- 1Department of Biochemistry, College of Medicine, Konyang University, Daejeon, South Korea
- 2Department of Neural Development and Disease, Korea Brain Research Institute (KBRI), Daegu, South Korea
- 3Department of Nursing, College of Nursing, Jeju National University, Jeju-si, South Korea
Although the major causative factors of Alzheimer’s disease (AD) are the accumulation of amyloid β and hyperphosphorylated tau, AD can also be caused by metabolic dysfunction. The major clinical symptom of AD is cognitive dysfunction. However, AD is also accompanied by various secondary symptoms such as depression, sleep–wake disturbances, and abnormal eating behaviors. Interestingly, the orexigenic hormone ghrelin has been suggested to have beneficial effects on AD-related metabolic syndrome and secondary symptoms. Ghrelin improves lipid distribution and alters insulin sensitivity, effects that are hypothesized to delay the progression of AD. Furthermore, ghrelin can relieve depression by enhancing the secretion of hormones such as serotonin, noradrenaline, and orexin. Moreover, ghrelin can upregulate the expression of neurotrophic factors such as brain-derived neurotrophic factor and modulate the release of proinflammatory cytokines such as tumor necrosis factor α and interleukin 1β. Ghrelin alleviates sleep–wake disturbances by increasing the levels of melatonin, melanin-concentrating hormone. Ghrelin reduces the risk of abnormal eating behaviors by increasing neuropeptide Y and γ-aminobutyric acid. In addition, ghrelin increases food intake by inhibiting fatty acid biosynthesis. However, despite the numerous studies on the role of ghrelin in the AD-related pathology and metabolic disorders, there are only a few studies that investigate the effects of ghrelin on secondary symptoms associated with AD. In this mini review, our purpose is to provide the insights of future study by organizing the previous studies for the role of ghrelin in AD-related pathology and metabolic disorders.
Introduction
Alzheimer’s disease (AD), characterized histopathologically by amyloid β aggregation and tau hyperphosphorylation, is the most common cause of dementia (Querfurth and LaFerla, 2010). Although AD is clinically characterized by progressive impairment of cognitive functions such as episodic memory, it is also accompanied by secondary symptoms such as depression, sleep–wake disturbances, and abnormal eating behaviors. Notably, some AD patients exhibit symptoms of major depressive episodes such as appetite changes, insomnia, and dysphoria (Merriam et al., 1988; Novais and Starkstein, 2015; Okuda et al., 2019). In addition, subjects with mild cognitive or behavioral impairment are more likely to experience accelerated progression to AD or onset of dementia if they have a history of depression (Wilson et al., 2002). Furthermore, metabolic syndrome such as hyperglycemia, hyperinsulinemia, and hypercholesterolemia is known to be the risk factor for AD (Lane and Farlow, 2005; Nelson and Alkon, 2005; Razay et al., 2007). Psychiatric and metabolic deficits are not only symptoms of AD, but also markers of AD prognosis. Although there are drugs that effectively delay AD-related cognitive impairment, thus far, no therapeutic strategy has been established to treat the psychiatric and metabolic symptoms of AD thus far.
Ghrelin is an orexigenic hormone which regulates body weight, energy homeostasis, and metabolism through the hypothalamus, and plays an enhancing role in insulin resistance and growth hormone secretion (Pradhan et al., 2013; Muller et al., 2015; Yanagi et al., 2018). Remarkably, extensive evidence has indicated that ghrelin may alleviate AD-related pathology such as Aβ accumulation (Dhurandhar et al., 2013; Jeong et al., 2018), tau hyperphosphorylation (Kang et al., 2015), mitochondrial dysfunction (Chung et al., 2007), impaired adult neurogenesis (Moon et al., 2014), and neuroinflammation (Moon et al., 2011; Sibilia et al., 2012). Therefore, due to its potential for mitigating AD-related pathologies, ghrelin could be a possible therapeutic target for AD (Jeon et al., 2019). In addition, several studies have reported that ghrelin plays a protective role in metabolic syndrome (Broglio et al., 2004) and various psychiatric disorders, including depression (Carlini et al., 2012), sleep–wake disturbances (Yannielli et al., 2007), and abnormal eating behaviors (Overduin et al., 2012). However, the possible roles of ghrelin in AD-related metabolic syndrome and psychiatric disorders have not yet been investigated. Furthermore, although ghrelin plays a pivotal role in energy metabolism and homeostasis (Yanagi et al., 2018), the effects of ghrelin on metabolic disorders and secondary symptoms of AD remain unclear. In this review, we discuss the possibility of using ghrelin as a therapeutic target for AD by presenting evidence for the potential roles of ghrelin in the metabolic symptoms and secondary symptoms associated with AD.
The Role of Ghrelin in Metabolic Syndrome and Secondary Symptoms of AD
The Role of Ghrelin in AD-Related Metabolic Syndrome
Alzheimer disease is considered to be another type of diabetes, and hyperinsulinemia and hypercholesterolemia are known to promote AD pathogenesis (de La Monte and Wands, 2008; Merlo et al., 2010). Hyperinsulinemia inhibits the activity of AMP-activated protein kinase (AMPK) (Valentine et al., 2014), and inhibition of AMPK activity as a result of metabolic syndrome inactivates the pentose phosphate pathway (Saito et al., 2015). Abnormal metabolic conditions including diabetes mellitus may induce impairment of energy metabolism by increasing the production of reactive oxygen species and mitochondrial dysfunction (Bonomini et al., 2015; Bhatti et al., 2017) and may accelerate cognitive impairment by promoting abnormal release of neurotransmitters, particularly γ-aminobutyric acid (GABA) (van Bussel et al., 2016). Several studies have suggested that in neurodegenerative diseases, there exists a link between insulin and cholesterol levels (Laws et al., 1991; Nelson and Alkon, 2005). Indeed, insulin increases the activity of 3-hydroxy-3-methylglutaryl-CoA reductase, the enzyme that catalyzes an intermediate in cholesterol synthesis (Nelson and Alkon, 2005). In a previous study, individuals with type 2 diabetes mellitus exhibited decreased cholesterol absorption and increased cholesterol synthesis regardless of obesity (Simonen et al., 2002). In the case of AD, Aβ-induced metabolic imbalance involving AMPK results in tau phosphorylation and neuroinflammation (Martinez de Morentin et al., 2010; Thornton et al., 2011; Lee et al., 2013). Furthermore, AD patients suffer from insulin signaling dysfunction due to a reduction in activity of tyrosine kinase, an important effector system for insulin receptors (Frolich et al., 1999), and decreased activities of elements of insulin–PI3K–AKT signaling, which results in elevated tau phosphorylation and decreased glucose metabolism (Liu et al., 2011). In particular, apolipoprotein E (ApoE), a protein responsible for the metabolism of plasma lipids (Jones et al., 2019), is also associated with AD (Lane and Farlow, 2005). Reportedly, polymorphism of ApoE allele, especially ApoE ε4, attributes to risk of AD development by increasing Aβ and Tau aggregation, whereas ApoE ε2 exhibits protective effects on risk of AD development (Verghese et al., 2011). Moreover, ApoE ε4 induces dysregulation of cerebral metabolism by decreasing lipid and glucose metabolism (Brandon et al., 2018). Interestingly, the control of insulin and plasma glucose by ghrelin administration can vary depending on the details of administration (i.e., duration, route, and dose) (Nieminen and Mustonen, 2004; Theander-Carrillo et al., 2006; Barazzoni et al., 2007a; Goshadrou et al., 2015). In rats, acute (1 day) administration of ghrelin increased levels of insulin and fasting plasma glucose, but chronic (21 days) administration of ghrelin normalized these upregulations (Goshadrou et al., 2015). The mechanism of insulin and glucose regulation after administration of exogenous ghrelin has not yet been clearly identified. Known mechanisms through which insulin inhibits ghrelin include upregulation of the AMPK- uncoupling protein 2 (UCP2) pathway through AMPK phosphorylation and UCP2 expression (Chmielewska et al., 2010; Wang et al., 2010), and the IA-2β pathway, which inhibits glucose-stimulated insulin through induction of IA-2β (Doi et al., 2006). These two pathways independently inhibit insulin. Remarkably, ghrelin not only regulates insulin but also regulates nigrostriatal dopamine function in a UCP2-dependent manner (Andrews et al., 2009). In addition, upregulation of UCP2 has been demonstrated to have a protective effect in animal models of ischemic stroke and Parkinson disease (Andrews et al., 2009; Liu et al., 2009).
The concentration of ghrelin is decreased in the middle-aged and elderly people with metabolic syndrome compared to individuals of the same age who do not have metabolic syndrome, and its concentration rapidly is decreased as metabolic abnormalities intensify (Ukkola et al., 2006; Serra-Prat et al., 2009; Mora et al., 2014). Several studies have suggested that ghrelin may be involved in the metabolism of insulin and glucose. In healthy subjects, administration of acyl-ghrelin reduced insulin levels and increased glucose levels (Broglio et al., 2004). By contrast, administration of des-acyl-ghrelin improved glucose metabolism and insulin sensitivity in subjects (Benso et al., 2012). In addition, administration of acyl-ghrelin alone to growth hormone–deficient patients increases insulin and glucose levels rapidly but decreases insulin sensitivity, whereas administration of acyl-ghrelin and des-acyl-ghrelin increases insulin sensitivity (Gauna et al., 2004). Moreover, transgenic mice overexpressing des-acyl-ghrelin exhibited a reduction in white adipose tissue weight and improvement in glucose tolerance and insulin sensitivity (Zhang et al., 2008). In a previous study, obese children with metabolic syndrome exhibited decreased levels of des-acyl-ghrelin and an increased acyl-ghrelin/des-acyl-ghrelin ratio compared to obese children without metabolic syndrome (Pacifico et al., 2009). Similarly, obese individuals with normoglycemia and type 2 diabetes mellitus exhibited increased plasma levels of acyl-ghrelin and decreased levels of des-acyl-ghrelin compared to lean individuals (Rodriguez et al., 2009). Therefore, individuals with metabolic syndrome and obesity have a higher acyl-ghrelin/des-acyl-ghrelin ratio than non-obese individuals with metabolic syndrome, suggesting that excessive acyl-ghrelin levels may promote insulin resistance (Barazzoni et al., 2007b). Moreover, administration of ghrelin causes tissue-specific changes in the activity of mitochondrial oxidative enzyme, the expression of gene involved in lipid metabolism, and triglyceride content in rats, suggesting that ghrelin may be involved in the regulation of lipid distribution and metabolism (Barazzoni et al., 2005).
Patients with AD exhibited lower lean mass compared to controls. Although patients with AD and controls exhibited similar basal levels of ghrelin, the area under the curve value was lower in male patients with AD than in control males (Theodoropoulou et al., 2012). Although further evidence and investigation are required, a previous study by Yoshino et al. (2018) showed increased levels of serum acyl-ghrelin in AD subjects compared to control subjects that might be a result of changes of the ghrelin pathway in brain (Yoshino et al., 2018). Thus, further deliberate examination and interpretation should be made. Given that ghrelin-O-acyltransferase blockade reduces the acyl-ghrelin/des-acyl-ghrelin ratio, des-acyl-ghrelin administration could be a promising therapeutic approach for metabolic dysfunction (Barnett et al., 2010). It is possible that the increased acyl-ghrelin/des-acyl-ghrelin ratio in individuals with obesity may promote insulin resistance and hyperinsulinemia (Barazzoni et al., 2007b). Insulin resistance and hyperinsulinism may increase the prevalence of AD by increasing Aβ-related metabolism and inflammation in the brain (Craft, 2007). Additionally, insulin transport to the brain is reduced, causing insulin deficiency (Baura et al., 1996). Furthermore, neurofibrillary tangles containing phosphorylated tau were observed in the hippocampus of insulin receptor substrate 2 knockout mice, indicating that insulinlike growth factor-1 and insulin are associated with tau phosphorylation (Schubert et al., 2003). These results suggest that metabolic abnormalities such as hyperinsulinemia and insulin resistance promote AD development (DiStefano et al., 2007). Both in vitro and in vivo studies have reported that an optimal concentration of insulin reduced Aβ production through increasing the levels of α-secretase ADAM10, sAPPα, and C83 and decreasing the levels of β-secretase BACE1, sAPPβ, and C99 (Vandal et al., 2014; Wang et al., 2014). Furthermore, antidiabetic drugs such as metformin and peroxisome proliferator-activated receptor-γ agonists may have beneficial effects on preventing or improving cognitive dysfunction and pathogenesis of AD (Crisby et al., 2002; Cong et al., 2010; Akter et al., 2011). Therefore, given that ghrelin plays major roles in metabolism, it may be a noteworthy therapeutic target for AD (Gahete et al., 2011; Eslami et al., 2018). Nonetheless, considering the fact that the area under the curve value of ghrelin was increased by glucose loading only in male patients with AD, not in female patients (Theodoropoulou et al., 2012), and the higher basal ghrelin levels in female healthy and opposite-sex twin pair subjects than men (Makovey et al., 2007; Song et al., 2017), difference in effects of ghrelin for AD-related metabolic syndrome according to gender should be examined in the future.
The Role of Ghrelin in AD-Related Depression
Depression is the most common secondary symptom in patients with AD and is associated with accelerated cognitive impairment (Bassuk et al., 1998; Modrego, 2010). In particular, late-onset depression is considered to be a risk factor for AD development and is more strongly associated with cognitive decline than early-onset depression (Devanand et al., 1996; van Reekum et al., 1999; Wilson et al., 2002). An increase in glucocorticoid production is characteristic of early AD (Rasmuson et al., 2001), and hypothalamic–pituitary–adrenal (HPA) axis dysfunction caused by excessive glucocorticoid secretion and reactivity promotes the development of depression (Zunszain et al., 2011). In addition, the limbic lobe, hippocampus, amygdala, and anterior and posterior cingulate cortices are involved in the pathophysiology of depression; a decrease in the density/structural plasticity of these areas has been identified in patients with depression (Rajkowska, 2000; Nestler et al., 2002; Ries et al., 2009) and in patients with early AD (Braak et al., 1993; Minoshima et al., 1997; Gastard et al., 2003; Poulin et al., 2011). Moreover, dysfunction of the monoaminergic system, in particular the serotonergic and noradrenergic systems, has been shown to occur in both depression and AD (Ressler and Nemeroff, 2000; Versijpt et al., 2003; Kepe et al., 2006; Chalermpalanupap et al., 2013).
Chronic stress–induced glucocorticoid upregulation promotes neuronal damage, induces structural changes, and decreases the expression of brain-derived neurotrophin-3 and neurotrophic factor (BDNF) mRNA in the hippocampus (Smith et al., 1995; Nestler et al., 2002). Ghrelin, which has a protective effect on metabolic disturbances induced by chronic stress, has been reported to also have protective effects against depressive-like responses in experimental animals (Lutter et al., 2008; Labarthe et al., 2014). In addition, the rat model of diabetes exhibits lower hippocampal BDNF mRNA levels compared to control rats, while administration of ghrelin significantly upregulates BDNF mRNA levels in a rat model of diabetes (Ma et al., 2011). Olfactory bulbectomy induced depressive-like behavior in mice, and this deficit was reversed by ghrelin administration, indicating that ghrelin exhibits an antidepressant-like effect (Carlini et al., 2012). Moreover, olfactory bulbectomy decreased noradrenaline levels and serotonin turnover and increased the levels of proinflammatory cytokines such as interleukin 1β (IL-1β) and tumor necrosis factor α (TNF-α) (Hellweg et al., 2007; Song et al., 2009; Yang et al., 2014; Chang et al., 2016). However, exogenous ghrelin inhibited the release of proinflammatory cytokines and increased noradrenaline levels and serotonin turnover, further demonstrating the antidepressant-like effect of ghrelin (Date et al., 2006; Kawakami et al., 2008; Waseem et al., 2008; Hansson et al., 2014). Moreover, increased ghrelin levels induced by calorie restriction led to anti–depressant-like effects. By contrast, the calorie restriction–induced anti–depressive-like effects were not observed in growth hormone secretagogue receptor (GHS-R) null mice, and these animals exhibited increased social avoidance compared to their wild-type littermates (Lutter et al., 2008). Notably, GHS-R1 is known to be involved in various psychological conditions, including depression (Guo et al., 2019). Thus, ghrelin may alleviate depressive-like responses by acting on GHS-R1–expressing neurons (Abizaid et al., 2006; Diano et al., 2006; Lutter et al., 2008).
Mechanisms related to the pathogenesis of depression include HPA axis dysfunction, monoaminergic system deficiency, inflammation, and neurodegeneration (Zunszain et al., 2011). Therefore, ghrelin may alleviate depressive symptoms by upregulating BDNF mRNA, decreasing glucocorticoid levels, rebalancing the monoaminergic system, stimulating GHS-R1–expressing neurons to modulate mood and synapse formation, and regulating the release of proinflammatory cytokines such as IL-1β and TNF-α. Unfortunately, few studies have investigated the role of ghrelin in AD-related depression. However, given the antidepressant-like effect of ghrelin observed in previous animal studies, we hypothesize that ghrelin may have a therapeutic effect on depression in AD patients.
Other neuropeptides, including neurotensin and neuropeptide Y (NPY), have been shown to be involved in the pathogenesis of depression. Interestingly, the effects of neurotensin were opposite to those of ghrelin on food intake (Cooke et al., 2009). In addition, neurotensin neurons are known to play important roles in regulation of energy balance controlled by ghrelin and leptin (Brown et al., 2017). Notably, mRNA levels of ghrelin and expression of its G protein–coupled receptors (neurotensin receptors 1 and 2) are decreased, whereas levels of neurotensin tend to decrease in the temporal lobe of patients with AD (Gahete et al., 2010). In another study, density of amyloid plaque in the occipital cortex was negatively correlated with density of neurotensin neurons in postmortem suprachiasmatic nucleus (SCN) (Hu et al., 2013). Moreover, neurotensin receptor 1 knockout mice showed increased depressive-like behaviors in the tail suspension test (Fitzpatrick et al., 2012). Despite the conflicting results from clinical studies examining the roles of NPY in depression, evidence strongly supports the involvement of NPY in pathogenesis of depression (Morales-Medina et al., 2010). In addition, levels of NPY vary by the locations of sampling and models of AD (Duarte-Neves et al., 2016). Considering, ghrelin cross talks with NPY neurons in the arcuate nucleus (ARC) in rats (Kohno et al., 2003) and the evidence that ghrelin increases gene expression of NPY in the ARC in hypothalamic cultures of rats (Goto et al., 2006), the regulatory effect of ghrelin on NPY in AD-related depression should be examined in the future. Although the interacting mechanisms among ghrelin, neurotensin, and NPY in AD-related depression remain to be examined, neurotensin and NPY, at least, seem to be mediating some AD-related depression-like behaviors by interacting with ghrelin.
The Role of Ghrelin in AD-Related Sleep–Wake Disturbances
Maintaining a normal circadian rhythm is essential in order to optimize quality of life and preserve health. Sleep–wake disturbances are common secondary symptoms of AD that have been observed in studies on patients with AD (Uddin et al., 2020) and on the 3×Tg and 5×FAD mouse models of AD (Sterniczuk et al., 2010; Sethi et al., 2015). Moreover, the pineal gland, which adjusts sleep patterns by producing melatonin, and the SCN, which is involved in the regulation and production of biological rhythms, are vulnerable regions in AD (Buijs and Kalsbeek, 2001; Wu and Swaab, 2005; Roy et al., 2019). A recent study using magnetic resonance imaging of the brain showed that the pineal volume was decreased in mild cognitive impairment (MCI) patients who converted to AD than in MCI patients who did not convert to AD (Matsuoka et al., 2020). Furthermore, sleep–wake cycle disturbances showed to increase Aβ plaques in the brain of AD mouse models (Kang et al., 2009; Rothman et al., 2013). The level of Aβ42 protein in cerebrospinal fluid of healthy middle-aged individuals was increased in the sleep deprivation group compared to that in the unrestricted sleep group (Ooms et al., 2014). In particular, sundowning, a common symptom of AD with circadian rhythm disruption, occurs in the afternoon and evening and is accompanied by seven destructive actions: combativeness, agitation or purposeless movement, wandering, prolonged incoherent vocalization, hallucinations, confusion, and disorientation (Gallagher-Thompson et al., 1992; Volicer et al., 2001). Regulations of sleep and brain functions are related to regulatory pathways including hippocampal signaling pathway and common neurotransmitter systems such as orexinergic and GABAergic systems (Prince and Abel, 2013). However, dysfunction of sleep function destabilizes physiology, disturbs sleep–wake timing, and promotes other pathological symptoms such as cognitive and metabolic deficits (Wulff et al., 2010). Surprisingly, the orexigenic peptide ghrelin regulates circadian rhythm (Yannielli et al., 2007; LeSauter et al., 2009; Steiger et al., 2011). Studies have been shown that administration of ghrelin decreased REM sleep and increased slow wave sleep in elderly men (Kluge et al., 2010) and promoted non-REM sleep in male mice (Obal et al., 2003). Several studies have been reported that GHS-R1 mRNA is highly expressed in the SCN (Zigman et al., 2006) and ARC (Jeon et al., 2019). It is well known that neurons in the SCN are projected to the dorsal parvocellular paraventricular nucleus (PVHd), and neurons in the PVHd are projected to sympathetic preganglionic neurons, which in turn regulate melatonin secretion by the pineal gland (Saper et al., 2005). Therefore, ghrelin could alleviate sleep–wake disturbances through increasing melatonin secretion by binding to GHS-R1 in the SCN and enhancing the regulatory pathways that stimulate the pineal gland. Additionally, the ARC neurons innervate to the ventrolateral preoptic nucleus (VLPO) and lateral hypothalamus (LH) via the dorsomedial hypothalamus. The VLPO is involved in sleep, and the LH is associated with wakefulness by regulating melanin-concentrating hormone (Saper et al., 2005). Thus, ghrelin could enhance sleep–wake cycle by stimulating the VLPO and LH through binding to GHS-R1 in the ARC. Moreover, ghrelin affects circadian locomotor output cycles kaput (CLOCK)–dependent functions (Garaulet et al., 2011). Taken together, these data indicate that ghrelin may alleviate sleep-wake disturbances by stimulating the SCN and ARC and ultimately regulate the function of CLOCK-related activity.
Areas such as the SCN, ARC, and pineal gland that influence the regulation and production of biological rhythms are damaged in patients and mouse models of AD, and these damaged regions cause sleep–wake disturbances (Do et al., 2018; Roy et al., 2019; Matsuoka et al., 2020). In addition, sleep–wake disturbances increase level of Aβ protein and plaques in healthy individuals and mouse models of AD (Kang et al., 2009; Rothman et al., 2013). Accumulating evidence has demonstrated that ghrelin not only has beneficial effects on sleep–wake cycle, but also stimulates areas involved in biological rhythms (Yannielli et al., 2007; LeSauter et al., 2009; Steiger et al., 2011). Because there is almost no study on the effects of ghrelin in AD-related sleep–wake disorders, further well-controlled clinical trials regarding the positive effects of ghrelin on disruption in the circadian rhythm and quality of life in patients with AD are needed.
The Role of Ghrelin in AD-Related Abnormal Eating Behaviors
In a previous clinical study, patients with AD exhibited weight loss (Barrett-Connor et al., 1998). Aging causes changes in appetite and growth hormone secretion (Creyghton et al., 2004), and these changes are referred to as “anorexia of aging.” Anorexia of aging causes several sequelae such as undernutrition, frailty, and sarcopenia (Cox et al., 2019). In addition, aging increases insulin resistance and reduces glucose metabolism (Shou et al., 2020). Insulin levels increase with age, and insulin may promote the development of anorexia. Moreover, aging-related leptin and ghrelin resistance may be related to anorexia of aging (Chapman et al., 2002; Chapman, 2004, 2007; Di Francesco et al., 2007).
Ghrelin is known to increase food intake (Tschop et al., 2000; Wren et al., 2000; Wren et al., 2001) and promote gastric emptying (Inui et al., 2004; Overduin et al., 2012). In particular, ghrelin regulates fatty acid metabolism in the ventromedial nuclei of the hypothalamus (VMH) to regulate food intake. The orexigenic effect of ghrelin is mediated via the phosphorylation of hypothalamic AMPK, which decreases malonyl-CoA levels and increases carnitine palmitoyltransferase-1 activity (Lopez et al., 2008). In the hypothalamic ARC, agouti-related protein and NPY are expressed in orexigenic neurons, and proopiomelanocortin (POMC) and amphetamine- and cocaine-regulated transcript are expressed in anorexigenic neurons (Zheng et al., 2003; Chen et al., 2004). In a previous study, ghrelin suppressed the activity of POMC-expressing neurons in the ARC by activating NPY-expressing neurons, which promoted the release of GABA (Cowley et al., 2003). Moreover, ghrelin reduced malonyl-CoA levels by suppressing the expression of fatty acid synthase in the VMH (Lopez et al., 2008). Indeed, intracerebroventricular infusion of ghrelin stimulated food intake via a mechanism involving the dopamine D1 receptor in rats (Overduin et al., 2012). In addition, ghrelin administration stimulated cerebral responses to food in the amygdala, anterior insula, orbitofrontal cortex, and striatum of healthy subjects (Malik et al., 2008). Interestingly, rivastigmine administration increased appetite by increasing acyl-ghrelin/des-acyl-ghrelin ratio in AD patients (Furiya et al., 2018) implying AD-related cachexia could potentially be alleviated by promoting appetite through ghrelin administration. Thus, the orexigenic effect of ghrelin may prevent the loss of body weight and lean mass in AD patients.
Conclusion
Taken together, there has been a lack of evidence demonstrating that ghrelin can alleviate metabolic syndrome and secondary symptoms associated with AD. However, it has been suggested that ghrelin may affect the progression of AD by alleviating metabolic syndrome. Moreover, it is thought that ghrelin may control secondary symptoms of AD such as depression, sleep–wake disturbances, and abnormal eating behaviors (Table 1 and Figure 1). Given the evidence for the involvement of ghrelin at various stages of AD progression, it is necessary to further examine the role of ghrelin in metabolic syndrome and in the secondary symptoms of AD.
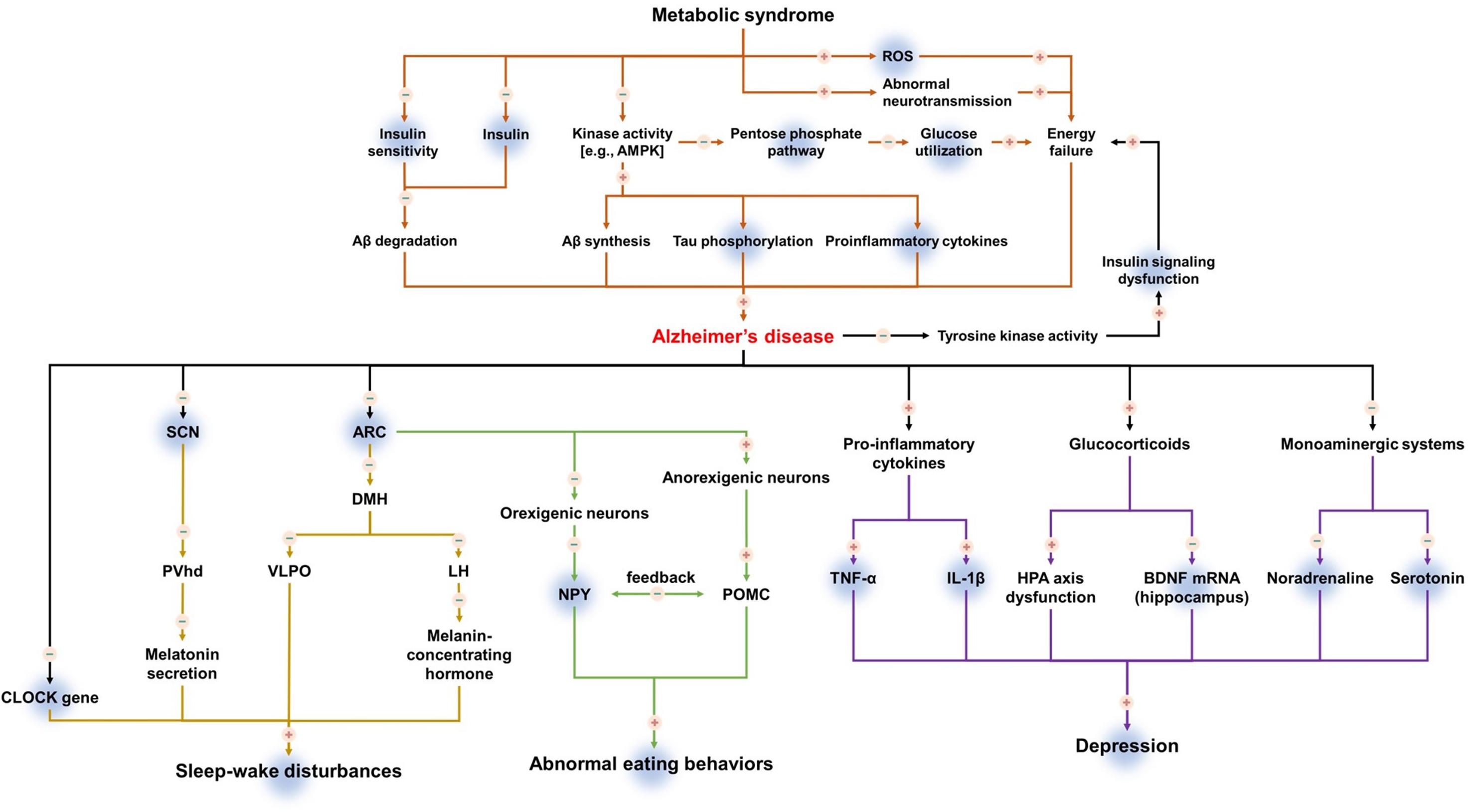
Figure 1. The mechanism of action of ghrelin in metabolic syndrome and secondary symptoms of Alzheimer’s disease. Upregulation is indicated by plus (+), downregulation is indicated by minus (–). Shadowed background color indicates the beneficial effects of ghrelin on metabolic syndrome and secondary symptoms of Alzheimer’s disease.
Author Contributions
SK, YN, SJS, YHP, SGJ, J-iK, and MM wrote this review article. SK, YN, SJS, YHP, SGJ, J-iK, M-JK, and MM revised this review article. All authors approved the submitted version.
Funding
This work was supported by the Basic Science Research Program of the National Research Foundation of Korea (NRF), which is funded by the Ministry of Science, ICT and Future Planning (NRF-2018R1D1A3B07041059) as well as by the Cooperative Research Program for Agriculture Science and Technology Development (project nos. PJ01319901 and PJ01428603), Rural Development Administration, South Korea.
Conflict of Interest
The authors declare that the research was conducted in the absence of any commercial or financial relationships that could be construed as a potential conflict of interest.
Abbreviations
AMPK, AMP-activated protein kinase; ARC, arcuate nucleus; BDNF, brain-derived neurotrophic factor; GABA, γ-aminobutyric acid; LHA, lateral hypothalamus; NPY, neuropeptide Y; POMC, proopiomelanocortin; PVhd, dorsal parvocellular paraventricular nucleus; ROS, reactive oxygen species; SCN, suprachiasmatic nucleus; VLPO, ventrolateral preoptic nucleus.
References
Abizaid, A., Liu, Z. W., Andrews, Z. B., Shanabrough, M., Borok, E., Elsworth, J. D., et al. (2006). Ghrelin modulates the activity and synaptic input organization of midbrain dopamine neurons while promoting appetite. J. Clin. Invest. 116, 3229–3239. doi: 10.1172/jci29867
Akter, K., Lanza, E. A., Martin, S. A., Myronyuk, N., Rua, M., and Raffa, R. B. (2011). Diabetes mellitus and Alzheimer’s disease: shared pathology and treatment? Br. J. Clin. Pharmacol. 71, 365–376. doi: 10.1111/j.1365-2125.2010.03830.x
Andrews, Z. B., Erion, D., Beiler, R., Liu, Z. W., Abizaid, A., Zigman, J., et al. (2009). Ghrelin promotes and protects nigrostriatal dopamine function via a UCP2-dependent mitochondrial mechanism. J. Neurosci. 29, 14057–14065. doi: 10.1523/jneurosci.3890-09.2009
Asakawa, A., Inui, A., Fujimiya, M., Sakamaki, R., Shinfuku, N., Ueta, Y., et al. (2005). Stomach regulates energy balance via acylated ghrelin and desacyl ghrelin. Gut 54, 18–24. doi: 10.1136/gut.2004.038737
Barazzoni, R., Bosutti, A., Stebel, M., Cattin, M. R., Roder, E., Visintin, L., et al. (2005). Ghrelin regulates mitochondrial-lipid metabolism gene expression and tissue fat distribution in liver and skeletal muscle. Am. J. Physiol. Endocrinol. Metab. 288, E228–E235.
Barazzoni, R., Zanetti, M., Cattin, M. R., Visintin, L., Vinci, P., Cattin, L., et al. (2007a). Ghrelin enhances in vivo skeletal muscle but not liver AKT signaling in rats. Obesity 15, 2614–2623. doi: 10.1038/oby.2007.313
Barazzoni, R., Zanetti, M., Ferreira, C., Vinci, P., Pirulli, A., Mucci, M., et al. (2007b). Relationships between desacylated and acylated ghrelin and insulin sensitivity in the metabolic syndrome. J. Clin. Endocrinol. Metab. 92, 3935–3940. doi: 10.1210/jc.2006-2527
Barnett, B. P., Hwang, Y., Taylor, M. S., Kirchner, H., Pfluger, P. T., Bernard, V., et al. (2010). Glucose and weight control in mice with a designed ghrelin O-acyltransferase inhibitor. Science 330, 1689–1692. doi: 10.1126/science.1196154
Barrett-Connor, E., Edelstein, S., Corey-Bloom, J., and Wiederholt, W. (1998). Weight loss precedes dementia in community-dwelling older adults. J. Nutr. Health Aging 2, 113–114.
Bassuk, S. S., Berkman, L. F., and Wypij, D. (1998). Depressive symptomatology and incident cognitive decline in an elderly community sample. Arch. Gen. Psychiatry 55, 1073–1081.
Baura, G. D., Foster, D. M., Kaiyala, K., Porte, D. Jr., Kahn, S. E., Schwartz, M. W., et al. (1996). Insulin transport from plasma into the central nervous system is inhibited by dexamethasone in dogs. Diabetes 45, 86–90. doi: 10.2337/diab.45.1.86
Benso, A., St-Pierre, D. H., Prodam, F., Gramaglia, E., Granata, R., Van Der Lely, A. J., et al. (2012). Metabolic effects of overnight continuous infusion of unacylated ghrelin in humans. Eur. J. Endocrinol. 166, 911–916. doi: 10.1530/eje-11-0982
Bhatti, J. S., Bhatti, G. K., and Reddy, P. H. (2017). Mitochondrial dysfunction and oxidative stress in metabolic disorders — A step towards mitochondria based therapeutic strategies. Biochim. Biophys. Acta Mol. Basis Dis. 1863, 1066–1077. doi: 10.1016/j.bbadis.2016.11.010
Bonomini, F., Rodella, F. L., and Rezzani, R. (2015). Metabolic syndrome, aging and involvement of oxidative stress. Aging Dis. 6, 109–120. doi: 10.14336/ad.2014.0305
Braak, H., Braak, E., and Bohl, J. (1993). Staging of Alzheimer-related cortical destruction. Eur. Neurol. 33, 403–408. doi: 10.1159/000116984
Brandon, J. A., Farmer, B. C., Williams, H. C., and Johnson, L. A. (2018). APOE and Alzheimer’s disease: neuroimaging of metabolic and cerebrovascular dysfunction. Front. Aging Neurosci. 10:180. doi: 10.3389/fnagi.2018.00180
Broglio, F., Gottero, C., Prodam, F., Gauna, C., Muccioli, G., Papotti, M., et al. (2004). Non-acylated ghrelin counteracts the metabolic but not the neuroendocrine response to acylated ghrelin in humans. J. Clin. Endocrinol. Metab. 89, 3062–3065. doi: 10.1210/jc.2003-031964
Brown, J. A., Bugescu, R., Mayer, T. A., Gata-Garcia, A., Kurt, G., Woodworth, H. L., et al. (2017). Loss of action via neurotensin-leptin receptor neurons disrupts leptin and ghrelin-mediated control of energy balance. Endocrinology 158, 1271–1288. doi: 10.1210/en.2017-00122
Buijs, R. M., and Kalsbeek, A. (2001). Hypothalamic integration of central and peripheral clocks. Nat. Rev. Neurosci. 2, 521–526. doi: 10.1038/35081582
Carlini, V. P., Machado, D. G., Buteler, F., Ghersi, M., Ponzio, M. F., Martini, A. C., et al. (2012). Acute ghrelin administration reverses depressive-like behavior induced by bilateral olfactory bulbectomy in mice. Peptides 35, 160–165. doi: 10.1016/j.peptides.2012.03.031
Chalermpalanupap, T., Kinkead, B., Hu, W. T., Kummer, M. P., Hammerschmidt, T., Heneka, M. T., et al. (2013). Targeting norepinephrine in mild cognitive impairment and Alzheimer’s disease. Alzheimers Res. Ther. 5:21.
Chang, X.-R., Wang, L., Li, J., and Wu, D.-S. (2016). Analysis of anti-depressant potential of curcumin against depression induced male albino wistar rats. Brain Res. 1642, 219–225. doi: 10.1016/j.brainres.2016.03.010
Chapman, I. M. (2004). Endocrinology of anorexia of ageing. Best Pract. Res. Clin. Endocrinol. Metab. 18, 437–452. doi: 10.1016/j.beem.2004.02.004
Chapman, I. M., Macintosh, C. G., Morley, J. E., and Horowitz, M. (2002). The anorexia of ageing. Biogerontology 3, 67–71. doi: 10.1016/0047-6374(94)90047-7
Chen, H. Y., Trumbauer, M. E., Chen, A. S., Weingarth, D. T., Adams, J. R., Frazier, E. G., et al. (2004). Orexigenic action of peripheral ghrelin is mediated by neuropeptide Y and agouti-related protein. Endocrinology 145, 2607–2612. doi: 10.1210/en.2003-1596
Chmielewska, J., Szczepankiewicz, D., Skrzypski, M., Kregielska, D., Strowski, M. Z., and Nowak, K. W. (2010). Ghrelin but not obestatin regulates insulin secretion from INS1 beta cell line via UCP2-dependent mechanism. J. Biol. Regul. Homeost Agents 24, 397–402.
Chung, H., Kim, E., Lee, D. H., Seo, S., Ju, S., Lee, D., et al. (2007). Ghrelin inhibits apoptosis in hypothalamic neuronal cells during oxygen-glucose deprivation. Endocrinology 148, 148–159. doi: 10.1210/en.2006-0991
Cong, W. N., Golden, E., Pantaleo, N., White, C. M., Maudsley, S., and Martin, B. (2010). Ghrelin receptor signaling: a promising therapeutic target for metabolic syndrome and cognitive dysfunction. CNS Neurol. Disord. Drug Targets 9, 557–563. doi: 10.2174/187152710793361513
Cooke, J. H., Patterson, M., Patel, S. R., Smith, K. L., Ghatei, M. A., Bloom, S. R., et al. (2009). Peripheral and central administration of xenin and neurotensin suppress food intake in rodents. Obesity 17, 1135–1143.
Cowley, M. A., Smith, R. G., Diano, S., Tschop, M., Pronchuk, N., Grove, K. L., et al. (2003). The distribution and mechanism of action of ghrelin in the CNS demonstrates a novel hypothalamic circuit regulating energy homeostasis. Neuron 37, 649–661. doi: 10.1016/s0896-6273(03)00063-1
Cox, N. J., Ibrahim, K., Sayer, A. A., Robinson, S. M., and Roberts, H. C. (2019). Assessment and treatment of the anorexia of aging: a systematic review. Nutrients 11:144. doi: 10.3390/nu11010144
Craft, S. (2007). Insulin resistance and Alzheimer’s disease pathogenesis: potential mechanisms and implications for treatment. Curr. Alzheimer Res. 4, 147–152. doi: 10.2174/156720507780362137
Creyghton, W. M., Van Dam, P. S., and Koppeschaar, H. P. (2004). The role of the somatotropic system in cognition and other cerebral functions. Semin. Vasc. Med. 4, 167–172. doi: 10.1055/s-2004-835375
Crisby, M., Carlson, L. A., and Winblad, B. (2002). Statins in the prevention and treatment of Alzheimer disease. Alzheimer Dis. Assoc. Disord. 16, 131–136. doi: 10.1097/00002093-200207000-00001
Date, Y., Shimbara, T., Koda, S., Toshinai, K., Ida, T., Murakami, N., et al. (2006). Peripheral ghrelin transmits orexigenic signals through the noradrenergic pathway from the hindbrain to the hypothalamus. Cell. Metab. 4, 323–331. doi: 10.1016/j.cmet.2006.09.004
de La Monte, S. M., and Wands, J. R. (2008). Alzheimer’s disease is type 3 diabetes-evidence reviewed. J. Diabetes Sci. Technol. 2, 1101–1113. doi: 10.1177/193229680800200619
Devanand, D. P., Sano, M., Tang, M. X., Taylor, S., Gurland, B. J., Wilder, D., et al. (1996). Depressed mood and the incidence of Alzheimer’s disease in the elderly living in the community. Arch. Gen. Psychiatry 53, 175–182. doi: 10.1001/archpsyc.1996.01830020093011
Dhurandhar, E. J., Allison, D. B., Van Groen, T., and Kadish, I. (2013). Hunger in the absence of caloric restriction improves cognition and attenuates Alzheimer’s disease pathology in a mouse model. PLoS One 8:e60437. doi: 10.1371/journal.pone.0060437
Di Francesco, V., Fantin, F., Omizzolo, F., Residori, L., Bissoli, L., Bosello, O., et al. (2007). The anorexia of aging. Dig. Dis. 25, 129–137.
Diano, S., Farr, S. A., Benoit, S. C., Mcnay, E. C., Da Silva, I., Horvath, B., et al. (2006). Ghrelin controls hippocampal spine synapse density and memory performance. Nat. Neurosci. 9, 381–388. doi: 10.1038/nn1656
DiStefano, P. S., Curtis, R., and Geddes, B. J. (2007). Insulin resistance, glycemic control and adiposity: key determinants of healthy lifespan. Curr. Alzheimer Res. 4, 153–157. doi: 10.2174/156720507780362038
Do, K., Laing, B. T., Landry, T., Bunner, W., Mersaud, N., Matsubara, T., et al. (2018). The effects of exercise on hypothalamic neurodegeneration of Alzheimer’s disease mouse model. PLoS One 13:e0190205. doi: 10.1371/journal.pone.0190205
Doi, A., Shono, T., Nishi, M., Furuta, H., Sasaki, H., and Nanjo, K. (2006). IA-2beta, but not IA-2, is induced by ghrelin and inhibits glucose-stimulated insulin secretion. Proc. Natl. Acad. Sci. U.S.A. 103, 885–890. doi: 10.1073/pnas.0502470102
Duarte-Neves, J., Pereira De Almeida, L., and Cavadas, C. (2016). Neuropeptide Y (NPY) as a therapeutic target for neurodegenerative diseases. Neurobiol. Dis. 95, 210–224. doi: 10.1016/j.nbd.2016.07.022
Eslami, M., Sadeghi, B., and Goshadrou, F. (2018). Chronic ghrelin administration restores hippocampal long-term potentiation and ameliorates memory impairment in rat model of Alzheimer’s disease. Hippocampus 28, 724–734. doi: 10.1002/hipo.23002
Fitzpatrick, K., Winrow, C. J., Gotter, A. L., Millstein, J., Arbuzova, J., Brunner, J., et al. (2012). Altered sleep and affect in the neurotensin receptor 1 knockout mouse. Sleep 35, 949–956. doi: 10.5665/sleep.1958
Frolich, L., Blum-Degen, D., Riederer, P., and Hoyer, S. (1999). A disturbance in the neuronal insulin receptor signal transduction in sporadic Alzheimer’s disease. Ann. N.Y. Acad. Sci. 893, 290–293. doi: 10.1111/j.1749-6632.1999.tb07839.x
Furiya, Y., Tomiyama, T., Izumi, T., Ohba, N., and Ueno, S. (2018). Rivastigmine improves appetite by increasing the plasma Acyl/Des-Acyl ghrelin ratio and cortisol in Alzheimer disease. Dement. Geriatr. Cogn. Disord. Extra 8, 77–84. doi: 10.1159/000487358
Gahete, M. D., Cordoba-Chacon, J., Kineman, R. D., Luque, R. M., and Castano, J. P. (2011). Role of ghrelin system in neuroprotection and cognitive functions: implications in Alzheimer’s disease. Peptides 32, 2225–2228. doi: 10.1016/j.peptides.2011.09.019
Gahete, M. D., Rubio, A., Córdoba-Chacón, J., Gracia-Navarro, F., Kineman, R. D., Avila, J., et al. (2010). Expression of the ghrelin and neurotensin systems is altered in the temporal lobe of Alzheimer’s disease patients. J. Alzheimers Dis. 22, 819–828. doi: 10.3233/jad-2010-100873
Gallagher-Thompson, D., Brooks, J. O. III, Bliwise, D., Leader, J., and Yesavage, J. A. (1992). The relations among caregiver stress, “sundowning” symptoms, and cognitive decline in Alzheimer’s disease. J. Am. Geriatr. Soc. 40, 807–810. doi: 10.1111/j.1532-5415.1992.tb01853.x
Garaulet, M., Sanchez-Moreno, C., Smith, C. E., Lee, Y. C., Nicolas, F., and Ordovas, J. M. (2011). Ghrelin, sleep reduction and evening preference: relationships to CLOCK 3111 T/C SNP and weight loss. PLoS One 6:e17435. doi: 10.1371/journal.pone.0017435
Gastard, M. C., Troncoso, J. C., and Koliatsos, V. E. (2003). Caspase activation in the limbic cortex of subjects with early Alzheimer’s disease. Ann. Neurol. 54, 393–398. doi: 10.1002/ana.10680
Gauna, C., Meyler, F. M., Janssen, J. A., Delhanty, P. J., Abribat, T., Van Koetsveld, P., et al. (2004). Administration of acylated ghrelin reduces insulin sensitivity, whereas the combination of acylated plus unacylated ghrelin strongly improves insulin sensitivity. J. Clin. Endocrinol. Metab. 89, 5035–5042. doi: 10.1210/jc.2004-0363
Goshadrou, F., Kazerouni, F., Mehranfard, N., and Sadeghi, B. (2015). Chronic administration of ghrelin regulates plasma glucose and normalizes insulin levels following fasting hyperglycemia and hyperinsulinemia. Gen. Comp. Endocrinol. 224, 113–120. doi: 10.1016/j.ygcen.2015.07.001
Goto, M., Arima, H., Watanabe, M., Hayashi, M., Banno, R., Sato, I., et al. (2006). Ghrelin increases neuropeptide y and agouti-related peptide gene expression in the arcuate nucleus in rat hypothalamic organotypic cultures. Endocrinology 147, 5102–5109. doi: 10.1210/en.2006-0104
Guo, L., Niu, M., Yang, J., Li, L., Liu, S., Sun, Y., et al. (2019). GHS-R1a deficiency alleviates depression-related behaviors after chronic social defeat stress. Front. Neurosci. 13:364. doi: 10.3389/fnins.2019.00364
Hansson, C., Alvarez-Crespo, M., Taube, M., Skibicka, K. P., Schmidt, L., Karlsson-Lindahl, L., et al. (2014). Influence of ghrelin on the central serotonergic signaling system in mice. Neuropharmacology 79, 498–505. doi: 10.1016/j.neuropharm.2013.12.012
Hellweg, R., Zueger, M., Fink, K., Hörtnagl, H., and Gass, P. (2007). Olfactory bulbectomy in mice leads to increased BDNF levels and decreased serotonin turnover in depression-related brain areas. Neurobiol. Dis. 25, 1–7. doi: 10.1016/j.nbd.2006.07.017
Hu, K., Harper, D. G., Shea, S. A., Stopa, E. G., and Scheer, F. A. J. L. (2013). Noninvasive fractal biomarker of clock neurotransmitter disturbance in humans with dementia. Sci. Rep. 3:2229.
Inui, A., Asakawa, A., Bowers, C. Y., Mantovani, G., Laviano, A., Meguid, M. M., et al. (2004). Ghrelin, appetite, and gastric motility: the emerging role of the stomach as an endocrine organ. FASEB J. 18, 439–456. doi: 10.1096/fj.03-0641rev
Jeon, S. G., Hong, S. B., Nam, Y., Tae, J., Yoo, A., Song, E. J., et al. (2019). Ghrelin in Alzheimer’s disease: pathologic roles and therapeutic implications. Ageing Res. Rev. 55:100945. doi: 10.1016/j.arr.2019.100945
Jeong, Y. O., Shin, S. J., Park, J. Y., Ku, B. K., Song, J. S., Kim, J. J., et al. (2018). MK-0677, a ghrelin agonist, alleviates amyloid beta-related pathology in 5XFAD mice, an animal model of Alzheimer’s disease. Int. J. Mol. Sci. 19:1800. doi: 10.3390/ijms19061800
Jones, N. S., Watson, K. Q., and Rebeck, G. W. (2019). Metabolic disturbances of a high-fat diet are dependent on APOE genotype and sex. eNeuro 6:ENEURO.0267-19.2019.
Kang, J. E., Lim, M. M., Bateman, R. J., Lee, J. J., Smyth, L. P., Cirrito, J. R., et al. (2009). Amyloid-beta dynamics are regulated by orexin and the sleep-wake cycle. Science 326, 1005–1007. doi: 10.1126/science.1180962
Kang, S., Moon, N. R., Kim, D. S., Kim, S. H., and Park, S. (2015). Central acylated ghrelin improves memory function and hippocampal AMPK activation and partly reverses the impairment of energy and glucose metabolism in rats infused with beta-amyloid. Peptides 71, 84–93. doi: 10.1016/j.peptides.2015.07.005
Kawakami, A., Okada, N., Rokkaku, K., Honda, K., Ishibashi, S., and Onaka, T. (2008). Leptin inhibits and ghrelin augments hypothalamic noradrenaline release after stress. Stress 11, 363–369. doi: 10.1080/10253890701820257
Kepe, V., Barrio, J. R., Huang, S. C., Ercoli, L., Siddarth, P., Shoghi-Jadid, K., et al. (2006). Serotonin 1A receptors in the living brain of Alzheimer’s disease patients. Proc. Natl. Acad. Sci. U.S.A. 103, 702–707. doi: 10.1073/pnas.0510237103
Kluge, M., Gazea, M., Schussler, P., Genzel, L., Dresler, M., Kleyer, S., et al. (2010). Ghrelin increases slow wave sleep and stage 2 sleep and decreases stage 1 sleep and REM sleep in elderly men but does not affect sleep in elderly women. Psychoneuroendocrinology 35, 297–304. doi: 10.1016/j.psyneuen.2009.07.007
Kohno, D., Gao, H.-Z., Muroya, S., Kikuyama, S., and Yada, T. (2003). Ghrelin directly interacts with neuropeptide-y-containing neurons in the rat arcuate nucleus. <span class=“subtitle”>Ca2+ signaling via protein kinase A and N-type channel-dependent mechanisms and cross-talk with leptin and orexin </span>. Diabetes 52, 948–956. doi: 10.2337/diabetes.52.4.948
Kumar, R., Salehi, A., Rehfeld, J. F., Hoglund, P., Lindstrom, E., and Hakanson, R. (2010). Proghrelin peptides: desacyl ghrelin is a powerful inhibitor of acylated ghrelin, likely to impair physiological effects of acyl ghrelin but not of obestatin A study of pancreatic polypeptide secretion from mouse islets. Regul. Pept. 164, 65–70. doi: 10.1016/j.regpep.2010.06.005
Labarthe, A., Fiquet, O., Hassouna, R., Zizzari, P., Lanfumey, L., Ramoz, N., et al. (2014). Ghrelin-derived peptides: a link between appetite/reward, GH axis, and psychiatric disorders? Front. Endocrinol. 5:163. doi: 10.3389/fendo.2014.00163
Lane, R. M., and Farlow, M. R. (2005). Lipid homeostasis and apolipoprotein E in the development and progression of Alzheimer’s disease. J. Lipid Res. 46, 949–968. doi: 10.1194/jlr.m400486-jlr200
Laws, A., King, A. C., Haskell, W. L., and Reaven, G. M. (1991). Relation of fasting plasma insulin concentration to high density lipoprotein cholesterol and triglyceride concentrations in men. Arterioscler. Thromb. 11, 1636–1642. doi: 10.1161/01.atv.11.6.1636
Lee, C. W., Shih, Y. H., Wu, S. Y., Yang, T., Lin, C., and Kuo, Y. M. (2013). Hypoglycemia induces tau hyperphosphorylation. Curr. Alzheimer Res. 10, 298–308. doi: 10.2174/1567205011310030009
LeSauter, J., Hoque, N., Weintraub, M., Pfaff, D. W., and Silver, R. (2009). Stomach ghrelin-secreting cells as food-entrainable circadian clocks. Proc. Natl. Acad. Sci. U.S.A. 106, 13582–13587. doi: 10.1073/pnas.0906426106
Liu, Y., Chen, L., Xu, X., Vicaut, E., and Sercombe, R. (2009). Both ischemic preconditioning and ghrelin administration protect hippocampus from ischemia/reperfusion and upregulate uncoupling protein-2. BMC Physiol. 9:17. doi: 10.1186/1472-6793-9-17
Liu, Y., Liu, F., Grundke-Iqbal, I., Iqbal, K., and Gong, C.-X. (2011). Deficient brain insulin signalling pathway in Alzheimer’s disease and diabetes. J. Pathol. 225, 54–62. doi: 10.1002/path.2912
Lopez, M., Lage, R., Saha, A. K., Perez-Tilve, D., Vazquez, M. J., Varela, L., et al. (2008). Hypothalamic fatty acid metabolism mediates the orexigenic action of ghrelin. Cell. Metab. 7, 389–399. doi: 10.1016/j.cmet.2008.03.006
Lutter, M., Sakata, I., Osborne-Lawrence, S., Rovinsky, S. A., Anderson, J. G., Jung, S., et al. (2008). The orexigenic hormone ghrelin defends against depressive symptoms of chronic stress. Nat. Neurosci. 11, 752–753. doi: 10.1038/nn.2139
Ma, L. Y., Zhang, D. M., Tang, Y., Lu, Y., Zhang, Y., Gao, Y., et al. (2011). Ghrelin-attenuated cognitive dysfunction in streptozotocin-induced diabetic rats. Alzheimer Dis. Assoc. Disord. 25, 352–363. doi: 10.1097/wad.0b013e31820ce536
Makovey, J., Naganathan, V., Seibel, M., and Sambrook, P. (2007). Gender differences in plasma ghrelin and its relations to body composition and bone – an opposite-sex twin study. Clin. Endocrinol. 66, 530–537.
Malik, S., Mcglone, F., Bedrossian, D., and Dagher, A. (2008). Ghrelin modulates brain activity in areas that control appetitive behavior. Cell Metab. 7, 400–409. doi: 10.1016/j.cmet.2008.03.007
Martinez de Morentin, P. B., Gonzalez, C. R., and Lopez, M. (2010). AMP-activated protein kinase: ‘a cup of tea’ against cholesterol-induced neurotoxicity. J. Pathol. 222, 329–334. doi: 10.1002/path.2778
Matsuoka, T., Oya, N., Yokota, H., Akazawa, K., Yamada, K., Narumoto, J., et al. (2020). Pineal volume reduction in patients with mild cognitive impairment who converted to Alzheimer’s disease. Psychiatry Clin. Neurosci. doi: 10.1111/pcn.13103 [Epub ahead of print].
Merlo, S., Spampinato, S., Canonico, P. L., Copani, A., and Sortino, M. A. (2010). Alzheimer’s disease: brain expression of a metabolic disorder? Trends Endocrinol. Metab. 21, 537–544.
Merriam, A. E., Aronson, M. K., Gaston, P., Wey, S. L., and Katz, I. (1988). The psychiatric symptoms of Alzheimer’s disease. J. Am. Geriatr. Soc. 36, 7–12.
Minoshima, S., Giordani, B., Berent, S., Frey, K. A., Foster, N. L., and Kuhl, D. E. (1997). Metabolic reduction in the posterior cingulate cortex in very early Alzheimer’s disease. Ann. Neurol. 42, 85–94. doi: 10.1002/ana.410420114
Modrego, P. J. (2010). Depression in Alzheimer’s disease. Pathophysiology, diagnosis, and treatment. J. Alzheimers Dis. 21, 1077–1087. doi: 10.3233/jad-2010-100153
Moon, M., Cha, M. Y., and Mook-Jung, I. (2014). Impaired hippocampal neurogenesis and its enhancement with ghrelin in 5XFAD mice. J. Alzheimers Dis. 41, 233–241. doi: 10.3233/jad-132417
Moon, M., Choi, J. G., Nam, D. W., Hong, H. S., Choi, Y. J., Oh, M. S., et al. (2011). Ghrelin ameliorates cognitive dysfunction and neurodegeneration in intrahippocampal amyloid-beta1-42 oligomer-injected mice. J. Alzheimers Dis. 23, 147–159. doi: 10.3233/jad-2010-101263
Mora, M., Mansego, M. L., Serra-Prat, M., Palomera, E., Boquet, X., Chaves, J. F., et al. (2014). Glucose impairment and ghrelin gene variants are associated to cognitive dysfunction. Aging Clin. Exp. Res. 26, 161–169. doi: 10.1007/s40520-014-0203-5
Morales-Medina, J. C., Dumont, Y., and Quirion, R. (2010). A possible role of neuropeptide Y in depression and stress. Brain Res. 1314, 194–205. doi: 10.1016/j.brainres.2009.09.077
Muccioli, G., Pons, N., Ghe, C., Catapano, F., Granata, R., and Ghigo, E. (2004). Ghrelin and des-acyl ghrelin both inhibit isoproterenol-induced lipolysis in rat adipocytes via a non-type 1a growth hormone secretagogue receptor. Eur. J. Pharmacol. 498, 27–35. doi: 10.1016/j.ejphar.2004.07.066
Muller, T. D., Nogueiras, R., Andermann, M. L., Andrews, Z. B., Anker, S. D., Argente, J., et al. (2015). Ghrelin. Mol. Metab. 4, 437–460.
Nelson, T. J., and Alkon, D. L. (2005). Insulin and cholesterol pathways in neuronal function, memory and neurodegeneration. Biochem. Soc. Trans. 33, 1033–1036. doi: 10.1042/bst0331033
Nestler, E. J., Barrot, M., Dileone, R. J., Eisch, A. J., Gold, S. J., and Monteggia, L. M. (2002). Neurobiology of depression. Neuron 34, 13–25.
Nieminen, P., and Mustonen, A. M. (2004). Effects of peripheral ghrelin on the carbohydrate and lipid metabolism of the tundra vole (Microtus oeconomus). Gen. Comp. Endocrinol. 138, 182–187. doi: 10.1016/j.ygcen.2004.06.001
Novais, F., and Starkstein, S. (2015). Phenomenology of depression in Alzheimer’s disease. J. Alzheimers Dis. 47, 845–855.
Obal, F. Jr., Alt, J., Taishi, P., Gardi, J., and Krueger, J. M. (2003). Sleep in mice with nonfunctional growth hormone-releasing hormone receptors. Am. J. Physiol. Regul. Integr. Comp. Physiol. 284, R131–R139.
Okuda, S., Tetsuka, J., Takahashi, K., Toda, Y., Kubo, T., and Tokita, S. (2019). Association between sleep disturbance in Alzheimer’s disease patients and burden on and health status of their caregivers. J. Neurol. 266, 1490–1500. doi: 10.1007/s00415-019-09286-0
Ooms, S., Overeem, S., Besse, K., Rikkert, M. O., Verbeek, M., and Claassen, J. A. (2014). Effect of 1 night of total sleep deprivation on cerebrospinal fluid beta-amyloid 42 in healthy middle-aged men: a randomized clinical trial. JAMA Neurol. 71, 971–977. doi: 10.1001/jamaneurol.2014.1173
Overduin, J., Figlewicz, D. P., Bennett-Jay, J., Kittleson, S., and Cummings, D. E. (2012). Ghrelin increases the motivation to eat, but does not alter food palatability. Am. J. Physiol. Regul. Integr. Comp. Physiol. 303, R259–R269.
Pacifico, L., Poggiogalle, E., Costantino, F., Anania, C., Ferraro, F., Chiarelli, F., et al. (2009). Acylated and nonacylated ghrelin levels and their associations with insulin resistance in obese and normal weight children with metabolic syndrome. Eur. J. Endocrinol. 161, 861–870. doi: 10.1530/eje-09-0375
Poulin, S. P., Dautoff, R., Morris, J. C., Barrett, L. F., and Dickerson, B. C., and Alzheimer’s Disease Neuroimaging (2011). Amygdala atrophy is prominent in early Alzheimer’s disease and relates to symptom severity. Psychiatry Res. 194, 7–13. doi: 10.1016/j.pscychresns.2011.06.014
Pradhan, G., Samson, S. L., and Sun, Y. (2013). Ghrelin: much more than a hunger hormone. Curr. Opin. Clin. Nutr. Metab. Care 16, 619–624. doi: 10.1097/mco.0b013e328365b9be
Prince, T.-M., and Abel, T. (2013). The impact of sleep loss on hippocampal function. Learn. Mem. 20, 558–569. doi: 10.1101/lm.031674.113
Rajkowska, G. (2000). Postmortem studies in mood disorders indicate altered numbers of neurons and glial cells. Biol. Psychiatry 48, 766–777. doi: 10.1016/s0006-3223(00)00950-1
Rasmuson, S., Andrew, R., Nasman, B., Seckl, J. R., Walker, B. R., and Olsson, T. (2001). Increased glucocorticoid production and altered cortisol metabolism in women with mild to moderate Alzheimer’s disease. Biol. Psychiatry 49, 547–552. doi: 10.1016/s0006-3223(00)01015-5
Razay, G., Vreugdenhil, A., and Wilcock, G. (2007). The metabolic syndrome and Alzheimer disease. Arch. Neurol. 64, 93–96.
Ressler, K. J., and Nemeroff, C. B. (2000). Role of serotonergic and noradrenergic systems in the pathophysiology of depression and anxiety disorders. Depress Anxiety 12(Suppl. 1), 2–19. doi: 10.1002/1520-6394(2000)12:1
Ries, M. L., Wichmann, A., Bendlin, B. B., and Johnson, S. C. (2009). Posterior cingulate and lateral parietal gray matter volume in older adults with depressive symptoms. Brain Imaging Behav. 3, 233–239. doi: 10.1007/s11682-009-9065-4
Rodriguez, A., Gomez-Ambrosi, J., Catalan, V., Gil, M. J., Becerril, S., Sainz, N., et al. (2009). Acylated and desacyl ghrelin stimulate lipid accumulation in human visceral adipocytes. Int. J. Obes. 33, 541–552. doi: 10.1038/ijo.2009.40
Rothman, S. M., Herdener, N., Frankola, K. A., Mughal, M. R., and Mattson, M. P. (2013). Chronic mild sleep restriction accentuates contextual memory impairments, and accumulations of cortical Abeta and pTau in a mouse model of Alzheimer’s disease. Brain Res. 1529, 200–208. doi: 10.1016/j.brainres.2013.07.010
Roy, U., Heredia-Munoz, M. T., Stute, L., Hofling, C., Matysik, J., Meijer, J. H., et al. (2019). Degeneration of the suprachiasmatic nucleus in an Alzheimer’s disease mouse model monitored by in vivo magnetic resonance relaxation measurements and immunohistochemistry. J. Alzheimers Dis. 69, 363–375. doi: 10.3233/jad-190037
Saito, Y., Chapple, R. H., Lin, A., Kitano, A., and Nakada, D. (2015). AMPK protects leukemia-initiating cells in myeloid leukemias from metabolic stress in the bone marrow. Cell Stem Cell 17, 585–596. doi: 10.1016/j.stem.2015.08.019
Saper, C. B., Lu, J., Chou, T. C., and Gooley, J. (2005). The hypothalamic integrator for circadian rhythms. Trends Neurosci. 28, 152–157. doi: 10.1016/j.tins.2004.12.009
Schubert, M., Brazil, D. P., Burks, D. J., Kushner, J. A., Ye, J., Flint, C. L., et al. (2003). Insulin receptor substrate-2 deficiency impairs brain growth and promotes tau phosphorylation. J. Neurosci. 23, 7084–7092. doi: 10.1523/jneurosci.23-18-07084.2003
Serra-Prat, M., Alfaro, S. R., Palomera, E., Casamitjana, R., Buquet, X., Fernandez-Fernandez, C., et al. (2009). Relationship between ghrelin and the metabolic syndrome in the elderly: a longitudinal population-based study. Clin. Endocrinol. 70, 227–232. doi: 10.1111/j.1365-2265.2008.03307.x
Sethi, M., Joshi, S. S., Webb, R. L., Beckett, T. L., Donohue, K. D., Murphy, M. P., et al. (2015). Increased fragmentation of sleep-wake cycles in the 5XFAD mouse model of Alzheimer’s disease. Neuroscience 290, 80–89. doi: 10.1016/j.neuroscience.2015.01.035
Shou, J., Chen, P.-J., and Xiao, W.-H. (2020). Mechanism of increased risk of insulin resistance in aging skeletal muscle. Diabetol. Metab. Syndrome 12:14.
Sibilia, V., Pagani, F., Mrak, E., Dieci, E., Tulipano, G., and Ferrucci, F. (2012). Pharmacological characterization of the ghrelin receptor mediating its inhibitory action on inflammatory pain in rats. Amino Acids 43, 1751–1759. doi: 10.1007/s00726-012-1260-8
Simonen, P. P., Gylling, H. K., and Miettinen, T. A. (2002). Diabetes contributes to cholesterol metabolism regardless of obesity. Diabetes Care 25, 1511–1515. doi: 10.2337/diacare.25.9.1511
Smith, M. A., Makino, S., Kvetnansky, R., and Post, R. M. (1995). Stress and glucocorticoids affect the expression of brain-derived neurotrophic factor and neurotrophin-3 mRNAs in the hippocampus. J. Neurosci. 15, 1768–1777. doi: 10.1523/jneurosci.15-03-01768.1995
Song, C., Zhang, X. Y., and Manku, M. (2009). Increased phospholipase A2 activity and inflammatory response but decreased nerve growth factor expression in the olfactory bulbectomized rat model of depression: effects of chronic ethyl-eicosapentaenoate treatment. J. Neurosci. 29, 14–22. doi: 10.1523/jneurosci.3569-08.2009
Song, N., Wang, W., Jia, F., Du, X., Xie, A., He, Q., et al. (2017). Assessments of plasma ghrelin levels in the early stages of parkinson’s disease. Mov. Disord. 32, 1487–1491. doi: 10.1002/mds.27095
Steiger, A., Dresler, M., Schussler, P., and Kluge, M. (2011). Ghrelin in mental health, sleep, memory. Mol. Cell. Endocrinol. 340, 88–96. doi: 10.1016/j.mce.2011.02.013
Sterniczuk, R., Dyck, R. H., Laferla, F. M., and Antle, M. C. (2010). Characterization of the 3xTg-AD mouse model of Alzheimer’s disease: part 1. Circadian changes. Brain Res. 1348, 139–148. doi: 10.1016/j.brainres.2010.05.013
Szentirmai, E., Kapas, L., and Krueger, J. M. (2007). Ghrelin microinjection into forebrain sites induces wakefulness and feeding in rats. Am. J. Physiol. Regul. Integr. Comp. Physiol. 292, R575–R585.
Theander-Carrillo, C., Wiedmer, P., Cettour-Rose, P., Nogueiras, R., Perez-Tilve, D., Pfluger, P., et al. (2006). Ghrelin action in the brain controls adipocyte metabolism. J. Clin. Invest. 116, 1983–1993. doi: 10.1172/jci25811
Theodoropoulou, A., Metallinos, I. C., Psyrogiannis, A., Vagenakis, G. A., and Kyriazopoulou, V. (2012). Ghrelin and leptin secretion in patients with moderate Alzheimer’s disease. J. Nutr. Health Aging 16, 472–477. doi: 10.1007/s12603-012-0058-4
Thornton, C., Bright, N. J., Sastre, M., Muckett, P. J., and Carling, D. (2011). AMP-activated protein kinase (AMPK) is a tau kinase, activated in response to amyloid beta-peptide exposure. Biochem. J. 434, 503–512. doi: 10.1042/bj20101485
Tschop, M., Smiley, D. L., and Heiman, M. L. (2000). Ghrelin induces adiposity in rodents. Nature 407, 908–913. doi: 10.1038/35038090
Uddin, M. S., Tewari, D., Mamun, A. A., Kabir, M. T., Niaz, K., Wahed, M. I. I., et al. (2020). Circadian and sleep dysfunction in Alzheimer’s disease. Ageing Res. Rev. 60:101046.
Ukkola, O., Poykko, S. M., and Antero Kesaniemi, Y. (2006). Low plasma ghrelin concentration is an indicator of the metabolic syndrome. Ann. Med. 38, 274–279. doi: 10.1080/07853890600622192
Valentine, R. J., Coughlan, K. A., Ruderman, N. B., and Saha, A. K. (2014). Insulin inhibits AMPK activity and phosphorylates AMPK Ser(4)(8)(5)/(4)(9)(1) through Akt in hepatocytes, myotubes and incubated rat skeletal muscle. Arch. Biochem. Biophys. 562, 62–69. doi: 10.1016/j.abb.2014.08.013
van Bussel, F. C., Backes, W. H., Hofman, P. A., Puts, N. A., Edden, R. A., Van Boxtel, M. P., et al. (2016). Increased GABA concentrations in type 2 diabetes mellitus are related to lower cognitive functioning. Medicine 95:e4803. doi: 10.1097/md.0000000000004803
van Reekum, R., Simard, M., Clarke, D., Binns, M. A., and Conn, D. (1999). Late-life depression as a possible predictor of dementia: cross-sectional and short-term follow-up results. Am. J. Geriatr. Psychiatry 7, 151–159. doi: 10.1097/00019442-199921720-00009
Vandal, M., White, P. J., Tremblay, C., St-Amour, I., Chevrier, G., Emond, V., et al. (2014). Insulin reverses the high-fat diet-induced increase in brain Abeta and improves memory in an animal model of Alzheimer disease. Diabetes 63, 4291–4301. doi: 10.2337/db14-0375
Verghese, P. B., Castellano, J. M., and Holtzman, D. M. (2011). Apolipoprotein E in Alzheimer’s disease and other neurological disorders. Lancet Neurol. 10, 241–252.
Versijpt, J., Van Laere, K. J., Dumont, F., Decoo, D., Vandecapelle, M., Santens, P., et al. (2003). Imaging of the 5-HT2A system: age-, gender-, and Alzheimer’s disease-related findings. Neurobiol. Aging 24, 553–561.
Volicer, L., Harper, D. G., Manning, B. C., Goldstein, R., and Satlin, A. (2001). Sundowning and circadian rhythms in Alzheimer’s disease. Am. J. Psychiatry 158, 704–711. doi: 10.1176/appi.ajp.158.5.704
Wang, X., Yu, S., Gao, S. J., Hu, J. P., Wang, Y., and Liu, H. X. (2014). Insulin inhibits Abeta production through modulation of APP processing in a cellular model of Alzheimer’s disease. Neuro Endocrinol. Lett. 35, 224–229.
Wang, Y., Nishi, M., Doi, A., Shono, T., Furukawa, Y., Shimada, T., et al. (2010). Ghrelin inhibits insulin secretion through the AMPK-UCP2 pathway in beta cells. FEBS Lett. 584, 1503–1508. doi: 10.1016/j.febslet.2010.02.069
Waseem, T., Duxbury, M., Ito, H., Ashley, S. W., and Robinson, M. K. (2008). Exogenous ghrelin modulates release of pro-inflammatory and anti-inflammatory cytokines in LPS-stimulated macrophages through distinct signaling pathways. Surgery 143, 334–342. doi: 10.1016/j.surg.2007.09.039
Wilson, R. S., Barnes, L. L., Mendes De Leon, C. F., Aggarwal, N. T., Schneider, J. S., Bach, J., et al. (2002). Depressive symptoms, cognitive decline, and risk of AD in older persons. Neurology 59, 364–370. doi: 10.1212/wnl.59.3.364
Wren, A. M., Seal, L. J., Cohen, M. A., Brynes, A. E., Frost, G. S., Murphy, K. G., et al. (2001). Ghrelin enhances appetite and increases food intake in humans. J. Clin. Endocrinol. Metab. 86:5992. doi: 10.1210/jcem.86.12.8111
Wren, A. M., Small, C. J., Ward, H. L., Murphy, K. G., Dakin, C. L., Taheri, S., et al. (2000). The novel hypothalamic peptide ghrelin stimulates food intake and growth hormone secretion. Endocrinology 141, 4325–4328. doi: 10.1210/endo.141.11.7873
Wu, Y. H., and Swaab, D. F. (2005). The human pineal gland and melatonin in aging and Alzheimer’s disease. J. Pineal Res. 38, 145–152. doi: 10.1111/j.1600-079x.2004.00196.x
Wulff, K., Gatti, S., Wettstein, J. G., and Foster, R. G. (2010). Sleep and circadian rhythm disruption in psychiatric and neurodegenerative disease. Nat. Rev. Neurosci. 11, 589–599. doi: 10.1038/nrn2868
Yanagi, S., Sato, T., Kangawa, K., and Nakazato, M. (2018). The homeostatic force of ghrelin. Cell Metab. 27, 786–804. doi: 10.1016/j.cmet.2018.02.008
Yang, S. J., Yu, H. Y., Kang, D. Y., Ma, Z. Q., Qu, R., Fu, Q., et al. (2014). Antidepressant-like effects of salidroside on olfactory bulbectomy-induced pro-inflammatory cytokine production and hyperactivity of HPA axis in rats. Pharmacol. Biochem. Behav. 124, 451–457. doi: 10.1016/j.pbb.2014.07.015
Yannielli, P. C., Molyneux, P. C., Harrington, M. E., and Golombek, D. A. (2007). Ghrelin effects on the circadian system of mice. J. Neurosci. 27, 2890–2895. doi: 10.1523/jneurosci.3913-06.2007
Yoshino, Y., Funahashi, Y., Nakata, S., Ozaki, Y., Yamazaki, K., Yoshida, T., et al. (2018). Ghrelin cascade changes in the peripheral blood of Japanese patients with Alzheimer’s disease. J. Psychiatr. Res. 107, 79–85. doi: 10.1016/j.jpsychires.2018.10.011
Zhang, W., Chai, B., Li, J. Y., Wang, H., and Mulholland, M. W. (2008). Effect of des-acyl ghrelin on adiposity and glucose metabolism. Endocrinology 149, 4710–4716. doi: 10.1210/en.2008-0263
Zheng, H., Corkern, M., Stoyanova, I., Patterson, L. M., Tian, R., and Berthoud, H. R. (2003). Peptides that regulate food intake: appetite-inducing accumbens manipulation activates hypothalamic orexin neurons and inhibits POMC neurons. Am. J. Physiol. Regul. Integr. Comp. Physiol. 284, R1436–R1444.
Zigman, J. M., Jones, J. E., Lee, C. E., Saper, C. B., and Elmquist, J. K. (2006). Expression of ghrelin receptor mRNA in the rat and the mouse brain. J. Comp. Neurol. 494, 528–548. doi: 10.1002/cne.20823
Keywords: ghrelin, Alzheimer’s disease, metabolic syndrome, depression, sleep–wake disturbances, abnormal eating behaviors
Citation: Kim S, Nam Y, Shin SJ, Park YH, Jeon SG, Kim J, Kim M-J and Moon M (2020) The Potential Roles of Ghrelin in Metabolic Syndrome and Secondary Symptoms of Alzheimer’s Disease. Front. Neurosci. 14:583097. doi: 10.3389/fnins.2020.583097
Received: 15 July 2020; Accepted: 26 August 2020;
Published: 24 September 2020.
Edited by:
Riccarda Granata, University of Turin, ItalyCopyright © 2020 Kim, Nam, Shin, Park, Jeon, Kim, Kim and Moon. This is an open-access article distributed under the terms of the Creative Commons Attribution License (CC BY). The use, distribution or reproduction in other forums is permitted, provided the original author(s) and the copyright owner(s) are credited and that the original publication in this journal is cited, in accordance with accepted academic practice. No use, distribution or reproduction is permitted which does not comply with these terms.
*Correspondence: Minho Moon, aG9taW5tb29uQGtvbnlhbmcuYWMua3I=; aG9taW5tb29uQGRhdW0ubmV0
†These authors have contributed equally to this work