- 1Laboratory of Reproductive Neurobiology, Institute of Experimental Medicine, Budapest, Hungary
- 2Department of Exotic Animal and Wildlife Medicine, University of Veterinary Medicine, Budapest, Hungary
- 3Department of Obstetrics and Food Animal Medicine Clinic, University of Veterinary Medicine, Üllõ, Hungary
- 4MTA-SZIE Large Animal Clinical Research Group, University of Veterinary Medicine, Üllõ, Hungary
- 5INSERM U1215, Neurocentre Magendie, University of Bordeaux, Bordeaux, France
Neurons co-synthesizing kisspeptin (KP), neurokinin B (NKB), and dynorphin (“KNDy neurons”) in the hypothalamic arcuate/infundibular nucleus (INF) form a crucial component of the gonadotropin-releasing hormone (GnRH)/luteinizing hormone (LH) “pulse generator.” The goal of our study was to characterize KP neuron distribution, neuropeptide phenotype and connectivity to GnRH cells in ovariectomized (OVX) dogs and cats with immunohistochemistry on formalin-fixed hypothalamic tissue sections. In both species, KP and NKB neurons occurred in the INF and the two cell populations overlapped substantially. Dynorphin was detected in large subsets of canine KP (56%) and NKB (37%) cells and feline KP (64%) and NKB (57%) cells; triple-labeled (“KNDy”) somata formed ∼25% of all immunolabeled neurons. Substance P (SP) was present in 20% of KP and 29% of NKB neurons in OVX cats but not dogs, although 26% of KP and 24% of NKB neurons in a gonadally intact male dog also contained SP signal. Only in cats, cocaine- and amphetamine regulated transcript was also colocalized with KP (23%) and NKB (7%). In contrast with reports from mice, KP neurons did not express galanin in either carnivore. KP neurons innervated virtually all GnRH neurons in both species. Results of this anatomical study on OVX animals reveal species-specific features of canine and feline mediobasal hypothalamic KP neurons. Anatomical and neurochemical similarities to and differences from the homologous KP cells of more extensively studied rodent, domestic and primate species will enhance our understanding of obligate and facultative players in the molecular mechanisms underlying pulsatile GnRH/LH secretion.
Introduction
Pulsatile secretion of hypothalamic gonadotropin-releasing hormone (GnRH) and adenohypophysial luteinizing hormone (LH) is crucial for mammalian fertility (Belchetz et al., 1978; Santoro et al., 1986). Evidence accumulated over the past two decades indicates that two populations of kisspeptin (KP) synthesizing neurons located in the preoptic region and in the arcuate (ARC)/infundibular (INF) nucleus of the mediobasal hypothalamus, respectively, are key regulators of GnRH/LH secretion. Kisspeptin stimulates LH release (Gottsch et al., 2004; Dhillo et al., 2005; Messager et al., 2005; Plant et al., 2006; Albers-Wolthers et al., 2014, 2016) via directly activating GnRH neurons, which express the KP receptor (Kiss1r) (Irwig et al., 2004; Han et al., 2005; Messager et al., 2005). This mechanism is so basic for reproduction that ablation of Kiss1r from GnRH neurons causes infertility, and conversely, fertility can be reinstated to mice bearing global Kiss1r deletion via the selective reinsertion of the Kiss1r into GnRH neurons (Kirilov et al., 2013).
While preoptic KP neurons are implicated in positive sex steroid feedback and the mid-cycle GnRH/LH surge (Herbison, 2008), KP neurons of the hypothalamic ARC/INF are important regulators of negative sex steroid feedback (Mittelman-Smith et al., 2012) and form key component of the long-sought GnRH/LH pulse generator network (Clarkson et al., 2017). Arcuate KP neurons, widely referred to as “KNDy” neurons (Lehman et al., 2010a), co-synthesize neurokinin B (NKB) and dynorphin (Dyn) in ovine, caprine, and rodent species (Burke et al., 2006; Ciofi et al., 2006; Foradori et al., 2006; Goodman et al., 2007; Navarro et al., 2009; Cheng et al., 2010; Wakabayashi et al., 2010). Abundant network communication of these cells with each other (Burke et al., 2006; Foradori et al., 2006; Goodman et al., 2007; Navarro et al., 2009; Cheng et al., 2010; Wakabayashi et al., 2010) uses multiple peptidergic mechanisms which are critical for shaping the GnRH/LH secretory pulses (Navarro et al., 2009). In mice, excitatory NKB neurotransmission via NK3 autoreceptors and inhibitory Dyn signaling via κ-opioid autoreceptors play crucial roles in this communication (Navarro et al., 2011; de Croft et al., 2013; Ruka et al., 2013; Qiu et al., 2016).
While KP itself does not change the electric activity of KNDy neurons (de Croft et al., 2013), it provides the major output signal from the KNDy neuron network to GnRH neurons which express the Kiss1r (Irwig et al., 2004; Han et al., 2005; Messager et al., 2005).
Against the common assumption that the molecular mechanism of pulsatile GnRH/LH secretion are conserved across mammals, some unexpected species differences have been noticed with respect to the neuropeptide composition of ARC/INF KNDy neurons. Likewise, while NKB has been observed in KP neurons of all species investigated so far, this colocalization is far less complete in humans than suggested earlier for sheep (Goodman et al., 2007) or mice (Navarro et al., 2009) and depends significantly on the sex and age of subjects (Hrabovszky et al., 2011, 2012; Molnar et al., 2012). Accordingly, only ∼36% of NKB neurons express KP immunoreactivity in young human males, which increases to ∼69% in males aged above 50 years (Molnar et al., 2012). In addition, Dyn, which may be present in virtually all KP neurons in sheep (Goodman et al., 2007) and mice (Navarro et al., 2009), can only be visualized very rarely with immunohistochemistry in human KP cells (Hrabovszky et al., 2012, 2019; Skrapits et al., 2015a). Similarly, galanin exhibits a high-degree of colocalization with KP in mice, but not in humans (Skrapits et al., 2015a). To the contrary, postmenopausal women show substance P (SP) immunoreactivity in ∼25% and ∼31% of KP and NKB neurons, respectively (Hrabovszky et al., 2013), whereas these instances of colocalization have not been reported in rodents and stated as non-existing in non-human primates (Kalil et al., 2016). Further, cocaine- and amphetamine-regulated transcript (CART) is present in ∼48% of KP-IR and ∼30% of NKB-IR perikarya in postmenopausal women and also colocalized with KP in non-human primates (True et al., 2017), but not in rodents (True et al., 2013). The above species differences suggest that some co-expressed neuropeptides can play obligate roles in the molecular mechanisms of episodic GnRH/LH secretion, whereas the contribution of others might be facultative.
Additional species-specific features in the neuroanatomy of the pulse generator include the variable topography of GnRH neurons (King and Anthony, 1984) and the relative importance of the ARC/INF KP cell population as a source for KP fibers innervating the somatodendritic compartment of GnRH neurons. Likewise, GnRH neurons in mice are located in the preoptic area and only ∼4% of their KP inputs contain NKB, a distinctive marker of KP fibers originating in the ARC (Kallo et al., 2012). In contrast, GnRH neurons in humans are widely distributed throughout the hypothalamus (Dudas et al., 2000) and at least 10–30% of their KP input originates in the INF and thus, contain both KP and NKB (Hrabovszky et al., 2011; Molnar et al., 2012).
Anatomical comparison of phylogenetically distant mammalian species can provide deeper insight into common and variable operative mechanisms of the GnRH/LH pulse generator. Here we chose to investigate mediobasal hypothalamic KP neuron topography, neurochemistry and connectivity to GnRH neurons in two poorly studied carnivore species, the dog and the cat.
Materials and Methods
Statement of Ethics
All studies were carried out with the permission from the Animal Welfare Committee of the Institute of Experimental Medicine (No. MAB 1/2016) and in accordance with legal requirements of the European Community (Decree 86/609/EEC).
Section Preparation for Immunohistochemistry
Tissue samples were collected from the brain of four ovariectomized (OVX) dogs, one gonadally intact male dog and six OVX cats (for details, see Table 1). All pets were euthanized by a veterinarian because of incurable and severe health issues. The cadavers were decapitated and, following the removal of the calvaria, the brain was removed and immersed immediately into 4% formaldehyde in 0.1 M phosphate buffered saline (PBS; pH 7.4) for 10 days. Hypothalamic blocks were dissected out, infiltrated with 20% sucrose, and sectioned at 30 μm with a Leica SM 2000R freezing microtome (Leica Microsystems, Nussloch Gmbh, Germany). The sections were stored permanently in cryoprotective solution (30% ethylene glycol; 25% glycerol; 0.05 M phosphate buffer; pH 7.4) at −20°C before immunohistochemical processing.
Experiment 1. Mapping the Distribution of Canine and Feline Kisspeptin Neurons and GnRH Cells
To study the topography of canine and feline KP and GnRH neurons, every 12–24th hypothalamic section was used from each brain. The sections were rinsed in PBS for 3 × 5 min and pretreated with a mixture of 3% H2O2 and 0.5% Triton X-100 for 30 min, followed by antigen retrieval with 0.1 M citrate buffer (pH 6.0) at 80°C for 30 min. The sections were incubated overnight at room temperature in different polyclonal antibodies against GnRH or KP (Table 2), followed by biotinylated secondary antibodies (Jackson ImmunoResearch Laboratories, West Grove, PA, United States; 1:500, 60 min) and the ABC Elite reagent (Vector, Burlingame, CA, United States; 1:1,000, 60 min). The peroxidase signal was developed with nickel-intensified diaminobenzidine chromogen. The sections were mounted on microscope slides from Elvanol, air-dried, dehydrated with 70 and 95% (5 min each), followed by 100% (2 × 5 min) ethanol, cleared with xylene (2 × 5 min) and coverslipped with DPX mounting medium (Merck Group, Darmstadt, Germany). Representative light microscopic images were prepared with an AxioCam MRc 5 digital camera mounted on a Zeiss AxioImager M1 microscope, using the AxioVision 4.6 software (Carl Zeiss, Göttingen, Germany). The distribution of GnRH neurons was illustrated with green triangles, each corresponding to a single GnRH neuron of a representative animal. Ten KP neurons found in a given region of a representative animal were illustrated with a red dot. Schemas were created with PowerPoint using modified canine and feline coronal atlas plates from BrainMaps.org(Mikula et al., 2007).
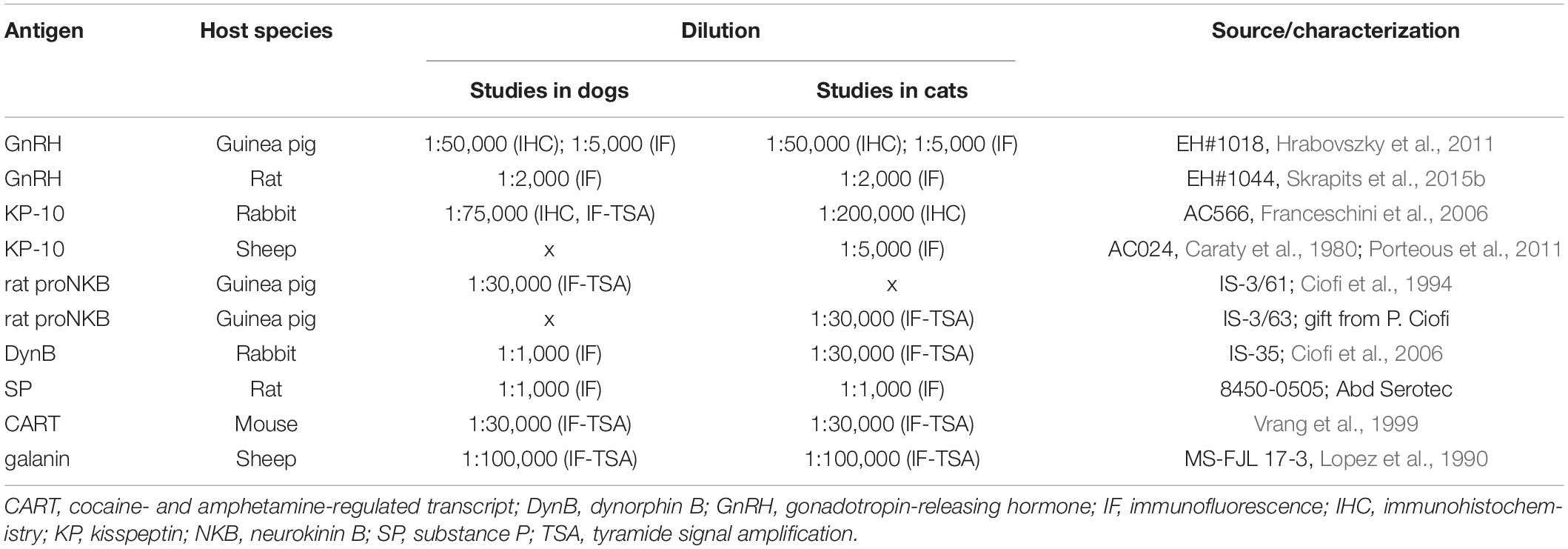
Table 2. Specification, source and dilution of primary antibodies used in immunohistochemical and immunofluorescence experiments.
Experiment 2. Colocalization Studies of Neuropeptide Cotransmitters in Canine and Feline Kisspeptin Neurons
To address the expression of Dyn, SP, CART, and galanin in canine and feline KP and NKB neurons, four separate triple-immunofluorescent experiments were carried out. After section pretreatments, the first neuropeptide antigen was visualized using sequential incubation in polyclonal primary antibodies (48 h, 4°C), followed by biotinylated secondary antibodies (Jackson ImmunoResearch; 1:500, 120 min), ABC Elite reagent (Vector; 1:1,000, 60 min) and finally, Cy3-tyramide (1:1,000 in 0.05 M Tris-HCl buffer, pH 7.6, containing 0.005% H2O2, 30 min) (Hrabovszky et al., 2013). Then, horseradish peroxidase was inactivated with 1% H2O2 for 30 min and the sections were incubated in a cocktail of two additional primary antibodies (48 h, 4°C) to detect the remaining two neuropeptides. One of these was visualized using peroxidase-conjugated secondary antibodies (Jackson ImmunoResearch; 1:250, 120 min), followed by FITC-tyramide (1:1,000 in 0.05 M Tris-HCl buffer, pH 7.6, containing 0.005% H2O2) for 30 min. The third primary antibody was detected with Cy5-conjugated secondary antibodies (Jackson ImmunoResearch; 1:500, 120 min). Specification, source and optimal dilution of primary antibodies are provided in Table 2. One triple-labeling experiment had to use two primary antibodies raised in the same host species. As in a former KP study (Goodman et al., 2007), the strategy to eliminate cross-reaction of secondary antibodies was adapted from a previous technical report (Hunyady et al., 1996). First, the rabbit anti-KP-10 antibodies (#566, Caraty, Franceschini et al., 2006) were applied to the sections at an extremely low concentration (1:75,000) and visualized using biotinylated secondary antibodies (Jackson ImmunoResearch; 1:500, 120 min), ABC Elite reagent (Vector; 1:1,000, 60 min) and finally, Cy3-tyramide (1:1,000 in 0.05 M Tris-HCl buffer, pH 7.6, containing 0.005% H2O2, 30 min). Subsequently, the rabbit anti-DynB (IS-35; Ciofi et al., 2006) antibodies were used at a high concentration (1:1,000), and visualized with Cy5-conjugated anti-rabbit secondary antibodies (Jackson ImmunoResearch; 1:500, 120 min). To verify that the Cy5-conjugated antibodies do not bind to the anti-KP-10 antibodies, control experiments were carried out in parallel. Cy5-conjugated anti-rabbit antibodies did not produce any Cy5 signal in control sections preincubated with highly diluted anti-KP-10 antibodies (#566, 1:75,000), indicating that the KP/DynB colocalization strategy with two rabbit primary antibodies was devoid of non-specific Dyn signal.
In each triple-labeling assay, Z-projected confocal slices were analyzed to study neuropeptide co-expression in KP-IR and NKB-IR perikarya. Representative photomicrographs (1-4/animal) were prepared from triple-labeled sections of the INF of 2-5 dogs and 4 cats. ∼200–900 labeled neurons were analyzed in each experiment and the extents of colocalization were determined separately in each animal and expressed as Mean ± SEM of the animals.
Experiment 3. Analysis of the Connectivity Between Canine and Feline Kisspeptin Neurons and GnRH Cells
Triple-immunofluorescent labeling for KP, NKB and GnRH was carried out to visualize KP and NKB inputs to the somatodendritic compartment of GnRH neurons. The number of KP and NKB contacts were counted on 7–14 randomly selected GnRH neurons from each of 3 OVX dogs and 3 OVX cats and results were expressed as the Mean ± SEM. Appositions were defined as contacts with no gap between the juxtaposed profiles in confocal slices and illustrated in orthogonal side-views of z-stacks. Considering the absence of sharp transition between the somatic and dendritic compartments in typical GnRH neurons, contacts identified on GnRH-IR profiles thinner than 5 μm were arbitrarily categorized as axodendritic. The total length of GnRH dendrites analyzed from dogs and cats were 1160.3 and 1787.7 μm, respectively. The frequency of dendritic afferents was expressed by dividing the number of contacts with the dendrite length of each animal and expressed as Mean ± SEM. Presence of axo-axonal appositions between KP and GnRH fibers was assessed qualitatively via studying the median eminence (ME).
Confocal Microscopy
The immunofluorescent specimens were mounted on glass slides from 0.1M Tris-HCl buffer (pH 7.6) and cover slipped with the aqueous mounting medium Mowiol. Signals were studied with a Zeiss LSM780 confocal microscope. High-resolution images were captured using a 20×/0.8 NA objective, a 0.6–1× optical zoom and the Zen software (CarlZeiss). In innervation studies, a 63×/1.4 NA objective lens with a 1–2.3× optical zoom was used. Different fluorochromes were detected with the following laser lines: 488 nm for FITC, 561 nm for Cy3, 633 nm for Cy5. Emission filters were as follows: 493–556 nm for FITC, 570–624 nm for Cy3 and 638–759 nm for Cy5. To prevent emission crosstalk between the fluorophores, the red channel (Cy3) was recorded separately from the green (FITC)/far-red (Cy5) channels (“smart setup” function). To illustrate the results, confocal Z-stacks (Z-steps: 0.85–1 μm, pixel dwell time: 0.79–1.58 μs, resolution: 1024 × 1024 pixels, pinhole size: set at 1 Airy unit) were merged using maximum intensity Z-projection (ImageJ). Orthogonal side-views were created by Zen software. The final figures were adjusted in Adobe Photoshop and saved as TIF files.
Results
Experiment 1. Topography of Canine and Feline Kisspeptin Neurons and GnRH Cells
Distribution of Kisspeptin-Immunoreactive Neurons in the Infundibular Nucleus
Immunohistochemical studies of tissue sections from different rostro-caudal levels of the hypothalamus (Figures 1A–I) visualized numerous heavily labeled KP-immunoreactive (IR) perikarya in the canine and feline mediobasal hypothalami (Figures 1E–I). The majority of these neurons were concentrated in the caudal part of the INF in both dogs (Figure 1G’) and cats (Figure 1H’). In OVX cats, fewer perikarya also occurred in the rostral INF and occasionally, in the periventricular nucleus. In both species, KP-IR fibers formed dense plexuses in the septal-preoptic region, the periventricular nucleus, the INF, the ventromedial and dorsomedial hypothalamic nuclei and the ME.
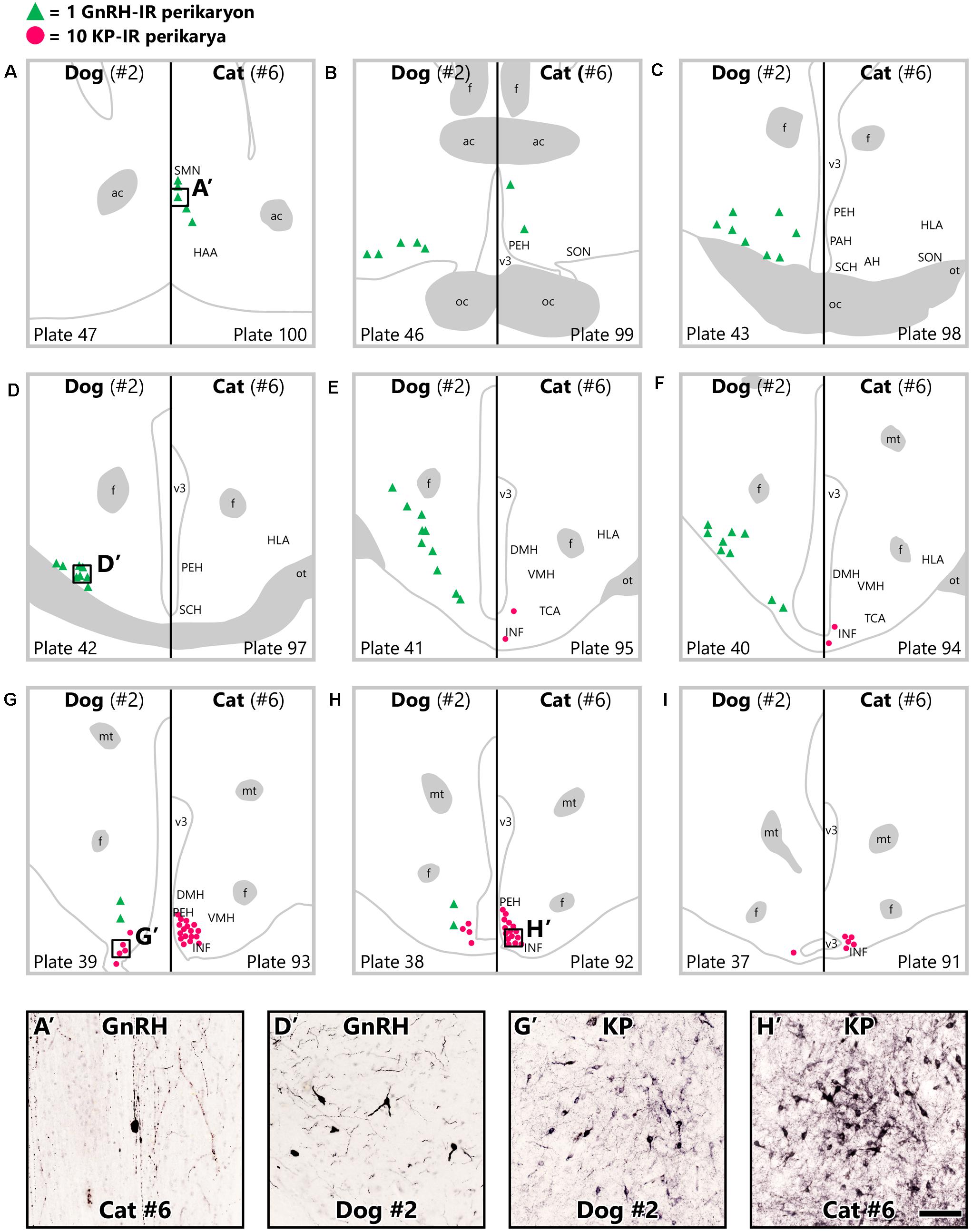
Figure 1. Topography of gonadotropin-releasing hormone and kisspeptin neurons in ovariectomized dogs and cats. Gonadotropin-releasing hormone (GnRH) neurons (green triangles) are scattered throughout the hypothalamus in ovariectomized (OVX) dogs and restricted to the septal-preoptic region in OVX cats. While kisspeptin-immunoreactive (KP-IR) neurons (red dots) are concentrated in the caudal infundibular nucleus in OVX cats as well as dogs, they also occur somewhat more rostrally in the feline mediobasal hypothalamus. Serial coronal schemas were (A–I) obtained from BrainMaps: An Interactive Multiresolution Brain Atlas (BrainMaps.org) (Mikula et al., 2007). Each green triangle corresponds to a single GnRH neuron and each red dot represents 10 KP-IR somata from animals D#2 and C#6. Representative photomicrographs at the bottom display immunolabeled GnRH and KP neurons from OVX cats (A’,H’) and dogs (D’,G’), respectively. ac, anterior commissure; AH, anterior hypothalamic nucleus; DMH, dorsomedial hypothalamic nucleus; f, fornix; HAA, anterior hypothalamic area; HLA, lateral hypothalamic area; INF, infundibular nucleus; mt, mammillothalamic tract; oc, optic chiasm; ot, optic tract; PAH, paraventricular nucleus of the hypothalamus; PEH, periventricular complex of the hypothalamus; SCH, suprachiasmatic nucleus; SMN, medial septal nucleus; SON, supraoptic nucleus; TCA, area of the tuber cinereum; v3, third cerebral ventricle; VMH, ventromedial hypothalamic nucleus. Scale bar: 100 μm.
Differential Distribution of Canine and Feline GnRH Neurons
While KP cells displayed similar basic topography in the canine and feline mediobasal hypothalami with relatively minor species differences, the distribution of GnRH neurons considerably differed between the two species (Figures 1A–H). Accordingly, the distribution of canine GnRH neurons covered wide areas from the preoptic region to the mediobasal hypothalamus (Figures 1B–H), including the supraoptic nucleus, the medial preoptic area, the ventrolateral preoptic nucleus, the lateroanterior hypothalamic nucleus (Figure 1D’), the area of the tuber cinereum-lateral hypothalamic area continuum, the medial tuberal nucleus and the INF. In contrast, GnRH cells of the cat exhibited a much more restricted distribution in the septal-preoptic area (Figures 1A,B), including the medial septal nucleus (Figure 1A’) and the periventricular complex of the hypothalamus; only few scattered neurons were observed in the INF. The bulk of GnRH-IR fibers targeted the ME in both species.
Experiment 2. Neuropeptide Phenotype of Canine and Feline Kisspeptin Neurons
Partial Overlap of Neurokinin B and Dynorphin Neurons With Kisspeptin Neurons
Triple-immunofluorescent studies provided evidence for the presence of both NKB and Dyn in canine and feline KP neurons (Figures 2A,B). The quantitative analysis of 833 immunoreactive cell bodies from two OVX dogs (D#1, D#2) and 931 immunoreactive somata from four OVX cats (C#3, C#4, C#5, C#6) identified Dyn signal in ∼60% of KP neurons in both species (55.7 ± 5.2% in dogs, Figure 2A, upper column; 64.4 ± 7.3% in cats, Figure 2B, upper column), in 36.9 ± 3.8% of NKB neurons in dogs (Figure 2A, bottom column) and in 56.6 ± 9.6% of NKB neurons in cats (Figure 2B, bottom column). 66.8 ± 2.4% of KP perikarya contained NKB (Figure 2B, upper column) and 84.0 ± 3.0% of NKB neurons contained KP in cats (Figure 2B, bottom column), whereas virtually all (98.8 ± 0.8%) KP neurons expressed NKB (Figure 2A, upper column) and 49.3 ± 5.3% of NKB neurons expressed KP (Figure 2A, bottom column) in dogs. Triple-labeled (“KNDy”) somata contributed to 25.9 ± 1.4% and 22.3 ± 2.5% of the labeled neurons in dogs and cats, respectively (white slices of pie charts in Figures 2A,B). Interestingly, the most frequently encountered phenotypes were NKB-only neurons in dogs (38.9 ± 0.7%; Figure 2A, green slice of pie chart), and Dyn-only neurons in cats (38.2 ± 1.8%; Figure 2B, blue slice of pie chart).
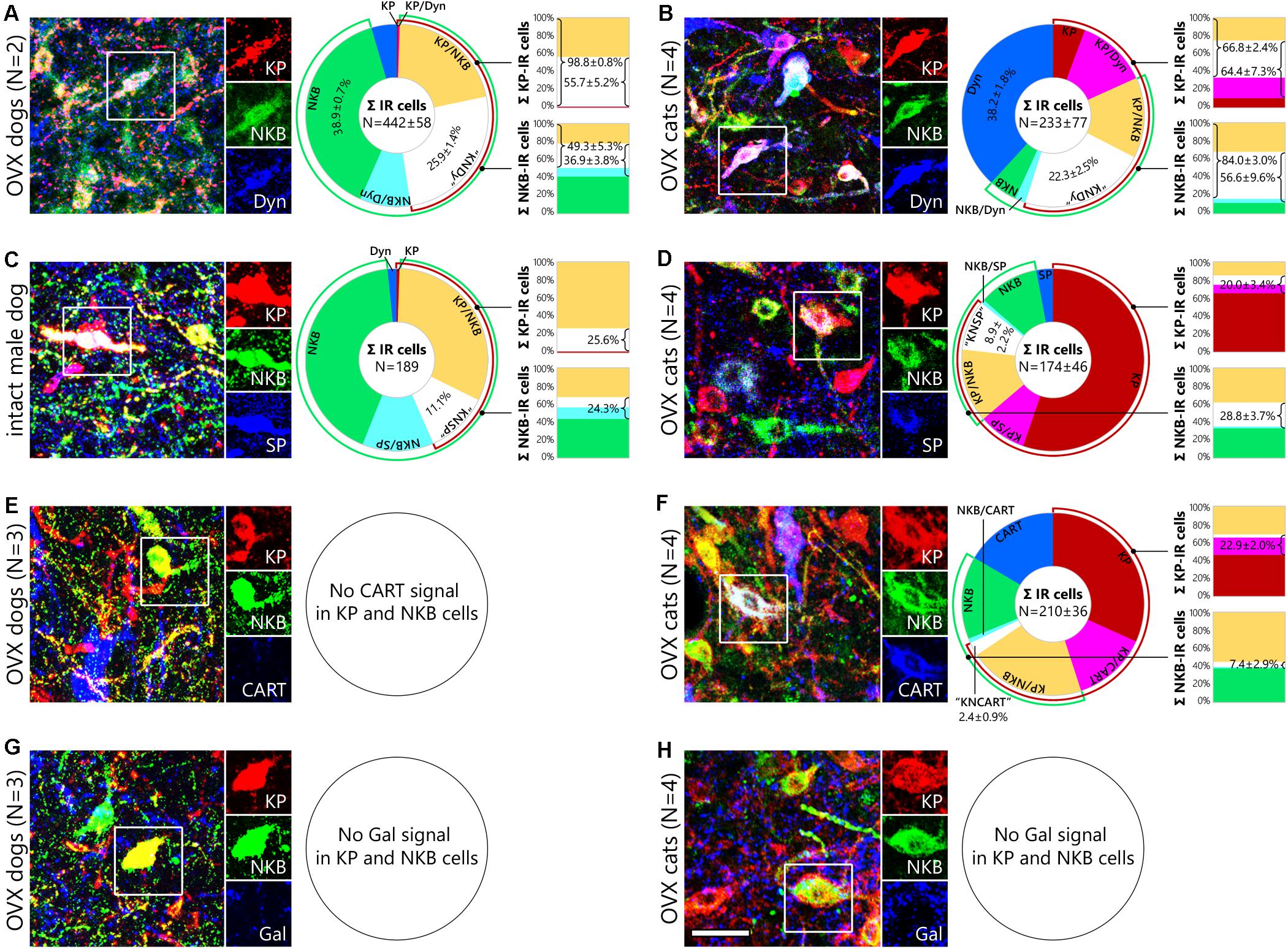
Figure 2. Neurochemistry of canine and feline kisspeptin and neurokinin B cells. Representative photomicrographs illustrate the neuropeptide phenotype of canine and feline kisspeptin (KP; red) and neurokinin B (NKB; green) neurons. Cells framed in the three-color figures are shown in separate color channels on the right side of each panel. KP and NKB immunoreactivities overlap considerably but incompletely in the infundibular nucleus of dogs (A,C,E,G) and ovariectomized (OVX) cats (B,D,F,H). Pie charts illustrate the percent distribution of distinct neuronal phenotypes from triple-immunofluorescence experiments addressing dynorphin (Dyn; A), substance P (SP; B) and cocaine- and amphetamine-regulated transcript (CART; C) co-expression in KP and NKB neurons. Bar graphs show percentages of neuropeptide colocalization in KP neurons (upper columns) and NKB neurons (bottom columns). Dynorphin signal (blue) occurs in large subsets of canine (A) and feline (B) KP and NKB cells; ∼25% of the immunolabeled infundibular neurons are triple-labeled (“KNDy”) cells in both dogs and cats. Substance P (blue) is detectable in 26% of KP and 24% of NKB neurons in an intact male dog (C) as well as in 20% of KP and 29% of NKB cells in OVX cats (D). CART (blue) is also present in 23% of KP and 7% of NKB neurons in OVX cats (F), but no CART signal is detectable in canine KP and NKB cells (E). Galanin is not expressed in KP and NKB neurons of either species (G,H). CART, cocaine- and amphetamine regulated transcript; Dyn, dynorphin; Gal, galanin; KP, kisspeptin; “KNCART”, kisspeptin/neurokinin B/cocaine- and amphetamine regulated transcript-positive cells; “KNDy”, kisspeptin/neurokinin B/dynorphin-positive cells; “KNSP”, kisspeptin/neurokinin B/substance P-positive cells; NKB, neurokinin B; SP, substance P. Scale bar: 50 μm.
Substance P Synthesis in Canine and Feline Kisspeptin and Neurokinin B Neurons
The putative expression of SP in KP and NKB neurons was addressed in additional triple-immunofluorescent experiments. Only two (D#1, D#2) out of the four OVX dogs contained SP signal in KP and NKB cells and SP was present in less than 2% of these cells. In contrast, quantitative analysis of 189 immunolabeled perikarya from a gonadally intact male dog (D#5) revealed SP signal in 25.6% of KP neurons (Figure 2C, upper column) and 24.3% of NKB neurons (Figure 2C, bottom column); 11.1% of the labeled neurons were triple-labeled (white slice of the pie chart in Figure 2C). As opposed to OVX dogs, OVX cats (C#2, C#3, C#4, C#6) contained SP signal in 20.0 ± 3.4% of KP perikarya (Figure 2D, upper column) and 28.8 ± 3.7 of NKB perikarya (Figure 2D, bottom column); 8.9 ± 2.2% of the 695 immunolabeled neurons were KP/NKB/SP-positive (white slice of pie chart in Figure 2D).
Cocaine- and Amphetamine Regulated Transcript in Kisspeptin and Neurokinin B Neurons of Cats but Not Dogs
Analysis of sections triple-labeled for KP, NKB and CART provided evidence that subpopulation of feline KP and NKB neurons also synthesize CART. The quantitative analysis of 838 cell bodies from four OVX cats (C#1, C#2, C#4, C#6) provided evidence for the presence of CART in 22.9 ± 2.0% of KP-IR (Figure 2F, upper column) and 7.4 ± 2.9% of NKB-IR (Figure 2F, bottom column) neurons; triple-labeled somata contributed to 2.4 ± 0.9% of the IR perikarya (white slice of pie chart in Figure 2F). CART was not detectable in canine KP or NKB neurons (Figure 2E).
Absence of Galanin in Kisspeptin and Neurokinin B Cells
Galanin, which was previously detected in KP neurons of mice (Porteous et al., 2011; Kallo et al., 2012) but not humans (Skrapits et al., 2015a), was not present in KP and NKB cells of OVX dogs (D#2, D#3, D#4) or cats (C#3, C#4, C#5, C#6) (Figures 2G,H).
Experiment 3. Kisspeptin Innervation of GnRH Cells
Kisspeptin Inputs to the Somatodendritic Compartment of GnRH Neurons
In OVX cats (C#3, C#4, C#6), KP neurons sent dense projections to the septal-preoptic region where most GnRH neurons were located. Virtually all (94.4 ± 5.6%) GnRH neurons received at least one KP-IR input; appositions were observed on both the somatic (6.9 ± 0.2 inputs/soma, Figure 3C’) and the dendritic (7.5 ± 1.4 contacts/100 μm dendrite, Figure 3C”) compartments. The feline hypothalamus showed very poor axonal labeling for the INF KP neuron markers NKB or Dyn, making it impossible to assess whether and to what extent, KP afferents to GnRH neurons arise from the INF. In OVX dogs (D#4, D#5, D#6), 77.6 ± 17.6% of GnRH neurons (75.8 ± 18.3% in the preoptic region and 83.3 ± 16.7% in the INF) were innervated by at least one KP fiber and GnRH neurons in the INF also received more KP-IR contacts than those located in more rostral hypothalamic regions [6.3 ± 1.0 (Figure 3A’) vs. 2.0 ± 0.7 inputs/soma and 7.9 ± 5.5 (Figure 3A”) vs. 0.9 ± 0.6 inputs/100 μm dendrite]. 57.6 ± 14.1% of inputs (93.0 ± 1.5% in the INF) targeting the somatodendritic compartment were also immunopositive for NKB, indicating that more than half of these KP afferents arise from the INF (Figure 3A).
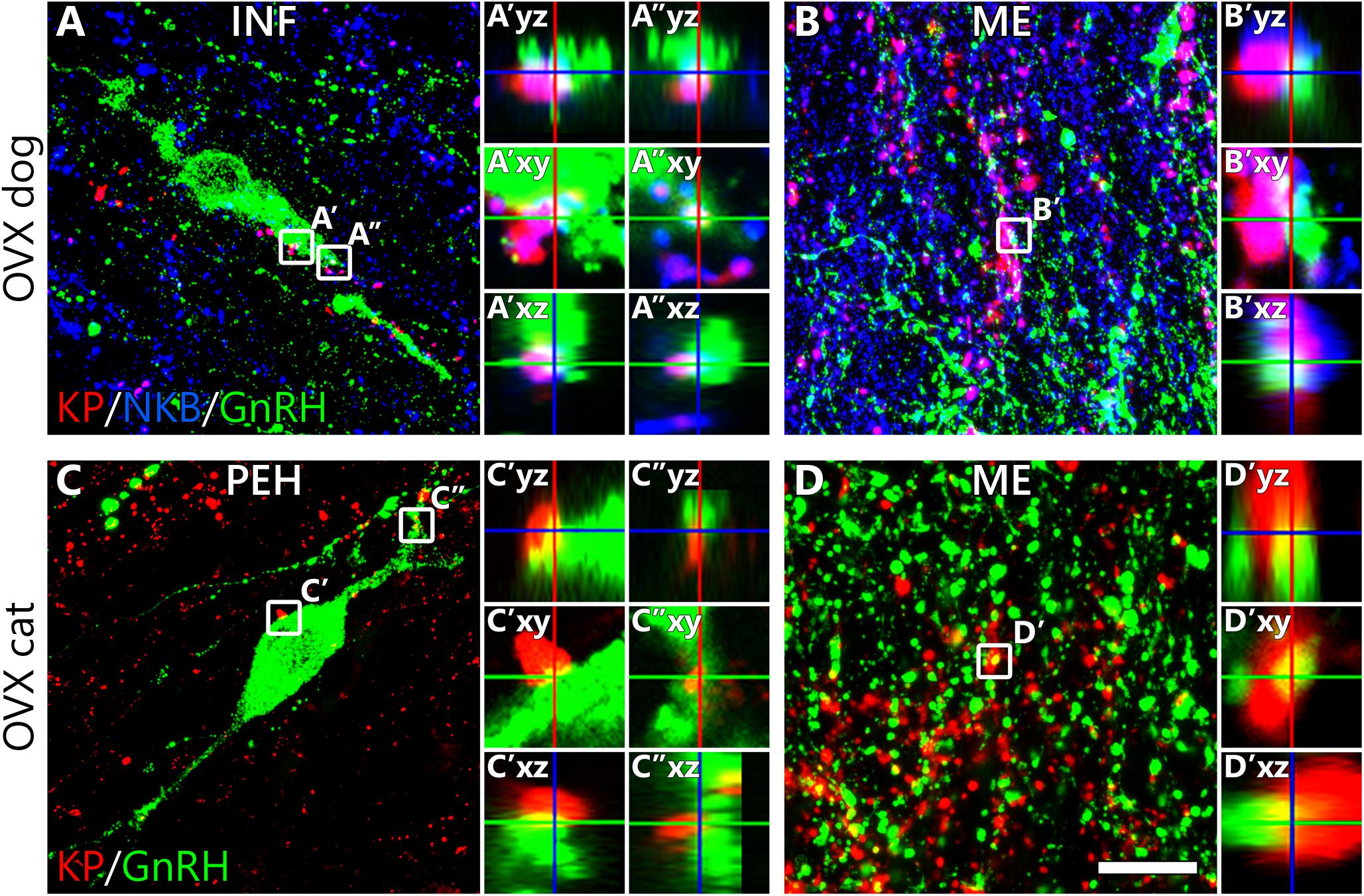
Figure 3. Kisspeptin innervation of canine and feline gonadotropin-releasing hormone neurons. Results of triple- and dual-immunofluorescent studies on ovariectomized dogs (A,B) and cats (C,D), respectively, reveal that kisspeptin (KP) fibers (red) establish axo-somatic (A’,C’), axo-dendritic (A”,C”) and axo-axonal (B’,D’) contacts with gonadotropin-releasing hormone (GnRH)-immunoreactive (green) neurons. Successful detection of neurokinin B (NKB) in fibers of the canine hypothalamus allows the identification of KP fibers that arise from KP/NKB dual-phenotype neurons of the infundibular nucleus. High-power images of framed regions show absence of gaps between the juxtaposed profiles in orthogonal side-views of contacts. INF, infundibular nucleus; ME, median eminence; PEH, periventricular complex of the hypothalamus; Scale bar: 20 μm in panels (A,C) (4 μm in insets), 16 μm in panels (B,D) (3 μm in insets).
Axo-axonal Communication Between Kisspeptin and GnRH Neurons in the Median Eminence
The distribution of hypophysiotropic GnRH fibers in the ME overlapped with a dense KP-IR fiber plexus in both dogs and cats (Figures 3B,D). Contacts suggesting axo-axonal interactions were often detected between the juxtaposed GnRH and KP profiles. In dogs, many KP fibers contained NKB signal as well, indicating their mediobasal hypothalamic origin (Figure 3B).
Discussion
The topography of GnRH and KP neurons and the peptide neurochemistry of the putative pulse generator KP neurons within the mediobasal hypothalamus differ substantially between the most widely studied laboratory rodent models and humans. Although the GnRH/LH pulse generator function is thought to be conserved across mammals, differences indicate that the anatomical basis and molecular mechanisms of episodic GnRH secretion can vary between species. Here we carried out the neuroanatomical characterization of KP and GnRH neurons in OVX dogs and cats. Information about the pulse generator network of these two poorly studied carnivore species may provide important insight into evolutionarily conserved vs. variable mechanisms of episodic GnRH/LH secretion.
Distribution of GnRH and Kisspeptin Neurons in Dogs and Cats
GnRH neurons are not concentrated within distinct hypothalamic nuclei and their distribution varies considerably between species (King and Anthony, 1984). For example, while GnRH cell bodies are located exclusively within the septal-preoptic region in laboratory rodents (Merchenthaler et al., 1980), in humans they are scattered quite widely from the septo-preoptic region to the retromammillary area (Dudas et al., 2000; Hrabovszky and Liposits, 2013). Similarly to human, OVX dogs used in our study contained GnRH-IR perikarya throughout the hypothalamus, including the INF. The functional difference between the rostral and the caudal GnRH cell groups of this species requires clarification. In sheep, both the preoptic and the mediobasal hypothalamic GnRH neuron populations express Fos during the LH surge (Moenter et al., 1993), whereas Fos only occurs in GnRH neurons of the ARC at times of enhanced pulsatile GnRH/LH secretion (Boukhliq et al., 1999).
In contrast with dogs, OVX cats contained the majority of GnRH neurons in the septal-preoptic region. This observation is in line with the previously reported distribution of GnRH-immunopositive perikarya in cats (Barry and Dubois, 1975; Mikami et al., 1985; Belda et al., 2000), except that in one study adult male (Belda et al., 2000) cats also contained GnRH cell bodies in the INF.
A variety of mammals, including humans (Hrabovszky et al., 2010; Rumpler et al., 2020), contain two major KP cell populations in the preoptic region and in the ARC/INF of the mediobasal hypothalamus, respectively (Lehman et al., 2010b). An additional small group of KP neurons has been identified recently in the medial amygdala of rodents (Stephens and Kauffman, 2017). In rats and mice, preoptic KP neurons play important roles in positive estrogen feedback and the mid-cycle GnRH/LH surge (Herbison, 2008). Kiss1 expression in these cells is regulated positively by estrogens (Adachi et al., 2007). The largest KP cell group in all species studied so far has been localized to the ARC/INF of the mediobasal hypothalamus (Lehman et al., 2010b); these neurons have been implicated in negative sex steroid feedback (Mittelman-Smith et al., 2012) and their Kiss1 expression is inhibited by gonadal sex steroid hormones (Smith et al., 2005). There is accumulating evidence that KP neurons of the ARC/INF play a critical role in the generation of GnRH/LH pulses (Clarkson et al., 2017).
Our mapping experiments localized the majority of KP neurons to the INF of OVX dogs and cats. In addition, the feline, but not the canine hypothalamus contained scattered IR neurons in the periventricular nucleus, in line with results of a recent immunohistochemical study by Amelkina et al. (2019). These authors studied intact female cats and identified two additional KP cell populations in the anterior periventricular nucleus and the amygdaloid complex, respectively (Amelkina et al., 2019). From these, the first population may be homologous with KP neurons of the rodent anteroventral periventricular nucleus which are activated during positive feedback (Herbison, 2008). On the other hand, the role of KP neurons in the cat amygdala is less clear and it was proposed that these KP neurons do not correspond to KP cells described in the medial amygdala in rodents (Amelkina et al., 2019).
Kisspeptin neurons of the anterior periventricular nucleus and the amygdaloid complex remained undetected in our present study. This is most likely explained by the use of OVX animals, in view that the expression of Kiss1 is positively regulated by estrogens both in the preoptic region (Adachi et al., 2007) and the amygdala (Stephens and Kauffman, 2017). In the context of ovulation, it is important to note that the cat is a reflex ovulator species in which the preovulatory GnRH/LH surge is induced by the receipt of genital somatosensory stimuli during mating. In such species, activation of brainstem noradrenergic neurons is thought to act in the mediobasal hypothalamus to promote GnRH release from nerve terminals in the ME (Bakker and Baum, 2000). While induced ovulating species generally do not show steroid-induced preovulatory LH surges, the cat seems to be an exception in this respect because group-housed females are capable of ovulating spontaneously (Gudermuth et al., 1997). It remains to be clarified how the hypothalamic KP and GnRH neuronal systems of the cat are integrated into the neuronal networks regulating spontaneous vs. reflex ovulation.
Connections Between Kisspeptin Cells and GnRH Neurons in Dogs and Cats
Kisspeptin input to GnRH neurons is critical for reproduction in mice (Kirilov et al., 2013) and humans (de Roux et al., 2003; Seminara et al., 2003), and unlikely to be less important in other mammals like dogs and cats. KP fibers in different species innervate the somatodendritic compartment of GnRH neurons (Kinoshita et al., 2005; Clarkson and Herbison, 2006; Ramaswamy et al., 2008; Smith et al., 2008; Hrabovszky et al., 2010). In mice, these inputs tend to arise from the preoptic region because most of them are devoid of the arcuate nucleus marker NKB (Kallo et al., 2012). The excitatory responses of GnRH neurons to KP (Han et al., 2005; Dumalska et al., 2008; Pielecka-Fortuna et al., 2008) are likely mediated by somatodendritic Kiss1r during positive estrogen feedback. Humans whose GnRH neurons are rather scattered within the hypothalamus (Dudas et al., 2000) differ from mice in that at least 10–30% of their KP afferents contain the INF marker NKB (Hrabovszky et al., 2011; Molnar et al., 2012). In our present study on OVX dogs and cats, the cell bodies and dendrites of GnRH neurons received abundant innervation from KP axons. In dogs, successful detection of NKB signal in over 90% of KP-IR afferents also allowed us to conclude that a high number of these KP inputs originate in the INF. In contrast, lack of sufficient axonal signal for NKB and Dyn within KP axons of the cat did not allow us to firmly establish the importance of KP inputs arising from the INF in this species.
It is known that KP-IR neurons of the mediobasal hypothalamus also establish axo-axonal contacts with hypophysiotropic GnRH fiber varicosities in the ME of various species (Ramaswamy et al., 2008; Krajewski et al., 2010; Matsuyama et al., 2011; Borsay et al., 2014). Kisspeptin likely binds to Kiss1r on these GnRH fibers when inducing GnRH release from mediobasal hypothalamic explants (which contain only a few GnRH cell bodies in rodents) in an action potential-independent manner (d’Anglemont de Tassigny et al., 2008). Systemic KP injection is also thought to stimulate LH secretion via acting on these processes (Matsui et al., 2004; Dhillo et al., 2005; Navarro et al., 2005; Shahab et al., 2005). Finally, axo-axonal contacts may also play a paramount role in the communication between the putative pulse generator KNDy neurons and the GnRH neurosecretory system. Temporal correlation between the pulsatile KP output and GnRH secretory pulses in the ME of monkeys provide strong support for this notion (Keen et al., 2008). Results of our present study established that, similarly to other species, dogs and cats also contain axo-axonal appositions between GnRH-IR and KP-IR fibers in the ME. In addition to these bona fide axo-axonal appositions, it remains possible that KP axons also innervate more proximal segments of GnRH fibers before they spray into terminal axon branches. In mice, GnRH fibers bear mixed dendritic and axonal properties (called “dendrons”) and at the border of the ME they receive a particularly high density of synaptic inputs. This connectivity may represent an important anatomical pathway in the regulation of episodic GnRH/LH secretion (Moore et al., 2018). The existence of such GnRH dendrons and their synaptic regulation in other species, including cats and dogs, will require clarification.
Species Differences in the Neurochemistry of Kisspeptin Cells
The most important message of our present study is the unique neurochemistry of canine and feline mediobasal hypothalamic KP neurons, with both similarities to and differences from the KP system of other species.
Similarities to rodents (Navarro et al., 2009) and the sheep (Goodman et al., 2007) include the co-expression of the three “KNDy” neuropeptides in the same cells. This evolutionarily conserved phenomenon suggests that all three neuropeptides play obligate roles in the generation of GnRH/LH pulses. However, it is worth to note that KP neurons in the INF of postmortem human brains only show partial overlap between the KNDy neuropeptides which also varies considerably with sex and age. For example, only ∼36% of NKB neurons express KP immunoreactivity in young human males (Hrabovszky et al., 2012; Molnar et al., 2012). In addition, Dyn immunoreactivity can only be detected very rarely in human KP and NKB neurons and their processes (Hrabovszky et al., 2012, 2019; Skrapits et al., 2015a), in contrast with much higher degrees of co-expression in sheep (Goodman et al., 2007) and mice (Navarro et al., 2009). An interesting similarity between dogs and cats and the human is the relatively high abundance of NKB neurons without KP signal, whereas most KP neurons in all three species contain NKB signal. Our immunofluorescence results on OVX dogs and cats indicate that about ∼25% of labeled neurons in the canine and feline INF are triple-labeled (“KNDy”) neurons, supporting the notion that the overlap of the three KNDy peptides might be functionally important, although far from being complete. It will be important to more thoroughly investigate the relationship between the reproductive status and the co-synthesis/co-secretion of each KNDy peptide in different species.
In addition to identifying the above species similarities, we also observed clear differences between OVX cats and dogs and the more extensively studied rodent and primate species. As opposed to mice (True et al., 2013) and similarly to humans (Hrabovszky et al., 2013; Skrapits et al., 2014), OVX cats showed a considerable co-expression of CART and SP in KP and NKB neurons. Interestingly, the two carnivores differed from each other in this context; canine KP and NKB neurons did not co-contain CART signal, whereas feline KP and NKB neurons did. Similarly, we did not observe SP signal in KP and NKB neurons in OVX dogs. Despite this negative finding, SP co-synthesis likely plays some role in the physiology of these neurons in this species because a gonadally intact male dog included in our study showed SP signal in many KP and NKB neurons. This finding raises the possibility that sex and/or the gonadal steroid status exert critical effects on the SP co-synthesis of KP neurons. Finally, galanin, which has only been observed in murine KP neurons (Porteous et al., 2011; Kallo et al., 2012), was absent from KP cells of both dogs and cats which is similar to our earlier observation on human KP neurons (Skrapits et al., 2015a). Neurochemical differences and common features of different species suggest that some neuropeptides play facultative roles in the generation or fine-tuning of GnRH/LH pulses, whereas the role of others is evolutionarily more conserved. One should keep in mind that colocalization results from different species were obtained using different antibodies and different immunohistochemical techniques. Therefore, comparison of the exact colocalization percentages has innate pitfalls.
Conclusion
In this study we characterize the distribution of GnRH and KP neurons in the hypothalamus of OVX dogs and cats and identify conspicuous anatomical similarities to and differences from laboratory rodents and primates. We show that the majority of GnRH neurons receive input from KP neurons. The vast majority of these inputs, at least in OVX dogs, originate from KP neurons of the INF. Finally, we determine the unique neurochemistry of KP and NKB neurons in the INF of OVX dogs and cats. Results of immunofluorescent colocalization studies indicate that the three KNDy peptides show partial co-expression, with ∼25% of neurons expressing all three substances. Galanin, observed earlier in murine KNDy neurons only, is absent from canine and feline KNDy neurons. SP, localized previously to human KP neurons but not to rodent KP cells, is present in subsets of KP and NKB neurons in OVX cats but not in OVX dogs, although KP and NKB neurons of a gonadally intact male dog also synthesized SP. Finally, CART, identified earlier as a cotransmitter of KP cells both in human and in non-human primates, occurs in KP and NKB neurons of OVX cats but not OVX dogs.
Anatomical and neurochemical similarities to and differences from homologous and more extensively investigated rodent, domestic and primate KP cells will contribute to our understanding of obligate and facultative players in the molecular mechanisms underlying episodic GnRH/LH secretion in mammals.
Data Availability Statement
The raw data supporting the conclusions of this article will be made available by the authors, without undue reservation.
Ethics Statement
The animal study was reviewed and approved by Animal Welfare Committee of the Institute of Experimental Medicine, Institute of Experimental Medicine, Budapest, Hungary. Written informed consent for participation was not obtained from the owners because all pets were euthanized by an authorized veterinarian because of incurable and severe health issues. No animal sacrifice was needed to carry out these studies.
Author Contributions
KS supervised the work. KS, ÉR, and EH wrote the manuscript with support from all listed co-authors. Tissue samples were provided by FB, OS, and AH. PC provided important reagents. All authors were involved in conceiving the experiments.
Funding
The research leading to these results has received funding from the National Science Foundation of Hungary (PD125393 and PD134837 to KS and K128317 to EH) and the Hungarian Brain Research Program (2017-1.2.1-NKP-2017-00002 to EH).
Conflict of Interest
The authors declare that the research was conducted in the absence of any commercial or financial relationships that could be construed as a potential conflict of interest.
Acknowledgments
The authors would like to thank Drs. J.T. Clausen (Novo Nordisk), A. Caraty (Nouzilly, France), and I. Merchenthaler for kindly providing CART, KP, and galanin antibodies, respectively for these studies.
References
Adachi, S., Yamada, S., Takatsu, Y., Matsui, H., Kinoshita, M., Takase, K., et al. (2007). Involvement of anteroventral periventricular metastin/kisspeptin neurons in estrogen positive feedback action on luteinizing hormone release in female rats. J. Reprod. Dev. 53, 367–378. doi: 10.1262/jrd.18146
Albers-Wolthers, C. H., De Gier, J., Rutten, V. P., Van Kooten, P. J., Leegwater, P. A., Schaefers-Okkens, A. C., et al. (2016). The effects of kisspeptin agonist canine KP-10 and kisspeptin antagonist p271 on plasma LH concentrations during different stages of the estrous cycle and anestrus in the bitch. Theriogenology 86, 589–595. doi: 10.1016/j.theriogenology.2016.02.009
Albers-Wolthers, K. H., De Gier, J., Kooistra, H. S., Rutten, V. P., Van Kooten, P. J., De Graaf, J. J., et al. (2014). Identification of a novel kisspeptin with high gonadotrophin stimulatory activity in the dog. Neuroendocrinology 99, 178–189. doi: 10.1159/000364877
Amelkina, O., Tanyapanyachon, P., Thongphakdee, A., and Chatdarong, K. (2019). Identification of feline Kiss1 and distribution of immunoreactive kisspeptin in the hypothalamus of the domestic cat. J. Reprod. Dev. 65, 335–343. doi: 10.1262/jrd.2018-101
Bakker, J., and Baum, M. J. (2000). Neuroendocrine regulation of GnRH release in induced ovulators. Front. Neuroendocrinol. 21, 220–262. doi: 10.1006/frne.2000.0198
Barry, J., and Dubois, M. P. (1975). Immunofluorescence study of LRF-producing neurons in the cat and the dog. Neuroendocrinology 18, 290–298. doi: 10.1159/000122410
Belchetz, P. E., Plant, T. M., Nakai, Y., Keogh, E. J., and Knobil, E. (1978). Hypophysial responses to continuous and intermittent delivery of hypopthalamic gonadotropin-releasing hormone. Science 202, 631–633. doi: 10.1126/science.100883
Belda, M., Covenas, R., Narvaez, J. A., Aguirre, J. A., and Tramu, G. (2000). Distribution of luteinizing hormone-releasing hormone in the upper brainstem and diencephalon of the cat: an immunocytochemical study. Brain Res. Bull. 51, 281–291. doi: 10.1016/s0361-9230(99)00221-x
Borsay, B. A., Skrapits, K., Herczeg, L., Ciofi, P., Bloom, S. R., Ghatei, M. A., et al. (2014). Hypophysiotropic gonadotropin-releasing hormone projections are exposed to dense plexuses of kisspeptin, neurokinin B and substance P immunoreactive fibers in the human: a study on tissues from postmenopausal women. Neuroendocrinology 100, 141–152. doi: 10.1159/000368362
Boukhliq, R., Goodman, R. L., Berriman, S. J., Adrian, B., and Lehman, M. N. (1999). A subset of gonadotropin-releasing hormone neurons in the ovine medial basal hypothalamus is activated during increased pulsatile luteinizing hormone secretion. Endocrinology 140, 5929–5936. doi: 10.1210/endo.140.12.7216
Burke, M. C., Letts, P. A., Krajewski, S. J., and Rance, N. E. (2006). Coexpression of dynorphin and neurokinin B immunoreactivity in the rat hypothalamus: morphologic evidence of interrelated function within the arcuate nucleus. J. Comp. Neurol. 498, 712–726. doi: 10.1002/cne.21086
Caraty, A., De Reviers, M. M., Pelletier, J., and Dubois, M. P. (1980). Reassessment of LRF radioimmunoassay in the plasma and hypothalamic extracts of rats and rams. Reprod. Nutr. Dev. 20, 1489–1501. doi: 10.1051/rnd:19800808
Cheng, G., Coolen, L. M., Padmanabhan, V., Goodman, R. L., and Lehman, M. N. (2010). The kisspeptin/neurokinin B/dynorphin (KNDy) cell population of the arcuate nucleus: sex differences and effects of prenatal testosterone in sheep. Endocrinology 151, 301–311. doi: 10.1210/en.2009-0541
Ciofi, P., Krause, J. E., Prins, G. S., and Mazzuca, M. (1994). Presence of nuclear androgen receptor-like immunoreactivity in neurokinin B-containing neurons of the hypothalamic arcuate nucleus of the adult male rat. Neurosci. Lett. 182, 193–196. doi: 10.1016/0304-3940(94)90795-1
Ciofi, P., Leroy, D., and Tramu, G. (2006). Sexual dimorphism in the organization of the rat hypothalamic infundibular area. Neuroscience 141, 1731–1745. doi: 10.1016/j.neuroscience.2006.05.041
Clarkson, J., Han, S. Y., Piet, R., Mclennan, T., Kane, G. M., Ng, J., et al. (2017). Definition of the hypothalamic GnRH pulse generator in mice. Proc. Natl. Acad. Sci. U.S.A. 114, E10216–E10223.
Clarkson, J., and Herbison, A. E. (2006). Postnatal development of kisspeptin neurons in mouse hypothalamus; sexual dimorphism and projections to gonadotropin-releasing hormone neurons. Endocrinology 147, 5817–5825. doi: 10.1210/en.2006-0787
d’Anglemont de Tassigny, X., Fagg, L. A., Carlton, M. B., and Colledge, W. H. (2008). Kisspeptin can stimulate gonadotropin-releasing hormone (GnRH) release by a direct action at GnRH nerve terminals. Endocrinology 149, 3926–3932. doi: 10.1210/en.2007-1487
de Croft, S., Boehm, U., and Herbison, A. E. (2013). Neurokinin B activates arcuate kisspeptin neurons through multiple tachykinin receptors in the male mouse. Endocrinology 154, 2750–2760. doi: 10.1210/en.2013-1231
de Roux, N., Genin, E., Carel, J. C., Matsuda, F., Chaussain, J. L., and Milgrom, E. (2003). Hypogonadotropic hypogonadism due to loss of function of the KiSS1-derived peptide receptor GPR54. Proc. Natl. Acad. Sci. U.S.A. 100, 10972–10976. doi: 10.1073/pnas.1834399100
Dhillo, W. S., Chaudhri, O. B., Patterson, M., Thompson, E. L., Murphy, K. G., Badman, M. K., et al. (2005). Kisspeptin-54 stimulates the hypothalamic-pituitary gonadal axis in human males. J. Clin. Endocrinol. Metab. 90, 6609–6615. doi: 10.1210/jc.2005-1468
Dudas, B., Mihaly, A., and Merchenthaler, I. (2000). Topography and associations of luteinizing hormone-releasing hormone and neuropeptide Y-immunoreactive neuronal systems in the human diencephalon. J. Comp. Neurol. 427, 593–603. doi: 10.1002/1096-9861(20001127)427:4<593::aid-cne7>3.0.co;2-c
Dumalska, I., Wu, M., Morozova, E., Liu, R., Van Den Pol, A., and Alreja, M. (2008). Excitatory effects of the puberty-initiating peptide kisspeptin and group I metabotropic glutamate receptor agonists differentiate two distinct subpopulations of gonadotropin-releasing hormone neurons. J. Neurosci. 28, 8003–8013. doi: 10.1523/jneurosci.1225-08.2008
Foradori, C. D., Amstalden, M., Goodman, R. L., and Lehman, M. N. (2006). Colocalisation of dynorphin a and neurokinin B immunoreactivity in the arcuate nucleus and median eminence of the sheep. J. Neuroendocrinol. 18, 534–541. doi: 10.1111/j.1365-2826.2006.01445.x
Franceschini, I., Lomet, D., Cateau, M., Delsol, G., Tillet, Y., and Caraty, A. (2006). Kisspeptin immunoreactive cells of the ovine preoptic area and arcuate nucleus co-express estrogen receptor alpha. Neurosci. Lett. 401, 225–230. doi: 10.1016/j.neulet.2006.03.039
Goodman, R. L., Lehman, M. N., Smith, J. T., Coolen, L. M., De Oliveira, C. V., Jafarzadehshirazi, M. R., et al. (2007). Kisspeptin neurons in the arcuate nucleus of the ewe express both dynorphin A and neurokinin B. Endocrinology 148, 5752–5760. doi: 10.1210/en.2007-0961
Gottsch, M. L., Cunningham, M. J., Smith, J. T., Popa, S. M., Acohido, B. V., Crowley, W. F., et al. (2004). A role for kisspeptins in the regulation of gonadotropin secretion in the mouse. Endocrinology 145, 4073–4077. doi: 10.1210/en.2004-0431
Gudermuth, D. F., Newton, L., Daels, P., and Concannon, P. (1997). Incidence of spontaneous ovulation in young, group-housed cats based on serum and faecal concentrations of progesterone. J. Reprod. Fertil. Suppl. 51, 177–184.
Han, S. K., Gottsch, M. L., Lee, K. J., Popa, S. M., Smith, J. T., Jakawich, S. K., et al. (2005). Activation of gonadotropin-releasing hormone neurons by kisspeptin as a neuroendocrine switch for the onset of puberty. J. Neurosci. 25, 11349–11356. doi: 10.1523/jneurosci.3328-05.2005
Herbison, A. E. (2008). Estrogen positive feedback to gonadotropin-releasing hormone (GnRH) neurons in the rodent: the case for the rostral periventricular area of the third ventricle (RP3V). Brain Res. Rev. 57, 277–287. doi: 10.1016/j.brainresrev.2007.05.006
Hrabovszky, E., Borsay, B. A., Rácz, K., Herczeg, L., Ciofi, P., Bloom, S. R., et al. (2013). Substance P immunoreactivity exhibits frequent colocalization with kisspeptin and neurokinin B in the human infundibular region. PLoS One 8:e72369. doi: 10.1371/journal.pone.0072369
Hrabovszky, E., Ciofi, P., Vida, B., Horvath, M. C., Keller, E., Caraty, A., et al. (2010). The kisspeptin system of the human hypothalamus: sexual dimorphism and relationship with gonadotropin-releasing hormone and neurokinin B neurons. Eur. J. Neurosci. 31, 1984–1998. doi: 10.1111/j.1460-9568.2010.07239.x
Hrabovszky, E., and Liposits, Z. (2013). Afferent neuronal control of type-I gonadotropin releasing hormone neurons in the human. Front. Endocrinol. 4:130. doi: 10.3389/fendo.2013.00130
Hrabovszky, E., Molnar, C. S., Sipos, M. T., Vida, B., Ciofi, P., Borsay, B. A., et al. (2011). Sexual dimorphism of kisspeptin and neurokinin B immunoreactive neurons in the infundibular nucleus of aged men and women. Front. Endocrinol. 2:80. doi: 10.3389/fendo.2011.00080
Hrabovszky, E., Sipos, M. T., Molnar, C. S., Ciofi, P., Borsay, B. A., Gergely, P., et al. (2012). Low degree of overlap between kisspeptin, neurokinin B, and dynorphin immunoreactivities in the infundibular nucleus of young male human subjects challenges the KNDy neuron concept. Endocrinology 153, 4978–4989. doi: 10.1210/en.2012-1545
Hrabovszky, E., Takacs, S., Gocz, B., and Skrapits, K. (2019). New perspectives for anatomical and molecular studies of kisspeptin neurons in the aging human brain. Neuroendocrinology 109, 230–241. doi: 10.1159/000496566
Hunyady, B., Krempels, K., Harta, G., and Mezey, E. (1996). Immunohistochemical signal amplification by catalyzed reporter deposition and its application in double immunostaining. J. Histochem. Cytochem. 44, 1353–1362. doi: 10.1177/44.12.8985127
Irwig, M. S., Fraley, G. S., Smith, J. T., Acohido, B. V., Popa, S. M., Cunningham, M. J., et al. (2004). Kisspeptin activation of gonadotropin releasing hormone neurons and regulation of KiSS-1 mRNA in the male rat. Neuroendocrinology 80, 264–272. doi: 10.1159/000083140
Kalil, B., Ramaswamy, S., and Plant, T. M. (2016). The distribution of substance p and kisspeptin in the mediobasal hypothalamus of the male rhesus monkey and a comparison of intravenous administration of these peptides to release GnRH as reflected by LH secretion. Neuroendocrinology 103, 711–723. doi: 10.1159/000442420
Kallo, I., Vida, B., Deli, L., Molnar, C. S., Hrabovszky, E., Caraty, A., et al. (2012). Co-localisation of kisspeptin with galanin or neurokinin B in afferents to mouse GnRH neurones. J. Neuroendocrinol. 24, 464–476. doi: 10.1111/j.1365-2826.2011.02262.x
Keen, K. L., Wegner, F. H., Bloom, S. R., Ghatei, M. A., and Terasawa, E. (2008). An increase in kisspeptin-54 release occurs with the pubertal increase in luteinizing hormone-releasing hormone-1 release in the stalk-median eminence of female rhesus monkeys in vivo. Endocrinology 149, 4151–4157. doi: 10.1210/en.2008-0231
King, J. C., and Anthony, E. L. (1984). LHRH neurons and their projections in humans and other mammals: species comparisons. Peptides 5(Suppl. 1), 195–207. doi: 10.1016/0196-9781(84)90277-8
Kinoshita, M., Tsukamura, H., Adachi, S., Matsui, H., Uenoyama, Y., Iwata, K., et al. (2005). Involvement of central metastin in the regulation of preovulatory luteinizing hormone surge and estrous cyclicity in female rats. Endocrinology 146, 4431–4436. doi: 10.1210/en.2005-0195
Kirilov, M., Clarkson, J., Liu, X., Roa, J., Campos, P., Porteous, R., et al. (2013). Dependence of fertility on kisspeptin-Gpr54 signaling at the GnRH neuron. Nat. Commun. 4:2492.
Krajewski, S. J., Burke, M. C., Anderson, M. J., Mcmullen, N. T., and Rance, N. E. (2010). Forebrain projections of arcuate neurokinin B neurons demonstrated by anterograde tract-tracing and monosodium glutamate lesions in the rat. Neuroscience 166, 680–697. doi: 10.1016/j.neuroscience.2009.12.053
Lehman, M. N., Coolen, L. M., and Goodman, R. L. (2010a). Minireview: kisspeptin/neurokinin B/dynorphin (KNDy) cells of the arcuate nucleus: a central node in the control of gonadotropin-releasing hormone secretion. Endocrinology 151, 3479–3489. doi: 10.1210/en.2010-0022
Lehman, M. N., Merkley, C. M., Coolen, L. M., and Goodman, R. L. (2010b). Anatomy of the kisspeptin neural network in mammals. Brain Res. 1364, 90–102. doi: 10.1016/j.brainres.2010.09.020
Lopez, F. J., Meade, E. H. Jr., and Negro-Vilar, A. (1990). Development and characterization of a specific and sensitive radioimmunoassay for rat galanin: measurement in brain tissue, hypophyseal portal and peripheral serum. Brain Res. Bull. 24, 395–399. doi: 10.1016/0361-9230(90)90095-h
Matsui, H., Takatsu, Y., Kumano, S., Matsumoto, H., and Ohtaki, T. (2004). Peripheral administration of metastin induces marked gonadotropin release and ovulation in the rat. Biochem. Biophys. Res. Commun. 320, 383–388. doi: 10.1016/j.bbrc.2004.05.185
Matsuyama, S., Ohkura, S., Mogi, K., Wakabayashi, Y., Mori, Y., Tsukamura, H., et al. (2011). Morphological evidence for direct interaction between kisspeptin and gonadotropin-releasing hormone neurons at the median eminence of the male goat: an immunoelectron microscopic study. Neuroendocrinology 94, 323–332. doi: 10.1159/000331576
Merchenthaler, I., Lengvari, I., Horvath, J., and Setalo, G. (1980). Immunohistochemical study of the LHRH-synthesizing neuron system of aged female rats. Cell Tissue Res. 209, 499–503.
Messager, S., Chatzidaki, E. E., Ma, D., Hendrick, A. G., Zahn, D., Dixon, J., et al. (2005). Kisspeptin directly stimulates gonadotropin-releasing hormone release via G protein-coupled receptor 54. Proc. Natl. Acad. Sci. U.S.A. 102, 1761–1766. doi: 10.1073/pnas.0409330102
Mikami, S., Ichino, K., Yamada, S., and Taniguchi, K. (1985). Immunocytochemical localization of neuropeptides in the ventral hypothalamus of the cat. Nihon Juigaku Zasshi 47, 931–942. doi: 10.1292/jvms1939.47.931
Mikula, S., Trotts, I., Stone, J. M., and Jones, E. G. (2007). Internet-enabled high-resolution brain mapping and virtual microscopy. Neuroimage 35, 9–15. doi: 10.1016/j.neuroimage.2006.11.053
Mittelman-Smith, M. A., Williams, H., Krajewski-Hall, S. J., Lai, J., Ciofi, P., Mcmullen, N. T., et al. (2012). Arcuate kisspeptin/neurokinin B/dynorphin (KNDy) neurons mediate the estrogen suppression of gonadotropin secretion and body weight. Endocrinology 153, 2800–2812. doi: 10.1210/en.2012-1045
Moenter, S. M., Karsch, F. J., and Lehman, M. N. (1993). Fos expression during the estradiol-induced gonadotropin-releasing hormone (GnRH) surge of the ewe: induction in GnRH and other neurons. Endocrinology 133, 896–903. doi: 10.1210/endo.133.2.8344224
Molnar, C. S., Vida, B., Sipos, M. T., Ciofi, P., Borsay, B. A., Racz, K., et al. (2012). Morphological evidence for enhanced kisspeptin and neurokinin B signaling in the infundibular nucleus of the aging man. Endocrinology 153, 5428–5439. doi: 10.1210/en.2012-1739
Moore, A. M., Prescott, M., Czieselsky, K., Desroziers, E., Yip, S. H., Campbell, R. E., et al. (2018). Synaptic innervation of the GnRH neuron distal dendron in female mice. Endocrinology 159, 3200–3208. doi: 10.1210/en.2018-00505
Navarro, V. M., Castellano, J. M., Fernandez-Fernandez, R., Tovar, S., Roa, J., Mayen, A., et al. (2005). Characterization of the potent luteinizing hormone-releasing activity of KiSS-1 peptide, the natural ligand of GPR54. Endocrinology 146, 156–163. doi: 10.1210/en.2004-0836
Navarro, V. M., Gottsch, M. L., Chavkin, C., Okamura, H., Clifton, D. K., and Steiner, R. A. (2009). Regulation of gonadotropin-releasing hormone secretion by kisspeptin/dynorphin/neurokinin B neurons in the arcuate nucleus of the mouse. J. Neurosci. 29, 11859–11866. doi: 10.1523/jneurosci.1569-09.2009
Navarro, V. M., Gottsch, M. L., Wu, M., Garcia-Galiano, D., Hobbs, S. J., Bosch, M. A., et al. (2011). Regulation of NKB pathways and their roles in the control of Kiss1 neurons in the arcuate nucleus of the male mouse. Endocrinology 152, 4265–4275. doi: 10.1210/en.2011-1143
Pielecka-Fortuna, J., Chu, Z., and Moenter, S. M. (2008). Kisspeptin acts directly and indirectly to increase gonadotropin-releasing hormone neuron activity and its effects are modulated by estradiol. Endocrinology 149, 1979–1986. doi: 10.1210/en.2007-1365
Plant, T. M., Ramaswamy, S., and Dipietro, M. J. (2006). Repetitive activation of hypothalamic G protein-coupled receptor 54 with intravenous pulses of kisspeptin in the juvenile monkey (Macaca mulatta) elicits a sustained train of gonadotropin-releasing hormone discharges. Endocrinology 147, 1007–1013. doi: 10.1210/en.2005-1261
Porteous, R., Petersen, S. L., Yeo, S. H., Bhattarai, J. P., Ciofi, P., De Tassigny, X. D., et al. (2011). Kisspeptin neurons co-express met-enkephalin and galanin in the rostral periventricular region of the female mouse hypothalamus. J. Comp. Neurol. 519, 3456–3469. doi: 10.1002/cne.22716
Qiu, J., Nestor, C. C., Zhang, C., Padilla, S. L., Palmiter, R. D., Kelly, M. J., et al. (2016). High-frequency stimulation-induced peptide release synchronizes arcuate kisspeptin neurons and excites GnRH neurons. eLife 5:e16246.
Ramaswamy, S., Guerriero, K. A., Gibbs, R. B., and Plant, T. M. (2008). Structural interactions between kisspeptin and GnRH neurons in the mediobasal hypothalamus of the male rhesus monkey (Macaca mulatta) as revealed by double immunofluorescence and confocal microscopy. Endocrinology 149, 4387–4395. doi: 10.1210/en.2008-0438
Ruka, K. A., Burger, L. L., and Moenter, S. M. (2013). Regulation of arcuate neurons coexpressing kisspeptin, neurokinin B, and dynorphin by modulators of neurokinin 3 and kappa-opioid receptors in adult male mice. Endocrinology 154, 2761–2771. doi: 10.1210/en.2013-1268
Rumpler, E., Skrapits, K., Takacs, S., Gocz, B., Trinh, S. H., Racz, G., et al. (2020). Characterization of kisspeptin neurons in the human rostral hypothalamus. Neuroendocrinology [Epub ahead of print].
Santoro, N., Filicori, M., and Crowley, W. F. Jr. (1986). Hypogonadotropic disorders in men and women: diagnosis and therapy with pulsatile gonadotropin-releasing hormone. Endocr. Rev. 7, 11–23. doi: 10.1210/edrv-7-1-11
Seminara, S. B., Messager, S., Chatzidaki, E. E., Thresher, R. R., Acierno, J. S. Jr., Shagoury, J. K., et al. (2003). The GPR54 gene as a regulator of puberty. N. Engl. J. Med. 349, 1614–1627.
Shahab, M., Mastronardi, C., Seminara, S. B., Crowley, W. F., Ojeda, S. R., and Plant, T. M. (2005). Increased hypothalamic GPR54 signaling: a potential mechanism for initiation of puberty in primates. Proc. Natl. Acad. Sci. U.S.A. 102, 2129–2134. doi: 10.1073/pnas.0409822102
Skrapits, K., Borsay, B. A., Herczeg, L., Ciofi, P., Bloom, S. R., Ghatei, M. A., et al. (2014). Colocalization of cocaine- and amphetamine-regulated transcript with kisspeptin and neurokinin B in the human infundibular region. PLoS One 9:e103977. doi: 10.1371/journal.pone.0103977
Skrapits, K., Borsay, B. A., Herczeg, L., Ciofi, P., Liposits, Z., and Hrabovszky, E. (2015a). Neuropeptide co-expression in hypothalamic kisspeptin neurons of laboratory animals and the human. Front. Neurosci. 9:29. doi: 10.3389/fnins.2015.00029
Skrapits, K., Kanti, V., Savanyu, Z., Maurnyi, C., Szenci, O., Horvath, A., et al. (2015b). Lateral hypothalamic orexin and melanin-concentrating hormone neurons provide direct input to gonadotropin-releasing hormone neurons in the human. Front. Cell. Neurosci. 9:348. doi: 10.3389/fncel.2015.00348
Smith, J. T., Coolen, L. M., Kriegsfeld, L. J., Sari, I. P., Jaafarzadehshirazi, M. R., Maltby, M., et al. (2008). Variation in kisspeptin and RFamide-related peptide (RFRP) expression and terminal connections to gonadotropin-releasing hormone neurons in the brain: a novel medium for seasonal breeding in the sheep. Endocrinology 149, 5770–5782. doi: 10.1210/en.2008-0581
Smith, J. T., Dungan, H. M., Stoll, E. A., Gottsch, M. L., Braun, R. E., Eacker, S. M., et al. (2005). Differential regulation of KiSS-1 mRNA expression by sex steroids in the brain of the male mouse. Endocrinology 146, 2976–2984. doi: 10.1210/en.2005-0323
Stephens, S. B. Z., and Kauffman, A. S. (2017). Regulation and possible functions of kisspeptin in the medial amygdala. Front. Endocrinol. 8:191. doi: 10.3389/fendo.2017.00191
True, C., Takahashi, D., Kirigiti, M., Lindsley, S. R., Moctezuma, C., Arik, A., et al. (2017). Arcuate nucleus neuropeptide coexpression and connections to gonadotrophin-releasing hormone neurones in the female rhesus macaque. J. Neuroendocrinol. 29:e12491.
True, C., Verma, S., Grove, K. L., and Smith, M. S. (2013). Cocaine- and amphetamine-regulated transcript is a potent stimulator of GnRH and kisspeptin cells and may contribute to negative energy balance-induced reproductive inhibition in females. Endocrinology 154, 2821–2832. doi: 10.1210/en.2013-1156
Vrang, N., Larsen, P. J., Clausen, J. T., and Kristensen, P. (1999). Neurochemical characterization of hypothalamic cocaine- amphetamine-regulated transcript neurons. J. Neurosci. 19:RC5.
Wakabayashi, Y., Nakada, T., Murata, K., Ohkura, S., Mogi, K., Navarro, V. M., et al. (2010). Neurokinin B and dynorphin A in kisspeptin neurons of the arcuate nucleus participate in generation of periodic oscillation of neural activity driving pulsatile gonadotropin-releasing hormone secretion in the goat. J. Neurosci. 30, 3124–3132. doi: 10.1523/jneurosci.5848-09.2010
Keywords: arcuate nucleus, carnivores, hypothalamus, KNDy neurons, neurokinin B, neuropeptides, reproduction
Citation: Rumpler É, Takács S, Göcz B, Baska F, Szenci O, Horváth A, Ciofi P, Hrabovszky E and Skrapits K (2020) Kisspeptin Neurons in the Infundibular Nucleus of Ovariectomized Cats and Dogs Exhibit Unique Anatomical and Neurochemical Characteristics. Front. Neurosci. 14:598707. doi: 10.3389/fnins.2020.598707
Received: 25 August 2020; Accepted: 09 November 2020;
Published: 02 December 2020.
Edited by:
Jacques Epelbaum, Institut National de la Santé et de la Recherche Médicale (INSERM), FranceReviewed by:
Allan Herbison, University of Cambridge, United KingdomAleisha Marie Moore, Kent State University, United States
Copyright © 2020 Rumpler, Takács, Göcz, Baska, Szenci, Horváth, Ciofi, Hrabovszky and Skrapits. This is an open-access article distributed under the terms of the Creative Commons Attribution License (CC BY). The use, distribution or reproduction in other forums is permitted, provided the original author(s) and the copyright owner(s) are credited and that the original publication in this journal is cited, in accordance with accepted academic practice. No use, distribution or reproduction is permitted which does not comply with these terms.
*Correspondence: Katalin Skrapits, skrapits.katalin@koki.hu; Erik Hrabovszky, hrabovszky.erik@koki.hu