- 1Department of Radiology, Beijing Friendship Hospital, Capital Medical University, Beijing, China
- 2Department of Otolaryngology Head and Neck Surgery, Beijing Friendship Hospital, Capital Medical University, Beijing, China
This study aimed to explore brain surface-based morphometry cortical thickness changes in patients with idiopathic tinnitus before and after 24 weeks of sound therapy. In this prospective observational study, we recruited 33 tinnitus patients who had undergone 24 weeks of sound therapy and 26 matched healthy controls. For the two groups of subjects, a 3D-BRAVO pulse sequence was acquired both at baseline and at the 24th week. Structural image data preprocessing was performed using the DPABISurf toolbox. The Tinnitus Handicap Inventory (THI) score was assessed to determine the severity of tinnitus before and after treatment. Two-way mixed-model analysis of variance (ANOVA) and Pearson’s correlation analysis were used in the statistical analysis. Student–Newman–Keuls (SNK) tests were used in the post hoc analysis. Significantly lower cortical thickness was found in the left somatosensory and motor cortex (SMC), left posterior cingulate cortex (PCC), and right orbital and polar frontal cortex (OPFC) of the participants in the tinnitus group at baseline than in the participants in the HC group at baseline and after 24 weeks; in the tinnitus group, significantly higher cortical thickness was found after the 24 weeks sound therapy in comparison to the baseline in the left SMC, bilateral superior parietal cortex (SPC), left inferior parietal cortex (IPC), left PCC, and right OPFC. In the HC group, no statistically significant difference in cortical thickness was found after the 24 weeks treatment in comparison to the baseline in the bilateral SMC, bilateral SPC, left IPC, left PCC, or right OPFC. The changes in cortical thickness before and after sound therapy can provide certain reference values for clinical tinnitus treatment. These brain regions could serve as potential targets for neuroimaging.
Introduction
Tinnitus is characterized by the ability to hear sound in the ears or head without any external stimulation (Wegger et al., 2017; Bauer, 2018). The adult prevalence rate is 10–15% (Henry et al., 2020), and the prevalence rate in elderly individuals is approximately 32.0% (Chang et al., 2019). Tinnitus patients exhibit varying degrees of hearing impairment (Cheng et al., 2020). In addition to hearing impairments, annoying tinnitus is often associated with sleep disorders, depression, anxiety, and other mental illnesses and severely impairs patients’ quality of life (Reynolds et al., 2004; Langguth et al., 2013; Zeman et al., 2014). In turn, these disorders can further exacerbate tinnitus (Bhatt et al., 2017).
One promising avenue to help in the search for therapeutic markers is neuroimaging biomarker research (Liu et al., 2020). Tinnitus was indicated to be a disease characterized by abnormal resting-state functional magnetic resonance imaging (rs-fMRI) in our previous research (Han et al., 2015; Lv et al.,2016a,b, 2018b). We also used various network-based approaches, such as graph-theoretical methods and tract-based spatial statistics (TBSS), to investigate associations with clinical variables (Lv et al., 2018a; Han et al., 2019b; Chen et al., 2020b). The brain regions involved include sound detection regions, such as the insula and hippocampus (van der Loo et al., 2011; Hofmeier et al., 2018), and auditory and non-auditory brain regions (Vanneste and De Ridder, 2012), such as the parahippocampal gyrus (Vanneste et al., 2011), posterior cingulate cortex (PCC) (Vanneste et al., 2010), and anterior cingulate cortex (Dirk et al., 2011). Previous studies have also shown that tinnitus can cause significant changes in brain function and structure, which were closely related to the clinical manifestations of patients (Ryu et al., 2016; Schmidt et al., 2017; Han et al., 2019a).
In recent years, an increasing number of studies have begun to focus on the microstructure of the brain. Our previous studies reported brain structural and white matter (WM) microstructural reorganization in the early stage of tinnitus (Chen et al.,2020a,b). Therefore, it is very important to fully understand the abnormal brain structure associated with tinnitus. Microstructural changes in the brain have also been reported in some tinnitus studies (Tae et al., 2018; Besteher et al., 2019). In these studies, the measurements of the brain structure’s microstructural changes have usually focused on two methods: voxel-based morphometry (VBM) and surface-based morphometry (SBM). Some studies have reported microstructural changes in the brains of tinnitus patients with VBM (Husain et al., 2011), which can quantitatively detect the volume of brain tissue at the voxel level (Ashburner and Friston, 2000). Our previous study also applied VBM and found significantly decreased gray matter (GM) volume in the left thalamus, right thalamus, and cochlear nucleus in tinnitus patients before sound therapy (baseline) compared to the healthy control (HC) group (Wei et al., 2020b). By contrast, SBM focuses on cortical structural characteristics. This important difference between SBM and VBM is how these morphological methods identify different brain regions. In recent years, individual studies have reported that the results of VBM cannot be directly compared with the results of SBM because the latter is a more innovative method in studying the structural characteristics associated with tinnitus (Leaver et al., 2012). The differences in VBM and SBM findings are due to the targeted structures using each technique and the variability in brain morphometry techniques (Adjamian et al., 2014). Unlike VBM analyses that mainly focus on subtle distinctions in volume (Frydman et al., 2016), SBM focuses on cortical structural characteristics such as thickness, volume, surface area, and curvature of the cerebral cortex (Khan et al., 2014). Meyer et al. (2016) revealed that cortical thickness differentially correlated with tinnitus distress and duration. Yoo et al. (2016) found that the cortical thickness changes related to hearing loss overlapped with those related to normal aging. However, to date, changes in cortical thickness have not been examined in the evaluation of the treatment efficacy of tinnitus.
Having a basic understanding of brain function and anatomical changes is necessary to effectively treat tinnitus. Many treatment modalities have been applied to tinnitus patients, such as repetitive transcranial magnetic stimulation (rTMS) (Poeppl et al., 2018), drug therapy (Zenner et al., 2017), tinnitus counseling and cognitive behavioral therapy (CBT) (Langguth et al., 2013), hearing aids (Yakunina et al., 2019), cochlear implants (Olze, 2015), and tinnitus retraining therapy (Lee et al., 2019). A previous study applied music therapy and observed changes in the GM volume of tinnitus patients; after the Heidelberg model of music therapy intervention, GM volume in the medial superior frontal areas, auditory cortex, and precuneus increased in acute tinnitus patients, accompanied by significantly decreased tinnitus-related distress (Krick et al., 2015). In recent years, narrowband noise sound therapy has been one of the most commonly used methods for the treatment of tinnitus (Henry et al., 2002). In our study, narrowband sound was defined as a sound center frequency of 400 Hz or higher: the 1/3 octave band is the narrowest and the 1/2 octave band is the widest. This narrowband noise sound therapy is often used clinically to improve short-term effects, and most current therapies are modified using only one characteristic of the individual and/or their tinnitus (Searchfield et al., 2017). Our previous studies demonstrated functional changes in the brain with this sound therapy (Han et al., 2019a,b). However, there are few related reports on surface-based morphological changes, especially changes in cortical thickness, before and after narrowband noise sound therapy.
In this study, tinnitus patients who underwent 24 weeks of narrowband noise sound therapy were enrolled. SBM was applied to analyze the anatomical changes in the brain in patients before and after sound therapy as well as to acquire data from HCs at baseline and at 24 weeks to explore morphological feature alterations. A previous study reported that the left auditory cortex and subcallosal anterior cingulate show correlation between tinnitus duration and cortical thickness (Meyer et al., 2016). However, very few studies have paid attention to the effect of sound therapy on the thickness of the cortex and on which brain regions are more involved. Therefore, we hypothesize that the brain regions associated with tinnitus in SBM may show cortical thickness increase after sound therapy. We hypothesized that sound therapy can return the brain structure to a relatively normal range. Hence, we attempt to provide deeper insight into the changes in the brain after long-term treatment for tinnitus from a surface-based morphological and neuroanatomical perspective.
Materials and Methods
Standard Protocol Approval, Registration, and Patient Consent
This research involved human participants. All authors have declared that this research was approved by the institutional review board (IRB). This study was approved by the Ethics Committees of our Research Institution (Beijing Friendship Hospital, Capital Medical University, 2016-P2-012). Written informed consent was obtained from all study subjects.
Subjects
All patients and healthy volunteers were recruited in our institution. In this study, 33 patients with idiopathic tinnitus were enrolled. Two patients had right laterality and five had left laterality; the rest had bilateral laterality. The tinnitus sound was described as a persistent, low- or high-pitched sound in one or both ears. A clinically considered high-frequency sound is more than 4 kHz. The inclusion criteria were as follows: (1) age of 18 to 65 years; (2) right handedness; (3) tinnitus duration ranging from 6 to 48 months; (4) no significant hearing loss (hearing thresholds ≥ 25 dB HL at frequencies of 0.250, 0.500, 1, 2, 3, 4, 6, and 8 kHz determined by pure tone audiometry (PTA) examination; and (5) willingness to receive sound therapy for 24 weeks and then return for re-examination after treatment. The exclusion criteria included: (1) other kinds of tinnitus (such as pulsatile tinnitus), Meniere’s disease, sudden deafness, or otosclerosis; (2) neurological signs and/or a history of neurological disease; (3) current chronic medical illness; (4) a history of head trauma; (5) cardiovascular, pulmonary, or systemic disease; (6) claustrophobia experienced during the MRI simulator session; and (7) inability to pitch-match their tinnitus. Twenty-six age-, sex-, education-, and handedness-matched HC subjects were enrolled as controls. None of the HCs had suffered from tinnitus in the past year. Other exclusion criteria were the same as previously described. The characteristics of the subjects are presented in Table 1.
Sound Therapy and Clinical Evaluation
We used the TinniTest® (TTS-1000A, China) tinnitus comprehensive diagnosis and treatment instrument for psychoacoustic testing and the SpeechEasy eMasker® (Micro-DSP Technology Co., Ltd, China) for narrowband noise sound therapy. We advised patients to use it in a quiet environment to achieve the best therapeutic effect. All of the enrolled tinnitus patients were examined for tinnitus loudness matching (L = loudness of tinnitus), pitch matching (Tf = tinnitus frequency), minimum masking level, and residual inhibition to characterize the tinnitus and prepare for treatment. Narrowband noise sound therapy was administered to the participants in the tinnitus group for 24 weeks, three times a day for 20 min each time. For each tinnitus patient, the loudness of sound for treatment was set as L-5 dB. The bandwidth is changed according to the center frequency, and the bandwidth is 1/3 octave (for example, Tf = 3 kHz, low cut = 3 kHz × 2–1/6, high cut = 3 kHz × 21/6).
We also asked the patients to complete the Tinnitus Handicap Inventory (THI) to assess the severity of tinnitus before and after treatment. The primary outcome of this prospective study was the change in THI score after treatment. A reduction of at least 16 points in the THI score was considered effective treatment (Newman et al., 1996). The HC group was not given any kind of sound during the study.
Data Acquisition
For each patient, to evaluate the change in brain activity following treatment, structural MRI data were collected at baseline and at the end of therapy (24th week). The HC group was also scanned at baseline as well as at the 24th week. Images were obtained using a 3.0 T MRI system (Prisma, Siemens, Erlangen, Germany) with a 64-channel phase-array head coil. All imaging studies were performed at the Medical Imaging Research Center of our hospital. Parallel imaging was employed for data acquisition. High-resolution three-dimensional (3D) structural T1-weighted (T1w) images were acquired using a 3D magnetization-prepared rapid gradient echo (MP-RAGE) sequence with the following parameters: repetition time (TR) = 2,530 ms, echo time (TE) = 2.98 ms, inversion time (TI) = 1,100 ms, flip angle (FA) = 7°, number of slices = 192, slice thickness = 1 mm, bandwidth = 240 Hz/Px, field of view (FOV) = 256 mm × 256 mm, and matrix = 256 × 256, resulting in an isotropic voxel size of 1 mm × 1 mm × 1 mm.
Anatomical Data Preprocessing
The results included in this manuscript come from the preprocessing performed by DPABISurf using fMRIPrep 20.0.5 (Esteban et al., 2019), which is based on Nipype 1.4.2 (Gorgolewski et al., 2011). For more details about the pipeline, see the section corresponding to workflows in fMRIPrep’s documentation.
The T1w image was corrected for intensity non-uniformity (INU) with N4BiasFieldCorrection (Tustison et al., 2010), distributed with ANTs 2.2.0 (Avants et al., 2008), and used as T1w-reference throughout the workflow. The T1w-reference was then skull-stripped with a Nipype implementation of the antsBrainExtraction.sh workflow (from ANTs), using OASIS30ANTs as the target template. Brain tissue segmentation of cerebrospinal fluid (CSF), WM, and GM was performed on the brain-extracted T1w using fast (FSL 5.0.9) (Zhang et al., 2001). Brain surfaces were reconstructed using recon-all (Dale et al., 1999), and the previously estimated brain mask was refined to reconcile ANT-derived and FreeSurfer-derived segmentations of the cortical GM with a custom variation in the method of Mindboggle (Klein et al., 2017). Volume-based spatial normalization to one standard space (MNI152NLin2009cAsym) was performed through non-linear registration with ANTs registration (ANTs 2.2.0) using brain-extracted versions of both the T1w-reference and the T1w template. The following template was selected for spatial normalization: ICBM 152 non-linear asymmetrical template version 2009c (Fonov et al., 2009). A Human Connectome Project (HCP) template is a built-in template in DPABISurf software, and it is used to divide the cortex.
Statistical Analysis
Demographic data were compared through two-sample t-tests and paired two-sample t-tests using SPSS 19.0 software (SPSS, Inc., Chicago, IL). P < 0.05 were considered statistically significant. Longitudinal changes in THI scores were also analyzed by using paired two-sample t-tests.
We used DPABISurf1 for preprocessing the neuroimaging statistics. For surface-based cortical thickness data, to determine the group × time interaction effect between the two groups and the two scans, the main effects of group (the tinnitus patient group and the HC group) and time (baseline and 24 weeks follow-up period), two-way mixed model ANOVA and post hoc analyses were performed. SBM analyses were conducted using whole-brain analyses. A P-value of cortical thickness analysis less than 0.05 (P < 0.025 for each hemisphere) was considered statistically significant (Monte Carlo simulation corrected). We looked up tables of P-values based on simulations in which a Z field was synthesized on the atlas surface. The tables are distributed in DPABISurf. The P-value for the cluster is determined by indexing into the table based on the size of the cluster, the threshold used to form the cluster, and an estimate of the global full width/half maximum (FWHM). Clusters are extracted separately for both hemispheres. In post hoc analyses, Student–Newman–Keuls (SNK) tests were used for pairwise comparisons. Pearson’s correlation analyses were further conducted to investigate the relationships between the change in cortical thickness and 24 weeks of sound therapy of tinnitus patients (ΔTHI score = THIpre - THIpost). P < 0.05 was set as the threshold to determine significance. The cortical thickness results were visualized with DPABISurf (see Footnote 1). Cortical thickness was quantified from the T1w images using DPABISurf V1.4 software. Pearson’s correlation analysis between the THI scores was performed using SPSS 19 software (SPSS, Inc., Chicago, IL).
Results
Demographics and Behavioral Outcomes of Study Participants
In this study, we enrolled 33 patients with idiopathic tinnitus, and we applied DPABISurf to analyze the cortical thickness in the brain in this group before and after sound therapy. Concurrently, 26 HC individuals were enrolled. Each group of subjects was age-, sex-, and handedness-matched (Table 1). THI scores were acquired before and after sound therapy. Significant longitudinal decreases in THI scores were observed. The results are summarized in Table 1.
Statistical Analysis Results
Group Differences in Cortical Thickness
As shown in Figure 1 and Table 2, in the comparison among four data sets, significant group × time interaction effects between the two groups (tinnitus patients and HCs) and two scans (at baseline and at the 24th week) on cortical thickness were observed at the bilateral somatosensory and motor cortex (SMC), bilateral dorsal stream visual cortex (DSVC), bilateral paracentral lobular and midcingulate cortex (PCL and MCC), bilateral superior parietal cortex (SPC), left inferior parietal cortex (IPC), left PCC, left primary visual cortex (PVC), and right orbital and polar frontal cortex (OPFC) [P < 0.05 (P < 0.025 for each hemisphere) corrected by Monte Carlo simulation (L, left; R, right)].
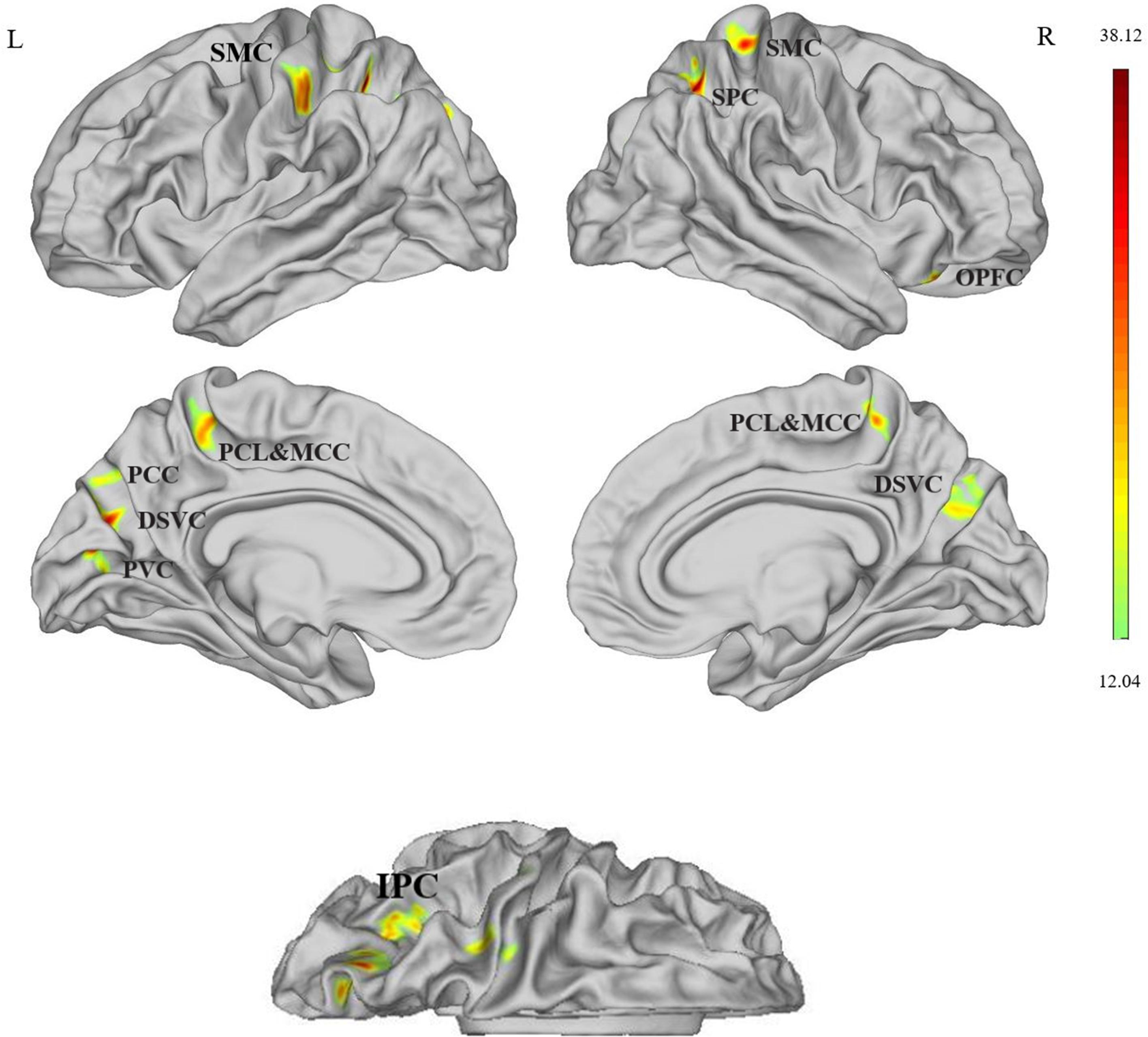
Figure 1. Cortical thickness differences assessed by ANOVA, compared among the four data sets, significant group × time interaction effects between the two groups (tinnitus patients and healthy controls) and two scans (at baseline and at the 24th week) on cortical thickness were observed at the left SMC, bilateral DSVC, bilateral PCL and MCC, bilateral SPC, left IPC, left PCC, left PVC, left PCC, and right OPFC [P < 0.05 (P < 0.025 for each hemisphere) corrected by Monte Carlo simulation (L, left; R, right)]. Abbreviations: SMC, somatosensory and motor cortex; SPC, superior parietal cortex; PCL and MCC, paracentral lobular and midcingulate cortex; DSVC, dorsal stream visual cortex; PVC, primary visual cortex; PCC, posterior cingulate cortex; OPFC, orbital and polar frontal cortex; IPC, inferior parietal cortex.
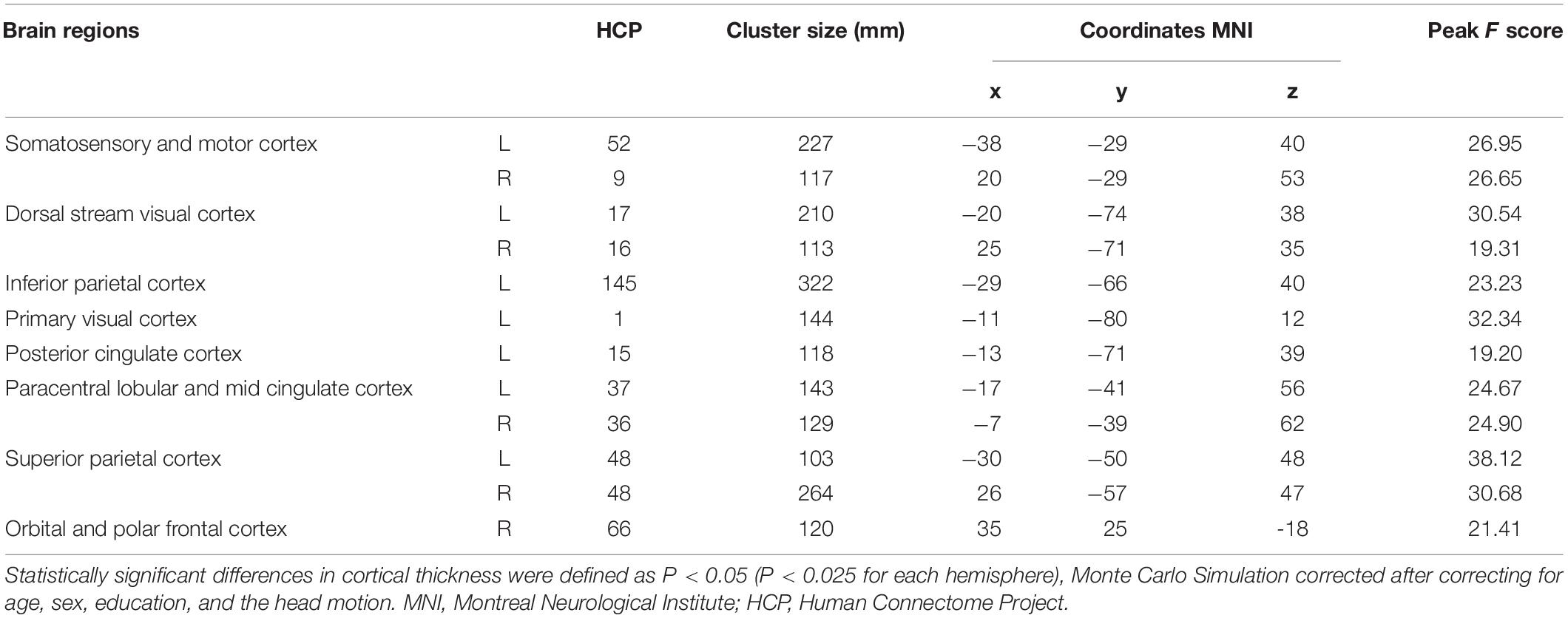
Table 2. Difference in cortical thickness of the left and right hemispheres between the two groups (tinnitus patients and healthy controls) and two scans (at baseline and at the 24th week).
Post hoc Analyses
Significantly lower cortical thickness was found in the left SMC, left PCC, and right OPFC in the tinnitus group at baseline than in the HC group at baseline and after 24 weeks (Figure 2).
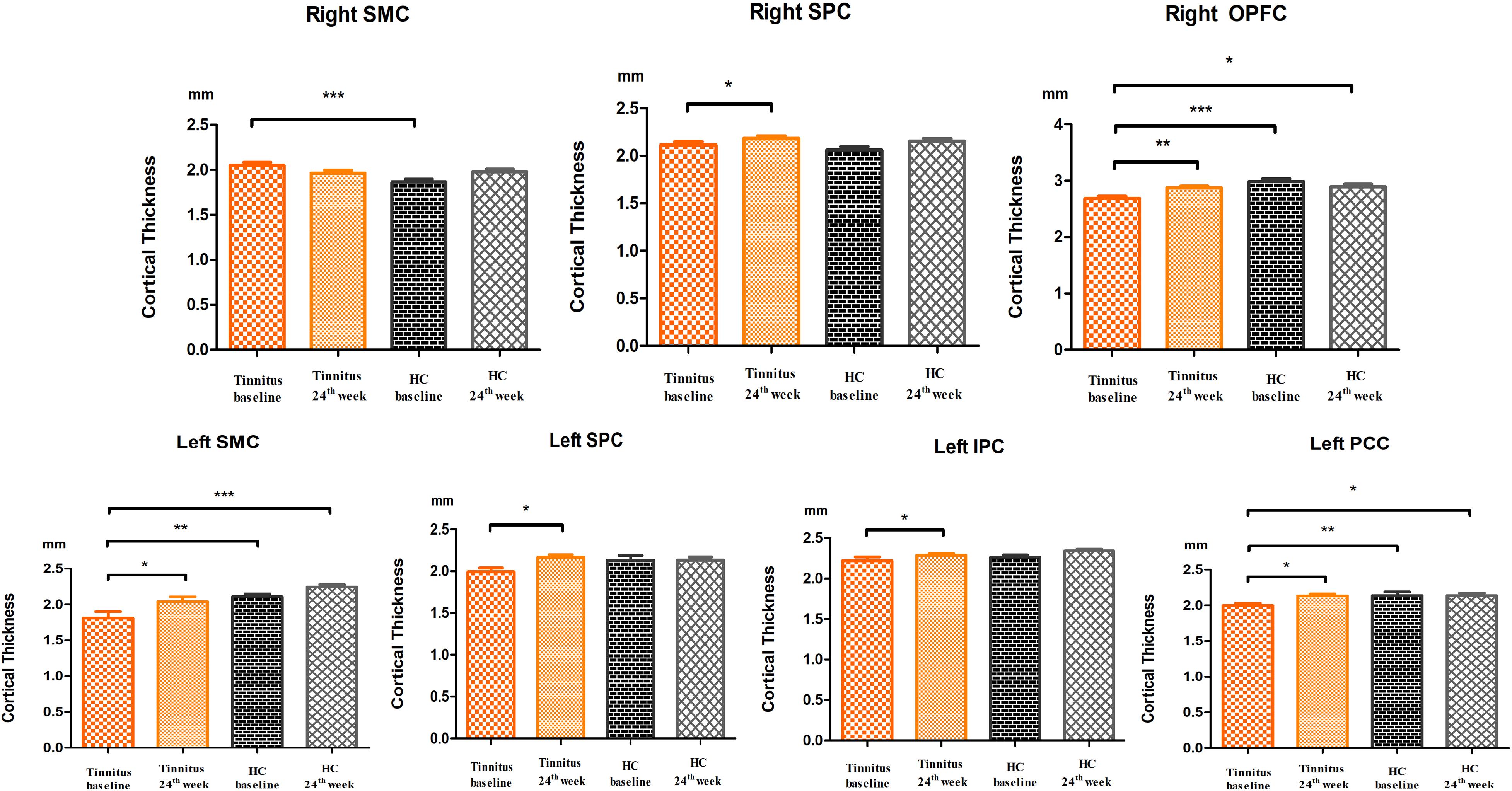
Figure 2. Post hoc analyses showed differences in cortical thickness among the baseline and 24-week sound treatment and the HC baseline and HC 24 weeks groups in both hemispheres. *P < 0.05, **P < 0.01, ***P < 0.001. SMC, somatosensory and motor cortex; SPC, superior parietal cortex; PVC, primary visual cortex; PCC, posterior cingulate cortex; IPC, inferior parietal cortex; OPFC, orbital and polar frontal cortex.
In the tinnitus group, significantly higher cortical thickness was found after the 24 weeks sound therapy in comparison to the baseline in the left SMC, bilateral SPC, left IPC, left PCC, and right OPFC. In this comparison, the difference in the right SMC did not reach statistical significance (Figure 2).
In the HC group, no statistically significant difference in cortical thickness was found after the 24 weeks sound therapy in comparison to the baseline in the bilateral SMC, bilateral SPC, left IPC, left PCC, or right OPFC (Figure 2).
Compared with the HC baseline group and the HC 24 weeks group, the 24 weeks sound therapy tinnitus group showed slightly lower or similar cortical thickness in the bilateral SMC, bilateral SPC, right OPFC, left IPC, and left PCC, and these differences did not reach statistical significance (Figure 2).
In post hoc analysis, there were no significant differences between the tinnitus baseline group and the 24 weeks sound therapy tinnitus group in the bilateral PCL and MCC, bilateral DSVC, or left PVC.
Correlation
Decreased THI scores and cortical thickness changes in the above brain regions were not significantly correlated.
Discussion
This is a meaningful SBM investigation that analyzed changes in cortical thickness in tinnitus patients from baseline to after 24 weeks of sound therapy. Cortical thickness changes in the brain were found in patients after longer-term sound therapy, mainly in the left SMC, bilateral SPC, left IPC, left PCC, and right OPFC. These brain regions are mainly concentrated in the visual cortex, prefrontal cortex, somatosensory cortex, motor cortex, and default-mode network (DMN), which are closely related to the abnormal brain morphology associated with tinnitus. Our data confirmed our hypothesis that sound therapy can gradually return the brain structure to a relatively normal range, especially cortical thickness.
In our study, we observed a significant increase in cortical thickness in the left SMC, bilateral SPC, left IPC, left PCC, and right OPFC in the patients after treatment compared with baseline. Among the above brain regions, the left PCC, right medial prefrontal cortex (mPFC), and left IPC are parts of the DMN. DMN is a brain system involved in internal cognitive patterns, and cognitive dysfunction may be linked with neuropathological changes in tinnitus (Vanneste et al., 2016; Wang et al., 2018). The PCC, as the central hub, is a metabolically active and highly connected region in the brain (Fransson and Marrelec, 2008) and plays a key role in stimulus-independent processing (Wei et al., 2020a). Chen et al. (2018a) reported that increased functional connectivity (FC) patterns of the precuneus and inferior parietal lobule (IPL) might be responsible for disrupting the DMN in tinnitus patients. Chen et al. (2018b) also showed that decreased FC within the DMN was associated with poorer cognitive performance in chronic tinnitus patients. This shows that tinnitus has a very close relationship with DMN.
The orbitofrontal cortex and PFC constitute the 20th section of the HCP template—the OPFC (Glasser et al., 2016). The orbitofrontal cortex is a large but heterogeneous cortical area on the ventral surface of the frontal lobe and has been closely related to emotion and executive function (Rudebeck and Rich, 2018). The PFC is involved in the executive control of attention and tinnitus perception (Aldhafeeri et al., 2012; Schmidt et al., 2013). Cortical thickness reductions and disrupted connectivity of the PFC might also be associated with the negative emotions experienced by tinnitus sufferers (Aldhafeeri et al., 2012). The IPC is also involved in the combination of auditory, visual, somatosensory, and attention processes. The results of our study indicated that lower DMN cortical thickness correlated with performance in the above domains in tinnitus patients, and our results are more focused on explaining morphological changes. In addition to DMN, the superior parietal lobule (SPL) is also involved in the cognitive process, and early along the auditory pathway, different cognitive resources are required for the filtering out of tinnitus (Vanneste et al., 2018). After sound therapy, the cortical thickness in these brain regions was slightly increased, and whether their corresponding functions are restored still needs further research.
The precentral gyrus (PreCG) is a typical representative of the somatosensory and primary motor cortex from the HCP brain template, and it plays a role in auditory-associated functions (Zhang et al., 2015; Glasser et al., 2016). Some studies have reported that the PreCG might associate with word recognition and phonological processing (Xu et al., 2019). Another study found significant functional changes between the PreCG and some other regions in tinnitus patients and inferred that the changes may suggest that tinnitus was caused or modulated by signals from the somatosensory, somatomotor, and visuomotor systems in some patients (Feng et al., 2018). Our results also indicated that sound therapy can affect cortical thickness at the morphological level. Although the change in the PreCG may not be as significant as functional changes, the results also reflect the efficacy of sound therapy in altering SBM properties to a certain extent.
In our post hoc analysis, there were no significant differences in the bilateral PCL and MCC, bilateral DSVC, or right PVC, but there were group differences. The PCL and MCC are included in the HCP template, and the former is adjacent to the somatosensory area (SMA), which was proposed to be involved in sensorimotor integration (Edwards et al., 2019; Umeda et al., 2019). The DSVC and PVC are visual cortexes. Some studies have reported that auditory spatial information is processed by the visual centers of the brain in addition to auditory brain regions (Bedny et al., 2010; Fiehler and Rosler, 2010). A recent study reported increased activity in the right middle occipital gyrus in tinnitus patients; the middle occipital gyrus is associated with visual processing, which may process cross-modal information in phantom auditory perception (Cheng et al., 2020). These brain regions are also irreplaceable neuroimaging targets in assessing tinnitus treatment efficacy.
Although different studies have used different morphological analysis methods, the correct treatment method and the duration of treatment are the most critical factors for achieving therapeutic effects. Schmidt et al. (2018) used SBM to investigate GM changes and diffusion tensor imaging to assess changes in orientation of WM tracts and found that whole-brain analyses revealed decreased cortical thickness in the left parahippocampal gyrus in those with more severe tinnitus compared to a group with a milder reaction. However, the individuals in that study were not being treated. A previous study with the Heidelberg model of music therapy analyzed the average treatment time to be approximately 2 weeks (Krick et al., 2015) and found GM volume changes in the precuneus, medial superior frontal areas, and auditory cortex. In our study, we applied narrowband noise sound therapy over a relatively long (6 months) treatment time. The morphological changes that may be found with this type of treatment and duration of treatment are different from those of previous studies, which is supported by our results. Our previous study showed that FC of the right insula, IPL, bilateral thalami, and left middle temporal gyrus was significantly modified with sound and predicted the clinical outcome of adjusted narrowband noise sound therapy (Han et al., 2019a). As in our former study (Lv et al., 2020) proved, from a functional perspective, the function of the brain regions mentioned above were basically improved after sound therapy. The results of this study are morphologically proven after a longer-term treatment, and the cortical thickness in these brain regions was slightly increased.
Limitations
First, there was no significant relationship between ΔTHI scores and cortical thickness changes in tinnitus patients, which may be due to the small sample size. In future studies, we need to further expand the sample size to verify the correlation between them. Second, due to the huge amount of high-precision cortical reconstruction calculations, the preprocessing time of SBM is longer; therefore, no studies have reported the treatment effect of tinnitus from the perspective of SBM. In future research, we need to use a more computationally intensive computer to analyze the morphology of tinnitus from all dimensions of SBM, such as cortical volume, curvature, cortical areas, and sulcal depth. Lastly, the current findings should not be overgeneralized to the entire tinnitus population as sound therapy is not the only possible treatment for tinnitus patients, and measurable tinnitus pitch was included in this study, which did not account for patients who reported other tinnitus sounds or characteristics.
Conclusion
This study analyzed anatomical changes in tinnitus patients before and after treatment for 6 months. Sound therapy can change cortical thickness in some brain regions and systems that are important for SMC, superior parietal cortex, inferior parietal cortex, PCC, and OPFC. Surface-based morphometry to assess cortical thickness can potentially provide neuroimaging research value to the study of tinnitus-related neuroanatomical changes before and after sound therapy in tinnitus patients. The findings of this study may better support the reduction in tinnitus severity or increase in cortical thickness.
Data Availability Statement
The original contributions presented in the study are included in the article/supplementary material, further inquiries can be directed to the corresponding author/s.
Ethics Statement
The studies involving human participants were reviewed and approved by the Ethics Committees of our Research Institution (Beijing Friendship Hospital, Capital Medical University, 2016-P2-012). The patients/participants provided their written informed consent to participate in this study. Written informed consent was obtained from the individual(s) for the publication of any potentially identifiable images or data included in this article.
Author Contributions
XW designed the experiments, performed the statistical analysis, and wrote the manuscript. QC conducted the statistical analysis. PZ contributed to the manuscript revision. HL, ZW, and CL collected the data. SG, ZY, and ZW were guarantors of this work. HL and ZW were the corresponding authors of this manuscript, full access to all the data in the study, take responsibility for the integrity of the data, and the accuracy of the data analysis. All authors contributed to the article and approved the submitted version.
Funding
This work was supported by Grant Nos. 61801311 and 61931013 from the National Natural Science Foundation of China, No. (2015) 160 from Beijing Scholars Program; Grant No. 7182044 from Beijing Natural Science Foundation; No. PX2018001 from Beijing Hospitals Authority; QML20180103 from Beijing Hospitals Authority Youth Programme; Nos. 2019M660717, 2020T130083ZX, and 2020M680607 from China Postdoctoral Science Foundation; No. 2020-Z2-023 from Beijing Postdoctoral Research Foundation; and No. yyqdkt2019-31 from the Research Foundation of Beijing Friendship Hospital, Capital Medical University.
Conflict of Interest
The authors declare that the research was conducted in the absence of any commercial or financial relationships that could be construed as a potential conflict of interest.
Acknowledgments
We especially thank the Beijing Friendship Hospital, Capital Medical University, for their support in our research. We thank doctors from the Department of Otolaryngology Head and Neck Surgery, Beijing Friendship Hospital, Capital Medical University, for their assistance with the data collection and audiological tests.
Footnotes
References
Adjamian, P., Hall, D. A., Palmer, A. R., Allan, T. W., and Langers, D. R. (2014). Neuroanatomical abnormalities in chronic tinnitus in the human brain. Neurosci. Biobehav. Rev. 45, 119–133. doi: 10.1016/j.neubiorev.2014.05.013
Aldhafeeri, F. M., Mackenzie, I., Kay, T., Alghamdi, J., and Sluming, V. (2012). Neuroanatomical correlates of tinnitus revealed by cortical thickness analysis and diffusion tensor imaging. Neuroradiology 54, 883–892. doi: 10.1007/s00234-012-1044-6
Ashburner, J., and Friston, K. J. (2000). Voxel-based morphometry—the methods. Neuroimage 11, 805–821.
Avants, B. B., Epstein, C. L., Grossman, M., and Gee, J. C. (2008). Symmetric diffeomorphic image registration with cross-correlation: evaluating automated labeling of elderly and neurodegenerative brain. Med. Image Anal. 12, 26–41. doi: 10.1016/j.media.2007.06.004
Bedny, M., Konkle, T., Pelphrey, K., Saxe, R., and Pascual-Leone, A. (2010). Sensitive period for a multimodal response in human visual motion area MT/MST. Curr. Biol. 20, 1900–1906. doi: 10.1016/j.cub.2010.09.044
Besteher, B., Gaser, C., Ivansic, D., Guntinas-Lichius, O., Dobel, C., and Nenadic, I. (2019). Chronic tinnitus and the limbic system: reappraising brain structural effects of distress and affective symptoms. Neuroimage Clin. 24:101976. doi: 10.1016/j.nicl.2019.101976
Bhatt, J. M., Bhattacharyya, N., and Lin, H. W. (2017). Relationships between tinnitus and the prevalence of anxiety and depression. Laryngoscope 127, 466–469. doi: 10.1002/lary.26107
Chang, N. C., Dai, C. Y., Lin, W. Y., Yang, H. L., Wang, H. M., Chien, C. Y., et al. (2019). Prevalence of persistent tinnitus and dizziness in an elderly population in southern taiwan. J. Int. Adv. Otol. 15, 99–105. doi: 10.5152/iao.2019.6257
Chen, Q., Lv, H., Wang, Z., Wei, X., Zhao, P., Yang, Z., et al. (2020a). Outcomes at 6 months are related to brain structural and white matter microstructural reorganization in idiopathic tinnitus patients treated with sound therapy. Hum. Brain Mapp. 42, 753–765. doi: 10.1002/hbm.25260
Chen, Q., Wang, Z., Lv, H., Zhao, P., Yang, Z., Gong, S., et al. (2020b). Reorganization of brain white matter in persistent idiopathic tinnitus patients without hearing loss: evidence from baseline data. Front. Neurosci. 14:591. doi: 10.3389/fnins.2020.00591
Chen, Y. C., Liu, S., Lv, H., Bo, F., Feng, Y., Chen, H., et al. (2018a). Abnormal resting-state functional connectivity of the anterior cingulate cortex in unilateral chronic tinnitus patients. Front. Neurosci. 12:9. doi: 10.3389/fnins.2018.00009
Chen, Y. C., Zhang, H., Kong, Y., Lv, H., Cai, Y., Chen, H., et al. (2018b). Alterations of the default mode network and cognitive impairment in patients with unilateral chronic tinnitus. Quant. Imaging Med. Surg. 8, 1020–1029. doi: 10.21037/qims.2018.11.04
Cheng, S., Xu, G., Zhou, J., Qu, Y., Li, Z., He, Z., et al. (2020). A multimodal meta-analysis of structural and functional changes in the brain of tinnitus. Front. Hum. Neurosci. 14:28. doi: 10.3389/fnhum.2020.00028
Dale, A. M., Fischl, B., and Sereno, M. I. (1999). Cortical surface-based analysis. I. Segmentation and surface reconstruction. Neuroimage 9, 179–194. doi: 10.1006/nimg.1998.0395
Dirk, D. R., Sven, V., Marco, C., and Thomas, K. (2011). The distressed brain: a group blind source separation analysis on tinnitus. Plos One 6:e24273.
Edwards, L. L., King, E. M., Buetefisch, C. M., and Borich, M. R. (2019). Putting the “Sensory” into sensorimotor control: the role of sensorimotor integration in goal-directed hand movements after stroke. Front. Integr. Neurosci. 13:16. doi: 10.3389/fnint.2019.00016
Esteban, O., Markiewicz, C. J., Blair, R. W., Moodie, C. A., Isik, A. I., Erramuzpe, A., et al. (2019). fMRIPrep: a robust preprocessing pipeline for functional MRI. Nat. Methods 16, 111–116. doi: 10.1038/s41592-018-0235-4
Feng, Y., Chen, Y. C., Lv, H., Xia, W., Mao, C. N., Bo, F., et al. (2018). Increased resting-state cerebellar-cerebral functional connectivity underlying chronic tinnitus. Front. Aging Neurosci. 10:59. doi: 10.3389/fnagi.2018.00059
Fiehler, K., and Rosler, F. (2010). Plasticity of multisensory dorsal stream functions: evidence from congenitally blind and sighted adults. Restor. Neurol. Neurosci. 28, 193–205. doi: 10.3233/RNN-2010-0500
Fonov, V. S., Evans, A. C., McKinstry, R. C., Almli, C. R., and Collins, D. L. (2009). Unbiased nonlinear average age-appropriate brain templates from birth to adulthood. NeuroImage 47:S102.
Fransson, P., and Marrelec, G. (2008). The precuneus/posterior cingulate cortex plays a pivotal role in the default mode network: evidence from a partial correlation network analysis. Neuroimage 42, 1178–1184. doi: 10.1016/j.neuroimage.2008.05.059
Frydman, I., de Salles Andrade, J. B., Vigne, P., and Fontenelle, L. F. (2016). Can neuroimaging provide reliable biomarkers for obsessive-compulsive disorder? a narrative review. Curr. Psychiatry Rep. 18:90. doi: 10.1007/s11920-016-0729-7
Glasser, M. F., Coalson, T. S., Robinson, E. C., Hacker, C. D., Harwell, J., Yacoub, E., et al. (2016). A multi-modal parcellation of human cerebral cortex. Nature 536, 171–178. doi: 10.1038/nature18933
Gorgolewski, K., Burns, C. D., Madison, C., Clark, D., Halchenko, Y. O., Waskom, M. L., et al. (2011). Nipype: a flexible, lightweight and extensible neuroimaging data processing framework in python. Front. Neuroinform. 5:13. doi: 10.3389/fninf.2011.00013
Han, L., Na, Z., Chunli, L., Yuchen, C., Pengfei, Z., Hao, W., et al. (2019a). Baseline functional connectivity features of neural network nodes can predict improvement after sound therapy through adjusted narrow band noise in tinnitus patients. Front. Neurosci. 13:614. doi: 10.3389/fnins.2019.00614
Han, L., Yawen, L., Hao, W., Chunli, L., Pengfei, Z., Zhengyu, Z., et al. (2019b). Effects of sound therapy on resting-state functional brain networks in patients with tinnitus: a graph-theoretical-based study. J. Magn. Reson. Imaging 50, 1731–1741. doi: 10.1002/jmri.26796
Han, L., Zhaohui, L., Fei, Y., Pengfei, Z., Ting, L., Cheng, D., et al. (2015). Disrupted neural activity in unilateral vascular pulsatile tinnitus patients in the early stage of disease: evidence from resting-state fMRI. Prog. Neuropsychopharmacol. Biol. Psychiatry 59, 91–99. doi: 10.1016/j.pnpbp.2015.01.013
Henry, J. A., Reavis, K. M., Griest, S. E., Thielman, E. J., Theodoroff, S. M., Grush, L. D., et al. (2020). Tinnitus: an epidemiologic perspective. Otolaryngol. Clin. North Am. 53, 481–499. doi: 10.1016/j.otc.2020.03.002
Henry, J. A., Schechter, M. A., Nagler, S. M., and Fausti, S. A. (2002). Comparison of tinnitus masking and tinnitus retraining therapy. J. Am. Acad. Audiol. 13, 559–581.
Hofmeier, B., Wolpert, S., Aldamer, E. S., Walter, M., Thiericke, J., Braun, C., et al. (2018). Reduced sound-evoked and resting-state BOLD fMRI connectivity in tinnitus. NeuroImage Clin. 20, 637–649. doi: 10.1016/j.nicl.2018.08.029
Husain, F. T., Medina, R. E., Davis, C. W., Szymko-Bennett, Y., Simonyan, K., Pajor, N. M., et al. (2011). Neuroanatomical changes due to hearing loss and chronic tinnitus: a combined VBM and DTI study. Brain Res. 1369, 74–88. doi: 10.1016/j.brainres.2010.10.095
Khan, U. A., Liu, L., Provenzano, F. A., Berman, D. E., Profaci, C. P., Sloan, R., et al. (2014). Molecular drivers and cortical spread of lateral entorhinal cortex dysfunction in preclinical Alzheimer’s disease. Nat. Neurosci. 17, 304–311. doi: 10.1038/nn.3606
Klein, A., Ghosh, S. S., Bao, F. S., Giard, J., Hame, Y., Stavsky, E., et al. (2017). Mindboggling morphometry of human brains. PLoS Comput. Biol. 13:e1005350. doi: 10.1371/journal.pcbi.1005350
Krick, C. M., Grapp, M., Daneshvar-Talebi, J., Reith, W., Plinkert, P. K., and Bolay, H. V. (2015). Cortical reorganization in recent-onset tinnitus patients by the heidelberg model of music therapy. Front. Neurosci. 9:49. doi: 10.3389/fnins.2015.00049
Langguth, B., Kreuzer, P. M., Kleinjung, T., and De Ridder, D. (2013). Tinnitus: causes and clinical management. Lancet Neurol. 12, 920–930. doi: 10.1016/S1474-4422(13)70160-1
Leaver, A. M., Seydell-Greenwald, A., Turesky, T. K., Morgan, S., Kim, H. J., and Rauschecker, J. P. (2012). Cortico-limbic morphology separates tinnitus from tinnitus distress. Front. Syst. Neurosci. 6:21. doi: 10.3389/fnsys.2012.00021
Lee, S. Y., Rhee, J., Shim, Y. J., Kim, Y., Koo, J. W., De Ridder, D., et al. (2019). Changes in the resting-state cortical oscillatory activity 6 months after modified tinnitus retraining therapy. Front. Neurosci. 13:1123. doi: 10.3389/fnins.2019.01123
Liu, X., Hou, Z., Yin, Y., Xie, C., Zhang, H., Zhang, H., et al. (2020). Decreased cortical thickness of left premotor cortex as a treatment predictor in major depressive disorder. Brain Imaging Behav. doi: 10.1007/s11682-020-00341-3
CrossRef Full Text. [Epub ahead of print]. | PubMed Abstract | Google Scholar
Lv, H., Liu, C., Wang, Z., Zhao, P., Cheng, X., Yang, Z., et al. (2020). Altered functional connectivity of the thalamus in tinnitus patients is correlated with symptom alleviation after sound therapy. Brain Imaging Behav. 14, 2668–2678. doi: 10.1007/s11682-019-00218-0
Lv, H., Wang, Z., Tong, E., Williams, L. M., Zaharchuk, G., Zeineh, M., et al. (2018a). Resting-state functional mri: everything that nonexperts have always wanted to know. AJNR Am. J. Neuroradiol. 39, 1390–1399. doi: 10.3174/ajnr.A5527
Lv, H., Zhao, P., Liu, Z., Liu, X., Ding, H., Liu, L., et al. (2018b). Lateralization effects on functional connectivity of the auditory network in patients with unilateral pulsatile tinnitus as detected by functional MRI. Prog. Neuropsychopharmacol. Biol. Psychiatry 81, 228–235. doi: 10.1016/j.pnpbp.2017.09.020
Lv, H., Zhao, P., Liu, Z., Li, R., Zhang, L., Wang, P., et al. (2016a). Abnormal resting-state functional connectivity study in unilateral pulsatile tinnitus patients with single etiology: a seed-based functional connectivity study. Eur. J. Radiol. 85, 2023–2029. doi: 10.1016/j.ejrad.2016.09.011
Lv, H., Zhao, P., Liu, Z., Wang, G., Zeng, R., Yan, F., et al. (2016b). Frequency-dependent neural activity in patients with unilateral vascular pulsatile tinnitus. Neural. Plast. 2016:4918186. doi: 10.1155/2016/4918186
Meyer, M., Neff, P., Liem, F., Kleinjung, T., Weidt, S., Langguth, B., et al. (2016). Differential tinnitus-related neuroplastic alterations of cortical thickness and surface area. Hear. Res. 342, 1–12. doi: 10.1016/j.heares.2016.08.016
Newman, C. W., Jacobson, G. P., and Spitzer, J. B. (1996). Development of the tinnitus handicap inventory. Arch. Otolaryngol. Head Neck Surg. 122, 143–148. doi: 10.1001/archotol.1996.01890140029007
Poeppl, T. B., Langguth, B., Lehner, A., Frodl, T., Rupprecht, R., Kreuzer, P. M., et al. (2018). Brain stimulation-induced neuroplasticity underlying therapeutic response in phantom sounds. Hum. Brain Mapp. 39, 554–562. doi: 10.1002/hbm.23864
Reynolds, P., Gardner, D., and Lee, R. (2004). Tinnitus and psychological morbidity: a cross-sectional study to investigate psychological morbidity in tinnitus patients and its relationship with severity of symptoms and illness perceptions. Clin. Otolaryngol. Allied Sci. 29, 628–634. doi: 10.1111/j.1365-2273.2004.00879.x
Rudebeck, P. H., and Rich, E. L. (2018). Orbitofrontal cortex. Curr. Biol. 28, R1083–R1088. doi: 10.1016/j.cub.2018.07.018
Ryu, C. W., Park, M. S., Byun, J. Y., Jahng, G. H., and Park, S. (2016). White matter integrity associated with clinical symptoms in tinnitus patients: a tract-based spatial statistics study. Eur. Radiol. 26, 2223–2232. doi: 10.1007/s00330-015-4034-3
Schmidt, S. A., Akrofi, K., Carpenter-Thompson, J. R., and Husain, F. T. (2013). Default mode, dorsal attention and auditory resting state networks exhibit differential functional connectivity in tinnitus and hearing loss. PLoS One 8:e76488. doi: 10.1371/journal.pone.0076488
Schmidt, S. A., Carpenter-Thompson, J., and Husain, F. T. (2017). Connectivity of precuneus to the default mode and dorsal attention networks: a possible invariant marker of long-term tinnitus. NeuroImage Clin. 16, 196–204. doi: 10.1016/j.nicl.2017.07.015
Schmidt, S. A., Zimmerman, B., Bido Medina, R. O., Carpenter-Thompson, J. R., and Husain, F. T. (2018). Changes in gray and white matter in subgroups within the tinnitus population. Brain Res. 1679, 64–74. doi: 10.1016/j.brainres.2017.11.012
Searchfield, G. D., Durai, M., and Linford, T. (2017). A state-of-the-art review: personalization of tinnitus sound therapy. Front. Psychol. 8:1599. doi: 10.3389/fpsyg.2017.01599
Tae, W. S., Yakunina, N., Lee, W. H., Ryu, Y. J., Ham, H. K., Pyun, S. B., et al. (2018). Changes in the regional shape and volume of subcortical nuclei in patients with tinnitus comorbid with mild hearing loss. Neuroradiology 60, 1203–1211. doi: 10.1007/s00234-018-2093-2
Tustison, N. J., Avants, B. B., Cook, P. A., Zheng, Y., Egan, A., Yushkevich, P. A., et al. (2010). N4ITK: improved N3 bias correction. IEEE Trans. Med. Imaging 29, 1310–1320. doi: 10.1109/TMI.2010.2046908
Umeda, T., Isa, T., and Nishimura, Y. (2019). The somatosensory cortex receives information about motor output. Sci. Adv. 5:eaaw5388. doi: 10.1126/sciadv.aaw5388
van der Loo, E., Congedo, M., Vanneste, S., Van De Heyning, P., and De Ridder, D. (2011). Insular lateralization in tinnitus distress. Auton Neurosci. 165, 191–194. doi: 10.1016/j.autneu.2011.06.007
Vanneste, S., and De Ridder, D. (2012). The auditory and non-auditory brain areas involved in tinnitus. An emergent property of multiple parallel overlapping subnetworks. Front. Syst. Neurosci. 6:31. doi: 10.3389/fnsys.2012.00031
Vanneste, S., Faber, M., Langguth, B., and De Ridder, D. (2016). The neural correlates of cognitive dysfunction in phantom sounds. Brain Res. 1642, 170–179. doi: 10.1016/j.brainres.2016.03.016
Vanneste, S., Joos, K., Ost, J., and De Ridder, D. (2018). Influencing connectivity and cross-frequency coupling by real-time source localized neurofeedback of the posterior cingulate cortex reduces tinnitus related distress. Neurobiol. Stress 8, 211–224. doi: 10.1016/j.ynstr.2016.11.003
Vanneste, S., Plazier, M., der Loo, E., de Heyning, P. V., Congedo, M., and De Ridder, D. (2010). The neural correlates of tinnitus-related distress. Neuroimage 52, 470–480. doi: 10.1016/j.neuroimage.2010.04.029
Vanneste, S. S., Heyning, P. V. D., and Ridder, D. D. (2011). Contralateral parahippocampal gamma-band activity determines noise-like tinnitus laterality: a region of interest analysis. Neuroscience 199, 481–490.
Wang, Y., Zhang, J. N., Hu, W., Li, J. J., Zhou, J. X., Zhang, J. P., et al. (2018). The characteristics of cognitive impairment in subjective chronic tinnitus. Brain Behav. 8:e00918. doi: 10.1002/brb3.918
Wegger, M., Ovesen, T., and Larsen, D. G. (2017). Acoustic coordinated reset neuromodulation: a systematic review of a novel therapy for tinnitus. Front. Neurol. 8:36. doi: 10.3389/fneur.2017.00036
Wei, X., Li, W., Chen, J., Li, Y., Zhu, J., Shi, H., et al. (2020a). Assessing drug cue-induced brain response in heroin dependents treated by methadone maintenance and protracted abstinence measures. Brain Imaging Behav. 14, 1221–1229. doi: 10.1007/s11682-019-00051-5
Wei, X., Lv, H., Wang, Z., Liu, C., Ren, P., Zhang, P., et al. (2020b). Neuroanatomical alterations in patients with tinnitus before and after sound therapy: a voxel-based morphometry study. Front. Neurosci. 14:911. doi: 10.3389/fnins.2020.00911
Xu, X. M., Jiao, Y., Tang, T. Y., Lu, C. Q., Zhang, J., Salvi, R., et al. (2019). Altered spatial and temporal brain connectivity in the salience network of sensorineural hearing loss and tinnitus. Front. Neurosci. 13:246. doi: 10.3389/fnins.2019.00246
Yakunina, N., Lee, W. H., Ryu, Y. J., and Nam, E. C. (2019). Tinnitus suppression effect of hearing aids in patients with high-frequency hearing loss: a randomized double-blind controlled trial. Otol. Neurotol. 40, 865–871. doi: 10.1097/MAO.0000000000002315
Yoo, H. B., De Ridder, D., and Vanneste, S. (2016). The importance of aging in gray matter changes within tinnitus patients shown in cortical thickness, surface area and volume. Brain Topogr. 29, 885–896. doi: 10.1007/s10548-016-0511-5
Zeman, F., Koller, M., Langguth, B., and Landgrebe, M. Tinnitus Research Initiative database study group. (2014). Which tinnitus-related aspects are relevant for quality of life and depression: results from a large international multicentre sample. Health Qual. Life Outcomes 12:7. doi: 10.1186/1477-7525-12-7
Zenner, H. P., Delb, W., Kroner-Herwig, B., Jager, B., Peroz, I., Hesse, G., et al. (2017). A multidisciplinary systematic review of the treatment for chronic idiopathic tinnitus. Eur. Arch. Otorhinolaryngol. 274, 2079–2091. doi: 10.1007/s00405-016-4401-y
Zhang, J., Chen, Y. C., Feng, X., Yang, M., Liu, B., Qian, C., et al. (2015). Impairments of thalamic resting-state functional connectivity in patients with chronic tinnitus. Eur. J. Radiol. 84, 1277–1284. doi: 10.1016/j.ejrad.2015.04.006
Keywords: tinnitus, sound therapy, surface-based morphometry, thickness, structural magnetic resonance imaging
Citation: Wei X, Lv H, Chen Q, Wang Z, Liu C, Zhao P, Gong S, Yang Z and Wang Z (2021) Cortical Thickness Alterations in Patients With Tinnitus Before and After Sound Therapy: A Surface-Based Morphometry Study. Front. Neurosci. 15:633364. doi: 10.3389/fnins.2021.633364
Received: 27 November 2020; Accepted: 12 February 2021;
Published: 05 March 2021.
Edited by:
Fatima T. Husain, University of Illinois at Urbana-Champaign, United StatesReviewed by:
Yihsin Tai, Ball State University, United StatesYu-Chen Chen, Nanjing Medical University, China
Copyright © 2021 Wei, Lv, Chen, Wang, Liu, Zhao, Gong, Yang and Wang. This is an open-access article distributed under the terms of the Creative Commons Attribution License (CC BY). The use, distribution or reproduction in other forums is permitted, provided the original author(s) and the copyright owner(s) are credited and that the original publication in this journal is cited, in accordance with accepted academic practice. No use, distribution or reproduction is permitted which does not comply with these terms.
*Correspondence: Han Lv, Y2hyaXNsdmhhbkAxMjYuY29t; Zhenchang Wang, Y2pyLnd6aGNoQHZpcC4xNjMuY29t