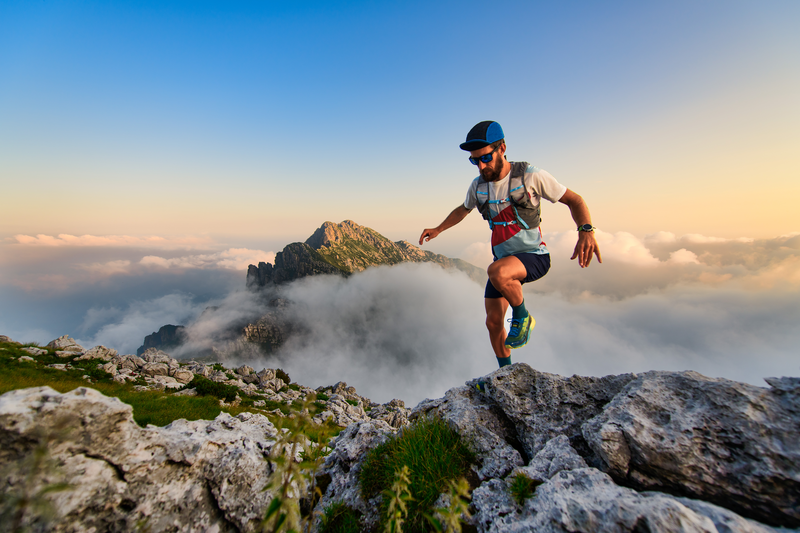
94% of researchers rate our articles as excellent or good
Learn more about the work of our research integrity team to safeguard the quality of each article we publish.
Find out more
REVIEW article
Front. Neurosci. , 02 August 2021
Sec. Perception Science
Volume 15 - 2021 | https://doi.org/10.3389/fnins.2021.695670
This article is part of the Research Topic Neurobiological Mechanism of Acupuncture for Pain and Itch View all 25 articles
Inflammatory pain is caused by peripheral tissue injury and inflammation. Inflammation leads to peripheral sensitization, which may further cause central sensitization, resulting in chronic pain and progressive functional disability. Neuroimmune crosstalk plays an essential role in the development and maintenance of inflammatory pain. Studies in recent years have shown that acupuncture can exert anti-inflammatory and analgesic effects by regulating peripheral (i.e., involving local acupoints and inflamed regions) and central neuroimmune interactions. At the local acupoints, acupuncture can activate the TRPV1 and TRPV2 channels of mast cells, thereby promoting degranulation and the release of histamine, adenosine, and other immune mediators, which interact with receptors on nerve endings and initiate neuroimmune regulation. At sites of inflammation, acupuncture enables the recruitment of immune cells, causing the release of opioid peptides, while also exerting direct analgesic effects via nerve endings. Furthermore, acupuncture promotes the balance of immune cells and regulates the release of inflammatory factors, thereby reducing the stimulation of nociceptive receptors in peripheral organs. Acupuncture also alleviates peripheral neurogenic inflammation by inhibiting the release of substance P (SP) and calcitonin gene-related peptide from the dorsal root ganglia. At the central nervous system level, acupuncture inhibits the crosstalk between glial cells and neurons by inhibiting the p38 MAPK, ERK, and JNK signaling pathways and regulating the release of inflammatory mediators. It also reduces the excitability of the pain pathway by reducing the release of excitatory neurotransmitters and promoting the release of inhibitory neurotransmitters from neurons and glial cells. In conclusion, the regulation of neuroimmune crosstalk at the peripheral and central levels mediates the anti-inflammatory and analgesic effects of acupuncture on inflammatory pain in an integrated manner. These findings provide novel insights enabling the clinical application of acupuncture in the treatment of inflammatory diseases.
Acupuncture, a complementary and alternative therapy, is widely used around the world and has been proven to provide substantial pain relief (Qiao et al., 2020). Of the patients receiving acupuncture treatment for pain relief, 41% have diseases involving inflammatory pain (Wang et al., 2018). Currently, the World Health Organization recommends acupuncture for the treatment of 16 inflammatory pain-related diseases, including rheumatoid arthritis (RA), acute gastritis, chronic gastritis, frozen shoulder, and allergic rhinitis (WHO, 2013). Evidence shows that acupuncture can be a low-cost, low-risk therapy with few and minor adverse effects and it can help in the management of diseases involving inflammatory pain and reduce reliance on analgesics, including morphine (Qin et al., 2016; Wu et al., 2018; A-Wang et al., 2020; Zhang et al., 2020a). In patients with knee osteoarthritis (KOA), acupuncture can significantly relieve pain, attenuate the decline in physical function, and improve quality of life (Corbett et al., 2013). Further, acupuncture appears to be more effective and safe than conventional drug therapy in treating the abdominal pain symptoms associated with ulcerative colitis (UC; A-Wang et al., 2020; Cheng et al., 2020).
Tissue damage or infection can induce an inflammatory response and promote the release of inflammatory mediators from immune cells. These inflammatory mediators activate peripheral nociceptors and nociceptive neurons in the dorsal root ganglion (DRG), causing them to release large amounts of substance P (SP) and calcitonin-gene-related peptide (CGRP; Ronchetti et al., 2017). The release of these signaling molecules aggravates the inflammatory response, mediates peripheral sensitization, and transmits pain signals to the spinal dorsal horn (SDH) via the DRG (Pinho-Ribeiro et al., 2017). Centrally, the stimulation of primary afferent neurons causes the release of inflammatory mediators, which in turn activate central neurons and glial cells. These cells interact with each other, enhancing neuronal function and the circuits involved in pain sensation, and also mediating central sensitization (Muley et al., 2016). Therefore, neuroimmune interactions are crucial for the induction and maintenance of inflammatory pain. Previous studies on the mechanism via which acupuncture relieves inflammatory pain have largely focused on analgesic substances, even though the primary cause of inflammatory pain is the inflammatory response. Recent studies have shown that acupuncture can provide both anti-inflammatory and analgesic effects by regulating neuron and immune cell activity in the central and peripheral systems.
In this review, we first summarize the animal models and acupuncture interventions used for mechanistic studies of acupuncture therapy for inflammatory pain. More importantly, we discuss the neuroimmune crosstalk that is likely involved in these mechanisms at three levels: the local acupoint where acupuncture is performed, the sites of inflammation, and the central nervous system (CNS). Accordingly, we aim to provide a basis for future studies on acupuncture and its application in the treatment of inflammatory pain.
Using the PubMed database, we retrieved studies published between January 2010 and December 2020 using the keywords (“acupuncture” or “electroacupuncture” or “EA” or “manual acupuncture” or “transcutaneous acupoint electrical stimulation” or “TAES”) and (“pain” or “analgesia” or “analgesic”). Only studies in English were included, and 3,206 articles were retrieved in the primary search. Of the identified articles, 69 were excluded due to the absence of an abstract, and the titles and abstracts of the remaining 3,137 articles were screened further to determine whether the studies met the inclusion criteria. At this stage, 2,839 articles were excluded because they were unrelated to acupuncture and inflammatory pain. Of the remaining 298 articles, 97 were basic research articles, 113 were clinical research articles, and 88 were review articles or meta-analyses. The search procedure is depicted in Figure 1.
The aim of the literature search was to analyze the mechanism underlying the effect of acupuncture on inflammatory pain. Information from the 97 basic research articles is listed in Table 1 and Supplementary Table 1. First, three authors (BD, YL, and JM) jointly created a database of the studies that examined the mechanism underlying the effects of acupuncture on inflammatory pain. Variables such as the models of inflammatory pain, interventions, and outcome measures were added to this database. The data were extracted by three authors (BD, YL, and JM) and checked by the other authors (ZX, XL, and LT).
Multiple animal models have been used to study the mechanism underlying the effect of acupuncture on inflammatory pain. These include Complete Freund’s adjuvant (CFA)-, carrageenan-, monosodium iodoacetate-, formalin- and collagenase-induced inflammatory pain models, an inflammatory bowel disease (IBD) model, incision-induced pain models, and spontaneous senescence-associated osteoarthritis (OA) models. Of these, the CFA-induced adjuvant arthritis model, which mimics RA pathogenesis, is the primary one used to study the mechanistic effects of acupuncture in the treatment of inflammatory pain owing to the simplicity of model development and the stability of acupuncture efficacy in this model. More importantly, CFA models share similarities with human RA in terms of chronic pain and pathological manifestations such as synovial inflammation, bone destruction, and joint dysfunction and pathological findings such as inflammatory cell infiltration, vascular proliferation, and expansion (Weng et al., 2021), making this model popular in studies on inflammatory pain treated by acupuncture.
Of the reviewed animal studies, 70 used ST36 (Zusanli) as the site of acupuncture intervention, whereas 18 used GB30 (Huantiao) and BL60 (Kunlun), 11 used SP6 (Sanyinjiao), and 10 used GB34 (Yanglingquan). This was consistent with the acupoints typically used for the clinical treatment of OA. Electroacupuncture (EA) was the most frequently used intervention in animal studies, and low-frequency (1, 2, and 10 Hz) or variable-frequency (2/100 Hz) stimulation, a stimulation intensity of 1–2 mA, and a stimulation duration of 30 min were the most commonly used parameters for EA. Further, some studies mentioned that EA inhibits inflammatory pain more effectively at 2–10 Hz than at 100 Hz (Kim et al., 2004; Zhang R. X. et al., 2005). Manual acupuncture (MA) was used in 14 of the included studies. In most MA studies, stimulation was performed 2–3 times per second, and the duration of continuous stimulation was relatively short—generally 1–2 min with an interval of 4–5 min—and the total treatment duration was approximately 30 min. However, few studies compared therapeutic effects between MA and EA, and most of the included studies using EA did not provide detailed descriptions of the wave type and amplitude of electrical stimulation.
The initial effects of acupuncture take place at the acupoint, and mechanical stimulation is converted into chemical signals at these sites (Mingfu et al., 2013; Wu et al., 2014). Acupuncture significantly increases extracellular adenosine levels at the ST36 acupoint. In mouse models of inflammatory pain, MA or a local injection of a specific adenosine A1 receptor (A1R) agonist at ST36 significantly inhibits mechanical allodynia and thermal hyperalgesia (Goldman et al., 2010). In CFA-treated rats, EA at ST36 and BL60 reduces the levels of SP, neurokinin-1 receptor (NK-1R), interleukin-6 (IL-6), IL-1β, and tumor necrosis factor (TNF)-α in the DRG by promoting adenosine release and activating the A1Rs of nerve endings at local acupoints. Therefore, it appears that the acupuncture-activated acupoint–A1R pathway contributes to the anti-inflammatory action of acupuncture (Zhang et al., 2020d). Further, in CFA-induced rat models of pain, MA at ST36 can directly induce neural regulation by activating the mechanically sensitive transient receptor potential ion channel vanilloid 1 (TRPV1) channel receptors expressed on nerve terminals and immune cells and promote adenosine triphosphate (ATP) release via calcium wave propagation across nearby nerve endings. The injection of capsaicin, a TRPV1 agonist, can recapitulate the analgesic effect of acupuncture when injected at ST36 (Wu et al., 2014). In CFA models, EA at ST36 can activate mast cells and promote mast cell degranulation by activating TRPV2 and inducing the release of histamine and adenosine, which increases the levels of β-endorphins (β-ENDs) in the cerebrospinal fluid and results in analgesia (Huang et al., 2018). Meanwhile, acupuncture can also produce immune regulation at local acupoints by recruiting and activating immune cells and activating the nuclear factor kappa B (NFκB) pathway, which then induces the release of immune mediators [monocyte chemoattractant protein-1 (MCP-1) and IL-6] that bind to receptors at adjacent nerve endings, thereby transmitting acupuncture signals (Huang et al., 2018; Zhang et al., 2020b).
The initiation of the effects of acupuncture involves several factors and is dependent on several substances in the local acupoint microenvironment. As demonstrated by the aforementioned evidence, acupuncture may directly activate nociceptive terminals and immune cells, particularly mast cells, via mechanically sensitive channel receptors. This leads to the release of bioactive chemicals such as ATP and its degradation product, adenosine, which activate nociceptive nerve endings (Figure 2). However, the complicated neuroimmune network in the acupoint microenvironment remains to be elucidated.
Figure 2. Role of neuroimmune crosstalk at the acupoint in mediating the anti-inflammatory and analgesic effects of acupuncture on inflammatory pain. The names of immune factors are presented in red and green, respectively. The factors in red are up-regulated by acupuncture, while those in green are down-regulated by acupuncture. NF-κB, nuclear factor kappa-B; TRPV1/2/4, transient receptor potential ion channel vanilloid 1/2/4; MCP-1, monocyte chemoattractant protein-1; IL-16, interleukin- 6.
Inflammation is a hallmark of inflammatory pain. The increased release of inflammatory mediators results in the sensitization of peripheral nociceptors, which is characterized by a lowered activation threshold in these nociceptors (Yu et al., 2020). Activated DRG nociceptor neurons produce and release a large number of neuropeptides, such as SP and CGRP, from their peripheral terminals, which further aggravates local inflammatory responses and peripheral sensitization (Basbaum et al., 2009). Hence, the literature suggests that neuroimmune interactions are crucial in this process. Recently, acupuncture has also been found to alleviate inflammatory pain via neuroimmune crosstalk.
T cells can be classified into several sub-populations, including T helper 1 (Th1) cells, Th2 cells, Th17 cells, and T regulatory (Treg) cells. These cells regulate immune responses by producing specific pro- or anti-inflammatory cytokines (Shaw et al., 2018). For instance, Th1 cells release pro-inflammatory factors such as IL-1, IL-2, interferon-γ (IFN-γ), and TNF-α, while Th2 cells produce IL-10, IL-5, IL-13, IL-4, IL-6, and other anti-inflammatory factors. Pro-inflammatory factors bind to their corresponding receptors and stimulate peripheral nerve endings, while anti-inflammatory factors reduce the excitability of peripheral nerves. A significant imbalance in the Th1/Th2 ratio is observed in RA patients and animal models of RA. In CFA-induced rat models of pain, MA at ST36 induces anti-inflammatory and analgesic effects at inflamed joints. Analyses of cell–cell communication networks have shown that Th1 and Th2 cells act as key mediators in the CFA model and are also the main mediators of MA action (Xu et al., 2018). In the inflamed plantar tissues of mice treated with CFA, EA can inhibit inflammatory responses and pain by reducing the levels of IL-2 and INF-γ produced by Th1 cells and increasing the levels of IL-13, IL-4, and IL-5 produced by Th2 cells. Therefore, the maintenance of the Th1–Th2 balance decreases the sensitivity of peripheral nociceptors and mediates the anti-inflammatory and analgesic effects of EA (Wang et al., 2017).
Treg cells are anti-inflammatory, whereas Th17 cells are pro-inflammatory (Benedetti and Miossec, 2014). Multiple studies have confirmed that in collagen-induced arthritis (CIA) and UC models, EA at ST36 and GB39 (Xuanzhong) or RN4 (Guanyuan) induces Treg cell proliferation and simultaneously inhibits Th17 differentiation in the spleen, leading to the increased expression of anti-inflammatory factors like Transforming growth factor (TGF-β), IL-10, and IL-2 and the reduced expression of pro-inflammatory factors like IL-6, IL-17A, and IL-17F, ultimately reducing inflammatory hyperalgesia (Jimeno et al., 2012; Zhu et al., 2015; Sun et al., 2017). Similarly, EA at ST36 and SP6 can promote the activation of Treg cells and decrease the expression of the pro-inflammatory mediators IL-1β, TNF-α, and NOD-like receptor family 3 (NLRP3) in the hind paws of CFA-treated mice, reducing the stimulation of peripheral nociceptors in these animals and thereby relieving inflammatory pain (Yu et al., 2020).
Macrophages are highly heterogeneous and plastic immune cells that can polarize into different phenotypes. The polarization of these cells is dependent on different microenvironmental signals, and the polarized macrophages have specific functions in tissue homeostasis or host defense (Murray, 2017). The balance between pro- and anti-inflammatory macrophages also plays an important role in the regulation of inflammatory pain. In CFA-induced rat models of adjuvant arthritis, MA at ST36 can alleviate joint inflammation and pain by increasing the M2/M1 ratio. Further, such stimulation can decrease the levels of the pro-inflammatory cytokines TNF-α, IL-1α, IL-1β, IL-6, IL-7, and IL-18 and increase the levels of the anti-inflammatory cytokines IL-4 and IL-5 (Fuming et al., 2021). In inflammatory muscle pain models, MA at SP6 induces phenotypic changes in muscle macrophages by reducing the number of M1 macrophages and increasing the number of M2 macrophages (major source of IL-10) in the gastrocnemius muscle, thereby reducing thermal and mechanical hyperalgesia and decreasing avoidance behaviors and edema (da Silva et al., 2015). In the OA model, the activation of the NLRP3 inflammasomes leads to the production of IL-1β and TNF-α, resulting in the promotion of synovial inflammation, cartilage degeneration, and chondrocyte apoptosis (Nasi et al., 2017). In OA models, EA at Ex-LE4 (Neixiyan) and ST35 (Dubi) can significantly inhibit the activation of the NLRP3 inflammasome and the protein expression of matrix metalloproteinase-13, caspase-1, and IL-1β in cartilage tissue, thereby reducing mechanical hyperalgesia, improving the structure of articular cartilage, and reducing cartilage surface fibrillation (B-Wang et al., 2020). Recently studies have shown that EA at ST36 excites the vagus nerve via its dorsal nucleus, activates the α7 nicotinic acetylcholine receptor (α7nAChR)-mediated Janus kinase 2/signal transducer and activator of transcription 3 (JAK2/STAT3) signaling pathway in macrophages, inhibits the expression of the inflammatory factors IL-6 and TNF-α, reduces the local immune responses in the gastrointestinal tract, and promotes the recovery of gastrointestinal motility in mice with postoperative intestinal paralysis (Yang et al., 2021). These pieces of evidence suggest that macrophage polarization and the balance of related cytokines may be the main targets of acupuncture in its regulation of inflammatory pain.
Monocyte- and macrophage-related chemokines are also regulated by acupuncture. The MCP-1/chemokine receptor 2 (CCR2) axis mediates the recruitment of monocytes and macrophages in the early stage of inflammatory pain, causes the secretion of inflammatory factors and nerve growth factors (NGFs), and aggravates OA progression (Raghu et al., 2017). In the KOA model, MA at ST35 and ST36 can inhibit the MCP-1/CCR2 axis, thereby inhibiting the recruitment of monocytes/macrophages and down-regulating IL-1β, TNF-α, and NGFs and alleviating hyperalgesia and cartilage degeneration in the knee joint (Li et al., 2020). In rats treated with CFA, EA at GB30 up-regulates chemokine C-X-C motif chemokine ligand 10 (CXCL10) expression and increases the number of chemokine C-X-C motif receptor 3 (CXCR3)+ (receptor of CXCL10) macrophages containing opioids at sites of inflammation (Wang et al., 2014).
Together, this evidence shows that acupuncture can promote the balance of immune cells by regulating immune mediators, thus reducing the inflammatory response at local nociceptors. However, it remains unclear how acupuncture, as a body distal stimulation, inhibits the inflammatory response. Recent studies have reported that acupuncture can activate distinct sympathetic or parasympathetic nerves that project to immune-related organs and modulate systemic inflammation. Future studies of acupuncture treatment for inflammatory pain will be directed toward mapping the somatosensory pathways that drive distinct autonomic pathways.
Electroacupuncture at GB30 can increase the number of opioid-containing macrophages and therefore increase the release of β-ENDs in the inflamed paw tissue of rats treated with CFA (Zhang G. G. et al., 2005). Interestingly, the long-term analgesic effect of EA is antagonized by β-END and enkephalin (ENK) antagonists, but not by dynorphin A (DYN) antagonists, indicating that the β-ENDs released due to acupuncture stimulation act on μ- and δ-opioid receptors and inhibit peripheral inflammatory pain (Wang et al., 2014). In monosodium urate-induced rat models of acute gouty arthritis, variable frequency EA (2/100 Hz) at ST36 and BL60 can up-regulate the expression of β-END in the local ankle tissue, and this β-END can act on μ- and κ-opioid receptors to exert peripheral analgesic effects (Chai et al., 2018). In addition, in carrageenan-induced rat models of pain, EA at ST36 activates μ-, δ-, and κ-opioid receptors, exerting analgesic effects by reducing the excitability of peripheral neurons and inhibiting the release of pro-inflammatory neuropeptides (such as SP) at peripheral nerve endings (Taguchi et al., 2010). These studies show that acupuncture may promote the aggregation of immune cells containing opioid peptides in inflammatory tissues and promote the release of β-END and ENK, which may act on μ-, δ-, and κ-opioid receptors at peripheral sensory endings to exert peripheral analgesic effects.
The endocannabinoid system is a vital neuromodulation system for pain sensation, and it includes two G protein-coupled receptors: cannabinoid receptor 1 (CB1R) and CB2R. EA at GB30 and GB34 enhances the expression of CB2R in immune cells (keratinocytes, macrophages, and T-lymphocytes) in inflamed skin tissue and reduces pain in CFA-treated rats (Chen et al., 2009; Zhang et al., 2010). In the CFA-treated and KOA pain models, EA can inhibit the release of the pro-inflammatory factors TNF-α, IL-1β, and IL-6 by activating CB2R, thereby suppressing peripheral inflammatory pain (Su et al., 2012; Yuan et al., 2018a; Cristino et al., 2020). EA can also exert anti-inflammatory and analgesic effects by reducing the activation of NLRP3 inflammasomes in skin macrophages via the activation of CB2R, and CB2R knockout can reduce NLRP3 inflammasome activation and weaken the analgesic effect of EA (Gao et al., 2018). In addition, cannabinoids and β-ENDs synergistically mediate the anti-inflammatory and analgesic effects of acupuncture. Interestingly, EA at GB30 and GB34 increases the levels of β-END by activating CB2R in keratinocytes, macrophages, and T lymphocytes in inflamed skin tissue to inhibit inflammatory pain (Su et al., 2011).
There are four receptors for adenosine: A1R, A2aR, A2bR, and A3R. Adenosine mainly inhibits inflammatory pain through A1R and A2aR (Andreas et al., 2008; Sawynok, 2016). A2aRs bind to adenosine-activated protein kinase A (PKA), which promotes the production of immunosuppressive cells and increases their infiltration, further inducing an anti-inflammatory response (Lv et al., 2021). EA at BL25 increases the expression of A1R, A2aR, and A3R in the colon of mouse models of IBD. Further, A2bR mediates the acupuncture-induced inhibition of the release of the pro-inflammatory factor IL-1β and reduces visceral pain (Hou et al., 2019). Similarly, EA at ST36 and SP6 can increase A2aR expression and inhibit the release of TNF-α in the ankle joint of CIA mice, thus exerting anti-inflammatory and tissue protection effects (Li et al., 2015). However, the role of A1R in the immune regulation caused by acupuncture has not been explored.
Peripheral nerve fibers and their cell bodies in the DRG relay inflammatory pain-related afferent input to the spinal cord (Lai et al., 2019). In the DRG, nociceptive ion channels (such as TRPV1) on primary sensory neurons open after peripheral noxious stimulation and activate neurons while releasing active substances such as SP and CGRP. Repeated long-term stimulation causes neurogenic inflammation and enhances neuronal excitability and primary afferent input, causing peripheral sensitization (Yoo et al., 2011).
Transient receptor potential ion channel vanilloid 1 is generally considered to be involved in inflammation and perceived thermal pain. Studies have found that acupuncture can down-regulate the expression and sensitivity of TRPV1 by reducing the release and expression of pro-inflammatory neuropeptides (SP and CGRP), pro-inflammatory cytokines (TNF-α, IL-1β, and IL-6), and NGF and reducing the sensitization of sensory neurons (McDonald et al., 2013). For example, TRPV1 is the main downstream target of Mas-associated G protein-coupled receptor C (MrgprC) activation in the acute inflammatory pain signaling pathway (Huang et al., 2006). EA at bilateral ST36 and BL60 in CFA-treated rats can inhibit the phosphorylation of TRPV1 residues by protein kinase C (PKC) via the down-regulation of MrgprC. This could reduce the sensitivity and openness of TRPV1 channels, thereby reducing internal Na+ and K+ flow and inhibiting pain transmission (Liu et al., 2018). EA at bilateral ST36 can inhibit the expression of TRPV1 and its downstream signaling molecules in the DRG in CFA-induced animal models of pain, thus down-regulating the activation of p-PKA, p-extracellular signal-regulated kinases (ERK), p-c-Jun-N-terminal kinase (JNK), p38-mitogen-activated protein kinase (MAPK), several transcription factors, p-cAMP response element binding protein (CREB), p-NFκB, and the noxious ion channel Nav1.7 (Liao et al., 2017; Yang et al., 2017). In TRPV1–/– mice, EA treatment does not produce analgesic effects, indicating that TRPV1 is key for EA-mediated analgesia in cases of inflammatory pain (Lu et al., 2016). EA at ST36 can inhibit the expression of acid sensation ion channels 3 (a sensor of acidic environments and mechanical stimuli) and TRPV4 (a sensor of osmotic pressure and mechanical stimuli) in DRG neurons and relieve inflammatory pain in both carrageenan- and CFA-induced models of pain via the inhibition of peripheral sensitization (Chen et al., 2011, 2012).
Substance P is a neuropeptide released by sensory nerve endings and it is the main mediator of neurogenic inflammation. The combination of SP and NK-1R can increase the secretion of pro-inflammatory cytokines and aggravate inflammatory pain (Yang et al., 2013). EA at ST36 can reduce the levels of SP and NK-1R and the expression of IL-6, TNF-α, and IL-1β in the DRG of CFA-treated rats, abating mechanical sensitivity and thermal pain. Interestingly, when the dorsal nerve root is cut or an SP receptor antagonist is injected, the inhibitory effect of EA on SP, NK-1R, and other downstream inflammatory factors is attenuated, which significantly reduces the analgesic effect of EA. Hence, EA may also prevent the transmission of pain signals to the CNS by inhibiting the expression of SP in the DRG (Zhang et al., 2020d).
The purinergic P2 × 3 receptor (P2 × 3R) is a ligand-gated non-selective cation channel that is selectively expressed in primary sensory neurons. When inflammatory pain occurs, ATP is released from damaged tissues or inflammatory cells and binds to P2 × 3R in DRG neurons to activate nociceptors and transmit pain signals (Bernier et al., 2018). EA at ST36 and BL60 can significantly reduce the number of P2 × 3R-positive neurons in the L4-6 DRG and reduce the expression of the P2 × 3R protein in the L6 DRG. Further, P2 × 3R agonists can reduce EA-mediated analgesia in CFA-treated rats (Fang et al., 2018). In CFA-treated rats, both short- and long-term 100-Hz EA at ST36 can reduce the expression of P2 × 3R in the DRG. Moreover, the inhibition or activation of P2 × 3R in the DRG may contribute to or weaken the analgesic effect of EA, respectively (Xiang et al., 2019). In addition, in rat models of IBD, EA at ST37 and ST25 can down-regulate the expression of P2 × 3R in the colon myenteric plexus and reduce sensitivity to visceral pain (Weng et al., 2015).
Tyrosine hydroxylase (TH) is the rate-limiting enzyme in the synthesis of catecholamines, and it is a key component of the peripheral sympathetic nervous system (Dunkley and Dickson, 2019). When the DRG receives pain signals, sympathetic nerve fibers protrude and invade the region around sensory neurons, participating in pain signal transduction. For example, in trinitrobenzene sulfonic acid (TNBS)-induced rat models of colitis, the germination of TH immunoreactive fibers toward DRG sensory neurons contributes to the maintenance and aggravation of peripheral inflammatory pain. EA at ST36 and ST37 can reduce hyperalgesia and inflammatory damage in the distal colon by inhibiting the expression of TH in the L6 DRG. This may be one mechanism via which EA relieves the symptoms of colitis (Wang et al., 2019).
In summary, the interactions between the peripheral nervous system and immune system that underly the effect of acupuncture on inflammatory pain include the following: (1) Acupuncture can induce the migration of immune cells containing analgesic substances to target organs, where they release opioid peptides, cannabinoids, and other analgesic substances to achieve analgesia by blocking peripheral sensitization. (2) Acupuncture regulates the balance of immune cells and reduces the release of pro-inflammatory factors, thereby reducing the stimulation of peripheral nociceptive nerves by inflammatory factors. (3) Acupuncture inhibits the release of SP, CGRP, and other neuropeptides from nociceptive DRG neurons, inhibits TRPV1 and its downstream signaling pathways, reduces peripheral neurogenic inflammation, and inhibits the transmission of pain signals to the CNS (Figure 3).
Figure 3. Role of neuroimmune crosstalk at the sites of inflammation in mediating the anti-inflammatory and analgesic effects of acupuncture on inflammatory pain. The names of immune factors are presented in red and green, respectively. The factors in red are up-regulated by acupuncture, while those in green are down-regulated by acupuncture. IL-1β/2/4/5/6/10/13/17, interleukin-1β/2/4/5/6/10/13/17; IFN-γ, interferon-γ; TGF-β, transforming growth factor; CXCL10, chemokine (C-X-C motif) ligand 10; NLRP3, NOD-like receptor family 3; MMP13, matrix metalloproteinase-13; A1R, adenosine 1 receptor; CytR, cytokine receptor; OpioidR, opioid receptor; A2aR, adenosine 2a receptor; SP, substance P; CB2R, cannabinoid receptor 2; P2 × 3, Purinergic P2 × 3 receptor; NK-1R, neurokinin-1 receptor; ASIC3, acid sensation ion channels 3; CGRP, calcitonin-gene-related peptide; TRPV1/2, transient receptor potential ion channel vanilloid 1/2; DRG, dorsal root ganglion; SCDH, spinal cord dorsal horn.
Emerging clinical and preclinical studies have indicated that neuron–glia interactions in the spinal cord are involved in the pathogenesis of chronic pain (Gwak et al., 2017; Haight et al., 2019). Inflammatory pain signals are transmitted via peripheral afferent nerves. When a nerve impulse arrives, neurotransmitters such as glutamate, ATP, SP, and CGRP are produced and released from primary DRG neurons, and information is transmitted to the spinal cord. These neurotransmitters act on the receptors present on glial cells and neurons, modulating glial and neuronal activity (Wei et al., 2008; Roberts et al., 2009). Glial cells (microglia and astrocytes) respond to increased input from peripheral nerves by changing their morphology, increasing in number, and releasing pronociceptive mediators such as cytokines and chemokines. These gliotransmitters can sensitize neurons by activating their homologous receptors, thereby promoting central sensitivity, which is fundamental for the generation of allodynia, hyperalgesia, and spontaneous pain (Old et al., 2015). The interaction between glial cells and neurons creates an integrated network that coordinates the immune response and modulates the excitability of pain pathways (Han et al., 2015).
Recent studies have shown that acupuncture can relieve inflammatory hyperalgesia by inhibiting the interaction between spinal cord neurons and glial cells. In formalin- and CFA-induced animal models of inflammatory pain, EA can relieve pain by decreasing the expression of the pain-related factors SP, CGRP, IFN-γ, IL-6, IL-1β, and TNF-α and increasing the levels of the anti-inflammatory factors IL-4 and IL-10 in the spinal cord (Liu et al., 2019). Neuron-derived chemokine C-X3-C motif chemokine ligand 1 (CX3CL1) activates C-X3-C chemokine receptor 1 (CX3CR1) on microglia, leading to the phosphorylation of p38 MAPK in microglia (Zhang et al., 2017). EA (2 Hz, 1–2 mA) at ST36 in CFA-induced pain models can reduce pain significantly by decreasing CX3CL1 expression in the spinal cord, inhibiting the activation of the p38 MAPK pathway in microglia, and reducing the release of downstream cytokines (IL-6, IL-1β, and TNF-α) (Li et al., 2019).These cytokines are important messengers that transmit signals between glia and neurons. The reduction in IL-1β and TNF-α attenuates N-methyl-D-aspartate (NMDA) receptor phosphorylation, inhibits the change in synaptic strength, and reduces behavioral hyperalgesia (Zhang et al., 2011a; Li et al., 2019). Purinergic signaling is also involved in the maintenance of pain. After peripheral tissue injury, a significant amount of ATP is released from satellite glial cells. This ATP acts on P2 × 7R and promotes the activation of CX3CR1 in microglia, and the interaction between CX3CL1 and CX3CR1 is initiated by the activation of P2 × 7R (Clark and Malcangio, 2014). The reduction in ATP/P2 × 7R signaling observed after three session of EA inhibits the downstream CX3CL1/CX3CR1 signaling pathway and p38 MAPK phosphorylation in microglia (Gao et al., 2017). Accordingly, EA suppresses ATP/P2 × 7R/CX3CL1/CX3CR1/p38 MAPK-mediated neuroglial crosstalk and thus exerts an analgesic effect.
When pain signals are relayed to the spinal cord, glutamate released from primary neurons activates glutamate receptors on astrocytes, increasing Ca2+ mobilization in these cells. The release of a series of mediators from activated astrocytes in turn modulates neuronal activity. For example, IL-10 can regulate long-term potentiation (LTP) of the synapses between primary afferent C-fibers and secondary neurons in order to prevent long-term mechanical and thermal hyperalgesia (Clark et al., 2015). EA at SP6 and GB34 relieves incision-related pain and suppresses spinal LTP via an increase in IL-10 levels in spinal astrocytes (Clark et al., 2015; Dai et al., 2019). The binding of IL-33 to growth stimulation expressed gene 2 (ST2) can activate downstream MAPK signaling pathways and aggravate inflammation. This activation plays an important role in central sensitization and pain modulation (Crown, 2012). EA reduces the paw lifting time and paw licking time in mouse models of formalin-induced pain, inhibits the expression of IL-33 in astrocytes and that of ST2 in astrocytes and neurons, and further inhibits the phosphorylation of ERK and JNK. Subcutaneous or intrathecal injection of recombinant IL-33 weakens the analgesic effect of EA and reverses the EA-induced suppression of ERK and JNK phosphorylation. Therefore, EA alleviates inflammatory pain by inhibiting IL-33/ST2 signaling and the downstream ERK and JNK pathways (Han et al., 2015). Even one session of EA at ST36 and BL60 can significantly increase the pain thresholds of CFA-treated rats and remarkably suppress ERK1/2 activation and cyclo-oxygenase 2 (COX-2) expression. Three sessions of EA decrease NK-1 expression and the DNA binding activity of CREB, a transcription factor downstream to ERK1/2. Therefore, acupuncture produces an analgesic effect by preventing the activation of the ERK1/2-COX-2 pathway and ERK1/2-CREB-NK-1 pathway at different stages of inflammatory pain progression (Fang et al., 2014).
These studies together show that acupuncture inhibits the communication and interaction between glial cells and neurons by reducing the phosphorylation of p38 MAPK, inhibiting the ERK and JNK pathways, decreasing the levels of pro-inflammatory factors, and increasing the levels of anti-inflammatory factors, thereby relieving inflammatory pain.
Glutamate is an excitatory neurotransmitter that is widely distributed in the CNS, and it plays a key role in the induction of CNS sensitization. Glutamate has three receptors, namely NMDA receptors, α-amino-3-hydroxy-5-methyl-4-isoxazole-propionic acid receptors, and kainate or metabotropic receptors (Swartjes et al., 2011). IL-1β and TNF-α, which are released from activated glial cells after the induction of inflammatory pain, lead to the phosphorylation of glutamate receptors. This enhances the excitability of spinal neurons and promotes pain transmission via the regulation of glutamate receptor activity and Ca2+-dependent signaling (Zhou et al., 2001; Liu et al., 2015). EA and acupoint catgut embedding (ACE) can inhibit the phosphorylation of the GluN1 subunit and thus inhibit the activation of NMDA receptors in the spinal cord. Further, these treatments can also inhibit Ca2+-dependent signals (calmodulin-dependent protein kinase II, ERK, and CREB) in CFA-treated rats, thus relieving inflammatory pain (Cui et al., 2019). In CFA-induced rat models of inflammatory pain, there is an increase in GluR2 phosphorylation in the ipsilateral SDH. EA (2 Hz, 1 mA) at ST36 and SP6 produces analgesic effects through the down-regulation of GluR2 phosphorylation (Lee et al., 2013). In addition, glutamate transporter (GT-1) in astrocytes prevents excessive activation of postsynaptic glutamate receptors by buffering the glutamate released into synapses (Nie and Weng, 2009). Nevertheless, injuries result in decreased GT-1 expression and an alteration of the glutamate homeostasis in synapses between astrocytes and neurons, leading to increased dorsal horn excitability and the development of inflammatory pain (Sung et al., 2003). In CFA-induced models of inflammatory pain, EA treatment can increase GT-1 expression in astrocytes, resulting in the clearance of excess glutamate in the synaptic cleft and a reduction in pain signals (Kim et al., 2012). Taken together, these data suggest that acupuncture modulates inflammatory pain by reducing neuroglial interactions and inhibiting the expression and phosphorylation of glutamate receptors and that it promotes glutamate reuptake by increasing the expression of glutamate transporters in astrocytes.
The activation of the endogenous opioid system is the best-understood mechanism underlying acupuncture-induced analgesia. This system mainly involves ENDs, ENKs, and DYNs, and the μ-, δ-, and κ-opioid receptors (Zhang et al., 2016). Different frequencies of EA are known to activate different opioid receptors. Low-frequency (2 Hz) EA promotes the release of ENKs, which binds to μ- and δ-opioid receptors, while high-frequency (100 Hz) EA promotes the release of DYNs, which bind to μ-opioid receptors (Seo et al., 2013; Zhang et al., 2014). Acupuncture can reduce inflammatory pain by activating different opioid receptors in different inflammatory pain models. In CFA- and capsaicin-induced inflammatory pain models, the inhibition of thermal and mechanical hyperalgesia caused by EA is blocked by the intrathecal administration of μ- and δ-opioid receptor antagonists but not by that of κ-opioid receptor antagonists (Zhang et al., 2004; Kim et al., 2009). In carrageenan-induced inflammatory pain models, the analgesic effects of acupuncture are blocked by the intrathecal administration of a μ-opioid receptor antagonist but not by that of a δ- or κ-opioid receptor antagonist (Yang et al., 2011). The differential involvement of opioid receptors might be due to changes in receptor sensitivity during the development of inflammatory pain. Interestingly, EA induces the release of ENDs in CFA-treated rats and inhibits the release of γ-aminobutyrate (GABA) by activating μ-opioid receptors on GABAergic neurons, thus activating serotonergic neurons in the rostral ventromedial medulla and causing them to release 5-hydroxytryptamine (5-HT) and suppress pain (Zhang et al., 2011b). This indicates that opioids and their receptors contribute to the increased release of analgesic transmitters, producing analgesic effects.
The endocannabinoid system is involved in the control of pain transmission and is largely dependent on two ligands: N-arachidonoylethanolamide and 2-arachidonoyl glycerol (2-AG) (Finn et al., 2021). CB1R is a cannabinoid receptor that is widely distributed in the nerve endings of both GABAergic and glutamatergic neurons in the periaqueductal gray (PAG; Hu et al., 2014; Samineni et al., 2017). EA (2 Hz, 1 mA) at Ex-LE4 (Neixiyan) and ST35 relieves the inflammatory pain caused by KOA via an increase in the levels of 2-AG, the induction of CB1R expression on GABAergic neurons (but not on glutamatergic neurons), and a reduction in 5-HT levels. The microinjection of CB1R antagonists in the ventrolateral PAG (vlPAG) can reverse the effects of EA. In GABA-CB1–/– mice, 5-HT levels do not increase after EA stimulation, confirming that EA potentiates the pain-inhibition effects of 5-HT in the descending inhibition pathway via CB1Rs on GABAergic neurons (Yuan et al., 2018b). EA can also simultaneously and bidirectionally inhibit GABAergic neurons and excite glutamatergic neurons by increasing CB1R expression in the vlPAG, thereby allowing serotonergic neurons to be sufficiently excited, resulting in antinociceptive effects (Zhu et al., 2019). Repeated EA at ST36 and BL60 increases the gene expression of CB1R and dopamine D1 and D2 receptors in the striatum. The injection of CB1R antagonists reverses the analgesic effects of repeated EA stimulation, indicating that the endocannabinoid system contributes to acupuncture-induced inflammatory pain reduction via the regulation of dopamine release in the striatum (Shou et al., 2013).
Therefore, endocannabinoid-CB1R-GABAergic/glutamatergic neurons and neurotransmitters (5-HT, dopamine, and norepinephrine) may form a novel pathway that mediates the acupuncture-induced inhibition of inflammatory pain. Future research should focus on the role of the endocannabinoid system in neural circuits and its interactions with other systems.
Serotonergic neurons in the raphe nucleus release 5-HT, targeting receptors along the descending pain circuit, and participate in the regulation of pain perception (Boadas-Vaello et al., 2017; Tao et al., 2019). Countless studies have shown that 5-HT and 5-HT receptors (5-HTRs) participate in acupuncture-induced analgesia. EA at GB30 in CFA-treated rats can activate serotonergic neurons in the nucleus raphes magnus and cause them to release 5-HT, which binds to spinal 5-HT1ARs and produces analgesic effects. Moreover, serotonin depletion and treatment with a 5-HT1AR antagonist prevent the effects of EA (Li et al., 2007; Zhang et al., 2011c). In collagenase-induced osteoarthritis rat models, EA at ST36 produces analgesic effects, and the analgesic effect of 2-Hz EA is reduced after pretreatment with 5-HT1R and 5-HT3R antagonists, although no such effect is observed when 5-HT2R antagonists are used (Seo et al., 2016). In CFA-induced pain models, 10-Hz EA activates 5-HT1ARs, but not 5-HT2BRs, 5-HT2CRs, or 5-HT3Rs in the spinal cord (Zhang et al., 2012b). Therefore, EA inhibits hyperalgesia by activating serotonergic neurons in the spinal cord and causing them to release 5-HT, which acts on 5-HT1ARs in the spinal cord.
The activation of 5-HT1AR has been reported to block GluN1 phosphorylation (Liu et al., 2011), and EA and ACE also inhibit the activation of GluN1. Importantly, intrathecal injection of a 5-HT1AR antagonist and agonist can block and mimic, respectively, the effects of EA and ACE. EA can alleviate inflammatory pain by activating 5-HT1ARs and preventing the phosphorylation of GluN1 and Ca2+-dependent signaling (Cui et al., 2019). Taken together, the data suggest that 5-HT mediates the effects of acupuncture against inflammatory pain through the activation of various receptor subtypes and the inhibition of glutamate receptor phosphorylation.
According to the International Association for the Study of Pain, pain has sensory, emotional, cognitive, and social components. Anxiety, depression, and other negative emotions can be caused by nociceptive stimulation (Williams and Craig, 2016). The main brain region associated with pain-related emotions is the anterior cingulate cortex (ACC; Zeng et al., 2018). NMDARs and μ-opioid receptors are co-expressed in the ACC, and EA at GB30 relieves pain-induced place aversion in CFA-treated rats through the promotion of μ-opioid receptor expression and inhibition of NMDA excitation (Zhang et al., 2012a). Protein kinase M zeta (PKM zeta) and GluR1 are involved in pain and the neuroplasticity induced by pain-related emotions (Adzovic and Domenici, 2014). EA at ST36 and BL60 inhibits the phosphorylation of PKM zeta and its downstream target GluR1 and reduces ACC-mediated LTP, thereby alleviating the anxiety-like behavior induced by inflammatory pain (Du et al., 2017). The neuropeptide S/neuropeptide S receptor (NPS/NPSR) system is involved in the regulation of the anxiety induced by chronic inflammation. EA at ST36 and BL60 enhances ipsilateral NPS and NPSR protein expression in the ACC and reduces the anxiety-like behavior associated with pain (Du et al., 2020).
Pain memory is an endogenous factor in intractable chronic pain. The secondary messenger cAMP, its downstream protein kinase PKA, and the transcription factor CREB regulate pain-related learning and memory and neuroplasticity (Wu et al., 2013). EA can modulate pain memory by inhibiting the cAMP/PKA/CREB signaling pathway (Shao et al., 2016). Therefore, there has been a switch from the conventional notion of a single mode of nociceptive sensation to a multi-dimensional mode of pain–emotion–cognition in studies involving pain research. Acupuncture can not only reduce pain sensation, but can also help manage pain-related emotion and cognition by regulating neurotransmitters and neuroplasticity, thus alleviating pain and related factors in an all-round manner.
In the CNS, neurons and glial cells contribute to the neuroimmune interactions involved in the production and maintenance of inflammatory pain. Acupuncture can reduce the levels of neuropeptides released by primary neurons, reduce the release of immune mediators from activated glial cells, and inhibit the interaction between glial cells and secondary neurons, thus inhibiting the transmission of pain signals. Acupuncture can also inhibit the release of central excitatory neurotransmitters and promote the release of inhibitory neurotransmitters, reduce the interaction between neurotransmitters, and relieve pain and pain-related emotions and cognition (Figure 4). Current evidence has confirmed that acupuncture regulates the glial cell–cytokine–neuron interaction. Most studies on the regulation of neurotransmitters and neuropeptides by acupuncture focus on the interactions between neurotransmitters and neural circuits. There are few studies on the relationship between neurotransmitters and glial cells, and more attention should be paid to this aspect in future studies.
Figure 4. Role of neuroimmune crosstalk within the central nervous system in mediating the anti-inflammatory and analgesic effects of acupuncture on inflammatory pain. The names of neurotransmitters, neuropeptides, and immune factors are presented in red, green, and purple, respectively. The factors in red are up-regulated by acupuncture, while those in green are down-regulated by acupuncture. The factors in purple are not regulated by acupuncture. SP, substance P; SPR, substance P receptor; NK1, neurokinin-1; CGRP, calcitonin-gene-related peptide; CRLR, calcitonin-gene-related peptide receptor; ATP, adenosine triphosphate; P2 × 7R, purinergic P2 × 7 receptor; CX3CL1, C-X3-C motif chemokine ligand 1; CX3CR1, C-X3-C chemokine receptor 1; p38 MARK, p38-mitogen-activated protein kinase; COX-2, cyclo-oxygen-ase 2; CREB, cAMP response element binding protein; ERK, extracellular signal-regulated kinases; JNK, c-Jun-N-terminal kinase; AMPAR, α-amino-3-hydroxy-5-methyl-4- isoxazolepropionic acid receptor; NMDAR, N-methyl-D-aspartate receptor; GABA, γ-aminobutyrate; 5-HT, 5-hydroxytryptamine; 5-HTR, 5-hydroxytryptamine receptor; CB1, cannabinoid 1; CB1R, cannabinoid receptor 1; OpioidR, opioid receptor; GABA, γ-aminobutyrate; GABAR, γ-aminobutyrate receptor; 2-AG, 2-arachidonoyl glycerol; IL-1β/4/6/10, interleukin-1β/4/6/10; TNF-α, tumor necrosis factor-α.
With in-depth research on the mechanism underlying the effects of acupuncture in treating inflammatory pain, we now fully appreciate the importance of neuroimmune interactions in this process. In peripheral regions, injury and inflammatory responses are the root causes of pain. Acupuncture can reduce the activation of pain pathways by promoting the balance between immune cells and inhibiting inflammatory responses. Acupuncture can also recruit immune cells that secrete analgesic neurotransmitters, which then act on receptors to produce analgesic effects. Long-term stimulation during peripheral inflammation leads to central sensitization. Acupuncture activates local neuroimmune regulation at acupoints and transmits acupuncture signals to the CNS. By regulating the release of neuropeptides and the interaction between glial cells and neurons, acupuncture inhibits neuroimmune crosstalk, which is vital to central sensitization. Meanwhile, acupuncture also promotes the release of analgesic neurotransmitters, inhibits the release of pain-promoting neurotransmitters, reduces the excitability of neurons and synaptic strength, and changes pain sensitivity. Accordingly, the regulation of neuroimmune crosstalk at the peripheral and central levels mediates the anti-inflammatory and analgesic effects of acupuncture on inflammatory pain in an integrated manner.
Although the reviewed studies provide reliable evidence for the application of acupuncture in the treatment of inflammatory pain, they have some limitations. First, the brain mechanisms underpinning the regulation of neuroimmune interactions by acupuncture during the treatment of inflammatory pain are not as well-studied as peripheral and spinal mechanisms. In cases of inflammatory pain, many inflammatory mediators at the inflamed site and in the spinal cord participate in the acupuncture-regulated neuroimmune crosstalk, but whether these mediators play a similar role in the brain remains unclear. Second, the interaction between spinal glial cells and neurons is involved in the acupuncture-mediated reduction in inflammatory pain, but microglia and astrocytes produce different mechanistic effects after acupuncture. It is still unclear which factors influence the timing of their activation and the molecular signal transduction within the cell. Third, some neurotransmitters can also be produced by glial cells, and glial cells also express the homologous receptors of neurotransmitters. However, it is not clear whether any interaction between neurotransmitters and glial cells mediates the effects of acupuncture in the treatment of inflammatory pain. Finally, current animal models have drawbacks because they show an acute inflammatory response and short-lived hyperalgesia, both of which become attenuated over time. Most studies have only examined the protective effect of acupuncture in the initial phase of inflammatory pain. Better models are needed to explore the effectiveness of acupuncture against chronic inflammatory pain.
Overall, this review of studies published over the last decade provides strong evidence for the usefulness of acupuncture in the treatment of inflammatory pain. The elucidation of the mechanisms underlying the effects of acupuncture in the treatment of inflammatory pain will open a variety of opportunities for further applications of acupuncture and a combination of acupuncture and drugs for treating, managing, and controlling inflammation and pain. Therefore, the continuation of research on this topic is extremely important.
BD and YLi: concept design, data collection, and manuscript writing. JM, NL, and WF: preparation of figures and the graphical abstract. LT, ZC, YGo, and ZL: data collection and analysis. ZX, SW, YX, YLiu, BC, YF, and YGu: language modification and review of the manuscript text. YGuo and XL: concept design and manuscript review. All authors contributed to the article and approved the submitted version.
This study was financially supported by the National Natural Science Foundation of China (NSFC) (Nos. 82030125, 81704146, 81873369, and 81873368); the National Key R&D Program of China (No. 2019YFC1709003); the National Natural Science Foundation of Tianjin (No. 20JCQNJC00280); the Graduate Research and Innovation Project of Tianjin (No. 2020YJSB197); the Graduate Research and Innovation Project of Tianjin University of Traditional Chinese Medicine (No. YJSKC-20201001).
The authors declare that the research was conducted in the absence of any commercial or financial relationships that could be construed as a potential conflict of interest.
All claims expressed in this article are solely those of the authors and do not necessarily represent those of their affiliated organizations, or those of the publisher, the editors and the reviewers. Any product that may be evaluated in this article, or claim that may be made by its manufacturer, is not guaranteed or endorsed by the publisher.
The Supplementary Material for this article can be found online at: https://www.frontiersin.org/articles/10.3389/fnins.2021.695670/full#supplementary-material
2-AG, 2-arachidonoyl glycerol; 5-HTRs, 5-HT receptors; 5-HT , 5-hydroxytryptamine; A1R, A1 receptor; ACE, acupoint catgut embedding; ATP, adenosine triphosphate; ACC, anterior cingulate cortex; CGRP, aalcitonin-gene-related peptide; CREB, cAMP response element binding protein; CB1R, cannabinoid receptor 1; CNS, central nervous system; CX3CL1, chemokine C-X 3-C motif chemokine ligand 1; CXCL10, chemokine C-X -C motif chemokine ligand 10; CXCR3, chemokine C-X -C motif receptor 3; CCR2, chemokine receptor 2; CIA, collagen-induced arthritis; CFA, complete Freund’s adjuvant; CX3CR1, C-X 3-C chemokine receptor 1; COX-2, cyclo-oxygenase 2; DRG, dorsal root ganglion; DYN, dynorphin; EA, electroacupuncture; ERK, extracellular signal-regulated kinases; GT-1, glutamate transporter; ST2, growth stimulation expressed gene 2; IBD, inflammatory bowel disease; IFN- γ , interferon- γ; IL-6, interleukin-6; JAK2, Janus kinase 2; JNK, Jun-N-terminal kinase; KOA, knee osteoarthritis; LTP, long-term potentiation; MA, manual acupuncture; MrgprC, Mas-associated G protein-coupled receptor C; ENK, enkephalin; MAPK, mitogen-activated protein kinase; MCP-1, monocyte chemoattractant protein-1; NGFs, nerve growth factors; NK-1R, neurokinin-1 receptor; NPS, neuropeptide S; NPSR, neuropeptide S receptor; NMDA, N-methyl -D-aspartate; NLRP3, NOD-like receptor family 3; NF κ B, nuclear factor kappa B; OA, osteoarthritis; PAG, periaqueductal gray; PKA, protein kinase A; PKC, protein kinase C; PKM zeta, protein kinase M zeta; P2 × 3R, purinergic P2 × 3 receptor; RA, rheumatoid arthritis; STAT3, signal transducer and activator of transcription 3; SDH, spinal dorsal horn; SP, substance P; Th1, T helper 1; Treg, T regulatory; TGF- β , transforming growth factor; TRPV1, transient receptor potential ion channel vanilloid 1; TNBS, trinitrobenzene sulfonic; TNF- α , tumor necrosis factor- α; TH, tyrosine hydroxylase; UC, ulcerative colitis; vlPAG, ventrolateral PAG; α 7nAChR, α 7 nicotinic acetylcholine receptor; β-ENDs , β-endorphins; GABA, γ-aminobutyrate .
Adzovic, L., and Domenici, L. (2014). Insulin induces phosphorylation of the AMPA receptor subunit GluR1, reversed by ZIP, and over-expression of Protein Kinase M zeta, reversed by amyloid beta. J. Neurochem. 131, 582–587.
Andreas, K., Lübke, C., Häupl, T., Dehne, T., Morawietz, L., Ringe, J., et al. (2008). Key regulatory molecules of cartilage destruction in rheumatoid arthritis: an in vitro study. Arthritis Res. Ther. 10:R9. doi: 10.1186/ar2358
A-Wang, X., Zhao, N. Q., Sun, Y. X., Bai, X., Si, J. T., Liu, J. P., et al. (2020). Acupuncture for ulcerative colitis: a systematic review and meta-analysis of randomized clinical trials. BMC Complement. Med. Ther. 20:309. doi: 10.1186/s12906-020-03101-4
Basbaum, A. I., Bautista, D. M., Scherrer, G., and Julius, D. (2009). Cellular and molecular mechanisms of pain. Cell 139, 267–284. doi: 10.1016/j.cell.2009.09.028
Benedetti, G., and Miossec, P. (2014). Interleukin 17 contributes to the chronicity of inflammatory diseases such as rheumatoid arthritis. Eur. J. Immunol. 44, 339–347. doi: 10.1002/eji.201344184
Bernier, L. P., Ase, A. R., and Séguéla, P. (2018). P2X receptor channels in chronic pain pathways. Br. J. Pharmacol. 175, 2219–2230. doi: 10.1111/bph.13957
Boadas-Vaello, P., Homs, J., Reina, F., Carrera, A., and Verdú, E. (2017). Neuroplasticity of supraspinal structures associated with pathological pain. Anat. Rec. 300, 1481–1501. doi: 10.1002/ar.23587
B-Wang, Z., Chen, M., Wang, B., Kang, W., Yu, H., Li, X., et al. (2020). Electroacupuncture alleviates osteoarthritis by suppressing NLRP3 inflammasome activation in guinea pigs. Evid. Based Complement. Alternat. Med. 2020:5476064. doi: 10.1155/2020/5476064
Chai, W., Tai, Y., Shao, X., Liang, Y., Zheng, G. Q., Wang, P., et al. (2018). Electroacupuncture alleviates pain responses and inflammation in a rat model of acute gout arthritis. Evid. Based Complement. Alternat. Med. 2018:2598975. doi: 10.1155/2018/2598975
Chen, L., Zhang, J., Li, F., Qiu, Y., Wang, L., Li, Y. H., et al. (2009). Endogenous anandamide and cannabinoid receptor-2 contribute to electroacupuncture analgesia in rats. J. Pain 10, 732–739. doi: 10.1016/j.jpain.2008.12.012
Chen, W. H., Hsieh, C. L., Huang, C. P., Lin, T. J., Tzen, J. T., Ho, T. Y., et al. (2011). Acid-sensing ion channel 3 mediates peripheral anti-hyperalgesia effects of acupuncture in mice inflammatory pain. J. Biomed. Sci. 18:82. doi: 10.1186/1423-0127-18-82
Chen, W. H., Tzen, J. T., Hsieh, C. L., Chen, Y. H., Lin, T. J., Chen, S. Y., et al. (2012). Attenuation of TRPV1 and TRPV4 expression and function in mouse inflammatory pain models using electroacupuncture. Evid. Based Complement. Alternat. Med. 2012:636848. doi: 10.1155/2012/636848
Cheng, S., Zhou, J., Xu, G., Xin, M., Cheng, Y., Qu, Y., et al. (2020). Acupuncture and moxibustion for pain relief and quality of life improvement in patients with knee osteoarthritis: a protocol for systematic review and meta-analysis. Medicine 99:e20171. doi: 10.1097/MD.0000000000020171
Clark, A. K., Gruber-Schoffnegger, D., Drdla-Schutting, R., Gerhold, K. J., Malcangio, M., and Sandkühler, J. (2015). Selective activation of microglia facilitates synaptic strength. J. Neurosci. 35, 4552–4570. doi: 10.1523/JNEUROSCI.2061-14.2015
Clark, A. K., and Malcangio, M. (2014). Fractalkine/CX3CR1 signaling during neuropathic pain. Front. Cell. Neurosci. 8:121. doi: 10.3389/fncel.2014.00121
Corbett, M. S., Rice, S. J., Madurasinghe, V., Slack, R., Fayter, D. A., Harden, M., et al. (2013). Acupuncture and other physical treatments for the relief of pain due to osteoarthritis of the knee: network meta-analysis. Osteoarthr. Cartil. 21, 1290–1298. doi: 10.1016/j.joca.2013.05.007
Cristino, L., Bisogno, T., and Di Marzo, V. (2020). Cannabinoids and the expanded endocannabinoid system in neurological disorders. Nat. Rev. Neurol. 16, 9–29. doi: 10.1038/s41582-019-0284-z
Crown, E. D. (2012). The role of mitogen activated protein kinase signaling in microglia and neurons in the initiation and maintenance of chronic pain. Exp. Neurol. 234, 330–339. doi: 10.1016/j.expneurol.2011.10.019
Cui, W. Q., Sun, W. S., Xu, F., Hu, X. M., Yang, W., Zhou, Y., et al. (2019). Spinal serotonin 1A receptor contributes to the analgesia of acupoint catgut embedding by inhibiting phosphorylation of the N-Methyl-d-Aspartate Receptor GluN1 subunit in complete freund’s adjuvant-induced inflammatory pain in rats. J. Pain 20, 16.e1–16.e16. doi: 10.1016/j.jpain.2018.07.011
da Silva, M. D., Bobinski, F., Sato, K. L., Kolker, S. J., Sluka, K. A., and Santos, A. R. (2015). IL-10 cytokine released from M2 macrophages is crucial for analgesic and anti-inflammatory effects of acupuncture in a model of inflammatory muscle pain. Mol. Neurobiol. 51, 19–31. doi: 10.1007/s12035-014-8790-x
Dai, W. J., Sun, J. L., Li, C., Mao, W., Huang, Y. K., Zhao, Z. Q., et al. (2019). Involvement of interleukin-10 in analgesia of electroacupuncture on incision pain. Evid. Based Complement. Alternat. Med. 2019:8413576. doi: 10.1155/2019/8413576
Du, J., Fang, J., Wen, C., Shao, X., Liang, Y., and Fang, J. (2017). The effect of electroacupuncture on PKMzeta in the ACC in regulating anxiety-like behaviors in rats experiencing chronic inflammatory pain. Neural Plast. 2017:3728752. doi: 10.1155/2017/3728752
Du, J., Fang, J., Xu, Z., Xiang, X., Wang, S., Sun, H., et al. (2020). Electroacupuncture suppresses the pain and pain-related anxiety of chronic inflammation in rats by increasing the expression of the NPS/NPSR system in the ACC. Brain Res. 1733:146719. doi: 10.1016/j.brainres.2020.146719
Dunkley, P. R., and Dickson, P. W. (2019). Tyrosine hydroxylase phosphorylation in vivo. J. Neurochem. 149, 706–728. doi: 10.1111/jnc.14675
Fang, J. Q., Du, J. Y., Fang, J. F., Xiao, T., Le, X. Q., Pan, N. F., et al. (2018). Parameter-specific analgesic effects of electroacupuncture mediated by degree of regulation TRPV1 and P2X3 in inflammatory pain in rats. Life Sci. 200, 69–80. doi: 10.1016/j.lfs.2018.03.028
Fang, J. Q., Fang, J. F., Liang, Y., and Du, J. Y. (2014). Electroacupuncture mediates extracellular signal-regulated kinase 1/2 pathways in the spinal cord of rats with inflammatory pain. BMC Complement. Altern. Med. 14:285. doi: 10.1186/1472-6882-14-285
Finn, D. P., Haroutounian, S., Hohmann, A. G., Krane, E., Soliman, N., and Rice, A. S. (2021). Cannabinoids, the endocannabinoid system, and pain: a review of preclinical studies. Pain 162(Suppl 1), S5–S25. doi: 10.1097/j.pain.0000000000002268
Fuming, Y., Yinan, G., Nannan, Y., Lin, Y., Xue, Z., Shouhai, H., et al. (2021). ST36 acupuncture alleviates the inflammation of adjuvant-induced arthritic rats by targeting monocyte/macrophage modulation. Evid. Based Complement. Alternat. Med. 2021:9430501.
Gao, F., Xiang, H. C., Li, H. P., Jia, M., Pan, X. L., Pan, H. L., et al. (2018). Electroacupuncture inhibits NLRP3 inflammasome activation through CB2 receptors in inflammatory pain. Brain Behav. Immun. 67, 91–100. doi: 10.1016/j.bbi.2017.08.004
Gao, Y. H., Li, C. W., Wang, J. Y., Tan, L. H., Duanmu, C. L., Jing, X. H., et al. (2017). Effect of electroacupuncture on the cervicospinal P2X7 receptor/fractalkine/CX3CR1 signaling pathway in a rat neck-incision pain model. Purinergic Signal. 13, 215–225. doi: 10.1007/s11302-016-9552-1
Goldman, N., Chen, M., Fujita, T., Xu, Q., Peng, W., Liu, W., et al. (2010). Adenosine A1 receptors mediate local anti-nociceptive effects of acupuncture. Nat. Neurosci. 13, 883–888. doi: 10.1038/nn.2562
Gwak, Y. S., Hulsebosch, C. E., and Leem, J. W. (2017). Neuronal-glial interactions maintain chronic neuropathic pain after spinal cord injury. Neural Plast. 2017:2480689. doi: 10.1155/2017/2480689
Haight, E. S., Forman, T. E., Cordonnier, S. A., James, M. L., and Tawfik, V. L. (2019). Microglial modulation as a target for chronic pain: from the bench to the bedside and back. Anesth. Analg. 128, 737–746. doi: 10.1213/ANE.0000000000004033
Han, K., Zhang, A., Mo, Y., Mao, T., Ji, B., Li, D., et al. (2019). Islet-cell autoantigen 69 mediates the antihyperalgesic effects of electroacupuncture on inflammatory pain by regulating spinal glutamate receptor subunit 2 phosphorylation through protein interacting with C-kinase 1 in mice. Pain 160, 712–723. doi: 10.1097/j.pain.0000000000001450
Han, P., Liu, S., Zhang, M., Zhao, J., Wang, Y., Wu, G., et al. (2015). Inhibition of spinal interlukin-33/ST2 signaling and downstream ERK and JNK pathways in electroacupuncture analgesia in formalin mice. PLoS One 10:e0129576. doi: 10.1371/journal.pone.0129576
Hou, T., Xiang, H., Yu, L., Su, W., Shu, Y., Li, H., et al. (2019). Electroacupuncture inhibits visceral pain via adenosine receptors in mice with inflammatory bowel disease. Purinergic Signal. 15, 193–204. doi: 10.1007/s11302-019-09655-4
Hsu, H. C., Hsieh, C. L., Wu, S. Y., and Lin, Y. W. (2019). Toll-like receptor 2 plays an essential role in electroacupuncture analgesia in a mouse model of inflammatory pain. Acupunct. Med. 37, 356–364. doi: 10.1136/acupmed-2017-011469
Hu, S. S., Ho, Y. C., and Chiou, L. C. (2014). No more pain upon Gq-protein-coupled receptor activation: role of endocannabinoids. Eur. J. Neurosci. 39, 467–484. doi: 10.1111/ejn.12475
Huang, J., Zhang, X., and McNaughton, P. A. (2006). Modulation of temperature-sensitive TRP channels. Semin. Cell Dev. Biol. 17, 638–645. doi: 10.1016/j.semcdb.2006.11.002
Huang, M., Wang, X., Xing, B., Yang, H., Sa, Z., Zhang, D., et al. (2018). Critical roles of TRPV2 channels, histamine H1 and adenosine A1 receptors in the initiation of acupoint signals for acupuncture analgesia. Sci. Rep. 8:6523. doi: 10.1038/s41598-018-24654-y
Huang, M., Zhang, D., Sa, Z. Y., Xie, Y. Y., Gu, C. L., and Ding, G. H. (2012). In adjuvant-induced arthritic rats, acupuncture analgesic effects are histamine dependent: potential reasons for acupoint preference in clinical practice. Evid. Based Complement. Alternat. Med. 2012:810512. doi: 10.1155/2012/810512
Inprasit, C., and Lin, Y. W. (2020). TRPV1 responses in the cerebellum lobules V, VIa and VII using electroacupuncture treatment for inflammatory hyperalgesia in murine model. Int. J. Mol. Sci. 21:3312. doi: 10.3390/ijms21093312
Jimeno, R., Leceta, J., Martínez, C., Gutiérrez-Cañas, I., Pérez-García, S., Carrión, M., et al. (2012). Effect of VIP on the balance between cytokines and master regulators of activated helper T cells. Immunol. Cell Biol. 90, 178–186. doi: 10.1038/icb.2011.23
Kim, H. N., Kim, Y. R., Jang, J. Y., Shin, H. K., and Choi, B. T. (2012). Electroacupuncture confers antinociceptive effects via inhibition of glutamate transporter downregulation in complete freund’s adjuvant-injected rats. Evid. Based Complement. Alternat. Med. 2012:643973. doi: 10.1155/2012/643973
Kim, H. Y., Wang, J., Lee, I., Kim, H. K., Chung, K., and Chung, J. M. (2009). Electroacupuncture suppresses capsaicin-induced secondary hyperalgesia through an endogenous spinal opioid mechanism. Pain 145, 332–340. doi: 10.1016/j.pain.2009.06.035
Kim, J. H., Min, B. I., Na, H. S., and Park, D. S. (2004). Relieving effects of electroacupuncture on mechanical allodynia in neuropathic pain model of inferior caudal trunk injury in rat: mediation by spinal opioid receptors. Brain Res. 998, 230–236. doi: 10.1016/j.brainres.2003.11.045
Lai, H. C., Lin, Y. W., and Hsieh, C. L. (2019). Acupuncture-analgesia-mediated alleviation of central sensitization. Evid. Based Complement. Alternat. Med. 2019:6173412. doi: 10.1155/2019/6173412
Lee, Y. S., Lee, J. H., Lee, I. S., and Choi, B. T. (2013). Effects of electroacupuncture on spinal α-amino-3-hydroxy-5-methyl-4-isoxazole propionic acid receptor in rats injected with complete Freund’s adjuvant. Mol. Med. Rep. 8, 1130–1134. doi: 10.3892/mmr.2013.1633
Li, A., Wang, Y., Xin, J., Lao, L., Ren, K., Berman, B. M., et al. (2007). Electroacupuncture suppresses hyperalgesia and spinal Fos expression by activating the descending inhibitory system. Brain Res. 1186, 171–179. doi: 10.1016/j.brainres.2007.10.022
Li, B., Jing, L., Jia, L., Qian, T., Jianyi, C., Zhongsheng, H., et al. (2020). Acupuncture reduces pain in rats with osteoarthritis by inhibiting MCP2/CCR2 signaling pathway. Exp. Biol. Med. 245, 1722–1731. doi: 10.1177/1535370220952342
Li, Q. H., Xie, W. X., Li, X. P., Huang, K. T., Du, Z. H., Cong, W. J., et al. (2015). Adenosine A2A receptors mediate anti-inflammatory effects of electroacupuncture on synovitis in mice with collagen-induced arthritis. Evid. Based Complement. Alternat. Med. 2015:809560. doi: 10.1155/2015/809560
Li, Y., Fang, Z., Gu, N., Bai, F., Ma, Y., Dong, H., et al. (2019). Inhibition of chemokine CX3CL1 in spinal cord mediates the electroacupuncture-induced suppression of inflammatory pain. J. Pain Res. 12, 2663–2672. doi: 10.2147/JPR.S205987
Liao, H. Y., Hsieh, C. L., Huang, C. P., and Lin, Y. W. (2017). Electroacupuncture Attenuates CFA-induced inflammatory pain by suppressing Nav1.8 through S100B. TRPV1, opioid, and adenosine pathways in mice. Sci. Rep. 7:42531. doi: 10.1038/srep42531
Liu, Q., Liu, Y., Bian, J., Li, Q., and Zhang, Y. (2019). The preemptive analgesia of pre-electroacupuncture in rats with formalin-induced acute inflammatory pain. Mol. Pain 15:1744806919866529. doi: 10.1177/1744806919866529
Liu, S., Liu, W. T., Liu, Y. P., Dong, H. L., Henkemeyer, M., Xiong, L. Z., et al. (2011). Blocking EphB1 receptor forward signaling in spinal cord relieves bone cancer pain and rescues analgesic effect of morphine treatment in rodents. Cancer Res. 71, 4392–4402. doi: 10.1158/0008-5472.CAN-10-3870
Liu, S., Mi, W. L., Li, Q., Zhang, M. T., Han, P., Hu, S., et al. (2015). Spinal IL-33/ST2 signaling contributes to neuropathic pain via neuronal CaMKII-CREB and Astroglial JAK2-STAT3 cascades in mice. Anesthesiology 123, 1154–1169. doi: 10.1097/ALN.0000000000000850
Liu, Y. J., Lin, X. X., Fang, J. Q., and Fang, F. (2018). Involvement of MrgprC in electroacupuncture analgesia for attenuating CFA-induced thermal hyperalgesia by suppressing the TRPV1 pathway. Evid. Based Complement. Alternat. Med. 2018:9102107. doi: 10.1155/2018/9102107
Lu, K. W., Hsu, C. K., Hsieh, C. L., Yang, J., and Lin, Y. W. (2016). Probing the effects and mechanisms of electroacupuncture at ipsilateral or contralateral ST36-ST37 acupoints on CFA-induced inflammatory pain. Sci. Rep. 6:22123. doi: 10.1038/srep22123
Lv, Z. Y., Yang, Y. Q., and Yin, L. M. (2021). Role of purinergic signaling in acupuncture therapeutics. Am. J. Chin. Med. 49, 645–659. doi: 10.1142/S0192415X21500294
McDonald, J. L., Cripps, A. W., Smith, P. K., Smith, C. A., Xue, C. C., and Golianu, B. (2013). The anti-inflammatory effects of acupuncture and their relevance to allergic rhinitis: a narrative review and proposed model. Evid. Based Complement. Alternat. Med. 2013:591796. doi: 10.1155/2013/591796
Mingfu, L., Xiaotong, D., Xiaojing, S., Jin, J., Jinling, Z., and Ying, H. (2013). Study on the dynamic compound structure composed of mast cells, blood vessels, and nerves in rat acupoint. Evid. Based Complement. Alternat. Med. 2013:160651. doi: 10.1155/2013/160651
Muley, M. M., Krustev, E., and McDougall, J. J. (2016). Preclinical assessment of inflammatory pain. CNS Neurosci. Ther. 22, 88–101. doi: 10.1111/cns.12486
Murray, P. J. (2017). Macrophage polarization. Annu. Rev. Physiol. 79, 541–566. doi: 10.1146/annurev-physiol-022516-034339
Nasi, S., Ea, H. K., So, A., and Busso, N. (2017). Revisiting the role of interleukin-1 Pathway in osteoarthritis: interleukin-1α and -1β, and NLRP3 inflammasome are not involved in the pathological features of the murine menisectomy model of osteoarthritis. Front. Pharmacol. 8:282. doi: 10.3389/fphar.2017.00282
Nie, H., and Weng, H. R. (2009). Glutamate transporters prevent excessive activation of NMDA receptors and extrasynaptic glutamate spillover in the spinal dorsal horn. J. Neurophysiol. 101, 2041–2051. doi: 10.1152/jn.91138.2008
Old, E. A., Clark, A. K., and Malcangio, M. (2015). The role of glia in the spinal cord in neuropathic and inflammatory pain. Handb. Exp. Pharmacol. 227, 145–170. doi: 10.1007/978-3-662-46450-2_8
Pinho-Ribeiro, F. A., Verri, W. A. Jr., and Chiu, I. M. (2017). Nociceptor sensory neuron-immune interactions in pain and inflammation. Trends Immunol. 38, 5–19. doi: 10.1016/j.it.2016.10.001
Qiao, L., Guo, M., Qian, J., Xu, B., Gu, C., and Yang, Y. (2020). Research advances on acupuncture analgesia. Am. J. Chin. Med. 48, 245–258. doi: 10.1142/S0192415X20500135
Qin, Z., Wu, J., Tian, J., Zhou, J., Liu, Y., and Liu, Z. (2016). Network meta-analysis of the efficacy of acupuncture, alpha-blockers and antibiotics on chronic prostatitis/chronic pelvic pain syndrome. Sci. Rep. 6:35737. doi: 10.1038/srep35737
Raghu, H., Lepus, C. M., Wang, Q., Wong, H. H., Lingampalli, N., Oliviero, F., et al. (2017). CCL2/CCR2, but not CCL5/CCR5, mediates monocyte recruitment, inflammation and cartilage destruction in osteoarthritis. Ann. Rheum. Dis. 76, 914–922. doi: 10.1136/annrheumdis-2016-210426
Roberts, J., Ossipov, M. H., and Porreca, F. (2009). Glial activation in the rostroventromedial medulla promotes descending facilitation to mediate inflammatory hypersensitivity. Eur. J. Neurosci. 30, 229–241. doi: 10.1111/j.1460-9568.2009.06813.x
Ronchetti, S., Migliorati, G., and Delfino, D. V. (2017). Association of inflammatory mediators with pain perception. Biomed. Pharmacother. 96, 1445–1452. doi: 10.1016/j.biopha.2017.12.001
Samineni, V. K., Grajales-Reyes, J. G., Copits, B. A., O’Brien, D. E., Trigg, S. L., Gomez, A. M., et al. (2017). Divergent modulation of nociception by glutamatergic and GABAergic neuronal subpopulations in the periaqueductal gray. eNeuro 4:ENEURO.0129-16.2017. doi: 10.1523/ENEURO.0129-16.2017
Sawynok, J. (2016). Adenosine receptor targets for pain. Neuroscience 338, 1–18. doi: 10.1016/j.neuroscience.2015.10.031
Seo, B. K., Park, D. S., and Baek, Y. H. (2013). The analgesic effect of electroacupuncture on inflammatory pain in the rat model of collagenase-induced arthritis: mediation by opioidergic receptors. Rheumatol. Int. 33, 1177–1183. doi: 10.1007/s00296-012-2502-5
Seo, B. K., Sung, W. S., Park, Y. C., and Baek, Y. H. (2016). The electroacupuncture-induced analgesic effect mediated by 5-HT1, 5-HT3 receptor and muscarinic cholinergic receptors in rat model of collagenase-induced osteoarthritis. BMC Complement. Altern. Med. 16:212. doi: 10.1186/s12906-016-1204-z
Shao, X. M., Sun, J., Jiang, Y. L., Liu, B. Y., Shen, Z., Fang, F., et al. (2016). Inhibition of the cAMP/PKA/CREB pathway contributes to the analgesic effects of electroacupuncture in the anterior cingulate cortex in a rat pain memory model. Neural Plast. 2016:5320641. doi: 10.1155/2016/5320641
Shaw, D. M., Merien, F., Braakhuis, A., and Dulson, D. (2018). T-cells and their cytokine production: the anti-inflammatory and immunosuppressive effects of strenuous exercise. Cytokine 104, 136–142. doi: 10.1016/j.cyto.2017.10.001
Shou, Y., Yang, Y., Xu, M. S., Zhao, Y. Q., Ge, L. B., and Zhang, B. M. (2013). Electroacupuncture inhibition of hyperalgesia in rats with adjuvant arthritis: involvement of cannabinoid receptor 1 and dopamine receptor subtypes in striatum. Evid. Based Complement. Alternat. Med. 2013:393460. doi: 10.1155/2013/393460
Su, T. F., Zhang, L. H., Peng, M., Wu, C. H., Pan, W., Tian, B., et al. (2011). Cannabinoid CB2 receptors contribute to upregulation of β-endorphin in inflamed skin tissues by electroacupuncture. Mol. Pain 7:98. doi: 10.1186/1744-8069-7-98
Su, T. F., Zhao, Y. Q., Zhang, L. H., Peng, M., Wu, C. H., Pei, L., et al. (2012). Electroacupuncture reduces the expression of proinflammatory cytokines in inflamed skin tissues through activation of cannabinoid CB2 receptors. Eur. J. Pain 16, 624–635. doi: 10.1002/j.1532-2149.2011.00055.x
Sun, J., Zhang, H., Wang, C., Yang, M., Chang, S., Geng, Y., et al. (2017). Regulating the balance of Th17/Treg via electroacupuncture and moxibustion: an ulcerative colitis mice model based study. Evid. Based Complement. Alternat. Med. 2017:7296353. doi: 10.1155/2017/7296353
Sung, B., Lim, G., and Mao, J. (2003). Altered expression and uptake activity of spinal glutamate transporters after nerve injury contribute to the pathogenesis of neuropathic pain in rats. J. Neurosci. 23, 2899–2910.
Swartjes, M., Morariu, A., Niesters, M., Aarts, L., and Dahan, A. (2011). Nonselective and NR2B-selective N-methyl-D-aspartic acid receptor antagonists produce antinociception and long-term relief of allodynia in acute and neuropathic pain. Anesthesiology 115, 165–174. doi: 10.1097/ALN.0b013e31821bdb9b
Taguchi, R., Taguchi, T., and Kitakoji, H. (2010). Involvement of peripheral opioid receptors in electroacupuncture analgesia for carrageenan-induced hyperalgesia. Brain Res. 1355, 97–103. doi: 10.1016/j.brainres.2010.08.014
Tao, Z. Y., Wang, P. X., Wei, S. Q., Traub, R. J., Li, J. F., and Cao, D. Y. (2019). The role of descending pain modulation in chronic primary pain: potential application of drugs targeting serotonergic system. Neural Plast. 2019:1389296. doi: 10.1155/2019/1389296
Wang, H., Yang, G., Wang, S., Zheng, X., Zhang, W., and Li, Y. (2018). The most commonly treated acupuncture indications in the united states: a cross-sectional study. Am. J. Chin. Med. doi: 10.1142/S0192415X18500738 [Epub ahead of print].
Wang, Y., Gehringer, R., Mousa, S. A., Hackel, D., Brack, A., and Rittner, H. L. (2014). CXCL10 controls inflammatory pain via opioid peptide-containing macrophages in electroacupuncture. PLoS One 9:e94696. doi: 10.1371/journal.pone.0094696
Wang, Y. L., Su, Y. S., He, W., and Jing, X. H. (2019). Electroacupuncture relieved visceral and referred hindpaw hypersensitivity in colitis rats by inhibiting tyrosine hydroxylase expression in the sixth lumbar dorsal root ganglia. Neuropeptides 77:101957. doi: 10.1016/j.npep.2019.101957
Wang, Z., Chen, T., Long, M., Chen, L., Wang, L., Yin, N., et al. (2017). Electro-acupuncture at Acupoint ST36 Ameliorates Inflammation and Regulates Th1/Th2 Balance in Delayed-Type Hypersensitivity. Inflammation 40, 422–434. doi: 10.1007/s10753-016-0487-z
Wei, F., Guo, W., Zou, S., Ren, K., and Dubner, R. (2008). Supraspinal glial-neuronal interactions contribute to descending pain facilitation. J. Neurosci. 28, 10482–10495. doi: 10.1523/JNEUROSCI.3593-08.2008
Weng, W., Wang, F., He, X., Zhou, K., Wu, X., and Wu, X. (2021). Protective effect of Corynoline on the CFA induced Rheumatoid arthritis via attenuation of oxidative and inflammatory mediators. Mol. Cell. Biochem. 476, 831–839. doi: 10.1007/s11010-020-03948-8
Weng, Z. J., Wu, L. Y., Zhou, C. L., Dou, C. Z., Shi, Y., Liu, H. R., et al. (2015). Effect of electroacupuncture on P2X3 receptor regulation in the peripheral and central nervous systems of rats with visceral pain caused by irritable bowel syndrome. Purinergic Signal. 11, 321–329. doi: 10.1007/s11302-015-9447-6
Williams, A., and Craig, K. D. (2016). Updating the definition of pain. Pain 157, 2420–2423. doi: 10.1097/j.pain.0000000000000613
Wu, L. C., Weng, P. W., Chen, C. H., Huang, Y. Y., Tsuang, Y. H., and Chiang, C. J. (2018). Literature review and meta-analysis of transcutaneous electrical nerve stimulation in treating chronic back pain. Reg. Anesth. Pain Med. 43, 425–433. doi: 10.1097/AAP.0000000000000740
Wu, S. Y., Chen, W. H., Hsieh, C. L., and Lin, Y. W. (2014). Abundant expression and functional participation of TRPV1 at Zusanli acupoint (ST36) in mice: mechanosensitive TRPV1 as an “acupuncture-responding channel”. BMC Complement. Altern. Med. 14:96. doi: 10.1186/1472-6882-14-96
Wu, W., Yu, X., Luo, X. P., Yang, S. H., and Zheng, D. (2013). Tetramethylpyrazine protects against scopolamine-induced memory impairments in rats by reversing the cAMP/PKA/CREB pathway. Behav. Brain Res. 253, 212–216. doi: 10.1016/j.bbr.2013.07.052
Xiang, X., Wang, S., Shao, F., Fang, J., Xu, Y., Wang, W., et al. (2019). Electroacupuncture stimulation alleviates CFA-induced inflammatory pain via suppressing P2X3 expression. Int. J. Mol. Sci. 20:3248. doi: 10.3390/ijms20133248
Xu, Y., Hong, S., Zhao, X., Wang, S., Xu, Z., Ding, S., et al. (2018). Acupuncture alleviates rheumatoid arthritis by immune-network modulation. Am. J. Chin. Med. 46, 997–1019. doi: 10.1142/S0192415X18500520
Yang, E. J., Koo, S. T., Kim, Y. S., Lee, J. E., Hwang, H. S., Lee, M. S., et al. (2011). Contralateral electroacupuncture pretreatment suppresses carrageenan-induced inflammatory pain via the opioid-mu receptor. Rheumatol. Int. 31, 725–730. doi: 10.1007/s00296-010-1364-y
Yang, J., Hsieh, C. L., and Lin, Y. W. (2017). Role of transient receptor potential vanilloid 1 in electroacupuncture analgesia on chronic inflammatory pain in mice. Biomed. Res. Int. 2017:5068347. doi: 10.1155/2017/5068347
Yang, N. N., Yang, J. W., Ye, Y., Huang, J., Wang, L., Wang, Y., et al. (2021). Electroacupuncture ameliorates intestinal inflammation by activating α7nAChR-mediated JAK2/STAT3 signaling pathway in postoperative ileus. Theranostics 11, 4078–4089. doi: 10.7150/thno.52574
Yang, Y., Yan, M., Zhang, H., and Wang, X. (2013). Substance P participates in immune-mediated hepatic injury induced by concanavalin A in mice and stimulates cytokine synthesis in Kupffer cells. Exp. Ther. Med. 6, 459–464. doi: 10.3892/etm.2013.1152
Yoo, Y. C., Oh, J. H., Kwon, T. D., Lee, Y. K., and Bai, S. J. (2011). Analgesic mechanism of electroacupuncture in an arthritic pain model of rats: a neurotransmitter study. Yonsei Med. J. 52, 1016–1021. doi: 10.3349/ymj.2011.52.6.1016
Yu, M. L., Wei, R. D., Zhang, T., Wang, J. M., Cheng, Y., Qin, F. F., et al. (2020). Electroacupuncture relieves pain and attenuates inflammation progression through inducing IL-10 Production in CFA-Induced Mice. Inflammation 43, 1233–1245. doi: 10.1007/s10753-020-01203-2
Yuan, X. C., Wang, Q., Su, W., Li, H. P., Wu, C. H., Gao, F., et al. (2018a). Electroacupuncture potentiates peripheral CB2 receptor-inhibited chronic pain in a mouse model of knee osteoarthritis. J. Pain Res. 11, 2797–2808. doi: 10.2147/JPR.S171664
Yuan, X. C., Zhu, B., Jing, X. H., Xiong, L. Z., Wu, C. H., Gao, F., et al. (2018b). Electroacupuncture potentiates cannabinoid receptor-mediated descending inhibitory control in a mouse model of knee osteoarthritis. Front. Mol. Neurosci. 11:112. doi: 10.3389/fnmol.2018.00112
Zeng, J., Li, S., Zhang, C., Huang, G., and Yu, C. (2018). The mechanism of hyperalgesia and anxiety induced by remifentanil: phosphorylation of GluR1 receptors in the anterior cingulate cortex. J. Mol. Neurosci. 65, 93–101. doi: 10.1007/s12031-018-1072-8
Zhang, F., Zhou, H., Ding, S., Zhang, D., Lian, D., Chen, X., et al. (2020a). Efficacy and safety of acupuncture for recurrent aphthous stomatitis: a systematic review protocol. BMJ Open 10:e037603. doi: 10.1136/bmjopen-2020-037603
Zhang, G. G., Yu, C., Lee, W., Lao, L., Ren, K., and Berman, B. M. (2005). Involvement of peripheral opioid mechanisms in electroacupuncture analgesia. Explore 1, 365–371. doi: 10.1016/j.explore.2005.06.006
Zhang, J., Chen, L., Su, T., Cao, F., Meng, X., Pei, L., et al. (2010). Electroacupuncture increases CB2 receptor expression on keratinocytes and infiltrating inflammatory cells in inflamed skin tissues of rats. J. Pain 11, 1250–1258. doi: 10.1016/j.jpain.2010.02.013
Zhang, K., Zhao, X., Ding, S., Liu, Y., Xu, Y., Yan, Y., et al. (2020b). Applying complex network and cell-cell communication network diagram methods to explore the key cytokines and immune cells in local acupoint involved in acupuncture treating inflammatory pain. Evid. Based Complement. Alternat. Med. 2020:2585960.
Zhang, K., Zhao, X., Yang, T., Zhang, Y. F., Yan, Y. W., Xu, Z. F., et al. (2020c). Initiation of acupoint molecular mechanisms for manual acupuncture analgesia-nuclear factor κB signaling pathway. Chin. J. Integr. Med. doi: 10.1007/s11655-020-3435-6 [Epub ahead of print].
Zhang, L., Berta, T., Xu, Z. Z., Liu, T., Park, J. Y., and Ji, R. R. (2011a). TNF-α contributes to spinal cord synaptic plasticity and inflammatory pain: distinct role of TNF receptor subtypes 1 and 2. Pain 152, 419–427. doi: 10.1016/j.pain.2010.11.014
Zhang, Q., Yue, J., Sun, Z., and Lu, Y. (2016). Acupuncture for chronic knee pain: a protocol for an updated systematic review. BMJ Open 6:e008027. doi: 10.1136/bmjopen-2015-008027
Zhang, R., Lao, L., Ren, K., and Berman, B. M. (2014). Mechanisms of acupuncture-electroacupuncture on persistent pain. Anesthesiology 120, 482–503. doi: 10.1097/ALN.0000000000000101
Zhang, R. X., Lao, L., Wang, L., Liu, B., Wang, X., Ren, K., et al. (2004). Involvement of opioid receptors in electroacupuncture-produced anti-hyperalgesia in rats with peripheral inflammation. Brain Res. 1020, 12–17. doi: 10.1016/j.brainres.2004.05.067
Zhang, R. X., Lao, L., Wang, X., Fan, A., Wang, L., Ren, K., et al. (2005). Electroacupuncture attenuates inflammation in a rat model. J. Altern. Complement. Med. 11, 135–142. doi: 10.1089/acm.2005.11.135
Zhang, R. Y., Zhu, B. F., Wang, L. K., Song, Y., Zhao, J. G., Guo, Y., et al. (2020d). Electroacupuncture alleviates inflammatory pain via adenosine suppression and its mediated substance P expression. Arq. Neuropsiquiatr. 78, 617–623. doi: 10.1590/0004-282X20200078
Zhang, Y., Li, A., Lao, L., Xin, J., Ren, K., Berman, B. M., et al. (2011b). Rostral ventromedial medulla μ, but not κ, opioid receptors are involved in electroacupuncture anti-hyperalgesia in an inflammatory pain rat model. Brain Res. 1395, 38–45. doi: 10.1016/j.brainres.2011.04.037
Zhang, Y., Li, A., Xin, J., Lao, L., Ren, K., Berman, B. M., et al. (2011c). Involvement of spinal serotonin receptors in electroacupuncture anti-hyperalgesia in an inflammatory pain rat model. Neurochem. Res. 36, 1785–1792. doi: 10.1007/s11064-011-0495-1
Zhang, Y., Meng, X., Li, A., Xin, J., Berman, B. M., Lao, L., et al. (2012a). Electroacupuncture alleviates affective pain in an inflammatory pain rat model. Eur. J. Pain 16, 170–181. doi: 10.1016/j.ejpain.2011.07.002
Zhang, Y., Zhang, R. X., Zhang, M., Shen, X. Y., Li, A., Xin, J., et al. (2012b). Electroacupuncture inhibition of hyperalgesia in an inflammatory pain rat model: involvement of distinct spinal serotonin and norepinephrine receptor subtypes. Br. J. Anaesth. 109, 245–252. doi: 10.1093/bja/aes136
Zhang, Z. J., Jiang, B. C., and Gao, Y. J. (2017). Chemokines in neuron-glial cell interaction and pathogenesis of neuropathic pain. Cell. Mol. Life Sci. 74, 3275–3291. doi: 10.1007/s00018-017-2513-1
Zhou, Q. Q., Imbe, H., Zou, S., Dubner, R., and Ren, K. (2001). Selective upregulation of the flip-flop splice variants of AMPA receptor subunits in the rat spinal cord after hindpaw inflammation. Mol. Brain Res. 88, 186–193. doi: 10.1016/s0169-328x(01)00041-9
Zhu, H., Xiang, H. C., Li, H. P., Lin, L. X., Hu, X. F., Zhang, H., et al. (2019). Inhibition of GABAergic neurons and excitation of glutamatergic neurons in the ventrolateral periaqueductal gray participate in electroacupuncture analgesia mediated by cannabinoid receptor. Front. Neurosci. 13:484. doi: 10.3389/fnins.2019.00484
Zhu, J., Chen, X. Y., Li, L. B., Yu, X. T., Zhou, Y., Yang, W. J., et al. (2015). Electroacupuncture attenuates collagen-induced arthritis in rats through vasoactive intestinal peptide signalling-dependent re-establishment of the regulatory T cell/T-helper 17 cell balance. Acupunct. Med. 33, 305–311. doi: 10.1136/acupmed-2014-010732
Keywords: acupuncture, inflammatory pain, analgesia, neuroimmune crosstalk, anti-inflammation
Citation: Dou B, Li Y, Ma J, Xu Z, Fan W, Tian L, Chen Z, Li N, Gong Y, Lyu Z, Fang Y, Liu Y, Xu Y, Wang S, Chen B, Guo Y, Guo Y and Lin X (2021) Role of Neuroimmune Crosstalk in Mediating the Anti-inflammatory and Analgesic Effects of Acupuncture on Inflammatory Pain. Front. Neurosci. 15:695670. doi: 10.3389/fnins.2021.695670
Received: 15 April 2021; Accepted: 05 July 2021;
Published: 02 August 2021.
Edited by:
Man Li, Huazhong University of Science and Technology, ChinaReviewed by:
Yong Tang, Chengdu University of Traditional Chinese Medicine, ChinaCopyright © 2021 Dou, Li, Ma, Xu, Fan, Tian, Chen, Li, Gong, Lyu, Fang, Liu, Xu, Wang, Chen, Guo, Guo and Lin. This is an open-access article distributed under the terms of the Creative Commons Attribution License (CC BY). The use, distribution or reproduction in other forums is permitted, provided the original author(s) and the copyright owner(s) are credited and that the original publication in this journal is cited, in accordance with accepted academic practice. No use, distribution or reproduction is permitted which does not comply with these terms.
*Correspondence:
Disclaimer: All claims expressed in this article are solely those of the authors and do not necessarily represent those of their affiliated organizations, or those of the publisher, the editors and the reviewers. Any product that may be evaluated in this article or claim that may be made by its manufacturer is not guaranteed or endorsed by the publisher.
Research integrity at Frontiers
Learn more about the work of our research integrity team to safeguard the quality of each article we publish.