W., Kolla, N. J., Brown, L., and Müller, D. J. (2022). The Gut Microbiome in depression and potential benefit of prebiotics, probiotics and synbiotics: A systematic review of clinical trials and observational studies. Int. J. Mol. Sci. 23:4494. doi: 10.3390/ijms23094494
Alshaya, D. S. (2022). Genetic and epigenetic factors associated with depression: An updated overview. Saudi J. Biol. Sci. 29:103311. doi: 10.1016/j.sjbs.2022.103311
Alvarez-Mon, M. A., Gomez-Lahoz, A. M., Orozco, A., Lahera, G., Sosa-Reina, M. D., Diaz, D., et al. (2020). Blunted expansion of regulatory T lymphocytes is associated with increased bacterial translocation in patients with major depressive disorder. Front. Psychiatry 11:591962. doi: 10.3389/fpsyt.2020.591962
Amirani, E., Milajerdi, A., Mirzaei, H., Jamilian, H., Mansournia, M. A., Hallajzadeh, J., et al. (2020). The effects of probiotic supplementation on mental health, biomarkers of inflammation and oxidative stress in patients with psychiatric disorders: A systematic review and meta-analysis of randomized controlled trials. Complement. Therap. Med. 49:102361. doi: 10.1016/j.ctim.2020.102361
An, Q., Li, C., Chen, Y., Yang, Y., Song, R., Zhou, L., et al. (2020). Scaffold hopping of agomelatine leads to enhanced antidepressant effects by modulation of gut microbiota and host immune responses. Pharmacol. Biochem. Behav. 192:172910. doi: 10.1016/j.pbb.2020.172910
Bai, S., Xie, J., Bai, H., Tian, T., Zou, T., and Chen, J.-J. (2021). Gut microbiota-derived inflammation-related serum metabolites as potential biomarkers for major depressive disorder. J. Inflammat. Res. 14, 3755–3766. doi: 10.2147/JIR.S324922
Bakken, J. S., Borody, T., Brandt, L. J., Brill, J. V., Demarco, D. C., Franzos, M. A., et al. (2011). Treating clostridium difficile infection with fecal microbiota transplantation. Clin. Gastroenterol. Hepatol. 9, 1044–1049. doi: 10.1016/j.cgh.2011.08.014
Barrio, C., Arias-Sánchez, S., and Martín-Monzón, I. (2022). The gut microbiota-brain axis, psychobiotics and its influence on brain and behaviour: A systematic review. Psychoneuroendocrinology 137:105640. doi: 10.1016/j.psyneuen.2021.105640
Behl, T., Rana, T., Alotaibi, G. H., Shamsuzzaman, M., Naqvi, M., Sehgal, A., et al. (2022). Polyphenols inhibiting MAPK signalling pathway mediated oxidative stress and inflammation in depression. Biomed. Pharmacother. Biomed. Pharmacother. 146:112545. doi: 10.1016/j.biopha.2021.112545
Boku, S., Nakagawa, S., Toda, H., and Hishimoto, A. (2018). Neural basis of major depressive disorder: Beyond monoamine hypothesis. Psychiatry Clin. Neurosci. 72, 3–12. doi: 10.1111/pcn.12604
Bolte, L. A., Vich Vila, A., Imhann, F., Collij, V., Gacesa, R., Peters, V., et al. (2021). Long-term dietary patterns are associated with pro-inflammatory and anti-inflammatory features of the gut microbiome. Gut 70, 1287–1298. doi: 10.1136/gutjnl-2020-322670
Bravo, J. A., Forsythe, P., Chew, M. V., Escaravage, E., Savignac, H. M., Dinan, T. G., et al. (2011). Ingestion of Lactobacillus strain regulates emotional behavior and central GABA receptor expression in a mouse via the vagus nerve. Proc. Natl. Acad. Sci. U.S.A. 108, 16050–16055. doi: 10.1073/pnas.1102999108
Cani, P. D. (2018). Human gut microbiome: Hopes, threats and promises. Gut 67, 1716–1725. doi: 10.1136/gutjnl-2018-316723
Cao, C., Liu, M., Qu, S., Huang, R., Qi, M., Zhu, Z., et al. (2020). Chinese medicine formula Kai-Xin-San ameliorates depression-like behaviours in chronic unpredictable mild stressed mice by regulating gut microbiota-inflammation-stress system. J. Ethnopharmacol. 261:113055. doi: 10.1016/j.jep.2020.113055
Carrard, A., Elsayed, M., Margineanu, M., Boury-Jamot, B., Fragnière, L., Meylan, E. M., et al. (2018). Peripheral administration of lactate produces antidepressant-like effects. Mol. Psychiatry 23, 392–399. doi: 10.1038/mp.2016.179
Cawthon, C. R., and de La Serre, C. B. (2018). Gut bacteria interaction with vagal afferents. Brain Res. 1693(Pt B), 134–139. doi: 10.1016/j.brainres.2018.01.012
Chang, L., Wei, Y., and Hashimoto, K. (2022). Brain-gut-microbiota axis in depression: A historical overview and future directions. Brain Res. Bull. 182, 44–56. doi: 10.1016/j.brainresbull.2022.02.004
Cheiran Pereira, G., Piton, E., Moreira Dos Santos, B., Ramanzini, L. G., Muniz Camargo, L. F., Menezes, et al. (2021). Microglia and HPA axis in depression: An overview of participation and relationship. World J. Biol. Psychiatry 23, 165–182. doi: 10.1080/15622975.2021.1939154
Chen, W.-G., Zheng, J.-X., Xu, X., Hu, Y.-M., and Ma, Y.-M. (2018). Hippocampal FXR plays a role in the pathogenesis of depression: A preliminary study based on lentiviral gene modulation. Psychiatry Res. 264, 374–379. doi: 10.1016/j.psychres.2018.04.025
Chi, X., Wang, S., Baloch, Z., Zhang, H., Li, X., Zhang, Z., et al. (2019). Research progress on classical traditional chinese medicine formula lily bulb and rehmannia decoction in the treatment of depression. Biomed. Pharmacother. Biomed. Pharmacother. 112:108616. doi: 10.1016/j.biopha.2019.108616
Chinna Meyyappan, A., Sgarbossa, C., Vazquez, G., Bond, D. J., Muller, D. J., and Milev, R. (2021). The safety and efficacy of microbial ecosystem therapeutic-2 in people with major depression: Protocol for a phase 2, double-blind, placebo-controlled study. JMIR Res. Protoc. 10:e31439. doi: 10.2196/31439
Couto, M. R., Gonçalves, P., Magro, F., and Martel, F. (2020). Microbiota-derived butyrate regulates intestinal inflammation: Focus on inflammatory bowel disease. Pharmacol. Res. 159:104947. doi: 10.1016/j.phrs.2020.104947
Czarny, P., Bialek, K., Ziolkowska, S., Strycharz, J., Barszczewska, G., and Sliwinski, T. (2021). The importance of epigenetics in diagnostics and treatment of major depressive disorder. J. Pers. Med. 11:167. doi: 10.3390/jpm11030167
Czarny, P., Wigner, P., Galecki, P., and Sliwinski, T. (2018). The interplay between inflammation, oxidative stress, DNA damage, DNA repair and mitochondrial dysfunction in depression. Prog. Neuropsychopharmacol. Biol. Psychiatry 80(Pt C), 309–321. doi: 10.1016/j.pnpbp.2017.06.036
Dall’Aglio, L., Lewis, C. M., and Pain, O. (2021). Delineating the genetic component of gene expression in major depression. Biol. Psychiatry 89, 627–636. doi: 10.1016/j.biopsych.2020.09.010
Dalvi-Garcia, F., Fonseca, L. L., Vasconcelos, A. T. R., Hedin-Pereira, C., and Voit, E. O. (2021). A model of dopamine and serotonin-kynurenine metabolism in cortisolemia: Implications for depression. PLoS Comput. Biol. 17:e1008956. doi: 10.1371/journal.pcbi.1008956
de Weerth, C. (2017). Do bacteria shape our development? Crosstalk between intestinal microbiota and HPA axis. Neurosci. Biobehav. Rev. 83, 458–471. doi: 10.1016/j.neubiorev.2017.09.016
Deng, S.-L., Chen, J.-G., and Wang, F. (2020). Microglia: A central player in depression. Curr. Med. Sci. 40, 391–400. doi: 10.1007/s11596-020-2193-1
Desbonnet, L., Garrett, L., Clarke, G., Kiely, B., Cryan, J. F., and Dinan, T. G. (2010). Effects of the probiotic Bifidobacterium infantis in the maternal separation model of depression. Neuroscience 170, 1179–1188. doi: 10.1016/j.neuroscience.2010.08.005
Dhaliwal, J., Singh, D. P., Singh, S., Pinnaka, A. K., Boparai, R. K., Bishnoi, M., et al. (2018). Lactobacillus plantarum MTCC 9510 supplementation protects from chronic unpredictable and sleep deprivation-induced behaviour, biochemical and selected gut microbial aberrations in mice. J. Appl. Microbiol. 125, 257–269. doi: 10.1111/jam.13765
Ding, Y., Bu, F., Chen, T., Shi, G., Yuan, X., Feng, Z., et al. (2021). A next-generation probiotic: Akkermansia muciniphila ameliorates chronic stress-induced depressive-like behavior in mice by regulating gut microbiota and metabolites. Appl. Microbiol. Biotechnol. 105, 8411–8426. doi: 10.1007/s00253-021-11622-2
Dion-Albert, L., Cadoret, A., Doney, E., Kaufmann, F. N., Dudek, K. A., Daigle, B., et al. (2022). Vascular and blood-brain barrier-related changes underlie stress responses and resilience in female mice and depression in human tissue. Nat. Commun. 13:164. doi: 10.1038/s41467-021-27604-x
Doll, J. P. K., Vazquez-Castellanos, J. F., Schaub, A. C., Schweinfurth, N., Kettelhack, C., Schneider, E., et al. (2022). Fecal microbiota transplantation (FMT) as an adjunctive therapy for depression-case report. Front. Psychiatry 13:815422. doi: 10.3389/fpsyt.2022.815422
Dong, Z., Xie, Q., Xu, F., Shen, X., Hao, Y., Li, J., et al. (2022). Neferine alleviates chronic stress-induced depression by regulating monoamine neurotransmitter secretion and gut microbiota structure. Front. Pharmacol. 13:974949. doi: 10.3389/fphar.2022.974949
Doran, C. M., and Kinchin, I. (2019). A review of the economic impact of mental illness. Austr. Health Rev.Publicat. Austr. Hosp. Associat. 43, 43–48. doi: 10.1071/AH16115
Du, Y., Gao, X.-R., Peng, L., and Ge, J.-F. (2020). Crosstalk between the microbiota-gut-brain axis and depression. Heliyon 6:e04097. doi: 10.1016/j.heliyon.2020.e04097
Dudek, K. A., Dion-Albert, L., Kaufmann, F. N., Tuck, E., Lebel, M., and Menard, C. (2021). Neurobiology of resilience in depression: Immune and vascular insights from human and animal studies. Eur. J. Neurosci. 53, 183–221. doi: 10.1111/ejn.14547
Erny, D., Hrabì de Angelis, A. L., Jaitin, D., Wieghofer, P., Staszewski, O., David, E., et al. (2015). Host microbiota constantly control maturation and function of microglia in the CNS. Nat. Neurosci. 18, 965–977. doi: 10.1038/nn.4030
Fan, L., Peng, Y., Wang, J., Ma, P., Zhao, L., and Li, X. (2021). Total glycosides from stems of Cistanche tubulosa alleviate depression-like behaviors: Bidirectional interaction of the phytochemicals and gut microbiota. Phytomedicine Int. J. Phytother. Phytopharmacol. 83:153471. doi: 10.1016/j.phymed.2021.153471
Fond, G. B., Lagier, J.-C., Honore, S., Lancon, C., Korchia, T., Sunhary De Verville, P.-L., et al. (2020). Microbiota-orientated treatments for major depression and schizophrenia. Nutrients 12:1024. doi: 10.3390/nu12041024
Gecys, D., Dambrauskiene, K., Simonyte, S., Patamsyte, V., Vilkeviciute, A., Musneckis, A., et al. (2022). Circulating hsa-let-7e-5p and hsa-miR-125a-5p as possible biomarkers in the diagnosis of major depression and bipolar disorders. Dis. Mark. 2022:3004338. doi: 10.1155/2022/3004338
Gonzalez-Santana, A., and Diaz Heijtz, R. (2020). Bacterial Peptidoglycans from microbiota in neurodevelopment and behavior. Trends Mol. Med. 26, 729–743. doi: 10.1016/j.molmed.2020.05.003
Grogans, S. E., Fox, A. S., and Shackman, A. J. (2022). The amygdala and depression: A sober reconsideration. Am. J. Psychiatry 179, 454–457. doi: 10.1176/appi.ajp.20220412
Gu, Q., and Li, P. (2016). Biosynthesis of vitamins by probiotic bacteria,” in Probiotics and prebiotics in human nutrition and health. London: In tech open, 135–148.
Guo, L.-T., Wang, S.-Q., Su, J., Xu, L.-X., Ji, Z.-Y., Zhang, R.-Y., et al. (2019). Baicalin ameliorates neuroinflammation-induced depressive-like behavior through inhibition of toll-like receptor 4 expression via the PI3K/AKT/FoxO1 pathway. J. Neuroinflam. 16:95. doi: 10.1186/s12974-019-1474-8
Han, S.-K., Kim, J.-K., Park, H.-S., Shin, Y.-J., and Kim, D.-H. (2021). Chaihu-Shugan-San (Shihosogansan) alleviates restraint stress-generated anxiety and depression in mice by regulating NF-κB-mediated BDNF expression through the modulation of gut microbiota. Chin. Med. 16:77. doi: 10.1186/s13020-021-00492-5
Hao, Z., Wang, W., Guo, R., and Liu, H. (2019). Faecalibacterium prausnitzii (ATCC 27766) has preventive and therapeutic effects on chronic unpredictable mild stress-induced depression-like and anxiety-like behavior in rats. Psychoneuroendocrinology 104, 132–142. doi: 10.1016/j.psyneuen.2019.02.025
Hayley, S., Hakim, A. M., and Albert, P. R. (2021). Depression, dementia and immune dysregulation. Brain J. Neurol. 144, 746–760. doi: 10.1093/brain/awaa405
Herman, J. P., McKlveen, J. M., Ghosal, S., Kopp, B., Wulsin, A., Makinson, R., et al. (2016). Regulation of the hypothalamic-pituitary-adrenocortical stress response. Compr. Physiol. 6, 603–621. doi: 10.1002/cphy.c150015
Ho, L. K. H., Tong, V. J. W., Syn, N., Nagarajan, N., Tham, E. H., Tay, S. K., et al. (2020). Gut microbiota changes in children with autism spectrum disorder: A systematic review. Gut Pathog. 12:6. doi: 10.1186/s13099-020-0346-1
Hua, H., Huang, C., Liu, H., Xu, X., Xu, X., Wu, Z., et al. (2022). Depression and antidepressant effects of ketamine and its metabolites: The pivotal role of gut microbiota. Neuropharmacology 220:109272. doi: 10.1016/j.neuropharm.2022.109272
Huang, F., and Wu, X. (2021). Brain neurotransmitter modulation by gut microbiota in anxiety and depression. Front. Cell Dev. Biol. 9:649103. doi: 10.3389/fcell.2021.649103
Huang, N., Hua, D., Zhan, G., Li, S., Zhu, B., Jiang, R., et al. (2019). Role of Actinobacteria and Coriobacteriia in the antidepressant effects of ketamine in an inflammation model of depression. Pharmacol. Biochem. Behav. 176, 93–100. doi: 10.1016/j.pbb.2018.12.001
Jaglin, M., Rhimi, M., Philippe, C., Pons, N., Bruneau, A., Goustard, B., et al. (2018). Indole, a signaling molecule produced by the gut microbiota, negatively impacts emotional behaviors in rats. Front. Neurosci. 12:216. doi: 10.3389/fnins.2018.00216
Jesulola, E., Micalos, P., and Baguley, I. J. (2018). Understanding the pathophysiology of depression: From monoamines to the neurogenesis hypothesis model - are we there yet? Behav. Brain Res. 341, 79–90. doi: 10.1016/j.bbr.2017.12.025
Ji, S., Han, S., Yu, L., Du, L., You, Y., Chen, J., et al. (2022). Jia Wei Xiao Yao San ameliorates chronic stress-induced depression-like behaviors in mice by regulating the gut microbiome and brain metabolome in relation to purine metabolism. Phytomedicine Int. J. Phytother.Phytopharmacol. 98:153940. doi: 10.1016/j.phymed.2022.153940
Jia, X., Gao, Z., and Hu, H. (2021). Microglia in depression: Current perspectives. Sci. China. Life Sci. 64, 911–925. doi: 10.1007/s11427-020-1815-6
Jiang, X., Yi, S., Liu, Q., Su, D., Li, L., Xiao, C., et al. (2022). Asperosaponin VI ameliorates the CMS-induced depressive-like behaviors by inducing a neuroprotective microglial phenotype in hippocampus via PPAR-γ pathway. J. Neuroinflam. 19:115. doi: 10.1186/s12974-022-02478-y
Jiang, Y., Zou, D., Li, Y., Gu, S., Dong, J., Ma, X., et al. (2022). Monoamine neurotransmitters control basic emotions and affect major depressive disorders. Pharmaceuticals 15:1203. doi: 10.3390/ph15101203
Karaca, Z., Grossman, A., and Kelestimur, F. (2021). Investigation of the Hypothalamo-pituitary-adrenal (HPA) axis: A contemporary synthesis. Rev. Endocr. Metab. Disord. 22, 179–204. doi: 10.1007/s11154-020-09611-3
Karbownik, M. S., Mokros, Ł, Dobielska, M., Kowalczyk, M., and Kowalczyk, E. (2022). Association between consumption of fermented food and food-derived prebiotics with cognitive performance, depressive, and anxiety symptoms in psychiatrically healthy medical students under psychological stress: A prospective cohort study. Front. Nutr. 9:850249. doi: 10.3389/fnut.2022.850249
Karen, C., Shyu, D. J. H., and Rajan, K. E. (2021). Supplementation prevents early life stress-induced anxiety and depressive-like behavior in maternal separation model-possible involvement of microbiota-gut-brain axis in differential regulation of microRNA124a/132 and glutamate receptors. Front. Neurosci. 15:719933. doi: 10.3389/fnins.2021.719933
Karnib, N., El-Ghandour, R., El Hayek, L., Nasrallah, P., Khalifeh, M., Barmo, N., et al. (2019). Lactate is an antidepressant that mediates resilience to stress by modulating the hippocampal levels and activity of histone deacetylases. Neuropsychopharmacology 44, 1152–1162. doi: 10.1038/s41386-019-0313-z
Kendler, K. S. (2017). The genealogy of major depression: Symptoms and signs of melancholia from 1880 to 1900. Mol. Psychiatry 22, 1539–1553. doi: 10.1038/mp.2017.148
Kim, C.-S., Cha, L., Sim, M., Jung, S., Chun, W. Y., Baik, H. W., et al. (2021). Probiotic supplementation improves cognitive function and mood with changes in gut microbiota in community-dwelling older adults: A randomized, double-blind, placebo-controlled, multicenter trial. J. Gerontol. Ser. A Biol. Sci. Med. Sci. 76, 32–40. doi: 10.1093/gerona/glaa090
Kim, I. B., and Park, S.-C. (2021). The entorhinal cortex and adult neurogenesis in major depression. Int. J. Mol. Sci. 22:11725. doi: 10.3390/ijms222111725
Kosuge, A., Kunisawa, K., Arai, S., Sugawara, Y., Shinohara, K., Iida, T., et al. (2021). Heat-sterilized Bifidobacterium breve prevents depression-like behavior and interleukin-1beta expression in mice exposed to chronic social defeat stress. Brain Behav. Immun. 96, 200–211. doi: 10.1016/j.bbi.2021.05.028
Kuti, D., Winkler, Z., Horváth, K., Juhász, B., Paholcsek, M., Stágel, A., et al. (2020). Gastrointestinal (non-systemic) antibiotic rifaximin differentially affects chronic stress-induced changes in colon microbiome and gut permeability without effect on behavior. Brain Behav. Immun. 84, 218–228. doi: 10.1016/j.bbi.2019.12.004
Lai, W.-T., Deng, W.-F., Xu, S.-X., Zhao, J., Xu, D., Liu, Y.-H., et al. (2021). Shotgun metagenomics reveals both taxonomic and tryptophan pathway differences of gut microbiota in major depressive disorder patients. Psychol. Med. 51, 90–101. doi: 10.1017/S0033291719003027
Li, H., Wang, P., Huang, L., Li, P., and Zhang, D. (2019). Effects of regulating gut microbiota on the serotonin metabolism in the chronic unpredictable mild stress rat model. Neurogastroenterol. Motil. 31:e13677. doi: 10.1111/nmo.13677
Li, H., Xiang, Y., Zhu, Z., Wang, W., Jiang, Z., Zhao, M., et al. (2021). Rifaximin-mediated gut microbiota regulation modulates the function of microglia and protects against CUMS-induced depression-like behaviors in adolescent rat. J. Neuroinflam. 18:254. doi: 10.1186/s12974-021-02303-y
Li, J., Hou, L., Wang, C., Jia, X., Qin, X., and Wu, C. (2018). Short term intrarectal administration of sodium propionate induces antidepressant-like effects in rats exposed to chronic unpredictable mild stress. Front. Psychiatry 9:454. doi: 10.3389/fpsyt.2018.00454
Li, J., Li, Y., Duan, W., Zhao, Z., Yang, L., Wei, W., et al. (2022). Shugan granule contributes to the improvement of depression-like behaviors in chronic restraint stress-stimulated rats by altering gut microbiota. CNS Neurosci. Ther. 28, 1409–1424. doi: 10.1111/cns.13881
Li, J.-H., Duan, R., Li, L., Wood, J. D., Wang, X.-Y., Shu, Y., et al. (2020). [Unique characteristics of “the second brain” - The enteric nervous system]. Sheng Li Xue Bao Acta Physiol. Sin. 72, 382–390.
Li, N., Wang, Q., Wang, Y., Sun, A., Lin, Y., Jin, Y., et al. (2019). Fecal microbiota transplantation from chronic unpredictable mild stress mice donors affects anxiety-like and depression-like behavior in recipient mice via the gut microbiota-inflammation-brain axis. Stress 22, 592–602. doi: 10.1080/10253890.2019.1617267
Liang, S., Wu, X., Hu, X., Wang, T., and Jin, F. (2018). Recognizing depression from the microbiota(-)Gut(-)Brain Axis. Int. J. Mol. Sci. 19:1592. doi: 10.3390/ijms19061592
Liang, X.-Q., Mai, P.-Y., Qin, H., Li, S., Ou, W.-J., Liang, J., et al. (2022). Integrated 16S rRNA sequencing and metabolomics analysis to investigate the antidepressant role of Yang-Xin-Jie-Yu decoction on microbe-gut-metabolite in chronic unpredictable mild stress-induced depression rat model. Front. Pharmacol. 13:972351. doi: 10.3389/fphar.2022.972351
Lin, S., Li, Q., Jiang, S., Xu, Z., Jiang, Y., Liu, L., et al. (2021). Crocetin ameliorates chronic restraint stress-induced depression-like behaviors in mice by regulating MEK/ERK pathways and gut microbiota. J. Ethnopharmacol. 268:113608. doi: 10.1016/j.jep.2020.113608
Liu, L., Wang, H., Chen, X., Zhang, Y., Li, W., Rao, X., et al. (2021a). Integrative analysis of long non-coding RNAs, messenger RNAs, and MicroRNAs indicates the neurodevelopmental dysfunction in the hippocampus of gut microbiota-dysbiosis mice. Front. Mol. Neurosci. 14:745437. doi: 10.3389/fnmol.2021.745437
Liu, L., Wang, H., Rao, X., Yu, Y., Li, W., Zheng, P., et al. (2021b). Comprehensive analysis of the lysine acetylome and succinylome in the hippocampus of gut microbiota-dysbiosis mice. J. Adv. Res. 30, 27–38. doi: 10.1016/j.jare.2020.12.00
Liu, R. T., Rowan-Nash, A. D., Sheehan, A. E., Walsh, R. F. L., Sanzari, C. M., Korry, B. J., et al. (2020). Reductions in anti-inflammatory gut bacteria are associated with depression in a sample of young adults. Brain Behav. Immun. 88, 308–324. doi: 10.1016/j.bbi.2020.03.026
Liu, S., da Cunha, A. P., Rezende, R. M., Cialic, R., Wei, Z., Bry, L., et al. (2016). The host shapes the gut microbiota via fecal MicroRNA. Cell Host Microb. 19, 32–43. doi: 10.1016/j.chom.2015.12.005
Liu, W.-H., Chuang, H.-L., Huang, Y.-T., Wu, C.-C., Chou, G.-T., Wang, S., et al. (2016). Alteration of behavior and monoamine levels attributable to Lactobacillus plantarum PS128 in germ-free mice. Behav. Brain Res. 298(Pt B), 202–209. doi: 10.1016/j.bbr.2015.10.046
Liu, X., Wei, F., Liu, H., Zhao, S., Du, G., and Qin, X. (2021c). Integrating hippocampal metabolomics and network pharmacology deciphers the antidepressant mechanisms of Xiaoyaosan. J. Ethnopharmacol. 268:113549. doi: 10.1016/j.jep.2020.113549
Louis, P., and Flint, H. J. (2017). Formation of propionate and butyrate by the human colonic microbiota. Environ. Microbiol. 19, 29–41. doi: 10.1111/1462-2920.13589
Lowes, D. C., Chamberlin, L. A., Kretsge, L. N., Holt, E. S., Abbas, A. I., Park, A. J., et al. (2021). Ventral tegmental area GABA neurons mediate stress-induced blunted reward-seeking in mice. Nat. Commun. 12:3539. doi: 10.1038/s41467-021-23906-2
Lu, X., Yang, R.-R., Zhang, J.-L., Wang, P., Gong, Y., Hu, W.-F., et al. (2018). Tauroursodeoxycholic acid produces antidepressant-like effects in a chronic unpredictable stress model of depression via attenuation of neuroinflammation, oxido-nitrosative stress, and endoplasmic reticulum stress. Fundament. Clin. Pharmacol. 32, 363–377. doi: 10.1111/fcp.12367
Luca, M., Di Mauro, M., Di Mauro, M., and Luca, A. (2019). Gut microbiota in Alzheimer’s disease, depression, and Type 2 diabetes mellitus: The role of oxidative stress. Oxidat. Med. Cell. Long. 2019:4730539. doi: 10.1155/2019/4730539
Lukiæ, I., Getselter, D., Ziv, O., Oron, O., Reuveni, E., Koren, O., et al. (2019). Antidepressants affect gut microbiota and Ruminococcus flavefaciens is able to abolish their effects on depressive-like behavior. Transl. Psychiatry 9:133. doi: 10.1038/s41398-019-0466-x
Lv, M., Wang, Y., Qu, P., Li, S., Yu, Z., Qin, X., et al. (2021). A combination of cecum microbiome and metabolome in CUMS depressed rats reveals the antidepressant mechanism of traditional Chinese medicines: A case study of Xiaoyaosan. J. Ethnopharmacol. 276:114167. doi: 10.1016/j.jep.2021.114167
Lyu, C., Zhao, W., Peng, C., Hu, S., Fang, H., Hua, Y., et al. (2018). Exploring the contributions of two glutamate decarboxylase isozymes in Lactobacillus brevis to acid resistance and γ-aminobutyric acid production. Microb. Cell Fact. 17:180. doi: 10.1186/s12934-018-1029-1
Ma, H., Marti-Gutierrez, N., Park, S.-W., Wu, J., Hayama, T., Darby, H., et al. (2018). Ma et al. reply. Nature 560, E10–E23. doi: 10.1038/s41586-018-0381-y
Ma, S. R., Yu, J. B., Fu, J., Pan, L. B., Yu, H., Han, P., et al. (2021). Determination and application of nineteen monoamines in the gut microbiota targeting phenylalanine, tryptophan, and glutamic acid metabolic pathways. Molecules 26:1377. doi: 10.3390/molecules26051377
Maes, M., Simeonova, D., Stoyanov, D., and Leunis, J.-C. (2019). Upregulation of the nitrosylome in bipolar disorder type 1 (BP1) and major depression, but not BP2: Increased IgM antibodies to nitrosylated conjugates are associated with indicants of leaky gut. Nitr. Oxide Biol. Chem. 91, 67–76. doi: 10.1016/j.niox.2019.07.003
Makris, A. P., Karianaki, M., Tsamis, K. I., and Paschou, S. A. (2021). The role of the gut-brain axis in depression: Endocrine, neural, and immune pathways. Hormones (Athens) 20, 1–12. doi: 10.1007/s42000-020-00236-4
Marin, I. A., Goertz, J. E., Ren, T., Rich, S. S., Onengut-Gumuscu, S., Farber, E., et al. (2017). Microbiota alteration is associated with the development of stress-induced despair behavior. Sci. Rep. 7:43859. doi: 10.1038/srep43859
Markowiak, P., and Śliżewska, K. (2017). Effects of Probiotics, prebiotics, and synbiotics on human health. Nutrients 9:1021. doi: 10.3390/nu9091021
Marx, W., Lane, M., Hockey, M., Aslam, H., Berk, M., Walder, K., et al. (2021). Diet and depression: Exploring the biological mechanisms of action. Mol. Psychiatry 26, 134–150. doi: 10.1038/s41380-020-00925-x
Matsuno, H., Tsuchimine, S., O’Hashi, K., Sakai, K., Hattori, K., Hidese, S., et al. (2022). Association between vascular endothelial growth factor-mediated blood-brain barrier dysfunction and stress-induced depression. Mol. Psychiatry 27, 3822–3832. doi: 10.1038/s41380-022-01618-3
McCoy, C. R., Rana, S., Stringfellow, S. A., Day, J. J., Wyss, J. M., Clinton, S. M., et al. (2016). Neonatal maternal separation stress elicits lasting DNA methylation changes in the hippocampus of stress-reactive Wistar Kyoto rats. Eur. J. Neurosci. 44, 2829–2845. doi: 10.1111/ejn.13404
McGuinness, A. J., Davis, J. A., Dawson, S. L., Loughman, A., Collier, F., O’Hely, M., et al. (2022). A systematic review of gut microbiota composition in observational studies of major depressive disorder, bipolar disorder and schizophrenia. Mol. Psychiatry 27, 1920–1935. doi: 10.1038/s41380-022-01456-3
McVey Neufeld, K.-A., O’Mahony, S. M., Hoban, A. E., Waworuntu, R. V., Berg, B. M., Dinan, T. G., et al. (2019). Neurobehavioural effects of Lactobacillus rhamnosus GG alone and in combination with prebiotics polydextrose and galactooligosaccharide in male rats exposed to early-life stress. Nutr. Neurosci. 22, 425–434. doi: 10.1080/1028415X.2017.1397875
Medina-Rodriguez, E. M., Madorma, D., O’Connor, G., Mason, B. L., Han, D., Deo, S. K., et al. (2020). Identification of a signaling mechanism by which the microbiome regulates Th17 cell-mediated depressive-like behaviors in mice. Am. J. Psychiatry 177, 974–990. doi: 10.1176/appi.ajp.2020.19090960
Meng, C., Feng, S., Hao, Z., Dong, C., and Liu, H. (2022). Antibiotics exposure attenuates chronic unpredictable mild stress-induced anxiety-like and depression-like behavior. Psychoneuroendocrinology 136:105620. doi: 10.1016/j.psyneuen.2021.105620
Merchak, A., and Gaultier, A. (2020). Microbial metabolites and immune regulation: New targets for major depressive disorder. Brain Behav. Immun. Health 9:100169. doi: 10.1016/j.bbih.2020.100169
Mikulska, J., Juszczyk, G., Gawronska-Grzywacz, M., and Herbet, M. (2021). HPA axis in the pathomechanism of depression and schizophrenia: New therapeutic strategies based on its participation. Brain Sci. 11:1298. doi: 10.3390/brainsci11101298
Misiak, B., Łoniewski, I., Marlicz, W., Frydecka, D., Szulc, A., Rudzki, L., et al. (2020). The HPA axis dysregulation in severe mental illness: Can we shift the blame to gut microbiota? Prog. Neuropsychopharmacol. Biol. Psychiatry 102:109951. doi: 10.1016/j.pnpbp.2020.109951
Mitchell, B. L., Campos, A. I., Whiteman, D. C., Olsen, C. M., Gordon, S. D., Walker, A. J., et al. (2022). The australian genetics of depression study: New risk loci and dissecting heterogeneity between subtypes. Biol. Psychiatry 92, 227–235. doi: 10.1016/j.biopsych.2021.10.021
Moludi, J., Khedmatgozar, H., Nachvak, S. M., Abdollahzad, H., Moradinazar, M., and Sadeghpour Tabaei, A. (2022). The effects of co-administration of probiotics and prebiotics on chronic inflammation, and depression symptoms in patients with coronary artery diseases: A randomized clinical trial. Nutr. Neurosci. 25, 1659–1668. doi: 10.1080/1028415X.2021.1889451
Monroe, S. M., and Harkness, K. L. (2022). Major depression and its recurrences: Life course matters. Annu. Rev. Clin. Psychol. 18, 329–357. doi: 10.1146/annurev-clinpsy-072220-021440
Moya-Perez, A., Perez-Villalba, A., Benitez-Paez, A., Campillo, I., and Sanz, Y. (2017). Bifidobacterium CECT 7765 modulates early stress-induced immune, neuroendocrine and behavioral alterations in mice. Brain Behav. Immun. 65, 43–56. doi: 10.1016/j.bbi.2017.05.011
Moya-Pérez, A., Perez-Villalba, A., Benítez-Páez, A., Campillo, I., and Sanz, Y. (2017). Bifidobacterium CECT 7765 modulates early stress-induced immune, neuroendocrine and behavioral alterations in mice. Brain Behav. Immun. 65, 43–56.
Nikolova, V. L., Cleare, A. J., Young, A. H., and Stone, J. M. (2021). updated review and meta-analysis of probiotics for the treatment of clinical depression: Adjunctive vs. stand-alone treatment. J. Clin. Med. 10:647. doi: 10.3390/jcm10040647
Nuzum, N. D., Loughman, A., Szymlek-Gay, E. A., Hendy, A., Teo, W.-P., and Macpherson, H. (2020). Gut microbiota differences between healthy older adults and individuals with Parkinson’s disease: A systematic review. Neurosci. Biobehav. Rev. 112, 227–241. doi: 10.1016/j.neubiorev.2020.02.003
Ogłodek, E. (2022). Changes in the serum levels of cytokines: IL-1β, IL-4, IL-8 and IL-10 in depression with and without posttraumatic stress disorder. Brain Sci. 12:387. doi: 10.3390/brainsci12030387
Ohlsson, L., Gustafsson, A., Lavant, E., Suneson, K., Brundin, L., Westrin, Å, et al. (2019). Leaky gut biomarkers in depression and suicidal behavior. Acta Psychiatr. Scandin. 139, 185–193. doi: 10.1111/acps.12978
Ortega, M. A., Alvarez-Mon, M. A., Garcia-Montero, C., Fraile-Martinez, O., Guijarro, L. G., Lahera, G., et al. (2022). Gut microbiota metabolites in major depressive disorder-deep insights into their pathophysiological role and potential translational applications. Metabolites 12:50. doi: 10.3390/metabo12010050
Oungpasuk, K., Li, Y. M., and Stettler, I. (2020). Association between depression and chronic gastrointestinal symptoms. Clin. Gastroenterol. Hepatol. 18:1898. doi: 10.1016/j.cgh.2019.12.042
Öztürk, M., Yalın Sapmaz, Ş., Kandemir, H., Taneli, F., and Aydemir, Ö (2021). The role of the kynurenine pathway and quinolinic acid in adolescent major depressive disorder. Int. J. Clin. Pract. 75:e13739. doi: 10.1111/ijcp.13739
Park, A. J., Collins, J., Blennerhassett, P. A., Ghia, J. E., Verdu, E. F., Bercik, P., et al. (2013). Altered colonic function and microbiota profile in a mouse model of chronic depression. Neurogastroenterol. Motil. 25, 733–e575. doi: 10.1111/nmo.12153
Park, M., Choi, J., and Lee, H.-J. (2020). Flavonoid-rich orange juice intake and altered gut microbiome in young adults with depressive symptom: A randomized controlled study. Nutrients 12:1815. doi: 10.3390/nu12061815
Paternain, L., Martisova, E., Campión, J., Martínez, J. A., Ramírez, M. J., and Milagro, F. I. (2016). Methyl donor supplementation in rats reverses the deleterious effect of maternal separation on depression-like behaviour. Behav. Brain Res. 299, 51–58. doi: 10.1016/j.bbr.2015.11.031
Paton, J. F., Li, Y. W., Deuchars, J., and Kasparov, S. (2000). Properties of solitary tract neurons receiving inputs from the sub-diaphragmatic vagus nerve. Neuroscience 95, 141–153. doi: 10.1016/s0306-4522(99)00416-9
Pellissier, S., and Bonaz, B. (2017). The place of stress and emotions in the irritable bowel syndrome. Vitam. Horm. 103, 327–354. doi: 10.1016/bs.vh.2016.09.005
Peng, X., Luo, Z., He, S., Zhang, L., and Li, Y. (2021). Blood-brain barrier disruption by lipopolysaccharide and sepsis-associated encephalopathy. Front. Cell. Infect. Microbiol. 11:768108. doi: 10.3389/fcimb.2021.768108
Perez-Caballero, L., Torres-Sanchez, S., Romero-López-Alberca, C., González-Saiz, F., Mico, J. A., and Berrocoso, E. (2019). Monoaminergic system and depression. Cell Tissue Res. 377, 107–113. doi: 10.1007/s00441-018-2978-8
Petty, F., Trivedi, M. H., Fulton, M., and Rush, A. J. (1995). Benzodiazepines as antidepressants: Does GABA play a role in depression? Biol. Psychiatry 38, 578–591.
Philippe, C., Szabo de Edelenyi, F., Naudon, L., Druesne-Pecollo, N., Hercberg, S., Kesse-Guyot, E., et al. (2021). Relation between mood and the host-microbiome Co-metabolite 3-indoxylsulfate: Results from the Observational Prospective NutriNet-Santé study. Microorganisms 9:716. doi: 10.3390/microorganisms9040716
Price, R. B., and Duman, R. (2020). Neuroplasticity in cognitive and psychological mechanisms of depression: An integrative model. Mol. Psychiatry 25, 530–543. doi: 10.1038/s41380-019-0615-x
Pu, Y., Tan, Y., Qu, Y., Chang, L., Wang, S., Wei, Y., et al. (2021). A role of the subdiaphragmatic vagus nerve in depression-like phenotypes in mice after fecal microbiota transplantation from Chrna7 knock-out mice with depression-like phenotypes. Brain Behav. Immun. 94, 318–326. doi: 10.1016/j.bbi.2020.12.032
Qiu, J., Liu, R., Ma, Y., Li, Y., Chen, Z., He, H., et al. (2020). Lipopolysaccharide-induced depression-like behaviors is ameliorated by sodium butyrate via inhibiting neuroinflammation and oxido-nitrosative stress. Pharmacology 105, 550–560. doi: 10.1159/000505132
Qu, Y., Dai, C., Zhang, X., and Ma, Q. (2019). [A new interspecies and interkingdom signaling molecule-Indole]. Sheng Wu Gong Cheng Xue Bao = Chin. J. Biotechnol. 35, 2177–2188. doi: 10.13345/j.cjb.190158
Qu, Y., Yang, C., Ren, Q., Ma, M., Dong, C., and Hashimoto, K. (2017). Comparison of (R)-ketamine and lanicemine on depression-like phenotype and abnormal composition of gut microbiota in a social defeat stress model. Sci. Rep. 7:15725. doi: 10.1038/s41598-017-16060-7
Ramaker, M. J., and Dulawa, S. C. (2017). Identifying fast-onset antidepressants using rodent models. Mol. Psychiatry 22, 656–665. doi: 10.1038/mp.2017.36
Rao, M., and Gershon, M. D. (2016). The bowel and beyond: The enteric nervous system in neurological disorders. Nat. Rev. Gastroenterol. Hepatol. 13, 517–528. doi: 10.1038/nrgastro.2016.107
Ren, M., Zhang, H., Qi, J., Hu, A., Jiang, Q., Hou, Y., et al. (2020). An Almond-based low carbohydrate diet improves depression and glycometabolism in patients with type 2 diabetes through modulating gut microbiota and GLP-1: A randomized controlled Trial. Nutrients 12:3036. doi: 10.3390/nu12103036
Rincon-Cortes, M., and Grace, A. A. (2020). Antidepressant effects of ketamine on depression-related phenotypes and dopamine dysfunction in rodent models of stress. Behav. Brain Res. 379:112367. doi: 10.1016/j.bbr.2019.112367
Roager, H. M., and Licht, T. R. (2018). Microbial tryptophan catabolites in health and disease. Nature Commun. 9:3294. doi: 10.1038/s41467-018-05470-4
Rong, H., Xie, X.-H., Zhao, J., Lai, W.-T., Wang, M.-B., Xu, D., et al. (2019). Similarly in depression, nuances of gut microbiota: Evidences from a shotgun metagenomics sequencing study on major depressive disorder versus bipolar disorder with current major depressive episode patients. J. Psychiatr. Res. 113, 90–99. doi: 10.1016/j.jpsychires.2019.03.017
Rosa, J. M., Formolo, D. A., Yu, J., Lee, T. H., and Yau, S.-Y. (2022). The role of MicroRNA and microbiota in depression and anxiety. Front. Behav. Neurosci. 16:828258. doi: 10.3389/fnbeh.2022.828258
Rosenblat, J. D., Carvalho, A. F., Li, M., Lee, Y., Subramanieapillai, M., and McIntyre, R. S. (2019). Oral ketamine for depression: A systematic review. J. Clin. Psychiatry 80:18r12475. doi: 10.4088/JCP.18r12475
Rostila, M. (2020). Invited commentary: Social Cohesion, depression, and the role of welfare states. Am. J. Epidemiol. 189, 354–357. doi: 10.1093/aje/kwz207
Rothhammer, V., Mascanfroni, I. D., Bunse, L., Takenaka, M. C., Kenison, J. E., Mayo, L., et al. (2016). Type I interferons and microbial metabolites of tryptophan modulate astrocyte activity and central nervous system inflammation via the aryl hydrocarbon receptor. Nat. Med. 22, 586–597. doi: 10.1038/nm.4106
Rush, A. J., Sackeim, H. A., Conway, C. R., Bunker, M. T., Hollon, S. D., Demyttenaere, K., et al. (2022). Clinical research challenges posed by difficult-to-treat depression. Psychol. Med. 52, 419–432. doi: 10.1017/S0033291721004943
Ryan, K. M., Allers, K. A., McLoughlin, D. M., and Harkin, A. (2020). Tryptophan metabolite concentrations in depressed patients before and after electroconvulsive therapy. Brain Behav. Immun. 83, 153–162. doi: 10.1016/j.bbi.2019.10.005
Shao, S., Jia, R., Zhao, L., Zhang, Y., Guan, Y., Wen, H., et al. (2021). Xiao-Chai-Hu-Tang ameliorates tumor growth in cancer comorbid depressive symptoms via modulating gut microbiota-mediated TLR4/MyD88/NF-kappaB signaling pathway. Phytomedicine 88:153606. doi: 10.1016/j.phymed.2021.153606
Smith, E. A., and Macfarlane, G. T. (1996). Enumeration of human colonic bacteria producing phenolic and indolic compounds: Effects of pH, carbohydrate availability and retention time on dissimilatory aromatic amino acid metabolism. J. Appl. Bacteriol. 81, 288–302. doi: 10.1111/j.1365-2672.1996.tb04331.x
Smith, K. B., Murray, E., Gregory, J. G., Liang, J., and Ismail, N. (2021). Pubertal probiotics mitigate lipopolysaccharide-induced programming of the hypothalamic-pituitary-adrenal axis in male mice only. Brain Res. Bull. 177, 111–118. doi: 10.1016/j.brainresbull.2021.09.017
Sorbara, M. T., and Pamer, E. G. (2022). Microbiome-based therapeutics. Nat. Rev. Microbiol. 20, 365–380. doi: 10.1038/s41579-021-00667-9
Struijs, S. Y., de Jong, P. J., Jeronimus, B. F., van der Does, W., Riese, H., and Spinhoven, P. (2021). Psychological risk factors and the course of depression and anxiety disorders: A review of 15 years NESDA research. J. Affect. Disord. 295, 1347–1359. doi: 10.1016/j.jad.2021.08.086
Suda, K., and Matsuda, K. (2022). How microbes affect depression: Underlying mechanisms via the gut-brain axis and the modulating role of probiotics. Int. J. Mol. Sci. 23:1172. doi: 10.3390/ijms23031172
Sun, J., Wang, F., Hong, G., Pang, M., Xu, H., Li, H., et al. (2016). Antidepressant-like effects of sodium butyrate and its possible mechanisms of action in mice exposed to chronic unpredictable mild stress. Neurosci. Lett. 618, 159–166. doi: 10.1016/j.neulet.2016.03.003
Sun, J., Wang, F., Hu, X., Yang, C., Xu, H., Yao, Y., et al. (2018). Clostridium butyricum attenuates chronic unpredictable mild stress-induced depressive-like behavior in mice via the gut-brain axis. J. Agric. Food Chem. 66, 8415–8421. doi: 10.1021/acs.jafc.8b02462
Sun, L., Zhang, H., Cao, Y., Wang, C., Zhao, C., Wang, H., et al. (2019). Fluoxetine ameliorates dysbiosis in a depression model induced by chronic unpredicted mild stress in mice. Int. J. Med. Sci. 16, 1260–1270. doi: 10.7150/ijms.37322
Sun, Y., Yan, T., Gong, G., Li, Y., Zhang, J., Wu, B., et al. (2020). Antidepressant-like effects of Schisandrin on lipopolysaccharide-induced mice : Gut microbiota, short chain fatty acid and TLR4/NF-κB signaling pathway. Int. Immunopharmacol. 89(Pt A):107029. doi: 10.1016/j.intimp.2020.107029
Tartt, A. N., Mariani, M. B., Hen, R., Mann, J. J., and Boldrini, M. (2022). Dysregulation of adult hippocampal neuroplasticity in major depression: Pathogenesis and therapeutic implications. Mol. Psychiatry 27, 2689–2699. doi: 10.1038/s41380-022-01520-y
Tian, P., Chen, Y., Zhu, H., Wang, L., Qian, X., Zou, R., et al. (2022). Bifidobacterium breve CCFM1025 attenuates major depression disorder via regulating gut microbiome and tryptophan metabolism: A randomized clinical trial. Brain Behav. Immun. 100, 233–241. doi: 10.1016/j.bbi.2021.11.023
Tian, P., Wang, G., Zhao, J., Zhang, H., and Chen, W. (2019). Bifidobacterium with the role of 5-hydroxytryptophan synthesis regulation alleviates the symptom of depression and related microbiota dysbiosis. J. Nutr. Biochem. 66, 43–51. doi: 10.1016/j.jnutbio.2019.01.007
Tremblay, A., Lingrand, L., Maillard, M., Feuz, B., and Tompkins, T. A. (2021). The effects of psychobiotics on the microbiota-gut-brain axis in early-life stress and neuropsychiatric disorders. Prog. Neuropsychopharmacol. Biol. Psychiatry 105:110142. doi: 10.1016/j.pnpbp.2020.110142
Tsavkelova, E. A., Botvinko, I. V., Kudrin, V. S., and Oleskin, A. V. (2000). Detection of neurotransmitter amines in microorganisms with the use of high-performance liquid chromatography. Doklady Biochem. Proc. Acad. Sci. USSR Biochem. Sec. 372, 115–117.
Vagnerová, K., Vodièka, M., Hermanová, P., Ergang, P., Šrùtková, D., Klusoòová, P., et al. (2019). Interactions between gut microbiota and acute restraint stress in peripheral structures of the hypothalamic-pituitary-adrenal axis and the intestine of male mice. Front. Immunol. 10:2655. doi: 10.3389/fimmu.2019.02655
Valles-Colomer, M., Falony, G., Darzi, Y., Tigchelaar, E. F., Wang, J., Tito, R. Y., et al. (2019). The neuroactive potential of the human gut microbiota in quality of life and depression. Nat. Microbiol. 4, 623–632. doi: 10.1038/s41564-018-0337-x
van de Wouw, M., Boehme, M., Lyte, J. M., Wiley, N., Strain, C., O’Sullivan, O., et al. (2018). Short-chain fatty acids: Microbial metabolites that alleviate stress-induced brain-gut axis alterations. J. Physiol. 596, 4923–4944. doi: 10.1113/JP276431
Wahlström, A., Sayin, S. I., Marschall, H.-U., and Bäckhed, F. (2016). Intestinal crosstalk between bile acids and microbiota and its impact on host metabolism. Cell Metabol. 24, 41–50. doi: 10.1016/j.cmet.2016.05.005
Wan, X., Eguchi, A., Fujita, Y., Ma, L., Wang, X., Yang, Y., et al. (2022). Effects of (R)-ketamine on reduced bone mineral density in ovariectomized mice: A role of gut microbiota. Neuropharmacology 213:109139. doi: 10.1016/j.neuropharm.2022.109139
Wang, H.-Q., Wang, Z.-Z., and Chen, N.-H. (2021). The receptor hypothesis and the pathogenesis of depression: Genetic bases and biological correlates. Pharmacol. Res. 167:105542. doi: 10.1016/j.phrs.2021.105542
Wang, S., Ishima, T., Qu, Y., Shan, J., Chang, L., Wei, Y., et al. (2021). Ingestion of Faecalibaculum rodentium causes depression-like phenotypes in resilient Ephx2 knock-out mice: A role of brain-gut-microbiota axis via the subdiaphragmatic vagus nerve. J. Affect. Disord. 292, 565–573. doi: 10.1016/j.jad.2021.06.006
Wang, S., Ishima, T., Zhang, J., Qu, Y., Chang, L., Pu, Y., et al. (2020). Ingestion of Lactobacillus intestinalis and Lactobacillus reuteri causes depression- and anhedonia-like phenotypes in antibiotic-treated mice via the vagus nerve. J. Neuroinflam. 17:241. doi: 10.1186/s12974-020-01916-z
Wang, X., Chang, L., Wan, X., Tan, Y., Qu, Y., Shan, J., et al. (2022). (R)-ketamine ameliorates demyelination and facilitates remyelination in cuprizone-treated mice: A role of gut-microbiota-brain axis. Neurobiol. Dis. 165:105635. doi: 10.1016/j.nbd.2022.105635
Wang, Y., Shi, Y.-H., Xu, Z., Fu, H., Zeng, H., and Zheng, G.-Q. (2019). Efficacy and safety of Chinese herbal medicine for depression: A systematic review and meta-analysis of randomized controlled trials. J. Psychiatr. Res. 117, 74–91. doi: 10.1016/j.jpsychires.2019.07.003
Wang, Z., and Zhao, Y. (2018). Gut microbiota derived metabolites in cardiovascular health and disease. Prot. Cell 9, 416–431. doi: 10.1007/s13238-018-0549-0
Wang, Z., Roberts, A. B., Buffa, J. A., Levison, B. S., Zhu, W., Org, E., et al. (2015). Non-lethal inhibition of gut microbial trimethylamine production for the treatment of atherosclerosis. Cell 163, 1585–1595. doi: 10.1016/j.cell.2015.11.055
Wei, C.-L., Wang, S., Yen, J.-T., Cheng, Y.-F., Liao, C.-L., Hsu, C.-C., et al. (2019). Antidepressant-like activities of live and heat-killed Lactobacillus paracasei PS23 in chronic corticosterone-treated mice and possible mechanisms. Brain Res. 1711, 202–213. doi: 10.1016/j.brainres.2019.01.025
Wei, Y., Chang, L., Liu, G., Wang, X., Yang, Y., and Hashimoto, K. (2022). Long-lasting beneficial effects of maternal intake of sulforaphane glucosinolate on gut microbiota in adult offspring. J. Nutr. Biochem. 109:109098. doi: 10.1016/j.jnutbio.2022.109098
Wei, Y., Melas, P. A., Wegener, G., Mathe, A. A., and Lavebratt, C. (2014). Antidepressant-like effect of sodium butyrate is associated with an increase in TET1 and in 5-hydroxymethylation levels in the Bdnf gene. Int. J. Neuropsychopharmacol. 18:yu032. doi: 10.1093/ijnp/pyu032
Weltens, N., Iven, J., Van Oudenhove, L., and Kano, M. (2018). The gut-brain axis in health neuroscience: Implications for functional gastrointestinal disorders and appetite regulation. Ann. N Y Acad. Sci. 1428, 129–150. doi: 10.1111/nyas.13969
Włodarczyk, A., Cubała, W. J., and Stawicki, M. (2021). Ketogenic diet for depression: A potential dietary regimen to maintain euthymia? Prog. Neuropsychopharmacol. Biol. Psychiatry 109:110257. doi: 10.1016/j.pnpbp.2021.110257
Wu, J., Wang, K., Wang, X., Pang, Y., and Jiang, C. (2021). The role of the gut microbiome and its metabolites in metabolic diseases. Prot. Cell 12, 360–373. doi: 10.1007/s13238-020-00814-7
Wu, Y., Wang, L., Zhang, C.-Y., Li, M., and Li, Y. (2022). Genetic similarities and differences among distinct definitions of depression. Psychiatry Res. 317:114843. doi: 10.1016/j.psychres.2022.114843
Yamawaki, Y., Yoshioka, N., Nozaki, K., Ito, H., Oda, K., Harada, K., et al. (2018). Sodium butyrate abolishes lipopolysaccharide-induced depression-like behaviors and hippocampal microglial activation in mice. Brain Res. 1680, 13–38. doi: 10.1016/j.brainres.2017.12.004
Yan, T., Nian, T., Liao, Z., Xiao, F., Wu, B., Bi, K., et al. (2020). Antidepressant effects of a polysaccharide from okra (Abelmoschus esculentus (L) Moench) by anti-inflammation and rebalancing the gut microbiota. Int. J. Biol. Macromol. 144, 427–440. doi: 10.1016/j.ijbiomac.2019.12.138
Yan, T., Wang, N., Liu, B., Wu, B., Xiao, F., He, B., et al. (2021). Schisandra chinensis ameliorates depressive-like behaviors by regulating microbiota-gut-brain axis via its anti-inflammation activity. Phytother. Res. PTR 35, 289–296. doi: 10.1002/ptr.6799
Yang, C., Qu, Y., Fujita, Y., Ren, Q., Ma, M., Dong, C., et al. (2017). Possible role of the gut microbiota-brain axis in the antidepressant effects of (R)-ketamine in a social defeat stress model. Transl. Psychiatry 7:1294. doi: 10.1038/s41398-017-0031-4
Yang, W., Yu, T., Huang, X., Bilotta, A. J., Xu, L., Lu, Y., et al. (2020). Intestinal microbiota-derived short-chain fatty acids regulation of immune cell IL-22 production and gut immunity. Nat. Commun. 11:4457. doi: 10.1038/s41467-020-18262-6
Yang, Y., Eguchi, A., Wan, X., Chang, L., Wang, X., Qu, Y., et al. (2022). A role of gut-microbiota-brain axis via subdiaphragmatic vagus nerve in depression-like phenotypes in Chrna7 knock-out mice. Prog. Neuropsychopharmacol. Biol. Psychiatry 120:110652. doi: 10.1016/j.pnpbp.2022.110652
Yogeswara, I. B. A., Maneerat, S., and Haltrich, D. (2020). Glutamate decarboxylase from lactic acid bacteria-a key enzyme in GABA synthesis. Microorganisms 8:1923. doi: 10.3390/microorganisms8121923
Yu, Y., Wang, H., Rao, X., Liu, L., Zheng, P., Li, W., et al. (2021). Proteomic profiling of lysine acetylation indicates mitochondrial dysfunction in the hippocampus of gut microbiota-absent mice. Front. Mol. Neurosci. 14:594332. doi: 10.3389/fnmol.2021.594332
Yunes, R. A., Poluektova, E. U., Vasileva, E. V., Odorskaya, M. V., Marsova, M. V., Kovalev, G. I., et al. (2020). A multi-strain potential probiotic formulation of GABA-Producing Lactobacillus plantarum 90sk and Bifidobacterium adolescentis 150 with antidepressant effects. Probiot. Antimicrob. Prot. 12, 973–979. doi: 10.1007/s12602-019-09601-1
Zhang, J., Ma, L., Chang, L., Pu, Y., Qu, Y., and Hashimoto, K. (2020). A key role of the subdiaphragmatic vagus nerve in the depression-like phenotype and abnormal composition of gut microbiota in mice after lipopolysaccharide administration. Transl. Psychiatry 10:186. doi: 10.1038/s41398-020-00878-3
Zhang, X., Liu, Y., Hong, X., Li, X., Meshul, C. K., Moore, C., et al. (2021). NG2 glia-derived GABA release tunes inhibitory synapses and contributes to stress-induced anxiety. Nat. Commun. 12:5740. doi: 10.1038/s41467-021-25956-y
Zhang, Y., Fan, Q., Hou, Y., Zhang, X., Yin, Z., Cai, X., et al. (2022). Bacteroides species differentially modulate depression-like behavior via gut-brain metabolic signaling. Brain Behavi. Immun. 102, 11–22. doi: 10.1016/j.bbi.2022.02.007
Zhang, Y., Huang, R., Cheng, M., Wang, L., Chao, J., Li, J., et al. (2019). Gut microbiota from NLRP3-deficient mice ameliorates depressive-like behaviors by regulating astrocyte dysfunction via circHIPK2. Microbiome 7:116. doi: 10.1186/s40168-019-0733-3
Zheng, Z.-H., Tu, J.-L., Li, X.-H., Hua, Q., Liu, W.-Z., Liu, Y., et al. (2021). Neuroinflammation induces anxiety- and depressive-like behavior by modulating neuronal plasticity in the basolateral amygdala. Brain Behav. Immun. 91, 505–518. doi: 10.1016/j.bbi.2020.11.007
Zhong, Q., Chen, J.-J., Wang, Y., Shao, W.-H., Zhou, C.-J., and Xie, P. (2022). Differential Gut microbiota compositions related with the severity of major depressive disorder. Front. Cell. Infect. Microbiol. 12:907239. doi: 10.3389/fcimb.2022.907239
Zhou, B., Zhu, Z., Ransom, B. R., and Tong, X. (2021). Oligodendrocyte lineage cells and depression. Mol. Psychiatry 26, 103–117. doi: 10.1038/s41380-020-00930-0
Zhou, L., Wang, T., Yu, Y., Li, M., Sun, X., Song, W., et al. (2022). The etiology of poststroke-depression: A hypothesis involving HPA axis. Biomed. Pharmacother. Biomed. Pharmacother. 151:113146. doi: 10.1016/j.biopha.2022.113146
Zhou, L., Zhang, M., Wang, Y., Dorfman, R. G., Liu, H., Yu, T., et al. (2018). Faecalibacterium prausnitzii produces butyrate to maintain Th17/treg balance and to ameliorate colorectal colitis by inhibiting histone deacetylase 1. Inflammat. Bowel Dis. 24, 1926–1940. doi: 10.1093/ibd/izy182
Zhou, N., Gu, X., Zhuang, T., Xu, Y., Yang, L., and Zhou, M. (2020). Gut microbiota: A pivotal hub for polyphenols as antidepressants. J. Agric. Food Chem. 68, 6007–6020. doi: 10.1021/acs.jafc.0c01461
Keywords: major depressive disorder (MDD), microbiota-gut-brain axis, metabolite, inflammation, neuroendocrine, gut microbiota
Citation: Han W, Wang N, Han M, Ban M, Sun T and Xu J (2022) Reviewing the role of gut microbiota in the pathogenesis of depression and exploring new therapeutic options. Front. Neurosci. 16:1029495. doi: 10.3389/fnins.2022.1029495
Received: 29 August 2022; Accepted: 24 November 2022;
Published: 08 December 2022.
Edited by:
Chun Yang, Nanjing Medical University, ChinaReviewed by:
Valentina Caputi, University College Cork, IrelandKenji Hashimoto, Chiba University, Japan
Copyright © 2022 Han, Wang, Han, Ban, Sun and Xu. This is an open-access article distributed under the terms of the Creative Commons Attribution License (CC BY). The use, distribution or reproduction in other forums is permitted, provided the original author(s) and the copyright owner(s) are credited and that the original publication in this journal is cited, in accordance with accepted academic practice. No use, distribution or reproduction is permitted which does not comply with these terms.
*Correspondence: Junnan Xu, eGpuMDAyQDEyNi5jb20=