- 1Rehabilitation Innovation Centre, Health and Rehabilitation Research Institute, School of Clinical Science, Auckland University of Technology, Auckland, New Zealand
- 2Department of Pharmacology and Toxicology, School of Biomedical Sciences, The Brain Health Research Centre, University of Otago, Dunedin, New Zealand
- 3Eisdell Moore Centre for Hearing and Balance Research, University of Auckland, Auckland, New Zealand
- 4Department of Physiology, University of Auckland, Auckland, New Zealand
Objective: Noisy galvanic vestibular stimulation (nGVS) has been used to boost vestibular afferent information to the central nervous system. This has the potential to improve postural control for people for whom vestibular signals are weak, such as in bilateral vestibulopathy (BVP). The aim of this systematic review and meta-analysis is to investigate the evidence for nGVS as a modality to improve postural control in people with BVP.
Methods: A comprehensive systematic search was conducted of five databases up to July 2022 to find studies applying nGVS to people with BVP, with the aim of improving postural control. Two independent reviewers screened and identified eligible studies, completed a risk of bias evaluation (Cochrane) and extracted relevant data. The standardized mean difference (SMD) based on Hedges' g was calculated as a measure of effect size for the primary outcome measure that best identified postural control, and a forest plot generated.
Results: Seven studies met the eligibility criteria, with five being suitable for meta-analysis. Meta-analysis revealed a moderate effect in favor of nGVS improving postural control during standing and walking [pooled SMD = 0.47 95% CI (0.25, 0.7)]. nGVS-mediated improvements in postural control were most evident in observations of reduced sway velocity when standing on a firm surface with eyes closed, and in the reduced variability of gait parameters, particularly those measuring lateral stability.
Conclusions: Coincident nGVS in people with BVP improves postural control during standing and walking. This improvement appears to be context specific, in that vestibular augmentation is most effective in situations where visual inputs are limited, and where reliable context specific proprioceptive cues are available. Further research is warranted investigating additional circumstances in which nGVS improves postural control, including investigating the residual, and sustained effects of nGVS.
Systematic review registration: https://www.crd.york.ac.uk/prospero/display_record.php?RecordID=342147, identifier: 342147.
Introduction
The vestibular system and bilateral vestibulopathy
The vestibular system is a closed system residing in the bony vault of the temporal bone and is extensively involved in the control of balance and eye movement. Consisting of the three semicircular canals and the two otolith organs (the utricle and saccule), the vestibular system relies on the inertial drag of endolymph and the static effects of gravity on its membranous labyrinth, to mechanically stimulate sensors that provide information to the brain about head position and movement of the head in space (Schubert and Minor, 2004). At a basic level it contributes to visual stability via the vestibulo-ocular reflexes and the maintenance of muscle tone, body and head posture and balance via the vestibulospinal reflexes (Schubert and Minor, 2004; Smith, 2017). See Schubert and Minor (2004) and Cullen (2019) for an extensive review of the physiological function of the vestibular system.
Bilateral vestibulopathy (BVP) is a diagnosis indicating that the neural signals that travel from the vestibular apparatus to the central nervous system are either absent or significantly reduced on both sides (Lucieer et al., 2020). There are multiple potential causes for BVP. Damage to the sensory organs of the vestibular system, the semicircular canals, utricle or saccule may occur in Meniere's disease, mechanical trauma, labyrinthitis or aminoglycoside toxicity (Kim and Kim, 2022) or the vestibular nerve may be impaired through neuritis, vestibular schwannoma or resection of the vestibular nerve (Kim and Kim, 2022). Around half of BVP cases are idiopathic, making the pathological process difficult to ascertain (Hain et al., 2018; Kim and Kim, 2022).
The reduced neural signals traveling from the vestibular apparatus to the central nervous system in people with BVP lead to imbalance, oscillopsia (visual blurring with head movement) and difficulty walking in darkness or over uneven surfaces (Ward et al., 2013; Lucieer et al., 2018, 2020). People also report deficits in spatial navigation, orientation (Schoberl et al., 2021), concentration, memory (Lucieer et al., 2018) and mood (Lucieer et al., 2020). As a result of these symptoms, the majority of patients report altering their behavior by avoiding activities, assigning more concentration to tasks, or completing tasks more slowly (Lucieer et al., 2020), and 35% of BVP patients perceive their vestibular loss as severely impacting their participation in daily activities (Dobbels et al., 2020).
Vestibular hair cells and neurons do not regenerate, and there is currently no established remedial medical treatment for BVP once damage has occurred (Guinand et al., 2012; Kim and Kim, 2022). To date, the gold standard treatment has been vestibular rehabilitation, which relies on central compensation and reweighting of other sensory inputs (Hall et al., 2016; Hain et al., 2018). However, vestibular rehabilitation has had modest and somewhat inconsistent effects (Herdman et al., 2015). An emerging treatment option is noisy galvanic vestibular stimulation (nGVS), which is a low-level noisy current that has been used successfully to facilitate both postural stability and vestibular ocular reflex activity (Stefani et al., 2020).
Noisy galvanic vestibular stimulation
Noisy galvanic vestibular stimulation (nGVS) applied to the bilateral mastoid processes has been investigated over the past two decades as a treatment to improve balance and postural control (Iwasaki et al., 2014, 2018; Fujimoto et al., 2016, 2018; Wuehr et al., 2016a,b; Inukai et al., 2018a, 2020a,b,c; Ko et al., 2020; Chen et al., 2021; Nooristani et al., 2021). Delivered via electrodes placed bilaterally over the mastoid processes, nGVS is a zero mean, noisy galvanic current applied over a fixed bandwidth. While the exact mechanism of nGVS is unknown, the benefits have been observed primarily at low amplitudes (Iwasaki et al., 2014), leading to the assumption nGVS amplifies weak vestibular afferent signals via stochastic resonance (Wuehr et al., 2018). Stochastic resonance is a phenomenon during which a non-linear system that is operating at a subthreshold level is boosted by adding noise, bringing the system up to threshold (Galvan-Garza et al., 2018). In the vestibular system this increases the likelihood of neural firing and facilitates the restoration of missing sensory information to the central nervous system (Moss et al., 2004). When a weak vestibular signal is boosted by the addition of a small nGVS signal, the performance of the vestibular system is enhanced, and balance improves (Dlugaiczyk et al., 2019). The aim of this systematic review and meta-analysis is to evaluate the efficacy of nGVS as a modality to improve postural control in people with BVP.
Methods
A literature search was undertaken using EBSCO (CINAHL plus, MEDLINE, SPORTDiscus), Scopus, Ovid (AMED) and Medline (PubMed). We used the search terms “bilateral vestibulopathy OR BVP OR bilateral vestibular weakness OR bilateral vestibular OR bilateral vestibular hypofunction OR BVH”; “AND nGVS OR noisy galvanic stimulation OR noisy vestibular stimulation OR galvanic vestibular stimulation OR GVS OR SVS OR stochastic vestibular stimulation.” Additional studies were identified by hand-searching the reference lists of key articles. Studies were restricted to peer reviewed journals with full text available in English, no limit was placed on the publication date or study design.
Two independent reviewers (RM, SR) screened titles and abstracts, and where necessary, the full text for eligibility according to the criteria in Table 1. In the case of any uncertainty, a third reviewer (DT) was consulted until consensus was achieved. The literature search was last performed on July 13, 2022.
Data were extracted directly from the text, tables, supplementary files and where necessary from graphs using online software (https://apps.automeris.io/wpd/). Data extracted included, study design, sample size, participant characteristics, primary and secondary outcome measurements related to postural control, and study findings. Normalized ratio or percentage were calculated as appropriate.
Risk of bias and trial quality assessment
The Cochrane risk of bias assessment (RoB2) for a randomized crossover design was applied to studies in the meta-analysis (Higgins et al., 2011). Data were extracted from journal articles and directly from the authors where available. Using the RoB2 algorithm, studies were assessed with respect to the randomization process (domain 1), bias arising from period and carryover effects (domain S), deviations from the intended interventions (domain 2), missing outcome data (domain 3), measurement of the outcome (domain 4), and selection of the reported results (domain 5). Each domain was categorized as low risk, some concerns or high risk of bias and subsequently an overall risk category was given for each study.
Meta-analysis
To be included in the meta-analysis studies were required to assess a postural control measure coincident to nGVS as well as a sham or no nGVS condition. Data were extracted for the primary outcome measure that best identified postural control. Only one outcome measure was included from each study. As the studies differed in the reported outcome measures, a standardized mean difference (SMD) along with its standard error (SE) was estimated for each study using Hedges' g formula for repeated measures (Borenstein et al., 2009). The SMD and SE calculation was derived from means, standard deviations, standard errors and 95% confidence intervals (CIs) reported by the studies. The SMD represented the treatment effect of active nGVS compared to sham nGVS or no nGVS on postural control of people with BVP. The SMDs were pooled using a fixed effects meta-analysis model using the metafor package version 3.4 in the R environment for statistical computing (Viechtbauer, 2010). A fixed effects model rather than a random effects model was used as the Cochrane test of heterogeneity suggested a non-significant heterogeneity at a type-I error rate of 5%. Heterogeneity across studies was also reported using the I2 statistic. The pooled SMD was considered statistically significant if its 95% confidence interval did not cross zero.
Results
After duplicates were removed the search yielded a total of 141 articles. After the title and abstract screening, 129 were removed as irrelevant and 12 articles went forward for full text review. Five articles were excluded at this stage and seven were retained for data extraction (Figure 1).
Description of included studies
A total of seven studies were included in this systematic review involving 112 participants with BVP. All participants met the Bárány Society criteria for either full or probable BVP (Table 2) (Strupp et al., 2017). For 66 of the 112 participants the underlying pathology was reported (Table 3), for the others it was not.
Study design
Studies identified in this review are summarized in Table 4. Five studies used a crossover design and assessed the coincident effect of nGVS on postural control (Iwasaki et al., 2014, 2018; Wuehr et al., 2016a; Sprenger et al., 2020; Chen et al., 2021). The meta-analysis was performed on these five studies. One study used a pre/post experimental design and assessed the post-stimulation effects of nGVS on postural control (Fujimoto et al., 2018) and one was a randomized controlled pilot study (Eder et al., 2022). Six studies explored the efficacy of a single session of nGVS (Iwasaki et al., 2014, 2018; Wuehr et al., 2016a; Fujimoto et al., 2018; Sprenger et al., 2020; Chen et al., 2021). One study evaluated the cumulative effect of multiple sessions of nGVS combined with vestibular rehabilitation (Eder et al., 2022). All studies utilized some method of determining the optimum amplitude of nGVS (Table 4). The methodological quality of the crossover studies used to generate the meta-analysis demonstrated a range from no risk to some risk of bias over the six domains, with all studies categorized overall by the Cochrane RoB2 algorithm as having some risk of bias (Figure 2). The primary risk noted in studies was related to the risk of bias arising from period effects in the Cochrane RoB2 (domain S).
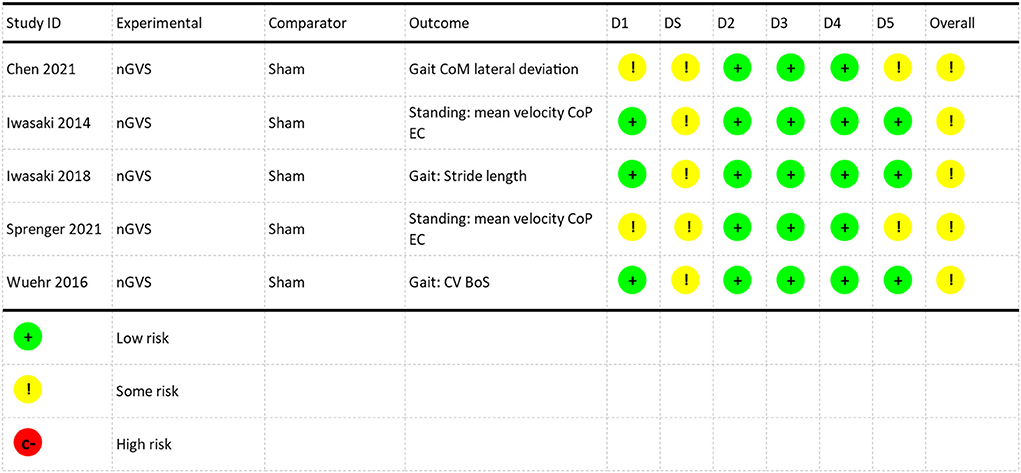
Figure 2. Risk of bias assessment. D1: Randomization Process, DS: Bias arising from period and carryover effects, D2: Deviations from the intended interventions, D3: missing outcome data, D4: Measurement of the outcome, D5: Selection of the reported results. NGVS, Noisy galvanic vestibular stimulation; CoM, center of mass; CoP, center of pressure; EC, eyes closed; CV, coefficient of variation; BoS, base of support.
Effect of nGVS on standing balance
Meta-analysis of the five studies assessing the coincident effect of nGVS revealed a moderate effect in favor of nGVS improving postural control during standing and walking (pooled SMD = 0.47 95% CI, [0.25,0.07]) (Figure 3) (Brydges, 2019). There was non-significant heterogeneity in the sample (I2 = 2.1%, p = 0.39).
The coincident effect of nGVS on postural control was evaluated with nGVS actively stimulating the participant during the measurement period. The post-stimulation effect of nGVS on postural control was measured after active nGVS ceased, with the period described as time since the nGVS current stopped.
Standing with vision available
One study (Sprenger et al., 2020) investigated the coincident effect of nGVS on sway velocity whilst standing with eyes open on a firm surface and on foam. They found no significant effect of nGVS on postural control (Table 5).
Standing with vision eliminated
Three studies investigated nGVS in standing with vision eliminated on a firm surface (Table 6) (Iwasaki et al., 2014; Fujimoto et al., 2018; Sprenger et al., 2020). They found an immediate reduction in sway velocity with coincident nGVS of 1.19 cm/s (normalized ratio (NR) 0.68, p < 0.001) (Iwasaki et al., 2014) and 1.52 cm/s (NR 0.75, p < 0.006) (Sprenger et al., 2020) with a post- stimulation effect lasting for 3 h post-stimulation (NR 0.77, p < 0.01 at zero mins post stimulation and NR 0.85, p < 0.001 at 3 h post stimulation) (Fujimoto et al., 2018). Sprenger et al. (2020) noted the benefit appeared to be weighted toward BVP participants with higher motion perception thresholds when stimulated with galvanic vestibular stimulation (NR 0.75, p < 0.006) compared to participants with BVP who had a motion perception threshold within the normal range (NR 0.92, p > 0.05). In the only trial that measured standing on a foam surface with eyes closed, Sprenger et al. (2020) reported that during stimulation nGVS did not have a significant effect on mean sway velocity in this condition (Table 7).
Effect of nGVS on gait
Spatiotemporal gait parameters
During over-ground walking, gait speed and stride length increased, and stride time decreased with coincident nGVS (Iwasaki et al., 2018). There was no effect during paced treadmill walking (Table 8) (Wuehr et al., 2016a).
Gait variance measures
Of the three studies that measured the effect of coincident nGVS on gait variance, there was a significantly reduced variability in gait parameters in two of the studies (Table 9). This was particularly evident in base of support and step width measures with a 51.1% reduction in SD of step width (p = 0.009) (Chen et al., 2021) and a 13.1% (p < 0.05) reduction in the coefficient of variation of base of support (Wuehr et al., 2016a) during walking at the participants' preferred speed. There was no significant change in the coefficient of variation of stride time, except at slow speed (Wuehr et al., 2016a) where it was 32.2% less variable (p < 0.05). This reduction in gait variance and improvement in stability was also noted in more complex gait tasks, for example, whilst walking with head turns (Chen et al., 2021).
nGVS augmented vestibular rehabilitation
In the only study to report the effects of nGVS-augmented rehabilitation, Eder et al. (2022) reported that 6 sessions of nGVS over 2 weeks together with vestibular rehabilitation did not significantly reduce postural sway in people with BVP. There was no difference in postural control measures between the experimental group receiving nGVS plus vestibular rehabilitation and the control group receiving sham nGVS plus vestibular rehabilitation (Eder et al., 2022).
Discussion
As the first systematic review and meta-analysis of the effectiveness of nGVS in people with BVP, this work advances the understanding of this modality and the contexts where nGVS may have the most efficacy. Meta-analysis demonstrated that the coincident effect of nGVS on postural stability in people with BVP was to improve balance. The very low heterogeneity of the results indicates that the results across studies were consistent, with little variability. All sampled studies favored nGVS with consistent effect sizes (>0.5), excepting one study which reported a smaller effect size (0.22).
Effect of pathology, location, and severity on efficacy of nGVS
While the neurophysiological basis of nGVS is not fully understood, it seems likely that the extent of peripheral vestibular integrity and baseline postural stability makes a difference to a person's responsiveness to nGVS (Fujimoto et al., 2019a; Herssens and McCrum, 2019; Lajoie et al., 2021). Evidence points to nGVS being most effective in people with suboptimum vestibular function, yet at the same time some intact vestibular hair cells and nerve afferents are required to enable a vestibular afferent signal to be transmitted and reach the CNS (Dlugaiczyk et al., 2019; Lajoie et al., 2021). Clinical evidence supports this assertion. nGVS was not effective in people with complete bilateral loss (Schniepp et al., 2019), whereas people with incomplete vestibular loss exhibited a significant improvement in postural control with nGVS compared to those with normal vestibular function (Nooristani et al., 2021).
The Barány Society's criteria for the diagnosis of BVP is based on horizontal semicircular canal function. Consequently, the significance of residual function from the remaining vestibular organs in determining responsiveness to nGVS is unclear. Two studies in people with BVP included individual patient data on saccular function based on raw cervical vestibular evoked myogenic potential (cVEMP) amplitudes (Wuehr et al., 2016a; Iwasaki et al., 2018). cVEMP amplitudes represent modulation of motor potentials recorded from the sternocleidomastoid muscle in response to acoustic stimulation of the saccule and are used to illustrate the integrity of the sacculocollic neural pathway (Colebatch and Rothwell, 2004). No significant association has been found between saccular function and responsiveness to nGVS to date (Wuehr et al., 2016a). However, the nuances around residual function of the vestibular system require further investigation, in particular, the role of high frequency vestibular afferents (Bae et al., 2021) and otolith function (Keywan et al., 2019) in the potential for restoration of postural control. Due to the rarity of BVP, we encourage more authors to make supplementary files available, with deidentified information regarding individual vestibular assessment results and the participants' response to nGVS, in order to contribute to the identification of participants for whom nGVS is most likely to be effective in the future.
Standing balance
Coincident effects of nGVS on standing postural control
nGVS had no significant effect on postural control when people stood with their eyes open (Table 9), regardless of proprioceptive afferent input (i.e., standing on a firm surface or standing on foam) (Sprenger et al., 2020). In contrast, older adults without BVP had a significant reduction in mean sway velocity standing with eyes open (p = 0.005) (Inukai et al., 2018a), and in studies of healthy young people the results have been mixed: showing benefits with nGVS for some but not all participants (Inukai et al., 2020a,b; Matsugi et al., 2020). In people with BVP it is likely that there is longstanding CNS reweighting of the balance response toward utilization of visual afferent input when vision is available (Medendorp et al., 2018; Helmchen et al., 2019). CNS reweighting to adapt to the facilitated vestibular input by nGVS is likely to require longer than a 30 s exposure. This may contribute to the lack of immediate benefit from nGVS augmented vestibular input in people with BVP. Conversely, older adults have unavoidable deterioration in vision due to age related changes to the anatomy of the eye, reducing the quality of visual input to the CNS (Saftari and Kwon, 2018). Whilst vision is still considered a critical component of the older adult balance response (Saftari and Kwon, 2018), adaptive changes to the CNS reweighting of afferent inputs may predispose them to having greater sensitivity to vestibular augmentation.
Two studies investigating the balance response of people with BVP standing on a firm surface with eyes closed demonstrated a significant reduction in sway velocity (Iwasaki et al., 2014, 2018; Sprenger et al., 2020). In the absence of vision, reweighting of the postural control mechanism to increase reliance on vestibular and proprioceptive afferent information occurs (Asslander and Peterka, 2016). Accordingly, once the visual system was removed from the balance response, augmentation of the vestibular pathway appears to have benefit. Sprenger et al. (2020) found that this response was specific to people in their high threshold group. The high threshold group required higher amplitude GVS stimulation to reach vestibular firing threshold (as determined by perception of motion in response to galvanic stimulation). This group also had higher baseline sway velocity, suggesting greater initial postural instability. These findings are consistent with conclusions drawn in other studies. Nooristani et al. (2021) found older adults with vestibular impairment showed more responsiveness to nGVS stimulation than those with intact vestibular systems, and Inukai et al. (2018a,b); Inukai et al. (2020c) found nGVS was more effective at improving balance in people who present with some baseline postural instability.
Once balance is heavily weighted to be reliant on the vestibular system by removing vision and reducing proprioceptive information (standing on foam with their eyes closed), postural sway has high variability in all populations (Rashid et al., 2021). In the one study that investigated people with BVP standing on foam with their eyes closed nGVS did not have a significant effect on sway velocity (Sprenger et al., 2020). This pattern has also been seen in healthy young adults (Inukai et al., 2020a,b; Matsugi et al., 2020; Asslander et al., 2021), and while overall Mulavara et al. (2011) and Goel et al. (2015) concluded nGVS improved postural stability, when we look at individual participant results, only approximately half the participants in these two studies had reduced sway velocity standing on foam with their eyes closed. While it seems intuitive that augmenting the vestibular system should improve balance when the task is dependent on vestibular inputs, there are several reasons why we may not see the anticipated improvement in healthy populations or in people with BVP. Firstly, standing on a compliant surface is a complex postural task influenced not only by sensory information but also by the task, environment, and context (Shumway-Cook and Woollacott, 2007). The vestibular system provides information on position and movement of the head with respect to gravity, however, without the context of visual or somatosensory information, the vestibular system cannot distinguish the importance and relevance of postural change (Bayot et al., 2018). Therefore, even with facilitation to bring the vestibular signal up to threshold, in the absence of proprioception and vision, the vestibular system may not be sufficiently sensitive to maintain stability. Secondly, measuring velocity of the center of pressure is not a true record of body sway but more accurately measures the activity of the motor system moving the center of pressure (Ruhe et al., 2011). Therefore, a task such as standing on foam with eyes closed, where maintaining the center of mass within the base of support requires extensive exertion of the muscles around the ankles, may reduce the sensitivity of this measure as an indication of postural sway (Matsugi et al., 2020). This is a thought-provoking area for further research that will help us understand the potential for nGVS as a treatment modality in more complex multifactorial tasks with diminished afferent sensory information.
Lasting effects of nGVS on postural control
The sustained effects of nGVS on postural control are not yet clear. Fujimoto et al. (2018) investigated the sustained effects of nGVS on people with BVP, finding that 30 mins of stimulation significantly reduced sway velocity for 3 h after nGVS was removed. These findings are comparable to those found in older adults where a significant effect on postural sway was evident for up to 4 h after stimulation with a second burst of 30 mins nGVS sustaining this effect for a further 4 h (Fujimoto et al., 2016). However, these studies have been criticized for the absence of a comparative control group using sham stimulation, as the use of repeated measures creates risk of a learning effect that may influence the results. Nooristani et al. (2019b) investigated post-stimulation effects of nGVS in healthy people, using a comparative sham group. They found a significant reduction in sway velocity immediately after, and at 1 h post stimulation, in both the nGVS and sham nGVS groups with no significant difference between groups. Similarly, in a sham-controlled crossover study Keywan et al. (2020) found no significant effect on motion perception immediately after, or 30 mins subsequent to nGVS or sham nGVS. These findings support the concerns of bias in earlier studies. Further investigations using a sham stimulation control group will help clarify any continued effects of stimulation.
Effect of nGVS on gait
Spatiotemporal parameters of gait
Two studies have investigated the effect of nGVS on the spatiotemporal parameters of gait in people with BVP. Iwasaki et al. (2018) found that nGVS increased gait speed and stride length, and decreased stride time, in people with BVP during overground walking. A similar effect was found in healthy young people during overground walking and walking on unsteady surfaces (Piccolo et al., 2020). To the contrary, Wuehr et al. (2016a,b) found there was no change in spatiotemporal gait parameters in healthy or BVP populations during walking at slow, preferred, and fast speeds. However, because their studies were on a treadmill and gait speed was predetermined, the influence of the treadmill pacing may have had an overriding influence (Wuehr et al., 2016a,b). Further research is required to determine whether gait speed and its associated parameters are affected by nGVS.
Three studies have reported on gait variance measures in people with BVP, with studies reporting significantly reduced gait variability particularly in step width and base of support, the lateral components of gait stability (Wuehr et al., 2016a; Iwasaki et al., 2018; Chen et al., 2021). This finding is noteworthy, as lateral gait variance has been cited as a defining feature of gait imbalance in people with BVP (Schniepp et al., 2019). Decreased step width variability (Wuehr et al., 2016b; Piccolo et al., 2020) and improved stability during lateral perturbation of the support surface (Mulavara et al., 2015) have also been found in nGVS studies on young healthy people, strengthening support for the role of nGVS to augment vestibular afferents and maintain stable gait (Schniepp et al., 2019).
Gait with head turns
The vestibular system has a critical role stabilizing vision via the vestibulo-ocular and vestibulospinal reflexes. To this end researchers have looked at gait with head turns to challenge the vestibular system during gait. This task is a demanding one for both healthy people and people with BVP (Ko et al., 2020). Ko et al. (2020) assessed coherence to 2 Hz turning during gait and discovered significantly improved coherence to 2 Hz head turns during gait with nGVS in people with BVP (0.35 ± 0.24 Hz without nGVS, 0.46 ± 0.28 Hz with nGVS), and healthy young people (p = 0.018). Nevertheless, despite the challenge of gait with head turns, Chen et al. (2021) found a significant reduction in the standard deviation of stride width walking with head turns with both eyes open and closed, suggesting that nGVS can offer increased stability during gait even during a more challenging task. They also found reduced gait deviation during gait with head turns when the eyes were closed, but not when the eyes were open. This supports the visual system's dominant role in the control of gait trajectory when vision is available. Further research into gait in complex situations will help us understand more about the vestibular contribution to stability and how nGVS may influence mobility in real life situations which are innately more complex and unpredictable than laboratory-based investigations.
Overall, there is limited research into the effect of nGVS on gait. However, research to date has identified the potential of nGVS to improve gait stability in people with BVP even during challenging tasks. This is particularly relevant, as gait stability is one of the primary long term deficits affecting people with BVP (Wuehr et al., 2022a).
Effect of nGVS over the longer term
While there is good evidence for the immediate benefit of nGVS to improve postural stability during standing and walking with some residual effect following the removal of the stimulation, there has been speculation about the effect of nGVS as an adjunct to rehabilitation (Iwasaki et al., 2014; Chen et al., 2021; Lotfi et al., 2021). Eder et al. (2022) piloted nGVS as an adjunct to vestibular rehabilitation in people with BVP for 30 mins three times a week for 2 weeks. They found that vestibular rehabilitation for 2 weeks yielded moderate improvements in functional mobility as measured by the timed up and go, and functional gait analysis, but found no significant difference between the nGVS and sham group on any outcome measures. There were no reports of dropouts or adverse events in this study, suggesting this higher dose of nGVS spread over weeks is feasible and acceptable. Future research would benefit from investigating augmentation of vestibular rehabilitation over a longer duration, more in line with clinical practice (Hall et al., 2021). Ensuring the outcome measures used have been demonstrated to be sensitive to nGVS in people with BVP may also provide a more accurate representation of any effect.
Limitations
While research into nGVS as a modality to improve balance has been investigated for several decades now, there remains limited high-quality research in people with BVP, and notably, a lack of research including a comparative control group (Nooristani et al., 2019b; Sprenger et al., 2020). Future research will benefit from inclusion of individual participants' vestibular assessment results and responsiveness to nGVS, helping build our understanding of who is most likely to respond to nGVS.
While there is acknowledgment in the literature that the parameters of the nGVS waveform are important (Dlugaiczyk et al., 2019; Fujimoto et al., 2019b; Herssens and McCrum, 2019; Stefani et al., 2020; Lajoie et al., 2021), the limited systematic evaluation of parameter settings, and variability in individual's response to nGVS make it unclear how the choice of stimulation parameters may affect responsiveness and outcomes. Within the studies evaluated in this review some of the contradictory results of the effect of nGVS on postural stability may be related to the different methods utilized to optimize the parameters. In the five studies used for meta-analysis, current amplitude was determined by four different means; postural sway velocity (Iwasaki et al., 2014; Chen et al., 2021), cutaneous sensory threshold (Wuehr et al., 2016a), motion perception threshold (Sprenger et al., 2020) and gait velocity (Iwasaki et al., 2018). Further understanding of the science underpinning the choice of nGVS parameters and how to determine their optimal settings is required to develop this work.
As much of the initial nGVS research was exploratory there are some methodological issues that are only now being redressed. The research upon which our theoretical underpinnings are based has been primarily performed in young healthy adults whom we now understand respond differently to nGVS than those with higher postural sway or vestibular impairment (Fujimoto et al., 2019a; Nooristani et al., 2021). These initial studies have often looked at multiple intensities, yet only reported on the intensity related to optimal performance (Goel et al., 2015; Mulavara et al., 2015; Fujimoto et al., 2016; Iwasaki et al., 2018) or have examined one intensity compared to sham stimulation (Inukai et al., 2018a,b, 2020a,b,c; Nooristani et al., 2019a,b; Matsugi et al., 2020). Research investigating performance alterations across a broad spectrum of stimulation intensities to account for the presence or absence of stimulation-induced stochastic resonance is starting to fill these gaps in our knowledge (Galvan-Garza et al., 2018; Asslander et al., 2021; Wuehr et al., 2022b). Investigation of parameter induced patterns of performance across a range of frequencies and amplitudes in people with reduced vestibular function or high baseline postural sway is required to advance our understanding of nGVS.
Conclusions
The meta-analysis demonstrated that nGVS has a moderate coincident effect to improve postural stability in people with BVP. However, these improvements are context specific. Where the visual system can dominate, enhancing the vestibular system does not improve postural stability. When the visual input is eliminated but there is sufficient somatosensory afferent feedback, nGVS reduces sway velocity improving balance, particularly in the unsteady; those with high baseline sway. When visual input and somatosensory input are removed (standing on foam with eyes closed), augmenting the vestibular system has no effect on postural control. During gait, even challenging gait with eyes closed and head turns, nGVS improved stability by reducing gait variability. Overall, these findings support further research into the use of nGVS over longer periods with the potential to use this stimulation as an orthotic.
Preliminary research into using nGVS as an adjunct to vestibular rehabilitation suggests it is a feasible treatment. However, further research is required with treatment over a longer duration, using outcome measures that have been found to be sensitive to vestibular rehabilitation and nGVS before we can draw any reliable conclusions about its efficacy to augment rehabilitation.
Data availability statement
The original contributions presented in the study are included in the article/supplementary materials, further inquiries can be directed to the corresponding author.
Author contributions
RM and DT conceptualized the study. RM performed the literature search, extracted and critically reviewed the data, and wrote the first draft of the manuscript. RM and SR independently reviewed the articles with help from DT. UR performed the statistical analysis. PS, RT, and DT provided feedback at all stages of manuscript development. All authors reviewed and approved the final manuscript.
Funding
This study was supported by the Health Research Council of New Zealand, Grant Numbers: HRC22/363 and HRC19/632.
Conflict of interest
The authors declare that the research was conducted in the absence of any commercial or financial relationships that could be construed as a potential conflict of interest.
Publisher's note
All claims expressed in this article are solely those of the authors and do not necessarily represent those of their affiliated organizations, or those of the publisher, the editors and the reviewers. Any product that may be evaluated in this article, or claim that may be made by its manufacturer, is not guaranteed or endorsed by the publisher.
References
Asslander, L., Giboin, L. S., Gruber, M., Schniepp, R., and Wuehr, M. (2021). No evidence for stochastic resonance effects on standing balance when applying noisy galvanic vestibular stimulation in young healthy adults. Sci. Rep. 11, 12327. doi: 10.1038/s41598-021-91808-w
Asslander, L., and Peterka, R. J. (2016). Sensory reweighting dynamics following removal and addition of visual and proprioceptive cues. J. Neurophysiol. 116, 272–285. doi: 10.1152/jn.01145.2015
Bae, S. H., Nam, G. S., Kwak, S. H., and Kim, S. H. (2021). Importance of high-frequency vestibular function in the prognosis of bilateral vestibulopathy. Clin. Exp. Otorhinolaryngol. 14, 192–199. doi: 10.21053/ceo.2020.01739
Bayot, M., Dujardin, K., Tard, C., Defebvre, L., Bonnet, C. T., Allart, E., et al. (2018). The interaction between cognition and motor control: a theoretical framework for dual-task interference effects on posture, gait initiation, gait and turning. Neurophysiol. Clin. 48, 361–375. doi: 10.1016/j.neucli.2018.10.003
Borenstein, M., Hedges, L. V., Higgins, J. P., and Rothstein, H. R. (2009). Effect Sizes Based on Means. New York, NY: Wiley. doi: 10.1002/9780470743386
Brydges, C. R. (2019). Effect size guidelines, sample size calculations, and statistical power in gerontology. Innov Aging. 3, igz036. doi: 10.1093/geroni/igz036
Chen, P. Y., Jheng, Y. C., Wang, C. C., Huang, S. E., Yang, T. H., Hsu, P. C., et al. (2021). Effect of noisy galvanic vestibular stimulation on dynamic posture sway under visual deprivation in patients with bilateral vestibular hypofunction. Sci. Rep. 11, 4229. doi: 10.1038/s41598-021-83206-z
Colebatch, J. G., and Rothwell, J. C. (2004). Motor unit excitability changes mediating vestibulocollic reflexes in the sternocleidomastoid muscle. Clin. Neurophysiol. 115, 2567–2573. doi: 10.1016/j.clinph.2004.06.012
Cullen, K. E. (2019). Vestibular processing during natural self-motion: implications for perception and action. Nat. Rev. Neurosci. 20, 346–363. doi: 10.1038/s41583-019-0153-1
Dlugaiczyk, J., Gensberger, K. D., and Straka, H. (2019). Galvanic vestibular stimulation: from basic concepts to clinical applications. J. Neurophysiol. 121, 2237–2255. doi: 10.1152/jn.00035.2019
Dobbels, B., Lucieer, F., Mertens, G., Gilles, A., Moyaert, J., van de Heyning, P., et al. (2020). Prospective cohort study on the predictors of fall risk in 119 patients with bilateral vestibulopathy. PLoS ONE 15, e0228768. doi: 10.1371/journal.pone.0228768
Eder, J., Kellerer, S., Amberger, T., Keywan, A., Dlugaiczyk, J., Wuehr, M., et al. (2022). Combining vestibular rehabilitation with noisy galvanic vestibular stimulation for treatment of bilateral vestibulopathy. J. Neurol. doi: 10.1007/s00415-022-11033-x
Fujimoto, C., Egami, N., Kawahara, T., Uemura, Y., Yamamoto, Y., Yamasoba, T., et al. (2018). Noisy galvanic vestibular stimulation sustainably improves posture in bilateral vestibulopathy. Front. Neurol. 9, 900. doi: 10.3389/fneur.2018.00900
Fujimoto, C., Kinoshita, M., Kamogashira, T., Egami, N., Kawahara, T., Uemura, Y., et al. (2019a). Noisy galvanic vestibular stimulation has a greater ameliorating effect on posture in unstable subjects: a feasibility study. Sci. Rep. 9, 17189. doi: 10.1038/s41598-019-53834-7
Fujimoto, C., Yagi, M., and Murofushi, T. (2019b). Recent advances in idiopathic bilateral vestibulopathy: a literature review. Orphanet J. Rare Dis. 14, 202. doi: 10.1186/s13023-019-1180-8
Fujimoto, C., Yamamoto, Y., Kamogashira, T., Kinoshita, M., Egami, N., Uemura, Y., et al. (2016). Noisy galvanic vestibular stimulation induces a sustained improvement in body balance in elderly adults. Sci. Rep. 6, 37575. doi: 10.1038/srep37575
Galvan-Garza, R. C., Clark, T. K., Mulavara, A. P., and Oman, C. M. (2018). Exhibition of stochastic resonance in vestibular tilt motion perception. Brain Stimul. 11, 716–722. doi: 10.1016/j.brs.2018.03.017
Goel, R., Kofman, I., Jeevarajan, J., De Dios, Y., Cohen, H. S., Bloomberg, J. J., et al. (2015). Using low levels of stochastic vestibular stimulation to improve balance function. PLoS ONE 10, e0136335. doi: 10.1371/journal.pone.0136335
Guinand, N., Boselie, F., Guyot, J. P., and Kingma, H. (2012). Quality of life of patients with bilateral vestibulopathy. Ann. Otol. Rhinol. Laryngol. 12, 471–477. doi: 10.1177/000348941212100708
Hain, T. C., Cherchi, M., and Yacovino, D. A. (2018). Bilateral vestibular weakness. Front. Neurol. 9, 344. doi: 10.3389/fneur.2018.00344
Hall, C. D., Herdman, S. J., Whitney, S. L., Anson, E. R., Carender, W. J., Hoppes, C. W., et al. (2021). Vestibular rehabilitation for peripheral vestibular hypofunction: an updated clinical practice guideline from the academy of neurologic physical therapy of the American Physical Therapy Association. J. Neurol. Phys. Ther. 46:118. doi: 10.1097/NPT.0000000000000382
Hall, C. D., Herdman, S. J., Whitney, S. L., Cass, S. P., Clendaniel, R. A., Fife, T. D., et al. (2016). Vestibular rehabilitation for peripheral vestibular hypofunction: an evidence-based clinical practice guideline. J. Neurol. Phys. Ther. 40, 124–155. doi: 10.1097/NPT.0000000000000120
Helmchen, C., Rother, M., Spliethoff, P., and Sprenger, A. (2019). Increased brain responsivity to galvanic vestibular stimulation in bilateral vestibular failure. Neuroimage Clin. 24, 101942. doi: 10.1016/j.nicl.2019.101942
Herdman, S. J., Hall, C. D., Maloney, B., Knight, S., Ebert, M., and Lowe, J. (2015). Variables associated with outcome in patients with bilateral vestibular hypofunction: preliminary study. J. Vestib. Res. 25, 185–194. doi: 10.3233/VES-150556
Herssens, N., and McCrum, C. (2019). Stimulating balance: recent advances in vestibular stimulation for balance and gait. J. Neurophysiol. 122, 447–450. doi: 10.1152/jn.00851.2018
Higgins, J. P. T., Altman, D. G., Gotzsche, P. C., Juni, P., Moher, D., and Oxman, A. D. (2011). The Cochrane Collaboration's tool for assessing risk of bias in randomised trials BMJ 343. doi: 10.1136/bmj.d5928
Inukai, Y., Masaki, M., Otsuru, N., Saito, K., Miyaguchi, S., Kojima, S., et al. (2018a). Effect of noisy galvanic vestibular stimulation in community-dwelling elderly people: a randomised controlled trial. J. Neuroeng. Rehabil. 15, 63. doi: 10.1186/s12984-018-0407-6
Inukai, Y., Miyaguchi, S., Kobayashi, N., Otsuru, N., and Onishi, H. (2020a). Noisy galvanic vestibular stimulation effect on center of pressure sway during one-legged standing. J. Clin. Neurosci. 82(Pt A), 173–178. doi: 10.1016/j.jocn.2020.10.050
Inukai, Y., Miyaguchi, S., Saito, M., Otsuru, N., and Onishi, H. (2020b). Effects of different stimulation conditions on the stimulation effect of noisy galvanic vestibular stimulation. Front. Hum. Neurosci. 14, 581405. doi: 10.3389/fnhum.2020.581405
Inukai, Y., Otsuru, N., Masaki, M., Saito, K., Miyaguchi, S., Kojima, S., et al. (2018b). Effect of noisy galvanic vestibular stimulation on center of pressure sway of static standing posture. Brain Stimul. 11, 85–93. doi: 10.1016/j.brs.2017.10.007
Inukai, Y., Otsuru, N., Saito, K., Miyaguchi, S., Kojima, S., Yokota, H., et al. (2020c). The after-effect of noisy galvanic vestibular stimulation on postural control in young people: a randomized controlled trial. Neurosci. Lett. 729, 135009. doi: 10.1016/j.neulet.2020.135009
Iwasaki, S., Fujimoto, C., Egami, N., Kinoshita, M., Togo, F., Yamamoto, Y., et al. (2018). Noisy vestibular stimulation increases gait speed in normals and in bilateral vestibulopathy. Brain Stimul. 11, 709–715. doi: 10.1016/j.brs.2018.03.005
Iwasaki, S., Yamamoto, Y., Togo, F., Kinoshita, M., Yoshifuji, Y., Fujimoto, C., et al. (2014). Noisy vestibular stimulation improves body balance in bilateral vestibulopathy. Neurology 82, 969–975. doi: 10.1212/WNL.0000000000000215
Keywan, A., Badarna, H., Jahn, K., and Wuehr, M. (2020). No evidence for after-effects of noisy galvanic vestibular stimulation on motion perception. Sci Rep 10, 2545. doi: 10.1038/s41598-020-59374-9
Keywan, A., Jahn, K., and Wuehr, M. (2019). Noisy galvanic vestibular stimulation primarily affects otolith- mediated motion perception Neuroscience 399, 161–166. doi: 10.1016/j.neuroscience.2018.12.031
Kim, J. S., and Kim, H. J. (2022). Bilateral vestibulopathy: the causes, diagnosis, and treatments. Curr. Opin. Neurol. 35, 98–106. doi: 10.1097/WCO.0000000000001014
Ko, L. W., Chikara, R. K., Chen, P. Y., Jheng, Y. C., Wang, C. C., Yang, Y. C., et al. (2020). Noisy galvanic vestibular stimulation (stochastic resonance) changes electroencephalography activities and postural control in patients with bilateral vestibular hypofunction. Brain Sci. 10:740. doi: 10.3390/brainsci10100740
Lajoie, K., Marigold, D. S., Valdes, B. A., and Menon, C. (2021). The potential of noisy galvanic vestibular stimulation for optimizing and assisting human performance. Neuropsychologia 152, 107751. doi: 10.1016/j.neuropsychologia.2021.107751
Lotfi, Y., Farahani, A., Azimiyan, M., Moossavi, A., and Bakhshi, E. (2021). Comparison of efficacy of vestibular rehabilitation and noisy galvanic vestibular stimulation to improve dizziness and balance in patients with multiple sclerosis. J. Vestib. Res. 31, 541–551. doi: 10.3233/VES-201609
Lucieer, F., Duijn, S., Van Rompaey, V., Perez Fornos, A., Guinand, N., Guyot, J. P., et al. (2018). Full spectrum of reported symptoms of bilateral vestibulopathy needs further investigation—a systematic review Front Neurol 9, 352. doi: 10.3389/fneur.2018.00352
Lucieer, F. M. P., Van Hecke, R., van Stiphout, L., Duijn, S., Perez-Fornos, A., Guinand, N., et al. (2020). Bilateral vestibulopathy: beyond imbalance and oscillopsia. J. Neurol. 267(Suppl 1), 241–255. doi: 10.1007/s00415-020-10243-5
Matsugi, A., Oku, K., and Mori, N. (2020). The effects of stochastic galvanic vestibular stimulation on body sway and muscle activity. Front. Hum. Neurosci. 14, 591671. doi: 10.3389/fnhum.2020.591671
Medendorp, W. P., Alberts, B., Verhagen, W. I. M., Koppen, M., and Selen, L. P. J. (2018). Psychophysical evaluation of sensory reweighting in bilateral vestibulopathy. Front. Neurol. 9, 377. doi: 10.3389/fneur.2018.00377
Moss, F., Ward, L. M., and Sannita, W. G. (2004). Stochastic resonance and sensory information processing: a tutorial and review of application. Clin. Neurophysiol. 115, 267–281. doi: 10.1016/j.clinph.2003.09.014
Mulavara, A. P., Fiedler, M. J., Kofman, I. S., Wood, S. J., Serrador, J. M., Peters, B., et al. (2011). Improving balance function using vestibular stochastic resonance: optimizing stimulus characteristics. Exp. Brain Res. 210, 303–312. doi: 10.1007/s00221-011-2633-z
Mulavara, A. P., Kofman, I. S., De Dios, Y. E., Miller, C., Peters, B. T., Goel, R., et al. (2015). Using low levels of stochastic vestibular stimulation to improve locomotor stability. Front. Syst. Neurosci. 9, 117. doi: 10.3389/fnsys.2015.00117
Nooristani, M., Bigras, C., Lafontaine, L., Bacon, B. A., Maheu, M., and Champoux, F. (2021). Vestibular function modulates the impact of nGVS on postural control in older adults. J. Neurophysiol. 125, 489–495. doi: 10.1152/jn.00512.2020
Nooristani, M., Maheu, M., Bacon, B. A., and Champoux, F. (2019a). The importance of nGVS current density for postural control enhancement. Brain Stimul. 12, 1592–1594. doi: 10.1016/j.brs.2019.07.022
Nooristani, M., Maheu, M., Houde, M. S., Bacon, B. A., and Champoux, F. (2019b). Questioning the lasting effect of galvanic vestibular stimulation on postural control. PLoS ONE 14, e0224619. doi: 10.1371/journal.pone.0224619
Piccolo, C., Bakkum, A., and Marigold, D. S. (2020). Subthreshold stochastic vestibular stimulation affects balance-challenged standing and walking. PLoS ONE 15, e0231334. doi: 10.1371/journal.pone.0231334
Rashid, U., Barbado, D., Olsen, S., Alder, G., Elvira, J. L. L., Lord, S., et al. (2021). Validity and reliability of a smartphone app for gait and balance assessment. Sensors (Basel) 22, 124. doi: 10.3390/s22010124
Ruhe, A., Fejer, R., and Walker, B. (2011). Center of pressure excursion as a measure of balance performance in patients with non-specific low back pain compared to healthy controls: a systematic review of the literature. Eur. Spine J. 20, 358–368. doi: 10.1007/s00586-010-1543-2
Saftari, L. N., and Kwon, O. S. (2018). Ageing vision and falls: a review. J. Physiol. Anthropol. 37, 11. doi: 10.1186/s40101-018-0170-1
Schniepp, R., Mohwald, K., and Wuehr, M. (2019). Clinical and automated gait analysis in patients with vestibular, cerebellar, and functional gait disorders: perspectives and limitations. J. Neurol. 266(Suppl 1), 118–122. doi: 10.1007/s00415-019-09378-x
Schoberl, F., Pradhan, C., Grosch, M., Brendel, M., Jostes, F., Obermaier, K., et al. (2021). Bilateral vestibulopathy causes selective deficits in recombining novel routes in real space. Sci. Rep. 11, 2695. doi: 10.1038/s41598-021-82427-6
Schubert, M. C., and Minor, L. B. (2004). Vestibulo-ocular physiology underlying vestibular hypofunction. Phys. Ther. 84, 373–385. doi: 10.1093/ptj/84.4.393
Shumway-Cook, A., and Woollacott, M. H. (2007). “Normal postural control,” in Motor Control: Translating Research Into Clinical Practice. 3rd ed. (Philadelphia, PA: Lippincott Williams and Wilkins), 176–181.
Smith, P. F. (2017). Bionic balance organs: progress in the development of vestibular prostheses. New Zealand Med. J. 130, 56–65.
Sprenger, A., Spliethoff, P., Rother, M., Machner, B., and Helmchen, C. (2020). Effects of perceptible and imperceptible galvanic vestibular stimulation on the postural control of patients with bilateral vestibulopathy. J. Neurol. 267, 2383–2397. doi: 10.1007/s00415-020-09852-x
Stefani, S. P., Serrador, J. M., Breen, P. P., and Camp, A. J. (2020). Impact of galvanic vestibular stimulation-induced stochastic resonance on the output of the vestibular system: a systematic review. Brain Stimul. 13, 533–535. doi: 10.1016/j.brs.2020.01.006
Strupp, M., Kim, J. S., Murofushi, T., Straumann, D., Jen, J. C., Rosengren, S. M., et al. (2017). Bilateral vestibulopathy: diagnostic criteria consensus document of the Classification Committee of the Barany Society. J. Vestib. Res. 27, 177–189. doi: 10.3233/VES-170619
Viechtbauer, W. (2010). Conducting meta-analyses in {R} with the {metafor} package. J. Stat. Softw. 36, 1–48. doi: 10.18637/jss.v036.i03
Ward, B. K., Agrawal, Y., Hoffman, H. J., Carey, J. P., and Della Santina, C. C. (2013). Prevalence and impact of bilateral vestibular hypofunction: results from the 2008 US National Health Interview Survey. JAMA Otolaryngol. Head Neck Surg. 139, 803–810. doi: 10.1001/jamaoto.2013.3913
Wuehr, M., Boerner, J. C., Pradhan, C., Decker, J., Jahn, K., Brandt, T., et al. (2018). Stochastic resonance in the human vestibular system - Noise-induced facilitation of vestibulospinal reflexes. Brain Stimul. 11, 261–263. doi: 10.1016/j.brs.2017.10.016
Wuehr, M., Decker, J., Schenkel, F., Jahn, K., and Schniepp, R. (2022a). Impact on daily mobility and risk of falling in bilateral vestibulopathy. J. Neurol. 2022, 1–9. doi: 10.1007/s00415-022-11043-9
Wuehr, M., Nusser, E., Decker, J., Krafczyk, S., Straube, A., Brandt, T., et al. (2016a). Noisy vestibular stimulation improves dynamic walking stability in bilateral vestibulopathy. Neurology 86, 2196–2202. doi: 10.1212/WNL.0000000000002748
Wuehr, M., Nusser, E., Krafczyk, S., Straube, A., Brandt, T., Jahn, K., et al. (2016b). Noise-enhanced vestibular input improves dynamic walking stability in healthy subjects. Brain Stimul. 9, 109–116. doi: 10.1016/j.brs.2015.08.017
Keywords: vestibular rehabilitation, balance, neuromodulation, noisy galvanic vestibular stimulation, nGVS, physical therapy
Citation: McLaren R, Smith PF, Taylor RL, Ravindran S, Rashid U and Taylor D (2022) Efficacy of nGVS to improve postural stability in people with bilateral vestibulopathy: A systematic review and meta-analysis. Front. Neurosci. 16:1010239. doi: 10.3389/fnins.2022.1010239
Received: 02 August 2022; Accepted: 24 August 2022;
Published: 28 September 2022.
Edited by:
James Phillips, University of Washington, United StatesReviewed by:
Max Wuehr, Ludwig Maximilian University of Munich, GermanyShinichi Iwasaki, Nagoya City University, Japan
Copyright © 2022 McLaren, Smith, Taylor, Ravindran, Rashid and Taylor. This is an open-access article distributed under the terms of the Creative Commons Attribution License (CC BY). The use, distribution or reproduction in other forums is permitted, provided the original author(s) and the copyright owner(s) are credited and that the original publication in this journal is cited, in accordance with accepted academic practice. No use, distribution or reproduction is permitted which does not comply with these terms.
*Correspondence: Ruth McLaren, ruth.mclaren@aut.ac.nz