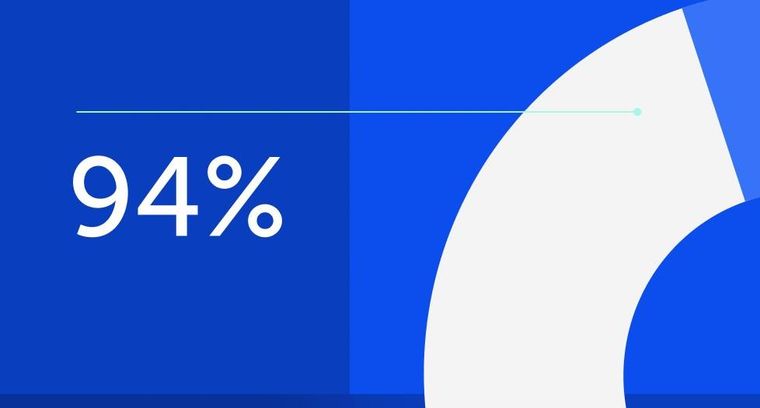
94% of researchers rate our articles as excellent or good
Learn more about the work of our research integrity team to safeguard the quality of each article we publish.
Find out more
ORIGINAL RESEARCH article
Front. Neurosci., 22 April 2021
Sec. Neuroendocrine Science
Volume 15 - 2021 | https://doi.org/10.3389/fnins.2021.670322
Endocrine state is an important determinant of learning and memory in animals. In Drosophila, rejection of male courtship overtures by mated females leads to an aversive response manifested as courtship memory. Here we report that ecdysis triggering hormone (ETH) is an obligatory enabler of long-term courtship memory (LTM). ETH deficiency suppresses LTM, whereas augmented ETH release reduces the minimum training period required for LTM induction. ETH receptor knockdown either in the mushroom body (MB) γ lobe or in octopaminergic dorsal-anterior-lateral (DAL) neurons impairs memory performance, indicating its direct action in these brain areas. Consistent with these findings, brain exposure to ETH mobilizes calcium in MB γ lobe neuropils and DAL neurons. ETH receptor (ETHR) knockdown in the corpus allatum (CA) to create juvenile hormone (JH) deficiency also suppresses LTM, as does knockdown of the JH receptor Met in the MB γ lobe, indicating a convergence of ETH and JH signaling in this region of the brain. Our findings identify endocrine-enabled neural circuit components in the brain that are critical for persistent behavioral changes resulting from aversive social experience.
Social experiences profoundly influence internal states and subsequent behavioral patterns in a broad range of animal species, including the fruit fly, Drosophila melanogaster (Chabaud et al., 2009; Shohat-Ophir et al., 2012). Such experience-based modulation of social interactions in fly communities may be critical for survival and propagation (Siegel and Hall, 1979; Svetec and Ferveur, 2005; Krupp et al., 2008). An example of social context-dependent behavioral plasticity in Drosophila is courtship memory, whereby rejection of male courtship overtures by mated females suppresses subsequent courtship attempts, even toward receptive virgin female partners (Siegel and Hall, 1979; McBride et al., 1999). Previous studies have reported structural and molecular components of courtship memory that are shared with olfaction-based memories. In particular, both are triggered by chemosensory inputs, chief among which are pheromones. However, courtship memory cannot be explained by olfactory influences alone, since males exposed to mated females experience multimodal aversive sensory inputs, in particular behavioral cues associated with rejection by the female during training (Keleman et al., 2012; Lee et al., 2017). Furthermore, distinct neural pathways involved in memory recall depend on the reproductive state (mated vs. virgin) of target females during the post-training test session (Ejima et al., 2007; Keleman et al., 2012). This type of associative memory may be influenced by additional internal components, among which could be endocrine state. While the steroid ecdysone promotes both short- and long-term courtship memories through distinct neural pathways (Ishimoto et al., 2009, 2013), how hormonal influences regulate learning and memory processes under courtship conditioning is still poorly understood.
Hormonal cascades drive ecdysis, a critically important innate behavior required for shedding of old cuticle and advancement to the next developmental stage (Zitnan et al., 2003). This fixed action pattern is initiated by circulating peptides called ecdysis triggering hormones (ETHs). ETHs, released by epitracheal gland Inka cells, orchestrate downstream peptidergic neuronal ensembles leading to sequential behaviors (Zitnan et al., 1996; Kim et al., 2006, 2015). ETH stimulates target cells via ETH receptor (ETHR)-mediated mobilization of calcium (Kim et al., 2006; Lee et al., 2017). Interestingly, Inka cells and associated transcripts of ETH and ETHRs persist into the adult stage of Drosophila (Park et al., 2002; Graveley et al., 2011). The adult roles of ETH signaling are just beginning to be unraveled and include reproductive fitness along with learning and memory.
We recently reported that ETH has an allatotropic function; i.e., promotion of juvenile hormone biosynthesis in adult Drosophila (Lee et al., 2017; Meiselman et al., 2017). ETHRs expressed in the corpus allatum (CA), the sole source of juvenile hormone (JH), regulate intracellular calcium levels in response to ETH. In females, ETH-driven JH synthesis is essential for reproductive fitness, while this hormonal cascade also affects male reproduction, possibly through regulation of accessory gland protein synthesis.
Another important functional role for the adult ETH-JH signaling cascade is regulation of short-term memory (STM) retention of males following courtship conditioning (Lee et al., 2017), whereby JH targets dopaminergic (DA) neurons. In this study, we provide evidence that hormonal states established by ETH and JH are also essential for long-term memory (LTM) performance following extended courtship conditioning. In this context, we report here that ETH actions on brain circuits are both indirect and direct: (1) ETH acts directly on dorsal anterior lateral (DAL) neurons and in concert with JH on mushroom body (MB) neurons, and (2) ETH acts indirectly through promotion of JH production. Our results provide evidence for a critical role of endocrine state in enabling social context-dependent behavioral modification and provide an approach for identification of neural circuit components through selective hormone receptor silencing.
Drosophila melanogaster stocks were maintained at 25°C on standard cornmeal-agar media under a 12-h light/dark (LD) regimen, except for certain experimental manipulations. Canton-S flies were used as wild-type. To reduce genetic background, all lines in this study were outcrossed to a wild-type line, wCS10 for at least five generations. JHAMT-GAL4 was obtained from B. Dauwalder (University of Houston) (Wijesekera et al., 2016). ETH-GAL4 was obtained from D. Anderson (California Institute of Technology). ETH-GS-GAL4, designated here as “EUG8,” was described previously (Cho et al., 2014). ETHR-GAL4 lines were obtained from B. White (National Institute of Mental Health) (Diao et al., 2016). UAS-hid,rpr was provided by P. Taghert (Washington University). An elav-GS-GAL4 driver GSG301 was provided by R. Davis (The Scripps Research Institute, Florida) (Osterwalder et al., 2001). 4.59 line was provided from U. Heberlein (Janelia Research Campus) (Kaun et al., 2011). UAS-ETHR RNAi lines (ETHRi-Sym, ETHRi-IR2) targeting independent sequences of the receptor are described previously (Kim et al., 2015). UAS-Met RNAi and UAS-gce RNAi lines were obtained from the Vienna Drosophila Research Center. The following Split-GAL4 lines were obtained from the Janelia Research Campus: MB009B, MB011B, MB093C, MB131B, and MB399B. Specificities of these driver lines are described on the Janelia Research “FlyLight Split Gal4 Driver Collection” website1. The following lines were obtained from the Bloomington Stock Center: OK107, 201Y, c739, 1471, TH-GAL4, Orco-GAL4, Dilp2-GAL4, G0338, G0431, UAS-dTrpA1, UAS-mCD8-GFP, UAS-Shits, and UAS-GCaMP5.
Animals were prepared for courtship conditioning as previously described (Lee et al., 2017). In short, males of appropriate strain were collected during the pupal stage and individually housed in clean glass tubes containing fresh food for 4 days posteclosion to prevent pre-test social interaction. To prevent anesthetic effects, we used a mouth aspirator to transfer or collect animals. Trainer females were prepared by housing day 3–5 virgin Canton-S females with Canton-S males in food-containing glass tubes overnight. Immobilized tester females were prepared by decapitating day 4–5 virgin Canton-S females with fine scissors under CO2 anesthesia. Protocols for long-term courtship conditioning followed those described previously (Keleman et al., 2007; Ishimoto et al., 2009), with some modifications. Briefly, a 4-day old male was paired with a mated Canton-S female for 5 h in the food chamber (2.0 ml tube). Trained (or sham-trained) males were individually housed in a fresh food tube for 24 h prior to testing, whereupon courtship activity of a trained (or sham-trained) male toward an immobilized (decapitated) tester female was recorded for 10 min using a high-frame digital camcorder (SONY HDR-XR260V). All assays were manually scored for courtship index, blind to the genotype and to the extent possible, the experimental condition. Positive contributions to courtship index (CI), defined as the percentage of time a male performs courtship behavior over a 10 min interval, included all courtship activities such as orienting, tapping, singing, licking, and attempting to copulate.
Courtship memory is quantified through computation of a memory performance index (MPI), which is the relative reduction of CI of individual trained male (iCIT) from the mean CI of sham-trained males (mCIS):
Statistical analyses were carried out using the Mann–Whitney U test for comparison between CI of trained and sham-trained males. For comparison between individual MPI of each control group and test group, a custom R script was developed to perform a 100,000 random permutation test (Keleman et al., 2007; Lee et al., 2017). Asterisks (∗) indicate statistical difference between MPI of test males and that of each control group, except in cases indicated in figure legends (∗p < 0.05; ∗∗p < 0.01; ∗∗∗p < 0.001; ns, not significant).
For TARGET experiments shown in Figure 2C, flies were raised at 19°C during the juvenile period, then transferred within a day of eclosion to 31°C for 4 days until training. Immediately following training at room temperature (20–21°C) for 5 h, flies were again transferred to 31°C for 24 h until testing at room temperature. For experiments depicted in Figure 4B, flies were raised at 19°C during the juvenile period and individually housed at 19°C for 6 days following eclosion. Flies were then transferred to 31°C until initiation of courtship conditioning, which was conducted at room temperature (20–21°C).
For experiments involving shibire (Figure 1C), flies were raised at 19°C during the juvenile period and housed at 31°C for 4 days following eclosion. For the training session and 24-h post-training interval, flies were maintained either at 21 or 31°C; testing was performed at room temperature.
Figure 1. ETH signaling regulates Drosophila courtship LTM. (A) Schematic of the long-term courtship conditioning procedure. (B) ETH deficiency was created by ablation of Inka cells through conditional ectopic expression of apoptosis genes hid and rpr under the control of RU486-activated GeneSwitch GAL4. Transgenic animals show significant reduction of MPI when treated with RU486 as compared to controls (n = 54–56). (C) Conditional block of vesicular secretion by Inka cells was achieved through expression of temperature-dependent dynamin mutant shibire in Inka cells. Courtship conditioning was conducted at the restrictive temperature, while testing occurred at room temperature. Transgenic flies exhibit less suppression of courtship activities following training than those of control groups (n = 48–52). Activation of adult Inka cells during 3- (D), and 1-h (D′) training with a mated female at 31°C utilizing dTrpA1 overexpression. ETH-GAL4/UAS-dTrpA1 males show 22.5% (D) and 16.9% (D′) courtship suppression following 3- and 1-h training at 31°C, respectively, compared to sham-trained males (n = 60–80). Light blue bars indicate MPI levels attained following a 5-h training protocol at 21°C, whereas dark blue bars depict significant reduction of MPI levels following 3- (D) or 1-h (D′) training protocols at 21°C. (E) Effect of Inka cell activation during 5-h training on persistence of memory performance. Training and sham-training of all animals were performed at 31°C. Asterisks represent statistical differences between data from test groups and GAL4 and UAS genetic control groups (n = 64–78). Mann–Whitney U test for CI and random permutation test for MPI; *p < 0.05, **p < 0.01, and ***p < 0.001. ns, no significance.
Figure 2. ETHR expression in the MB γ lobe is essential for courtship LTM performance. (A) Conditional knockdown of ETHR-encoding genes was achieved using the drug-inducible pan-neuronal GeneSwitch system (GSG301) by feeding RU486 for 3 days to day 4 males. LTM is lost in both GSG301/UAS-ETHR RNAi-Sym and GSG301/UAS-ETHR RNAi-IR2 males (n = 60–64). (B) ETHR knockdown using two independent RNAi lines in MB neurons sharply reduces MPI (n = 60–66). (C) Conditional ETHR silencing in adult MB neurons results in courtship LTM impairment (n = 64–72). (D,E) ETHR knockdown in MB γ-containing neurons impairs courtship LTM: (D) GAL4 lines utilized in this study: 201Y (αβ and γ), c739 (αβ), 4.59 (α′β′), and 1,471 (γ). Expression patterns were confirmed by crossing UAS-mCD8-GFP; (E) memory performances of MB lobe-specific ETHR-silenced males (n = 64–70). Random permutation test, *p < 0.05; **p < 0.01; and ***p < 0.001; ns, no significance.
For dTrpA1 experiments (Figures 1D,E), male flies were raised at 19°C during the first 4 days of adulthood, then housed at either 31 or 21°C with a mated female for the indicated training periods (3- and 1-h training). After a 24-h interval period at 19°C, memory performance was tested by pairing an individual male with an immobilized (decapitated) virgin female at room temperature.
Drug treatment associated with GeneSwitch experiments was described previously (McGuire et al.,2004a,b). Briefly, flies carrying the transgene EUG8-GAL4 were fed 200 μM mifepristone (RU486, Sigma) in normal fly food beginning immediately after eclosion for 4 days (Figure 1B), and from day 4 to 7 (Figures 2A, 5A) until courtship conditioning. Ethanol (1.6%) was used as vehicle. The JH analog methoprene (JHA; 1x, 64.4 pmol or 10×, 644 pmol) was applied topically in acetone to the ventral abdomen of day 0 posteclosion males held under cold anesthesia using a Nanoject II (Drummond).
Brains dissected from adults 4–5 days after eclosion were fixed in 4% paraformaldehyde at 4°C, washed sequentially with PBS and PBST (0.5% Triton X-100 in PBS), then blocked with 3% normal goat serum (NGS) for 1 h at room temperature. Primary antibody incubation in PBST with 3% NGS at 4°C overnight was followed by incubation in fluorophore-conjugated secondary antibodies overnight at 4°C. Brains were mounted in Aqua Poly/Mount on slides for imaging. Primary antibodies used were rabbit anti-GFP IgG (1:500, Invitrogen) and mouse anti-Bruchpilot (nc82) (1:50, DSHB). Fluorophore-conjugated secondary antibodies were Alexa Fluor 488 goat anti-rabbit (1:200, Invitrogen) and Alexa Fluor 568 goat anti-mouse (1:200, Invitrogen). Confocal images were acquired with a Zeiss LSM 510 microscope. To obtain clearer expression patterns for some GAL4 lines, image colors were inverted using raster graphic software (Adobe Photoshop CC).
Ca2+ dynamics were monitored via functional imaging utilizing the genetically encoded Ca2+ reporter GCaMP5 (Akerboom et al., 2012) expressed in specific neuronal populations using the GAL4/UAS system. Brains were extirpated and maintained in ice-chilled fly saline during recordings. Fluorescence responses were visualized with a TILL-Imago CCD camera mounted on an Olympus BX51W1 microscope; Live Acquisition software was used for control of a Polychrome V monochronometer (excitation wavelength 488 nm). Excitation pulses (488 nm, 50 ms duration) were applied at 1 Hz and emission was recorded continuously via a 515 long pass filter. Following 2-min of pre-application sampling, Drosophila ETH1 (DmETH1) was applied.
Mature virgin Drosophila females are generally receptive to male courtship advances, whereas recently mated females reject mating attempts. Following rejection or exposure to male-specific anti-aphrodisiac pheromones from mated females, males suppress subsequent courtship attempts regardless of female receptivity (Ejima et al., 2007). Furthermore, male courtship suppression toward a new virgin partner can be short- (∼1–2 h following 1-h training) or long-lasting (>24 h after 5–7-h training), depending on the duration of sexual rejection experienced during encounters with unreceptive females (McBride et al., 1999). Altered male behaviors following courtship conditioning for short or longer periods constitute courtship STM and LTM, respectively.
In a previous report, we showed that genetic disruption of the ETH-JH hormonal cascade impairs courtship STM, whereby normal males suppress courtship overtures by ∼30% toward an immobilized virgin female for up to 2 h following a 1-h training interval with a mated female (Lee et al., 2017). To investigate whether disruption of ETH signaling also affects courtship LTM, we applied an extended courtship conditioning protocol (see section “Materials and Methods” for details) (Figure 1A). Following a 5 h training period, we observed suppression of male courtship behavior between 14 and 20%, expressed as MPI when assayed 24 h after completion of training.
We first tested whether ETH deficiency affects LTM by conditionally ablating Inka cells posteclosion in order to avoid lethal ecdysis deficiencies during development (Park et al., 2002). This was accomplished by preparing transgenic flies expressing apoptosis genes (hid and rpr) specifically in Inka cells using the drug-inducible GeneSwitch driver (ETH-GS-GAL4, designated as EUG8) (Cho et al., 2014). We found that adult-specific ablation of Inka cells by feeding males (EUG8 > UAS-hid,rpr) the GAL4 activator RU486 impairs memory performance. RU486 feeding had no effect on memory performance of a genetic control group (Figure 1B). Likewise, Inka cell-ablated males showed no significant changes in basal courtship activities toward immobilized virgin females; i.e., the CI of sham-trained males was unchanged.
We next used an alternative strategy for creating ETH deficiency by blocking vesicular secretion by Inka cells via activation the dominant-negative temperature sensitive dynamin mutant shibire (UAS-Shits1) (Kitamoto, 2001). Blocking Inka cell release at the restrictive temperature during both maturation and courtship conditioning periods impaired LTM performance without affecting basal courtship activity (Figure 1C). These data indicate that Inka cell secretory activity is essential for normal courtship LTM performance of males.
We have shown that ETH deficiency during adulthood suppresses courtship LTM performance (Figure 1). To test whether augmented ETH release from Inka cells during the training period affects memory performance, we expressed the temperature-sensitive cation channel, Drosophila TrpA1 (dTrpA1) in Inka cells using the ETH-GAL4 driver. In our extended courtship conditioning protocol (described in the “Materials and Methods” section), male flies require a minimum of 5-h training with a mated female to show significant courtship suppression after 24 h (Figures 1D,D′; light blue bars). Reduction of the training period to 3 h or 1 h caused reduction of MPI to levels below 4% (Figures 1D,D′; dark blue bars).
We asked whether augmented Inka cell activity through dTrpA1 activation could enhance memory performance during shorter training periods. When paired with a mated female for a 3-h training period at the TrpA1 permissive temperature, substantial enhancement of MPI was observed (Figure 1D), reaching p < 0.01 compared to GAL4 and UAS controls. When the training period was reduced further to only 1-h, enhancement reached p < 0.05 for the UAS control and p = 0.057 for the GAL4 control, suggesting that significant enhancement also occurred following this minimal training period. (Figure 1D′). Genetic control groups did not show detectable courtship suppression by 3- or 1-h training at both temperature conditions. No significant difference in basal courtship activity was observed in sham-trained ETH-GAL4/UAS-dTrpA1 males toward an immobilized tester virgin female following heat-activation compared to the genetic control groups (Supplementary Figure 1).
We next asked whether augmented Inka cell activity affects maintenance of memory following long-term courtship conditioning. Although Drosophila courtship LTM following 5-h training with a mated female lasts at least several days, the intensity of memory performance decays gradually over this period (McBride et al., 1999). Significant enhancement of MPI for ETH-GAL4/UAS-dTrpA1 males trained for 5 h at 31°C over controls was not observed after 24 h, given the relatively high MPI of controls (see Figure 1E). However, the test male group showed a significant increase of MPI 48-h after training compared to both genetic control groups (Figure 1E). Thus, we conclude that augmented Inka cell activity during the 5-h training extends a high rate of memory performance (>20%) to 48 h after training.
Although functions of ETH-ETHR signaling in central neurons of juvenile animals have been described (Kim et al., 2006, 2015; Diao et al., 2016; Mena et al., 2016), presence of ETHRs in the adult brain (Supplementary Figure 2) suggests their possible roles in behavioral regulation in adults. In particular, a recent study described expression of ETHRs in several neuronal subtypes of memory-related circuits in the adult brain through cell-specific transcriptome analyses (Crocker et al., 2016). Although their findings suggest low levels of ETHR expression in MB neurons, we used the genotype ETHR-GAL4 (MH1506) > UAS-mCD8-GFP to identify a subset of cells (approximately 2 μm diameter) in the MB calyx area (Supplementary Figure 2C).
We first asked whether RNA knockdown of ETHR in the nervous system affects courtship LTM. We employed adult-specific RNA knockdown of ETHR genes using the conditional pan-neuronal GeneSwitch driver elav-GS-GAL4 (GSG301) enabled by RU486 (Osterwalder et al., 2001; Ford et al., 2007). Employing ETHR RNA knockdown with two independent RNAi constructs (Kim et al., 2015) targeting distinct regions common to the ETHR-A and ETHR-B sequences, we obtained strong suppression of courtship LTM performance (Figure 2A).
To examine the role of ETHR expression in MB neurons, we first used the pan-MB GAL4 driver OK107 to effect RNA knockdown of ETHRs and observed strong suppression of courtship LTM performance using two dsRNA constructs targeting distinct regions of the ETHR gene (UAS-ETHR RNAi-Sym and UAS-ETHR RNAi-IR2) (Figure 2B). In contrast, ETHR silencing in MB neurons did not show deficit in STM performance (Supplementary Figure 3A) following 1-h training, suggesting that these two forms of courtship memory arise through independent mechanisms. To confirm the requirement of ETHR expression in posteclosion adult MB neurons for courtship LTM, we applied the temporal and regional gene expression targeting (TARGET) system (McGuire et al.,2004a,b), again using the OK107 driver and found that RNA knockdown of ETHR genes in the entire MB during adulthood also severely impairs courtship LTM (Figure 2C). ETHR silencing did not affect the size or gross morphology of MB lobes when we performed the immunostaining against FasII (Supplementary Figure 3B).
The MB consists of three major classes of neuropils: the αβ, α′β′, and γ (Figure 2D). To more precisely identify specific functional targets of ETH regulating courtship LTM, we performed knockdown of ETHR genes using a set of GAL4 drivers specific for the following neuropil areas: αβ and γ (201Y), αβ (c739), α′β′ (4.59), and γ (1471). ETHR knockdown using the 201Y and 1,471 drivers significantly impaired courtship LTM, whereas memory performance was unaffected by ETHR silencing in the αβ lobe (c739) (Figure 2E). Although slight suppression of LTM performance occurred following ETHR knockdown with the 4.59 driver, reduction did not reach statistical significance. Taken together, our data thus suggest that ETHR expression in the MB γ lobe is required for courtship LTM performance.
Ecdysis triggering hormone receptor expression in the adult brain (Supplementary Figure 2; Crocker et al., 2016) suggests that ETH could target multiple neuronal circuits. We therefore selected an array of Split-GAL4 lines with high regional specificity to test whether ETHR silencing affects courtship LTM: MB009B and MB131B driving expression in MB γ neurons exclusively (Masek et al., 2015), along with MB093C, MB011B, and MB399B expressing in MB output neurons (MBONs) (Aso et al., 2014). To target the DAL neurons, the drivers G0338 and G0431 were employed (Chen et al., 2012).
Consistent with results shown in Figure 2E, RNA knockdown of ETHRs in the MB γ lobe abolished differences in CI between sham-trained and courtship-conditioned males (Figure 3A). MPI reduction following ETHR knockdown via the MB γ lobe-specific driver MB131B/UAS-ETHR RNAi-Sym was significant (p < 0.01), whereas the MB009B/UAS-ETHR RNAi-Sym trended close to significance at the 95% level (p = 0.057).
Figure 3. ETH targets specific memory circuit neurons to regulate courtship LTM. (A) ETHR knockdown in utilizing an array of candidate neuronal driver lines. Mann–Whitney U test for CI and random permutation test for MPI: *p < 0.05; **p < 0.01; ***p < 0.001; ns, no significance. (B) Expression patterns of MB131B and G0431 drivers, which show memory defects following ETHR knockdown in panel (A), were confirmed by crossing UAS-mCD8-GFP. To improve contrast, colors were inverted: purple, GFP; blue, Fas-II (MB). (C,C′) ETH mobilizes Ca2+ in the MB γ lobe [(C), MB131B/UAS-GCaMP5] and DAL neurons [(C′), G0431/UAS-GCaMP5]. Upper representative images show before (left) and after (right) exposure to 1 μM DmETH1. Bottom: averaged traces of ΔF/F0 following saline (black) and 1 μM DmETH1 (purple or blue). Arrowheads represent saline or ETH application. Darker lines show means, and the light envelopes represent SEM. Parentheses indicate the number of recordings.
Figure 4. ETH-JH hormonal cascade is essential for courtship LTM. (A) The role of ETH-JH signaling in courtship LTM was examined following ETHR knockdown in the CA to create JH deficiency. CA-specific ETHR silencing leads to memory defect, whereas both genetic control groups are normal (n = 50–64). (B) Conditional ETHR silencing in the CA via the TARGET system. Adult-specific ETHR silencing causes a significantly less memory performance by training compared to genetic controls (n = 58–66). (C) Rescue of memory phenotype in ETHR-silenced males by topical treatment with the JH analog methoprene (n = 48–62): “–,” vehicle (acetone); “+,” methoprene (1x, 64.4 pmol; 10x, 644 pmol per animal). Vehicle-treated JHAMT-GAL4/UAS-ETHR RNAi males suppress courtship by 2.3%, while and 1x JHA-treated males show 7.0% suppression of courtship; the MPI of 10x JHA-treated males is 10.9%. Mann–Whitney U test for CI and random permutation test for MPI: *p < 0.05; **p < 0.01; ns, no significance.
Figure 5. Hormonal convergence for courtship LTM regulation. (A) Loss of LTM in GSG301/UAS-gce RNAi;UAS-Met RNAi and GSG301/UAS-Met RNAi males, whereas GSG301/UAS-gce RNAi males does not show significantly different memory performance compared to vehicle-treated animals (n = 62–68). (B) RNA knockdown of Met in ETH-targeted neurons: MB γ lobe-containing neuronal drivers (OK107, 201Y, 1,471, MB131B, and MB009B) significantly impair LTM performance by Met-silencing (n = 60–62). Random permutation test, *p < 0.05; **p < 0.01; ns, no significance. (C) A model for the endocrine system function in courtship memory induced by long-term conditioning. Proposed model as described in the text depicting the direct and indirect pathways of ETH signaling and hormonal convergence of ETH and JH in regulation of Drosophila courtship LTM in response to repeated failure in courtship.
We also observed that ETHR knockdown using both DAL neurons drivers (G0431 and G0338) abolished CI differences between sham-trained and courtship-conditioned males (Figure 3A). While memory performance (MPI) using the DAL neuronal driver G0431 was impaired significantly (p < 0.05), results using the G0338 driver trended toward significance (p = 0.061).
While the CI comparisons were made with identical genotypes (sham-trained GAL4 > UAS vs trained GAL 4 > UAS), the MPI comparisons were made between different genotypes (GAL4 control vs Gal4 > UAS). This appears to have introduced an additional variability factor that may account for loss of significance at the p < 0.05 threshold for the MB009B-Gal4 and G0338-Gal4 drivers.
In contrast, ETHR knockdown using all MBON driver lines did not abolish CI differences between sham-trained and courtship-conditioned males and led to no statistically significant differences in MPI compared to genetic control groups. Adult-specific RNA knockdown of ETHRs in olfactory (Orco-GAL4), dopaminergic (TH-GAL4), and insulin-producing cells (Dilp2-GAL4) also did not result in significant changes in courtship LTM performance (Supplementary Figure 4A).
In vitro functional imaging of the entire MB shows gradual elevation of Ca2+-mediated fluorescence mainly in the lateral neuropils of the MB in response to Drosophila ETH (DmETH1) application (Supplementary Figure 4B). We tested the sensitivity of MB γ neurons and DAL neurons to ETH by crossing MB131B and G0431 driver lines (Figure 3B) associated with the strongest memory phenotype with the UAS-GcaMP5 line. DmETH1 application led to robust Ca2+ mobilization in both MB γ lobe and DAL neurons over a 30–40 min period (Figures 3C,C′). Together, these results suggest that ETH targets MB γ lobe and DAL neurons to regulate courtship LTM.
We previously reported that suppression of ETH signaling through ETHR knockdown specifically in the CA (JHAMT-GAL4/UAS-ETHR RNAi-Sym) leads to a 70% reduction in JH levels and a consequent reduction of STM performance (Lee et al., 2017). To investigate whether this hormonal cascade also contributes to courtship LTM regulation, we subjected the same genotype to long-term courtship conditioning and found that JH-deficient males show sharply reduced courtship LTM performance without a significant change in courtship activity toward tester virgin females (Figure 4A).
To confirm the necessity of JH in LTM performance, we employed two experimental strategies. We first delayed ETHR knockdown in the CA until 6 days posteclosion by applying the TARGET system (JHAMT-GAL4/UAS-ETHR RNAi-Sym;TubPGAL80ts). The objective of this experiment was to allow gene expression-dependent brain maturation to occur prior to alteration of hormonal state. We found that ETHR knockdown in these animals also impaired courtship LTM significantly, whereas both genetic control groups under identical experimental conditions showed normal memory performance (Figure 4B).
We next applied the JH analog methoprene at two doses (1x, 64 pmol and 10x, 640 pmol) to JH-deficient adult males and achieved rescue of memory performance (Figure 4C). The MPI rescue achieved by the higher methoprene dose was not statistically different from that of the GAL4 control. Methoprene treatment had no effect on courtship memory performance of a control group. Thus, the ETH-JH signaling cascade is essential for courtship LTM performance in mature adult males.
Since we have demonstrated that the ETH-JH signaling cascade is required for courtship LTM and that augmented Inka cell activity during the training session improves LTM performance (Figure 1), we also asked whether augmented CA activity through expression of dTrpA1 would enhance courtship LTM via increased JH levels. Previous studies showed that Ca2+ influx induces biosynthesis and release of JH in the CA (Kikukawa et al., 1987; Yamamoto et al., 1988; Allen et al., 1992; Hsieh et al., 2001; Chiang et al., 2002). We expressed dTrpA1 in the CA using the JHAMT-GAL4 driver, since this would be expected to depolarize CA cells. However, we and found that, unlike the improvement of memory performance obtained by augmented Inka cell activity, training JHAMT-GAL4/UAS-dTrpA1 males at 31°C did not alter courtship LTM performance (Supplementary Figure 5A). Therefore, improved MPI caused by Inka cell activation (Figure 1) appears not to be mediated by JH elevation, but instead through direct action of ETH on memory circuits. An important caveat associated with the negative result obtained following dTrPA1 expression in CA cells result is that depolarization-dependence of JH release in Drosophila has not been demonstrated. To that extent, the negative result reported here remains somewhat equivocal.
Genes encoding two JH receptor paralogs [Methoprene-tolerant (MET) and germ cell-expressed (GCE)] are expressed in the adult male brain (Baumann et al., 2010). We performed conditional RNA knockdown of JH receptor genes (Met and gce) using the elav-GS-GAL4 (GSG301) driver. Since functional redundancy and compensation between these two receptor types have been reported (Baumann et al., 2010; Abdou et al., 2011), we co-expressed RNAi constructs for Met and gce (UAS-gce RNAi;UAS-Met RNAi). RU486 treatment of transgenic animals significantly impaired courtship memory performance compared to the vehicle-treated group. We further demonstrated that Met-silencing alone is sufficient for courtship LTM impairment, whereas silencing of the gce gene alone results in no significant change in memory performance (Figure 5A).
We previously demonstrated that JH receptor (both MET and GCE) expression in TH (tyrosine hydroxylase)-positive neurons is required for courtship STM performance (Lee et al., 2017). We therefore asked whether these neurons are also involved in regulation of courtship LTM performance by silencing of both Met and gce genes using the TH-GAL4 driver. Interestingly, TH-GAL4/UAS-gce RNAi;UAS-Met RNAi males showed no significant change the memory performance following long-term courtship conditioning (Supplementary Figure 5B). This indicates that, although JH receptors in TH-positive neurons are required for regulation of courtship STM, they are not required for courtship LTM performance.
A recent study of JH receptor expression patterns in the brain of adult Drosophila (Baumann et al., 2017) demonstrated strong expression of Met in MB lobes and lateral dorsal neurons (LNd), including DAL neurons. We therefore tested whether Met RNA knockdown in these memory circuit neurons compromises courtship LTM (Figure 5B). Met silencing of the entire MB using the OK107 driver resulted in strong suppression of LTM performance without gross morphological changes of the MB (Supplementary Figure 3B). Similar to results obtained by silencing ETHR genes (Figure 2E), Met silencing in MB γ lobe-containing lines (201Y and 1,471) showed significant memory impairment, whereas silencing Met expression in αβ (c739) or α′β′ (4.59) line had no effect on courtship LTM performance. Since these GAL4 lines also drive gene expression in additional areas of the brain, specificity of action could be questioned. We therefore performed Met RNA knockdown using the more specific Split-GAL4 MB γ lobe-directed lines MB009B and MB131B (Figure 3A) and observed significant suppression of courtship LTM. We did not observe memory impairment from Met silencing in DAL neurons. These results indicate that Met expression in the MB γ lobe regulates courtship LTM. In summary, we conclude that converging influences of ETH and JH in this area of the MB is essential for courtship LTM.
We have demonstrated that hormonal state profoundly influences LTM performance in Drosophila courtship conditioning. ETH signaling operates both directly and indirectly to regulate memory performance in two distinct ways: (i) directly, by targeting MB γ lobe and DAL neurons; (ii) indirectly via allatotropic actions to promote JH production. Joint action of ETH and JH on MB γ lobe neurons is essential for courtship LTM. Augmentation of ETH levels during training enhances memory performance and reduces the minimum training period for LTM. Our findings implicate the joint action of ETH and JH as essential for courtship LTM and that this is independent of the courtship STM pathway described previously (Lee et al., 2017), leading us to propose a model for converging endocrine inputs in the CNS essential for LTM expression following aversive sexual experience (Figure 5C).
Disruption of Inka cell function in adult males impairs memory performance following long-term courtship conditioning. This result, together with loss of memory performance after targeted silencing of ETHR, provides evidence that deficiencies of either ligand or receptor compromise memory performance. These data clearly support the conclusion that ETH signaling is required for memory formation. We reported that the ecdysteroid hormones up-regulate ETH biosynthesis in juvenile (Cho et al., 2014) and adult (Meiselman et al., 2017) Drosophila. Ishimoto et al. (2009) found that long-term courtship conditioning leads to increased steroid levels in male flies. We therefore propose that repeated unsuccessful sexual experiences of males induce ETH production through elevation of ecdysteroids. The synthetic origin(s) of ecdysteroids in adult male flies remains unclear.
Augmented Inka cell activity during training enhances features of courtship memory—i.e., learning period and persistence of courtship suppression—without affecting basal courtship activity of naïve males. This suggests that the ETH levels are important not only for driving behavioral change but also for consolidating memory.
Our findings demonstrate direct actions of ETH on two distinct brain circuitries: MB γ lobe and DAL neurons. Several previous studies have revealed that these neural circuits play important roles in post-training memory processes such as consolidation, storage, and retrieval of information (Dubnau et al., 2001; Keleman et al., 2007; Chen et al., 2012; Qin et al., 2012). In particular, expression of Orb2A, a cytoplasmic polyadenylation element-binding (CPEB) protein in the MB γ lobe, is essential for Drosophila courtship memory formation (Keleman et al., 2007), by regulating synaptic strength through local protein synthesis in neurons (Kruttner et al., 2012, 2015). The role of synaptic Orb2A is CaMKII-dependent. Therefore, the ETHR-mediated signaling cascade in MB neurons may contribute to memory consolidation through this pathway. However, their courtship conditioning used mated females as tester, which contains anti-aphrodisiac pheromone cues whereas we employed mature virgin females. It is thus not clear whether ETHR-mediated signaling acts via Orb2A in MB neurons; this point requires further investigation.
Sakai et al. (2012a) reported that expression of the clock gene period (per) is essential for retrieval of courtship memory when they used virgin females as tester. Although per gene expression in DAL neurons contributes to memory consolidation after olfactory conditioning (Chen et al., 2012), courtship LTM does not require per expression in the DAL neurons. In future studies, it would be of great interest to identify interactions between intracellular signaling molecules controlling courtship memory in DAL neurons.
In line with previous reports of ETHR-mediated Ca2+ regulation in peptidergic neurons of juvenile flies (Kim et al., 2006; Diao et al., 2016), we find that ETH mobilizes Ca2+ in central neurons of mature adult males. We therefore hypothesize that ETHR-mediated intracellular Ca2+ signaling in memory circuit neurons provides a key modulatory input for courtship LTM. In a broad range of animal species, Ca2+ plays a critical role in memory formation. For example, Ca2+ entry through glutamate receptors in mammalian cortical neurons promotes phosphorylation of eEF2, a GTPase that mediates ribosomal translocation along mRNA following peptide bond formation, thereby contributing to long-lasting memory (Belelovsky et al., 2005). The crucial role of cytoplasmic or nuclear Ca2+ levels in memory formation has been suggested by several studies in both vertebrates and invertebrates (Meneses, 2007; Perisse et al., 2009; Weislogel et al., 2013). Previous studies also showed that a TRP channel encoded by painless (pain) (Sakai et al., 2012b) and the small conductance calcium-activated potassium (SK) channel (Abou Tayoun et al., 2012) expressions in the MB neurons are required for Drosophila courtship LTM, supporting our hypothesis of the essential role of Ca2+ dynamics in formation of this type of memory.
Ecdysis triggering hormone plays another important role in courtship LTM by manipulating JH levels. Suppression of JH production by ETHR silencing in the CA impairs male courtship LTM, while memory impairment can be rescued by the JH analog methoprene, thus demonstrating the essential contribution of the ETH-JH cascade to memory performance. Two observations in this study show that elevation of JH beyond normal levels does not improve memory performance. First, although methoprene rescues courtship LTM of JH-deficient males, high doses do not improve memory performance in either JH-deficient or control groups. Second, unlike the memory enhancement observed following Inka cell activation, reinforced CA function induced by TrpA1 activity during courtship conditioning does not affect LTM. We thus conclude that physiological levels of JH are sufficient for maintenance of memory circuits homeostasis. Our findings also demonstrate that the JH receptor Met is essential for courtship LTM. Importantly, knockdown of Met expression in the MB γ lobe, a target region of ETH, diminishes memory performance. Based on these findings, we propose a hormonal convergence model for courtship LTM (Figure 5C).
In mammals, interactions of neuromodulatory influences within strategic brain areas are essential for emotional memory consolidation. Stress-induced release of glucocorticoids (cortisol in humans) influences memory consolidation via both direct and indirect pathways that converge in the amygdala (McGaugh, 2004). In particular, secreted glucocorticoids promote activity of brainstem nuclei (nucleus of the solitary tract, NTS) that connect noradrenergic projections to the basolateral amygdala (BLA), thereby leading to memory formation. Glucocorticoid receptors present in the BLA also facilitate memory consolidation by potentiating noradrenergic signaling through interactions with G protein-mediated cascades.
Although ETH and JH converge on the MB γ lobe to regulate LTM, it remains unclear whether these hormones target the same MB neurons. Furthermore, even if they do target the same neurons, how signal transduction and possible new gene expression resulting from their actions contributes to LTM remain to be elucidated. As discussed, G protein-mediated Ca2+ signaling is critical for memory formation through diverse downstream mechanisms. Previous reports showing that JH promotes protein phosphorylation in moths and mosquitoes (Arif et al., 2002; Liu et al., 2015), provides some insights into possible joint actions of ETH-mediated GPCR signaling and JH-mediated Met function for promotion of diverse cellular responses. For example, ETHR-mediated Ca2+ mobilization may combine with JH receptor-mediated protein phosphorylation to enhance presynaptic protein synthesis. It will be important to investigate the detailed mechanisms underlying ETH- and JH-mediated signaling at the molecular level.
Previously, there has been a presumption that LTM is sequentially formed following the STM process (McGaugh, 1966). However, studies have reported that the circuit-based mechanisms underlying STM and LTM in diverse animal models can be independent and parallel (Botzer et al., 1998; Izquierdo et al., 1998, 1999; Blum et al., 2009; Sanderson et al., 2009; Trannoy et al., 2011; Yamagata et al., 2015). Our previous study showed that expression of JH receptors in dopaminergic (DA) neurons is essential for courtship STM (Lee et al., 2017). The function of JH in these neurons likely contributes to maintenance of information, even during the short-term interval following aversive experience. Nevertheless, we found in the current study that silencing of JH receptors in DA neurons does not affect courtship LTM performance. Instead, Met expression in the MB γ lobe neurons is critical for LTM. Thus, our findings argue for parallel pathways in regulation of courtship STM and LTM through independent neuronal targets of endocrine signals ETH and JH.
In summary, we have identified novel hormonal pathways that regulate 24-h lasting LTM following their induction by extended courtship conditioning in Drosophila. ETH modulates memory directly through Ca2+-mediated mechanisms in the MB lobe and DAL neurons, and indirectly through regulation of JH production. Furthermore, ETH and JH cascades converge in a functionally-distinct area of the MB that is critical for courtship LTM. Thus, we provide a testable model for the essential involvement of endocrine state in the formation of context-dependent social memories.
The raw data supporting the conclusions of this article will be made available by the authors, without undue reservation.
SL performed behavioral assays, immunohistochemistry, and functional Ca2+ imaging. Both authors designed the experiments and wrote the manuscript.
This work has been supported by the NIH (GM-067310), the UCR Agricultural Experiment Station, the UCR Office of Research, and the UCR Graduate Division.
The authors declare that the research was conducted in the absence of any commercial or financial relationships that could be construed as a potential conflict of interest.
We would like to thank Anandasankar Ray, Anupama Dahanukar, Naoki Yamanaka, and Xinping Cui for valuable discussions. We also would like to thank Ron Davis, Yoshi Aso, Ulrike Heberlein, Brigitte Dauwalder, David Anderson, Lynn Riddiford, and Paul Taghert for kindly providing fly lines. Facilities provided by the UCR Institute for Integrated Genome Biology were crucial to the success of the study.
The Supplementary Material for this article can be found online at: https://www.frontiersin.org/articles/10.3389/fnins.2021.670322/full#supplementary-material
Abdou, M. A., He, Q., Wen, D., Zyaan, O., Wang, J., Xu, J., et al. (2011). Drosophila met and gce are partially redundant in transducing juvenile hormone action. Insect. Biochem. Mol. Biol. 41, 938–945. doi: 10.1016/j.ibmb.2011.09.003
Abou Tayoun, A. N., Pikielny, C., and Dolph, P. J. (2012). Roles of the drosophila SK channel (dSK) in courtship memory. PLoS One 7:e34665. doi: 10.1371/journal.pone.0034665
Akerboom, J., Chen, T. W., Wardill, T. J., Tian, L., Marvin, J. S., Mutlu, S., et al. (2012). Optimization of a GCaMP calcium indicator for neural activity imaging. J. Neurosci. 32, 13819–13840. doi: 10.1523/JNEUROSCI.2601-12.2012
Allen, C. U., Janzen, W. P., and Granger, N. A. (1992). Manipulation of intracellular calcium affects in vitro juvenile hormone synthesis by larval corpora allata of Manduca sexta. Mol. Cell. Endocrinol. 84, 227–241. doi: 10.1016/0303-7207(92)90034-4
Arif, A., Shanavas, A., Murthy, ChR, and Dutta-Gupta, A. (2002). Juvenile hormone stimulated tyrosine kinase-mediated protein phosphorylation in the CNS of the silk worm, Bombyx mori. Arch. Insect. Biochem. Physiol. 50, 139–146. doi: 10.1002/arch.10038
Aso, Y., Sitaraman, D., Ichinose, T., Kaun, K. R., Vogt, K., Belliart-Guerin, G., et al. (2014). Mushroom body output neurons encode valence and guide memory-based action selection in drosophila. Elife 3:e04580. doi: 10.7554/eLife.04580
Baumann, A., Barry, J., Wang, S., Fujiwara, Y., and Wilson, T. G. (2010). Paralogous genes involved in juvenile hormone action in Drosophila melanogaster. Genetics 185, 1327–1336. doi: 10.1534/genetics.110.116962
Baumann, A. A., Texada, M. J., Chen, H. M., Etheredge, J. N., Miller, D. L., Picard, S., et al. (2017). Genetic tools to study juvenile hormone action in Drosophila. Sci. Rep. 7:2132. doi: 10.1038/s41598-017-02264-4
Belelovsky, K., Elkobi, A., Kaphzan, H., Nairn, A. C., and Rosenblum, K. (2005). A molecular switch for translational control in taste memory consolidation. Eur. J. Neurosci. 22, 2560–2568. doi: 10.1111/j.1460-9568.2005.04428.x
Blum, A. L., Li, W., Cressy, M., and Dubnau, J. (2009). Short- and long-term memory in Drosophila require cAMP signaling in distinct neuron types. Curr. Biol. 19, 1341–1350. doi: 10.1016/j.cub.2009.07.016
Botzer, D., Markovich, S., and Susswein, A. J. (1998). Multiple memory processes following training that a food is inedible in Aplysia. Learn. Mem. 5, 204–219.
Chabaud, M. A., Isabel, G., Kaiser, L., and Preat, T. (2009). Social facilitation of long-lasting memory retrieval in Drosophila. Curr. Biol. 19, 1654–1659. doi: 10.1016/j.cub.2009.08.017
Chen, C. C., Wu, J. K., Lin, H. W., Pai, T. P., Fu, T. F., Wu, C. L., et al. (2012). Visualizing long-term memory formation in two neurons of the Drosophila brain. Science 335, 678–685. doi: 10.1126/science.1212735
Chiang, A. S., Lin, W. Y., Liu, H. P., Pszczolkowski, M. A., Fu, T. F., Chiu, S. L., et al. (2002). Insect NMDA receptors mediate juvenile hormone biosynthesis. Proc. Natl. Acad. Sci. U.S.A. 99, 37–42. doi: 10.1073/pnas.012318899
Cho, K. H., Daubnerova, I., Park, Y., Zitnan, D., and Adams, M. E. (2014). Secretory competence in a gateway endocrine cell conferred by the nuclear receptor betaFTZ-F1 enables stage-specific ecdysone responses throughout development in Drosophila. Dev. Biol. 385, 253–262. doi: 10.1016/j.ydbio.2013.11.003
Crocker, A., Guan, X. J., Murphy, C. T., and Murthy, M. (2016). Cell-type-specific transcriptome analysis in the Drosophila mushroom body reveals memory-related changes in gene expression. Cell. Rep. 15, 1580–1596. doi: 10.1016/j.celrep.2016.04.046
Diao, F., Mena, W., Shi, J., Park, D., Diao, F., Taghert, P., et al. (2016). The splice isoforms of the Drosophila ecdysis triggering hormone receptor have developmentally distinct roles. Genetics 202, 175–189. doi: 10.1534/genetics.115.182121
Dubnau, J., Grady, L., Kitamoto, T., and Tully, T. (2001). Disruption of neurotransmission in Drosophila mushroom body blocks retrieval but not acquisition of memory. Nature 411, 476–480. doi: 10.1038/35078077
Ejima, A., Smith, B. P., Lucas, C., van der Goes van Naters, W., Miller, C. J., Carlson, J. R., et al. (2007). Generalization of courtship learning in Drosophila is mediated by cis-vaccenyl acetate. Curr. Biol. 17, 599–605. doi: 10.1016/j.cub.2007.01.053
Ford, D., Hoe, N., Landis, G. N., Tozer, K., Luu, A., Bhole, D., et al. (2007). Alteration of Drosophila life span using conditional, tissue-specific expression of transgenes triggered by doxycyline or RU486/Mifepristone. Exp. Gerontol. 42, 483–497. doi: 10.1016/j.exger.2007.01.004
Graveley, B. R., Brooks, A. N., Carlson, J. W., Duff, M. O., Landolin, J. M., Yang, L., et al. (2011). The developmental transcriptome of Drosophila melanogaster. Nature 471, 473–479. doi: 10.1038/nature09715
Hsieh, Y. C., Hsu, E. L., Chow, Y. S., and Kou, R. (2001). Effects of calcium channel antagonists on the corpora allata of adult male loreyi leafworm Mythimna loreyi: juvenile hormone acids release and intracellular calcium level. Arch. Insect. Biochem. Physiol. 48, 89–99. doi: 10.1002/arch.1061
Ishimoto, H., Sakai, T., and Kitamoto, T. (2009). Ecdysone signaling regulates the formation of long-term courtship memory in adult Drosophila melanogaster. Proc. Natl. Acad. Sci. U.S.A. 106, 6381–6386. doi: 10.1073/pnas.0810213106
Ishimoto, H., Wang, Z., Rao, Y., Wu, C. F., and Kitamoto, T. (2013). A novel role for ecdysone in Drosophila conditioned behavior: linking GPCR-mediated non-canonical steroid action to cAMP signaling in the adult brain. PLoS Genet. 9:e1003843. doi: 10.1371/journal.pgen.1003843
Izquierdo, I., Barros, D. M., Mello e Souza, T., de Souza, M. M., Izquierdo, L. A., and Medina, J. H. (1998). Mechanisms for memory types differ. Nature 393, 635–636. doi: 10.1038/31371
Izquierdo, I., Medina, J. H., Vianna, M. R., Izquierdo, L. A., and Barros, D. M. (1999). Separate mechanisms for short- and long-term memory. Behav. Brain Res. 103, 1–11. doi: 10.1016/s0166-4328(99)00036-4
Kaun, K. R., Azanchi, R., Maung, Z., Hirsh, J., and Heberlein, U. (2011). A Drosophila model for alcohol reward. Nat. Neurosci. 14, 612–619. doi: 10.1038/nn.2805
Keleman, K., Kruttner, S., Alenius, M., and Dickson, B. J. (2007). Function of the Drosophila CPEB protein Orb2 in long-term courtship memory. Nat. Neurosci. 10, 1587–1593. doi: 10.1038/nn1996
Keleman, K., Vrontou, E., Kruttner, S., Yu, J. Y., Kurtovic-Kozaric, A., and Dickson, B. J. (2012). Dopamine neurons modulate pheromone responses in Drosophila courtship learning. Nature 489, 145–149. doi: 10.1038/nature11345
Kikukawa, S., Tobe, S. S., Solowiej, S., Rankin, S. M., and Stay, B. (1987). Calcium as a regulator of juvenile hormone biosynthesis and release in the cockroach Diploptera punctata. Insect. Biochem. 17, 179–187.
Kim, D. H., Han, M. R., Lee, G., Lee, S. S., Kim, Y. J., and Adams, M. E. (2015). Rescheduling behavioral subunits of a fixed action pattern by genetic manipulation of peptidergic signaling. PLoS Genet. 11:e1005513. doi: 10.1371/journal.pgen.1005513
Kim, Y. J., Zitnan, D., Galizia, C. G., Cho, K. H., and Adams, M. E. (2006). A command chemical triggers an innate behavior by sequential activation of multiple peptidergic ensembles. Curr. Biol. 16, 1395–1407. doi: 10.1016/j.cub.2006.06.027
Kitamoto, T. (2001). Conditional modification of behavior in Drosophila by targeted expression of a temperature-sensitive shibire allele in defined neurons. J. Neurobiol. 47, 81–92. doi: 10.1002/neu.1018
Krupp, J. J., Kent, C., Billeter, J. C., Azanchi, R., So, A. K., Schonfeld, J. A., et al. (2008). Social experience modifies pheromone expression and mating behavior in male Drosophila melanogaster. Curr. Biol. 18, 1373–1383. doi: 10.1016/j.cub.2008.07.089
Kruttner, S., Stepien, B., Noordermeer, J. N., Mommaas, M. A., Mechtler, K., Dickson, B. J., et al. (2012). Drosophila CPEB Orb2A mediates memory independent of Its RNA-binding domain. Neuron 76, 383–395. doi: 10.1016/j.neuron.2012.08.028
Kruttner, S., Traunmuller, L., Dag, U., Jandrasits, K., Stepien, B., Iyer, N., et al. (2015). Synaptic Orb2A bridges memory acquisition and late memory consolidation in Drosophila. Cell. Rep. 11, 1953–1965. doi: 10.1016/j.celrep.2015.05.037
Lee, S. S., Ding, Y., Karapetians, N., Rivera-Perez, C., Noriega, F. G., and Adams, M. E. (2017). Hormonal signaling cascade during an early-adult critical period required for courtship memory retention in Drosophila. Curr. Biol. 27, 2798–2809.e3. doi: 10.1016/j.cub.2017.08.017
Liu, P., Peng, H. J., and Zhu, J. (2015). Juvenile hormone-activated phospholipase C pathway enhances transcriptional activation by the methoprene-tolerant protein. Proc. Natl. Acad. Sci. U.S.A. 112, E1871–E1879. doi: 10.1073/pnas.1423204112
Masek, P., Worden, K., Aso, Y., Rubin, G. M., and Keene, A. C. (2015). A dopamine-modulated neural circuit regulating aversive taste memory in Drosophila. Curr. Biol. 25, 1535–1541. doi: 10.1016/j.cub.2015.04.027
McBride, S. M., Giuliani, G., Choi, C., Krause, P., Correale, D., Watson, K., et al. (1999). Mushroom body ablation impairs short-term memory and long-term memory of courtship conditioning in Drosophila melanogaster. Neuron 24, 967–977. doi: 10.1016/s0896-6273(00)81043-0
McGaugh, J. L. (1966). Time-dependent processes in memory storage. Science 153, 1351–1358. doi: 10.1126/science.153.3742.1351
McGaugh, J. L. (2004). The amygdala modulates the consolidation of memories of emotionally arousing experiences. Annu. Rev. Neurosci. 27, 1–28. doi: 10.1146/annurev.neuro.27.070203.144157
McGuire, S. E., Mao, Z., and Davis, R. L. (2004a). Spatiotemporal gene expression targeting with the TARGET and gene-switch systems in Drosophila. Sci. Stke. 2004:l6. doi: 10.1126/stke.2202004pl6
McGuire, S. E., Roman, G., and Davis, R. L. (2004b). Gene expression systems in Drosophila: a synthesis of time and space. Trends Genet. 20, 384–391. doi: 10.1016/j.tig.2004.06.012
Meiselman, M., Lee, S. S., Tran, R. T., Dai, H., Ding, Y., Rivera-Perez, C., et al. (2017). Endocrine network essential for reproductive success in Drosophila melanogaster. Proc. Natl. Acad. Sci. U.S.A. 114, E3849–E3858. doi: 10.1073/pnas.1620760114
Mena, W., Diegelmann, S., Wegener, C., and Ewer, J. (2016). Stereotyped responses of Drosophila peptidergic neuronal ensemble depend on downstream neuromodulators. Elife 5:e19686. doi: 10.7554/eLife.19686
Meneses, A. (2007). Stimulation of 5-HT1A, 5-HT1B, 5-HT2A/2C, 5-HT3 and 5-HT4 receptors or 5-HT uptake inhibition: short- and long-term memory. Behav. Brain Res. 184, 81–90. doi: 10.1016/j.bbr.2007.06.026
Osterwalder, T., Yoon, K. S., White, B. H., and Keshishian, H. (2001). A conditional tissue-specific transgene expression system using inducible GAL4. Proc. Natl. Acad. Sci. U.S.A. 98, 12596–12601. doi: 10.1073/pnas.221303298
Park, Y., Filippov, V., Gill, S. S., and Adams, M. E. (2002). Deletion of the ecdysis-triggering hormone gene leads to lethal ecdysis deficiency. Development 129, 493–503.
Perisse, E., Raymond-Delpech, V., Neant, I., Matsumoto, Y., Leclerc, C., Moreau, M., et al. (2009). Early calcium increase triggers the formation of olfactory long-term memory in honeybees. BMC Biol. 7:30. doi: 10.1186/1741-7007-7-30
Qin, H., Cressy, M., Li, W., Coravos, J. S., Izzi, S. A., and Dubnau, J. (2012). Gamma neurons mediate dopaminergic input during aversive olfactory memory formation in Drosophila. Curr. Biol. 22, 608–614. doi: 10.1016/j.cub.2012.02.014
Sakai, T., Inami, S., Sato, S., and Kitamoto, T. (2012a). Fan-shaped body neurons are involved in period-dependent regulation of long-term courtship memory in Drosophila. Learn. Mem. 19, 571–574. doi: 10.1101/lm.028092.112
Sakai, T., Sato, S., Ishimoto, H., and Kitamoto, T. (2012b). Significance of the centrally expressed TRP channel painless in Drosophila courtship memory. Learn. Mem. 20, 34–40. doi: 10.1101/lm.029041.112
Sanderson, D. J., Good, M. A., Skelton, K., Sprengel, R., Seeburg, P. H., Rawlins, J. N., et al. (2009). Enhanced long-term and impaired short-term spatial memory in GluA1 AMPA receptor subunit knockout mice: evidence for a dual-process memory model. Learn. Mem. 16, 379–386. doi: 10.1101/lm.1339109
Shohat-Ophir, G., Kaun, K. R., Azanchi, R., Mohammed, H., and Heberlein, U. (2012). Sexual deprivation increases ethanol intake in Drosophila. Science 335, 1351–1355. doi: 10.1126/science.1215932
Siegel, R. W., and Hall, J. C. (1979). Conditioned responses in courtship behavior of normal and mutant Drosophila. Proc. Natl. Acad. Sci. U.S.A. 76, 3430–3434. doi: 10.1073/pnas.76.7.3430
Svetec, N., and Ferveur, J. F. (2005). Social experience and pheromonal perception can change male-male interactions in Drosophila melanogaster. J. Exp. Biol. 208(Pt 5), 891–898. doi: 10.1242/jeb.01454
Trannoy, S., Redt-Clouet, C., Dura, J. M., and Preat, T. (2011). Parallel processing of appetitive short- and long-term memories in Drosophila. Curr. Biol. 21, 1647–1653. doi: 10.1016/j.cub.2011.08.032
Weislogel, J. M., Bengtson, C. P., Muller, M. K., Hortzsch, J. N., Bujard, M., Schuster, C. M., et al. (2013). Requirement for nuclear calcium signaling in Drosophila long-term memory. Sci. Signal 6:ra33. doi: 10.1126/scisignal.2003598
Wijesekera, T. P., Saurabh, S., and Dauwalder, B. (2016). Juvenile hormone is required in adult males for Drosophila courtship. PLoS One 11:e0151912. doi: 10.1371/journal.pone.0151912
Yamagata, N., Ichinose, T., Aso, Y., Placais, P. Y., Friedrich, A. B., Sima, R. J., et al. (2015). Distinct dopamine neurons mediate reward signals for short- and long-term memories. Proc. Natl. Acad. Sci. U.S.A. 112, 578–583. doi: 10.1073/pnas.1421930112
Yamamoto, K., Chadarevian, A., and Pellegrini, M. (1988). Juvenile hormone action mediated in male accessory glands of Drosophila by calcium and kinase C. Science 239, 916–919. doi: 10.1126/science.3124270
Zitnan, D., Kingan, T. G., Hermesman, J. L., and Adams, M. E. (1996). Identification of ecdysis-triggering hormone from an epitracheal endocrine system. Science 271, 88–91. doi: 10.1126/science.271.5245.88
Keywords: long-term memory, Drosophila courtship conditioning, ecdysis triggering hormone, juvenile hormone, mushroom body, hormonal convergence
Citation: Lee SS and Adams ME (2021) Regulation of Drosophila Long-Term Courtship Memory by Ecdysis Triggering Hormone. Front. Neurosci. 15:670322. doi: 10.3389/fnins.2021.670322
Received: 21 February 2021; Accepted: 30 March 2021;
Published: 22 April 2021.
Edited by:
Ian Orchard, University of Toronto Mississauga, CanadaReviewed by:
Richard Isaac, University of Leeds, United KingdomCopyright © 2021 Lee and Adams. This is an open-access article distributed under the terms of the Creative Commons Attribution License (CC BY). The use, distribution or reproduction in other forums is permitted, provided the original author(s) and the copyright owner(s) are credited and that the original publication in this journal is cited, in accordance with accepted academic practice. No use, distribution or reproduction is permitted which does not comply with these terms.
*Correspondence: Michael E. Adams, bWljaGFlbC5hZGFtc0B1Y3IuZWR1
†Present address: Sang Soo Lee, Department of Neurology, Johns Hopkins University, Baltimore, MD, United States
Disclaimer: All claims expressed in this article are solely those of the authors and do not necessarily represent those of their affiliated organizations, or those of the publisher, the editors and the reviewers. Any product that may be evaluated in this article or claim that may be made by its manufacturer is not guaranteed or endorsed by the publisher.
Research integrity at Frontiers
Learn more about the work of our research integrity team to safeguard the quality of each article we publish.