- 1Center for Clinical Intervention and Neuropsychiatric Schizophrenia Research and Center for Neuropsychiatric Schizophrenia Research, Mental Health Centre Glostrup, University of Copenhagen, Glostrup, Denmark
- 2Department of Clinical Medicine, Faculty of Health and Medical Sciences, University of Copenhagen, Copenhagen, Denmark
- 3Functional Imaging Unit, Department of Clinical Physiology, Nuclear Medicine and PET, University of Copenhagen, Glostrup, Denmark
- 4Neurobiology Research Unit, Department of Neurology, Rigshospitalet, University of Copenhagen, Copenhagen, Denmark
- 5Department of Basic and Clinical Neuroscience, Institute of Psychiatry Psychology and Neuroscience, King’s College London, London, United Kingdom
- 6Medical Research Council Centre for Neurodevelopmental Disorders, King’s College London, London, United Kingdom
- 7Department of Clinical Physiology and Nuclear Medicine, Herlev Hospital, University of Copenhagen, Herlev, Denmark
Patients with chronic schizophrenia often display enlarged striatal volumes, and antipsychotic drugs may contribute via the dopamine D2/3 receptor (D2/3R) blockade. Separating the effects of disease from medication is challenging due to the lack of a proper placebo-group. To address this, we conducted a longitudinal study of antipsychotic-naïve, first-episode schizophrenia patients to test the hypothesis that selective blockade of D2/3R would induce a dose-dependent striatal volume increase. Twenty-one patients underwent structural magnetic resonance imaging (sMRI), single-photon emission computed tomography (SPECT), and symptom severity ratings before and after six weeks of amisulpride treatment. Twenty-three matched healthy controls underwent sMRI and baseline SPECT. Data were analyzed using repeated measures and multiple regression analyses. Correlations between symptom severity decrease, volume changes, dose and receptor occupancy were explored. Striatal volumes did not differ between patients and controls at baseline or follow-up, but a significant group-by-time interaction was found (p = 0.01). This interaction was explained by a significant striatal volume increase of 2.1% in patients (Cohens d = 0.45). Striatal increase was predicted by amisulpride dose, but not by either D2/3R occupancy or baseline symptom severity. A significant reduction in symptom severity was observed at a mean dose of 233.3 (SD = 109.9) mg, corresponding to D2/3R occupancy of 44.65%. Reduction in positive symptoms correlated significantly with striatal volume increase, driven by reductions in hallucinations. Our data demonstrate a clear link between antipsychotic treatment and striatal volume increase in antipsychotic-naïve schizophrenia patients. Moreover, the treatment-induced striatal volume increase appears clinically relevant by correlating to reductions in core symptoms of schizophrenia.
Introduction
Schizophrenia is a mental disorder affecting approximately 1% of the population worldwide (Salavati et al., 2015). The disorder typically manifests in puberty or adolescence and is characterized by so-called positive symptoms such as delusions and/or hallucinations (Howes and Kapur, 2009), but patients also exhibit negative symptoms and cognitive deficits. Studies using structural magnetic resonance imaging (sMRI) provide evidence that patients with schizophrenia display subtle volumetric brain aberrations at the time of diagnosis as compared to healthy controls (Brugger and Howes, 2017; Dietsche et al., 2017). Moreover, the brain of chronic, medicated patients appears to undergo progressive, structural changes over the course of the illness, with ventricular volume increases, cortical thinning, and basal ganglia enlargement among the most consistent findings (Brandt and Bonelli, 2008; Puri, 2010; Haijma et al., 2013; van Erp et al., 2016; Dietsche et al., 2017). Antipsychotic drugs (APD) are the gold standard for treatment of positive symptoms (Howes and Kapur, 2009), but since illness and treatment go hand in hand, separating the effects of medication and disease on brain structure is difficult (Fusar-Poli et al., 2013).
In 1976 it was discovered that antipsychotics exert their function by antagonizing the dopamine D2 receptors (D2R) in striatum, and that drug efficacy is directly proportional to the affinity for the receptor (Creese et al., 1976). This led to the dopamine hypothesis of schizophrenia, which suggests that a hyperactive striatal dopamine-system leads to ‘aberrant salience’, meaning that wrongful interpretations of harmless stimuli can eventually lead to core psychotic symptoms such as hallucinations and delusions (Kapur, 2003). Further studies of striatum have found increased presynaptic dopamine synthesis capacity and -release compared to controls, as well as higher dopamine concentrations in the synaptic cleft (Howes et al., 2009, 2012; Brunelin et al., 2013; Salavati et al., 2015). All currently marketed antipsychotics antagonize the D2R, thereby blocking the down-stream signaling in the post-synaptic neuron (Golan, 2012; Kusumi et al., 2015; Amato et al., 2017). However, most antipsychotics are characterized by broad receptor profiles, and bind to e.g., serotonin 2A-, histaminergic- and cholinergic receptor systems (Kusumi et al., 2015). This complex pharmacology has further limited the investigations of causal mechanisms linking antipsychotic treatment to structural brain changes. Nevertheless, longitudinal studies on antipsychotic-naïve patients as well as meta-analyses studies have reported associations between antipsychotic exposure and volumetric increase in basal ganglia (Glenthoj et al., 2007; Ebdrup et al., 2013; Jorgensen et al., 2016; Huhtaniska et al., 2017; Di Sero et al., 2019). Studies on rodents have replicated the basal ganglia volume increase in response to antipsychotic treatment (Vernon et al., 2012), and investigations in dopamine D2 or D3 receptor knock-out- and wild-type mice provide evidence that this increase is likely to be mediated through D2-like receptors (Guma et al., 2018, 2019).
In humans, dopamine D2-like receptor availability and blockade following antipsychotic treatment can be investigated with single-photon emission computed tomography (SPECT) examinations (Salavati et al., 2015). The association between antipsychotic treatment, dopamine D2/3 receptor occupancy, and basal ganglia enlargement has, however, yet to be established in a longitudinal study of antipsychotic-naïve patients with schizophrenia.
To address this gap in our knowledge, we completed a prospective study, wherein we examined a cohort of first-episode, antipsychotic-naïve schizophrenia patients, before and after 6 weeks of treatment with amisulpride, a relatively selective dopamine D2/3 receptor antagonist. Baseline- and follow-up examinations included sMRI, SPECT, and Positive and Negative Syndrome Scale (PANSS) examinations.
We hypothesized that selective blockade of dopamine D2/3R would lead to a dose-dependent striatal volume increase. Further, we explored correlations between symptom severity decrease, striatal volume increase, dose and receptor occupancy.
Materials and Methods
Participants
We included participants between the ages of 18–45 years from 2008 to 2014. Patients with schizophrenia were first-episode, antipsychotic-naïve, and were recruited from hospitals and psychiatric out-patient clinics in the capital region of Denmark, as a part of the PECANS I (Pan European Collaboration Antipsychotic-naïve Studies, PECANS) cohort. All patients met the International Classification of Diseases (ICD-10) criteria for schizophrenia (F20) verified by the structured diagnostic interview SCAN (Schedule of Clinical Assessment in Neuropsychiatry, version 2.1). Exclusion criteria included previous exposure to antipsychotic medication, methylphenidate, or use of antidepressants less than 1 month prior to baseline examinations. Healthy controls were recruited through advertisement, and matched to patients on age, gender and parental socioeconomic status. Exclusion criteria for the healthy controls were identical to the criteria for patients, but also comprised any former or current psychiatric illnesses, psychiatric diagnoses within first-degree relatives and/or any drug-abuse (classified by ICD-10). For all participants, previous or current medical history of serious head trauma, neurological diseases, developmental disorders or current drug dependency (by ICD-10 classification), and current pregnancy were exclusion criteria. All participants were screened for drug-use with urine samples (Rapid Response, Jepsen HealthCare) prior to SPECT scan. Included participants are a subsample of Wulff et al. (2015, 2019) from the PECANS I cohort. Wulff and colleagues also reported on binding potentials in their sample, although a different method of binding potential extraction was used. Subcortical volumes have not yet been investigated in this subgroup.
Medication
The atypical APD, amisulpride, was chosen as a tool compound because of its relative selectivity toward dopamine D2/3 receptors (Rosenzweig et al., 2002). Amisulpride treatment was initiated after completion of baseline examinations, and dosage was slowly increased and adjusted to the individual patient, according to clinical judgment and patients’ reports of adverse effects. Pharmaceutical treatment against adverse effects was not allowed. Follow-up examinations were conducted after six weeks, and treatment dose in mg was recorded. To ensure a steady concentration at examinations, dosage was kept stable in the week prior to follow-up. Compliance was continuously ensured through dialogue with the patient, and measurement of serum-amisulpride (S-amisulpride) levels at follow-up. Benzodiazepines were allowed on an “as-needed basis” to secure sleep and reduce anxiety but were not allowed 12 h prior to SPECT examinations. Healthy control subjects were not treated.
Symptom Severity
Symptom severity was assessed with PANSS (Kay et al., 1987) within the same week as MRI and SPECT scan examinations. PANSS total score as well as sub-scores (positive-, negative-, and general sub-scores) was assessed at baseline and at follow-up. To ensure consistency in PANSS ratings between clinicians, ratings were regularly evaluated using systematic video recordings of the interviews. Duration of untreated illness was assessed from the patient history of worsening in functions due to symptoms. Healthy controls did not undergo PANSS examinations.
Magnetic Resonance Imaging
T1-weighted scans of the whole head (sagittal 3D sequence, TR = 10 ms, TE = 4.6 ms, FA = 8°, voxel size = 0.79 mm × 0.79 mm × 0.80 mm) were acquired with an 8-channel SENSE head coil on a 3T Philips Achieva scanner (Philips Healthcare, Best, Netherlands) at baseline and after 6 weeks. MRI scans were acquired within the same week as SPECT and PANSS. Subcortical segmentation and volume extraction were performed with tools from the FSL, FMRIB software library v5.0.10 (Patenaude et al., 2011). In this study we focused on striatum as our region of interest, estimated as a sum of volumes from the bilateral subregions of caudate nucleus, putamen and nucleus accumbens (Figure 1). Anatomically, striatum is also referred to as a part of basal ganglia (Waschke and Paulsen, 2011).
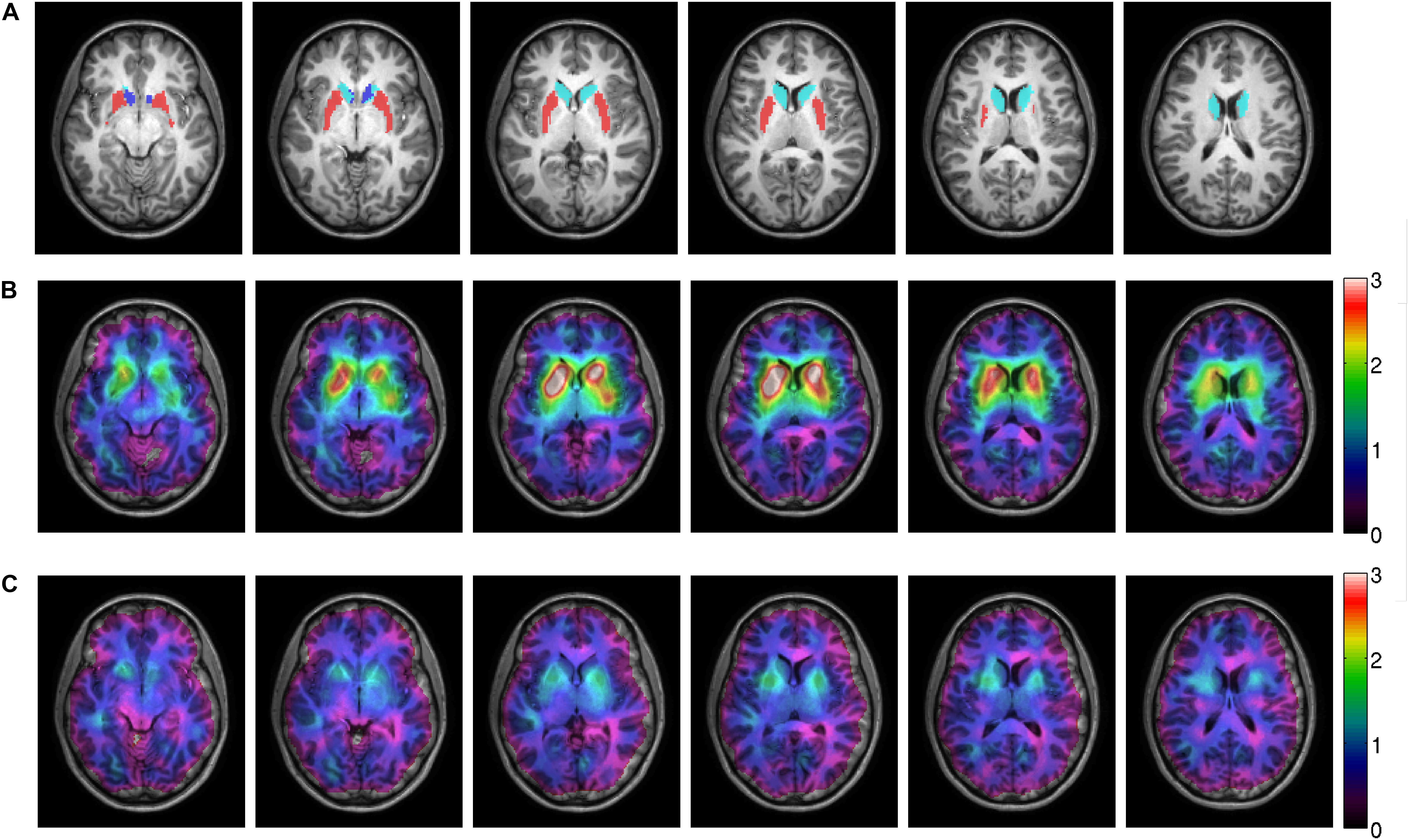
Figure 1. MRI and SPECT images of one patient, treated with 300 mg amisulpride displaying a mean dopamine D2 receptor occupancy of 56%. Panel (A) shows the sMRI image with the caudate nucleus (light blue), putamen (red) and accumbens (dark blue) from the subcortical Harvard-Oxford atlas depicted. Panels (B,C) show the co-registered SPECT image on top of the MRI image. The color scale corresponds to the specific binding potential before treatment (B) and after six weeks of treatment (C).
Single Photon Emission Computed Tomography
Single-photon emission computed tomography acquisition has previously been described (Wulff et al., 2015). In short, SPECT images were acquired using a Siemens Symbia T2 series SPECT-CT scanner, with the [123I]-Iodobenzamide ([123I]-IBZM) as the radioactive ligand, because of its dopamine D2/3R selectivity (Kung et al., 1990; Barnas et al., 2001). After 180 min of rest, a CT scout and 2 × 30 min tomography were performed. CT-scout and tomography were performed to optimize positioning in the scanner and for attenuation correction. Patients underwent both baseline and follow-up SPECT scans, whereas controls only underwent baseline SPECT to minimize their exposure to radiation. At follow-up, the individual dose of amisulpride was administered 3 h prior to the scan, and s-amisulpride was measured prior to and at 60, 120, 150, 180, 210, and 240 min after administration. The mean s-amisulpride during SPECT-scan was calculated.
Image Processing
Because SPECT images contain limited anatomical information, it was not possible to automatically extract SPECT counts (counts/s) directly from our regions of interest. First, we co-registered CT- and MR anatomical images using a statistical parametric mapping method (SPM8) to calculate the transformation matrix. This step was visually inspected with an image overlay method, and manually adjusted if needed (Willendrup et al., 2004). Next, the CT-MR transformation matrix was used to co-register the SPECT images to the MRIs, and FSL subcortical region segmentations were resliced to fit the individual SPECT images. Subsequently, SPECT counts were extracted from FSL-MRI defined regions.
Lastly, extracted SPECT counts were scatter- and decay corrected. The specific binding potentials were calculated by subtracting non-specific binding from a reference region from total binding in the regions of interest divided by the metabolite corrected plasma counts. Cerebellum as defined in Svarer et al. (2005) was used as reference region for non-specific binding, as in our previous study on binding potentials (Wulff et al., 2015). Dopamine receptor occupancy was calculated using the following equation:
Statistical Analyses
Statistical analyses were conducted using IBM SPSS version 25. Normal distributions were assessed by Shapiro–Wilk. Equality of variance was assessed by Box’s- or Levene’s test. For between-groups comparisons, unpaired students t-test was used for normally distributed data and Mann–Whitney for non-normally distributed data (demographics, volumes, and binding potentials). Within-group comparisons were analyzed with paired students t-test and Wilcoxon for non-normally distributed data. Cohens d was used to calculate effect sizes, with effect size 0.2 considered low, 0.5 considered medium and 0.8 considered high. Pearson’s Chi2 was used for nominal data. When correlating data, Pearson’s correlation coefficient was used for parametric data, otherwise Spearman’s rho was used.
Our primary hypothesis was tested in two steps. First, striatal volume changes over time were tested with a repeated measure analysis. Significant group-by-time interactions were further investigated with post-hoc t-tests. The repeated measures analysis was initially performed for striatum, and afterward we separately analyzed the striatal subregions, i.e., caudate nucleus, putamen and nucleus accumbens. Second, we applied a multiple regression analysis to investigate the individual predictive effect of a set of variables on striatal volume increase, whilst controlling for the following included variables: amisulpride dose, striatal receptor occupancy, and baseline PANSS positive score. PANSS baseline positive scores were included in the model to control for the disease severity. Assumptions of normal distribution and no multicollinearity (Variance Inflation Factor <10) were met. If variables were initially non-normally distributed, they were transformed to normal distributions using log10- or square root functions.
Finally, we explored Spearman correlations between changes in symptom severity, striatal volumes, amisulpride dose, and D2/3R occupancy. Explored correlations were Bonferroni corrected for number of hypotheses tested on the same data, with a threshold of α/m, where α-level was set at 0.05, and m was number of hypotheses tested. For all other analyses, a two-sided p-value less than 0.05 was accepted as significant.
Results
Patients Compared to Healthy Controls
We included 21 patients and 23 controls with full datasets in our analyses (Supplementary Figure S1). Patients had higher use of tobacco and fewer years of education compared to controls (Table 1) but did not differ in other demographic factors. No difference in mean striatal volumes between patients and controls was found at baseline (p = 0.82) or at follow-up (p = 0.28). No difference in mean specific binding potentials to dopamine D2/3R was found between patients (2.49 ± 0.82) and controls (2.68 ± 0.71) (p = 0.25).
Symptom Severity and Receptor Occupancy in Patients After Treatment
After six weeks of treatment, patients’ PANSS total-, positive- and general symptom scores were significantly decreased, but negative symptoms were not (Table 1). Patients were treated with a mean dose of 233.3 (SD = 109.9) mg amisulpride. Oral dose and s-amisulpride correlated positively (r2 = 0.76, p < 0.001). Mean receptor occupancy was 44.65% (SD = 18.7%) and correlated positively with oral dose (r2 = 0.60, p = 0.004) and s-amisulpride (r2 = 0.68, p = 0.001). Receptor occupancy is illustrated in Figure 1. Amisulpride dose did not correlate with symptom severity (PANSS total) at baseline (r2 = 0.292, p = 0.199).
Striatal Volume Increase Is Predicted by Amisulpride Dose, But Not D2/3R Occupancy
The repeated measures analysis revealed no volume difference between groups at either time-point, but instead a significant group-by-time interaction was observed (p = 0.01). The post hoc analysis revealed that the interaction was driven by a significant volume increase in striatum of 2.1% (95% CI = 0.52–3.68%, p = 0.01, Cohens d = 0.45) in patients. Sub-regional increases were observed in left and right caudate nucleus (2.6%) and right putamen (2.4%) (Table 2). The multiple regression model significantly predicted striatal volume increase (r2 = 0.411, p = 0.026) (Figure 2), with amisulpride oral dose as the only unique, predictive factor (beta = 0.553, p = 0.028) (Supplementary Table S1).
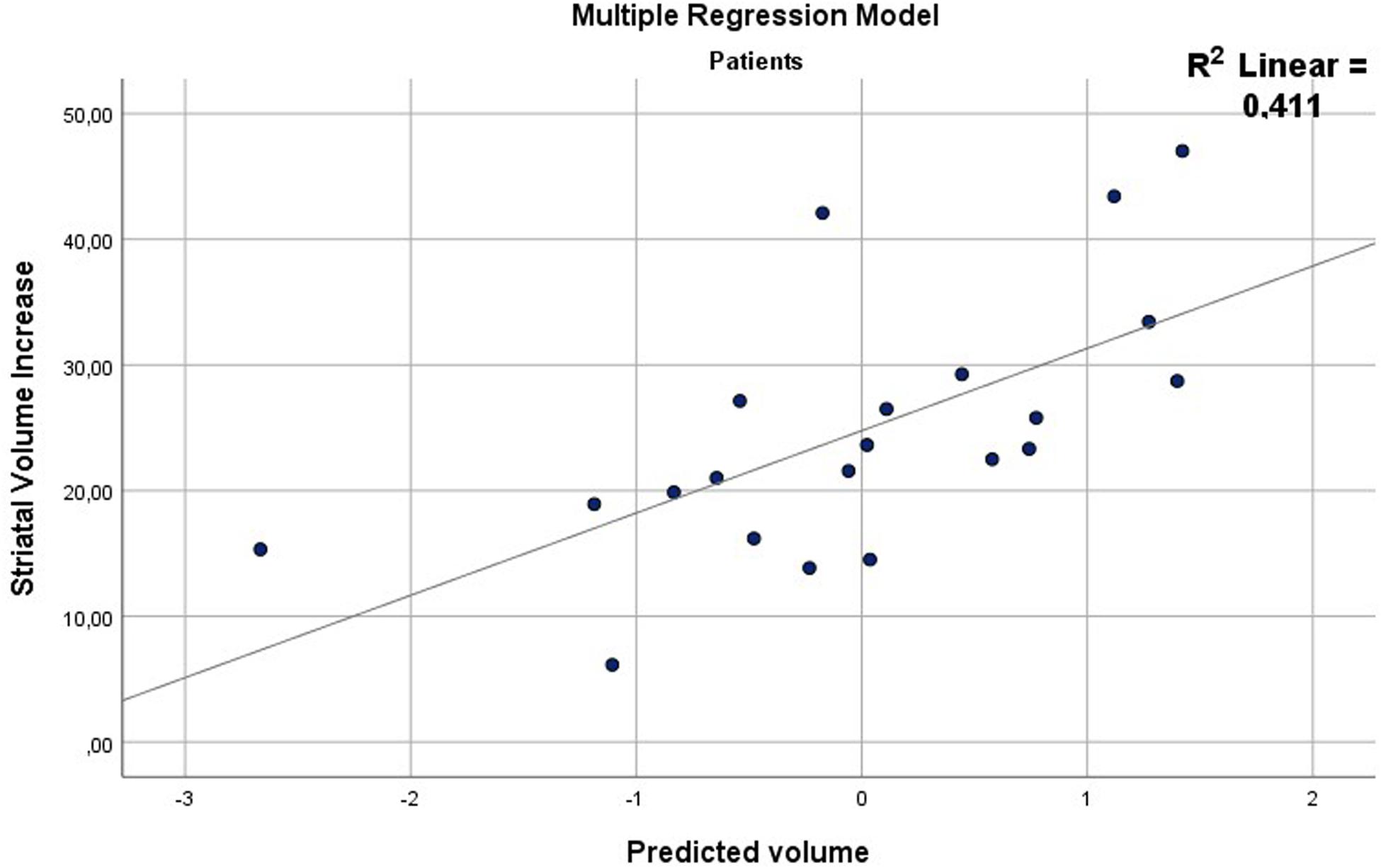
Figure 2. Scatter plot of the multiple regression model including dose, striatal receptor occupancy and PANSS positive score as predictive/independent variables. The dependent variable, striatal volume increase, is depicted on the y-axis, the independent variables on the x-axis. The model significantly predicted striatal volume increase (r2 = 0.411, p = 0.026). Only dose was a unique predictor of volume increase when controlling for the other variables (r2 = 0.553, p = 0.028). All model coefficients can be seen in Supplementary Table S1.
Symptom Severity Exploratory Correlations
Reduction in positive symptoms correlated significantly with striatal volume increase (r2 = −0.472, p = 0.031) and this correlation was driven by a reduction in hallucinations (r2 = −0.515, p = 0.017). The correlations did not survive Bonferroni correction. Changes in PANSS total- or subscores did not correlate to either amisulpride dose, s-amisulpride or receptor occupancy.
Discussion
Primary Findings
In line with our hypothesis, we found a significant volume increase in striatum in patients (2.1%) with a medium effect size (Cohens d = 0.45) after six weeks of amisulpride treatment. Our predictive model showed that dose was a predictor of volume increase, but positive symptom severity at baseline and D2/3R occupancy were not. Our exploratory correlation analyses indicated that striatal volume increase was associated with an improvement in positive symptoms, particularly hallucinations.
Results Compared to Previous Findings
Structural brain differences between patients and healthy controls at the time of diagnosis have previously been reported, but are not consistently replicated, and often no differences are found, indicating that changes are subtle (Glenthoj et al., 2007; Puri, 2010; Dietsche et al., 2017). Consistent with this, we did not find any significant differences in striatal volumes between patients and healthy controls. Specific D2/3R binding potentials did not differ between patients and controls prior to treatment, which is a replication of previous findings (Howes et al., 2009; Salavati et al., 2015). In this study, mean amisulpride dose was relatively low (233.3 mg), and approximately half of the dose used in phase one of the OPTiMiSE study (488.0 mg for completers) (Kahn et al., 2018). However, included patients in the OPTiMiSE study were not all antipsychotic-naïve, and due to a potential compensatory upregulation of D2R in response to antipsychotic treatment (Oda et al., 2015; Yin et al., 2017), this could explain the need for higher treatment doses compared to our antipsychotic-naïve patients. Furthermore, medication against adverse symptoms was not allowed in this study, which made clinicians upregulate dose slowly.
Treatment dose and blood levels are inherently linked to occupancy, and it is generally accepted that a striatal dopamine receptor occupancy of 65–80% is necessary for clinical response (Uchida et al., 2011; Yilmaz et al., 2012). However, we found a significant decrease in symptom severity at mean receptor occupancy-levels of 44.65%. Most recent studies investigating occupancy levels in patients use estimations, but a cautious comparison can be made to the CATIE data (Moriguchi et al., 2013). Authors found that for patients in stable remission, approximately half did not have continuous dopamine D2 blockade of ≥65%. A prospective PET study further found an optimal therapeutic window between 50 and 60% receptor occupancy on clinically stable patients with late-life schizophrenia (Graff-Guerrero et al., 2015). Altogether, this indicates that lower doses/D2 receptor occupancies in selected patient populations are sufficient, and also reduce risk of adverse effects such as extrapyramidal symptoms.
We found no correlation between reduction in positive symptoms and dose or occupancy as previously found for amisulpride (Sparshatt et al., 2009) and other antipsychotics (Yilmaz et al., 2012), but a negative finding has also been reported (Batail et al., 2014). Different patient groups, different antipsychotics and different methodology makes results difficult to compare. Considering that amisulpride in low doses has a higher affinity toward the presynaptic dopamine D2 auto receptors rather than post synaptic receptors (Rosenzweig et al., 2002), the discrepancy may be explained by the relatively low dose used in our study. We did, however, find a correlation between symptom reduction and volume increase. When specific positive symptoms were examined, this correlation was linked to a decrease in hallucinations. Similar results were found in a study by Li et al. (2012), in which PANSS decrease correlated to volume increase in putamen. Our results did not survive a Bonferroni correction and should be interpreted with caution. However, this plays well into hypotheses related to altered striatal structure and connectivity linked to symptom severity (Sarpal et al., 2015).
Basal Ganglia Volume Increases
Correlation between antipsychotic dose and volume changes in striatum is a subject of much debate (Roiz-Santianez et al., 2015; Huhtaniska et al., 2017) in part because separating the effect of disease and medication is inherently difficult. Vernon and colleagues found proof of concept in healthy rodent models, in which chronic (8 weeks) exposure to antipsychotics, but not other psychotropics (e.g., lithium) using clinically comparable dosing, leads to structural brain changes in naïve rats, including striatal enlargement (Vernon et al., 2012). In line with these data, we found a dose-dependent volume increase in the striatum after six weeks of treatment with an atypical APD with predominant D2/3R blockade. It is still, however, unknown what causes this volume increase. Investigation has been made into the cellular components of the volume increase, but linking structural MR changes to their cellular correlates is challenging, and although antipsychotic exposure has been found to moderate microglial activation, neuronal dendritic spine density and astrocytes (Vernon et al., 2014; Cotel et al., 2015; Amato et al., 2017), no studies to date have linked any of these changes to striatal volume increase.
Another explanation for the volume increase could be augmented blood flow to striatum. This was found in a functional MRI study in healthy males after one dose of APD (Hawkins et al., 2018), as well as in patients treated with a mean of 27 days (Corson et al., 2002). Increased blood flow could possibly lead to an “apparent” volume change, but the difference in flow did not seem to have an impact on volume changes or brain structure investigated by Hawkins et al. (2018). Notably, Vernon et al. (2012) reported that striatal volume increases in rodents chronically exposed to haloperidol (8 weeks) was normalized after an equivalent period of drug washout (Vernon et al., 2012). The same tendencies of volume decrease in putamen after withdrawal of antipsychotics was reported from a small schizophrenia patient cohort (Boonstra et al., 2011). Finally, our previous functional MRI study on a subset of the current cohort showed changes in the task-related blood oxygen level-dependent activation in striatal regions after amisulpride treatment (Nielsen et al., 2012). Collectively, these data suggest that the effects of antipsychotics on brain structure, including the basal ganglia, are dynamic and potentially reversible.
It has long been discussed whether striatal volume changes are specific to so-called typical antipsychotics (Ebdrup et al., 2013), but our findings together with (Jorgensen et al., 2016) and (Glenthoj et al., 2007) show that this is not the case. The assumption may have been due to striatal volume decreases seen in patients treated with atypical clozapine (Garcia et al., 2015; Jorgensen et al., 2016) or quetiapine (Ebdrup et al., 2011). Both drugs, however, have low affinity toward dopamine D2-like receptors, whereas typical antipsychotics have high affinities (Creese et al., 1976; Kusumi et al., 2015; Jorgensen et al., 2016). Dopamine D2-like receptor knock-out mice also show striatal volume increases, mirroring the effects of chronic exposure (9 weeks) to different APDs (Guma et al., 2018, 2019). Notably, when chronically exposed to the same antipsychotics as wild-type mice, no additional basal ganglia volume increases were found. Taken together, these data strongly support that volume increases following antipsychotic exposure are mediated via the dopamine D2-like receptor (Guma et al., 2018, 2019), rather than depending on the drug-class (‘typical’ vs. ‘atypical’ antipsychotic).
Dopamine Receptor Occupancy
We expected the volume increase to be predicted by striatal dopamine D2/3R occupancy, because occupancy may be considered a more direct measure of effect than oral dose. This was not the case. We speculate that it may be due to several issues regarding SPECT imaging. First, receptor occupancy is calculated as the difference in available dopamine receptors between baseline and follow-up, and is therefore subjective to interfering factors such as changes in endogenous dopamine levels, which in turn affect dopamine receptor availability and occupancy. Second, as previously mentioned, studies also suggest a possible compensatory upregulation of D2R in response to treatment (Oda et al., 2015; Yin et al., 2017), which again may affect occupancy, and a potentially decreased effect of drug dose in the long-term. Third, SPECT measurements are subjective to noise, which may have obscured a potential true correlation. Another issue to consider is that the multiple regression analysis assumes linearity, which might not be the case between volume increase and receptor occupancy. Lastly, a measure of cumulative dose (although likely correlated to the mean dose) might have been a more accurate measure, but unfortunately not possible within our study. To our knowledge, only one study has done a similar investigation, also reporting no association between occupancy and volume increase (Di Sero et al., 2019). However, since amisulpride primarily acts by blocking D2/3R, we argue that the observed striatal volumetric increases still can be mediated through occupancy, and we assign the negative association with occupancy to the aforementioned issues.
Strengths and Limitations
We conducted a clinically challenging prospective study on a cohort of antipsychotic-naïve first-episode schizophrenia patients and matched, healthy controls. Men and women were equally represented and confounding effects of previous exposure to antipsychotics could be ruled out. Amisulpride was chosen for treatment because of its selectivity toward dopamine D2/3R, thereby excluding potential involvement of other neuroreceptors.
Because of the extensive examination program, the study included a limited sample of patients, and therefore selection bias cannot be ruled out. On the other hand, with a mean baseline PANSS total score of 78.5, the patients in our study may be considered moderately ill (Leucht et al., 2005). The limited number of patients restricted the degrees of freedom in the multivariate linear regression model, and therefore it was not possible to include- and control for further variables in our analyses. The effect of nicotine on basal ganglia volumes is unresolved (Van Haren et al., 2010; Das et al., 2012).
Conclusion
We found a dose-dependent striatal volume increase in antipsychotic-naïve schizophrenia patients in response to six weeks dopamine D2/3 receptor blockade with an atypical antipsychotic compound. Thus, our findings contrast the notion that striatal volume increase is restricted to “typical” antipsychotics. However, the underlying mechanisms warrant further investigation. We found the striatal volume increase to be clinically relevant, since it appears correlated to a reduction in positive symptoms.
Data Availability Statement
The datasets generated for this study are available on request to the corresponding author.
Ethics Statement
The study was conducted in accordance with the Helsinki declaration II, and approved by the Danish research ethics committee (H-D-2008-088), as well as the Danish Data Committee (RHP-2016-025, I-suite no. 05181). Clinical Trials.gov Identifier: NCT01154829. The patients/participants provided their written informed consent to participate in this study.
Author Contributions
BG and BE conceived and designed the study. SW and MN collected the data. JR, CS, SW, ER, LJ, PA, and LP contributed with data processing and analysis tools. HA, JR, LBJ, ER and BE conducted the statistical analyses. HA and BE drafted the manuscript. HA, JR, CS, and SW particularly contributed with method section. AV contributed to the interpretation and discussion. All authors fulfill authorship criteria of the ICMJE by substantial contribution to the conception and design, to acquisition of data, or to the analysis and interpretation of the data contributed to manuscript revision, read and approved the submitted version.
Funding
HA was funded by a scholarship from the Lundbeck Foundation and a grant from A. P. Møller Fonden. The Centre for Clinical Intervention and Neuropsychiatric Schizophrenia Research, CINS is funded by Lundbeck Foundation grant number R25-A2701.
Conflict of Interest
BE has received lecture fees and/or is part of Advisory Boards of Bristol-Myers Squibb, Eli Lilly and Company, Janssen-Cilag, Otsuka Pharma Scandinavia AB, Takeda Pharmaceutical Company and Lundbeck Pharma A/S. BG is the leader of a Lundbeck Foundation Centre of Excellence for Clinical Intervention and Neuropsychiatric Schizophrenia Research (CINS), which is partially financed by an independent grant from the Lundbeck Foundation based on international review and partially financed by the Mental Health Services in the Capital Region of Denmark, the University of Copenhagen, and other foundations. Her group has also received a research grant from Lundbeck A/S for another independent investigator-initiated study. All grants are the property of the Mental Health Services in the Capital Region of Denmark and administrated by them. She has no other conflicts to disclose.
The remaining authors declare that the research was conducted in the absence of any commercial or financial relationships that could be construed as a potential conflict of interest.
Acknowledgments
We would like to thank patients and controls for their participation in this study, and the Lundbeck Foundation for sponsoring the scholar-stipendium to HA. We would also like to thank Anthony Vernon for accepting a visit from HA at the Maurice Wohl Neuroscience Institute.
Supplementary Material
The Supplementary Material for this article can be found online at: https://www.frontiersin.org/articles/10.3389/fnins.2020.00484/full#supplementary-material
References
Amato, D., Beasley, C. L., Hahn, M. K., and Vernon, A. C. (2017). Neuroadaptations to antipsychotic drugs: insights from pre-clinical and human post-mortem studies. Neurosci. Biobehav. Rev. 76, 317–335. doi: 10.1016/j.neubiorev.2016.10.004
Barnas, C., Quiner, S., Tauscher, J., Hilger, E., Willeit, M., Küfferle, B., et al. (2001). In vivo 123I IBZM SPECT imaging of striatal dopamine 2 receptor occupancy in schizophrenic patients. Psychopharmacology 157, 236–242. doi: 10.1007/s002130100813
Batail, J.-M., Langrée, B., Robert, G., Bleher, S., Verdier, M.-C., Bellissant, E., et al. (2014). Use of very-high-dose olanzapine in treatment-resistant schizophrenia. Schizophr. Res. 159, 411–414. doi: 10.1016/j.schres.2014.09.020
Boonstra, G., van Haren, N. E. M., Schnack, H. G., Cahn, W., Burger, H., Boersma, M., et al. (2011). Brain volume changes after withdrawal of atypical antipsychotics in patients with first-episode schizophrenia. J. Clin. Psychopharmacol. 31, 146–153. doi: 10.1097/JCP.0b013e31820e3f58
Brandt, G. N., and Bonelli, R. M. (2008). Structural neuroimaging of the basal ganglia in schizophrenic patients: a review. Wien. Med. Wochenschr. 158, 84–90. doi: 10.1007/s10354-007-0478-7
Brugger, S. P., and Howes, O. D. (2017). Heterogeneity and homogeneity of regional brain structure in schizophrenia: a meta-analysis. JAMA psychiatry 74, 1104–1111. doi: 10.1001/jamapsychiatry.2017.2663
Brunelin, J., Fecteau, S., and Suaud-Chagny, M.-F. (2013). Abnormal striatal dopamine transmission in schizophrenia. Curr. Med. Chem. 20, 397–404. doi: 10.2174/0929867311320030011
Corson, P. W., O’leary, D. S., Miller, D. D., and Andreasen, N. C. (2002). The effects of neuroleptic medications on basal ganglia blood flow in schizophreniform disorders: a comparison between the neuroleptic-naïve and medicated states. Biol. Psychiatry 52, 855–862. doi: 10.1016/s0006-3223(02)01421-x
Cotel, M.-C., Lenartowicz, E. M., Natesan, S., Modo, M. M., Cooper, J. D., Williams, S. C. R., et al. (2015). Microglial activation in the rat brain following chronic antipsychotic treatment at clinically relevant doses. Eur. Neuropsychopharmacol. 25, 2098–2107. doi: 10.1016/j.euroneuro.2015.08.004
Creese, I., Burt, D., and Snyder, S. (1976). Dopamine receptor binding predicts clinical and pharmacological potencies of antischizophrenic drugs. Science 192, 481–483. doi: 10.1126/science.3854
Das, D., Cherbuin, N., Anstey, K. J., Sachdev, P. S., and Easteal, S. (2012). Lifetime cigarette smoking is associated with striatal volume measures. Addict. Biol. 17, 817–825. doi: 10.1111/j.1369-1600.2010.00301.x
Di Sero, A., Jørgensen, K. N., Nerland, S., Melle, I., Andreassen, O. A., Jovicich, J., et al. (2019). Antipsychotic treatment and basal ganglia volumes: exploring the role of receptor occupancy, dosage and remission status. Schizophr. Res. 208, 114–123. doi: 10.1016/j.schres.2019.04.002
Dietsche, B., Kircher, T., and Falkenberg, I. (2017). Structural brain changes in schizophrenia at different stages of the illness: a selective review of longitudinal magnetic resonance imaging studies. Aust. N. Z. J. Psychiatry 51, 500–508. doi: 10.1177/0004867417699473
Ebdrup, B. H., Nørbak, H., Borgwardt, S., and Glenthøj, B. (2013). Volumetric changes in the basal ganglia after antipsychotic monotherapy: a systematic review. Curr. Med. Chem. 20, 438–447. doi: 10.2174/0929867311320030015
Ebdrup, B. H., Skimminge, A., Rasmussen, H., Aggernaes, B., Oranje, B., Lublin, H., et al. (2011). Progressive striatal and hippocampal volume loss in initially antipsychotic-naive, first-episode schizophrenia patients treated with quetiapine: relationship to dose and symptoms. Int. J. Neuropsychopharmacol. 14, 69–82. doi: 10.1017/S1461145710000817
Fusar-Poli, P., Smieskova, R., Kempton, M. J., Ho, B. C., Andreasen, N. C., and Borgwardt, S. (2013). Progressive brain changes in schizophrenia related to antipsychotic treatment? A meta-analysis of longitudinal MRI studies. Neurosci. Biobehav. Rev. 37, 1680–1691. doi: 10.1016/j.neubiorev.2013.06.001
Garcia, G. J., Chagas, M. H., Silva, C. H., Machado-De-Sousa, J. P., Crippa, J. A., and Hallak, J. E. (2015). Structural and functional neuroimaging findings associated with the use of clozapine in schizophrenia: a systematic review. Rev. Bras. Psiquiatr. 37, 71–79. doi: 10.1590/1516-4446-2014-1387
Glenthoj, A., Glenthoj, B. Y., Mackeprang, T., Pagsberg, A. K., Hemmingsen, R. P., Jernigan, T. L., et al. (2007). Basal ganglia volumes in drug-naive first-episode schizophrenia patients before and after short-term treatment with either a typical or an atypical antipsychotic drug. Psychiatry Res. Neuroimaging 154, 199–208. doi: 10.1016/j.pscychresns.2006.10.002
Golan, D. E. (2012). Principles of Pharmacology: The Pathophysiologic Basis of Drug Therapy, 3rd Edn. Philadelphia, PA: Lippincott Williams & Wilkins, 132–146.
Graff-Guerrero, A., Rajji, T. K., Mulsant, B. H., Nakajima, S., Caravaggio, F., Suzuki, T., et al. (2015). Evaluation of antipsychotic dose reduction in late-life schizophrenia: a prospective dopamine D2/3 receptor occupancy study. JAMA Psychiatry 72, 927–934. doi: 10.1001/jamapsychiatry.2015.0891
Guma, E., Rocchetti, J., Devenyi, G. A., Tanti, A., Mathieu, A., Lerch, J. P., et al. (2018). Regional brain volume changes following chronic antipsychotic administration are mediated by the dopamine D2 receptor. Neuroimage 176, 226–238. doi: 10.1016/j.neuroimage.2018.04.054
Guma, E., Rocchetti, J., Devenyi, G. A., Tanti, A., Mathieu, A. P., Lerch, J. P., et al. (2019). Role of D3 dopamine receptors in modulating neuroanatomical changes in response to antipsychotic administration. Sci. Rep. 9:7850. doi: 10.1038/s41598-019-43955-4
Haijma, S. V., Van Haren, N., Cahn, W., Koolschijn, P. C. M. P., Hulshoff Pol, H. E., and Kahn, R. S. (2013). Brain volumes in schizophrenia: a meta-analysis in over 18 000 subjects. Schizophr. Bull. 39, 1129–1138. doi: 10.1093/schbul/sbs118
Hawkins, P. C. T., Wood, T. C., Vernon, A. C., Bertolino, A., Sambataro, F., Dukart, J., et al. (2018). An investigation of regional cerebral blood flow and tissue structure changes after acute administration of antipsychotics in healthy male volunteers. Hum. Brain Mapp. 39, 319–331. doi: 10.1002/hbm.23844
Howes, O., Egerton, A., Allan, V., McGuire, P., Stokes, P., and Kapur, S. (2009). Mechanisms underlying psychosis and antipsychotic treatment response in schizophrenia: insights from PET and SPECT Imaging. Curr. Pharm. Des. 15, 2550–2559. doi: 10.2174/138161209788957528
Howes, O. D., Kambeitz, J., Kim, E., Stahl, D., Slifstein, M., Abi-Dargham, A., et al. (2012). The nature of dopamine dysfunction in schizophrenia and what this means for treatment. Arch. Gen. Psychiatry 69, 776–786. doi: 10.1001/archgenpsychiatry.2012.169
Howes, O. D., and Kapur, S. (2009). The dopamine hypothesis of schizophrenia: version III–the final common pathway. Schizophr. Bull. 35, 549–562. doi: 10.1093/schbul/sbp006
Huhtaniska, S., Jääskeläinen, E., Hirvonen, N., Remes, J., Murray, G. K., Veijola, J., et al. (2017). Long-term antipsychotic use and brain changes in schizophrenia – a systematic review and meta-analysis. Hum. Psychopharmacol. 32:e2574. doi: 10.1002/hup.2574
Jorgensen, K. N., Nesvag, R., Gunleiksrud, S., Raballo, A., Jonsson, E. G., and Agartz, I. (2016). First- and second-generation antipsychotic drug treatment and subcortical brain morphology in schizophrenia. Eur. Arch. Psychiatry Clin. Neurosci. 266, 451–460. doi: 10.1007/s00406-015-0650-9
Kahn, R. S., Winter van Rossum, I., Leucht, S., McGuire, P., Lewis, S. W., Leboyer, M., et al. (2018). Amisulpride and olanzapine followed by open-label treatment with clozapine in first-episode schizophrenia and schizophreniform disorder (OPTiMiSE): a three-phase switching study. Lancet Psychiatry 5, 797–807. doi: 10.1016/S2215-0366(18)30252-9
Kapur, S. (2003). Psychosis as a state of aberrant salience: a framework linking biology, phenomenology, and pharmacology in schizophrenia. Am. J. Psychiatry 160, 13–23. doi: 10.1176/appi.ajp.160.1.13
Kay, S. R., Fiszbein, A., and Opler, L. A. (1987). The positive and negative syndrome scale (PANSS) for schizophrenia. Schizophr. Bull. 13, 261–276. doi: 10.1093/schbul/13.2.261
Kung, H. F., Alavi, A., Chang, W., Kung, M. P., Keyes, J. W., Velchik, M. G., et al. (1990). In vivo SPECT imaging of CNS D-2 dopamine receptors: initial studies with iodine-123-IBZM in humans. J. Nucl. Med. 31, 573–579.
Kusumi, I., Boku, S., and Takahashi, Y. (2015). Psychopharmacology of atypical antipsychotic drugs: from the receptor binding profile to neuroprotection and neurogenesis. Psychiatry Clin. Neurosci. 69, 243–258. doi: 10.1111/pcn.12242
Leucht, S., Kane, J. M., Kissling, W., Hamann, J., Etschel, E., and Engel, R. R. (2005). What does the PANSS mean? Schizophr. Res. 79, 231–238. doi: 10.1016/j.schres.2005.04.008
Li, M., Chen, Z., Deng, W., He, Z., Wang, Q., Jiang, L., et al. (2012). Volume increases in putamen associated with positive symptom reduction in previously drug-naive schizophrenia after 6 weeks antipsychotic treatment. Psychol. Med. 42, 1475–1483. doi: 10.1017/S0033291711002157
Moriguchi, S., Bies, R. R., Remington, G., Suzuki, T., Mamo, D. C., Watanabe, K., et al. (2013). Estimated dopamine D2 receptor occupancy and remission in schizophrenia: analysis of the CATIE data. J. Clin. Psychopharmacol. 33, 682–685. doi: 10.1097/JCP.0b013e3182979a0a
Nielsen, M. O., Rostrup, E., Wulff, S., Bak, N., Broberg, B. V., Lublin, H., et al. (2012). Improvement of brain reward abnormalities by antipsychotic monotherapy in schizophrenia. Arch. Gen. Psychiatry 69, 1195–1204. doi: 10.1001/archgenpsychiatry.2012.847
Oda, Y., Kanahara, N., and Iyo, M. (2015). Alterations of dopamine D2 receptors and related receptor-interacting proteins in schizophrenia: the pivotal position of dopamine supersensitivity psychosis in treatment-resistant schizophrenia. Int. J. Mol. Sci. 16, 30144–30163. doi: 10.3390/ijms161226228
Patenaude, B., Smith, S. M., Kennedy, D. N., and Jenkinson, M. (2011). A Bayesian model of shape and appearance for subcortical brain segmentation. Neuroimage 56, 907–922. doi: 10.1016/j.neuroimage.2011.02.046
Puri, B. K. (2010). Progressive structural brain changes in schizophrenia. Expert Rev. Neurother. 10, 33–42. doi: 10.1586/ern.09.142
Roiz-Santianez, R., Suarez-Pinilla, P., and Crespo-Facorro, B. (2015). Brain structural effects of antipsychotic treatment in schizophrenia: a systematic review. Curr. Neuropharmacol. 13, 422–434. doi: 10.2174/1570159x13666150429002536
Rosenzweig, P., Canal, M., Patat, A., Bergougnan, L., Zieleniuk, I., and Bianchetti, G. (2002). A review of the pharmacokinetics, tolerability and pharmacodynamics of amisulpride in healthy volunteers. Hum. Psychopharmacol. Clin. Exp. 17, 1–13. doi: 10.1002/hup.320
Salavati, B., Rajji, T. K., Price, R., Sun, Y., Graff-Guerrero, A., and Daskalakis, Z. J. (2015). Imaging-based neurochemistry in schizophrenia: a systematic review and implications for dysfunctional long-term potentiation. Schizophr. Bull. 41, 44–56. doi: 10.1093/schbul/sbu132
Sarpal, D. K., Robinson, D. G., Lencz, T., Argyelan, M., Ikuta, T., Karlsgodt, K., et al. (2015). Antipsychotic treatment and functional connectivity of the striatum in first-episode schizophrenia. JAMA Psychiatry 72, 5–13. doi: 10.1001/jamapsychiatry.2014.1734
Sparshatt, A., Taylor, D., Patel, M. X., and Kapur, S. (2009). Amisulpride – dose, plasma concentration, occupancy and response: implications for therapeutic drug monitoring. Acta Psychiatr. Scand. 120, 416–428. doi: 10.1111/j.1600-0447.2009.01429.x
Svarer, C., Madsen, K., Hasselbalch, S. G., Pinborg, L. H., Haugbøl, S., Frøkjær, V. G., et al. (2005). MR-based automatic delineation of volumes of interest in human brain PET images using probability maps. Neuroimage 24, 969–979. doi: 10.1016/j.neuroimage.2004.10.017
Uchida, H., Takeuchi, H., Graff-Guerrero, A., Suzuki, T., Watanabe, K., and Mamo, D. C. (2011). Dopamine D2 receptor occupancy and clinical effects: a systematic review and pooled analysis. J. Clin. Psychopharmacol. 31, 497–502. doi: 10.1097/JCP.0b013e3182214aad
van Erp, T. G. M., Hibar, D. P., Rasmussen, J. M., Glahn, D. C., Pearlson, G. D., Andreassen, O. A., et al. (2016). Subcortical brain volume abnormalities in 2028 individuals with schizophrenia and 2540 healthy controls via the ENIGMA consortium. Mol. Psychiatry 21, 547–553. doi: 10.1038/mp.2015.63
Van Haren, N. E. M., Koolschijn, P. C. M. P., Cahn, W., Schnack, H. G., Hulshoff Pol, H. E., and Kahn, R. S. (2010). Cigarette smoking and progressive brain volume loss in schizophrenia. Eur. Neuropsychopharmacol. 20, 454–458. doi: 10.1016/j.euroneuro.2010.02.009
Vernon, A. C., Crum, W. R., Lerch, J. P., Chege, W., Natesan, S., Modo, M., et al. (2014). Reduced cortical volume and elevated astrocyte density in rats chronically treated with antipsychotic drugs – linking magnetic resonance imaging findings to cellular pathology. Biol. Psychiatry 75, 982–990. doi: 10.1016/j.biopsych.2013.09.012
Vernon, A. C., Natesan, S., Crum, W. R., Cooper, J. D., Modo, M., Williams, S. C. R., et al. (2012). Contrasting effects of haloperidol and lithium on rodent brain structure: a magnetic resonance imaging study with postmortem confirmation. Biol. Psychiatry 71, 855–863. doi: 10.1016/j.biopsych.2011.12.004
Waschke, J., and Paulsen, F. (2011). Sobotta, Atlas of Human Anatomy: Head, Neck and Neuroanatomy, 15th Edn. Munich: Urban & Fischer.
Willendrup, P., Pinborg, L. H., Hasselbalch, S. G., Adams, K. H., Stahr, K., Knudsen, G. M., et al. (2004). Assessment of the precision in co-registration of structural MR images and PET images with localized binding. Int. Congr. Ser. 1265, 275–280. doi: 10.1016/j.ics.2004.04.065
Wulff, S., Nielsen, M. Ø, Rostrup, E., Svarer, C., Jensen, L. T., Pinborg, L., et al. (2019). The relation between dopamine D2 receptor blockade and the brain reward system: a longitudinal study of first-episode schizophrenia patients. Psychol. Med. 50, 220–228. doi: 10.1017/S0033291718004099
Wulff, S., Pinborg, L. H., Svarer, C., Jensen, L. T., Nielsen, M. Ø, Allerup, P., et al. (2015). Striatal D2/3 binding potential values in drug-naïve first-episode schizophrenia patients correlate with treatment outcome. Schizophr. Bull. 41, 1143–1152. doi: 10.1093/schbul/sbu220
Yilmaz, Z., Zai, C. C., Hwang, R., Mann, S., Arenovich, T., Remington, G., et al. (2012). Antipsychotics, dopamine D2 receptor occupancy and clinical improvement in schizophrenia: a meta-analysis. Schizophr. Res. 140, 214–220. doi: 10.1016/j.schres.2012.06.027
Keywords: schizophrenia, dopamine receptor, first-episode antipsychotic-naïve, striatum, SPECT, sMRI, antipsychotic drug, longitudinal
Citation: Andersen HG, Raghava JM, Svarer C, Wulff S, Johansen LB, Antonsen PK, Nielsen MØ, Rostrup E, Vernon AC, Jensen LT, Pinborg LH, Glenthøj BY and Ebdrup BH (2020) Striatal Volume Increase After Six Weeks of Selective Dopamine D2/3 Receptor Blockade in First-Episode, Antipsychotic-Naïve Schizophrenia Patients. Front. Neurosci. 14:484. doi: 10.3389/fnins.2020.00484
Received: 31 January 2020; Accepted: 20 April 2020;
Published: 20 May 2020.
Edited by:
Andrew J. Grottick, Beacon Discovery Inc., United StatesReviewed by:
Doris Doudet, University of British Columbia, CanadaRobert Warren Gould, Wake Forest School of Medicine, United States
Copyright © 2020 Andersen, Raghava, Svarer, Wulff, Johansen, Antonsen, Nielsen, Rostrup, Vernon, Jensen, Pinborg, Glenthøj and Ebdrup. This is an open-access article distributed under the terms of the Creative Commons Attribution License (CC BY). The use, distribution or reproduction in other forums is permitted, provided the original author(s) and the copyright owner(s) are credited and that the original publication in this journal is cited, in accordance with accepted academic practice. No use, distribution or reproduction is permitted which does not comply with these terms.
*Correspondence: Bjørn H. Ebdrup, bebdrup@cnsr.dk