Corrigendum: The Mechanisms and Boundary Conditions of Drug Memory Reconsolidation
- 1Department of Forensic Science, School of Basic Medical Science, Central South University, Changsha, China
- 2Xiangya School of Medicine, Central South University, Changsha, China
- 3Key Laboratory of Molecular Epidemiology of Hunan Province, School of Medicine, Hunan Normal University, Changsha, China
- 4Department of Forensic Science, School of Basic Medical Science, Xinjiang Medical University, Urumqi, China
Drug addiction can be seen as a disorder of maladaptive learning characterized by relapse. Therefore, disrupting drug-related memories could be an approach to improving therapies for addiction. Pioneering studies over the last two decades have revealed that consolidated memories are not static, but can be reconsolidated after retrieval, thereby providing candidate pathways for the treatment of addiction. The limbic–corticostriatal system is known to play a vital role in encoding the drug memory engram. Specific structures within this system contribute differently to the process of memory reconsolidation, making it a potential target for preventing relapse. In addition, as molecular processes are also active during memory reconsolidation, amnestic agents can be used to attenuate drug memory. In this review, we focus primarily on the brain structures involved in storing the drug memory engram, as well as the molecular processes involved in drug memory reconsolidation. Notably, we describe reports regarding boundary conditions constraining the therapeutic potential of memory reconsolidation. Furthermore, we discuss the principles that could be employed to modify stored memories. Finally, we emphasize the challenge of reconsolidation-based strategies, but end with an optimistic view on the development of reconsolidation theory for drug relapse prevention.
Introduction
Memory in drug addiction is usually abnormal and is considered to reflect a learning disorder (Phelps and Hofmann, 2019). The central goal of addiction treatments is to prevent relapse and compulsive drug-seeking behavior. Drug-associated memories, therefore, provide an effective treatment target to reduce relapse (Lee et al., 2017). Generally, drug memory can be viewed as a kind of associative memory that combines a conditioned stimulus (CS) with a rewarding drug stimulus [the unconditioned stimulus (US)] (Xue et al., 2012). Researchers have made substantial progress in reducing negative effects related to drugs by disrupting associative memory. One of the factors contributing to this advance is the significant development of the theory of memory reconsolidation. Although early studies indicated that consolidated memory may be diminished after retrieval (Misanin et al., 1968; Przybyslawski and Sara, 1997), the mechanism underlying reconsolidation was not well understood. In 2000, Nader et al. (2000b) found that previously consolidated memories can be labile after retrieval, and that the synthesis of new proteins is necessary for long-term storage—a process they putatively termed reconsolidation. Initial research into reconsolidation mainly focused on fear memory, but because of the promising clinical therapeutic potential of this theory, later studies expanded the research to investigate drug memories with encouraging success (Lee et al., 2005; Xue et al., 2012). Editing well-established memories provides a means by which detrimental memories driving relapse can be disrupted (Xue et al., 2012, 2017b). Meanwhile, studies using a large variety of amnestic agents have contributed to elucidating neural circuits involved in memory updating. Here, we focus on reviewing the limbic–corticostriatal circuits recruited during reconsolidation, in addition to several molecular processes that may serve as potent targets to disrupt reconsolidation (see Figure 1). In addition, we discuss the boundary conditions that limit reconsolidation-based strategies.
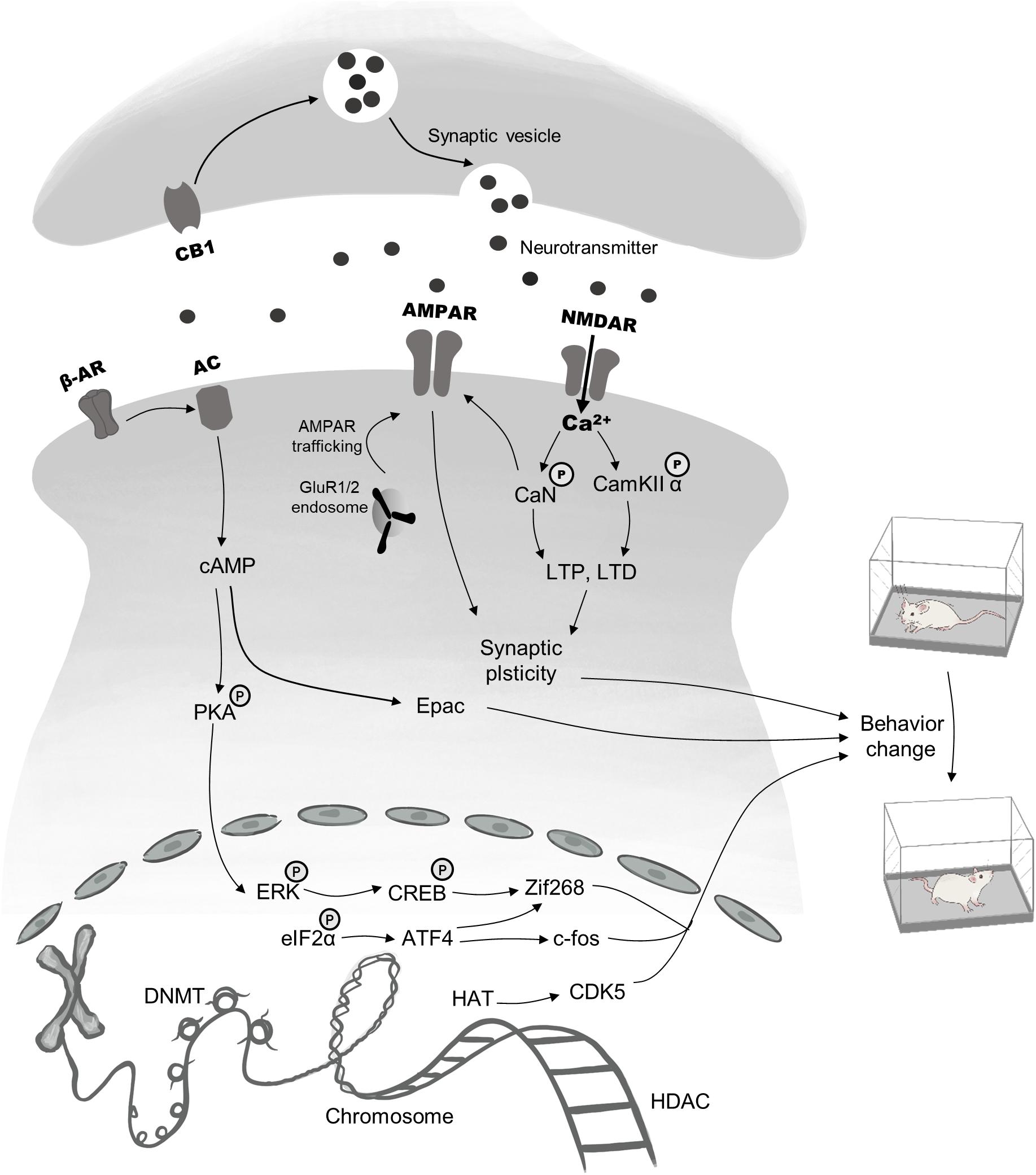
Figure 1. Brief description of pharmacological targets and signaling cascades recruited in reconsolidation. The process of drug memory reconsolidation requires a complicated regulatory network, including epigenetic mechanisms, gene transcription, and activation of membrane receptors, all of which are responsible for behavior changes. Targets for epigenetic modifications mainly lie in the HAT, the HDAC, the DNMT. Besides, the phosphorylation and dephosphorylation of ERK and eIF2α within the nucleus regulate the expression of immediate early genes, such as CREB, Zif 268, and c-fos, thus ultimately lead to changes in addiction behaviors. Finally, pre-and postsynaptic membrane receptors including AMPAR, NMDAR, β-AR, and CB1 have been proved to be effective targets. Main downstream mechanisms contain the second messenger (cAMP)-mediated pathway, AMPAR & NMDAR regulated synaptic plasticity and neurotransmitter transport.
Structures in the Brain Related to Reconsolidation
Several brain regions interact to form CS–US associative memories, thereby directing reward-seeking behaviors. Limbic-corticostriatal circuitry, including the amygdala, hippocampus, striatum, and prefrontal cortex, are required to form associative memories between stimuli and rewards. The underlying molecular mechanisms contributing to CS–US association memories in these brain areas and circuits are listed in Table 1.
Amygdala Is Required for Memory Retrieval
The amygdala is a brain structure that plays a critical role in emotion and motivation (Blundell et al., 2001) and that is actively involved in processing rewarding environmental stimuli (Janak and Tye, 2015). In terms of the subregions of the amygdala, the basolateral amygdala (BLA) is a key brain structure involved in CS-induced memory reconsolidation (Higginbotham et al., 2021a,b). BLA neurons store the associative emotional learning engrams that are recruited during retrieval (Pignatelli et al., 2019). BLA neurons receive dopaminergic input from the ventral tegmental area (VTA), and project to the nucleus accumbens (NAc) via glutamatergic neurons, contributing to the process underlying the incubation of craving (Lüscher, 2016). Remarkably, the central nucleus of the amygdala (CeA) and the BLA play different roles in memory reconsolidation (Kruzich and See, 2001). For example, Jian et al. (2014) found that CS-induced reconsolidation of morphine and cocaine memories in rats could be disrupted by selectively inhibiting the dephosphorylation of the eukaryotic initiation factor 2 α-subunit (eIF2α) in the BLA but not in the CeA. However, the CeA may play an essential role in US-induced drug memory reconsolidation. In another study, researchers found that US-induced but not CS-induced cocaine memory reconsolidation required β1-adrenergic signaling and de novo protein synthesis in the CeA, indicating that the CeA may be required for US retrieval but not CS retrieval (Zhu et al., 2018).
Hippocampus Is Required for the Storage of Drug-Paired Context
The hippocampus is known to organize episodic memory and is required for the formation of Pavlovian conditioned associations (also known as classical conditioning), as measured by conditioned place preference (CPP) (Taubenfeld et al., 2010; Liu et al., 2018). In terms of operant drug-seeking behavior, the hippocampus seems to be less directly required, as the cue or tone represents much less of a spatial object (Fuchs et al., 2005). Besides, the hippocampus does not appear to encode the memory trace of the conditioning context alone, as microinjections of the protein synthesis inhibitor anisomycin into the dorsal hippocampus (DH) do not disrupt cocaine memory reconsolidation (Ramirez et al., 2009). In contrast, contralateral BLA microinjections of the protein synthesis inhibitor baclofen/muscimol disrupt cocaine memory reconsolidation, suggesting that interaction between the DH and BLA is involved in editing the context–drug engram (Wells et al., 2011). Moreover, using optogenetic techniques, researchers found that the dorsal CA1 (dCA1) subregion of the hippocampus directly projects to the NAc, indicating that the spatial memory trace facilitates effective appetitive behavior via a limbic–motor interface (Trouche et al., 2019). Taken together, these studies demonstrate that the hippocampus is required for reward-motivated behavior and that it does not mediate reconsolidation alone.
Striatum Drives Cue–Reward Learning
The striatum is necessary for learning that actions result in reward and for executing actions. In rodents, the striatum is typically divided into three main subregions: dorsolateral striatum (DLS), dorsomedial striatum (DMS), and ventral striatum (VS) (Cox and Witten, 2019). The DLS plays a vital role in stimulus–response association, which is necessary for the formation of skills and for habituation (Barnes et al., 2005; O’Hare et al., 2016). In contrast, the DMS is involved in goal-directed behaviors that depend on response–outcome associations (Yin et al., 2005). In fact, there is a shift of action transitions from goal-directed to habitual after overtraining (Thorn et al., 2010). Both DLS and DMS are recruited in the process of drug-seeking and consumption, which rely on instrumental learning. Early studies proved that instrumental learning does not require protein synthesis-dependent memory reconsolidation (Hernandez and Kelley, 2004; Brown et al., 2008). However, recently it has been revealed that instrumental memories for drug addiction (e.g., to cocaine or nicotine) may go through reconsolidation (Exton-McGuinness et al., 2014, 2019; Piva et al., 2020), providing a new perspective on how to reduce drug abuse behaviors. With regard to the VS, its primary function can be attributed to its major component, the NAc, which is essential for the formation of stimulus–outcome associations in Pavlovian learning (Milton and Everitt, 2012). The NAc receives dopaminergic neuronal input from the VTA, which plays a key role in processing reward stimuli (Nutt et al., 2015). In addition, glutamatergic neurons in the BLA, prefrontal cortex (PFC), and ventral hippocampus also project to the NAc, contributing to its crucial role in drug-evoked synaptic plasticity (Lüscher, 2016).
Prefrontal Cortex Modulates Reward Circuits
Drug addiction was initially thought to be caused by the dysfunction of subcortical reward circuits. However, accumulating evidence indicates that the PFC is recruited during drug addiction via regulation of limbic reward regions (Goldstein and Volkow, 2011). The PFC is necessary for action selection and decision making based on the value of goals (Hyman et al., 2006; Szczepanski and Knight, 2014). Research has shown that associative learning during cocaine abuse induces plasticity in medial prefrontal cortex (mPFC) neurons to alter the reward system (Porter and Sepulveda-Orengo, 2020). Furthermore, the removal of perineuronal nets, which play an essential role in neural plasticity, from GABAergic interneurons modulating the activity of pyramidal neurons in the PFC impaired the reconsolidation of a cocaine CPP (Slaker et al., 2015), suggesting that the PFC is required for drug memory reconsolidation. In another study, β-adrenergic receptor (β-AR) blockade in the prelimbic medial prefrontal cortex (PL-mPFC) persistently reduced the expression of a cocaine CPP memory when administered before, but not after, cocaine memory retrieval (Otis et al., 2013). This indicates that blockade of the β-ARs disrupted the retrieval, but not the reconsolidation, of cocaine CPP memory. These studies illustrate the sophisticated role played by the PFC in the process of memory reconsolidation.
There are limitations in the conclusions that can be drawn from circuit-based studies targeting specific brain regions using lesion or inactivation, as these methods may disrupt neural communication between structures. The mechanisms underlying reconsolidation remain to be further clarified considering the neuronal projections within the limbic–corticostriatal system. With the advance of neuronal manipulation techniques, such as the newly developed wireless optogenetic technique (Yang et al., 2021), we are optimistic that these limitations can be addressed in future work.
Potential Targets for Relapse Prevention
While the brain areas required for associating environmental cues with drug stimuli have been well established, the underlying mechanisms of memory destabilization and re-stabilization at the molecular and synaptic levels are poorly understood. Here, we focus on reviewing novel potent targets for modulating drug memory reconsolidation.
Function of Epigenetic Mechanisms in Preventing Drug Relapse
Accumulating evidence indicates that epigenetic regulation plays a critical role in the process of drug-induced neuronal plasticity (Renthal and Nestler, 2008; Werner et al., 2021), which can involve long-lasting changes in gene expression and ultimately result in behavioral changes (Feng and Nestler, 2013). Here, we focus on the use of DNA demethylation and histone deacetylation during memory destabilization and their impact on relapse prevention.
DNA methylation in the VTA has been shown to play a role in associative memory combining environmental cues with drug reward (Day et al., 2013). Although DNA methylation was initially perceived as a stable process that cannot be rapidly modulated, subsequent studies have demonstrated that this is not the case (Miller et al., 2010; Zipperly et al., 2021). In the brain, DNA undergoes rapid methylation and demethylation, which is necessary for memory formation and synaptic plasticity (Feng et al., 2010; Miller et al., 2010; Day et al., 2013). This provides an avenue for disrupting drug memory during the reconsolidation window. For example, knockdown of the ten-eleven translocation 3 (TET3) gene of methylcytosine dioxygenase in pyramidal neurons of the DH was found to decrease the activation of pyramidal neurons, thus leading to the impairment of Pavlovian CPP memory reconsolidation (Liu et al., 2018). A putative explanation is that DNA demethylation promotes the binding of transcription factors (Miller et al., 2010; Jarome and Lubin, 2014), which in turn regulate the synthesis of new proteins necessary for memory updating. In another study, using a cocaine operant self-administration (SA) model, Massart et al. (2015) found that incubated cue-induced cocaine-seeking behavior was significantly reduced if the DNA methyltransferase (DNMT) inhibitor (RG108) was delivered intra-NAc on abstinence day 29 and immediately before the extinction test on day 30. This phenomenon could be reversed by the methyl donor S-adenosylmethionine (Massart et al., 2015), indicating that DNMT is a core target for relapse prevention. These results suggest that the signal cascade induced by memory reactivation opened a temporal window during which drug-related memories could be disrupted. In this study, the DNMT inhibitor RG108 was initially delivered one day before CS exposure and again immediately before CS presentation. In contrast, intra-BLA injection of the DNMT inhibitor 5-azacytidine (5-AZA) immediately after CS exposure, but not after a 6-h delay, disrupted cocaine memory reconsolidation (Shi et al., 2015). These two experiments reported similar results in spite of differences in the drug intervention times used, suggesting a complex mechanism underlying reconsolidation that will require more results to further clarify.
Experiments targeting the downstream gene, protein of the CDK5 (cyclin-dependent kinase 5), also found lower expression of the incubation of cocaine craving (Massart et al., 2015). Moreover, in another study, targeting protein kinase CDK5 in the BLA (but not CeA) immediately after memory reactivation, but not after a 6-h delay, abolished a cocaine CPP (Li et al., 2010). These studies suggest that DNA methylation is a core target for preventing relapse, and that protein expression, but not transcription, must be targeted within a specific reconsolidation time window (Nader et al., 2000a; Sorg et al., 2015).
Acetylation and deacetylation of chromatin is another signaling pathway involved in regulating the formation of drug context-associated memories contributing to addiction-like behaviors (Rogge et al., 2013; Bender and Torregrossa, 2020; Campbell et al., 2021). These processes are regulated by two kinds of functionally similar enzymes. Histone acetyltransferases (HATs) facilitate transcription (Korzus et al., 2004; Kouzarides, 2007; Barrett et al., 2011), while histone deacetylases (HDACs) repress transcription (Kumar et al., 2005; Kouzarides, 2007). Both HATs and HDACs are involved in long-term memory formation, and are necessary for Pavlovian cocaine memory consolidation (Malvaez et al., 2011; Taniguchi et al., 2012; Rogge et al., 2013). For example, mice showed significantly improved CPP acquisition after homozygous HDAC3 deletions, due to increased gene expression of c-Fos and nuclear receptor subfamily 4 group A member 2 (Nr4a2) (Rogge et al., 2013). As histone acetylation is required for drug-induced neuroplasticity, later studies have demonstrated that HATs and HDACs are actively involved in drug memory reconsolidation. For example, cue-induced cocaine reinstatement could be enhanced by infusion of trichostatin A (an HDAC inhibitor) into lateral amygdala (LA), and was disrupted by the amnestic agent garcinol (Monsey et al., 2019). These results suggest that changes in histone acetylation are required for memory reconsolidation specifically in the LA, and that targeting HDACs is a possible way to disrupt reconsolidation.
As epigenetic changes affect the initial stage of protein synthesis upstream of transcription, epigenetic manipulation often leads to non-specific consequences. To improve the therapeutic potential of the targeting epigenetic processes, downstream targets and their roles in drug relapse should be identified by future research.
Autophagy Is a Potent Target for Modifying Drug Memory
Autophagy is an essential pathway for maintaining proteostasis and plays a critical role in neuroplasticity (Liang, 2019). However, direct evidence of autophagy in drug memory consolidation and reconsolidation is rarely reported, although several studies have indicated that autophagy is involved in fear memory consolidation and reconsolidation. For example, one study found that autophagy is recruited in auditory fear memory consolidation by regulating inhibitory neurotransmission via GABA(A)R-associated protein (GABARAP) and its interaction with the GABA(A)R γ2 subunit (Li et al., 2019). Moreover, fear memory reactivation could be prevented by inhibiting synaptic protein degradation (Lee et al., 2008). This effect could be reversed by autophagy-induced synaptic α-amino-3-hydroxy-5-methyl-4-isoxazolepropionic acid receptor (AMPAR) endocytosis (Shehata et al., 2018), suggesting that autophagy may participate in memory reconsolidation via synaptic protein degradation. These reports reveal that the GABAR and AMPAR are potent targets for modulating memory consolidation and reconsolidation. A few studies have also reported that autophagy is required during the degradation of endocytosed GABARs in Caenorhabditis elegans (Rowland et al., 2006) and the degradation of AMPARs in hippocampal neurons (Shehata et al., 2012). As both the GABAR and AMPAR are required for learning and memory (Luo et al., 2015; Roth et al., 2020; Davenport et al., 2021), this presents an ideal opportunity to verify whether autophagy is involved in drug memory consolidation and reconsolidation. However, one pitfall of this approach may be that, as implied in the study (Shehata et al., 2018), autophagy does not directly disrupt reconsolidation, but rather helps to enhance memory destabilization, thus leading to changes in reconsolidation-resistant memories.
Beta-Adrenergic Signaling Is a Promising Safe Target for Preventing Relapse
Two potential explanations for the limited efficacy of reconsolidation-based pharmacological therapy in preventing relapse are the adverse effects of the amnestic agents administered and the huge surgical trauma involved in targeting specific brain areas. However, these challenges can be addressed by using an amnestic agent with little toxicity that can be delivered in a safe way. Propranolol, a non-specific β-AR blocker, has been reported to be a promising candidate for preventing nicotine, heroin, and cocaine relapse in the clinic (Zhao et al., 2011; Saladin et al., 2013; Xue et al., 2017b; Chen et al., 2021). Furthermore, propranolol has been shown to cross the blood–brain barrier and target β-ARs in the amygdala to modulate drug memory reconsolidation (Otis et al., 2015; Zhu et al., 2018). Propranolol has few side effects and has proved powerful in reducing drug craving. Traditionally, to reduce relapse, the association between drug-paired cues and drug reward is targeted to affect CS-induced reconsolidation. However, the application of this approach may be limited as environmental cues are diverse and the extinguished response to cues may be reinstated with the passage of time (Luo et al., 2015). Nevertheless, the limitations of targeting CS-induced reconsolidation could be addressed by also targeting US-induced reconsolidation (Luo et al., 2015). Propranolol is capable of disrupting US-induced reconsolidation via beta-adrenergic signaling (Xue et al., 2017a,b; Deng et al., 2020), making this signaling pathway a promising target for the prevention of relapse.
Editing Drug Memory at the Synaptic Level
Synaptic plasticity plays an essential role in neuroadaptations caused by drug addiction and the subsequent maladaptive learning (Kauer and Malenka, 2007). Drug abuse induces changes in synaptic strength, known as synaptic plasticity, to support the formation of associative memories between environment cues and drug reward. Technological advances in experimental methods have allowed precise observation of the process of reconsolidation. For example, one study observed that recognizing training contexts more precisely and more effectively during fear memory retrieval required transiently increased excitation of engram cells (neuroplasticity) (Pignatelli et al., 2019), indicating the dynamic nature of synapses during reconsolidation. Furthermore, in a SA model, researchers found that cocaine memory retrieval promoted the re-maturation of matured silent synapses during the destabilization window (Wright et al., 2020). Blocking silent synapse re-maturation in the NAc during this window gave rise to reduced cocaine-seeking behavior after cue exposure, suggesting that synaptic plasticity is required for memory reconsolidation. Intriguingly, independent of memory retrieval, Young et al. (2016) found that context-induced drug-seeking was disrupted by preventing synaptic actin polymerization in methamphetamine (METH) addiction. In addition, BLA spine dynamics have been shown to contribute to the formation and disruption of METH-associated memory (Young et al., 2020). As the impairment of METH-associated memories was independent of memory reactivation, this approach provides a novel way to edit drug memory outside of the reconsolidation window. These studies reveal the dynamic nature of synaptic plasticity in reconsolidation-dependent, as well as reconsolidation-independent, memory editing.
Boundary Conditions for Memory Reconsolidation
Boundaries for Memory Reactivation and Updating
Although drug-paired cues provide effective targets for editing addiction memory, there are several problems that limit reconsolidation-based therapy. Firstly, memory reactivation may be limited due to the requirements for specific retrieval conditions (e.g., context, schedule, or duration of retrieval) or memory features (e.g., age or strength) (Lee, 2009; Lee et al., 2017; Piva et al., 2019). In addition, to promote memory destabilization, a prediction error is usually required (Exton-McGuinness et al., 2015; Sinclair and Barense, 2019). Among people with drug addictions, inter-individual differences may be a boundary condition for the prediction error; this can be attributed to individual drug use histories and incentive value to cues (Kuijer et al., 2020). It would be a significant advance if future research was successful in identifying the biomarkers of drug memory destabilization (Wang et al., 2020). Secondly, CS-induced memory reconsolidation only helps to target specific drug-paired cues (Xue et al., 2012). Although US-induced reconsolidation seems to be a more effective target for preventing relapse (Luo et al., 2015; Dunbar and Taylor, 2017; Xue et al., 2017b), there may be ethical barriers to evoking drug memories using low-dose drug priming in the clinic. Lastly, memory updates may be ineffective in people with drug addictions who also suffer from psychiatric disorders. For instance, in a clinical study, compared with control subjects, patients diagnosed with schizophrenia displayed significant impairment of CS-induced recall of an extinguished memory (Holt et al., 2009).
Boundaries for Reconsolidation-Based Therapy
Reconsolidation-based therapy has not been appreciably improved by neuroscientific research. One boundary limiting this is that mechanistic studies using rodent models do not combine volitional social factors (Heilig et al., 2016). Recently, Venniro et al. (2018, 2019) and Venniro and Shaham (2020) introduced social context into the classic SA model, demonstrating that operant social interaction could prevent drug addiction, broadening the horizon of relapse prevention. To prevent relapse, methodological innovation is needed in order to provide new ways to understand addiction and to control drug abuse.
Conclusion
Over the last two decades, reconsolidation theory has progressed from a topic of debate to a basis for clinical therapy. Fundamental research in rodents has revealed the brain regions and molecular processes recruited during reconsolidation. However, boundary conditions limiting progress in memory destabilization and clinical translation remain a challenge for neurobiologists. Meanwhile, reports implying that drug memories can be modified without memory reactivation could provide a promising supplementary approach to reconsolidation-based therapy (Young et al., 2016, 2020). With the development of novel techniques and the accumulation of scientific evidence, we keep an open mind with regard to the potential role of reconsolidation theory in drug relapse prevention.
Author Contributions
LC developed the manuscript, corrected the style, reviewed and edited the manuscript, and discussed the central ideas of it. HY, YW, XF, SH, and FW developed the manuscript and discussed the central ideas of it. ZH and QL designed the graph and reviewed and edited the manuscript. JY developed the manuscript, proposed the central idea of it, reviewed and edited the manuscript, and acquired funding. All authors contributed to the article and approved the submitted version.
Funding
This work was supported in by National Natural Science Foundation of China (Nos. 81772024 and 82060339) and the Fundamental Research Funds for the Central Universities of Central South University (No. 2021zzts0939).
Conflict of Interest
The authors declare that the research was conducted in the absence of any commercial or financial relationships that could be construed as a potential conflict of interest.
Publisher’s Note
All claims expressed in this article are solely those of the authors and do not necessarily represent those of their affiliated organizations, or those of the publisher, the editors and the reviewers. Any product that may be evaluated in this article, or claim that may be made by its manufacturer, is not guaranteed or endorsed by the publisher.
References
Arguello, A. A., Hodges, M. A., Wells, A. M., Lara, H. III., Xie, X., and Fuchs, R. A. (2014). Involvement of amygdalar protein kinase A, but not calcium/calmodulin-dependent protein kinase II, in the reconsolidation of cocaine-related contextual memories in rats. Psychopharmacology (Berl) 231, 55–65. doi: 10.1007/s00213-013-3203-9
Barak, S., Liu, F., Hamida, S. B., Yowell, Q. V., Neasta, J., Kharazia, V., et al. (2013). Disruption of alcohol-related memories by mTORC1 inhibition prevents relapse. Nat. Neurosci. 16, 1111–1117. doi: 10.1038/nn.3439
Barnes, T. D., Kubota, Y., Hu, D., Jin, D. Z., and Graybiel, A. M. (2005). Activity of striatal neurons reflects dynamic encoding and recoding of procedural memories. Nature 437, 1158–1161. doi: 10.1038/nature04053
Barrett, R. M., Malvaez, M., Kramar, E., Matheos, D. P., Arrizon, A., Cabrera, S. M., et al. (2011). Hippocampal focal knockout of CBP affects specific histone modifications, long-term potentiation, and long-term memory. Neuropsychopharmacology 36, 1545–1556. doi: 10.1038/npp.2011.61
Bender, B. N., and Torregrossa, M. M. (2020). Molecular and circuit mechanisms regulating cocaine memory. Cell. Mol. Life Sci. 77, 3745–3768. doi: 10.1007/s00018-020-03498-8
Blundell, P., Hall, G., and Killcross, S. (2001). Lesions of the basolateral amygdala disrupt selective aspects of reinforcer representation in rats. J. Neurosci. 21, 9018–9026. doi: 10.1523/jneurosci.21-22-09018.2001
Brown, T. E., Lee, B. R., and Sorg, B. A. (2008). The NMDA antagonist MK-801 disrupts reconsolidation of a cocaine-associated memory for conditioned place preference but not for self-administration in rats. Learn. Mem. 15, 857–865. doi: 10.1101/lm.1152808
Campbell, R. R., Kramár, E. A., Pham, L., Beardwood, J. H., Augustynski, A. S., López, A. J., et al. (2021). HDAC3 Activity within the Nucleus Accumbens Regulates Cocaine-Induced Plasticity and Behavior in a Cell-Type-Specific Manner. J. Neurosci. 41, 2814–2827. doi: 10.1523/jneurosci.2829-20.2021
Chen, L., Huang, S., Yang, C., Wu, F., Zheng, Q., Yan, H., et al. (2021). Blockade of β-Adrenergic Receptors by Propranolol Disrupts Reconsolidation of Drug Memory and Attenuates Heroin Seeking. Front. Pharmacol. 12:686845. doi: 10.3389/fphar.2021.686845
Cox, J., and Witten, I. B. (2019). Striatal circuits for reward learning and decision-making. Nat. Rev. Neurosci. 20, 482–494. doi: 10.1038/s41583-019-0189-2
Davenport, C. M., Rajappa, R., Katchan, L., Taylor, C. R., Tsai, M. C., Smith, C. M., et al. (2021). Relocation of an Extrasynaptic GABA(A) Receptor to Inhibitory Synapses Freezes Excitatory Synaptic Strength and Preserves Memory. Neuron 109, 123–134.e4.
Day, J. J., Childs, D., Guzman-Karlsson, M. C., Kibe, M., Moulden, J., Song, E., et al. (2013). DNA methylation regulates associative reward learning. Nat. Neurosci. 16, 1445–1452. doi: 10.1038/nn.3504
Deng, J., Shi, L., Yuan, K., Yao, P., Chen, S., Que, J., et al. (2020). Propranolol-induced inhibition of unconditioned stimulus-reactivated fear memory prevents the return of fear in humans. Transl. Psychiatry 10:345.
Dunbar, A. B., and Taylor, J. R. (2017). Garcinol Blocks the Reconsolidation of Multiple Cocaine-Paired Cues after a Single Cocaine-Reactivation Session. Neuropsychopharmacology 42, 1884–1892. doi: 10.1038/npp.2017.27
Exton-McGuinness, M. T., Lee, J. L., and Reichelt, A. C. (2015). Updating memories–the role of prediction errors in memory reconsolidation. Behav. Brain Res. 278, 375–384. doi: 10.1016/j.bbr.2014.10.011
Exton-McGuinness, M. T., Patton, R. C., Sacco, L. B., and Lee, J. L. (2014). Reconsolidation of a well-learned instrumental memory. Learn. Mem. 21, 468–477. doi: 10.1101/lm.035543.114
Exton-McGuinness, M. T. J., Drame, M. L., Flavell, C. R., and Lee, J. L. C. (2019). On the Resistance to Relapse to Cocaine-Seeking Following Impairment of Instrumental Cocaine Memory Reconsolidation. Front. Behav. Neurosci. 13:242. doi: 10.3389/fnbeh.2019.00242
Feng, J., and Nestler, E. J. (2013). Epigenetic mechanisms of drug addiction. Curr. Opin. Neurobiol. 23, 521–528. doi: 10.1016/j.conb.2013.01.001
Feng, J., Zhou, Y., Campbell, S. L., Le, T., Li, E., Sweatt, J. D., et al. (2010). Dnmt1 and Dnmt3a maintain DNA methylation and regulate synaptic function in adult forebrain neurons. Nat. Neurosci. 13, 423–430. doi: 10.1038/nn.2514
Fuchs, R. A., Evans, K. A., Ledford, C. C., Parker, M. P., Case, J. M., Mehta, R. H., et al. (2005). The role of the dorsomedial prefrontal cortex, basolateral amygdala, and dorsal hippocampus in contextual reinstatement of cocaine seeking in rats. Neuropsychopharmacology 30, 296–309. doi: 10.1038/sj.npp.1300579
Goldstein, R. Z., and Volkow, N. D. (2011). Dysfunction of the prefrontal cortex in addiction: neuroimaging findings and clinical implications. Nat. Rev. Neurosci. 12, 652–669. doi: 10.1038/nrn3119
Heilig, M., Epstein, D. H., Nader, M. A., and Shaham, Y. (2016). Time to connect: bringing social context into addiction neuroscience. Nat. Rev. Neurosci. 17, 592–599. doi: 10.1038/nrn.2016.67
Hernandez, P. J., and Kelley, A. E. (2004). Long-term memory for instrumental responses does not undergo protein synthesis-dependent reconsolidation upon retrieval. Learn. Mem. 11, 748–754. doi: 10.1101/lm.84904
Higginbotham, J. A., Jones, N. M., Wang, R., Christian, R. J., Ritchie, J. L., Mclaughlin, R. J., et al. (2021a). Basolateral amygdala CB1 receptors gate HPA axis activation and context-cocaine memory strength during reconsolidation. Neuropsychopharmacology 46, 1554–1564. doi: 10.1038/s41386-020-00919-x
Higginbotham, J. A., Wang, R., Richardson, B. D., Shiina, H., Tan, S. M., Presker, M. A., et al. (2021b). CB1 Receptor Signaling Modulates Amygdalar Plasticity during Context-Cocaine Memory Reconsolidation to Promote Subsequent Cocaine Seeking. J. Neurosci. 41, 613–629. doi: 10.1523/jneurosci.1390-20.2020
Holt, D. J., Lebron-Milad, K., Milad, M. R., Rauch, S. L., Pitman, R. K., Orr, S. P., et al. (2009). Extinction memory is impaired in schizophrenia. Biol. Psychiatry 65, 455–463.
Hyman, S. E., Malenka, R. C., and Nestler, E. J. (2006). Neural mechanisms of addiction: the role of reward-related learning and memory. Annu. Rev. Neurosci. 29, 565–598. doi: 10.1146/annurev.neuro.29.051605.113009
Janak, P. H., and Tye, K. M. (2015). From circuits to behaviour in the amygdala. Nature 517, 284–292. doi: 10.1038/nature14188
Jarome, T. J., and Lubin, F. D. (2014). Epigenetic mechanisms of memory formation and reconsolidation. Neurobiol. Learn. Mem. 115, 116–127. doi: 10.1016/j.nlm.2014.08.002
Jian, M., Luo, Y. X., Xue, Y. X., Han, Y., Shi, H. S., Liu, J. F., et al. (2014). eIF2α dephosphorylation in basolateral amygdala mediates reconsolidation of drug memory. J. Neurosci. 34, 10010–10021. doi: 10.1523/jneurosci.0934-14.2014
Kauer, J. A., and Malenka, R. C. (2007). Synaptic plasticity and addiction. Nat. Rev. Neurosci. 8, 844–858.
Korzus, E., Rosenfeld, M. G., and Mayford, M. (2004). CBP histone acetyltransferase activity is a critical component of memory consolidation. Neuron 42, 961–972. doi: 10.1016/j.neuron.2004.06.002
Kouzarides, T. (2007). Chromatin modifications and their function. Cell 128, 693–705. doi: 10.1016/j.cell.2007.02.005
Kruzich, P. J., and See, R. E. (2001). Differential contributions of the basolateral and central amygdala in the acquisition and expression of conditioned relapse to cocaine-seeking behavior. J. Neurosci. 21:RC155.
Kuijer, E. J., Ferragud, A., and Milton, A. L. (2020). Retrieval-Extinction and Relapse Prevention: rewriting Maladaptive Drug Memories? Front. Behav. Neurosci. 14:23. doi: 10.3389/fnbeh.2020.00023
Kumar, A., Choi, K. H., Renthal, W., Tsankova, N. M., Theobald, D. E., Truong, H. T., et al. (2005). Chromatin remodeling is a key mechanism underlying cocaine-induced plasticity in striatum. Neuron 48, 303–314. doi: 10.1016/j.neuron.2005.09.023
Lee, J. L., Di Ciano, P., Thomas, K. L., and Everitt, B. J. (2005). Disrupting reconsolidation of drug memories reduces cocaine-seeking behavior. Neuron 47, 795–801. doi: 10.1016/j.neuron.2005.08.007
Lee, J. L., Milton, A. L., and Everitt, B. J. (2006). Cue-induced cocaine seeking and relapse are reduced by disruption of drug memory reconsolidation. J. Neurosci. 26, 5881–5887. doi: 10.1523/jneurosci.0323-06.2006
Lee, J. L. C. (2009). Reconsolidation: maintaining memory relevance. Trends Neurosci. 32, 413–420. doi: 10.1016/j.tins.2009.05.002
Lee, J. L. C., Nader, K., and Schiller, D. (2017). An Update on Memory Reconsolidation Updating. Trends Cogn. Sci. 21, 531–545. doi: 10.1016/j.tics.2017.04.006
Lee, S. H., Choi, J. H., Lee, N., Lee, H. R., Kim, J. I., Yu, N. K., et al. (2008). Synaptic protein degradation underlies destabilization of retrieved fear memory. Science 319, 1253–1256. doi: 10.1126/science.1150541
Li, F. Q., Xue, Y. X., Wang, J. S., Fang, Q., Li, Y. Q., Zhu, W. L., et al. (2010). Basolateral amygdala cdk5 activity mediates consolidation and reconsolidation of memories for cocaine cues. J. Neurosci. 30, 10351–10359. doi: 10.1523/jneurosci.2112-10.2010
Li, K., Chen, H. S., Li, D., Li, H. H., Wang, J., Jia, L., et al. (2019). SAR405, a Highly Specific VPS34 Inhibitor, Disrupts Auditory Fear Memory Consolidation of Mice via Facilitation of Inhibitory Neurotransmission in Basolateral Amygdala. Biol. Psychiatry 85, 214–225. doi: 10.1016/j.biopsych.2018.07.026
Liang, J., Li, J.-L., Han, Y., Luo, Y.-X., Xue, Y.-X., Zhang, Y., et al. (2017). Calpain-GRIP Signaling in Nucleus Accumbens Core Mediates the Reconsolidation of Drug Reward Memory. J. Neurosci. 37, 8938–8951. doi: 10.1523/jneurosci.0703-17.2017
Liang, Y. (2019). Emerging Concepts and Functions of Autophagy as a Regulator of Synaptic Components and Plasticity. Cells 8:34. doi: 10.3390/cells8010034
Liu, C., Sun, X., Wang, Z., Le, Q., Liu, P., Jiang, C., et al. (2018). Retrieval-Induced Upregulation of Tet3 in Pyramidal Neurons of the Dorsal Hippocampus Mediates Cocaine-Associated Memory Reconsolidation. Int. J. Neuropsychopharmacol. 21, 255–266. doi: 10.1093/ijnp/pyx099
Luo, Y.-X., Xue, Y.-X., Liu, J.-F., Shi, H.-S., Jian, M., Han, Y., et al. (2015). A novel UCS memory retrieval-extinction procedure to inhibit relapse to drug seeking. Nat. Commun. 6, 7675–7675.
Lüscher, C. (2016). The Emergence of a Circuit Model for Addiction. Annu. Rev. Neurosci. 39, 257–276. doi: 10.1146/annurev-neuro-070815-013920
Malvaez, M., Mhillaj, E., Matheos, D. P., Palmery, M., and Wood, M. A. (2011). CBP in the nucleus accumbens regulates cocaine-induced histone acetylation and is critical for cocaine-associated behaviors. J. Neurosci. 31, 16941–16948. doi: 10.1523/jneurosci.2747-11.2011
Massart, R., Barnea, R., Dikshtein, Y., Suderman, M., Meir, O., Hallett, M., et al. (2015). Role of DNA Methylation in the Nucleus Accumbens in Incubation of Cocaine Craving. J. Neurosci. 35, 8042–8058. doi: 10.1523/jneurosci.3053-14.2015
Miller, C. A., Gavin, C. F., White, J. A., Parrish, R. R., Honasoge, A., Yancey, C. R., et al. (2010). Cortical DNA methylation maintains remote memory. Nat. Neurosci. 13, 664–666. doi: 10.1038/nn.2560
Milton, A. L., and Everitt, B. J. (2012). The persistence of maladaptive memory: addiction, drug memories and anti-relapse treatments. Neurosci. Biobehav. Rev. 36, 1119–1139. doi: 10.1016/j.neubiorev.2012.01.002
Milton, A. L., Lee, J. L., Butler, V. J., Gardner, R., and Everitt, B. J. (2008). Intra-amygdala and systemic antagonism of NMDA receptors prevents the reconsolidation of drug-associated memory and impairs subsequently both novel and previously acquired drug-seeking behaviors. J. Neurosci. 28, 8230–8237. doi: 10.1523/jneurosci.1723-08.2008
Misanin, J. R., Miller, R. R., and Lewis, D. J. (1968). Retrograde amnesia produced by electroconvulsive shock after reactivation of a consolidated memory trace. Science 160, 554–555. doi: 10.1126/science.160.3827.554
Monsey, M. S., Ruiz, S. G., and Taylor, J. R. (2019). Regulation of Garcinol on Histone Acetylation in the Amygdala and on the Reconsolidation of a Cocaine-Associated Memory. Front. Behav. Neurosci. 13:281. doi: 10.3389/fnbeh.2019.00281
Nader, K., Schafe, G. E., and Le Doux, J. E. (2000a). Fear memories require protein synthesis in the amygdala for reconsolidation after retrieval. Nature 406, 722–726. doi: 10.1038/35021052
Nader, K., Schafe, G. E., and LeDoux, J. E. (2000b). The labile nature of consolidation theory. Nat. Rev. Neurosci. 1, 216–219. doi: 10.1038/35044580
Nutt, D. J., Lingford-Hughes, A., Erritzoe, D., and Stokes, P. R. (2015). The dopamine theory of addiction: 40 years of highs and lows. Nat. Rev. Neurosci. 16, 305–312. doi: 10.1038/nrn3939
O’Hare, J. K., Ade, K. K., Sukharnikova, T., Van Hooser, S. D., Palmeri, M. L., Yin, H. H., et al. (2016). Pathway-Specific Striatal Substrates for Habitual Behavior. Neuron 89, 472–479. doi: 10.1016/j.neuron.2015.12.032
Otis, J. M., Dashew, K. B., and Mueller, D. (2013). Neurobiological Dissociation of Retrieval and Reconsolidation of Cocaine-Associated Memory. J. Neurosci. 33, 1271–1281. doi: 10.1523/jneurosci.3463-12.2013
Otis, J. M., Werner, C. T., and Mueller, D. (2015). Noradrenergic regulation of fear and drug-associated memory reconsolidation. Neuropsychopharmacology 40, 793–803. doi: 10.1038/npp.2014.243
Phelps, E. A., and Hofmann, S. G. (2019). Memory editing from science fiction to clinical practice. Nature 572, 43–50. doi: 10.1038/s41586-019-1433-7
Pignatelli, M., Ryan, T. J., Roy, D. S., Lovett, C., Smith, L. M., Muralidhar, S., et al. (2019). Engram Cell Excitability State Determines the Efficacy of Memory Retrieval. Neuron 101, 274–284.e5.
Piva, A., Gerace, E., Di Chio, M., Padovani, L., Paolone, G., Pellegrini-Giampietro, D. E., et al. (2019). Reconsolidation of sucrose instrumental memory in rats: The role of retrieval context. Brain Res. 1714, 193–201. doi: 10.1016/j.brainres.2019.03.006
Piva, A., Pintori, N., Padovani, L., and Chiamulera, C. (2020). Protocols for instrumental memory reconsolidation in rodents: a methodological review. J. Neurosci. Methods 342:108766. doi: 10.1016/j.jneumeth.2020.108766
Porter, J. T., and Sepulveda-Orengo, M. T. (2020). Learning-induced intrinsic and synaptic plasticity in the rodent medial prefrontal cortex. Neurobiol. Learn. Mem. 169, 107117–107117. doi: 10.1016/j.nlm.2019.107117
Przybyslawski, J., and Sara, S. J. (1997). Reconsolidation of memory after its reactivation. Behav. Brain Res. 84, 241–246. doi: 10.1016/s0166-4328(96)00153-2
Ramirez, D. R., Bell, G. H., Lasseter, H. C., Xie, X., Traina, S. A., and Fuchs, R. A. (2009). Dorsal hippocampal regulation of memory reconsolidation processes that facilitate drug context-induced cocaine-seeking behavior in rats. Eur. J. Neurosci. 30, 901–912. doi: 10.1111/j.1460-9568.2009.06889.x
Renthal, W., and Nestler, E. J. (2008). Epigenetic mechanisms in drug addiction. Trends Mol. Med. 14, 341–350. doi: 10.1016/j.molmed.2008.06.004
Rich, M. T., Abbott, T. B., Chung, L., Gulcicek, E. E., Stone, K. L., Colangelo, C. M., et al. (2016). Phosphoproteomic Analysis Reveals a Novel Mechanism of CaMKIIα Regulation Inversely Induced by Cocaine Memory Extinction versus Reconsolidation. J. Neurosci. 36, 7613–7627. doi: 10.1523/jneurosci.1108-16.2016
Rich, M. T., Huang, Y. H., and Torregrossa, M. M. (2020). Calcineurin Promotes Neuroplastic Changes in the Amygdala Associated with Weakened Cocaine-Cue Memories. J. Neurosci. 40, 1344–1354. doi: 10.1523/jneurosci.0453-19.2019
Rogge, G. A., Singh, H., Dang, R., and Wood, M. A. (2013). HDAC3 is a negative regulator of cocaine-context-associated memory formation. J. Neurosci. 33, 6623–6632. doi: 10.1523/jneurosci.4472-12.2013
Roth, R. H., Cudmore, R. H., Tan, H. L., Hong, I., Zhang, Y., and Huganir, R. L. (2020). Cortical Synaptic AMPA Receptor Plasticity during Motor Learning. Neuron 105, 895–908.e5.
Rowland, A. M., Richmond, J. E., Olsen, J. G., Hall, D. H., and Bamber, B. A. (2006). Presynaptic terminals independently regulate synaptic clustering and autophagy of GABAA receptors in Caenorhabditis elegans. J. Neurosci. 26, 1711–1720. doi: 10.1523/jneurosci.2279-05.2006
Saladin, M. E., Gray, K. M., Mcrae-Clark, A. L., Larowe, S. D., Yeatts, S. D., Baker, N. L., et al. (2013). A double blind, placebo-controlled study of the effects of post-retrieval propranolol on reconsolidation of memory for craving and cue reactivity in cocaine dependent humans. Psychopharmacology (Berl) 226, 721–737. doi: 10.1007/s00213-013-3039-3
Shehata, M., Abdou, K., Choko, K., Matsuo, M., Nishizono, H., and Inokuchi, K. (2018). Autophagy Enhances Memory Erasure through Synaptic Destabilization. J. Neurosci. 38, 3809–3822. doi: 10.1523/jneurosci.3505-17.2018
Shehata, M., Matsumura, H., Okubo-Suzuki, R., Ohkawa, N., and Inokuchi, K. (2012). Neuronal stimulation induces autophagy in hippocampal neurons that is involved in AMPA receptor degradation after chemical long-term depression. J. Neurosci. 32, 10413–10422. doi: 10.1523/jneurosci.4533-11.2012
Shi, H. S., Luo, Y. X., Yin, X., Wu, H. H., Xue, G., Geng, X. H., et al. (2015). Reconsolidation of a cocaine associated memory requires DNA methyltransferase activity in the basolateral amygdala. Sci. Rep. 5:13327.
Sinclair, A. H., and Barense, M. D. (2019). Prediction Error and Memory Reactivation: how Incomplete Reminders Drive Reconsolidation. Trends Neurosci. 42, 727–739. doi: 10.1016/j.tins.2019.08.007
Slaker, M., Churchill, L., Todd, R. P., Blacktop, J. M., Zuloaga, D. G., Raber, J., et al. (2015). Removal of perineuronal nets in the medial prefrontal cortex impairs the acquisition and reconsolidation of a cocaine-induced conditioned place preference memory. J. Neurosci. 35, 4190–4202. doi: 10.1523/jneurosci.3592-14.2015
Sorg, B. A., Todd, R. P., Slaker, M., and Churchill, L. (2015). Anisomycin in the medial prefrontal cortex reduces reconsolidation of cocaine-associated memories in the rat self-administration model. Neuropharmacology 92, 25–33. doi: 10.1016/j.neuropharm.2014.12.029
Szczepanski, S. M., and Knight, R. T. (2014). Insights into human behavior from lesions to the prefrontal cortex. Neuron 83, 1002–1018. doi: 10.1016/j.neuron.2014.08.011
Taniguchi, M., Carreira, M. B., Smith, L. N., Zirlin, B. C., Neve, R. L., and Cowan, C. W. (2012). Histone deacetylase 5 limits cocaine reward through cAMP-induced nuclear import. Neuron 73, 108–120. doi: 10.1016/j.neuron.2011.10.032
Taubenfeld, S. M., Muravieva, E. V., Garcia-Osta, A., and Alberini, C. M. (2010). Disrupting the memory of places induced by drugs of abuse weakens motivational withdrawal in a context-dependent manner. Proc. Natl. Acad. Sci. U. S. A. 107, 12345–12350. doi: 10.1073/pnas.1003152107
Thorn, C. A., Atallah, H., Howe, M., and Graybiel, A. M. (2010). Differential dynamics of activity changes in dorsolateral and dorsomedial striatal loops during learning. Neuron 66, 781–795. doi: 10.1016/j.neuron.2010.04.036
Trouche, S., Koren, V., Doig, N. M., Ellender, T. J., El-Gaby, M., Lopes-Dos-Santos, V., et al. (2019). A Hippocampus-Accumbens Tripartite Neuronal Motif Guides Appetitive Memory in Space. Cell 176, 1393–1406.e16.
Venniro, M., Russell, T. I., Zhang, M., and Shaham, Y. (2019). Operant Social Reward Decreases Incubation of Heroin Craving in Male and Female Rats. Biol. Psychiatry 86, 848–856. doi: 10.1016/j.biopsych.2019.05.018
Venniro, M., and Shaham, Y. (2020). An operant social self-administration and choice model in rats. Nat. Protoc. 15, 1542–1559. doi: 10.1038/s41596-020-0296-6
Venniro, M., Zhang, M., Caprioli, D., Hoots, J. K., Golden, S. A., Heins, C., et al. (2018). Volitional social interaction prevents drug addiction in rat models. Nat. Neurosci. 21, 1520–1529. doi: 10.1038/s41593-018-0246-6
Wan, X., Torregrossa, M. M., Sanchez, H., Nairn, A. C., and Taylor, J. R. (2014). Activation of exchange protein activated by cAMP in the rat basolateral amygdala impairs reconsolidation of a memory associated with self-administered cocaine. PLoS One 9:e107359. doi: 10.1371/journal.pone.0107359
Wang, X.-Y., Zhao, M., Ghitza, U. E., Li, Y.-Q., and Lu, L. (2008). Stress Impairs Reconsolidation of Drug Memory via Glucocorticoid Receptors in the Basolateral Amygdala. J. Neurosci. 28, 5602–5610. doi: 10.1523/jneurosci.0750-08.2008
Wang, Z., Jin, T., Le, Q., Liu, C., Wang, X., Wang, F., et al. (2020). Retrieval-Driven Hippocampal NPTX2 Plasticity Facilitates the Extinction of Cocaine-Associated Context Memory. Biol. Psychiatry 87, 979–991. doi: 10.1016/j.biopsych.2019.10.009
Wells, A. M., Arguello, A. A., Xie, X., Blanton, M. A., Lasseter, H. C., Reittinger, A. M., et al. (2013). Extracellular signal-regulated kinase in the basolateral amygdala, but not the nucleus accumbens core, is critical for context-response-cocaine memory reconsolidation in rats. Neuropsychopharmacology 38, 753–762. doi: 10.1038/npp.2012.238
Wells, A. M., Lasseter, H. C., Xie, X., Cowhey, K. E., Reittinger, A. M., and Fuchs, R. A. (2011). Interaction between the basolateral amygdala and dorsal hippocampus is critical for cocaine memory reconsolidation and subsequent drug context-induced cocaine-seeking behavior in rats. Learn. Mem. 18, 693–702. doi: 10.1101/lm.2273111
Werner, C. T., Altshuler, R. D., Shaham, Y., and Li, X. (2021). Epigenetic Mechanisms in Drug Relapse. Biol. Psychiatry 89, 331–338. doi: 10.1016/j.biopsych.2020.08.005
Wright, W. J., Graziane, N. M., Neumann, P. A., Hamilton, P. J., Cates, H. M., Fuerst, L., et al. (2020). Silent synapses dictate cocaine memory destabilization and reconsolidation. Nat. Neurosci. 23, 32–46. doi: 10.1038/s41593-019-0537-6
Wu, Y., Li, Y., Yang, X., and Sui, N. (2014). Differential effect of beta-adrenergic receptor antagonism in basolateral amygdala on reconsolidation of aversive and appetitive memories associated with morphine in rats. Addict Biol. 19, 5–15. doi: 10.1111/j.1369-1600.2012.00443.x
Xue, Y. X., Chen, Y. Y., Zhang, L. B., Zhang, L. Q., Huang, G. D., Sun, S. C., et al. (2017a). Selective Inhibition of Amygdala Neuronal Ensembles Encoding Nicotine-Associated Memories Inhibits Nicotine Preference and Relapse. Biol. Psychiatry 82, 781–793. doi: 10.1016/j.biopsych.2017.04.017
Xue, Y. X., Deng, J. H., Chen, Y. Y., Zhang, L. B., Wu, P., Huang, G. D., et al. (2017b). Effect of Selective Inhibition of Reactivated Nicotine-Associated Memories With Propranolol on Nicotine Craving. JAMA Psychiatry 74, 224–232. doi: 10.1001/jamapsychiatry.2016.3907
Xue, Y. X., Luo, Y. X., Wu, P., Shi, H. S., Xue, L. F., Chen, C., et al. (2012). A memory retrieval-extinction procedure to prevent drug craving and relapse. Science 336, 241–245. doi: 10.1126/science.1215070
Yang, Y., Wu, M., Vázquez-Guardado, A., Wegener, A. J., Grajales-Reyes, J. G., Deng, Y., et al. (2021). Wireless multilateral devices for optogenetic studies of individual and social behaviors. Nat. Neurosci. 24, 1035–1045. doi: 10.1038/s41593-021-00849-x
Yim, A. J., Moraes, C. R., Ferreira, T. L., and Oliveira, M. G. (2006). Protein synthesis inhibition in the basolateral amygdala following retrieval does not impair expression of morphine-associated conditioned place preference. Behav. Brain Res. 171, 162–169. doi: 10.1016/j.bbr.2006.03.031
Yin, H. H., Ostlund, S. B., Knowlton, B. J., and Balleine, B. W. (2005). The role of the dorsomedial striatum in instrumental conditioning. Eur. J. Neurosci. 22, 513–523. doi: 10.1111/j.1460-9568.2005.04218.x
Young, E. J., Blouin, A. M., Briggs, S. B., Sillivan, S. E., Lin, L., Cameron, M. D., et al. (2016). Nonmuscle myosin IIB as a therapeutic target for the prevention of relapse to methamphetamine use. Mol. Psychiatry 21, 615–623. doi: 10.1038/mp.2015.103
Young, E. J., Lin, H., Kamenecka, T. M., Rumbaugh, G., and Miller, C. A. (2020). Methamphetamine Learning Induces Persistent and Selective Nonmuscle Myosin II-Dependent Spine Motility in the Basolateral Amygdala. J. Neurosci. 40, 2695–2707. doi: 10.1523/jneurosci.2182-19.2020
Zhang, F., Huang, S., Bu, H., Zhou, Y., Chen, L., Kang, Z., et al. (2021). Disrupting Reconsolidation by Systemic Inhibition of mTOR Kinase via Rapamycin Reduces Cocaine-Seeking Behavior. Front. Pharmacol. 12:652865. doi: 10.3389/fphar.2021.652865
Zhao, L. Y., Sun, L. L., Shi, J., Li, P., Zhang, Y., and Lu, L. (2011). Effects of β-adrenergic receptor blockade on drug-related memory reconsolidation in abstinent heroin addicts. Drug Alcohol Depend. 118, 224–229. doi: 10.1016/j.drugalcdep.2011.03.025
Zhu, H., Zhou, Y., Liu, Z., Chen, X., Li, Y., Liu, X., et al. (2018). β1-Adrenoceptor in the Central Amygdala Is Required for Unconditioned Stimulus-Induced Drug Memory Reconsolidation. Int. J. Neuropsychopharmacol. 21, 267–280. doi: 10.1093/ijnp/pyx104
Keywords: drug memory, addiction, reconsolidation, limbic–corticostriatal system, boundary condition
Citation: Chen L, Yan H, Wang Y, He Z, Leng Q, Huang S, Wu F, Feng X and Yan J (2021) The Mechanisms and Boundary Conditions of Drug Memory Reconsolidation. Front. Neurosci. 15:717956. doi: 10.3389/fnins.2021.717956
Received: 31 May 2021; Accepted: 20 July 2021;
Published: 06 August 2021.
Edited by:
Qi Wang, Southern Medical University, ChinaReviewed by:
Jie Zhu, Xi’an Jiaotong University, ChinaJingsong Yuan, Columbia University, United States
Copyright © 2021 Chen, Yan, Wang, He, Leng, Huang, Wu, Feng and Yan. This is an open-access article distributed under the terms of the Creative Commons Attribution License (CC BY). The use, distribution or reproduction in other forums is permitted, provided the original author(s) and the copyright owner(s) are credited and that the original publication in this journal is cited, in accordance with accepted academic practice. No use, distribution or reproduction is permitted which does not comply with these terms.
*Correspondence: Jie Yan, wills212156@csu.edu.cn