Corrigendum: Transcriptomic Signatures Associated With Gray Matter Volume Changes in Patients With Functional Constipation
- 1Department of Radiology, Shanghai Tenth People’s Hospital, School of Clinical Medicine of Nanjing Medical University, Shanghai, China
- 2Department of Radiology, The First Affiliated Hospital of Dalian Medical University, Dalian, China
- 3Department of Colorectal and Anal Surgery, Shanghai Tenth People’s Hospital, School of Clinical Medicine of Nanjing Medical University, Shanghai, China
Functional constipation, which belongs to the functional gastrointestinal disorder (FGID), is a common disease and significantly impacts daily life. FGID patients have been progressively proven with functional and structural alterations in various brain regions, but whether and how functional constipation affects the brain gray matter volume (GMV) remains unclear; besides, which genes are associated with the GMV changes in functional constipation is largely unknown. On account of the structural MRI image from the 30 functional constipation patients and 30 healthy controls (HCs), GMV analysis showed that functional constipation patients had significantly decreased GMV in the right orbital prefrontal cortex (OFC), left precentral gyrus (PreG), and bilateral thalamus (THA). Correlation analysis showed that the self-rating depressive scale, patient assessment of constipation quality of life (PAC-QOL), and Wexner constipation scores were negatively correlated with GMV of the OFC and negative correlations between PAC-QOL score and GMV of the bilateral THA. Based on the Allen Human Brain Atlas, a cross-sample spatial correlation was conducted and found that 18 genes’ expression values showed robust correlations with GMV changes in functional constipation patients. These outcomes highlight our recognition of the transcriptional features related to GMV changes in functional constipation and could be regarded as candidates to detect biological mechanisms of abnormality in functional constipation patients.
Introduction
Functional constipation, as one type of the functional gastrointestinal disorder (FGID), is portrayed by rare bowel movements, painful defecation, uncomfortable feeling of incomplete evacuation, hard/big stools, and frequently joined with abdominal distension and/or abdominal pain (Alame and Bahna, 2012). The incidence rate of functional constipation is relatively high in the entire community, about 0.7–79% (Mugie et al., 2011). Besides, an impressive proportion of functional constipation patients would accompany anxiety and varying severity of depression (Hosseinzadeh et al., 2011). These symptoms severely sway the quality of their daily life and emotional status (Glia and Lindberg, 1997; Bongers et al., 2009).
At present, robust evidence demonstrated that FGID results from physiological changes of the gastrointestinal system because of the bidirectional brain–gut axis, which may influence brain functional/structural alterations, and subsequently arising from anxiety and depression symptoms (Mayer et al., 2006; Mazur et al., 2012). Accordingly, neuroimaging methods have been progressively applied to investigate brain structural and functional anomalies of FGID patients (Blankstein et al., 2010; Zhu et al., 2016; Jin et al., 2019; Hu et al., 2020; Duan et al., 2021; Liu et al., 2021). They found the functional abnormalities in brain regions enrolled in emotion modulating, including the orbitofrontal cortex (OFC), anterior insula, dorsal anterior cingulate cortex, and hippocampus, and motor control, including the precentral gyrus and supplementary motor area. Besides, several pieces of research in FGID patients demonstrated functional impairments in the thalamus, assuming a fundamental part in sensory and motor signal processing (Tillisch et al., 2011; Liu et al., 2021). Moreover, a new structural MRI research had shown that functional constipation patients demonstrated significant diminished cortical thickness of different brain regions, including the left orbitofrontal cortex, left middle frontal gyrus, left medial prefrontal gyrus, left supplementary motor area, right dorsal anterior cingulate cortex, right middle temporal gyrus, and bilateral posterior cingulate cortex/precuneus; decreased cortical volume in the left middle temporal gyrus and bilateral posterior cingulate cortex/precuneus; and induced cortical surface area in the right precentral gyrus additionally (Hu et al., 2020). The above-mentioned brain regions are primarily associated with somatic movement controlling and emotion modulating (Zhu et al., 2016; Hu et al., 2020), and these alterations were implicated in the symptoms of functional constipation patients containing disorder of defecation and unhealthy mood (Ringel, 2002; Alame and Bahna, 2012; Al Omran and Aziz, 2014). Notwithstanding, there has been no study of transcriptional neuroimaging analysis to identify genes related to GMV alterations in functional constipation patients.
Currently, based on the Allen Human Brain Atlas (AHBA)1, we acquired the gene expression data and conducted the transcriptional neuroimaging analysis to find the genes related to GMV changes in functional constipation patients. We extracted gene expression data from each sample and calculated GMV changes depending on the high-resolution structural MRI image of functional constipation and controls, in sequence, cross-sample spatial association analysis between GMV changes and gene expression values. An illustration of the handling flow chart is displayed in Figure 1.
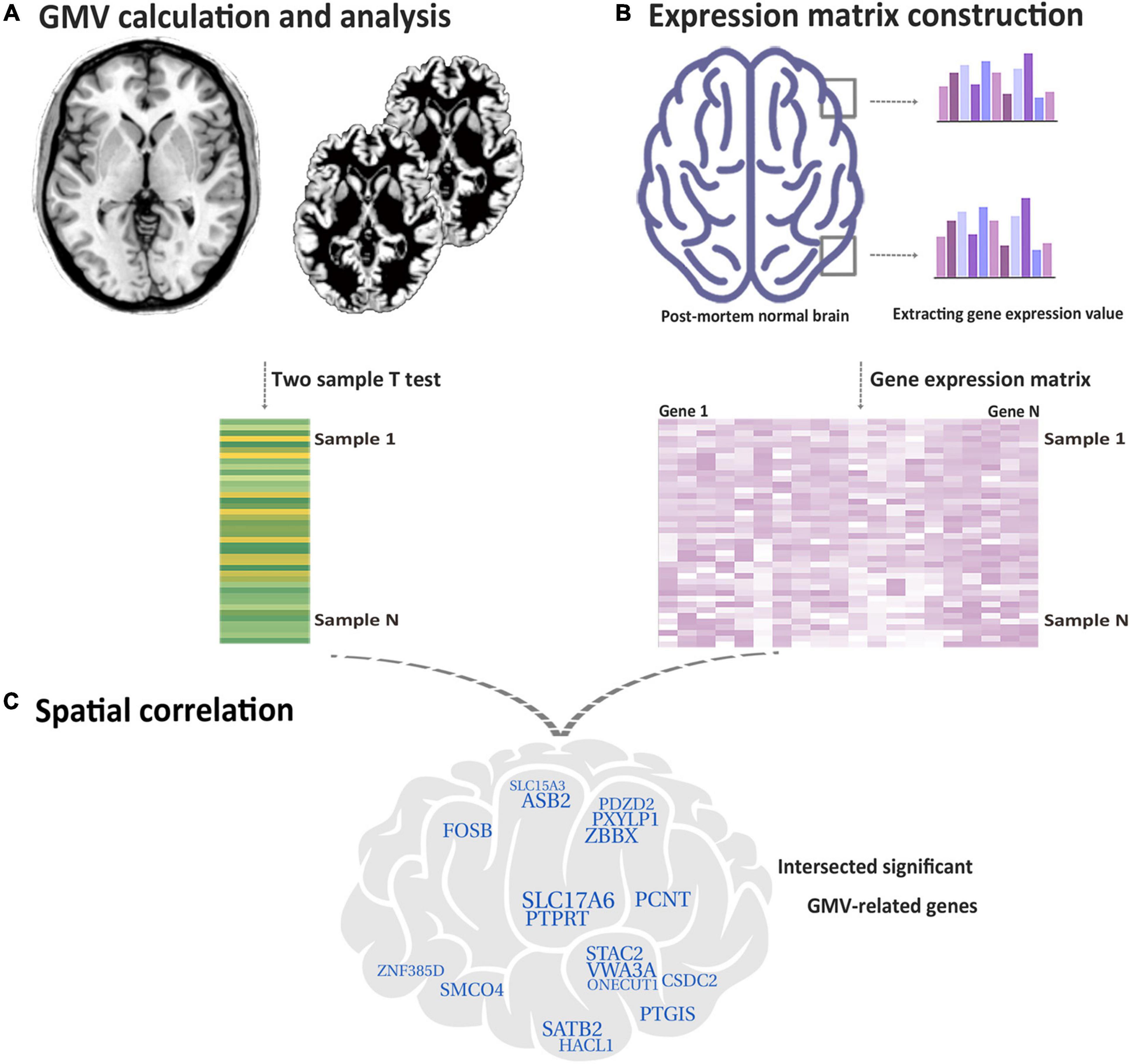
Figure 1. Flow chart of the study design. (A) Based on functional constipation and HC, utilizing the two-sample t-test to make voxel-wise GMV changes for each tissue sample. (B) Acquired gene expression values in each tissue sample. (C) Identifying genes related with GMV changes in functional constipation. The crossed specific genes are characterized as genes related with GMV changes in functional constipation.
Materials and Methods
Subjects
The experiment was approved by the Ethical Committee of Shanghai Tenth People’s Hospital, and each subject gave written informed consent before the study. Utilizing Rome IV criteria (Drossman, 2016), patients diagnosed by a gastroenterologist expert as having functional constipation with history over 1 year were enrolled in our study. Subjects were barred from the study if they had the following diseases, namely, congenital giant colon, excess sigmoid colon, and mental disease, or who were drug abusers. Besides, subjects with other brain disorders or abnormalities (such as severe white matter hyperintensity, lacunes, microbleeds, and tumors), as determined using T2 fluid-attenuated inversion recovery (FLAIR) sequence, were excluded. Finally, there were 30 right-handed patients with functional constipation (10 men, right-handed, 46.00 ± 18.03 years) who finished the MRI scans. The healthy control group comprised 30 subjects who were right-handed and age and gender matched (9 men, right-handed, 45.77 ± 14.63 years). Self-directed surveys, including the patient assessment of constipation quality of life (PAC-QOL) and Wexner constipation score, were displayed to all members to comprehensively assess the burden of constipation on patients’ regular working and life (Marquis et al., 2005). Patients were additionally approached to finish the Zung (1965) self-rating depressive scale (SDS) and Zung (1971) self-rating anxiety scale (SAS) to survey their seriousness of depression and anxiety.
Data Acquisition and Gray Matter Volume Calculation
Sagittal 3D high-resolution T1-weighted data were collected by a turbo field echo (TFE) sequence. The parameters are as followed: repetition time (TR)/echo time (TE) = 7.0 ms/3.2 ms; field of view = 256 mm × 256 mm; matrix = 256 × 256. The thickness slice is 1.0 mm, and there were 192 slices with no gap (Ingenia 3.0, Philips).
All the structural MRI data were preprocessed utilizing CAT12 software (version r1364) with the accompanying methodology: bias correction, segmentation, the creation of population-specific tissue templates, spatial normalization using the DARTEL technique, and smoothing with an 8 mm × 8 mm × 8 mm full-width. After these preprocessing steps, we acquired the normalized, modulated, and smoothed GMV images, and each voxel represented volume information.
Case–Control Gray Matter Volume Changes
We conducted the voxel-based comparisons to distinguish the brain regions that showed group differences in GMV by utilizing the two-sample t-test, controlling the impacts of gender, age, and whole intracranial volume. The multiple comparisons were adjusted by the false discovery rate method (p < 0.05) and a cluster size >200 voxels using the SPM12 software. Then, a region of interest (ROI)-based association analysis was applied to test relationships between the GMVs of the brain areas, which showed significant group differences with the SDS, SAS, PAC-QOL, and Wexner constipation scores.
Gene Expression Data Preprocessing
Freely accessible gene expression data of six postmortem human brains were obtained from the AHBA dataset (Hawrylycz et al., 2012), and they were handled utilizing a new flow chart to combine gene expression data with neuroimage data (Arnatkeviciute et al., 2019). We divided the AHBA dataset into two datasets: the first dataset consisted of two donors with the whole brain gene expression data, which have 820 samples, and the second dataset consisted of six donors only with the left-brain gene expression data, which have 1,782 samples. The short processing work was as follows: first, we reassigned probes to genes by using the most recent sequencing databases; second, we barred probes with lower expression signal intensity compared to background noise; third, the probes showing high consistency with the RNA-sequence data were picked; lastly, the expression data were normalized. After such handling process, we obtained 10,185 genes with a normalized expression value of each sample.
Genes Associated With Gray Matter Volume Changes in Functional Constipation Patients
After extracting gene expression data from each sample (820/1,782 samples) and calculating GMV changes (t-statistic values) in these samples derived from the two-sample t-test based on the high-resolution structural MRI image of functional constipation patients and HC, we performed a gene-wise cross-sample Spearman correlation analysis to decide the relationships between GMV changes and gene expression values independently (n = 10,185). Considering the multiple comparisons at the gene level (n = 10,185), we adjusted the Bonferroni correction method and set a p < 4.91 × 10–7 = 0.05/10,185 to identify the significant genes. At last, the genes related to GMV changes in functional constipation patients were characterized as those whose expression values derived from two expression datasets were prominently associated with GMV changes.
Results
Demographic and Clinical Characteristics
The general clinical characteristics of functional constipation patients and HC are summed up in Table 1. The two groups have not shown significant differences in gender (χ2 = 0.077, df = 1, p = 0.781), age (F = 2.451, df = 58, p = 0.123), body mass index (BMI) (F = 0.287, df = 58, p = 0.594), and anxiety (F = 1.582, df = 58, p = 0.213) between the two groups. There was significant group difference on depression (F = 7.474, df = 58, p = 0.008).
Gray Matter Volume Changes of Functional Constipation Patients
After analyzing voxel-wise GMV alterations (P < 0.05, FDR corrected) between the patients with functional constipation and HC, compared to HC groups, we found that functional constipation patients primarily demonstrated decreased GMV in the right orbital prefrontal cortex (OFC, peak MNI coordinates: x = 15, y = 37.5, z = −22.5; cluster size = 230 voxels), left precentral gyrus (PreG, peak MNI coordinates: x = −42, y = 24, z = 52.5; cluster size = 230 voxels), and bilateral thalamus (THA, peak MNI coordinates: x = 4.5, y = −19.5, z = −1.5; cluster size = 338 voxels) (Figure 2). In addition, there were no regions demonstrating a significant increased GMV in functional constipation patients.
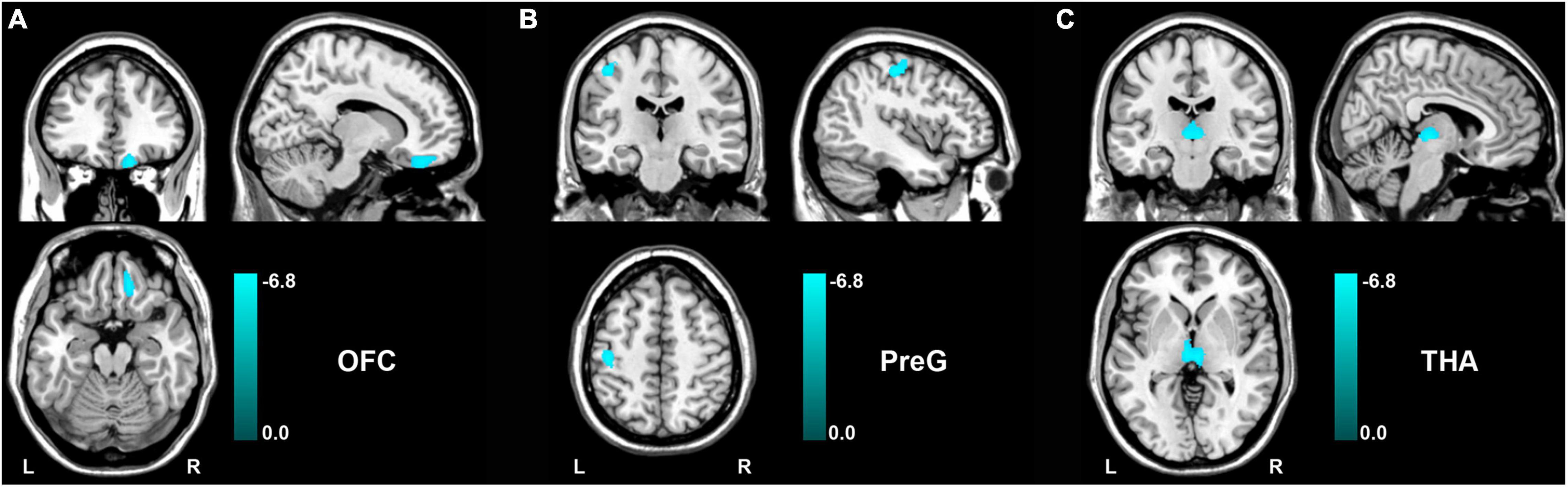
Figure 2. GMV changes between functional constipation and healthy controls (p < 0.05, FDR correction). The color bar showed the range of t-value. There was significantly decreased GMV in the right OFC (A), left PreG (B), and bilateral THA (C). GMV, gray matter volume; L, left; OFC, orbital prefrontal cortex; PreG, precentral gyrus; R, right; THA, thalamus.
Correlation analysis showed that the PAC-QOL, Wexner constipation score, and SDS score were negatively correlated with GMV of the OFC (r = −0.638, p < 0.001; r = −0.466, p = 0.009; and r = −0.412, p = 0.024, respectively) in functional constipation patients (Figure 3). There was negative correlation between PAC-QOL score and GMV of the bilateral THA (r = −0.415, p = 0.023) in functional constipation patients (Figure 3). However, the data showed expected heterogeneity due to the small sample size; we should restrict the wide application of such conclusions, as there exist correlated tendencies, which need further verification in the future.
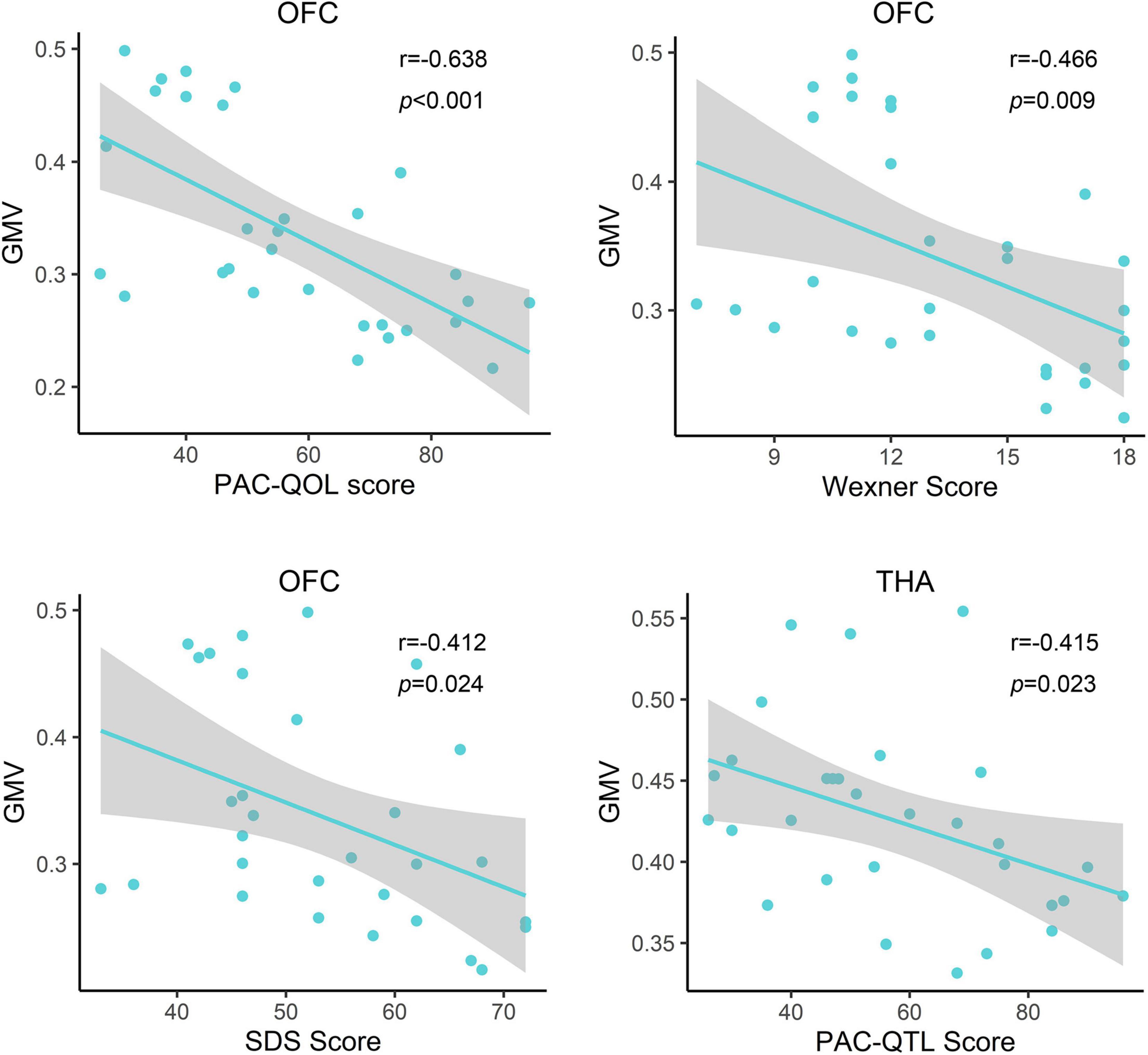
Figure 3. Relationship between clinical information and gray matter volume of the impaired brain region in functional constipation patients. In the functional constipation patients, the PAC-QOL, Wexner constipation score, and SDS score were negatively correlated with GMV of the right OFC. There was negative correlation between PAC-QOL score and GMV of the bilateral THA. GMV, gray matter volume; L, left; OFC, orbital prefrontal cortex; PAC-QOL, patient assessment of constipation quality of life; PreG, precentral gyrus; R, right; SDS, self-rating depressive scale; THA, thalamus.
Genes Related to Gray Matter Volume Changes in Functional Constipation Patients
After the gene expression data processing, we ultimately achieved 10,185 genes with normalized expression values for every 820 and 1,782 samples from the two AHBA datasets. A cross-sample spatial correlation was conducted between GMV changes in functional constipation patients and gene expression value. There were 345 genes that revealed a significant correlation with GMV changes in functional constipation in the first dataset and 208 genes in the second (p < 0.05, Bonferroni corrected). The crossed 18 genes of the two AHBA expression datasets were chosen. Detailed descriptions and correlation coefficients of these genes are exhibited in Table 2. Hence, the positive correlation that implies higher gene expression in brain samples manifested a more prominent GMV decrease in functional constipation patients. The negative correlation that implies lower gene expression in brain samples manifested a more noteworthy GMV decrease in functional constipation patients (Figure 4).
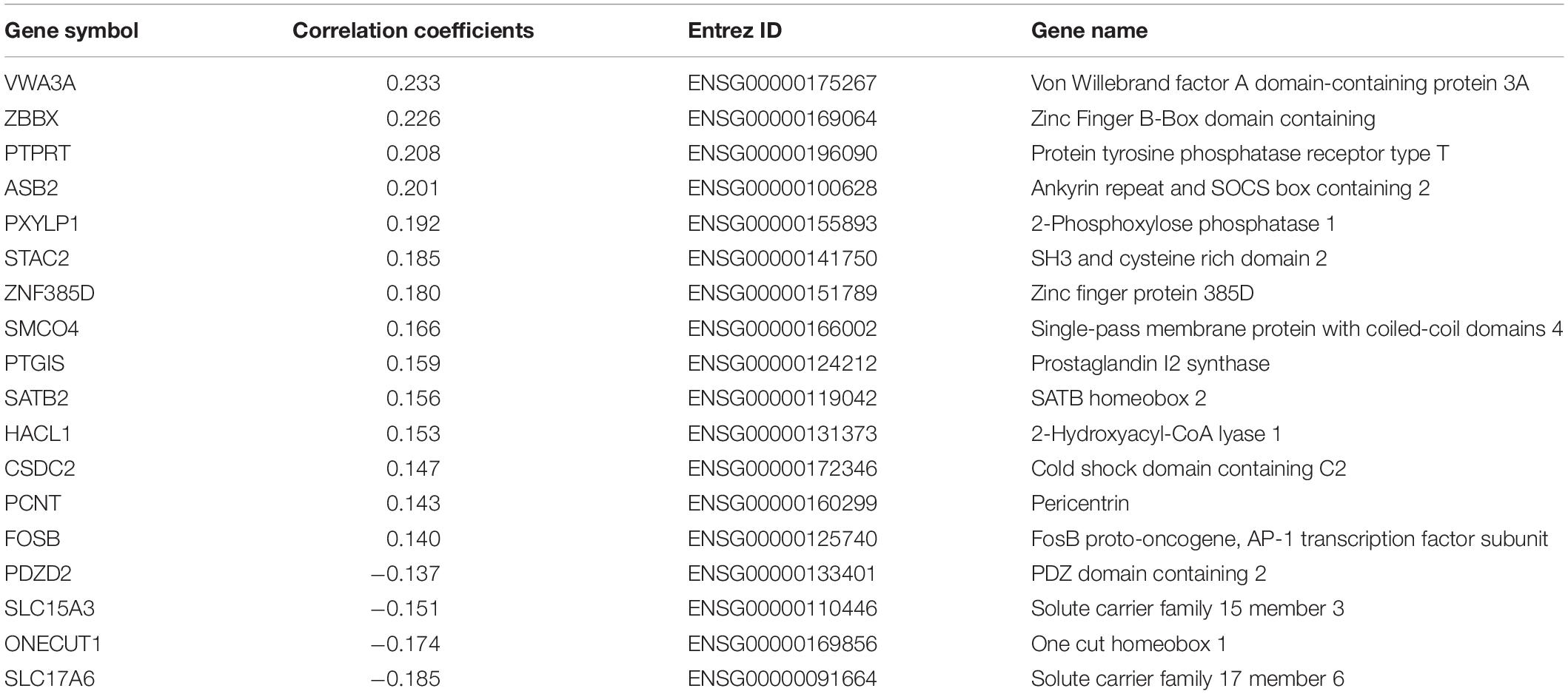
Table 2. The candidate 18 genes manifesting prominent relationships between gene expression and GMV alterations in functional constipation.
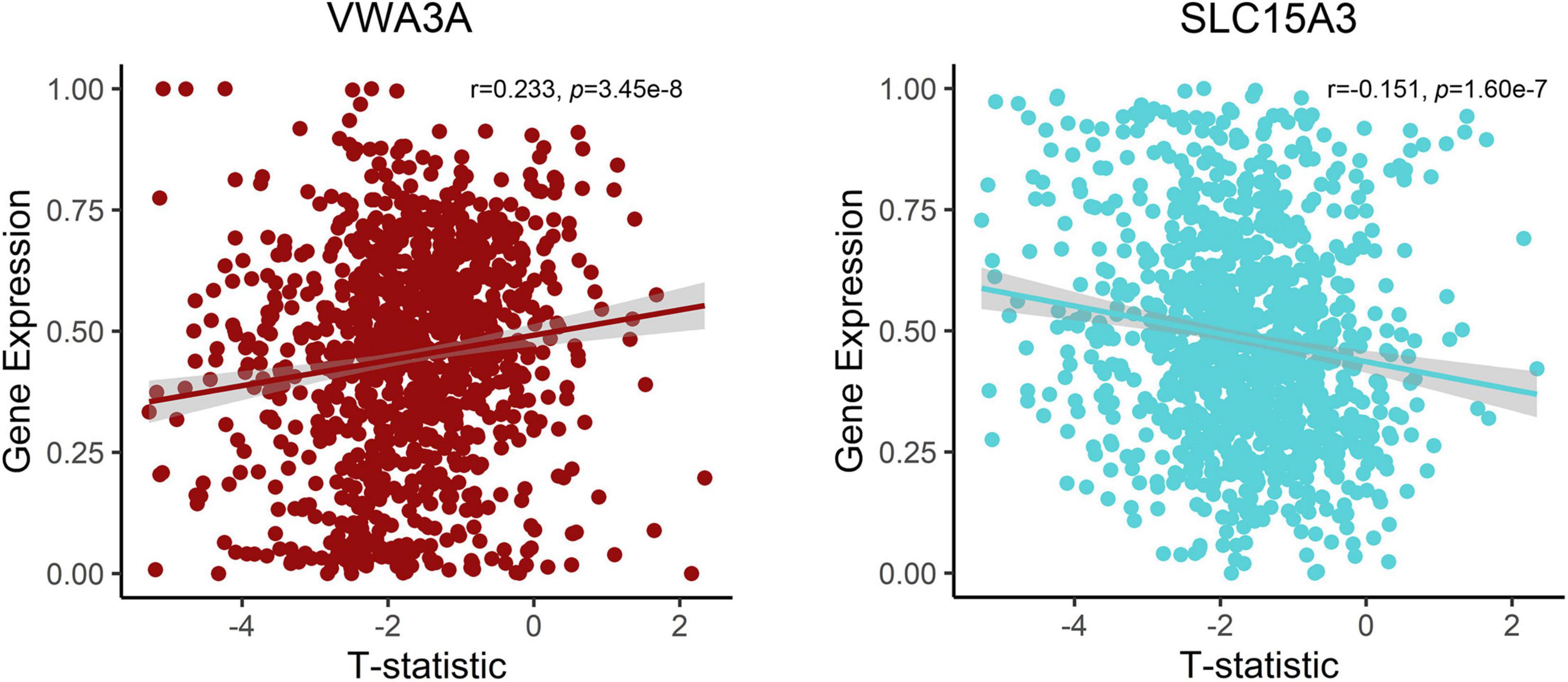
Figure 4. Correlations between expression of two representative genes and GMV changes in patients with functional constipation. The x-axis shows the T-statistic of GMV difference between functional constipation patients and healthy controls, and the y-axis is the gene expression value.
Discussion
In this study, we mainly identified genes preferentially correlated to GMV changes in functional constipation patients by linking gene expression patterns to GMV difference patterns in humans. We found 18 genes’ expression values showing robust correlations with GMV changes in functional constipation, including the orbitofrontal cortex, precentral gyrus, and thalamus. These outcomes could highlight our recognition of the transcriptional features correlated with GMV changes in functional constipation patients.
Here, we found the decreased GMV in the right OFC and its association with constipation scores and depression. As one of the least understood regions, OFC assumes a vital part in emotional regulation, visceral information integration (including sensory and motor information), and decision-making (Kringelbach and Rolls, 2004; Price, 2007; Rolls and Grabenhorst, 2008; Bongers et al., 2009; Rolls, 2019). Additionally, the OFC shows anatomical connection with the cingulate gyrus, amygdala, hypothalamus, and midbrain (Price, 2007), which subsequently portrays its contribution to emotional modulation and visceral coordination. Previous functional MRI (fMRI) studies have reported that the distension of the lower gastrointestinal tract will activate OFC (Derbyshire, 2003), followed by another study that showed that the painful and non-painful gastric stimulation will also activate the right OFC (Guleria et al., 2017). Thereby, these studies further support the vital role of OFC in regulating visceral function. Meanwhile, increased baseline activity in OFC was found in patients with functional constipation and showed a correlation with the sensation of incomplete evacuation (Zhu et al., 2016). Functional constipation is often accompanied by mental issues; the most common are anxiety and depression (Emmanuel et al., 2001; Waters et al., 2013). In this study, we found that the decreased GMV in the right OFC showed association with constipation scores and depression, indicating that the structural injury in OFC might cause the functional abnormality in visceral sensory and motor integration and emotional processing.
In addition, we noticed reduced GMV in the left precentral gyrus and the bilateral thalamus, and the GMV of the bilateral thalamus in patients with functional constipation showed association with PAC-QOL score. The precentral gyrus contributes to controlling the movement execution (Zhu et al., 2016). The structural abnormality of PreG in patients with functional constipation indicated the altered ability to control bowel movement (Hu et al., 2020). Thalamus, as an integrative hub, prominently participates in relaying/integrating/transmitting numerous inputs and connections with various cortical brain areas (Rouiller et al., 1999; Sherman, 2017). Some studies focusing on irritable bowel syndrome revealed the vital role of the thalamus in controlling sensory information (Keszthelyi et al., 2012; Labus et al., 2014) and displayed the activation when distending the rectum (Mayer et al., 2009). Neuroimaging studies depicted numerous functional abnormalities in the thalamus of the functional constipation patients, including the lower amplitude of low-frequency fluctuation in the female functional constipation patients and the decreased nodal degree based on the resting-state fMRI (Jin et al., 2019; Liu et al., 2021). Recently, a study that employed diffusion tensor imaging showed a decreased fractional anisotropy in the fibers communicating with the precentral gyrus, postcentral gyrus, amygdala, and hippocampus in patients with functional constipation, which may imply that the function of integrating the visceral sensory or motor inputs and connecting with other brain regions was impaired in functional constipation patients (Al Omran and Aziz, 2014; Liu et al., 2021).
Currently, we identified that the expression of genes (VWA3A, ZBBX, PTPRT, ASB2, PXYLP1, STAC2, ZNF385D, SMCO4, PTGIS, SATB2, HACL1, CSDC2, PCNT, and FOSB) showed positive correlations with GMV difference, and the expression of genes (ONECUT1, PDZD2, SLC15A3, and SLC17A6) showed negative correlations. For example, there was a positive correlation for SATB2, encoding the special AT-rich sequence-binding protein 2, which is a known member of the AT-rich matrix attachment region-binding transcription factor family with a role in the central nervous system and craniofacial development (FitzPatrick et al., 2003; Britanova et al., 2005), also highly expressed and specific for colorectal origins (Magnusson et al., 2011). The more prominent expression of SATB2 in brain samples with significant GMV decrease in functional constipation may be due to its aberrant expression influence on the brain–gut axis. In contrast, SLC17A6 acted a negative correlation, i.e., the lower gene expression in brain samples manifested a more notable GMV decrease in functional constipation. SLC17A6 is a protein-coding gene, also known as VGluT2, highly expressed in glutamatergic neurons. As a primary afferent neurotransmitter, glutamate transfers information from the mucosa to the enteric plexuses and brain. Changes in SLC17A6 expression could indicate glutamatergic dysfunction in bowel disease (Tong et al., 2001). Even though the genes related to GMV changes in functional constipation is a backhanded technique, we accept that the strategy can suggest valuable discovery on account of such firsthand datasets lacking (Richiardi et al., 2015).
Limitations
Some limitations should be considered when interpreting our findings. First, we have not collected a larger sample of patients with functional constipation and healthy controls, which restricted the wide application and weakened the statistical robustness. Second, all the functional constipation patients that we enrolled had a history over 1 year, and their medications are different. Some patients only took healthcare products, while some took laxatives occasionally. In this study, we did not take into account the effect of medicines on the results. Third, genes with undetectable expression variation across individuals were omitted in such analysis, since the gene expression data and neuroimage data were acquired from different individuals. Finally, our study is experimental, and further, we should explore whether the identified genes have a causal influence on the altered GMV in functional constipation patients.
Conclusion
In brief, we performed transcriptional neuroimaging association to define the genes that appear to have correlation with GMV changes in functional constipation. The identified 18 genes, accordantly manifesting prominent relationships between GMV alterations in functional constipation and gene expression value, could be regarded as candidates to detect biological mechanisms of abnormality in functional constipation patients.
Data Availability Statement
The original contributions presented in the study are included in the article/supplementary material, further inquiries can be directed to the corresponding author/s.
Ethics Statement
The studies involving human participants were reviewed and approved by the Ethical Committee of Shanghai Tenth People’s Hospital. The patients/participants provided their written informed consent to participate in this study.
Author Contributions
WC and YZ designed the research, analyzed the data, and wrote the manuscript. WC, LW, and RZ performed the research. WC, LW, RZ, and BY were involved in the clinical assessment. WC, YZ, TH, and JG processed part of the data. GT provided guidance and advice. All authors contributed to the article and approved the submitted version.
Funding
This study was funded by the National Natural Science Foundation of China (No. 81871325), Project of STCSM (20Y11911800 and 19411965300), and SCDSTC (CKY2021-41).
Conflict of Interest
The authors declare that the research was conducted in the absence of any commercial or financial relationships that could be construed as a potential conflict of interest.
Publisher’s Note
All claims expressed in this article are solely those of the authors and do not necessarily represent those of their affiliated organizations, or those of the publisher, the editors and the reviewers. Any product that may be evaluated in this article, or claim that may be made by its manufacturer, is not guaranteed or endorsed by the publisher.
Footnotes
References
Al Omran, Y., and Aziz, Q. (2014). Functional brain imaging in gastroenterology: to new beginnings. Nat. Rev. Gastroenterol. Hepatol. 11, 565–576. doi: 10.1038/nrgastro.2014.89
Arnatkeviciute, A., Fulcher, B. D., and Fornito, A. (2019). A practical guide to linking brain-wide gene expression and neuroimaging data. Neuroimage 189, 353–367. doi: 10.1016/j.neuroimage.2019.01.011
Blankstein, U., Chen, J., Diamant, N. E., and Davis, K. D. (2010). Altered brain structure in irritable bowel syndrome: potential contributions of pre-existing and disease-driven factors. Gastroenterology 138, 1783–1789. doi: 10.1053/j.gastro.2009.12.043
Bongers, M. E., van Dijk, M., Benninga, M. A., and Grootenhuis, M. A. (2009). Health related quality of life in children with constipation-associated fecal incontinence. J. Pediatr. 154, 749–753. doi: 10.1016/j.jpeds.2008.11.029
Britanova, O., Akopov, S., Lukyanov, S., Gruss, P., and Tarabykin, V. (2005). Novel transcription factor Satb2 interacts with matrix attachment region DNA elements in a tissue-specific manner and demonstrates cell-type-dependent expression in the developing mouse CNS. Eur. J. Neurosci. 21, 658–668. doi: 10.1111/j.1460-9568.2005.03897.x
Derbyshire, S. W. (2003). A systematic review of neuroimaging data during visceral stimulation. Am. J. Gastroenterol. 98, 12–20. doi: 10.1111/j.1572-0241.2003.07168.x
Drossman, D. A. (2016). Functional gastrointestinal disorders: history, pathophysiology, clinical features and Rome IV. Gastroenterology 150, 1262–1279E2. doi: 10.1053/j.gastro.2016.02.032
Duan, S., Liu, L., Li, G., Wang, J., Hu, Y., Zhang, W., et al. (2021). Altered functional connectivity within and between salience and sensorimotor networks in patients with functional constipation. Front. Neurosci. 15:628880. doi: 10.3389/fnins.2021.628880
Emmanuel, A. V., Mason, H. J., and Kamm, M. A. (2001). Relationship between psychological state and level of activity of extrinsic gut innervation in patients with a functional gut disorder. Gut 49, 209–213. doi: 10.1136/gut.49.2.209
FitzPatrick, D. R., Carr, I. M., McLaren, L., Leek, J. P., Wightman, P., Williamson, K., et al. (2003). Identification of SATB2 as the cleft palate gene on 2q32-q33. Hum. Mol. Genet. 12, 2491–2501. doi: 10.1093/hmg/ddg248
Glia, A., and Lindberg, G. (1997). Quality of life in patients with different types of functional constipation. Scand. J. Gastroenterol. 32, 1083–1089. doi: 10.3109/00365529709002985
Guleria, A., Karyampudi, A., Singh, R., Khetrapal, C. L., Verma, A., Ghoshal, U. C., et al. (2017). Mapping of brain activations to rectal balloon distension stimuli in male patients with irritable bowel syndrome using functional magnetic resonance imaging. J. Neurogastroenterol. Motil. 23, 415–427. doi: 10.5056/jnm16148
Hawrylycz, M. J., Lein, E. S., Guillozet-Bongaarts, A. L., Shen, E. H., Ng, L., Miller, J. A., et al. (2012). An anatomically comprehensive atlas of the adult human brain transcriptome. Nature 489, 391–399. doi: 10.1038/nature11405
Hosseinzadeh, S. T., Poorsaadati, S., Radkani, B., and Forootan, M. (2011). Psychological disorders in patients with chronic constipation. Gastroenterol. Hepatol. Bed Bench 4, 159–163.
Hu, C., Liu, L., Liu, L., Zhang, J., Hu, Y., Zhang, W., et al. (2020). Cortical morphometry alterations in brain regions involved in emotional, motor-control and self-referential processing in patients with functional constipation. Brain Imaging Behav. 14, 1899–1907. doi: 10.1007/s11682-019-00133-4
Jin, Q., Duan, S., Li, G., Sun, L., Hu, Y., Hu, C., et al. (2019). Sex-related differences in resting-state brain activity and connectivity in the orbital frontal cortex and insula in patients with functional constipation. Neurogastroenterol. Motil. 31:e13566. doi: 10.1111/nmo.13566
Keszthelyi, D., Troost, F. J., and Masclee, A. A. (2012). Irritable bowel syndrome: methods, mechanisms, and pathophysiology. Methods to assess visceral hypersensitivity in irritable bowel syndrome. Am. J. Physiol. Gastrointest. Liver Physiol. 303, G141–G154. doi: 10.1152/ajpgi.00060.2012
Kringelbach, M. L., and Rolls, E. T. (2004). The functional neuroanatomy of the human orbitofrontal cortex: evidence from neuroimaging and neuropsychology. Prog. Neurobiol. 72, 341–372. doi: 10.1016/j.pneurobio.2004.03.006
Labus, J. S., Dinov, I. D., Jiang, Z., Ashe-McNalley, C., Zamanyan, A., Shi, Y., et al. (2014). Irritable bowel syndrome in female patients is associated with alterations in structural brain networks. Pain 155, 137–149. doi: 10.1016/j.pain.2013.09.020
Liu, L., Hu, C., Hu, Y., Zhang, W., Zhang, Z., Ding, Y., et al. (2021). Abnormalities in the thalamo-cortical network in patients with functional constipation. Brain Imaging Behav. 15, 630–642. doi: 10.1007/s11682-020-00273-y
Magnusson, K., de Wit, M., Brennan, D. J., Johnson, L. B., McGee, S. F., Lundberg, E., et al. (2011). SATB2 in combination with cytokeratin 20 identifies over 95% of all colorectal carcinomas. Am. J. Surg. Pathol. 35, 937–948. doi: 10.1097/PAS.0b013e31821c3dae
Marquis, P., De La Loge, C., Dubois, D., McDermott, A., and Chassany, O. (2005). Development and validation of the patient assessment of constipation quality of life questionnaire. Scand. J. Gastroenterol. 40, 540–551. doi: 10.1080/00365520510012208
Mayer, E. A., Aziz, Q., Coen, S., Kern, M., Labus, J. S., Lane, R., et al. (2009). Brain imaging approaches to the study of functional GI disorders: a Rome working team report. Neurogastroenterol. Motil. 21, 579–596. doi: 10.1111/j.1365-2982.2009.01304.x
Mayer, E. A., Naliboff, B. D., and Craig, A. D. (2006). Neuroimaging of the brain-gut axis: from basic understanding to treatment of functional GI disorders. Gastroenterology 131, 1925–1942. doi: 10.1053/j.gastro.2006.10.026
Mazur, M., Furgala, A., Jablonski, K., Mach, T., and Thor, P. (2012). Autonomic nervous system activity in constipation-predominant irritable bowel syndrome patients. Med. Sci. Monit. 18, CR493–CR499. doi: 10.12659/msm.883269
Mugie, S. M., Benninga, M. A., and Di Lorenzo, C. (2011). Epidemiology of constipation in children and adults: a systematic review. Best Pract. Res. Clin. Gastroenterol. 25, 3–18. doi: 10.1016/j.bpg.2010.12.010
Price, J. L. (2007). Definition of the orbital cortex in relation to specific connections with limbic and visceral structures and other cortical regions. Ann. N. Y. Acad. Sci. 1121, 54–71. doi: 10.1196/annals.1401.008
Richiardi, J., Altmann, A., Milazzo, A. C., Chang, C., Chakravarty, M. M., Banaschewski, T., et al. (2015). BRAIN NETWORKS. Correlated gene expression supports synchronous activity in brain networks. Science 348, 1241–1244.
Ringel, Y. (2002). Brain research in functional gastrointestinal disorders. J. Clin. Gastroenterol. 35(1 Suppl), S23–S25.
Rolls, E. T. (2019). The orbitofrontal cortex and emotion in health and disease, including depression. Neuropsychologia 128, 14–43. doi: 10.1016/j.neuropsychologia.2017.09.021
Rolls, E. T., and Grabenhorst, F. (2008). The orbitofrontal cortex and beyond: from affect to decision-making. Prog. Neurobiol. 86, 216–244. doi: 10.1016/j.pneurobio.2008.09.001
Rouiller, E. M., Tanne, J., Moret, V., and Boussaoud, D. (1999). Origin of thalamic inputs to the primary, premotor, and supplementary motor cortical areas and to area 46 in macaque monkeys: a multiple retrograde tracing study. J. Comp. Neurol. 409, 131–152. doi: 10.1002/(sici)1096-9861(19990621)409:1<131::aid-cne10>3.0.co;2-a
Sherman, S. M. (2017). Functioning of circuits connecting thalamus and cortex. Compr. Physiol. 7, 713–739. doi: 10.1002/cphy.c160032
Tillisch, K., Mayer, E. A., and Labus, J. S. (2011). Quantitative meta-analysis identifies brain regions activated during rectal distension in irritable bowel syndrome. Gastroenterology 140, 91–100. doi: 10.1053/j.gastro.2010.07.053
Tong, Q., Ma, J., and Kirchgessner, A. L. (2001). Vesicular glutamate transporter 2 in the brain-gut axis. Neuroreport 12, 3929–3934. doi: 10.1097/00001756-200112210-00015
Waters, A. M., Schilpzand, E., Bell, C., Walker, L. S., and Baber, K. (2013). Functional gastrointestinal symptoms in children with anxiety disorders. J. Abnorm. Child Psychol. 41, 151–163.
Zhu, Q., Cai, W., Zheng, J., Li, G., Meng, Q., Liu, Q., et al. (2016). Distinct resting-state brain activity in patients with functional constipation. Neurosci. Lett. 632, 141–146. doi: 10.1016/j.neulet.2016.08.042
Keywords: functional constipation, Allen Human Brain Atlas, gene expression analysis, gray matter volume, imaging-genetics
Citation: Cai W, Zhou Y, Wan L, Zhang R, Hua T, Gong J, Yang B and Tang G (2022) Transcriptomic Signatures Associated With Gray Matter Volume Changes in Patients With Functional Constipation. Front. Neurosci. 15:791831. doi: 10.3389/fnins.2021.791831
Received: 09 October 2021; Accepted: 23 November 2021;
Published: 05 January 2022.
Edited by:
Jiajia Zhu, First Affiliated Hospital of Anhui Medical University, ChinaReviewed by:
Huaigui Liu, Tianjin Medical University General Hospital, ChinaHuawang Wu, The Affiliated Brain Hospital of Guangzhou Medical University, China
Copyright © 2022 Cai, Zhou, Wan, Zhang, Hua, Gong, Yang and Tang. This is an open-access article distributed under the terms of the Creative Commons Attribution License (CC BY). The use, distribution or reproduction in other forums is permitted, provided the original author(s) and the copyright owner(s) are credited and that the original publication in this journal is cited, in accordance with accepted academic practice. No use, distribution or reproduction is permitted which does not comply with these terms.
*Correspondence: Guangyu Tang, tgy17@126.com
†These authors have contributed equally to this work