- 1Duke Molecular Physiology Institute, Duke University Medical Center, Durham, NC, United States
- 2Queensland University of Technology, Centre for Genomics and Personalised Health, Faculty of Health, Institute of Health and Biomedical Innovation, Kelvin Grove, QLD, Australia
- 3Durham Veterans Affairs Health Care System, Durham, NC, United States
- 4VA Mid-Atlantic Mental Illness Research, Education, and Clinical Center, Durham, NC United States
- 5Department of Psychiatry and Behavioral Sciences, Duke University School of Medicine, Durham, NC, United States
- 6Department of Neurosurgery, Stanford University School of Medicine, Stanford, CA, United States
- 7Gallipoli Medical Research Foundation, Greenslopes Private Hospital, Greenslopes, QLD, Australia
- 8Faculty of Health, Queensland University of Technology, Kelvin Grove, QLD, Australia
- 9Department of Psychiatry, School of Medicine, University of California, San Diego, La Jolla, CA, United States
- 10Herbert Wertheim School of Public Health, University of California, San Diego, La Jolla, CA, United States
- 11VA San Diego Healthcare System, San Diego, CA, United States
Posttraumatic stress disorder (PTSD) is a complex psychiatric disorder that can develop following exposure to traumatic events. The Psychiatric Genomics Consortium PTSD group (PGC-PTSD) has collected over 20,000 multi-ethnic PTSD cases and controls and has identified both genetic and epigenetic factors associated with PTSD risk. To further investigate biological correlates of PTSD risk, we examined three PGC-PTSD cohorts comprising 977 subjects to identify differentially expressed genes among PTSD cases and controls. Whole blood gene expression was quantified with the HumanHT-12 v4 Expression BeadChip for 726 OEF/OIF veterans from the Veterans Affairs (VA) Mental Illness Research Education and Clinical Center (MIRECC), 155 samples from the Injury and Traumatic Stress (INTRuST) Clinical Consortium, and 96 Australian Vietnam War veterans. Differential gene expression analysis was performed in each cohort separately followed by meta-analysis. In the largest cohort, we performed co-expression analysis to identify modules of genes that are associated with PTSD and MDD. We then conducted expression quantitative trait loci (eQTL) analysis and assessed the presence of eQTL interactions involving PTSD and major depressive disorder (MDD). Finally, we utilized PTSD and MDD GWAS summary statistics to identify regions that colocalize with eQTLs. Although not surpassing correction for multiple testing, the most differentially expressed genes in meta-analysis were interleukin-1 beta (IL1B), a pro-inflammatory cytokine previously associated with PTSD, and integrin-linked kinase (ILK), which is highly expressed in brain and can rescue dysregulated hippocampal neurogenesis and memory deficits. Pathway analysis revealed enrichment of toll-like receptor (TLR) and interleukin-1 receptor genes, which are integral to cellular innate immune response. Co-expression analysis identified four modules of genes associated with PTSD, two of which are also associated with MDD, demonstrating common biological pathways underlying the two conditions. Lastly, we identified four genes (UBA7, HLA-F, HSPA1B, and RERE) with high probability of a shared causal eQTL variant with PTSD and/or MDD GWAS variants, thereby providing a potential mechanism by which the GWAS variant contributes to disease risk. In summary, we provide additional evidence for genes and pathways previously reported and identified plausible novel candidates for PTSD. These data provide further insight into genetic factors and pathways involved in PTSD, as well as potential regions of pleiotropy between PTSD and MDD.
Introduction
Posttraumatic stress disorder (PTSD) is a common psychiatric disorder that can occur following exposure to traumatic events. It is characterized by re-experiencing symptoms, avoidance, and persistent hyperarousal. While 7–8% of adults in the United States will experience PTSD over the course of their lifetime (Kessler et al., 2005; Roberts et al., 2011; Kilpatrick et al., 2013), rates are much higher among military veterans. The prevalence of PTSD in veterans returning from Iraq/Afghanistan is estimated to be 23% (Fulton et al., 2015), whereas an estimated 30% of Vietnam veterans have experienced lifetime PTSD (Kulka et al., 1990). Individuals with PTSD are at increased risk for many other comorbid conditions including major depressive disorder (MDD) (Cougle et al., 2010; Rytwinski et al., 2013; Flory and Yehuda, 2015; Ben Barnes et al., 2018), substance abuse disorder (SUD) (Petrakis et al., 2011; Seal et al., 2011; Blanco et al., 2013; Stein et al., 2017; Teeters et al., 2017), sleep disorders (Pigeon et al., 2013; Lind et al., 2020), and cardiovascular disease (Pollard et al., 2016; Koenen et al., 2017). Individuals with PTSD are also at increased risk for suicidal behaviors, particularly if they experience comorbid MDD (Oquendo et al., 2005; Panagioti et al., 2012; Kimbrel et al., 2016; Livingston et al., 2020; Ursano et al., 2020). For example, Kimbrel et al. (2016) found that among Iraq/Afghanistan-era war veterans, comorbid PTSD-depression was a robust prospective predictor of future suicide attempts over a 12-month period, even after controlling for the effects of sex, age, race, sexual orientation, and lifetime history of suicide attempts. Given the rate of death by suicide among veterans is approximately 1.5 times the rate among non-veteran adults (Department of Veterans Affairs, Office of Mental Health & Suicide Prevention, 20201), it is clear that an improved understanding of the etiology of PTSD and depression is of utmost importance.
While most people experience at least one traumatic event in their life, only some will subsequently develop PTSD (Benjet et al., 2016; Liu et al., 2017), suggesting that a heritable component to risk for PTSD exists. Based on family and twin studies, genetic susceptibility accounts for an estimated 30–70% of the variance in PTSD risk (True et al., 1993; Yehuda et al., 2001; Stein et al., 2002; Wolf et al., 2014). There is also evidence for a shared heritable influence on PTSD and MDD (Sartor et al., 2012). Utilizing over 20,000 individuals from 11 studies, the Psychiatric Genomics Consortium-Posttraumatic Stress Disorder group (PGC-PTSD) recently reported a single nucleotide polymorphism (SNP) heritability estimate of 29% for European-American females and found evidence for overlapping genetic risk between PTSD and schizophrenia, bipolar disorder, and MDD (Duncan et al., 2018). Even with large sample sizes, it has been difficult to identify robust regions of association with PTSD, illustrating the complex and multi-genic nature of the disorder. Recent GWAS reports from the PGC-PTSD and Million Veteran Program (MVP) have had greater success in identifying PTSD-associated loci when utilizing quantitative symptom severity and symptom subdomains instead of PTSD diagnosis (Maihofer et al., submitted; Stein et al., 2021). Nonetheless, the biological mechanisms underlying these largely non-coding regions of association have not yet been elucidated.
Gene expression profiling has been widely used to identify genes and pathways that are associated with specific biological processes and to study related molecular mechanisms. A recent transcriptome-wide study in prefrontal cortex (PFC) of post-mortem human brains identified co-regulated gene networks that are altered in PTSD, including differences between men and women which could help explain the increased prevalence of PTSD among women (Girgenti et al., 2021). However, due to the extremely limited availability of human brain tissue from PTSD-affected donors, the majority of studies have focused on gene expression in human peripheral blood samples. Although this is a limitation, evidence from both human and animal studies indicate it is likely that changes in the blood transcriptome reflect at least some changes occurring in the brain. For example, inflammation in the periphery has been associated with global brain transcriptome changes in mouse (Thomson et al., 2014). In humans, Huckins et al. (2020) showed that genetically regulated transcriptomic changes in the brain correlate with measured gene expression changes in peripheral blood.
Many previous gene expression studies of peripheral blood in PTSD patients and controls have reported that alterations in immune and inflammatory response pathways are important in PTSD pathogenesis, specifically the hypothalamic-pituitary-adrenal (HPA) axis and glucocorticoid (GC) function (Segman et al., 2005). For example, FK506 binding protein 5 (FKBP5), a GC receptor inhibitor, is downregulated in PTSD cases (Yehuda et al., 2009). This finding, along with other genes in the GC receptor signaling pathway, has been widely replicated across various trauma types (Mehta et al., 2011, 2018; Sarapas et al., 2011; van Zuiden et al., 2012; Logue et al., 2015; Kuan et al., 2017). Others have reported differentially expressed genes in pathways enriched for innate immunity and inflammatory response (Zieker et al., 2007; Neylan et al., 2011; Breen et al., 2015, 2018; Guardado et al., 2016; Rusch et al., 2019). Additionally, decreased interleukin 1A (IL1A) expression was observed in the dorsolateral prefrontal cortex (dlPFC) of post-mortem PTSD brains (Morrison et al., 2019), a finding that is concordant with reports of altered cytokine expression in peripheral blood of PTSD patients (Zieker et al., 2007; Mehta et al., 2011; Breen et al., 2018).
Taken together, the findings described above suggest that altered transcripts in peripheral blood can still provide meaningful insights into the pathophysiology of PTSD. However, most of the studies to date have relied upon small sample sizes and did not include subjects from diverse ancestry groups. Accordingly, the objective of the current study was to conduct the largest meta-analysis of differential PTSD gene expression to date (n = 977), including 383 PTSD cases and 594 trauma-exposed controls from three multi-ethnic cohorts. Additional analyses aimed at uncovering genetic regulators of expression and modules of co-expression were performed in the largest cohort (n = 726). Finally, we sought to explain previously reported PTSD and MDD GWAS regions by alterations in gene expression.
Materials and Methods
Study Participants
Nine hundred seventy-seven subjects with available gene expression, genotype, and clinical phenotype data were selected from three independent PGC-PTSD cohorts: veterans returning from Iraq/Afghanistan from the Veterans Affairs (VA) VISN-6 Mid-Atlantic Mental Illness Research Education and Clinical Center (MIRECC) as well as community civilians and all-era veterans enrolled in other trauma research studies at the Durham VA Health Care System and Duke University Medical Center (MIRECC/Duke; n = 726), the Injury and Traumatic Stress (INTRuST) Clinical Consortium (n = 155), and Australian Vietnam War veterans (GMFR-QUT; n = 96), which have been described previously (Ashley-Koch et al., 2015; McLeay et al., 2017; Akosile et al., 2018; Bomyea et al., 2019, 2020).
Posttraumatic stress disorder diagnosis for the MIRECC/Duke cohort was determined using either the Structured Clinical Interview for DSM-IV Disorders (SCID; First et al., 1994) or the Clinician-Administered PTSD Scale (CAPS; Blake et al., 1995), whereas PTSD severity was measured using the Davidson Trauma Scale (DTS; Davidson et al., 1997). For the INTRuST consortium, PTSD diagnosis was determined using either the PTSD Checklist-Civilian Version (PCL-C; Weathers et al., 1993), the CAPS (Blake et al., 1995), or the MINI International Neuropsychiatric Interview 6.0.0 (MINI; Sheehan et al., 1998), and for the GMRF-QUT cohort, PTSD diagnosis was determined with the CAPS-5 (Weathers et al., 2001). While the CAPS and SCID are considered gold standard interviews for PTSD, the MINI (compared to the SCID) has demonstrated a sensitivity of 0.85 and specificity of 0.96 for PTSD (Sheehan et al., 1998). Diagnosis based on a PCL cutoff of 50 (as used in the INTRuST cohort) has a sensitivity of 0.52 and a specificity of 0.94 for PTSD compared to the CAPS (Yeager et al., 2007). MDD was assessed in the MIRECC/Duke cohort using the SCID (First et al., 1994), whereas in the INTRuST consortium, depression was assessed using the Patient Health Questionnaire-9 (PHQ9; Kroenke et al., 2001) and scores ≥ 15 indicated the subject had MDD. For the GMRF-QUT cohort, MDD was assessed with the MINI (Sheehan et al., 1998). While the SCID is a gold standard interview for MDD, the PHQ-9 has established reliability and validity to detect a high probability of MDD. Using the Mental Health Professional Validation Interview as the criterion standard, a PHQ-9 score ≥ 15 (as used in the INTRuST consortium) had a sensitivity of 0.68 and a specificity of 0.95 for MDD (Kroenke et al., 2001). The MINI, which was used in the GMRF-QUT cohort, demonstrated a sensitivity of 0.96 and a specificity of 0.88 compared to the SCID (Sheehan et al., 1998). Smoking status for participants in each cohort was determined using study-specific questionnaires. Each study received approval from their respective ethics committee or institutional review board (IRB), and informed consent was obtained from each study participant prior to data collection.
Gene Expression Microarrays
Gene expression data was generated in the three cohorts separately using HumanHT-12 v4 Expression BeadChips (Illumina Inc., San Diego, CA, United States), which capture 47,231 expression probes. For the MIRECC/Duke and INTRuST samples, whole blood was collected in PAXgene blood RNA tubes and incubated at room temperature overnight before being transferred to −20°C for 24 h and finally stored at −80°C. Total RNA was extracted using the PAXgene Blood RNA System Kit following manufacturer’s guidelines (Qiagen, Germantown, MD, United States). Purified RNA was analyzed for integrity (RIN) using the Agilent Bioanalyzer 2100 with Agilent RNA 6000 LabChip kits (Agilent, Santa Clara, CA, United States) and only samples with RIN ≥ 6 were included in downstream analyses. All RNA samples were processed using the Ambion GLOBINclear-Human Globin mRNA Removal Kit to deplete alpha and beta globin mRNA and to increase sensitivity of gene detection (Life Technologies, Foster City, CA, United States). The enriched RNA was amplified and biotin-labeled using the Illumina TotalPrep Amplification Kit before hybridization to the microarrays. Details regarding the generation of gene expression data for the GMRF-QUT cohort have been described previously (Mehta et al., 2017, 2018).
Single Nucleotide Polymorphism Genotyping and Imputation
Information on SNP genotyping and imputation for all three cohorts have been previously described (Ashley-Koch et al., 2015; Mehta et al., 2017; Nievergelt et al., 2019). Briefly, DNA was hybridized to three different bead chips in three different batches for the MIRECC/Duke samples: HumanHap650 BeadChip, Human1M-Duo BeadChip, and HumanOmni2.5 BeadChip (Illumina, San Diego, CA, United States). Resulting genotypes were then merged and imputed using a global reference panel from 1000Genomes (1000 Genomes Project Consortium et al., 2015). For GMRF-QUT, genotypes were obtained from the Infinium PsychArray (Illumina Inc., San Diego, CA, United States) and were imputed with the 1000Genomes phase 3 reference panel on the Michigan Imputation Server (imputationserver.sph.umich.edu). INTRuST samples were genotyped using the Infinium PsychArray (Illumina Inc., San Diego, CA, United States) and were imputed with IMPUTE2 (Howie et al., 2009) using the 1000Genomes phase 3 reference panel. Principal component analysis (PCA) of SNPs from each cohort merged with hapmap3 data (International HapMap 3 Consortium et al., 2010) was performed and resulting PCs were plotted to visualize the population substructure present within each cohort using R.
Statistical Analysis
Sample characteristics were assessed for differences by cohort using SAS v9.4 (SAS Institute, Cary, NC, United States). Raw gene expression data was processed and quality control measures were taken for each cohort separately in R. For the MIRECC/Duke and INTRuST cohorts, probes below the detection threshold (detection p > 0.05) for more than half of the samples were removed. Background adjustment, log2 transformation, and quantile normalization were performed using limma (Ritchie et al., 2015). PCA was performed to check for batch effects, which were subsequently corrected using ComBat (Johnson et al., 2007). For GMRF-QUT, data were transformed and normalized using the variance stabilizing normalization (Huber et al., 2002), and probes detected in ≥5% of samples (detection p-value < 0.05) were retained for analysis (Mehta et al., 2017). For all cohorts, the proportion of monocytes and lymphocytes for each sample was estimated using an approach described in Logue et al. (2015). The following number of probes were available for analysis in each cohort: 17,776 for MIRECC/Duke, 13,586 for INTRuST, and 12,613 for GMRF-QUT. Differential gene expression analysis for PTSD was performed with a common pipeline in each cohort separately controlling for age, principal components (PCs), sex (if applicable), smoking status, and cell proportions using limma, followed by meta-analysis of the 11,502 overlapping probes using the inverse normal method (Marot et al., 2009; Ratanatharathorn et al., 2017). Inflation and bias were controlled using the empirical null distribution (van Iterson et al., 2017) and multiple testing was corrected using false discovery rate (FDR; Storey and Tibshirani, 2003). Pathway analysis was performed using DAVID (Huang da et al., 2009a,b). Unique genes corresponding to gene expression probes with meta-analysis p < 0.05 were used as the gene list of interest and all unique genes corresponding to the 11,502 gene expression probes common to all three cohorts were included as the background gene list. Finally, a volcano plot depicting the differential expression meta-analysis p-values and log fold changes obtained from the MIRECC/Duke cohort, which is the largest of the three cohorts included, was generated using R.
We next performed an expression quantitative trait loci (eQTL) analysis in the MIRECC/Duke subjects, stratified by ethnicity, using fastQTL (Ongen et al., 2016). Specifically, each gene expression probe was regressed on SNP genotypes within a 1MB window, using an additive genetic model and controlling for 10 PCs from PCA of normalized gene expression values and 10 PCs from PCA of SNP data. Only the most significant SNP for each probe was retained. Using the most significant SNP for each probe, we then tested for interactions with current PTSD status, controlling for the same 20 PCs listed above, in PLINK. Likewise, we interrogated SNP by current MDD interactions using the same approach.
Utilizing summary statistics from the most recent PGC-PTSD GWAS (Maihofer et al., submitted) and MDD GWAS (Wray et al., 2018), we tested for colocalization with eQTLs identified in 309 non-Hispanic white (NHW) MIRECC/Duke subjects. There were 20 GWAS-associated regions for PTSD and 44 regions for MDD. All eQTLs involving a gene which resides in these 64 regions were interrogated for evidence of a shared causal variant with SNPs from the PTSD and MDD GWAS studies using coloc (Giambartolomei et al., 2014), a Bayesian method that investigates five possible hypotheses: no association (H0), association with PTSD (MDD) but not gene expression (H1), association with gene expression but not PTSD (MDD) (H2), two distinct causal variants, each associated with one trait (H3), or a shared causal variant that is associated with both PTSD (MDD) and gene expression (H4). Regions with posterior probability for H4 (PPH4) > 0.90 were deemed to co-localize.
To identify modules of co-regulated gene expression, weighted correlation network analysis was performed with WGCNA (Zhang and Horvath, 2005; Langfelder and Horvath, 2008) in all MIRECC/Duke subjects (n = 726) and subsequently in 309 NHW MIRECC/Duke subjects and 417 non-Hispanic black (NHB) MIRECC/Duke subjects separately. Probes missing for > 15% of samples were removed. For genes with > 1 expression probe, only the most variable probe was retained. Finally, the distribution of probe variability was inspected and the 50% most variable probes were included in WGCNA (n = 5,070 probes for all subjects, n = 5,187 probes for NHW subjects, and n = 4,993 probes for NHB subjects). A soft-threshold parameter was chosen to approximate scale-free topology, modules were detected with a minimum size = 30, and resulting modules were merged when the correlation coefficient was ≥ 0.75. Logistic regression was used to test for association between each module eigengene and current PTSD, current MDD, and current smoking status, controlling for age, sex, PCs, and cell type estimates. Current smoking status was included as a covariate in the models for current PTSD and current MDD. Total DTS score was also tested for association with each module eigengene controlling for the same covariates and assuming a zero-inflated negative binomial distribution. Pathway analysis using DAVID was performed on resulting co-expression modules with module members as the gene list of interest and all genes input into WGCNA (described above) as the background gene list.
Results
A description of sample characteristics by cohort is shown in Table 1. Subjects from GMRF-QUT were significantly older, exclusively male, more likely to be non-smokers, and had a higher percentage of PTSD compared to those from MIRECC/Duke or INTRuST. The percentage of MDD in the MIRECC/Duke cohort was higher compared to INTRuST and GMRF-QUT. PTSD and MDD were significantly correlated in all three cohorts (p ≤ 0.0001) and Cramer’s V between PTSD and MDD was highest among INTRuST subjects (VGMRF–QUT = 0.38, VMIRECC/Duke = 0.43, VINTRuST = 0.53). The MIRECC/Duke subset utilized in this analysis was comprised of 309 NHW and 417 NHB subjects. PCA plots of each cohort overlaid with HapMap3 samples show that the GMRF-QUT samples were primarily of European descent, whereas the INTRuST samples were multi-ethnic (Supplementary Figures 1–3).
Despite these differences in cohort characteristics, meta-analysis revealed 558 genes that were differentially expressed between PTSD cases and controls (p < 0.05, Supplementary Table 1). Inflation and bias were sufficiently controlled (λGC = 1.1). Meta-analysis p-values and effect sizes from the largest cohort (MIRECC/Duke) are depicted in Figure 1. For nominally significant probes (p < 0.05), the direction of effect is concordant 91% of the time for MIRECC/Duke and INTRuST, and is concordant 72% of the time for MIRECC/Duke and GMRF-QUT. Although not surpassing correction for multiple testing, the most significant genes were interleukin-1 beta (IL1B; p = 2.15 × 10–5), and integrin-linked kinase (ILK; p = 1.10 × 10–4). PTSD cases displayed higher expression of IL1B compared to controls, whereas ILK expression was lower in PTSD cases compared to controls. Pathway analysis of all nominally associated genes (p < 0.05) revealed an enrichment of genes involved in Toll-interleukin 1 resistance (SM00255:TIR; p = 7.01 × 10–4), including TLR5, TLR6, TLR8, TLR10, IL1RAP, and IL18RAP, which represent seven unique expression probes that were all upregulated in PTSD cases.
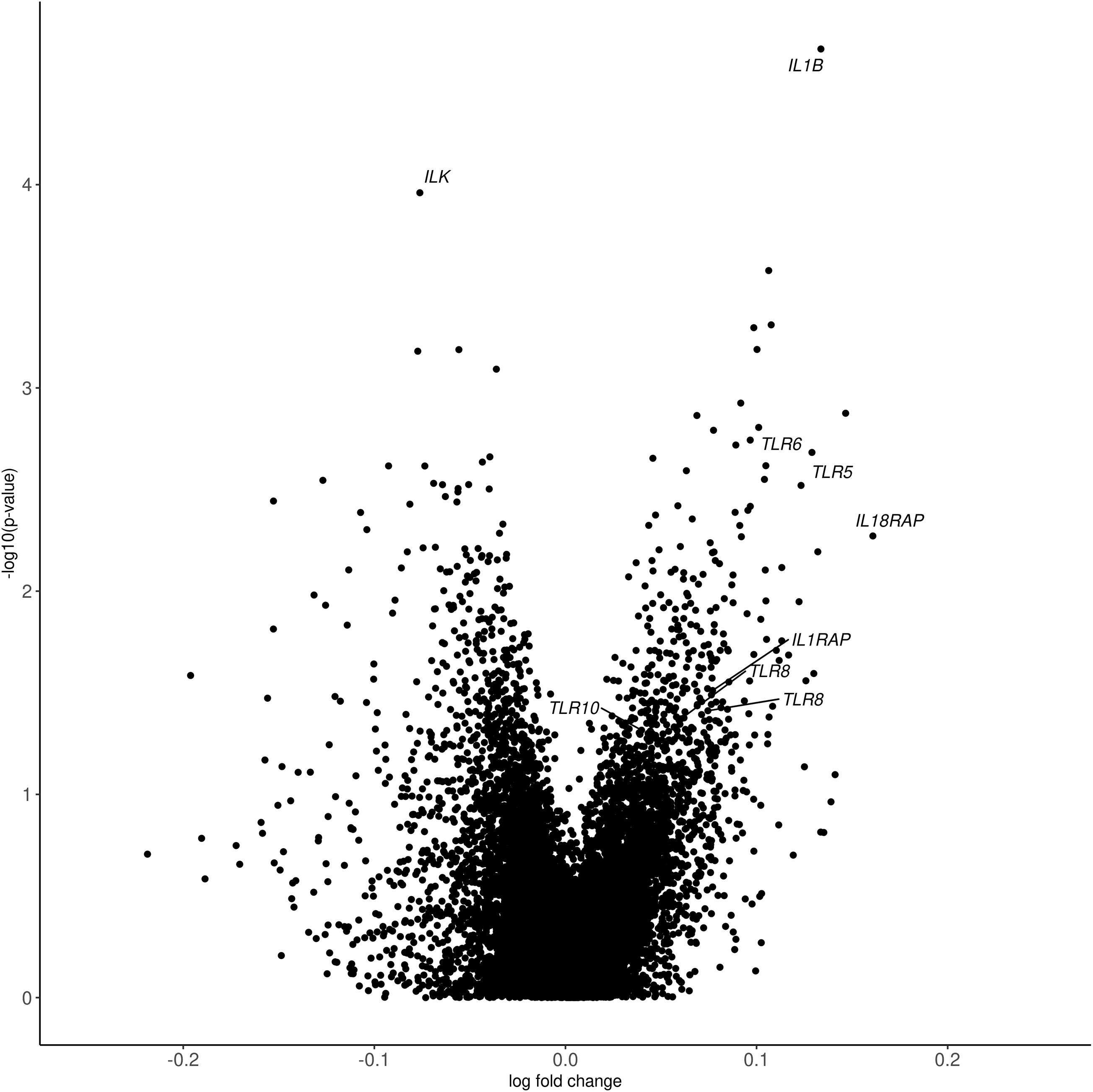
Figure 1. Volcano plot depicting differential gene expression p-values from meta-analysis and log fold change from the MIRECC/Duke cohort. In each cohort, differences in gene expression between PTSD cases and controls were interrogated controlling for age, PCs, sex (except in GMRF-QUT, which was exclusively male), smoking status, and cell proportions. The most significant genes (IL1B and ILK) and those from the enriched Toll-interleukin 1 resistance pathway (SM00255:TIR; TLR5, TLR6, TLR8, TLR10, IL1RAP, and IL18RAP) are labeled.
Quantitative trait loci analysis identified many SNPs associated with expression levels: 2,216 in the NHW MIRECC/Duke subset and 1018 in the NHB subset (FDR q < 0.05). Subsequently, we performed an interaction analysis and identified several SNPs that were associated with gene expression at levels surpassing correction for multiple testing, but only in those with either PTSD or MDD. In the NHB subset, rs28842268 was associated with ILMN_1764090 in AK3L1 (AK4), but only among those with PTSD (p = 7.9 × 10–6, q = 0.0473, Supplementary Figure 4A). Likewise, in the NHB subset, rs11823726 was associated with ILMN_1759789 in KAT5, but only among those with PTSD (p = 8.24 × 10–6, q = 0.0473, Supplementary Figure 4B). In the NHW subset, we observed two eQTLs that were significant only in those with MDD. rs28536123 was associated with ILMN_2134888 in TUBE1 (p = 3.43 × 10–9, q = 4.25 × 10–5, Supplementary Figure 4C) and rs687562 was associated with ILMN_1872122 in LCOR (p = 1.63 × 10–6, q = 0.0101, Supplementary Figure 4D), but only among those with MDD. These results should be viewed as preliminary due to small sample size and require replication in independent cohorts.
Next, Bayesian colocalization analysis was used to detect regions with high probability of a shared causal variant for PTSD or MDD and gene expression (Table 2). One eQTL in Ubiquitin Like Modifier Activating Enzyme 7 (UBA7; PPH4 = 0.9604) colocalized with PTSD associated variants, whereas two eQTLs were found to colocalize with MDD associated variants: one in HLA-F (PPH4 = 0.9844) and one in HSPA1B (PPH4 = 0.9509). Several other regions displayed suggestive evidence for colocalization, including two separate eQTLs in RERE (PPH4 = 0.8757 and 0.8488) with MDD associated variants. Interestingly, the eQTL in UBA7 deemed to colocalize with PTSD associated variants also showed suggestive evidence for colocalization with MDD associated variants (PPH4 = 0.8178).
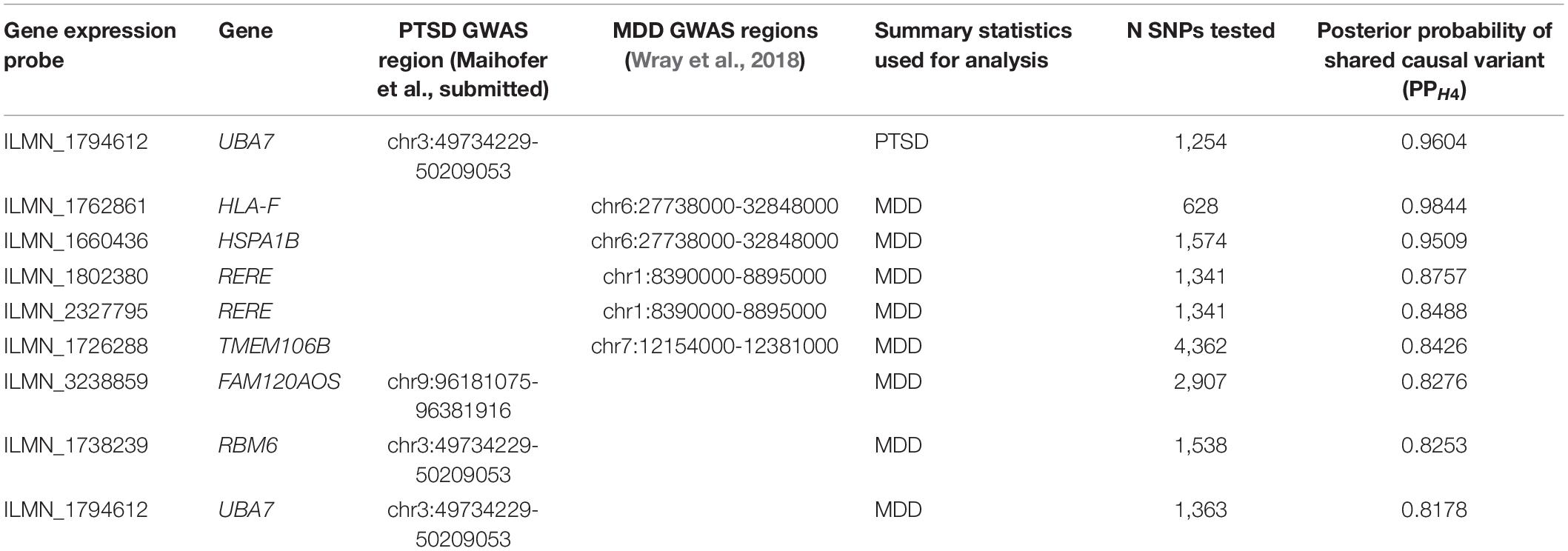
Table 2. Colocalization of eQTLs in NHW MIRECC/Duke subset and either PTSD or MDD associated GWAS regions (PPH4 > 0.8).
Weighted gene co-expression analysis in all MIRECC/Duke subjects identified 17 modules, none of which were associated with PTSD. When stratifying by ancestry, 16 independent co-expression modules were identified in the NHB subset and 18 were identified in the NHW subset. While none of the module eigengenes were associated with PTSD in the NHB subset, four modules significantly associated with PTSD status in the NHW subset (p ≤ 0.05, Figure 2). Two modules (MEtan and MEred) were upregulated in PTSD cases and two modules (MEpink and MEpurple) were downregulated in PTSD cases. MEpurple was also associated with PTSD severity as measured by total DTS score (p = 0.0356), and with current MDD status (p = 0.0007), an association that surpassed adjustment for multiple testing (Bonferroni adjustment; 18 tests; p = 0.0028). Additionally, MEred was also associated with MDD status (p = 0.0118) and MEtan was also associated with current smoking status (p = 0.041). These associations were in the same direction as were those for PTSD: MEpurple genes were downregulated in MDD and associated with decreasing total DTS score, MEred genes were upregulated in MDD, and MEtan genes were upregulated in smokers. Pathways significantly enriched in the PTSD-associated co-expression modules are listed in Table 3. The genes in MEtan showed significant enrichment for gene ontology (GO) terms involving plasma membrane/transmembrane, glycoprotein, and signal peptide (FDR q < 0.05), whereas genes in MEred were enriched for protein transport, alternative splicing, and phosphoprotein GO terms (FDR q < 0.05). MEpink genes were enriched for KEGG pathway term “metabolic pathways” (FDR q < 0.05) and MEpurple showed enrichment for actin binding, alternative splicing, and adaptive immunity GO terms (FDR q < 0.05).
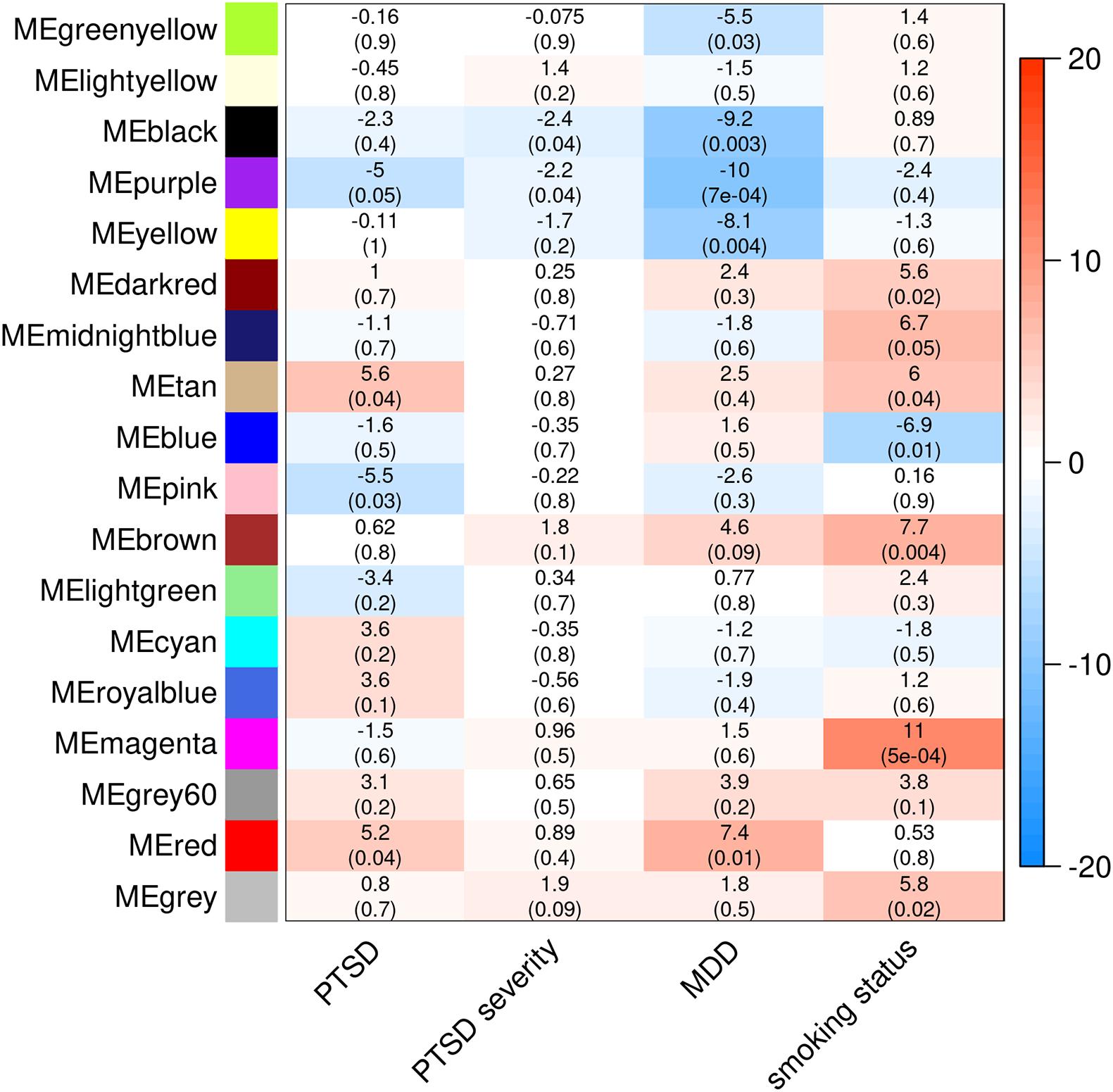
Figure 2. Co-expression modules detected in NHW MIRECC/Duke subjects and subsequent associations with PTSD, PTSD severity, MDD, and smoking status. The heatmap color scale represents effect sizes of each association (betas are listed in each cell, followed by corresponding p-value in parenthesis).
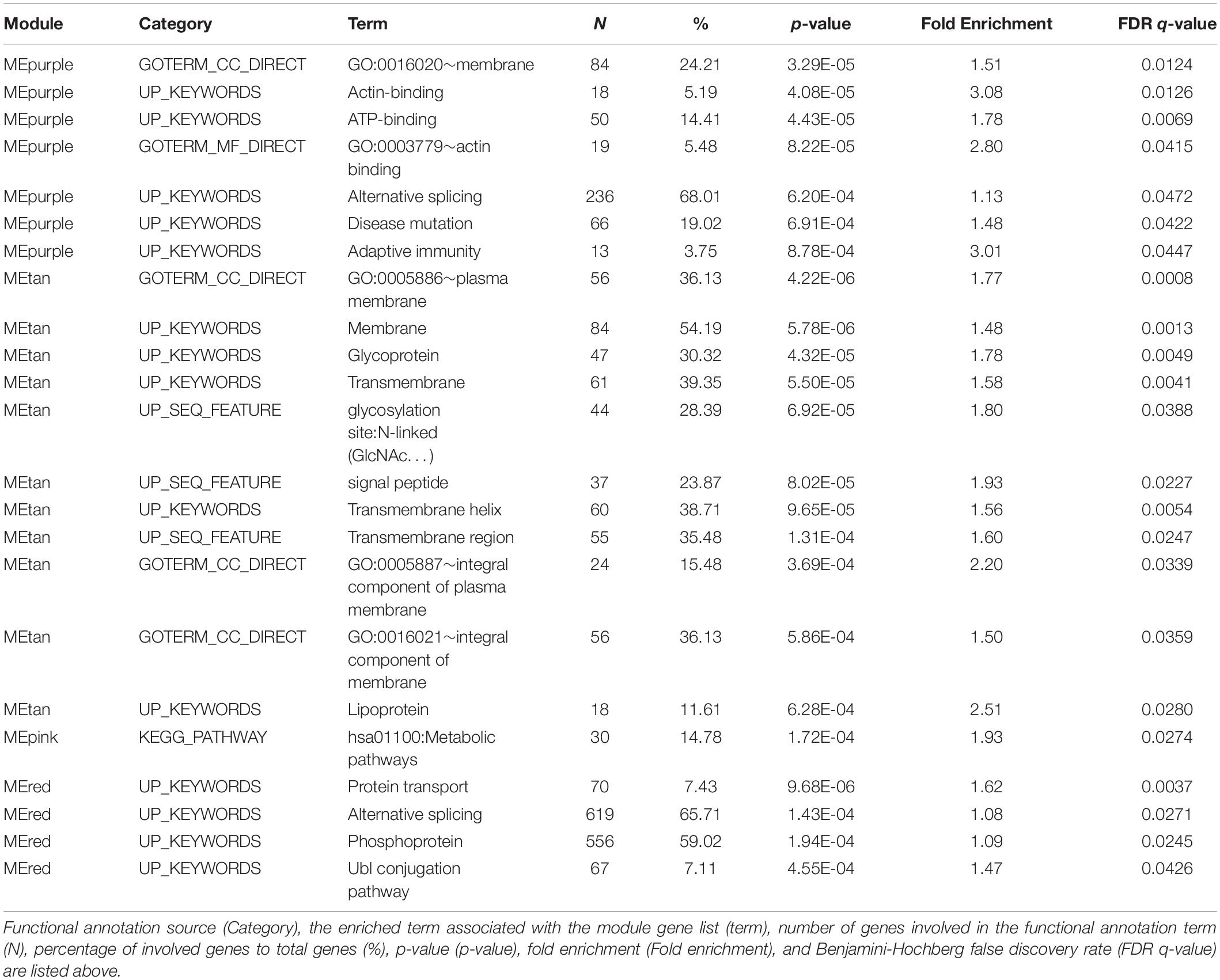
Table 3. Pathways enriched in co-expression modules that are also associated with PTSD (MEpurple, MEtan, MEpink, and MEred).
Discussion
This is the largest meta-analysis of differential PTSD gene expression to date. Results not only identified genes in pathways relevant to PTSD pathogenesis, but also showed PTSD and MDD GWAS signals may be driven by differences in gene expression. The most significant differentially expressed gene was interleukin-1ß (IL1B), a pro-inflammatory cytokine that is associated with innate immunity and has been extensively studied for its role in autoinflammatory diseases (Dinarello, 2011). Indeed, those with PTSD are at increased risk for autoimmune disorders including rheumatoid arthritis, systemic lupus erythematosus, inflammatory bowel diseases, and multiple sclerosis (Ali et al., 2014; O’Donovan et al., 2015; Song et al., 2018; Bookwalter et al., 2020), and display elevated levels of pro-inflammatory cytokines, including interleukin-1ß (Spivak et al., 1997; Tursich et al., 2014; Passos et al., 2015). Moreover, inflammation and dysregulation of the immune system has been associated with other psychiatric disorders that frequently co-occur with PTSD such as MDD (Howren et al., 2009; Dowlati et al., 2010), schizophrenia (Miller et al., 2011), and alcohol use disorder (Crews et al., 2017; Patel et al., 2019). Animal studies have shown that mice subjected to a model of depression display increased levels of interleukin-1ß in the hippocampus (Goshen et al., 2008), a brain region integral to the neuropathology of PTSD due to its role in fear learning and contextual processing (Maren et al., 2013), as well as memory formation and retrieval (Bremner et al., 1997); in fact, a recent study in post-mortem human brain observed increased IL1B expression in dlPFC of PTSD cases compared to controls (Girgenti et al., 2021). The increase in IL1B expression observed among PTSD cases in the present research adds additional evidence to the growing body of work demonstrating widespread inflammatory response and dysregulation of the immune system in PTSD pathology.
We also detected reduced expression of ILK, the gene encoding ILK, a key scaffold protein that localizes to focal adhesions and is involved in the regulation of many cellular processes including cell growth, survival, adhesion, invasion, and migration. ILK is highly expressed in several brain regions including hippocampus, cerebellum, and frontal cortex (Mills et al., 2003; Xu et al., 2015) and is essential for neurite outgrowth (Mills et al., 2003), dendritogenesis (Naska et al., 2006), and survival signaling in hippocampal neurons (Gary et al., 2003). Although this gene has not previously been implicated in PTSD, indirect evidence points to ILK as a plausible candidate for further interrogation. In mouse, knockdown of brain-derived neurotrophic factor (BDNF), a gene associated not only with PTSD, but also MDD, bipolar disorder, and schizophrenia, results in dysregulation of hippocampal neurogenesis and memory deficits (BDNF+/-; Ernfors et al., 1994). Overexpression of ILK in the hippocampus of these mice rescues the hippocampal neurogenesis and memory deficits (Xu et al., 2015). Additionally, in a rat model of fetal alcohol spectrum disorder, alcohol-exposed pups displayed impaired contextual fear conditioning and memory performance, along with reduced hippocampal ILK activity (Bhattacharya et al., 2015). This pattern has also been observed in a mouse model for Alzheimer’s disease (AD), where ILK protein levels are significantly decreased in the hippocampus of APP/PS1 mice, which display perturbed neurogenesis and memory deficits, and can be rescued by overexpressing ILK (Xu et al., 2018). The direction of the effects in these animal studies is consistent with our observation that ILK expression is reduced in PTSD cases. Relatedly, several studies have reported a bi-directional association between PTSD and dementia (reviewed in Desmarais et al., 2020); those with PTSD are at increased risk for dementia and vice versa. More research is necessary to investigate the potential therapeutic value of ILK signaling pathway targets in PTSD, as have been suggested for AD (Li et al., 2012; Xu et al., 2018).
In addition to IL1B and ILK, pathway analysis of all gene expression probes nominally associated with PTSD revealed an enrichment of toll-like receptor (TLR) and interleukin-1 receptor (IL-1R) genes that share the conserved toll/IL-1R homologous region (TIR) and are involved in innate antibacterial and antifungal immunity, consistent with previous reports from gene expression (Breen et al., 2015) and methylation studies (Uddin et al., 2010; Smith et al., 2011) in PTSD. TLRs play an important role in pathogen recognition and activate a signaling cascade which leads to upregulation of pro-inflammatory cytokines, including interleukin-1ß. Identification of not only IL1B, but also several TLR genes, demonstrates an increased burden of dysregulated innate immunity and inflammatory response genes among PTSD cases.
Correlation network analysis identified many modules of co-expression in the MIRECC/Duke cohort. Four modules identified in the NHW samples were associated with PTSD, two of which were also associated with MDD. Identification of coordinated expression associated with both PTSD and MDD confirms not only a strong correlation between the two phenotypes, but also shared biological pathways underlying the two conditions. We also identified eQTLs that were only significant in those with either PTSD or MDD, demonstrating genotype × environment interactions affecting gene expression. For instance, rs28842268 was associated with adenylate kinase 4 (AK4) expression, but only among PTSD cases; there was no effect among the control samples. AK4 localizes to the mitochondrial matrix and is involved in the cell survival response to oxidative stress (Liu et al., 2009; Kong et al., 2013), a molecular state that is activated by the persistent hyperarousal and fear experienced by those with PTSD (reviewed in Miller et al., 2018). Using a convergent functional genomics approach, Le-Niculescu et al. (2009) implicated AK4 in bipolar disorder, another neuropsychiatric condition which frequently co-occurs with PTSD (Cerimele et al., 2017). These data suggest there are genetic regulators of expression that are specific to the elevated stress and inflammatory state induced by PTSD.
Utilizing summary statistics from PTSD and MDD GWASs (Wray et al., 2018; Maihofer et al., submitted), we identified four genes (UBA7, HLA-F, HSPA1B, and RERE) with high probability of a shared causal eQTL variant with PTSD and/or MDD GWAS variants, thereby providing a potential mechanism by which the GWAS variant contributes to disease risk. UBA7 encodes an E1 ubiquitin-activating enzyme shown to be involved in STAT1-mediated interferon-α (INF-α) regulation of hippocampal neurogenesis and apoptosis (Borsini et al., 2018), processes triggered by a pro-inflammatory state. The UBA7 eQTL shares genetic effects with both PTSD and MDD GWAS variants, suggesting a potential pleiotropic mechanism at this locus. Recently, UBA7 was implicated in a transcriptome-wide association study (TWAS) of PTSD using brain tissue (Girgenti et al., 2021), confirming the association we observe in whole blood. The summary statistics utilized in Girgenti et al. were obtained from MVP (Stein et al., 2021) as opposed to the current study, which used summary statistics from PGC-PTSD (Maihofer et al., submitted), demonstrating an independent replication of the UBA7 finding. We also identified two eQTLs involving major histocompatibility complex (MHC) genes, HLA-F and HSPA1B, that displayed evidence for a shared genetic effect with MDD GWAS variants. The MHC group of genes encode proteins integral to immune response and variants in MHC genes have been associated with increased risk for many autoimmune diseases (reviewed in Matzaraki et al., 2017). As mentioned above, those with PTSD are at increased risk for autoimmune disorders and display increased levels of pro-inflammatory cytokines, including interleukin-1ß. The identification of multiple shared genetic effects of MHC gene expression and MDD risk increases the body of evidence implicating inflammatory and immune response pathways in both PTSD and MDD. Finally, two separate eQTLs for Arginine-Glutamic Acid Dipeptide Repeats (RERE) colocalize with MDD GWAS variants. Of note, a chromatin accessibility QTL (cQTL) in RERE identified in dlPFC of post-mortem human brains was shown to colocalize with schizophrenia GWAS variants (Bryois et al., 2018), another psychiatric disorder that co-occurs with PTSD.
The majority of these findings represent eQTLs from a PTSD-enriched dataset that colocalize with MDD GWAS variants. This further demonstrates not only the phenotypic comorbidity between PTSD and MDD, but identifies genomic regions of possible pleiotropy. Functional studies are necessary to further investigate these associations. Still, these results suggest biological mechanisms by which non-coding and common genetic variants may influence risk for PTSD and/or MDD.
While we have identified plausible novel candidates for PTSD, and confirmed many previously reported genes and pathways, this study is not without limitations. We acknowledge that the associations we observed in the differential expression analysis failed to achieve correction for multiple testing. While we have assembled the largest study of gene expression in PTSD to date, still larger sample sizes are required to reach an adequate power threshold to detect more modest differences. Despite this, IL1B has been repeatedly implicated in PTSD, therefore our replication of increased IL1B expression in PTSD cases is important. Because ILK has not previously been associated with PTSD, replication in independent datasets is necessary. Secondly, gene expression was measured in peripheral blood as opposed to brain tissue. We did attempt to impute genetically-regulated dlPFC expression in the NHW MIRECC/Duke subjects using a large eQTL reference panel (Huckins et al., 2019), but were only able to impute 6,928 genes with high confidence. Among the genes that were differentially expressed in both blood and imputed brain, nearly 70% showed a concordant direction of association with PTSD. The high percentage of concordance, as well as replication of pathways from the few brain-based studies available, lends credence to the hypothesis of at least partial overlap between blood and brain transcriptomes. Third, despite having compiled a large veteran cohort with respect to gene expression, it is difficult to identify robust interactions, particularly when SNPs involved have low minor allele frequency (MAF). As such, the eQTL interactions we have identified, particularly those involving SNPs with lower MAF, should be viewed as preliminary and need to be replicated in larger studies. Also, we did not investigate sex-specific associations due to the relatively small number of female samples available in the current study. Ascertainment of female veterans should be prioritized to facilitate research of possible sex-specific genetic factors influencing PTSD risk. Finally, gene expression in this study was determined using microarrays and not RNA-sequencing. Future studies that interrogate gene expression using sequencing approaches would be beneficial to obtain a truly global depiction of the transcriptome.
Conclusion
This is the largest study of differential gene expression between PTSD cases and controls to date, and these analyses have identified many logical candidate genes and pathways for further research. Notably, while we did not replicate the FKBP5 finding (p > 0.05), the present findings provide additional support for the role of IL1B and other inflammatory response and innate immunity pathway genes in PTSD, and have identified ILK as a novel candidate. Further, we identified modules of co-expressed genes that were associated with both PTSD and MDD, suggesting common biological pathways underlie the two comorbid disorders. Finally, we identified changes in gene expression that may help explain several PTSD and MDD GWAS signals. These data provide further insight into genetic factors and pathways associated with PTSD, as well as potential regions of pleiotropy between PTSD and MDD.
Members of the VA Mid-Atlantic Mental Illness Research, Education, and Clinical Center (MIRECC) Workgroup
Mira Brancu, Ph.D., Patrick S. Calhoun, Ph.D., Eric Dedert, Ph.D., Eric B. Elbogen, Ph.D., John A. Fairbank, Ph.D., Robin A. Hurley, M.D., Jason D. Kilts, Ph.D., Angela Kirby, M.S., Sara Martindale, Ph.D., Scott D. McDonald, Ph.D., Scott D. Moore, M.D., Ph.D., Rajendra A. Morey, M.D., M.S., Jennifer C. Naylor, Ph.D., Jared Rowland, Ph.D., Robert Shura, Ph.D., Cindy Swinkels, Ph.D., Steven T. Szabo, M.D., Ph.D., Katherine H. Taber, Ph.D., Larry A. Tupler, Ph.D., and Ruth E. Yoash-Gantz, Psy.D.
Members of the PTSD Initiative
Sarah McLeay, BSc(Hons), Ph.D., Wendy Harvey, BSc(Hons), MBBS, MPH, Madeline Romaniuk, BA, GradDipPsych, BBehSc(Hons), DPsych(Clinical), Darrell Crawford, MBBS, FRACP, M.D., David Colquhoun, MBBS, FRACP, Ross McD Young, Ph.D., Miriam Dwyer, BSc, HDipEd, John Gibson, MBBS, FRANZCP, Robyn O’Sullivan, MBBS, FRACP, Graham Cooksley, MBBS, M.D., FRACP, Christopher Strakosch, M.D., FRACP, Rachel Thomson, MBBS, GradDipClinEpi, Ph.D., FRACP, Joanne Voisey, BSc(Hons), Ph.D., Bruce Lawford, MBBS, FRANZCP, FAChAM (RACP).
Data Availability Statement
The datasets presented in this article are not readily available because the institutional review board (IRB) protocols governing these datasets do not allow sharing of individual level data. Requests to access the datasets should be directed to the senior author, AA-K at YWxsaXNvbi5hc2hsZXlrb2NoQGR1a2UuZWR1.
Ethics Statement
The studies involving human participants were reviewed and approved by the Durham (NC) VA Medical Center IRB, McGuire (Richmond, VA) VA Medical Center IRB, Hampton (VA) VA Medical Center IRB, W.G. (Bill) Hefner (Salisbury, NC) VA Medical Center IRB, Greenslopes Research and Ethics Committee, Queensland University of Technology Human Research Committee, San Diego (CA) VA Medical Center IRB, Togus (ME) VA Medical Center IRB, Cincinnati (OH) VA Medical Center IRB, Puget Sound (WA) VA Medical Center IRB, Dartmouth College (NH) IRB, University of California San Diego (CA) IRB, and Human Research Protections Office of the Department of Defense. The patients/participants provided their written informed consent to participate in this study.
Author Contributions
AA-K, MH, and MG contributed to the design of the study. DM, MD, CM, GG, MS, NK, JB, MH, and AA-K assisted in the data collection, generation, and preparation. MG, XQ, and DM performed the analyses. MG prepared the first draft of the manuscript. AA-K, MH, NK, and JB contributed to the comments and edits during manuscript drafting. All authors reviewed the manuscript, provided feedback, and approved the manuscript for submission.
Funding
Work utilizing the MIRECC/Duke cohort was supported by grant #I01BX002577 from the Biomedical and Laboratory Research and Development (BLR&D) Service of the Department of Veterans Affairs (VA) Office of Research and Development (ORD). In addition, JB was supported by a Research Career Scientist Award (#11S-RCS-009) from CSR&D. The views expressed in this article are those of the authors and do not necessarily reflect the position or policy of the VA or the US government. The PTSD Initiative was funded by the Queensland Branch of the Returned and Services League of Australia (RSL QLD), Australia. The Gallipoli Medical Research Foundation wishes to thank the RSL QLD for their generous donation, and Sullivan Nicolaides Pathology and Queensland X-Ray for their in-kind support. The authors thank the Faculty of Health QUT, and IHBI for financial and administrative support. DM was funded via the QUT Vice Chancellors Research Fellowship. Research using the INTRuST cohort was supported by the CDMRP award W81XWH-08-2-0159 (MS), the VA Office of Academic Affairs (J. Bomyea) and a Career Development Award from the US Department of Veteran Affairs (CSR&D CX001600-01A1; J. Bomyea).
Conflict of Interest
The authors declare that the research was conducted in the absence of any commercial or financial relationships that could be construed as a potential conflict of interest.
Publisher’s Note
All claims expressed in this article are solely those of the authors and do not necessarily represent those of their affiliated organizations, or those of the publisher, the editors and the reviewers. Any product that may be evaluated in this article, or claim that may be made by its manufacturer, is not guaranteed or endorsed by the publisher.
Acknowledgments
The authors thank the Gallipoli Medical Research Foundation for their support, especially Miriam Dwyer and Sarah McLeay for their project management support. The authors would also like to acknowledge Madeline Romaniuk for psychological expertise and John Gibson and the team at the Keith Payne Unit, and the staff and investigators at Greenslopes Private Hospital for their valuable contribution to the study. All authors extend their gratitude to the study participants for their generous provision of data and time. The authors thank the INTRuST consortium contributors for contributions to the study design and execution.
Supplementary Material
The Supplementary Material for this article can be found online at: https://www.frontiersin.org/articles/10.3389/fnins.2021.678548/full#supplementary-material
Footnotes
- ^ https://www.mentalhealth.va.gov/docs/data-sheets/2020/2020-National-Veteran-Suicide-Prevention-Annual-Report-11-2020-508.pdf
References
Akosile, W., Young, R., Lawford, B., Voisey, J., and Colquhoun, D. (2018). PTSD symptoms associated with myocardial infarction: practical clinical implications. Australas. Psychiatry 26, 60–64. doi: 10.1177/1039856217734738
Ali, T., Prammanasudh, C. S., Samuels, J. M., and Madhoun, M. F. (2014). Stress as a risk factor for inflammatory bowel disease: more evidence from our OEF/OIF veterans? Am. J. Gastroenterol. 109, 604–605. doi: 10.1038/ajg.2013.416
Ashley-Koch, A. E., Garrett, M. E., Gibson, J., Liu, Y., Dennis, M. F., Kimbrel, N. A., et al. (2015). Genome-wide association study of posttraumatic stress disorder in a cohort of Iraq-Afghanistan era veterans. J. Affect. Disord 184, 225–234. doi: 10.1016/j.jad.2015.03.049
Ben Barnes, J., Hayes, A. M., Contractor, A. A., Nash, W. P., and Litz, B. T. (2018). The structure of co-occurring PTSD and depression symptoms in a cohort of Marines pre- and post-deployment. Psychiatry Res. 259, 442–449. doi: 10.1016/j.psychres.2017.10.045
Benjet, C., Bromet, E., Karam, E. G., Kessler, R. C., Mclaughlin, K. A., Ruscio, A. M., et al. (2016). The epidemiology of traumatic event exposure worldwide: results from the world mental health survey consortium. Psychol. Med. 46, 327–343.
Bhattacharya, D., Dunaway, E. P., Bhattacharya, S., Bloemer, J., Buabeid, M., Escobar, M., et al. (2015). Impaired ILK function is associated with deficits in hippocampal based memory and synaptic plasticity in a FASD rat model. PLoS One 10:e0135700. doi: 10.1371/journal.pone.0135700
Blake, D. D., Weathers, F. W., Nagy, L. M., Kaloupek, D. G., Gusman, F. D., Charney, D. S., et al. (1995). The development of a clinician-administered PTSD scale. J. Trauma. Stress 8, 75–90.
Blanco, C., Xu, Y., Brady, K., Perez-Fuentes, G., Okuda, M., and Wang, S. (2013). Comorbidity of posttraumatic stress disorder with alcohol dependence among US adults: results from national epidemiological survey on alcohol and related conditions. Drug Alcohol. Depend. 132, 630–638. doi: 10.1016/j.drugalcdep.2013.04.016
Bomyea, J., Flashman, L. A., Zafonte, R., Andaluz, N., Coimbra, R., George, M. S., et al. (2019). Associations between neuropsychiatric and health status outcomes in individuals with probable mTBI. Psychiatry Res. 272, 531–539. doi: 10.1016/j.psychres.2018.12.021
Bomyea, J., Simmons, A. N., Shenton, M. E., Coleman, M. J., Bouix, S., Rathi, Y., et al. (2020). Neurocognitive markers of childhood abuse in individuals with PTSD: findings from the INTRuST Clinical Consortium. J. Psychiatr. Res. 121, 108–117. doi: 10.1016/j.jpsychires.2019.11.012
Bookwalter, D. B., Roenfeldt, K. A., Leardmann, C. A., Kong, S. Y., Riddle, M. S., and Rull, R. P. (2020). Posttraumatic stress disorder and risk of selected autoimmune diseases among US military personnel. BMC Psychiatry 20:23.
Borsini, A., Cattaneo, A., Malpighi, C., Thuret, S., Harrison, N. A., Consortium, M. R. C. I., et al. (2018). Interferon-alpha reduces human hippocampal neurogenesis and increases apoptosis via activation of distinct STAT1-dependent mechanisms. Int. J. Neuropsychopharmacol. 21, 187–200. doi: 10.1093/ijnp/pyx083
Breen, M. S., Maihofer, A. X., Glatt, S. J., Tylee, D. S., Chandler, S. D., Tsuang, M. T., et al. (2015). Gene networks specific for innate immunity define post-traumatic stress disorder. Mol. Psychiatry 20, 1538–1545. doi: 10.1038/mp.2015.9
Breen, M. S., Tylee, D. S., Maihofer, A. X., Neylan, T. C., Mehta, D., Binder, E. B., et al. (2018). PTSD blood transcriptome mega-analysis: shared inflammatory pathways across biological sex and modes of trauma. Neuropsychopharmacology 43, 469–481. doi: 10.1038/npp.2017.220
Bremner, J. D., Randall, P., Vermetten, E., Staib, L., Bronen, R. A., Mazure, C., et al. (1997). Magnetic resonance imaging-based measurement of hippocampal volume in posttraumatic stress disorder related to childhood physical and sexual abuse–a preliminary report. Biol. Psychiatry 41, 23–32. doi: 10.1016/s0006-3223(96)00162-x
Bryois, J., Garrett, M. E., Song, L., Safi, A., Giusti-Rodriguez, P., Johnson, G. D., et al. (2018). Evaluation of chromatin accessibility in prefrontal cortex of individuals with schizophrenia. Nat. Commun. 9:3121.
Cerimele, J. M., Bauer, A. M., Fortney, J. C., and Bauer, M. S. (2017). Patients with co-occurring bipolar disorder and posttraumatic stress disorder: a rapid review of the literature. J. Clin. Psychiatry 78, e506–e514.
Cougle, J. R., Feldner, M. T., Keough, M. E., Hawkins, K. A., and Fitch, K. E. (2010). Comorbid panic attacks among individuals with posttraumatic stress disorder: associations with traumatic event exposure history, symptoms, and impairment. J. Anxiety Disord 24, 183–188. doi: 10.1016/j.janxdis.2009.10.006
Crews, F. T., Lawrimore, C. J., Walter, T. J., and Coleman, L. G. Jr. (2017). The role of neuroimmune signaling in alcoholism. Neuropharmacology 122, 56–73. doi: 10.1016/j.neuropharm.2017.01.031
Davidson, J. R., Book, S. W., Colket, J. T., Tupler, L. A., Roth, S., David, D., et al. (1997). Assessment of a new self-rating scale for post-traumatic stress disorder. Psychol. Med. 27, 153–160. doi: 10.1017/s0033291796004229
Department of Veterans Affairs, Office of Mental Health & Suicide Prevention (2020). 2020 National Veterans Suicide Prevention Annual Report. Available online at: https://www.mentalhealth.va.gov/docs/data-sheets/2020/2020-National-Veteran-Suicide-Prevention-Annual-Report-11-2020-508.pdf (accessed December 3, 2020).
Desmarais, P., Weidman, D., Wassef, A., Bruneau, M. A., Friedland, J., Bajsarowicz, P., et al. (2020). The interplay between post-traumatic stress disorder and dementia: a systematic review. Am. J. Geriatr. Psychiatry 28, 48–60. doi: 10.1016/j.jagp.2019.08.006
Dinarello, C. A. (2011). Interleukin-1 in the pathogenesis and treatment of inflammatory diseases. Blood 117, 3720–3732. doi: 10.1182/blood-2010-07-273417
Dowlati, Y., Herrmann, N., Swardfager, W., Liu, H., Sham, L., Reim, E. K., et al. (2010). A meta-analysis of cytokines in major depression. Biol. Psychiatry 67, 446–457. doi: 10.1016/j.biopsych.2009.09.033
Duncan, L. E., Ratanatharathorn, A., Aiello, A. E., Almli, L. M., Amstadter, A. B., Ashley-Koch, A. E., et al. (2018). Largest GWAS of PTSD (N=20 070) yields genetic overlap with schizophrenia and sex differences in heritability. Mol. Psychiatry 23, 666–673. doi: 10.1038/mp.2017.77
Ernfors, P., Lee, K. F., and Jaenisch, R. (1994). Mice lacking brain-derived neurotrophic factor develop with sensory deficits. Nature 368, 147–150. doi: 10.1038/368147a0
First, M. B., Spitzer, R. L., Gibbon, M., and Williams, J. B. W. (1994). Structured Clinical Interview for Axis I DSM-IV Disorders, 2nd Edn. New York, NY: Biometrics Research Department.
Flory, J. D., and Yehuda, R. (2015). Comorbidity between post-traumatic stress disorder and major depressive disorder: alternative explanations and treatment considerations. Dialogues Clin. Neurosci. 17, 141–150. doi: 10.31887/dcns.2015.17.2/jflory
Fulton, J. J., Calhoun, P. S., Wagner, H. R., Schry, A. R., Hair, L. P., Feeling, N., et al. (2015). The prevalence of posttraumatic stress disorder in Operation Enduring Freedom/Operation Iraqi Freedom (OEF/OIF) Veterans: a meta-analysis. J. Anxiety Disord 31, 98–107. doi: 10.1016/j.janxdis.2015.02.003
Gary, D. S., Milhavet, O., Camandola, S., and Mattson, M. P. (2003). Essential role for integrin linked kinase in Akt-mediated integrin survival signaling in hippocampal neurons. J. Neurochem. 84, 878–890. doi: 10.1046/j.1471-4159.2003.01579.x
Genomes Project Consortium, Auton, A., Brooks, L. D., Durbin, R. M., Garrison, E. P., Kang, H. M., et al. (2015). A global reference for human genetic variation. Nature 526, 68–74. doi: 10.1038/nature15393
Giambartolomei, C., Vukcevic, D., Schadt, E. E., Franke, L., Hingorani, A. D., Wallace, C., et al. (2014). Bayesian test for colocalisation between pairs of genetic association studies using summary statistics. PLoS Genet. 10:e1004383. doi: 10.1371/journal.pgen.1004383
Girgenti, M. J., Wang, J., Ji, D., Cruz, D. A., Traumatic Stress Brain Research, Stein, M. B., et al. (2021). Transcriptomic organization of the human brain in post-traumatic stress disorder. Nat. Neurosci. 24, 24–33.
Goshen, I., Kreisel, T., Ben-Menachem-Zidon, O., Licht, T., Weidenfeld, J., Ben-Hur, T., et al. (2008). Brain interleukin-1 mediates chronic stress-induced depression in mice via adrenocortical activation and hippocampal neurogenesis suppression. Mol. Psychiatry 13, 717–728. doi: 10.1038/sj.mp.4002055
Guardado, P., Olivera, A., Rusch, H. L., Roy, M., Martin, C., Lejbman, N., et al. (2016). Altered gene expression of the innate immune, neuroendocrine, and nuclear factor-kappa B (NF-kappaB) systems is associated with posttraumatic stress disorder in military personnel. J. Anxiety Disord 38, 9–20. doi: 10.1016/j.janxdis.2015.12.004
Howie, B. N., Donnelly, P., and Marchini, J. (2009). A flexible and accurate genotype imputation method for the next generation of genome-wide association studies. PLoS Genet 5:e1000529. doi: 10.1371/journal.pgen.1000529
Howren, M. B., Lamkin, D. M., and Suls, J. (2009). Associations of depression with C-reactive protein, IL-1, and IL-6: a meta-analysis. Psychosom. Med. 71, 171–186. doi: 10.1097/psy.0b013e3181907c1b
Huang da, W., Sherman, B. T., and Lempicki, R. A. (2009a). Bioinformatics enrichment tools: paths toward the comprehensive functional analysis of large gene lists. Nucleic Acids Res. 37, 1–13. doi: 10.1093/nar/gkn923
Huang da, W., Sherman, B. T., and Lempicki, R. A. (2009b). Systematic and integrative analysis of large gene lists using DAVID bioinformatics resources. Nat. Protoc. 4, 44–57. doi: 10.1038/nprot.2008.211
Huber, W., Von Heydebreck, A., Sultmann, H., Poustka, A., and Vingron, M. (2002). Variance stabilization applied to microarray data calibration and to the quantification of differential expression. Bioinformatics 18(Suppl. 1) S96–S104.
Huckins, L. M., Chatzinakos, C., Breen, M. S., Hartmann, J., Klengel, T., Da Silva Almeida, A. C., et al. (2020). Analysis of genetically regulated gene expression identifies a prefrontal PTSD Gene. SNRNP35, specific to military cohorts. Cell Rep. 31:107716. doi: 10.1016/j.celrep.2020.107716
Huckins, L. M., Dobbyn, A., Ruderfer, D. M., Hoffman, G., Wang, W., Pardinas, A. F., et al. (2019). Gene expression imputation across multiple brain regions provides insights into schizophrenia risk. Nat. Genet. 51, 659–674. doi: 10.1038/s41588-019-0364-4
International HapMap 3 Consortium, Altshuler, D. M., Gibbs, R. A., Peltonen, L., Altshuler, D. M., Gibbs, R. A., et al. (2010). Integrating common and rare genetic variation in diverse human populations. Nature 467, 52–58. doi: 10.1038/nature09298
Johnson, W. E., Li, C., and Rabinovic, A. (2007). Adjusting batch effects in microarray expression data using empirical Bayes methods. Biostatistics 8, 118–127. doi: 10.1093/biostatistics/kxj037
Kessler, R. C., Berglund, P., Demler, O., Jin, R., Merikangas, K. R., and Walters, E. E. (2005). Lifetime prevalence and age-of-onset distributions of DSM-IV disorders in the National Comorbidity Survey Replication. Arch. Gen. Psychiatry 62, 593–602. doi: 10.1001/archpsyc.62.6.593
Kilpatrick, D. G., Resnick, H. S., Milanak, M. E., Miller, M. W., Keyes, K. M., and Friedman, M. J. (2013). National estimates of exposure to traumatic events and PTSD prevalence using DSM-IV and DSM-5 criteria. J. Trauma. Stress 26, 537–547. doi: 10.1002/jts.21848
Kimbrel, N. A., Meyer, E. C., Debeer, B. B., Gulliver, S. B., and Morissette, S. B. (2016). A 12-Month prospective study of the effects of PTSD-depression comorbidity on suicidal behavior in Iraq/Afghanistan-era veterans. Psychiatry Res. 243, 97–99. doi: 10.1016/j.psychres.2016.06.011
Koenen, K. C., Sumner, J. A., Gilsanz, P., Glymour, M. M., Ratanatharathorn, A., Rimm, E. B., et al. (2017). Post-traumatic stress disorder and cardiometabolic disease: improving causal inference to inform practice. Psychol. Med. 47, 209–225. doi: 10.1017/s0033291716002294
Kong, F., Binas, B., Moon, J. H., Kang, S. S., and Kim, H. J. (2013). Differential expression of adenylate kinase 4 in the context of disparate stress response strategies of HEK293 and HepG2 cells. Arch. Biochem. Biophys. 533, 11–17. doi: 10.1016/j.abb.2013.02.014
Kroenke, K., Spitzer, R. L., and Williams, J. B. (2001). The PHQ-9: validity of a brief depression severity measure. J. Gen. Intern. Med. 16, 606–613. doi: 10.1046/j.1525-1497.2001.016009606.x
Kuan, P. F., Waszczuk, M. A., Kotov, R., Clouston, S., Yang, X., Singh, P. K., et al. (2017). Gene expression associated with PTSD in World Trade Center responders: an RNA sequencing study. Transl. Psychiatry 7:1297.
Kulka, R. A., Schlenger, W. E., Fairbank, J. A., Hough, R. L., Jordan, B. K., Marmar, C. R., et al. (1990). Trauma and the Vietnam war generation: Report of Findings From the National Vietnam Veterans Readjustment Study. New York NY: Brunner/Mazel.
Langfelder, P., and Horvath, S. (2008). WGCNA: an R package for weighted correlation network analysis. BMC Bioinformatics 9:559.
Le-Niculescu, H., Patel, S. D., Bhat, M., Kuczenski, R., Faraone, S. V., Tsuang, M. T., et al. (2009). Convergent functional genomics of genome-wide association data for bipolar disorder: comprehensive identification of candidate genes, pathways and mechanisms. Am. J. Med. Genet. B Neuropsychiatr. Genet. 150B, 155–181. doi: 10.1002/ajmg.b.30887
Li, M., Dai, F. R., Du, X. P., Yang, Q. D., Zhang, X., and Chen, Y. (2012). Infusion of BDNF into the nucleus accumbens of aged rats improves cognition and structural synaptic plasticity through PI3K-ILK-Akt signaling. Behav. Brain Res. 231, 146–153. doi: 10.1016/j.bbr.2012.03.010
Lind, M. J., Brick, L. A., Gehrman, P. R., Duncan, L. E., Gelaye, B., Maihofer, A. X., et al. (2020). Molecular genetic overlap between posttraumatic stress disorder and sleep phenotypes. Sleep 43:zsz257.
Liu, H., Petukhova, M. V., Sampson, N. A., Aguilar-Gaxiola, S., Alonso, J., Andrade, L. H., et al. (2017). Association of DSM-IV Posttraumatic Stress Disorder With Traumatic Experience Type and History in the World Health Organization World Mental Health Surveys. JAMA Psychiatry 74, 270–281.
Liu, R., Strom, A. L., Zhai, J., Gal, J., Bao, S., Gong, W., et al. (2009). Enzymatically inactive adenylate kinase 4 interacts with mitochondrial ADP/ATP translocase. Int. J. Biochem. Cell Biol. 41, 1371–1380. doi: 10.1016/j.biocel.2008.12.002
Livingston, W. S., Fargo, J. D., Gundlapalli, A. V., Brignone, E., and Blais, R. K. (2020). Comorbid PTSD and depression diagnoses mediate the association of military sexual trauma and suicide and intentional self-inflicted injury in VHA-enrolled iraq/afghanistan veterans, 2004-2014. J. Affect. Disord 274, 1184–1190. doi: 10.1016/j.jad.2020.05.024
Logue, M. W., Smith, A. K., Baldwin, C., Wolf, E. J., Guffanti, G., Ratanatharathorn, A., et al. (2015). An analysis of gene expression in PTSD implicates genes involved in the glucocorticoid receptor pathway and neural responses to stress. Psychoneuroendocrinology 57, 1–13. doi: 10.1016/j.psyneuen.2015.03.016
Maren, S., Phan, K. L., and Liberzon, I. (2013). The contextual brain: implications for fear conditioning, extinction and psychopathology. Nat. Rev. Neurosci. 14, 417–428. doi: 10.1038/nrn3492
Marot, G., Foulley, J. L., Mayer, C. D., and Jaffrezic, F. (2009). Moderated effect size and P-value combinations for microarray meta-analyses. Bioinformatics 25, 2692–2699. doi: 10.1093/bioinformatics/btp444
Matzaraki, V., Kumar, V., Wijmenga, C., and Zhernakova, A. (2017). The MHC locus and genetic susceptibility to autoimmune and infectious diseases. Genome Biol. 18:76.
McLeay, S. C., Harvey, W. M., Romaniuk, M. N., Crawford, D. H., Colquhoun, D. M., Young, R. M., et al. (2017). Physical comorbidities of post-traumatic stress disorder in Australian Vietnam War veterans. Med. J. Aust. 206, 251–257.
Mehta, D., Bruenig, D., Carrillo-Roa, T., Lawford, B., Harvey, W., Morris, C. P., et al. (2017). Genomewide DNA methylation analysis in combat veterans reveals a novel locus for PTSD. Acta. Psychiatr. Scand. 136, 493–505. doi: 10.1111/acps.12778
Mehta, D., Gonik, M., Klengel, T., Rex-Haffner, M., Menke, A., Rubel, J., et al. (2011). Using polymorphisms in FKBP5 to define biologically distinct subtypes of posttraumatic stress disorder: evidence from endocrine and gene expression studies. Arch. Gen. Psychiatry 68, 901–910. doi: 10.1001/archgenpsychiatry.2011.50
Mehta, D., Voisey, J., Bruenig, D., Harvey, W., Morris, C. P., Lawford, B., et al. (2018). Transcriptome analysis reveals novel genes and immune networks dysregulated in veterans with PTSD. Brain Behav. Immun. 74, 133–142. doi: 10.1016/j.bbi.2018.08.014
Miller, B. J., Buckley, P., Seabolt, W., Mellor, A., and Kirkpatrick, B. (2011). Meta-analysis of cytokine alterations in schizophrenia: clinical status and antipsychotic effects. Biol. Psychiatry 70, 663–671. doi: 10.1016/j.biopsych.2011.04.013
Miller, M. W., Lin, A. P., Wolf, E. J., and Miller, D. R. (2018). Oxidative stress, inflammation, and neuroprogression in chronic PTSD. Harv. Rev. Psychiatry 26, 57–69. doi: 10.1097/hrp.0000000000000167
Mills, J., Digicaylioglu, M., Legg, A. T., Young, C. E., Young, S. S., Barr, A. M., et al. (2003). Role of integrin-linked kinase in nerve growth factor-stimulated neurite outgrowth. J. Neurosci. 23, 1638–1648. doi: 10.1523/jneurosci.23-05-01638.2003
Morrison, F. G., Miller, M. W., Wolf, E. J., Logue, M. W., Maniates, H., Kwasnik, D., et al. (2019). Reduced interleukin 1A gene expression in the dorsolateral prefrontal cortex of individuals with PTSD and depression. Neurosci. Lett. 692, 204–209. doi: 10.1016/j.neulet.2018.10.027
Naska, S., Park, K. J., Hannigan, G. E., Dedhar, S., Miller, F. D., and Kaplan, D. R. (2006). An essential role for the integrin-linked kinase-glycogen synthase kinase-3 beta pathway during dendrite initiation and growth. J. Neurosci. 26, 13344–13356. doi: 10.1523/jneurosci.4462-06.2006
Neylan, T. C., Sun, B., Rempel, H., Ross, J., Lenoci, M., O’donovan, A., et al. (2011). Suppressed monocyte gene expression profile in men versus women with PTSD. Brain Behav. Immun. 25, 524–531. doi: 10.1016/j.bbi.2010.12.001
Nievergelt, C. M., Maihofer, A. X., Klengel, T., Atkinson, E. G., Chen, C. Y., Choi, K. W., et al. (2019). International meta-analysis of PTSD genome-wide association studies identifies sex- and ancestry-specific genetic risk loci. Nat Commun 10:4558.
O’Donovan, A., Cohen, B. E., Seal, K. H., Bertenthal, D., Margaretten, M., Nishimi, K., et al. (2015). Elevated risk for autoimmune disorders in iraq and afghanistan veterans with posttraumatic stress disorder. Biol. Psychiatry 77, 365–374. doi: 10.1016/j.biopsych.2014.06.015
Ongen, H., Buil, A., Brown, A. A., Dermitzakis, E. T., and Delaneau, O. (2016). Fast and efficient QTL mapper for thousands of molecular phenotypes. Bioinformatics 32, 1479–1485. doi: 10.1093/bioinformatics/btv722
Oquendo, M., Brent, D. A., Birmaher, B., Greenhill, L., Kolko, D., Stanley, B., et al. (2005). Posttraumatic stress disorder comorbid with major depression: factors mediating the association with suicidal behavior. Am. J. Psychiatry 162, 560–566. doi: 10.1176/appi.ajp.162.3.560
Panagioti, M., Gooding, P. A., and Tarrier, N. (2012). A meta-analysis of the association between posttraumatic stress disorder and suicidality: the role of comorbid depression. Compr. Psychiatry 53, 915–930. doi: 10.1016/j.comppsych.2012.02.009
Passos, I. C., Vasconcelos-Moreno, M. P., Costa, L. G., Kunz, M., Brietzke, E., Quevedo, J., et al. (2015). Inflammatory markers in post-traumatic stress disorder: a systematic review, meta-analysis, and meta-regression. Lancet Psychiatry 2, 1002–1012. doi: 10.1016/s2215-0366(15)00309-0
Patel, R. R., Khom, S., Steinman, M. Q., Varodayan, F. P., Kiosses, W. B., Hedges, D. M., et al. (2019). IL-1beta expression is increased and regulates GABA transmission following chronic ethanol in mouse central amygdala. Brain Behav. Immun. 75, 208–219. doi: 10.1016/j.bbi.2018.10.009
Petrakis, I. L., Rosenheck, R., and Desai, R. (2011). Substance use comorbidity among veterans with posttraumatic stress disorder and other psychiatric illness. Am. J. Addict. 20, 185–189. doi: 10.1111/j.1521-0391.2011.00126.x
Pigeon, W. R., Campbell, C. E., Possemato, K., and Ouimette, P. (2013). Longitudinal relationships of insomnia, nightmares, and PTSD severity in recent combat veterans. J. Psychosom. Res. 75, 546–550. doi: 10.1016/j.jpsychores.2013.09.004
Pollard, H. B., Shivakumar, C., Starr, J., Eidelman, O., Jacobowitz, D. M., Dalgard, C. L., et al. (2016). “Soldier’s Heart”: a genetic basis for elevated cardiovascular disease risk associated with post-traumatic stress disorder. Front. Mol. Neurosci. 9:87.
Ratanatharathorn, A., Boks, M. P., Maihofer, A. X., Aiello, A. E., Amstadter, A. B., Ashley-Koch, A. E., et al. (2017). Epigenome-wide association of PTSD from heterogeneous cohorts with a common multi-site analysis pipeline. Am. J. Med. Genet. B Neuropsychiatr. Genet. 174, 619–630. doi: 10.1002/ajmg.b.32568
Ritchie, M. E., Phipson, B., Wu, D., Hu, Y., Law, C. W., Shi, W., et al. (2015). limma powers differential expression analyses for RNA-sequencing and microarray studies. Nucleic Acids Res. 43:e47. doi: 10.1093/nar/gkv007
Roberts, A. L., Gilman, S. E., Breslau, J., Breslau, N., and Koenen, K. C. (2011). Race/ethnic differences in exposure to traumatic events, development of post-traumatic stress disorder, and treatment-seeking for post-traumatic stress disorder in the United States. Psychol. Med. 41, 71–83. doi: 10.1017/s0033291710000401
Rusch, H. L., Robinson, J., Yun, S., Osier, N. D., Martin, C., Brewin, C. R., et al. (2019). Gene expression differences in PTSD are uniquely related to the intrusion symptom cluster: a transcriptome-wide analysis in military service members. Brain Behav. Immun. 80, 904–908. doi: 10.1016/j.bbi.2019.04.039
Rytwinski, N. K., Scur, M. D., Feeny, N. C., and Youngstrom, E. A. (2013). The co-occurrence of major depressive disorder among individuals with posttraumatic stress disorder: a meta-analysis. J. Trauma. Stress 26, 299–309. doi: 10.1002/jts.21814
Sarapas, C., Cai, G., Bierer, L. M., Golier, J. A., Galea, S., Ising, M., et al. (2011). Genetic markers for PTSD risk and resilience among survivors of the World Trade Center attacks. Dis Markers 30, 101–110. doi: 10.1155/2011/328054
Sartor, C. E., Grant, J. D., Lynskey, M. T., Mccutcheon, V. V., Waldron, M., Statham, D. J., et al. (2012). Common heritable contributions to low-risk trauma, high-risk trauma, posttraumatic stress disorder, and major depression. Arch. Gen. Psychiatry 69, 293–299. doi: 10.1001/archgenpsychiatry.2011.1385
Seal, K. H., Cohen, G., Waldrop, A., Cohen, B. E., Maguen, S., and Ren, L. (2011). Substance use disorders in Iraq and Afghanistan veterans in VA healthcare, 2001-2010: implications for screening, diagnosis and treatment. Drug Alcohol. Depend. 116, 93–101. doi: 10.1016/j.drugalcdep.2010.11.027
Segman, R. H., Shefi, N., Goltser-Dubner, T., Friedman, N., Kaminski, N., and Shalev, A. Y. (2005). Peripheral blood mononuclear cell gene expression profiles identify emergent post-traumatic stress disorder among trauma survivors. Mol. Psychiatry 10:425. doi: 10.1038/sj.mp.4001676
Sheehan, D. V., Lecrubier, Y., Sheehan, K. H., Amorim, P., Janavs, J., Weiller, E., et al. (1998). The Mini-International Neuropsychiatric Interview (M.I.N.I.): the development and validation of a structured diagnostic psychiatric interview for DSM-IV and ICD-10. J. Clin. Psychiatry 59 Suppl 20:quiz34–57.
Smith, A. K., Conneely, K. N., Kilaru, V., Mercer, K. B., Weiss, T. E., Bradley, B., et al. (2011). Differential immune system DNA methylation and cytokine regulation in post-traumatic stress disorder. Am. J. Med. Genet. B Neuropsychiatr. Genet. 156B, 700–708. doi: 10.1002/ajmg.b.31212
Song, H., Fang, F., Tomasson, G., Arnberg, F. K., Mataix-Cols, D., Fernandez De La Cruz, L., et al. (2018). Association of stress-related disorders with subsequent autoimmune disease. JAMA 319, 2388–2400. doi: 10.1001/jama.2018.7028
Spivak, B., Shohat, B., Mester, R., Avraham, S., Gil-Ad, I., Bleich, A., et al. (1997). Elevated levels of serum interleukin-1 beta in combat-related posttraumatic stress disorder. Biol. Psychiatry 42, 345–348. doi: 10.1016/s0006-3223(96)00375-7
Stein, M. B., Campbell-Sills, L., Gelernter, J., He, F., Heeringa, S. G., Nock, M. K., et al. (2017). Alcohol misuse and co-occurring mental disorders among new soldiers in the U.S. Army. Alcohol. Clin. Exp. Res. 41, 139–148. doi: 10.1111/acer.13269
Stein, M. B., Jang, K. L., Taylor, S., Vernon, P. A., and Livesley, W. J. (2002). Genetic and environmental influences on trauma exposure and posttraumatic stress disorder symptoms: a twin study. Am. J. Psychiatry 159, 1675–1681. doi: 10.1176/appi.ajp.159.10.1675
Stein, M. B., Levey, D. F., Cheng, Z., Wendt, F. R., Harrington, K., Pathak, G. A., et al. (2021). Genome-wide association analyses of post-traumatic stress disorder and its symptom subdomains in the Million Veteran Program. Nat. Genet. 53, 174–184. doi: 10.1038/s41588-020-00767-x
Storey, J. D., and Tibshirani, R. (2003). Statistical significance for genomewide studies. Proc. Natl. Acad. Sci. U.S.A. 100, 9440–9445. doi: 10.1073/pnas.1530509100
Teeters, J. B., Lancaster, C. L., Brown, D. G., and Back, S. E. (2017). Substance use disorders in military veterans: prevalence and treatment challenges. Subst. Abuse Rehabil. 8, 69–77. doi: 10.2147/sar.s116720
Thomson, C. A., Mccoll, A., Cavanagh, J., and Graham, G. J. (2014). Peripheral inflammation is associated with remote global gene expression changes in the brain. J. Neuroinflamm. 11:73. doi: 10.1186/1742-2094-11-73
True, W. R., Rice, J., Eisen, S. A., Heath, A. C., Goldberg, J., Lyons, M. J., et al. (1993). A twin study of genetic and environmental contributions to liability for posttraumatic stress symptoms. Arch. Gen. Psychiatry 50, 257–264. doi: 10.1001/archpsyc.1993.01820160019002
Tursich, M., Neufeld, R. W., Frewen, P. A., Harricharan, S., Kibler, J. L., Rhind, S. G., et al. (2014). Association of trauma exposure with proinflammatory activity: a transdiagnostic meta-analysis. Transl. Psychiatry 4:e413. doi: 10.1038/tp.2014.56
Uddin, M., Aiello, A. E., Wildman, D. E., Koenen, K. C., Pawelec, G., De Los Santos, R., et al. (2010). Epigenetic and immune function profiles associated with posttraumatic stress disorder. Proc. Natl. Acad. Sci. U.S.A. 107, 9470–9475. doi: 10.1073/pnas.0910794107
Ursano, R. J., Herberman Mash, H. B., Kessler, R. C., Naifeh, J. A., Fullerton, C. S., Aliaga, P. A., et al. (2020). Factors associated with suicide ideation in US Army Soldiers During Deployment in Afghanistan. JAMA Netw. Open 3:e1919935. doi: 10.1001/jamanetworkopen.2019.19935
van Iterson, M., Van Zwet, E. W., Consortium, B., and Heijmans, B. T. (2017). Controlling bias and inflation in epigenome- and transcriptome-wide association studies using the empirical null distribution. Genome Biol. 18:19.
van Zuiden, M., Geuze, E., Willemen, H. L., Vermetten, E., Maas, M., Amarouchi, K., et al. (2012). Glucocorticoid receptor pathway components predict posttraumatic stress disorder symptom development: a prospective study. Biol. Psychiatry 71, 309–316. doi: 10.1016/j.biopsych.2011.10.026
Weathers, F. W., Keane, T. M., and Davidson, J. R. (2001). Clinician-administered PTSD scale: a review of the first ten years of research. Depress Anxiety 13, 132–156. doi: 10.1002/da.1029
Weathers, F. W., Litz, B. T., Herman, D. S., Huska, J. A., and Keane, T. M. (1993). “The PTSD checklist (PCL): reliability, validity, and diagnostic utility,” in Proceedings of the Annual Conference for the International Society for Traumatic Stress Studies, (San Antonio, TX).
Wolf, E. J., Mitchell, K. S., Koenen, K. C., and Miller, M. W. (2014). Combat exposure severity as a moderator of genetic and environmental liability to post-traumatic stress disorder. Psychol. Med. 44, 1499–1509. doi: 10.1017/s0033291713002286
Wray, N. R., Ripke, S., Mattheisen, M., Trzaskowski, M., Byrne, E. M., Abdellaoui, A., et al. (2018). Genome-wide association analyses identify 44 risk variants and refine the genetic architecture of major depression. Nat. Genet. 50, 668–681.
Xu, X. F., Li, T., Wang, D. D., Chen, B., Wang, Y., and Chen, Z. Y. (2015). Integrin-linked kinase is essential for environmental enrichment enhanced hippocampal neurogenesis and memory. Sci. Rep. 5:11456.
Xu, X. F., Wang, Y. C., Zong, L., Chen, Z. Y., and Li, Y. (2018). Elevating Integrin-linked Kinase expression has rescued hippocampal neurogenesis and memory deficits in an AD animal model. Brain Res. 1695, 65–77. doi: 10.1016/j.brainres.2018.05.024
Yeager, D. E., Magruder, K. M., Knapp, R. G., Nicholas, J. S., and Frueh, B. C. (2007). Performance characteristics of the posttraumatic stress disorder checklist and SPAN in Veterans Affairs primary care settings. Gen. Hosp. Psychiatry 29, 294–301. doi: 10.1016/j.genhosppsych.2007.03.004
Yehuda, R., Cai, G., Golier, J. A., Sarapas, C., Galea, S., Ising, M., et al. (2009). Gene expression patterns associated with posttraumatic stress disorder following exposure to the World Trade Center attacks. Biol. Psychiatry 66, 708–711. doi: 10.1016/j.biopsych.2009.02.034
Yehuda, R., Halligan, S. L., and Bierer, L. M. (2001). Relationship of parental trauma exposure and PTSD to PTSD, depressive and anxiety disorders in offspring. J. Psychiatr. Res. 35, 261–270. doi: 10.1016/s0022-3956(01)00032-2
Zhang, B., and Horvath, S. (2005). A general framework for weighted gene co-expression network analysis. Stat. Appl. Genet. Mol. Biol. 4:17.
Keywords: posttraumatic stress disorder, gene expression, major depressive disorder, quantitative trait loci, multi-ethnic, meta-analysis
Citation: Garrett ME, Qin XJ, Mehta D, Dennis MF, Marx CE, Grant GA, VA Mid-Atlantic MIRECC Workgroup, PTSD Initiative, Injury and Traumatic Stress (2021) Clinical Consortium, Psychiatric Genomics Consortium PTSD Group, Stein MB, Kimbrel NA, Beckham JC, Hauser MA and Ashley-Koch AE (2021) Gene Expression Analysis in Three Posttraumatic Stress Disorder Cohorts Implicates Inflammation and Innate Immunity Pathways and Uncovers Shared Genetic Risk With Major Depressive Disorder. Front. Neurosci. 15:678548. doi: 10.3389/fnins.2021.678548
Received: 09 March 2021; Accepted: 07 July 2021;
Published: 29 July 2021.
Edited by:
Adriana Lori, Emory University, United StatesReviewed by:
Laura Beth Kozell, Oregon Health and Science University, United StatesErika J. Wolf, VA Boston Healthcare System, United States
Copyright © 2021 Garrett, Qin, Mehta, Dennis, Marx, Grant, VA Mid-Atlantic MIRECC Workgroup, PTSD Initiative, Injury and Traumatic Stress (INTRuST) Clinical Consortium, Psychiatric Genomics Consortium PTSD Group, Stein, Kimbrel, Beckham, Hauser and Ashley-Koch. This is an open-access article distributed under the terms of the Creative Commons Attribution License (CC BY). The use, distribution or reproduction in other forums is permitted, provided the original author(s) and the copyright owner(s) are credited and that the original publication in this journal is cited, in accordance with accepted academic practice. No use, distribution or reproduction is permitted which does not comply with these terms.
*Correspondence: Allison E. Ashley-Koch, YWxsaXNvbi5hc2hsZXlrb2NoQGR1a2UuZWR1