- 1The Key Laboratory of Oral Biomedicine, The Affiliated Stomatological Hospital of Nanchang University, Nanchang, China
- 2Department of Ophthalmology, The First Affiliated Hospital of Nanchang University, Nanchang, China
- 3Department of Radiology, The First Affiliated Hospital of Nanchang University, Nanchang, China
Toothache (TA) is a common and severe pain, but its effects on the brain are somewhat unclear. In this study, functional magnetic resonance imaging (fMRI) was used to compare regional homogeneity (ReHo) between TA patients and a normal control group and to explore the brain activity changes during TA, establishing the theoretical basis for the mechanism of neuropathic pain. In total, 20 TA patients and 20 healthy controls (HCs) were recruited and underwent assessment of pain, and then resting-state fMRI (rs-fMRI). The ReHo method was used to analyze the original whole-brain images. Pearson’s correlation analysis was used to assess the relationship between mean ReHo values in each brain region and clinical symptoms, and the receiver operating characteristic (ROC) curve was used to conduct correlation analysis on the brain regions studied. The ReHo values of the right lingual gyrus (RLG), right superior occipital gyrus (RSOG), left middle occipital gyrus (LMOG) and right postcentral gyrus (RPG) in the TA group were significantly higher than in HCs. The mean ReHo values in the RLG were positively correlated with the anxiety score (AS) (r = 0.723, p < 0.001), depression score (DS) (r = 0.850, p < 0.001) and visual analogue score (VAS) (r = 0.837, p < 0.001). The mean ReHo values of RSOG were also positively correlated with AS (r = 0.687, p = 0.001), DS (r = 0.661, p = 0.002) and VAS (r = 0.712, p < 0.001). The areas under the ROC curve of specific brain area ReHo values were as follows: RLG, 0.975; RSOG, 0.959; LMOG, 0.975; RPG, 1.000. Various degrees of brain activity changes reflected by ReHo values in different areas of the brain indicate the impact of TA on brain function. These findings may reveal related neural mechanisms underlying TA.
Introduction
Toothache (TA) is the most common and severe pain in the field of stomatology. The oral area is distinct from other parts of the body due to its special anatomical characteristics and receptors. Medical images of typical TA show that it is often accompanied by swollen gums and severe alveolar bone resorption. TA is a symptom of potential damage, such as pulp infection. Clinical treatment to alleviate pain mainly targets pathological changes and is based on the assumption: the pain sensation of peripheral innervation can often be accurate positioning. Studies have shown that the treatment of tooth lesions does not achieve the purpose of pain relief. The reason was that the root cause of TA in some TA patients was not the teeth themselves, but the pain in this area caused by trigeminal neuropathy and bone afferent pain (Nixdorf et al., 2012). In addition, the degree of TA and the severity of lesions do not show a linear relationship, and the pain experienced is instead closely related to personal characteristics, psychological factors, and other factors (Wiech et al., 2008). Therefore, it is important to understand the cognitive regulation of the pain system to explore brain function in TA.
Resting-state functional magnetic resonance imaging (rs-fMRI) is a functional brain imaging technique that records signals reflecting metabolic changes related to neural activity in specific brain regions. The consistency of blood oxygen level-dependent (BOLD) signals between adjacent voxels in the brain is measured to evaluate the brain activity of subjects at rest (Zang et al., 2004; Biswal, 2012; Lin et al., 2013a). At each location in the brain, neighboring voxel BOLD signals show similar modulation over time. The ReHo is calculated using the REST toolkit using Kendall’s coefficient of consistency (KCC) (Kippenhan et al., 1992). The ReHo (rs-ReHo) diagram of an individual’s resting state is generated by combining the KCC value of each voxel and its 26 nearest neighboring voxels (Zang et al., 2004). Individual rs-ReHo diagrams are standardized with their own average KCC. Based on this concept, the regional homogeneity (ReHo) method can be used to measure the degree of similarity of different voxel time series in brain regions, to obtain information about brain activity, and determine the spontaneous fMRI signal partial synchronization (Brügger et al., 2011, 2012). As one of the data-driven methods for the study of fMRI, ReHo can collect more regional functional activity information than the model-driven method without any prior assumptions about the hemodynamic model, to further analyze the functional activity changes of temporary specific brain regions performing corresponding tasks (Zang et al., 2004). One of the advantages of ReHo is that it does not require random seed assignment and can be used to detect changes in any region of the cerebral (Tononi et al., 1998). In addition, compared with other traditional ALFF sequences, ReHo sequences can observe synchronous signals of neural functional activity in cerebral regions with similar functional activity changes. Therefore, the sequence can be used to analyze the corresponding cerebral regions as a whole and expand the wholeness of observation (Yue et al., 2020). Up to now, ReHo has also been used by some researchers in the study of pathology in psychiatric, neurological, and ophthalmic diseases such as the pathogenesis of epilepsy (Tang et al., 2014), optic neuritis (Shao et al., 2015), and comitant strabismus (Huang et al., 2016b).
Neuro-electrophysiology and neuro-orbital procedures have shown that structural networks involved in the multidimensional aspects of pain perception mainly included the primary and secondary somatosensory cortex, insula cortex, the anterior cingulate, prefrontal cortex, the thalamus, and cerebellar cortex (Apkarian et al., 2005; Farrell et al., 2005). Studies have found that the influence of TA on brain-associated networks is strikingly similar to that reported for spinal pain (Iannetti and Mouraux, 2010). A previous study reported the changes in brain functional connections in TA and found that the functional activities of the right lingual gyrus, right precentral gyrus, and left middle temporal gyrus were significantly higher than those of healthy subjects (Wu et al., 2020). The study also first confirmed that the degree centrality (DC) value of the right gyrus was positively correlated with the anxiety and depression scores of the subjects, and the DC value of the left middle temporal gyrus was positively correlated with the pain score. Our other previous study using ALFF sequence to study the changes in brain functional activities in TA patients also showed similar results (Yang et al., 2019). Studies on the changes in functional activities in the corresponding brain regions of TA showed that different levels of TA stimulation were closely related to specific subregions in the cerebral cortex pain network, including the bilateral anterior insula and cingulate gyrus (Brügger et al., 2012). However, these pain-related brain changes which had been confirmed maybe not be caused by pain entirely, even if the location of the lesions and the nature of the stimulus are the same. The study of the changes in cerebral functional activity in patients with TA provides conditions for further study of the neuropathological mechanism of TA. To a certain extent, the severity of the disease and the exposure to clinical symptoms can be evaluated by monitoring the changes in cerebral function and activity, to provide personalized treatment for patients with TA. The purpose of this study was to analyze the relationship between changes in ReHo values alternations in specific brain regions and clinical outcomes in TA patients by processing fMRI data using the ReHo method.
Materials and methods
Participants
A total of 20 patients with TA and 20 healthy controls (HCs) were recruited at the first affiliated Hospital of Nanchang University. The inclusion criteria of TA patients were: (1) pulp and/or periodontal pain due to dental or non-dental disease; (2) acute and/or chronic TA (including trigeminal nerve pain); (3) no other comorbid pain diseases; (4) no contraindications to MRI examination; (5) no obvious abnormal signals showed in Routine MRI T1WI and T2WI sequences. Exclusion criteria included: (1) structural brain abnormalities, (2) systemic disease, recent intake of analgesic drugs, anti-inflammatory or narcotic drug allergy history, (3) long-term anxiety, depression, and other mental health abnormalities, (4) heart pacemakers and other metals in the body, contraindicating an MRI scan. A visual analog scale (VAS) was used to evaluate subjects’ pain (Bijur et al., 2001). A score of 0 represented painlessness, and a score of 10 denoted excruciating pain. In addition, all subjects completed the Hospital Anxiety and fepression Scale (HADS) (Bjelland et al., 2002). Subjects were instructed to take no alcohol for 12 h before testing and MRI measurements were performed between 1 PM and 9 PM.
This study met the requirements of the Medical Ethics Committee of the first affiliated Hospital of Nanchang University. The approval number is cdyfy2017021. Subjects participating in this study expressed their willingness to participate and signed a declaration of informed consent after learning about the purpose, methods, and potential risks of the study.
Magnetic resonance imaging parameters
The Trio 3T MR scanner (Siemens Healthcare, Erlangen, Germany) was used for MRI scans Whole-brain T1 images were weighted using prepared magnetic gradient-echo images (MPRAGE). The corresponding metamorphic gradient echo sequence was conducted using the following parameters: repetition time (TR) 1,900 ms, echo time TE 2.26 ms, thickness 1.0 mm, gap 0.5 mm, acquisition matrix 256 × 256, the field of view (FOV) 250 mm × 250 mm and turning angle 9°. Functional images were then obtained using the gradient echo plane image sequence in the static scanning session with parameters TR 2,000 ms, TE 30 ms, thickness 4.0 mm, gap 1.2 mm, acquisition matrix 64 × 64, FOV 220 mm × 220 mm, turning angle 90° and 29 axial slices. In addition, all TA patients did not use other analgesic drugs during the rS-FMRI examination.
Functional magnetic resonance imaging data processing
To analyze the functional images we first used MRIcro software to filter unusable data and then used fMRI data processing assistant and Statistical Parameter Mapping (SPM8) for data processing (Huang et al., 2016a). To ensure that the measurement signals from each individual were balanced, the first ten volumes recorded from each individual were deleted. A series of recent studies have shown that head movement can significantly affect the results of rs-fMRI. In this study, we used the Friston 24-head motion parameter model to reduce the potential impact of head movement on the results (Van Dijk et al., 2012; Zeng et al., 2014). In addition, we used linear regression to eliminate the effects of respiration, local small motion, and low-frequency drift on the variables from variation or movement effects. After the correction of head movement, the functional magnetic resonance image was normalized to Montreal Neurological Institute space (Tate et al., 2014). Then the images were de-trended by bandpass filtering (0.01–0.08 Hz) to reduce the influence of factors such as physiological high-frequency breathing, low-frequency drift, and cardiac noise. Through the above data preprocessing methods, we ensure the image quality included in the experiment. Voxel calculations were then made in 27 individual clusters using the KCC. Based on the ReHo method, KCC was used to calculate the similarity between the time series of a given voxel and its nearest time series at the voxel level, to judge the functional activity changes of the corresponding cerebral regions. The details of the KCC calculation can be found in the references (Zang et al., 2004). The remaining data were smoothed using a full width half maximum Gaussian of 6 mm × 6 mm × 6 mm.
Regional homogeneity statistical analysis
ReHo computations were conducted using REST software. ReHo analysis was calculated for a given time series and its neighboring voxel time series using KCC to assess the consistency and comparability of each voxel, assuming similarity with its neighbors.
Statistical analysis
In this study, SPSS version 16.0 (IBM Corp., USA) was used to analyze clinical variables and demographics. Comparisons were made using an independent samples t-test, with p values less than 0.05 indicating statistical significance. The SPM8 toolkit was then used for general linear model analysis. To compare ReHo between the TA and HC groups, the two-sample t-test was used and Gaussian random field theory was applied to adjust for multiple comparisons (P = 0.01, 40 voxels, AlphaSim correction).
The receiver operating characteristic (ROC) curve was used to analyze the correlation between the mean ReHo values of different brain regions in each group. After obtaining the corresponding results, Pearson’s correlation analysis was used to evaluate relationships between behavioral characteristics and ReHo values in the corresponding brain regions in the TA group, with P < 0.05 indicating a significant difference.
Results
Demographics
No significant difference in age was found between the two groups (P = 0.692). Demographic information and pain assessment of subjects are shown in Table 1. The mean ± standard deviation of TA duration was 0.19 ± 0.07 years.
Regional homogeneity differences
ReHo values were significantly higher in patients with TA than in HCs in the following brain regions: right lingual gyrus (RLG), right postcentral gyrus (RPG), left middle occipital gyrus (LMOG), and right superior occipital gyrus (RSOG) (Figures 1A,B, yellow and Table 2). Figure 1C shows differences in mean ReHo between TA and HC groups.
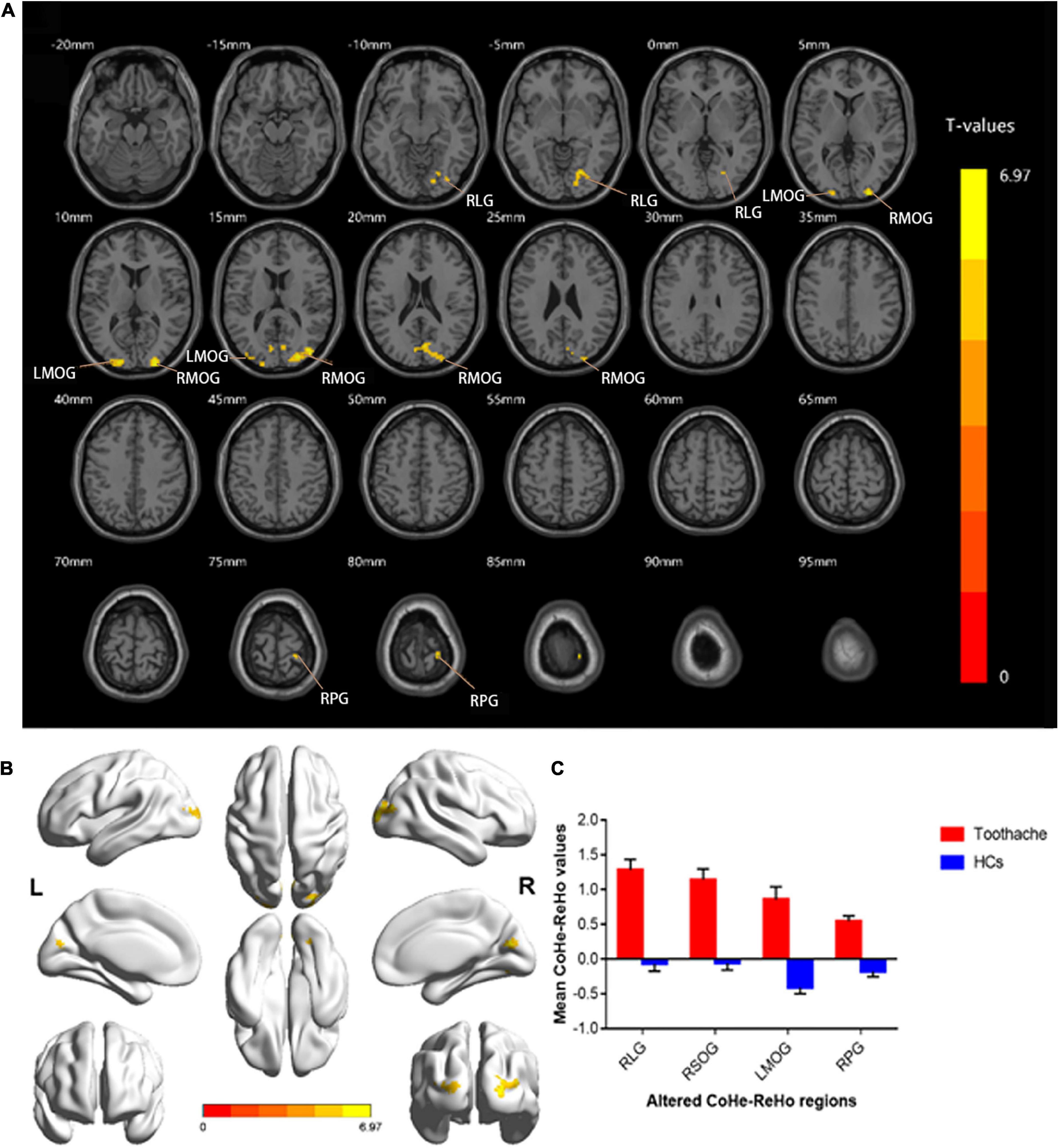
Figure 1. Spontaneous brain activity in the TA and HC groups. RLG, RSOG, LMOG, and RPG showed significant between-group differences in activity. Higher ReHo values are shown in red or yellow; lower values in blue [P < 0.01 for multiple comparisons using Gaussian random field theory (cluster 40 > voxels, AlphaSim corrected; z > 2.3, P < 0.01)] (A,B). The mean differences in ReHo values between the HC and TA groups (C). ReHo, regional homogeneity; RLG, right lingual gyrus; RSOG, right superior occipital gyrus; LMOG, left middle occipital gyrus; RPG, right postcentral gyrus; HCs, healthy controls.
Correlation analysis
In the TA group, the mean ReHo values in the RLG were positively correlated with the anxiety score (AS) (r = 0.723, p < 0.001), depression score (DS) (r = 0.850, p < 0.001) and VAS (r = 0.837, p < 0.001). ReHo values at the RSOG were also positively correlated with the AS (r = 0.687, p = 0.001), DS (r = 0.661, p = 0.002) and VAS (r = 0.712, p < 0.001) (Figure 2).
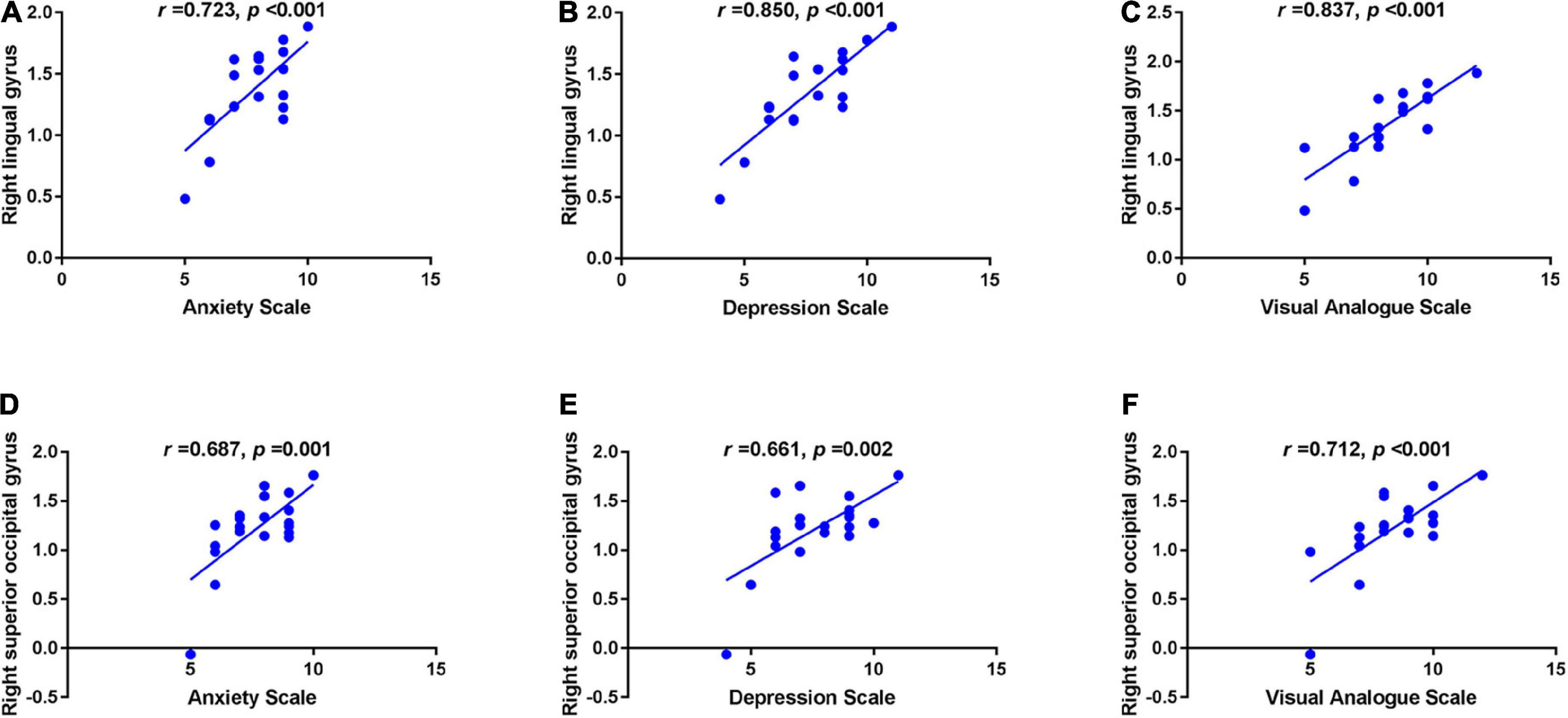
Figure 2. Correlations between the mean ReHo values in different brain regions and the clinical behaviors in the TA group. The mean ReHo value in the right lingual gyrus was positively correlated with the anxiety score (r = 0.723, p < 0.001) (A), depression score (r = 0.850, p < 0.001) (B) and visual analogue score (r = 0.837, p < 0.001) (C); and the right superior occipital gyrus value was positively correlated with the anxiety score (r = 0.687, p = 0.001) (D), depression score (r = 0.661, p = 0.002) (E), and visual analogue score (r = 0.712, p < 0.001) (F). ReHo, regional homogeneity; TA, toothache; RLG, right lingual gyrus; RSOG, right superior occipital gyrus.
Receiver operating characteristic curve
We adopted the ROC curve for differential diagnosis analysis, where the area under the curve (AUC) ranges from 0.5 to 0.7, indicating that the differential diagnosis accuracy of this indicator is low; when the AUC value ranges from 0.7 to 0.9, indicating that this indicator has moderate accuracy; when the AUC value is greater than 0.9, it means excellent precision in differential diagnosis (Chen et al., 2021). Areas under the curve (AUCs) for each region were as follows: RLG, 0.975; RSOG, 0.959 (Figure 3A); LMOG, 0.975; RPG, 1.000 (Figure 3B).
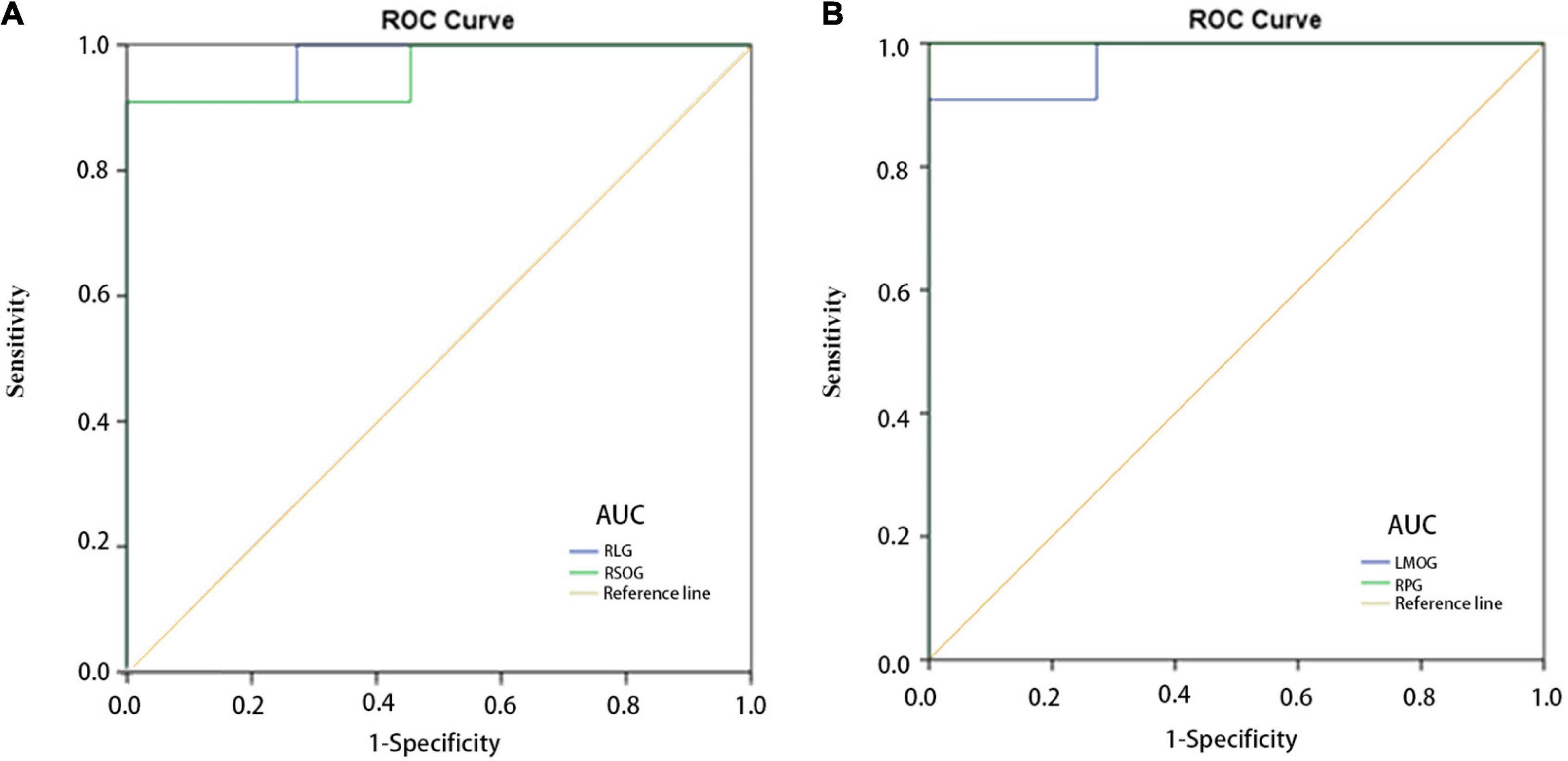
Figure 3. ROC curve analysis of the mean regional homogeneity values for altered brain regions. (A) The AUCs were 0.975 (P < 0.001; 95% CI: 0.918–1.000) for the RLG, and 0.959 (P < 0.001; 95% CI: 0.873–1.000) for the RSOG. (B) The AUCs were 0.975 (P < 0.001; 95% CI: 0.918–1.000) for the LMOG, and 1.000 (P < 0.001; 95% CI: 1.000–1.000) for the RPG. AUC, area under the curve; CI, confidence interval; HC, healthy control; RLG, right lingual gyrus; RSOG, right superior occipital gyrus; LMOG, left middle occipital gyrus; RPG, right postcentral gyrus; ROC, receiver operating characteristic.
Discussion
Analysis of central nervous system activity has been an area of intense interest for many years. Since the invention of the electroencephalogram (EEG), brain function has been explored using a range of tools under various conditions. In recent decades, the characteristics of brain activity in the resting state have become a subject of great interest to various medical departments (Raichle and Snyder, 2007; Buckner et al., 2008), providing a new perspective for related studies on brain functional organization. Resting-state analysis has been used to assess ReHo values alternations relating to, movement, language, vision, processing, and other functions (Moussa et al., 2012; Lee et al., 2014). The stimuli produced by TA generate activity that may be analyzed in the resting state.
Studies have shown the importance of treating and diagnosing TA. To some extent, controlling pain is as important as treating the injury, the latter being challenging due to the difficulty in locating the damage. Even when pain-associated lesions are identified, TA is an emotional cognition-related experience. The brain affects the memory of the pain input, and related factors such as emotion and cognition can affect the patient’s experience (Tracey and Mantyh, 2007). It is difficult to study the ability of the brain to respond to non-specific memory and pain, which is largely reflected in pain caused by noxious stimuli (Mouraux et al., 2011). However, through functional methods such as fMRI, relevant information about brain pain can be obtained (Apkarian et al., 2005; Dunckley et al., 2005; Iannetti et al., 2005); many studies have used ReHo methods to explore pain-related diseases and this approach has a broad scope for further development (Table 3; Yu et al., 2012; Xie et al., 2013; Wang et al., 2014, 2015; Zhang et al., 2014; Ke et al., 2015; Wu et al., 2016; Tang et al., 2018). In addition, a study of rs-fMRI in patients with chronic cervical spondylosis showed that ReHo values in the left sensorimotor cortex and temporal lobe were significantly higher than those in normal subjects (Chen et al., 2018). This study also plays a background role in our research. ReHo has the advantage of determining the “global changes” in brain activity during pain and changes in neural activity in areas of the brain associated with pain. The pain experience is regulated by psychological, social, and other factors, with extensive inter-individual variations. Understanding how TA affects related brain mechanisms is crucial to customizing pain control for individual patients with dental disease.
The results of this study showed significant increases in ReHo values in the RLG, RSOG, LMOG, and RPG (Figure 4), indicating that the stimulation caused by TA affects the above four cerebral areas.
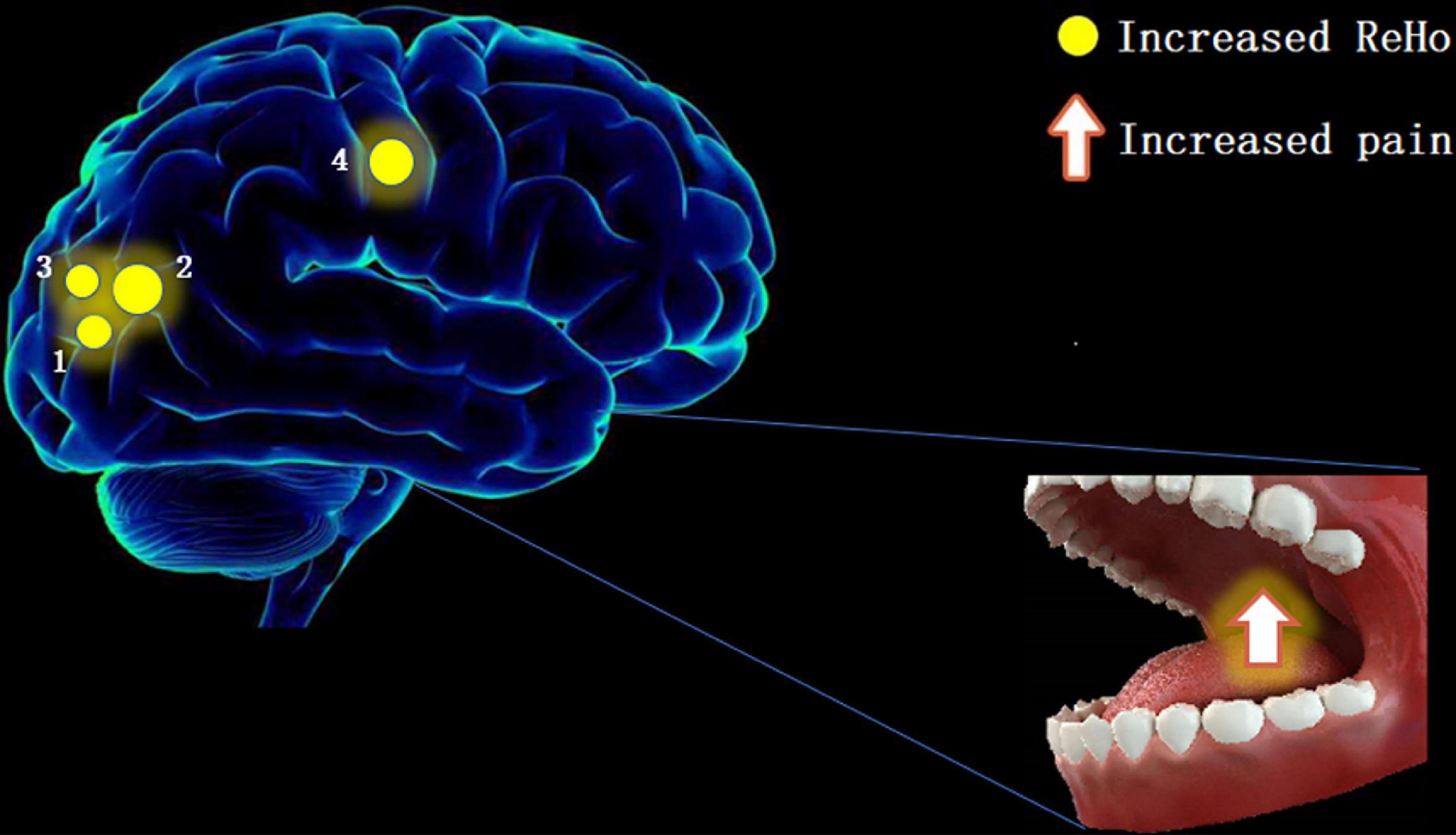
Figure 4. The ReHo results of brain activity in the TA group. Compared with the HCs, the ReHo value was increased in the following regions: 1—right lingual gyrus (BA19, t = 6.603), 2—right superior occipital gyrus (BA18, t = 6.7611), 3—left middle occipital gyrus (BA19, t = 6.4052), and 4—right postcentral gyrus (BA2, t = 6.9664). Spot size denotes the degree of quantitative changes. ReHo, regional homogeneity; TA, toothache; HC, healthy control.
The RLG is associated with emotional control and cognitive function. One study showed that the normal functioning of the lingual gyrus (LG) helped fight anxiety and depression and maintain normal cognitive function (Jung et al., 2014). Related studies further showed that in Alzheimer’s disease and depression, functional connectivity between LG and other cortical regions was decreased (Liu et al., 2017). Our study did not measure functional connectivity but we found that LG activity was increased in TA. Previous reports have indicated that TA experiences are associated with aversion emotions. Activation of the patient’s brain in disgust might mean that the individual was positively responding to painful stimuli, constantly reassessing the pain (Borsook et al., 2013; Baliki and Vania Apkarian, 2015). When the TA experience was related to aversion, activated brain areas included the frontal lobe, amygdala, hippocampus, and others (Duerden and Albanese, 2013; Lin et al., 2013b). These areas associated with TA have been described as the “cognitive-emotional” network, indicating that cognition and emotion play significant roles in the development of TA (Wiech et al., 2008). The main function of the occipital lobe is to process visual information. Furthermore, the RSOG responds preferentially to visual information about spatial characteristics (Renier et al., 2010). In addition, epidemiological studies have confirmed that patients with visual impairment have relatively poor periodontal health, and the incidence of gingival bleeding and dental calculus is higher than that of normal subjects, which increases the risk of toothache (Liu et al., 2019). However, the effect of toothache on visual dysfunction needs further study. On the other hand, other studies found a potential link between the lingual region of the occipital lobe and the hippocampus and amygdala, with a major change in mood due to TA associated with occipital gyrus activation (Isenberg et al., 1999; Cho et al., 2012). A related study in children also suggested that the SOG played a role in pediatric bipolar disorder (Wei et al., 2018). In addition, a resting-state imaging study showed that the functional state of the LMOG was associated with major depressive disorder in females (Teng et al., 2018). The alterations in RLG, RSOG, and LMOG activity in the present study can partially confirm these findings. The functional state of ReHo values in these three cerebral cortex regions was related to the body’s emotional and cognitive functions, and changes in their ReHo values may affect their functions.
Patients with TA often experience unbearable pain. It has been found that pain activates the primary (S1) and secondary (S2) somatosensory cortex, insular cortex, cingulate cortex, and parietal and thalamus regions. Some previous studies have suggested that many pain-related diseases activate the S1 region (Peyron et al., 2000; Apkarian et al., 2005; Frot et al., 2013). Gerstner et al. (2013) found by comparing harmful stimulation with a resting baseline that the somatosensory cortex, parietal lobe, and cingulate gyrus played key roles in oral pain. The right postcentral gyrus is part of the somatosensory cortex. Integrated stimulation of the somatosensory cortex, insula, and anterior central cingulate cortex may regulate the development of TA, and our experimental results can to some extent confirm this. We, therefore, consider that change in activity at the right postcentral gyrus was due to the stimulation of the brain caused by the TA itself. Table 4 shows the functions of the above cerebral regions and the diseases associated with dysfunction.
ROC curves can be used to distinguish between healthy and diseased states and higher AUC values (above 0.7) indicate higher diagnostic accuracy. In our research, the AUC values of each cortical region were all greater than 0.9, indicating good accuracy and suggesting that ReHo at the brain regions discussed above may be a practical method for the diagnosis of TA in the future. The present study had some limitations. First, the sample is small, limiting the reliability of the experimental results (Button et al., 2013). Second, there is a lack of standardized noxious stimulation in the field of pain research, so while many clinical factors can cause TA in patients, we could not evaluate these in a standardized manner. Third, the psychological characteristics of patients with TA vary, and we could not determine the connection between these characteristics and those related to brain activity.
In summary, using ReHo to study fMRI data was an effective way to study brain regional changes in a neural functional state in TA patients. Our results showed that areas of the brain in TA patients, including the somatosensory area, exhibited altered synchronous neural activity. These results provide a basis for further understanding of TA and relevant brain mechanisms and the development of customized pain control for individual dental patients.
Data availability statement
The original data can be obtained by contacting the corresponding author. Requests to access these datasets should be directed to Y-LH, MTczMzg2NDI0QHFxLmNvbQ==.
Ethics statement
This study was reviewed and approved by the Medical Ethics Committee of the first affiliated Hospital of Nanchang University (cdyfy2017021). All procedures performed in studies involving human participants were following the 1964 Helsinki declaration and its later amendments or comparable ethical standards.
Author contributions
JY and Y-LH contributed to the study inception and design. JY and YS equally contributed to the literature search, analysis, and writing of the manuscript. BiaL, BinL, Q-MG, Q-YL, Q-YY, R-BL, S-NW, and Y-CP contributed to the study design and study supervision. All authors approved the final version of the manuscript.
Funding
Key Research Foundation of Jiangxi Province (Nos: 20203BBG73059 and 20181BBG70004), Excellent Talents Development Project of Jiangxi Province (20192BCBL23020), Natural Science Foundation of Jiangxi Province (20181BAB205034), Grassroots Health Appropriate Technology “Spark Promotion Plan” Project of Jiangxi Province (No: 20188003), Health Development Planning Commission Science Foundation of Jiangxi Province (No: 20201032), and Health Development Planning Commission Science TCM Foundation of Jiangxi Province (No: 2018A060).
Conflict of interest
The authors declare that the research was conducted in the absence of any commercial or financial relationships that could be construed as a potential conflict of interest.
Publisher’s note
All claims expressed in this article are solely those of the authors and do not necessarily represent those of their affiliated organizations, or those of the publisher, the editors and the reviewers. Any product that may be evaluated in this article, or claim that may be made by its manufacturer, is not guaranteed or endorsed by the publisher.
References
Apkarian, A. V., Bushnell, M. C., Treede, R. D., and Zubieta, J. K. (2005). Human brain mechanisms of pain perception and regulation in health and disease. Eur. J. Pain 9, 463–463. doi: 10.1016/j.ejpain.2004.11.001
Baliki, M., and Vania Apkarian, A. (2015). Nociception, pain, negative moods, and behavior selection. Neuron 87, 474–491. doi: 10.1016/j.neuron.2015.06.005
Bijur, P. E., Silver, W., and Gallagher, E. J. (2001). Reliability of the visual analog scale for measurement of acute pain. Acad. Emerg. Med. 8, 1153–1157. doi: 10.1111/j.1553-2712.2001.tb01132.x
Biswal, B. B. (2012). Resting state fMRI: A personal history. Neuroimage 62, 938–944. doi: 10.1016/j.neuroimage.2012.01.090
Bjelland, I., Dahl, A. A., Haug, T. T., and Neckelmann, D. (2002). The validity of the hospital anxiety and depression scale. An updated literature review. J. Psychosom. Res. 52, 69–77. doi: 10.1016/S0022-3999(01)00296-3
Borsook, D., Edwards, R., Elman, I., Becerra, L., and Levine, J. (2013). Pain and analgesia: The value of salience circuits. Prog. Neurobiol. 104, 93–105. doi: 10.1016/j.pneurobio.2013.02.003
Brügger, M., Ettlin, D. A., Meier, M., Keller, T., Luechinger, R., Barlow, A., et al. (2011). Taking sides with pain–lateralization aspects related to cerebral processing of dental pain. Front. Hum. Neurosci. 5:12. doi: 10.3389/fnhum.2011.00012
Brügger, M., Lutz, K., Brönnimann, B., Meier, M., Luechinger, R., Barlow, A., et al. (2012). Tracing toothache intensity in the brain. J. Dent. Res. 91, 156–160. doi: 10.1177/0022034511431253
Buckner, R. L., Andrews-Hanna, J. R., and Schacter, D. L. (2008). The brain’s default network: Anatomy, function, and relevance to disease. Ann. N. Y. Acad. Sci. 1124, 1–38. doi: 10.1196/annals.1440.011
Button, K. S., Ioannidis, J. P., Mokrysz, C., Nosek, B. A., Flint, J., Robinson, E. S., et al. (2013). Power failure: Why small sample size undermines the reliability of neuroscience. Nat. Rev. Neurosci. 14, 365–376. doi: 10.1038/nrn3475
Chen, J., Wang, Z., Tu, Y., Liu, X., Jorgenson, K., Ye, G., et al. (2018). Regional homogeneity and multivariate pattern analysis of cervical spondylosis neck pain and the modulation effect of treatment. Front. Neurosci. 12:900. doi: 10.3389/fnins.2018.00900
Chen, S. Y., Cai, G. Q., Liang, R. B., Yang, Q. C., Min, Y. L., Ge, Q. M., et al. (2021). Regional brain changes in patients with diabetic optic neuropathy: A resting-state functional magnetic resonance imaging study. Quant. Imaging Med. Surg. 11, 2125–2137. doi: 10.21037/qims-20-453
Cho, S., Metcalfe, A. W., Young, C. B., Ryali, S., Geary, D. C., and Menon, V. (2012). Hippocampal–prefrontal engagement and dynamic causal interactions in the maturation of children’s fact retrieval. J. Cogn. Neurosci. 24, 1849–1866. doi: 10.1162/jocn_a_00246
Duerden, E. G., and Albanese, M. C. (2013). Localization of pain-related brain activation: A meta-analysis of neuroimaging data. Hum. Brain Mapp. 34, 109–149. doi: 10.1002/hbm.21416
Dunckley, P., Wise, R. G., Fairhurst, M., Hobden, P., Aziz, Q., Chang, L., et al. (2005). A comparison of visceral and somatic pain processing in the human brainstem using functional magnetic resonance imaging. J. Neurosci. 25, 7333–7341. doi: 10.1523/JNEUROSCI.1100-05.2005
Farrell, M. J., Laird, A. R., and Egan, G. F. (2005). Brain activity associated with painfully hot stimuli applied to the upper limb: A meta-analysis. Hum. Brain Mapp. 25, 129–139. doi: 10.1002/hbm.20125
Frot, M., Magnin, M., Mauguière, F., and Garcia-Larrea, L. (2013). Cortical representation of pain in primary sensory-motor areas (S1/M1)—a study using intracortical recordings in humans. Hum. Brain Mapp. 34, 2655–2668. doi: 10.1002/hbm.22097
Gerstner, G., Ichesco, E., and Schmidt-Wilcke, T. (2013). “Brain imaging of pain phenomena,” in Treatment of temporomandibular disorders: bridging the gap between advances in basic research and clinical pain management, eds S. G. Charles and D. M. Laskin (Milton Park: Taylor & Francis Ltd), 141–154.
Huang, X., Li, H.-J., Ye, L., Zhang, Y., Wei, R., Zhong, Y.-L., et al. (2016a). Altered regional homogeneity in patients with unilateral acute open-globe injury: a resting-state functional MRI study. Neuropsychiatr. Dis. Treat. 12:1901. doi: 10.2147/NDT.S110541
Huang, X., Li, S. H., Zhou, F. Q., Zhang, Y., Zhong, Y. L., Cai, F. Q., et al. (2016b). Altered intrinsic regional brain spontaneous activity in patients with comitant strabismus: A resting-state functional MRI study. Neuropsychiatr. Dis. Treat. 12, 1303–1308. doi: 10.2147/NDT.S105478
Iannetti, G. D., and Mouraux, A. (2010). From the neuromatrix to the pain matrix (and back). Exp. Brain Res. 205, 1–12. doi: 10.1007/s00221-010-2340-1
Iannetti, G., Zambreanu, L., Wise, R. G., Buchanan, T., Huggins, J., Smart, T., et al. (2005). Pharmacological modulation of pain-related brain activity during normal and central sensitization states in humans. Proc. Natl. Acad. Sci. U.S.A. 102, 18195–18200. doi: 10.1073/pnas.0506624102
Isenberg, N., Silbersweig, D., Engelien, A., Emmerich, S., Malavade, K., Beattie, B. A., et al. (1999). Linguistic threat activates the human amygdala. Proc. Natl. Acad. Sci. U.S.A. 96, 10456–10459. doi: 10.1073/pnas.96.18.10456
Jung, J., Kang, J., Won, E., Nam, K., Lee, M.-S., Tae, W. S., et al. (2014). Impact of lingual gyrus volume on antidepressant response and neurocognitive functions in major depressive disorder: A voxel-based morphometry study. J. Affect. Disord. 169, 179–187. doi: 10.1016/j.jad.2014.08.018
Ke, J., Qi, R., Liu, C., Xu, Q., Wang, F., Zhang, L., et al. (2015). Abnormal regional homogeneity in patients with irritable bowel syndrome: A resting-state functional MRI study. Neurogastroenterol. Motil. 27, 1796–1803. doi: 10.1111/nmo.12692
Kippenhan, J. S., Barker, W. W., Pascal, S., Nagel, J., and Duara, R. (1992). Evaluation of a neural-network classifier for PET scans of normal and Alzheimer’s disease subjects. J. Nucl. Med. 33, 1459–1467.
Lee, T.-W., Northoff, G., and Wu, Y.-T. (2014). Resting network is composed of more than one neural pattern: An fMRI study. Neuroscience 274, 198–208. doi: 10.1016/j.neuroscience.2014.05.035
Lin, C.-S., Hsieh, J.-C., Yeh, T.-C., Lee, S.-Y., and Niddam, D. M. (2013a). Functional dissociation within insular cortex: The effect of pre-stimulus anxiety on pain. Brain Res. 1493, 40–47. doi: 10.1016/j.brainres.2012.11.035
Lin, C.-S., Niddam, D., Hsu, M.-L., and Hsieh, J.-C. (2013b). Pain catastrophizing is associated with dental pain in a stressful context. J. Dent. Res. 92, 130–135. doi: 10.1177/0022034512467804
Liu, L., Zhang, Y., Wu, W., He, M., Lu, Z., Zhang, K., et al. (2019). Oral health status among visually impaired schoolchildren in Northeast China. BMC Oral Health 19:63. doi: 10.1186/s12903-019-0752-2
Liu, X., Chen, W., Hou, H., Chen, X., Zhang, J., Liu, J., et al. (2017). Decreased functional connectivity between the dorsal anterior cingulate cortex and lingual gyrus in Alzheimer’s disease patients with depression. Behav. Brain Res. 326, 132–138. doi: 10.1016/j.bbr.2017.01.037
Mouraux, A., Diukova, A., Lee, M. C., Wise, R. G., and Iannetti, G. D. (2011). A multisensory investigation of the functional significance of the “pain matrix”. Neuroimage 54, 2237–2249. doi: 10.1016/j.neuroimage.2010.09.084
Moussa, M. N., Steen, M. R., Laurienti, P. J., and Hayasaka, S. (2012). Consistency of network modules in resting-state FMRI connectome data. PLoS One 7:e44428. doi: 10.1371/journal.pone.0044428
Nixdorf, D. R., Drangsholt, M., Ettlin, D. A., Gaul, C., De Leeuw, R., Svensson, P., et al. (2012). Classifying orofacial pains: A new proposal of taxonomy based on ontology. J. Oral Rehabil. 39, 161–169. doi: 10.1111/j.1365-2842.2011.02247.x
Peyron, R., Laurent, B., and Garcia-Larrea, L. (2000). Functional imaging of brain responses to pain. A review and meta-analysis (2000). Neurophysiol. Clin. 30, 263–288. doi: 10.1016/S0987-7053(00)00227-6
Raichle, M. E., and Snyder, A. Z. (2007). A default mode of brain function: A brief history of an evolving idea. Neuroimage 37, 1083–1090. doi: 10.1016/j.neuroimage.2007.02.041
Renier, L. A., Anurova, I., De Volder, A. G., Carlson, S., VanMeter, J., and Rauschecker, J. P. (2010). Preserved functional specialization for spatial processing in the middle occipital gyrus of the early blind. Neuron 68, 138–148. doi: 10.1016/j.neuron.2010.09.021
Shao, Y., Cai, F. Q., Zhong, Y. L., Huang, X., Zhang, Y., Hu, P. H., et al. (2015). Altered intrinsic regional spontaneous brain activity in patients with optic neuritis: A resting-state functional magnetic resonance imaging study. Neuropsychiatr. Dis. Treat. 11, 3065–3073. doi: 10.2147/NDT.S92968
Tang, L.-Y., Li, H.-J., Huang, X., Bao, J., Sethi, Z., Ye, L., et al. (2018). Assessment of synchronous neural activities revealed by regional homogeneity in individuals with acute eye pain: A resting-state functional magnetic resonance imaging study. J. Pain Res. 11:843. doi: 10.2147/JPR.S156634
Tang, Y. L., Ji, G. J., Yu, Y., Wang, J., Wang, Z. J., Zang, Y. F., et al. (2014). Altered regional homogeneity in rolandic epilepsy: A resting-state FMRI study. Biomed. Res. Int. 2014:960395. doi: 10.1155/2014/960395
Tate, M. C., Herbet, G., Moritz-Gasser, S., Tate, J. E., and Duffau, H. (2014). Probabilistic map of critical functional regions of the human cerebral cortex: Broca’s area revisited. Brain 137(Pt 10), 2773–2782. doi: 10.1093/brain/awu168
Teng, C., Zhou, J., Ma, H., Tan, Y., Wu, X., Guan, C., et al. (2018). Abnormal resting state activity of left middle occipital gyrus and its functional connectivity in female patients with major depressive disorder. BMC Psychiatry 18:370. doi: 10.1186/s12888-018-1955-9
Tononi, G., McIntosh, A. R., Russell, D. P., and Edelman, G. M. (1998). Functional clustering: Identifying strongly interactive brain regions in neuroimaging data. Neuroimage 7, 133–149. doi: 10.1006/nimg.1997.0313
Tracey, I., and Mantyh, P. W. (2007). The cerebral signature for pain perception and its modulation. Neuron 55, 377–391. doi: 10.1016/j.neuron.2007.07.012
Van Dijk, K. R., Sabuncu, M. R., and Buckner, R. L. (2012). The influence of head motion on intrinsic functional connectivity MRI. Neuroimage 59, 431–438. doi: 10.1016/j.neuroimage.2011.07.044
Wang, P., Du, H., Chen, N., Guo, J., Gong, Q., Zhang, J., et al. (2014). Regional homogeneity abnormalities in patients with tensiontype headache: A resting-state fMRI study. Neurosci. Bull. 30, 949–955. doi: 10.1007/s12264-013-1468-6
Wang, Y., Zhang, X., Guan, Q., Wan, L., Yi, Y., and Liu, C.-F. (2015). Altered regional homogeneity of spontaneous brain activity in idiopathic trigeminal neuralgia. Neuropsychiatr. Dis. Treat. 11:2659. doi: 10.2147/NDT.S94877
Wei, M., Gao, W., Jiao, Q., Cui, D., Cao, W., Xu, Q., et al. (2018). Resting-state functional connectivity of occipital cortex in euthymic pediatric bipolar disorder patients. J. Med. Imaging Health Inform. 8, 28–32. doi: 10.1166/jmihi.2018.2227
Wiech, K., Ploner, M., and Tracey, I. (2008). Neurocognitive aspects of pain perception. Trends Cogn. Sci. 12, 306–313. doi: 10.1016/j.tics.2008.05.005
Wu, S. N., Zhang, M. Y., Shu, H. Y., Liang, R. B., Ge, Q. M., Pan, Y. C., et al. (2020). Changes in functional connectivity of specific cerebral regions in patients with toothache: A resting-state functional magnetic resonance imaging study. Dis. Markers 2020:6683161. doi: 10.1155/2020/6683161
Wu, T.-H., Tu, C.-H., Chao, H.-T., Li, W.-C., Low, I., Chuang, C.-Y., et al. (2016). Dynamic changes of functional pain connectome in women with primary dysmenorrhea. Sci. Rep. 6:24543. doi: 10.1038/srep24543
Xie, H., Xu, F., Chen, R., Luo, T., Chen, M., Fang, W., et al. (2013). Image formation of brain function in patients suffering from knee osteoarthritis treated with moxibustion. J. Trad. Chine. Med. 33, 181–186. doi: 10.1016/S0254-6272(13)60122-3
Yang, J., Li, B., Yu, Q. Y., Ye, L., Zhu, P. W., Shi, W. Q., et al. (2019). Altered intrinsic brain activity in patients with toothaches using the amplitude of low-frequency fluctuations: a resting-state fMRI study. Neuropsychiatr. Dis. Treat. 15, 283–291. doi: 10.2147/NDT.S189962
Yu, D., Yuan, K., Zhao, L., Zhao, L., Dong, M., Liu, P., et al. (2012). Regional homogeneity abnormalities in patients with interictal migraine without aura: A resting-state study. NMR Biomed. 25, 806–812. doi: 10.1002/nbm.1796
Yue, Y., Jiang, Y., Shen, T., Pu, J., Lai, H. Y., and Zhang, B. (2020). ALFF and ReHo mapping reveals different functional patterns in early- and late-onset Parkinson’s disease. Front. Neurosci. 14:141. doi: 10.3389/fnins.2020.00141
Zang, Y., Jiang, T., Lu, Y., He, Y., and Tian, L. (2004). Regional homogeneity approach to fMRI data analysis. Neuroimage 22, 394–400. doi: 10.1016/j.neuroimage.2003.12.030
Zeng, L. L., Wang, D., Fox, M. D., Sabuncu, M., Hu, D., Ge, M., et al. (2014). Neurobiological basis of head motion in brain imaging. Proc. Natl. Acad. Sci. U.S.A. 111, 6058–6062. doi: 10.1073/pnas.1317424111
Keywords: toothache, regional homogeneity, rs-fMRI, neuroimaging, disease markers
Citation: Yang J, Shao Y, Li B, Yu Q-Y, Ge Q-M, Li B, Pan Y-C, Liang R-B, Wu S-N, Li Q-Y and He Y-L (2022) Altered regional homogeneity of spontaneous brain activity in patients with toothache: A resting-state functional magnetic resonance imaging study. Front. Neurosci. 16:1019989. doi: 10.3389/fnins.2022.1019989
Received: 15 August 2022; Accepted: 05 September 2022;
Published: 28 September 2022.
Edited by:
Yuzhen Xu, Tongji University, ChinaReviewed by:
Shangkun Ou, Xiamen University, ChinaXuzhou Li, Yunnan Normal University, China
Ce Shi, Zhejiang University, China
Copyright © 2022 Yang, Shao, Li, Yu, Ge, Li, Pan, Liang, Wu, Li and He. This is an open-access article distributed under the terms of the Creative Commons Attribution License (CC BY). The use, distribution or reproduction in other forums is permitted, provided the original author(s) and the copyright owner(s) are credited and that the original publication in this journal is cited, in accordance with accepted academic practice. No use, distribution or reproduction is permitted which does not comply with these terms.
*Correspondence: Yu-Lin He, MTczMzg2NDI0QHFxLmNvbQ==
†These authors have contributed equally to this work