- 1IRCCS, Fondazione Don Carlo Gnocchi ONLUS, Milan, Italy
- 2Department of Neurology, Buffalo Neuroimaging Analysis Center, School of Medicine and Biomedical Sciences, University at Buffalo, State University of New York, Buffalo, NY, United States
Background: Motor rehabilitation is routinely used in clinical practice as an effective method to reduce progressive disability gain in multiple sclerosis (MS), but rehabilitation approaches are typically unstandardized, and only few studies have investigated the impact of rehabilitation on brain neuroplasticity.
Objective: To summarize and critically analyze studies applying MRI markers of functional connectivity and structural changes to assess the effect of motor rehabilitation on brain neuroplasticity in MS.
Methods: Literature search was performed using PubMed and EMBASE, selecting studies having as a subject motor rehabilitation and advanced MRI techniques investigating neuroplasticity in adult patients affected by MS.
Results: Seventeen out of 798 papers were selected, of which 5 applied structural MRI (4 diffusion tensor imaging, 1 volumetric measurements), 7 applied functional fMRI (5 task-related fMRI, 2 resting-state fMRI) whereas the remaining 5 applied both structural and functional imaging.
Discussion: The considerable data heterogeneity and the small sample sizes characterizing the studies limit interpretation and generalization of the results. Overall, motor rehabilitation promotes clinical improvement, paralleled by positive adaptive brain changes, whose features and extent depend upon different variables, including the type of rehabilitation approach. MRI markers of functional and structural connectivity should be implemented in studies testing the efficacy of motor rehabilitation. They allow for a better understanding of neuroplastic mechanisms underlying rehabilitation-mediated clinical achievements, facilitating the identification of rehabilitation strategies tailored to patients' needs and abilities.
Introduction
Multiple sclerosis (MS) is the primary cause of non-traumatic neurological disability in young adults (Goodin, 2014). In the last decades, the progressively expanding availability of pharmacological treatments has changed the disease evolution, with a dramatic impact on the inflammatory component of the relapsing-remitting (RR) phase (Comi et al., 2017). This translates into a reduced relapse rate as well as fewer new/active lesions on magnetic resonance imaging (MRI). Unfortunately, though, the available pharmaceutical armament is considerably less efficacious in treating the neurodegenerative aspect of the disease, with a less meaningful impact on disease progression, which in turns leads to a higher MS-related Global Burden of Disease (GBD) (GBD 2016 Multiple Sclerosis Collaborators, 2019). According to the last GBD Study, neurological diseases are the primary cause of disability-adjusted life years (DALYs), and whereas MS-related mortality has significantly decreased in the last decade, age-related DALYs have not changed (GBD 2016 Neurology Collaborators, 2019). Accordingly, morbidity has a stronger impact on GBD, with moderate-to-severe cases accounting for more than 60% of disease burden (GBD 2016 Multiple Sclerosis Collaborators, 2019; Kaufmann et al., 2020). Moreover, identified risk factors explain <10% of DALYs burden in most neurological conditions, including MS (GBD 2016 Multiple Sclerosis Collaborators, 2019). Therefore, the lack of preventive approaches and the limited ability of pharmacological therapies to prevent neurodegeneration provide a compelling need for strategies capable of mitigating progressive disability gain.
It is clear that physical activity in MS has a positive influence, as demonstrated in both experimental and clinical settings (Dalgas et al., 2009; Rossi et al., 2009; Latimer-Cheung et al., 2013). Whereas earlier studies highlighted the beneficial effects of exercise at a peripheral level (i.e., osteoarticular/muscular and cardiovascular systems), (Dalgas et al., 2009; Latimer-Cheung et al., 2013) it has become increasingly clear that physical activity has a major effect on brain reorganization as well (Prakash et al., 2007, 2010; Motl et al., 2015; El-Sayes et al., 2019; Guo et al., 2020; Lozinski and Yong, 2020). Neuroplasticity, intended as the ability of the brain to modify itself at a structural and functional level in response to aging, experiences and environmental stimuli, occurs throughout the lifespan, as evidenced by animal and MRI based-human studies (Maguire et al., 2000; Draganski et al., 2004; Bengtsson et al., 2005; Zatorre et al., 2012; Garthe et al., 2016; Lambert et al., 2019).
The more widespread use of advanced imaging techniques, particularly with MRI, has also shed light on the neuroplastic changes that occur in the context of neurological diseases (Figure 1). In particular, in MS, brain tissue repair mechanisms and functional reorganization, at least initially, counterbalance the effect of the two main pathogenic mechanisms involved in the disease progression, inflammation and neurodegeneration (Koudriavtseva and Mainero, 2016).
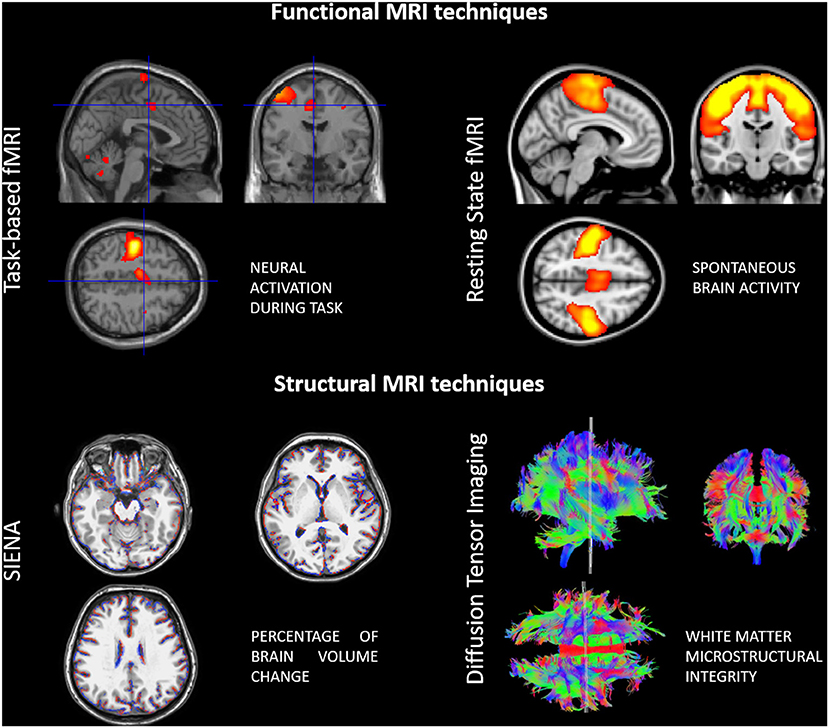
Figure 1. Examples of advanced functional and structural MRI techniques used for assessing neuroplasticity in multiple sclerosis patients.
Diffusion tensor imaging (DTI) is commonly used to study structural aspects, as DTI-derived markers [e.g., mean diffusivity (MD), axial diffusivity (AD), radial diffusivity (RD), and fractional anisotropy (FA)] reflect water mobility within the tissue and along fibers. Volumetric measures of the gray and white matter facilitate the quantification of structural changes over time as well. With functional MRI (fMRI) one can investigate regional or global brain activity, based on blood-oxygenation level dependent (BOLD) signal changes. Previously, fMRI studies were focused on brain activation in response to specific tasks executed by the patients during MRI acquisition (e.g., motor, visual, cognitive). More recently though, resting-state fMRI (rs-fMRI) has become increasingly popular as studying connectivity between different brain areas in resting conditions is possible. This overcomes limitations related to task-execution (standardization of movements in terms of rate and amplitude, possible confounding factors related movement) and also allows the exploration of functional interactions between different brain areas, usually integrated in so-called networks (Tahedl et al., 2018).
Advanced imaging has also been used to study physical activity, with consistent results of a positive effect of exercise on structural changes and functional connectivity (FC) (Prakash et al., 2011) as well as to evaluate the efficacy of neurorehabilitation (Tavazzi et al., 2018).
Structural changes described after rehabilitation treatment include remyelination, neuroaxonal regeneration, neuronal sprouting, synaptogenesis, whereas functional changes reflect adaptive network rearrangements. The net effect is thought to compensate for damaged tissue (Plautz et al., 2000). Importantly though, these processes cannot be studied at the cellular level with MRI, given its much coarser spatial resolution. Moreover, disentangling the role of disparate mechanisms acting simultaneously is challenging. Nevertheless, advanced MRI can still facilitate the study of structural and functional responses in the brain with respect to ongoing tissue damage (Tavazzi et al., 2020).
Despite the fact that rehabilitation is widely utilized for people with MS (pwMS), especially in mid-to-late disease stages, the lack of robust markers, together with the use of different variables (rehabilitative settings, as well as type, frequency, and duration of treatment) make literature studies difficult to compare.
The role of neurorehabilitation on disability accrual and functional impairment is potentially of great clinical relevance, and a better understanding of the underlying mechanisms related to neuroplasticity would have several benefits. First, it would allow for the creation of a more standardized and reproducible process regarding the screening and evaluation of patients eligible for rehabilitation, based not only on clinical but MRI criteria as well. Second, it would facilitate the selection of patients more suitable for rehabilitation intervention. Third, rehabilitative approaches could be tailored to the individual, according to the degrees of physical impairment and tissue injury.
With this background, the aim of this systematic review is to summarize and critically analyze literature data on MRI markers of FC and structural changes (SC) in pwMS undergoing motor rehabilitation.
Methods
PICOS Eligibility Criteria
Participants
The only eligibility criterion was the recruitment of adult patients (≥18 years old) affected by MS. All disease phenotypes (relapsing-remitting MS-RRMS; progressive MS-PMS) were considered eligible.
Interventions
Studies applying quantitative non-conventional MRI techniques on pwMS undergoing motor rehabilitation, both physical (physical exercise, resistance training, aerobic exercise, balance training, endurance training, action-observation therapy, motor rehabilitation using robotic devices for upper or lower limbs) and virtual (virtual reality) were selected. As such, studies reporting MRI results after a single rehabilitative session or studies applying MRI to the study of a specific task that was not part of a rehabilitative treatment were excluded. Moreover, studies using exclusively brain stimulation to enhance brain plasticity, not associated with motor rehabilitation, were excluded. Studies on cognitive rehabilitation of any sort, or studies aiming at improving cognitive functions, were excluded. Finally, treatment was considered “active” when the patient themselves performed the intended action while “passive” was defined as a treatment applied to the patient (e.g., a physiotherapist moving the patient's limb).
Comparisons
Both studies with a group of treated patients (i.e., patients undergoing motor rehabilitation of any sort) and a control group of patients not undergoing any treatment, studies comparing groups of patients undergoing different rehabilitative treatments and studies comparing patients undergoing rehabilitation and healthy subjects.
Outcomes
The outcome considered was the evaluation of motor rehabilitation on surrogate MRI markers representative of FC and/or SC. Most studies had two time points (e.g., baseline-before rehabilitation initiation-, and post-treatment), only few described a third time point, usually planned 1 month after the end of the rehabilitative treatment, or, in the case of randomized cross-over trials, performed before the 2 groups switched treatment.
Study Designs
Peer-reviewed Randomized and non-Randomized Controlled Trials including ≥5 subjects, and case studies were included in the analysis. Conference proceedings, reviews, book chapters, case reports and editorials were excluded.
Information Sources, Search, and Study Selection
Literature search was conducted using MEDLINE (via PubMed), Cochrane Central Register of Controlled Trials (CENTRAL), and EMBASE from inception to November 3rd 2020. The MeSH terms “multiple sclerosis” AND (“rehabilitation” OR “physiotherapy” OR “exercise” OR “virtual reality” OR “robotics”) AND (“MRI” OR “brain plasticity” OR “connectivity”). Papers written in languages other than English were excluded. References from the selected articles were then screened for further records. Three researchers (ET, NB, MC) independently assessed the selected articles to evaluate their eligibility, and disagreements were solved by discussion.
Data Extraction
For each study, the study design, number of subjects, rehabilitative setting (e.g., inpatient, outpatient, physiotherapist-supervised, home-based), MRI markers pre- and post-intervention were extracted and reported.
Testex Evaluation for Randomized Clinical Trials
The included RCTs were evaluated according to the Tool for the assessment of Study qualiTy and reporting in Exercise (TESTEX) scale, specifically developed to evaluate rehabilitative studies both in terms of study quality and reporting. The TESTEX scale consists of 12 criteria and a full scoring of 15 points (Smart et al., 2015). According to the scoring the studies were classified as “high quality” (12–15 points), “good quality” (7–11 points), or “low quality” (6 points or less) as previously suggested (Batalik et al., 2021). In the event that the clinical and the neuroimaging data were reported in separate manuscripts, details were extracted from the associated article that reported a complete description of the protocol.
The current review is reported according to PRISMA (Preferred Reporting Items for Systematic Reviews and Meta-Analyses) criteria (Page et al., 2021). The protocol for the review was not registered before the literature search began.
Results
The literature search retrieved, as of November 3rd, 2020, 798 papers using the abovementioned MeSH terms, to which we added 2 papers retrieved from references. Seven-hundred and eighty-three papers were eliminated for the following reasons: duplicates (248), not fitting with the topic of the review after reading the title/abstract (511) or the entire manuscript (7), editorials or opinion articles (2), reviews (11), case reports (2), papers reporting only information on planned trials (2). A total of 17 papers are discussed in details in this review. The flow chart summarizing the selection process is depicted in Figure 2.
Characteristics of the Studies
The key features of the studies are reported in Table 1 for clinical and rehabilitation aspects. Table 2 describes the imaging characteristics. Table 3 provides the TESTEX evaluations of the included studies.
The study designs were as follows: 10 randomized clinical trials (RCT) (Bonzano et al., 2014, 2019; Prosperini et al., 2014; Barghi et al., 2018; Kjolhede et al., 2018; Tavazzi et al., 2018; Rocca et al., 2019; Boffa et al., 2020; Prochazkova et al., 2020; Stellmann et al., 2020), plus 1 clinical RCT where the imaging component involved only one subgroup of the intervention arm (Fling et al., 2019). 7 non-randomized clinical trials [1 semi-RCT (Akbar et al., 2020), 2 case-control studies (CC) (Rasova et al., 2005; Ibrahim et al., 2011), 1 case series (CS) (Rasova et al., 2015), 1 parallel-group (PG) (Zuber et al., 2020), 1 self-controlled case series (SCCS) (Peran et al., 2020)].
With respect to patient characteristics, most studies recruited both RRMS and PMS (Bonzano et al., 2014, 2019; Prosperini et al., 2014; Rasova et al., 2015; Barghi et al., 2018; Tavazzi et al., 2018; Fling et al., 2019; Rocca et al., 2019; Akbar et al., 2020; Prochazkova et al., 2020; Zuber et al., 2020), 2 studies recruited only PMS (Boffa et al., 2020; Peran et al., 2020), 3 studies recruited exclusively RRMS (Ibrahim et al., 2011; Kjolhede et al., 2018; Stellmann et al., 2020), whereas disease phenotype was not reported for 1 study (Rasova et al., 2005).
Regarding the rehabilitative treatment, the setting was as follows: inpatient (IP, 3) (Tavazzi et al., 2018; Peran et al., 2020; Zuber et al., 2020), outpatient (OP, 4) (Rasova et al., 2005; Fling et al., 2019; Prochazkova et al., 2020; Stellmann et al., 2020), physiotherapist supervised without further information on the setting (7) (Ibrahim et al., 2011; Bonzano et al., 2014, 2019; Rasova et al., 2015; Kjolhede et al., 2018; Rocca et al., 2019; Boffa et al., 2020), home-based (1) (Prosperini et al., 2014), OP + home-based (2) (Barghi et al., 2018; Akbar et al., 2020). The mean duration of each rehabilitative session was 85 ± 46 min. The weekly frequency of the physiotherapy sessions ranged from 1 to 6 days/week, and the total duration of the rehabilitative program ranged from 2 to 16 weeks.
MRI was always acquired both before and at the end of the rehabilitative cycle, whereas in 5 studies there was also an additional MRI time-point, either within the cycle (Prosperini et al., 2014; Kjolhede et al., 2018), after 4 weeks (Akbar et al., 2020; Prochazkova et al., 2020), or after 3 months (Tavazzi et al., 2018). Among the 17 selected studies, 5 applied structural MRI [4 diffusion tensor imaging (DTI) (Ibrahim et al., 2011; Bonzano et al., 2014; Prosperini et al., 2014; Barghi et al., 2018), 1 volumetric measurements (Kjolhede et al., 2018)], 7 applied functional fMRI [5 task-related fMRI (Rasova et al., 2005; Bonzano et al., 2019; Peran et al., 2020; Prochazkova et al., 2020; Zuber et al., 2020)], 2 resting-state fMRI (Fling et al., 2019; Akbar et al., 2020) whereas the remaining 5 applied both structural and functional imaging (Rasova et al., 2015; Tavazzi et al., 2018; Rocca et al., 2019; Boffa et al., 2020; Stellmann et al., 2020).
Studies on Upper Limb Rehabilitation
Five studies described clinical and MRI results after upper limb rehabilitation (Bonzano et al., 2014, 2019; Barghi et al., 2018; Rocca et al., 2019; Boffa et al., 2020). All these studies consistently reported a beneficial clinical effect of motor rehabilitation (Barghi et al., 2018; Rocca et al., 2019; Boffa et al., 2020), although the benefits were not significantly different between the active group and the control group undergoing a passive treatment in two studies (Bonzano et al., 2014, 2019). Moreover, there was an association between improved clinical measures and MRI markers of brain reorganization (Barghi et al., 2018; Rocca et al., 2019; Boffa et al., 2020) although the direct correlation between clinical and MRI parameters was not reported in the studies in which passive treatment was utilized (Bonzano et al., 2014, 2019).
With respect to studies analyzing structural MRI markers, white matter structural changes in response to upper limb rehabilitation were less remarkable than functional rearrangements, being either completely absent and not associated with any clinical or behavioral measures (Rocca et al., 2019; Boffa et al., 2020), or small and confined to the corpus callosum and the cortico-spinal tract (Bonzano et al., 2014; Barghi et al., 2018). In this latter case, one study described also a correlation between improved arm function quantified by the Motor Activity Log and corpus callosum integrity (Barghi et al., 2018).
The only study investigating volumetric gray matter changes showed increased volumes in areas involved in the mirror neuron system (MNS) as a response to action-observation therapy (AOT), which is known to activate mirror neurons (Rocca et al., 2019). Furthermore, the volumetric changes of superior and inferior frontal gyrus were significantly associated with the main clinical measures of strength (Jamar dynamometers), hand dexterity (9-hole peg test), and finger tapping frequency, as well as with cognitive measures of attention and processing speed (Rocca et al., 2019).
Functional changes in response to rehabilitation, on the contrary, are reported in all the studies, with different activation patterns, which largely depended on the specific type of rehabilitation approach: MNS and cerebellum for AOT (Rocca et al., 2019), primary motor area, primary sensorimotor cortex, thalami, and cerebellum for task-oriented therapy (Bonzano et al., 2019; Boffa et al., 2020). Remarkably, in both rs-fMRI and task-related fMRI studies the cerebellum showed increased activation/connectivity with other brain areas. Moreover, Rocca et al. reported significant associations between improved strength and dexterity, measured, respectively, with dynamometers and 9-hole peg test and both functional connectivity as task-related activations of different cortical areas, but mainly of the inferior frontal gyrus (Rocca et al., 2019). Boffa et al. described significant associations between lower interhand interval at spontaneous and maximum velocity and the functional connectivity between primary motor cortex and primary somatosensory cortex, as well as between thalami and inferior frontal gyrus (Boffa et al., 2020).
Studies on Lower Limb Rehabilitation
Six studies focused on lower limb rehabilitation, intended as either aerobic exercise or resistance training (Ibrahim et al., 2011; Kjolhede et al., 2018; Tavazzi et al., 2018; Fling et al., 2019; Stellmann et al., 2020), and in one case balance training (Prosperini et al., 2014).
Clinical improvements, when investigated, were related to motor functions (Tavazzi et al., 2018), standing balance (Prosperini et al., 2014), and cognitive performance (Ibrahim et al., 2011).
Two DTI studies reported improved tissue integrity in structures belonging to the motor pathway, such as the corpus callosum (Ibrahim et al., 2011) and superior cerebellar peduncles (Prosperini et al., 2014), although other relevant regions investigated by Prosperini et al. (2014) (e.g., middle and inferior cerebellar peduncles, corpus callosum, corona radiata) did not show any change. In the latter study, improved balance reflected by reduced postural sway was strongly associated with both increased FA and reduced radial diffusivity of superior cerebellar peduncles (Prosperini et al., 2014).
Improved structural integrity reflected by increased white matter FA, together with within-hub structural integration and organization, was also found in the only connectome-based study, paralleled by increased FC and associated with Expanded Disability Status Scale (EDSS) change (Stellmann et al., 2020). On the contrary, another study reported no significant structural changes investigated by means of Tract-Based Spatial Statistics, as well as no associations with clinical parameters (Tavazzi et al., 2018).
All the rs-fMRI studies investigated exclusively region of interests targeted by the specific type of rehabilitation and reported increased FC within the sensorimotor networks (Tavazzi et al., 2018; Fling et al., 2019). The only study applying a connectome-based approach confirmed and expanded previously reported results, describing increased global FC, which was associated with improved structural organization but not with any clinical measures. In one study, the increased connectivity between supplemental motor area (SMA) and sensorimotor network was paralleled by reduced connectivity with the cerebellum (Fling et al., 2019).
Multimodal Rehabilitation
The last six studies described results from multimodal rehabilitation, intended as exercises focused at improving motor functions of both upper and lower limb, as well as trunk balance (Rasova et al., 2005, 2012, 2015; Akbar et al., 2020; Peran et al., 2020; Prochazkova et al., 2020; Zuber et al., 2020).
The only structural study reported improved tissue integrity represented by increased FA and reduced MD within the corpus callosum, as well as an improvement of several clinical measures, even though the association between clinical and MRI outcomes has not been described.
Task-related fMRI results in multimodal rehabilitation studies are discordant, reporting increased activation of SMA and premotor cortex significantly associated with improved clinical index only in patients clinically improved (Prochazkova et al., 2020), reduced cerebellar and prefrontal activation in the active group paralleling an increased accuracy in the execution of the motor task (Zuber et al., 2020), or no significant changes between active and control group (Rasova et al., 2005, 2012, 2015). Finally, another study showed reduced activation in areas usually involved in the specific task, whereas activation was increased in new cerebral areas. As the efficacy of the rehabilitation intervention was not an aim of the study, clinical measures were not reported (Peran et al., 2020).
One study investigated the effect of progressive resistance training of both upper and lower limbs on both fatigue and caudate connectivity, reporting a significant improvement of the physical and cognitive components of fatigue as well as increased functional connectivity between caudate and several cortical areas (Akbar et al., 2020). Furthermore, the increased functional connectivity between caudate and left inferior parietal lobule was significantly associated with increased physical activity level, and there was a trending correlation between the abovementioned increased FC and a decrease in cognitive fatigue quantified by the modified impact fatigue scale (Akbar et al., 2020).
Quality Ratings
Detailed information about the TESTEX scoring are reported for each item in Table 3.
Eleven out of 17 included studies were RCT and were evaluated with the TESTEX scale (Smart et al., 2015). Three out of 11 were classified as “high quality” studies (12–15 points), while 8 out of 11 were classified as “good quality” studies (7–11 points). Five out of 11 RCT were registered. One study involved only imaging of 14 patients in the experimental group (Fling et al., 2019), while the larger RCT was published in a different manuscript and was registered.
Discussion
Motor rehabilitation in MS has a global beneficial effect both subjectively perceived as reduced fatigue, increased motivation and level of physical activity and objectively quantified by several clinical scales. Considering that MRI is a fully integrated tool in the MS-related diagnostic and monitoring processes, the low number of studies applying MRI to evaluate the effect of rehabilitation with respect to the total amount of studies dedicated to rehabilitation in MS is somewhat surprising. Several studies here reported underline the crucial role of MRI markers in the evaluation of rehabilitation effects, even when clinical markers fail to do so (Bonzano et al., 2014, 2019). However, the scarceness of results, together with their heterogeneity, makes it difficult to clearly define the effects of rehabilitation on brain functional and structural changes. Moreover, the role of neurorehabilitation on brain plasticity is challenging, considering that MS is characterized by chronic, ongoing tissue damage, such that changes are happening in tandem with response to inflammation and neurodegeneration. Overall, most of the studies analyzed in this review show that motor rehabilitation has the potential to favorably impact brain neuroplasticity, although many factors, such as individual disease stage and duration, as well as rehabilitation type and duration, influence the type and degree of cerebral response.
Adaptive vs. Maladaptive Plasticity
Adaptive plasticity has been extensively studied, as the capacity of the brain to structurally and functionally change in response to experiences and environmental stimuli in healthy subjects, as well as in response to tissue injury, with the aim to restore homeostasis (Nava and Roder, 2011). However, changes can also be considered as reflecting so-called maladaptive plasticity, which represents an aberrant modification associated with a poor clinical outcome (Trojan and Pokorny, 1999; Nava and Roder, 2011). Often though, the two mechanisms are closely interrelated and the border between the two phenomena is very narrow. Maladaptive plasticity has been the subject of several studies in motor-related stroke patients (Jang, 2013), where it results in abnormal movement patterns or increased activation of the contralesional motor pathway, thus preventing the recovery of the damaged area. In MS, the distinction between adaptive and maladaptive mechanisms is fraught with challenges in the absence of associated clinical measures, as the underlying tissue damage renders it difficult to interpret changes unequivocally (Laura et al., 2018). Widespread functional brain activation in response to motor or cognitive tasks is generally increased in MS patients with respect to healthy subjects, leading to define it as a compensatory mechanism to preserve a satisfactory clinical status (Tavazzi et al., 2018; Rocca et al., 2019). However, some studies showed that better motor performances were associated with a reduction of diffuse task-related activation after rehabilitation intervention, interpreted as increased synaptic efficiency and recovery of specialized function within the damaged area (Tavazzi et al., 2018; Bonzano et al., 2019; Peran et al., 2020). It must be noted though that other studies reported increased task-related activation and increased FC in association with improved motor functions (Guerrera et al., 2014; Rocca et al., 2019; Akbar et al., 2020; Boffa et al., 2020). This apparent discrepancy confirms that the same plasticity mechanisms can result in opposite clinical outcomes and be considered either adaptive or maladaptive, likely depending on several factors including tissue damage entity and location along with disease stage, among others. It has also to be considered that new sophisticated tools to study global brain connectivity rather than task-related brain activations, such as the connectome-based approach, have shown a very complex hierarchical organization within the brain, consisting of networks and hubs interacting across disparate brain regions. Therefore, a dichotomic distinction of functional rearrangements between adaptive and maladaptive might be overly simplistic in MS, but only future longitudinal studies will clarify this aspect (Schoonheim et al., 2015).
Upper Limb Rehabilitation
Although walking impairment is frequently considered the most disabling aspect of MS, upper limb dysfunction is frequent and strongly impacts quality of life at multiple levels (Johansson et al., 2007; Bertoni et al., 2015). Results from the studies included in the present review show that neuromotor rehabilitation effectively improves upper limb function, although in two studies there was no significant difference between the active and the passive groups, in terms of clinical outcomes (Bonzano et al., 2014, 2019). This might be due to the fact that passive mobilization can be an effective stimulus on the corresponding sensorimotor cortex, or might be related to the choice of clinical measures that are not necessarily sensitive enough to detect a difference between the two groups. This latter hypothesis seems likely, considering that structural MRI markers were stable over time in the active group but worsened in patients undergoing the passive treatment (Bonzano et al., 2014), and functional MRI showed a trend toward brain activity normalization only in the active group (Bonzano et al., 2019).
Studies investigating structural brain reorganization elicited by motor rehabilitation reported very small changes, if any (Bonzano et al., 2014; Barghi et al., 2018; Rocca et al., 2019; Boffa et al., 2020). Even though structural changes have been reported early after training, the short treatment period and the long disease duration in the aforementioned studies might account for their results, related to an exhaustion of compensatory mechanisms in the brain, thereby preventing meaningful structural reorganization (Filippi et al., 2012; Bonzano et al., 2014).
Functional changes, instead, were described in all the studies, involving different areas mostly depend upon the rehabilitation approach used. However, a common finding of these studies was increased cerebellar connectivity with multiple brain areas. The cerebellum is a key structure involved in several cognitive and motor functions and is associated with multiple cortical areas through afferent and efferent cortico-cerebellar pathways (Stoodley and Schmahmann, 2010; Ruggieri et al., 2020). Recently, FC cerebellar changes were reported for both sensorimotor and cognitive compartments as a consequence of MS-related tissue damage, negatively associated with global and regional disability levels (Pasqua et al., 2020). This latter finding supports the adaptive role of cerebellar FC, highlighted also by the abovementioned results in the rehabilitative setting.
Lower Limb Rehabilitation
Motor dysfunctions involving the lower limbs are so frequent and relevant that the clinical scale primarily used to quantify physical disability, namely the Expanded Disability Status Scale (EDSS), weights this aspect above all others, and disability milestones are related to the gradual loss of walking autonomy. Therefore, rehabilitation of lower limbs, which includes treatment of weakness, spasticity and imbalance, is a relevant part of the clinical practice.
Most structural studies reported a positive effect of rehabilitation on motor pathway tissue integrity. An association between structural rearrangements in motor-related areas and improvement of clinical measures reflecting motor functions was also described, leading to interpret these changes as beneficially adaptive (Prosperini et al., 2014; Stellmann et al., 2020). The only study that failed to show rehabilitation-mediated structural changes as well as a significant association structural MRI markers and clinical outcomes, was hampered by a small sample size and a high drop-out rate (Tavazzi et al., 2018).
Functional results are concordantly positive, although the analysis of most studies was limited to regions of interests, known to be activated by the chosen rehabilitation approach. However, the connectome-based approach used in one single study showed a significant association between functional and structural reorganization at a global level (Stellmann et al., 2020).
Interestingly, reduced connectivity between SMA and the cerebellum has been reported (Fling et al., 2019). Although this finding, which is the opposite of what previously reported in upper-limb rehabilitation studies, can be ascribed to different aspects related to the rehabilitation approach or patient characteristics, it can also be hypothesized that upper-limb and lower-limb rehabilitation potentiate different pathways, in which the cerebellum can be variably involved. Overall, the association between structural and functional changes mediated by neuromotor rehabilitation and improved clinical outcomes strongly suggests a positive effect of rehabilitation on the brain.
Multimodal Rehabilitation
As MS is a neurodegenerative disease impacting several multifunctional systems, the consequent disability usually involves all 4 limbs, to a varying degree and mainly in the late stage, as well as the trunk in terms of imbalance and postural lack of control. Some rehabilitative approaches combine exercises for both upper and lower limbs, and so-called multimodal or multidisciplinary rehabilitation includes different types of treatment, such as motor rehabilitation and occupational therapy, with the aim of maintaining everyday life activities. The identification of structural and functional changes occurring in response to these rehabilitative therapies is challenging, due to the multiplicity of brain areas potentially recruited.
Structural brain reorganization in response to multimodal rehabilitation was concordantly reported and associated with improved motor and cognitive performance, suggesting a protective role of rehabilitation in MS-related neurodegeneration (Kjolhede et al., 2018). However, functional data are very heterogeneous both in terms of activation pattern and regions involved.
A general issue with multidisciplinary rehabilitation interventions relates to the fact they are typically tailored to individual patient needs and abilities, and as such vary between different centers and even within subjects of the same group. This aspect, while representing a significant advantage in the clinical practice, becomes a major issue in the research field as it potentially makes it more difficult to identify signals in the data and renders it challenging to compare studies and apply their results in different settings.
Methodological Considerations and Potential Bias
The design and conduction of studies focused on MRI markers of neuroplasticity in rehabilitation settings present several potential issues that are worth discussing (Table 2). In addition, it is important to consider possible sources of bias in the studies that have been reviewed. Although the techniques utilized in provide quantitative measures, it is difficult to directly compare the results from one study to another due to technical factors. For example, scanning platforms and sequence parameters of the image acquisition vary substantially between studies. Furthermore, it is typically the case that all details describing the specific options used in image post-processing are not fully provided in a given manuscript. Importantly, it may also be the case that the authors explored multiple different processing techniques but only chose to present the “best” results, without mentioning that other avenues had been pursued. To mitigate these sources of biases, authors can choose to register a protocol with their analysis plan before the study even starts such that subsequent findings can be directly linked to the original intent. As of now though, most reported studies have not registered a protocol ahead of time, resulting in a considerable risk of bias and thus preventing one from drawing concrete conclusions from individual studies. Of note, the analysis protocol was not published prior to study initiation for any of the reported studies in this review.
Disease Stage
Contrarily to what happens in other neurological conditions such as traumatic brain injury or a first episode of stroke, in which a monophasic event occurs in an otherwise healthy brain, MS is a chronically evolving disease. Therefore, the disease stage, and consequently the balance between the ongoing inflammation and neurodegeneration, is a relevant factor to be considered when analyzing the effect of rehabilitation on brain reorganization. Indeed, inflammation is known to impact neuroplasticity via several mechanisms, interfering with synaptic transmission, neurovascular coupling and signal transmission through non adjacent brain regions (Tomassini et al., 2016). Neurodegeneration is characterized by progressive loss of tissue integrity, with chronic demyelination in both the white and gray matter as well as neuroaxonal damage intrinsically affecting brain plasticity (Ksiazek-Winiarek et al., 2015). Whereas these notions might seem to suggest that late-stage MS patients would not benefit from rehabilitation, it has instead been demonstrated that the brain does not fully exhaust its neuroplastic abilities, even in severely disabled patients (Tomassini et al., 2011, 2012). However, clinical trials evaluating the efficacy of rehabilitation should recruit homogenous populations in terms of disease stage, or at least take into account this factor when interpreting the results. MRI markers of inflammation, such as gadolinium-enhancing lesions or new/enlarging T2-lesions, as well as markers of neurodegeneration, such as volumetric measures quantifying tissue atrophy or DTI parameters, could be relevant information to include, rather than the simple categorization of patients as RRMS or PMS.
Along this line, the presence of disease modifying treatments should also be considered, as it has been showed that a pharmacologically-driven reduction of inflammation parallels restoration of brain plasticity (Tomassini et al., 2016).
Control Group
The choice of an appropriate control group is a critical point when aiming to detect changes at a microstructural level (Thomas and Baker, 2013), as well as functional rearrangements, especially when the subject of the study is a chronic disease affecting both white and gray matter.
Comparing MS patients with healthy subjects undergoing the same rehabilitation intervention is not ideal (Ibrahim et al., 2011), as neuroplastic mechanisms depend on the underlying degree of tissue integrity. Even when recruiting MS patients as control groups, the choice of “placebo” treatment needs to be carefully evaluated. The complete absence of treatment (Rasova et al., 2005; Stellmann et al., 2020), as well as a shorter exposure time to physical activity makes it difficult to interpret whether the potential results could be attributed only to increased level of physical activity or be the consequence of brain reorganization. A control group with MS patients undergoing a different type of training, including passive movements, for the same amount of time, represents the best option (Bonzano et al., 2014, 2019; Barghi et al., 2018; Rocca et al., 2019; Akbar et al., 2020; Boffa et al., 2020; Prochazkova et al., 2020).
Follow-Up
Only 3 studies reported data on follow-up, which was short-term (4 weeks) in 2 of them (Rasova et al., 2015; Zuber et al., 2020), and 3 months in the remaining one (Tavazzi et al., 2018). Whereas the studies with short-term follow-up reported maintenance of functional (Zuber et al., 2020) and structural (Rasova et al., 2015) changes after rehabilitation, patients reverted to the baseline in the study with longer follow-up (Tavazzi et al., 2018). The persistence of clinical, as well as functional and structural improvements obtained with rehabilitation, is a crucial aspect that needs to be carefully investigated, as the main aim is for pwMS to be able to exploit the benefits of the rehabilitation intervention in a real-life setting. Surely several factors play a relevant role, such as the socio-familial support, psychological state, and environment that allows for the maintenance of a physically active lifestyle (Kever et al., 2021). Interestingly though, whereas neuroplastic abilities are preserved throughout the disease course as abovementioned, retention skills might be impaired in pwMS (Nguemeni et al., 2020), explaining the negative results of the longer-term follow-up study. Only studies with longer follow-ups can help better understand whether the clinical and MRI- related achievements obtained with motor rehabilitation are the mere results of increased physical activity or they reflect a more consolidated process of brain reorganization, and how to favor this second possibility.
Other Factors
Some other factors are worth mentioning, as they could hinder rehabilitation-mediated clinical and MRI results or act as confounders.
While investigating the efficacy of motor rehabilitation on brain reorganization, one aspect that should be taken into careful consideration is the overall integrity of the motor pathway. In stroke patients, structural damage of the pyramidal tract, including both neurons belonging to motor areas and axons of the corticospinal tract, considerably influence the type and extent of functional rearrangements after rehabilitation (Hamzei et al., 2006, 2008). In pwMS with moderate-to-severe disability, the likelihood of motor pathway damage is high. Nonetheless to characterize its extent might be useful when trying to explain different types of functional rearrangements, and interpreting structural data (Naismith et al., 2013; Oh et al., 2013; Hubbard et al., 2016).
Fatigue was quantified in 7 out of 17 studies (Rasova et al., 2005; Tavazzi et al., 2018; Akbar et al., 2020; Boffa et al., 2020; Peran et al., 2020; Stellmann et al., 2020; Zuber et al., 2020), and is another element that should be assessed in patients undergoing neuromotor rehabilitation. Fatigue is one of the most common and disabling symptoms affecting pwMS, negatively impacting quality of life and disease evolution (Eizaguirre et al., 2020; Vaughn et al., 2020), as well as reducing overall physical activity. Fatigue has a neuroimaging correlate, being associated with altered FC within the sensorimotor networks, and hyperactivation of different brain regions, such as the primary motor area (Bertoli and Tecchio, 2020). As such, fatigue could interfere with both clinical outcomes and neuroplastic changes mediated by rehabilitation. In the past, pwMS were advised to abstain from exercise to reduce symptoms as fatigue, but it has been demonstrated that, on the contrary, fatigue improves together with endurance in pwMS undergoing regular exercise (Stephens et al., 2019), or rehabilitation. Therefore, fatigue should be regularly assessed in MRI studies testing rehabilitation efficacy, as it could be both a marker of rehabilitation efficacy and a factor that needs to be considered when interpreting clinical and MRI results. A better understanding of the role of fatigue and its functional MRI correlate would lead to the inclusion of rehabilitation strategies specifically aimed at reducing its impact on quality of life in pwMS (Dobryakova et al., 2018; Akbar et al., 2020).
Future MRI-Rehabilitation Studies
Physical activity is now recommended as a strategy capable of impacting disability accrual in pwMS (Lozinski and Yong, 2020), but some studies described an effect of exercise also on cognition, mediated by neuroplasticity, proving a broad effect of physical exercise that goes beyond clinical motor outcomes and motor-related areas in the brain (Leavitt et al., 2014; Sandroff et al., 2017, 2018). In particular, enriched environmental stimuli result in greater neuroplastic abilities, as evidenced by experimental and human studies (Carey et al., 2005; You et al., 2005; Lambert et al., 2016; Saleh et al., 2017; Wu et al., 2019). Therefore, rehabilitation strategies exploiting virtual reality, robotic devices, and/or dual task approaches need to be further studied and subsequently implemented in clinical practice.
With respect to imaging techniques applied in the study of rehabilitation effects on brain reorganization, tools that simultaneously analyze structural and functional rearrangements of multiple brain areas, based on the hierarchical organization of brain networks, should be favored (Stellmann et al., 2020).
Finally, the wider availability of technological devices makes telerehabilitation an option that needs to be considered, at least complementing therapist-supervised treatments, as it could more easily reach pwMS with limited access to rehabilitation centers in addition to lowering overall MS-related economic burden. Few studies have been done in this context, but none have applied MRI markers to test the intervention efficacy, and the derived evidence is not robust enough to draw any conclusions (Khan et al., 2015). However, it has emerged as a need for pwMS even with mild disability (Remy et al., 2020), and the potential usefulness in more severely disabled patients highlights the need for further studies applying telerehabilitation and including MRI outcomes.
Summary and Conclusion
MS is a chronic, long-lasting disease affecting mainly young people in whom the maintenance of ambulatory self-sufficiency is crucial for psychological, relational, socio-economic and work-related reasons.
In this complex disease, the individual recovering abilities, in terms of both clinical disability and neuroplasticity, derive from several factors such as ongoing pathogenic mechanisms, disability level, cognitive impairment, as well as socio-economic and familial conditions. Neuromotor rehabilitation is a crucial aspect among all the interventions aimed at preserving quality of life and reducing MS-related GBD. However, studies investigating the effect of rehabilitation on brain reorganization are few and the validity of their results is hampered by the small sample size, potential bias in lack of protocol registration, and the extreme data heterogeneity in terms of patient characteristics, rehabilitation settings, MRI protocol acquisition and post-processing. Conversely, a better standardization of rehabilitation interventions is needed, to facilitate the identification of the appropriate rehabilitation approach for individual patient needs and abilities, and the generalization of results. In this context, MRI markers of structural and functional connectivity represent an important part of this assessment process and should be implemented in the clinical routine as well as in research studies investigating the effect of rehabilitation interventions. Indeed, they provide quantitative, reproducible information on neuroplastic changes underlying clinical achievements, furthering the community's knowledge on the role of rehabilitation modifying disease evolution, and contributing to tailored rehabilitation strategies at an individual level.
Finally, considering that an unequivocal interpretation of structural and functional findings is difficult and further complicated by the ongoing pathological mechanisms of a chronically evolving disease such as MS, associations between MRI markers and clinical/behavioral data should always be presented. This is fundamental for characterizing changes in terms of adaptive or maladaptive responses and facilitates the assessment of success for a given rehabilitative treatment.
Data Availability Statement
The original contributions presented in the study are included in the article/supplementary material, further inquiries can be directed to the corresponding author/s.
Author Contributions
ET: literature search, data interpretation, and scientific writing. MC: literature search, tables preparation, and scientific writing. AP: literature search and figure preparation. VB: data interpretation and analysis. MR: scientific writing and critical data analysis. NB: literature search, figure preparation, and scientific writing. FB: scientific supervision. All authors contributed to the article and approved the submitted version.
Funding
This study was funded by the Italian Ministry of Health (Ricerca Corrente Reti Program- Neurosciences and Neurorehabilitation Network). ET received a scholarship from Crespi Spano Foundation.
Conflict of Interest
The authors declare that the research was conducted in the absence of any commercial or financial relationships that could be construed as a potential conflict of interest.
Publisher's Note
All claims expressed in this article are solely those of the authors and do not necessarily represent those of their affiliated organizations, or those of the publisher, the editors and the reviewers. Any product that may be evaluated in this article, or claim that may be made by its manufacturer, is not guaranteed or endorsed by the publisher.
Acknowledgments
We acknowledge Fondazione Crespi-Spanò for the financial support to ET.
References
Akbar, N., Sandroff, B. M., Wylie, G. R., Strober, L. B., Smith, A., Goverover, Y., et al. (2020). Progressive resistance exercise training and changes in resting-state functional connectivity of the caudate in persons with multiple sclerosis and severe fatigue: a proof-of-concept study. Neuropsychol. Rehabil. 30, 54–66. doi: 10.1080/09602011.2018.1449758
Barghi, A., Allendorfer, J. B., Taub, E., Womble, B., Hicks, J. M., Uswatte, G., et al. (2018). Phase II randomized controlled trial of constraint-induced movement therapy in multiple sclerosis. Part 2: effect on white matter integrity. Neurorehabil. Neural Repair 32, 233–241. doi: 10.1177/1545968317753073
Batalik, L., Winnige, P., Dosbaba, F., Vlazna, D., and Janikova, A. (2021). Home-Based aerobic and resistance exercise interventions in cancer patients and survivors: a systematic review. Cancers 13:1951. doi: 10.3390/cancers13081915
Bengtsson, S. L., Nagy, Z., Skare, S., Forsman, L., Forssberg, H., and Ullen, F. (2005). Extensive piano practicing has regionally specific effects on white matter development. Nat. Neurosci. 8, 1148–1150. doi: 10.1038/nn1516
Bertoli, M., and Tecchio, F. (2020). Fatigue in multiple sclerosis: does the functional or structural damage prevail? Mult. Scler. 26, 1809–1815. doi: 10.1177/1352458520912175
Bertoni, R., Lamers, I., Chen, C. C., Feys, P., and Cattaneo, D. (2015). Unilateral and bilateral upper limb dysfunction at body functions, activity and participation levels in people with multiple sclerosis. Mult. Scler. 21, 1566–1574. doi: 10.1177/1352458514567553
Boffa, G., Tacchino, A., Sbragia, E., Schiavi, S., Droby, A., Piaggio, N., et al. (2020). Preserved brain functional plasticity after upper limb task-oriented rehabilitation in progressive multiple sclerosis. Eur. J. Neurol. 27, 77–84. doi: 10.1111/ene.14059
Bonzano, L., Pedulla, L., Tacchino, A., Brichetto, G., Battaglia, M. A., Mancardi, G. L., et al. (2019). Upper limb motor training based on task-oriented exercises induces functional brain reorganization in patients with multiple sclerosis. Neuroscience 410, 150–159. doi: 10.1016/j.neuroscience.2019.05.004
Bonzano, L., Tacchino, A., Brichetto, G., Roccatagliata, L., Dessypris, A., Feraco, P., et al. (2014). Upper limb motor rehabilitation impacts white matter microstructure in multiple sclerosis. Neuroimage 90, 107–116. doi: 10.1016/j.neuroimage.2013.12.025
Carey, J. R., Bhatt, E., and Nagpal, A. (2005). Neuroplasticity promoted by task complexity. Exerc. Sport Sci. Rev. 33, 24–31.
Comi, G., Radaelli, M., and Soelberg Sorensen, P. (2017). Evolving concepts in the treatment of relapsing multiple sclerosis. Lancet 389, 1347–1356. doi: 10.1016/S0140-6736(16)32388-1
Dalgas, U., Stenager, E., Jakobsen, J., Petersen, T., Hansen, H. J., Knudsen, C., et al. (2009). Resistance training improves muscle strength and functional capacity in multiple sclerosis. Neurology 73, 1478–1484. doi: 10.1212/WNL.0b013e3181bf98b4
Dobryakova, E., Hulst, H. E., Spirou, A., Chiaravalloti, N. D., Genova, H. M., Wylie, G. R., et al. (2018). Fronto-striatal network activation leads to less fatigue in multiple sclerosis. Mult. Scler. 24, 1174–1182. doi: 10.1177/1352458517717087
Draganski, B., Gaser, C., Busch, V., Schuierer, G., Bogdahn, U., and May, A. (2004). Neuroplasticity: changes in grey matter induced by training. Nature 427, 311–312. doi: 10.1038/427311a
Eizaguirre, M. B., Ciufia, N., Roman, M. S., Martinez Canyazo, C., Alonso, R., Silva, B., et al. (2020). Perceived fatigue in multiple sclerosis: the importance of highlighting its impact on quality of life, social network and cognition. Clin. Neurol. Neurosurg. 199:106265. doi: 10.1016/j.clineuro.2020.106265
El-Sayes, J., Harasym, D., Turco, C. V., Locke, M. B., and Nelson, A. J. (2019). Exercise-Induced neuroplasticity: a mechanistic model and prospects for promoting plasticity. Neuroscientist 25, 65–85. doi: 10.1177/1073858418771538
Filippi, M., Riccitelli, G., Mattioli, F., Capra, R., Stampatori, C., Pagani, E., et al. (2012). Multiple sclerosis: effects of cognitive rehabilitation on structural and functional MR imaging measures–an explorative study. Radiology 262, 932–940. doi: 10.1148/radiol.11111299
Fling, B. W., Martini, D. N., Zeeboer, E., Hildebrand, A., and Cameron, M. (2019). Neuroplasticity of the sensorimotor neural network associated with walking aid training in people with multiple sclerosis. Mult. Scler. Relat. Disord. 31, 1–4. doi: 10.1016/j.msard.2019.03.004
Garthe, A., Roeder, I., and Kempermann, G. (2016). Mice in an enriched environment learn more flexibly because of adult hippocampal neurogenesis. Hippocampus 26, 261–271. doi: 10.1002/hipo.22520
GBD 2016 Multiple Sclerosis Collaborators (2019). Global, regional, and national burden of multiple sclerosis 1990-2016: a systematic analysis for the global burden of disease study 2016. Lancet Neurol. 18, 269–285. doi: 10.1016/S1474-4422(18)30443-5
GBD 2016 Neurology Collaborators (2019). Global, regional, and national burden of neurological disorders, 1990-2016: a systematic analysis for the global burden of disease study 2016. Lancet Neurol. 18, 459–480. doi: 10.1016/S1474-4422(18)30499-X
Goodin, D. S. (2014). The epidemiology of multiple sclerosis: insights to disease pathogenesis. Handb. Clin. Neurol. 122, 231–266. doi: 10.1016/B978-0-444-52001-2.00010-8
Guerrera, S., Morabito, R., Baglieri, A., Corallo, F., Ciurleo, R., De Luca, R., et al. (2014). Cortical reorganization in multiple sclerosis after intrathecal baclofen therapy. Neurocase 20, 225–229. doi: 10.1080/13554794.2013.770872
Guo, L. Y., Lozinski, B., and Yong, V. W. (2020). Exercise in multiple sclerosis and its models: focus on the central nervous system outcomes. J. Neurosci. Res. 98, 509–523. doi: 10.1002/jnr.24524
Hamzei, F., Dettmers, C., Rijntjes, M., and Weiller, C. (2008). The effect of cortico-spinal tract damage on primary sensorimotor cortex activation after rehabilitation therapy. Exp. Brain Res. 190, 329–336. doi: 10.1007/s00221-008-1474-x
Hamzei, F., Liepert, J., Dettmers, C., Weiller, C., and Rijntjes, M. (2006). Two different reorganization patterns after rehabilitative therapy: an exploratory study with fMRI and TMS. Neuroimage 31, 710–720. doi: 10.1016/j.neuroimage.2005.12.035
Hubbard, E. A., Wetter, N. C., Sutton, B. P., Pilutti, L. A., and Motl, R. W. (2016). Diffusion tensor imaging of the corticospinal tract and walking performance in multiple sclerosis. J. Neurol. Sci. 363, 225–231. doi: 10.1016/j.jns.2016.02.044
Ibrahim, I., Tintera, J., Skoch, A., Jiru, F., Hlustik, P., Martinkova, P., et al. (2011). Fractional anisotropy and mean diffusivity in the corpus callosum of patients with multiple sclerosis: the effect of physiotherapy. Neuroradiology 53, 917–926. doi: 10.1007/s00234-011-0879-6
Jang, S. H. (2013). Motor function-related maladaptive plasticity in stroke: a review. NeuroRehabilitation 32, 311–316. doi: 10.3233/NRE-130849
Johansson, S., Ytterberg, C., Claesson, I. M., Lindberg, J., Hillert, J., Andersson, M., et al. (2007). High concurrent presence of disability in multiple sclerosis. Associations with perceived health. J. Neurol. 254, 767–773. doi: 10.1007/s00415-006-0431-5
Kaufmann, M., Puhan, M. A., Salmen, A., Kamm, C. P., Manjaly, Z. M., Calabrese, P., et al. (2020). 60/30: 60% of the morbidity-associated multiple sclerosis disease burden comes from the 30% of persons with higher impairments. Front. Neurol. 11:156. doi: 10.3389/fneur.2020.00156
Kever, A., Buyukturkoglu, K., Riley, C. S., De Jager, P. L., and Leavitt, V. M. (2021). Social support is linked to mental health, quality of life, and motor function in multiple sclerosis. J. Neurol. 268, 1827–1836. doi: 10.1007/s00415-020-10330-7
Khan, F., Amatya, B., Kesselring, J., and Galea, M. (2015). Telerehabilitation for persons with multiple sclerosis. Cochrane Database Syst Rev. 2015:CD010508. doi: 10.1002/14651858.CD010508.pub2
Kjolhede, T., Siemonsen, S., Wenzel, D., Stellmann, J. P., Ringgaard, S., Pedersen, B. G., et al. (2018). Can resistance training impact MRI outcomes in relapsing-remitting multiple sclerosis? Mult. Scler. 24, 1356–1365. doi: 10.1177/1352458517722645
Koudriavtseva, T., and Mainero, C. (2016). Neuroinflammation, neurodegeneration and regeneration in multiple sclerosis: intercorrelated manifestations of the immune response. Neural Regen. Res. 11, 1727–1730. doi: 10.4103/1673-5374.194804
Ksiazek-Winiarek, D. J., Szpakowski, P., and Glabinski, A. (2015). Neural plasticity in multiple sclerosis: the functional and molecular background. Neural Plast. 2015:307175. doi: 10.1155/2015/307175
Lambert, K., Eisch, A. J., Galea, L. A. M., Kempermann, G., and Merzenich, M. (2019). Optimizing brain performance: identifying mechanisms of adaptive neurobiological plasticity. Neurosci. Biobehav. Rev. 105, 60–71. doi: 10.1016/j.neubiorev.2019.06.033
Lambert, K., Hyer, M., Bardi, M., Rzucidlo, A., Scott, S., Terhune-Cotter, B., et al. (2016). Natural-enriched environments lead to enhanced environmental engagement and altered neurobiological resilience. Neuroscience 330, 386–394. doi: 10.1016/j.neuroscience.2016.05.037
Latimer-Cheung, A. E., Martin Ginis, K. A., Hicks, A. L., Motl, R. W., Pilutti, L. A., Duggan, M., et al. (2013). Development of evidence-informed physical activity guidelines for adults with multiple sclerosis. Arch. Phys. Med. Rehabil. 94, 1829–1836.e7. doi: 10.1016/j.apmr.2013.05.015
Laura, G., Silvia, T., Nikolaos, P., and Patrizia, P. (2018). The role of fMRI in the assessment of neuroplasticity in MS: a systematic review. Neural Plast. 2018:3419871. doi: 10.1155/2018/3419871
Leavitt, V. M., Cirnigliaro, C., Cohen, A., Farag, A., Brooks, M., Wecht, J. M., et al. (2014). Aerobic exercise increases hippocampal volume and improves memory in multiple sclerosis: preliminary findings. Neurocase 20, 695–697. doi: 10.1080/13554794.2013.841951
Lozinski, B. M., and Yong, V. W. (2020). Exercise and the brain in multiple sclerosis. Mult. Scler. 1352458520969099. doi: 10.1177/1352458520969099. [Epub ahead of print].
Maguire, E. A., Gadian, D. G., Johnsrude, I. S., Good, C. D., Ashburner, J., Frackowiak, R. S., et al. (2000). Navigation-related structural change in the hippocampi of taxi drivers. Proc. Natl. Acad. Sci. U.S.A. 97, 4398–4403. doi: 10.1073/pnas.070039597
Motl, R. W., Pilutti, L. A., Hubbard, E. A., Wetter, N. C., Sosnoff, J. J., and Sutton, B. P. (2015). Cardiorespiratory fitness and its association with thalamic, hippocampal, and basal ganglia volumes in multiple sclerosis. Neuroimage Clin. 7, 661–666. doi: 10.1016/j.nicl.2015.02.017
Naismith, R. T., Xu, J., Klawiter, E. C., Lancia, S., Tutlam, N. T., Wagner, J. M., et al. (2013). Spinal cord tract diffusion tensor imaging reveals disability substrate in demyelinating disease. Neurology 80, 2201–2209. doi: 10.1212/WNL.0b013e318296e8f1
Nava, E., and Roder, B. (2011). Adaptation and maladaptation insights from brain plasticity. Prog. Brain Res. 191, 177–194. doi: 10.1016/B978-0-444-53752-2.00005-9
Nguemeni, C., Nakchbandi, L., Homola, G., and Zeller, D. (2020). Impaired consolidation of visuomotor adaptation in patients with multiple sclerosis. Eur. J. Neurol. 28, 884–892. doi: 10.1111/ene.14599
Oh, J., Saidha, S., Chen, M., Smith, S. A., Prince, J., Jones, C., et al. (2013). Spinal cord quantitative MRI discriminates between disability levels in multiple sclerosis. Neurology 80, 540–547. doi: 10.1212/WNL.0b013e31828154c5
Page, M. J., McKenzie, J. E., Bossuyt, P. M., Boutron, I., Hoffmann, T. C., Mulrow, C. D., et al. (2021). The PRISMA 2020 statement: an updated guideline for reporting systematic reviews. Int. J. Surg. 88:105906. doi: 10.1016/j.ijsu.2021.105906
Pasqua, G., Tommasin, S., Bharti, K., Ruggieri, S., Petsas, N., Piervincenzi, C., et al. (2020). Resting-state functional connectivity of anterior and posterior cerebellar lobes is altered in multiple sclerosis. Mult. Scler. 27, 539–548. doi: 10.1177/1352458520922770
Peran, P., Nemmi, F., Dutilleul, C., Finamore, L., Falletta Caravasso, C., Troisi, E., et al. (2020). Neuroplasticity and brain reorganization associated with positive outcomes of multidisciplinary rehabilitation in progressive multiple sclerosis: a fMRI study. Mult. Scler. Relat. Disord. 42:102127. doi: 10.1016/j.msard.2020.102127
Plautz, E. J., Milliken, G. W., and Nudo, R. J. (2000). Effects of repetitive motor training on movement representations in adult squirrel monkeys: role of use versus learning. Neurobiol. Learn. Mem. 74, 27–55. doi: 10.1006/nlme.1999.3934
Prakash, R. S., Patterson, B., Janssen, A., Abduljalil, A., and Boster, A. (2011). Physical activity associated with increased resting-state functional connectivity in multiple sclerosis. J. Int. Neuropsychol. Soc. 17, 986–997. doi: 10.1017/S1355617711001093
Prakash, R. S., Snook, E. M., Erickson, K. I., Colcombe, S. J., Voss, M. W., Motl, R. W., et al. (2007). Cardiorespiratory fitness: a predictor of cortical plasticity in multiple sclerosis. Neuroimage 34, 1238–1244. doi: 10.1016/j.neuroimage.2006.10.003
Prakash, R. S., Snook, E. M., Motl, R. W., and Kramer, A. F. (2010). Aerobic fitness is associated with gray matter volume and white matter integrity in multiple sclerosis. Brain Res. 1341, 41–51. doi: 10.1016/j.brainres.2009.06.063
Prochazkova, M., Tintera, J., Spanhelova, S., Prokopiusova, T., Rydlo, J., Pavlikova, M., et al. (2020). Brain activity changes following neuroproprioceptive “facilitation, inhibition” physiotherapy in multiple sclerosis: a parallel group randomized comparison of two approaches. Eur. J. Phys. Rehabil. Med. 57, 356–365. doi: 10.23736/S1973-9087.20.06336-4
Prosperini, L., Fanelli, F., Petsas, N., Sbardella, E., Tona, F., Raz, E., et al. (2014). Multiple sclerosis: changes in microarchitecture of white matter tracts after training with a video game balance board. Radiology 273, 529–538. doi: 10.1148/radiol.14140168
Rasova, K., Dolezil, D., Kalistova, H., Kucera, P., Juzova, O., Zimova, D., et al. (2012). Physiotherapy as an immunoactive therapy? A pilot study. Neuro Endocrinol. Lett. 33, 67–75.
Rasova, K., Krasensky, J., Havrdova, E., Obenberger, J., Seidel, Z., Dolezal, O., et al. (2005). Is it possible to actively and purposely make use of plasticity and adaptability in the neurorehabilitation treatment of multiple sclerosis patients? A pilot project. Clin. Rehabil. 19, 170–181. doi: 10.1191/0269215505cr831oa
Rasova, K., Prochazkova, M., Tintera, J., Ibrahim, I., Zimova, D., and Stetkarova, I. (2015). Motor programme activating therapy influences adaptive brain functions in multiple sclerosis: clinical and MRI study. Int. J. Rehabil. Res. 38, 49–54. doi: 10.1097/MRR.0000000000000090
Remy, C., Valet, M., Stoquart, G., El Sankari, S., Van Pesch, V., De Haan, A., et al. (2020). Telecommunication and rehabilitation for patients with multiple sclerosis: access and willingness to use. A cross-sectional study. Eur. J. Phys. Rehabil. Med. 56, 403–411. doi: 10.23736/S1973-9087.20.06061-X
Rocca, M. A., Meani, A., Fumagalli, S., Pagani, E., Gatti, R., Martinelli-Boneschi, F., et al. (2019). Functional and structural plasticity following action observation training in multiple sclerosis. Mult. Scler. 25, 1472–1487. doi: 10.1177/1352458518792771
Rossi, S., Furlan, R., De Chiara, V., Musella, A., Lo Giudice, T., Mataluni, G., et al. (2009). Exercise attenuates the clinical, synaptic and dendritic abnormalities of experimental autoimmune encephalomyelitis. Neurobiol. Dis. 36, 51–59. doi: 10.1016/j.nbd.2009.06.013
Ruggieri, S., Bharti, K., Prosperini, L., Gianni, C., Petsas, N., Tommasin, S., et al. (2020). A comprehensive approach to disentangle the effect of cerebellar damage on physical disability in multiple sclerosis. Front. Neurol. 11:529. doi: 10.3389/fneur.2020.00529
Saleh, S., Fluet, G., Qiu, Q., Merians, A., Adamovich, S. V., and Tunik, E. (2017). Neural patterns of reorganization after intensive robot-assisted virtual reality therapy and repetitive task practice in patients with chronic stroke. Front. Neurol. 8:452. doi: 10.3389/fneur.2017.00452
Sandroff, B. M., Johnson, C. L., and Motl, R. W. (2017). Exercise training effects on memory and hippocampal viscoelasticity in multiple sclerosis: a novel application of magnetic resonance elastography. Neuroradiology 59, 61–67. doi: 10.1007/s00234-016-1767-x
Sandroff, B. M., Wylie, G. R., Sutton, B. P., Johnson, C. L., DeLuca, J., and Motl, R. W. (2018). Treadmill walking exercise training and brain function in multiple sclerosis: preliminary evidence setting the stage for a network-based approach to rehabilitation. Mult. Scler. J. Exp. Transl. Clin. 4:2055217318760641. doi: 10.1177/2055217318760641
Schoonheim, M. M., Meijer, K. A., and Geurts, J. J. (2015). Network collapse and cognitive impairment in multiple sclerosis. Front. Neurol. 6:82. doi: 10.3389/fneur.2015.00082
Smart, N. A., Waldron, M., Ismail, H., Giallauria, F., Vigorito, C., Cornelissen, V., et al. (2015). Validation of a new tool for the assessment of study quality and reporting in exercise training studies: TESTEX. Int. J. Evid. Based Healthc. 13, 9–18. doi: 10.1097/XEB.0000000000000020
Stellmann, J. P., Maarouf, A., Schulz, K. H., Baquet, L., Pottgen, J., Patra, S., et al. (2020). Aerobic exercise induces functional and structural reorganization of CNS networks in multiple sclerosis: a randomized controlled trial. Front. Hum. Neurosci. 14:255. doi: 10.3389/fnhum.2020.00255
Stephens, S., Shams, S., Lee, J., Grover, S. A., Longoni, G., Berenbaum, T., et al. (2019). Benefits of physical activity for depression and fatigue in multiple sclerosis: a longitudinal analysis. J. Pediatr. 209, 226–232.e2. doi: 10.1016/j.jpeds.2019.01.040
Stoodley, C. J., and Schmahmann, J. D. (2010). Evidence for topographic organization in the cerebellum of motor control versus cognitive and affective processing. Cortex 46, 831–844. doi: 10.1016/j.cortex.2009.11.008
Tahedl, M., Levine, S. M., Greenlee, M. W., Weissert, R., and Schwarzbach, J. V. (2018). Functional connectivity in multiple sclerosis: recent findings and future directions. Front. Neurol. 9:828. doi: 10.3389/fneur.2018.00828
Tavazzi, E., Bergsland, N., Cattaneo, D., Gervasoni, E., Lagana, M. M., Dipasquale, O., et al. (2018). Effects of motor rehabilitation on mobility and brain plasticity in multiple sclerosis: a structural and functional MRI study. J. Neurol. 265, 1393–1401. doi: 10.1007/s00415-018-8859-y
Tavazzi, E., Zivadinov, R., Dwyer, M. G., Jakimovski, D., Singhal, T., Weinstock-Guttman, B., et al. (2020). MRI biomarkers of disease progression and conversion to secondary-progressive multiple sclerosis. Expert Rev. Neurother. 20, 821–834. doi: 10.1080/14737175.2020.1757435
Thomas, C., and Baker, C. I. (2013). Teaching an adult brain new tricks: a critical review of evidence for training-dependent structural plasticity in humans. Neuroimage 73, 225–236. doi: 10.1016/j.neuroimage.2012.03.069
Tomassini, V., d'Ambrosio, A., Petsas, N., Wise, R. G., Sbardella, E., Allen, M., et al. (2016). The effect of inflammation and its reduction on brain plasticity in multiple sclerosis: MRI evidence. Hum. Brain Mapp. 37, 2431–2445. doi: 10.1002/hbm.23184
Tomassini, V., Johansen-Berg, H., Jbabdi, S., Wise, R. G., Pozzilli, C., Palace, J., et al. (2012). Relating brain damage to brain plasticity in patients with multiple sclerosis. Neurorehabil. Neural Repair 26, 581–593. doi: 10.1177/1545968311433208
Tomassini, V., Johansen-Berg, H., Leonardi, L., Paixao, L., Jbabdi, S., Palace, J., et al. (2011). Preservation of motor skill learning in patients with multiple sclerosis. Mult. Scler. 17, 103–115. doi: 10.1177/1352458510381257
Trojan, S., and Pokorny, J. (1999). Theoretical aspects of neuroplasticity. Physiol. Res. 48, 87–97. doi: 10.1007/BF03229822
Vaughn, C. B., Kavak, K. S., Dwyer, M. G., Bushra, A., Nadeem, M., Cookfair, D. L., et al. (2020). Fatigue at enrollment predicts EDSS worsening in the New York state multiple sclerosis consortium. Mult. Scler. 26, 99–108. doi: 10.1177/1352458518816619
Wu, Q., Yue, Z., Ge, Y., Ma, D., Yin, H., Zhao, H., et al. (2019). Brain functional networks study of subacute stroke patients with upper limb dysfunction after comprehensive rehabilitation including BCI training. Front. Neurol. 10:1419. doi: 10.3389/fneur.2019.01419
You, S. H., Jang, S. H., Kim, Y. H., Hallett, M., Ahn, S. H., Kwon, Y. H., et al. (2005). Virtual reality-induced cortical reorganization and associated locomotor recovery in chronic stroke: an experimenter-blind randomized study. Stroke 36, 1166–1171. doi: 10.1161/01.STR.0000162715.43417.91
Zatorre, R. J., Fields, R. D., and Johansen-Berg, H. (2012). Plasticity in gray and white: neuroimaging changes in brain structure during learning. Nat. Neurosci. 15, 528–536. doi: 10.1038/nn.3045
Keywords: multiple sclerosis, rehabilitation, neuroplastic changes, DTI (diffusion tensor imaging), fMRI
Citation: Tavazzi E, Cazzoli M, Pirastru A, Blasi V, Rovaris M, Bergsland N and Baglio F (2021) Neuroplasticity and Motor Rehabilitation in Multiple Sclerosis: A Systematic Review on MRI Markers of Functional and Structural Changes. Front. Neurosci. 15:707675. doi: 10.3389/fnins.2021.707675
Received: 10 May 2021; Accepted: 03 September 2021;
Published: 06 October 2021.
Edited by:
Xin Di, New Jersey Institute of Technology, United StatesReviewed by:
Kiran Karunakaran, Kessler Foundation, United StatesChristopher Tench, University of Nottingham, United Kingdom
Copyright © 2021 Tavazzi, Cazzoli, Pirastru, Blasi, Rovaris, Bergsland and Baglio. This is an open-access article distributed under the terms of the Creative Commons Attribution License (CC BY). The use, distribution or reproduction in other forums is permitted, provided the original author(s) and the copyright owner(s) are credited and that the original publication in this journal is cited, in accordance with accepted academic practice. No use, distribution or reproduction is permitted which does not comply with these terms.
*Correspondence: Niels Bergsland, nbergsland@dongnocchi.it
†These authors share senior authorship