- 1Department of Neurology, Affiliated Nanhua Hospital, University of South China, Hengyang, China
- 2Department of Neurology, The First Affiliated Hospital, University of South China, Hengyang, China
- 3Institute of Neurology, The First Affiliated Hospital, University of South China, Hengyang, China
- 4Department of Physiology, Hengyang Medical College, University of South China, Hengyang, China
Sleep deprivation (SD) induces hippocampal damage. Hydrogen sulfide (H2S) is a neuronal protective factor. Silence information regulating factor 1 (Sirt1) plays an important role in neuroprotection. Therefore, this study was aimed at exploring whether H2S meliorates SD-induced hippocampal damage and whether Sirt1 mediates this protective role of H2S. We found that sodium hydrosulfide (NaHS, a donor of H2S) alleviated SD-generated hippocampal oxidative stress, including increases in the activation of SOD and the level of GSH as well as a decrease in the level of MDA. Meanwhile, we found that NaHS reduced SD-exerted hippocampal endoplasmic reticulum (ER) Stress, including downregulations of GRP78, CHOP, and cleaved-caspase-12 expression. Moreover, NaHS reduced the apoptosis in the SD-exposed hippocampus, and this included decreases in the number of apoptotic cells and the activation of caspase-3, downregulation of Bax expression, and upregulation of Bcl-2 expression. NaHS upregulated the expression of Sirt1 in the hippocampus of SD-exposed rats. Furthermore, Sirtinol, the inhibitor of Sirt1, abrogated the protection of NaHS against SD-exerted hippocampal oxidative stress, ER stress, and apoptosis. These results suggested that H2S alleviates SD-induced hippocampal damage by upregulation of hippocampal Sirt1.
Introduction
Sleep is essential to the balance of human physical health. It has been shown that most adults do not get enough sleep (Centers for Disease and Prevention, 2011). Sleep deprivation (SD) causes physiological, psychological, and behavioral change (Walker and Stickgold, 2006; Ohayon et al., 2010; Dai et al., 2012), and it especially has an effect on the central nervous system (CNS) (Nabaee et al., 2018). In recent years, the effects of SD on cognitive function have attracted wide attention (Killgore, 2010; Kaliyaperumal et al., 2017; Nasehi et al., 2018). However, the underlying mechanisms of these effects remain unclear. The hippocampus, as a key component of the limbic systems, plays an important role in the regulation of cognition (Shohamy and Turk-Browne, 2013). The important role of the hippocampus for cognitive functions is well established (Squire and Zola, 1996; Eichenbaum, 2000). It has been demonstrated that paradoxical SD increases the hippocampal oxidative stress in rats (Singh et al., 2008). Moreover, SD increases endoplasmic reticulum (ER) stress (Hakim et al., 2015) and eventually leads to neuronal apoptosis in the brain (Qiu et al., 2016). In addition, SD causes hippocampal synaptic and membrane excitability changes, which indicated that SD determinately affects hippocampus damage (McDermott et al., 2003). Therefore, it is of great academic value to explore the potential therapeutic approaches for hippocampal damage, which may provide a new target for the treatment of SD-induced cognitive impairment.
Hydrogen sulfide (H2S), the third gasotransmitter after carbon monoxide (CO) and nitric oxide (NO) (Wang, 2010), exerts protective effect in the CNS (Zhang and Bian, 2014; Panthi et al., 2016; Elbassuoni and Nazmy, 2018). Emerging evidence has indicated that H2S has anti-oxidative, anti-ER stress, and antiapoptotic properties in the CNS (Jiang et al., 2011; Nie et al., 2013; Li et al., 2014; Zhang et al., 2014). Of note, our groups have demonstrated the protective effect of H2S against chronic unpredictable mild stress-induced oxidative stress and ER stress in the hippocampus (Hu et al., 2016; Liu et al., 2017). We previously provided evidence that H2S inhibits neuronal apoptosis in the hippocampus of homocysteine-induced rats (Wei et al., 2014). These data suggested that H2S plays an important role in counteracting hippocampal damage. Thus, we speculated that H2S ameliorates oxidative stress, ER stress, and apoptosis in the hippocampus of SD-treated rats.
Silence information regulating factor 1 (Sirt1), an NAD+ dependent class III histone deacetylases (Imai et al., 2000; Smith et al., 2000; Cohen et al., 2004), plays important roles in neuroprotection and neuroregulation (Han, 2009; Zhang et al., 2011; Donmez, 2012). It has been demonstrated that SD decreases the expression of Sirt1 in the hippocampus (Chang et al., 2009) and that Sirt1 deficiency induces hippocampal damage (Kim et al., 2018). Moreover, Sirt1 activation relieves ischemia-induced and D-galactose-induced hippocampal apoptosis (Meng et al., 2015; Wu et al., 2015). Furthermore, our previous study testified that H2S upregulates the expression of Sirt-1 in the hippocampus (Liu et al., 2017; Tang et al., 2018) and that H2S inhibits chronic restrain stress (CRS)-induced hippocampal damage in rats via upregulating the expression of Sirt-1 (Li et al., 2017). Therefore, we speculated that the protection of H2S against SD-induced hippocampal damage is also via upregulating hippocampal Sirt1.
In the present work, we demonstrated the suppressive effects of H2S on hippocampal oxidative Stress, ER stress, and apoptosis in SD-exposed rats. We also proved that H2S significantly upregulated Sirt1 expression in the hippocampus of SD-exposed rats. Furthermore, Sirtinol, a Sirt1 inhibitor, reversed the protective effects of H2S against SD-induced hippocampal oxidative Stress, ER stress, and apoptosis. Taken together, we identified a critical role of H2S on the protection against SD-induced hippocampal damage and the mediatory role of hippocampal Sirt1 in the protection of H2S against SD-induced hippocampal damage.
Materials and Methods
Reagents
Sodium hydrosulfide (NaHS, a donor of H2S), Sirtinol (a Specific Sirt-1 inhibitor), and Cleaved caspase-12-antibody were obtained from Sigma-Aldrich (St Louis, MO, United States). Pelltobarbitalum Natricum was bought from Germany. The Specific monoclonal antibodies of CPR78, CHOP, Bax Bcl2, and Sirt1 were purchased from Abcam (Hong Kong, China). The malondialdehyde (MDA) assay kit, superoxide dismutase (SOD) assay kit, glutathione (GSH) enzyme-linked immunosorbentassay (ELISA) kits, and caspase-3 Elisa Kit were purchased from USCN Life ScienceInc (Wuhan, Hubei, China).
Animals
The adult male Wistar rats (200 g∼220 g) were purchased from the SJA Lab Animal Center of Changsha (Changsha, Hunan, China). The rats were fed within single cage at a temperature of (25 ± 2)°C, constant humidity, natural illumination, and good ventilation. The rats were free to eat and drink, and the padding was changed regularly (two times a week). The subjects were touched for 3 min every day to eliminate the strangeness with the testers and to reduce the interference and influence of human factors and surrounding environment on the experimental rats. All the procedures and animal experiments were strictly conducted according to the National Institutes of Health Guide for the Care and Use of Laboratory Animals and were approved by the Animal Use and Protection Committee of University of South China.
Induction of Sleep Deprivation
The sleep deprivation model was established by the modified multiple platform method (MMPM) (Suchecki and Tufik, 2000). Animals were placed in a water tank (170 cm × 70 cm × 50 cm) that was filled with 19 small platforms (diameter 6.5 cm) surrounded by water (24 ± 1°C), and the horizontal plane was 1 cm below the small platform. The model was used to hinder the total sleep (especially REM sleep). When the rats entered REM sleep, muscle atonia caused animals to hit the water and wake up, leading to sleep deprivation (Machado et al., 2004). Rats were exposed to sleep deprivation for 72 h on the platform.
Drug Treatments and Experimental Schedule
After adaptation for 7 days, all rats were randomly divided into seven treatment groups: in the control group, rats were injected with PBS (i.p.) for 10 days; in the SD group, rats were exposed to the modified multiple platform method (MMPM) for 72 h; in the SD + NaHS (30 μmol/kg or 100 μmol/kg) group, rats were pretreated with NaHS (30 or 100 μmol/kg/d, ip) for 7days and then cotreated with SD for 72 h; in the SD + NaHS (100 μmol/kg) + Sirtinol (10 nm, i.c.v.) group, rats were pretreated with NaHS (100 μmol/kg/d, ip) for 7 days, and then cotreated with SD for 72 h and Sirtinol (10 nmol/d, icv) for 3 days; and in the NaHS (100 μmol/kg) alone group and Sirtinol alone group, rats were injected with NaHS (100 μmol/kg, 10 days, i.p.), and Sirtinol (10 nmol, 10 d, i.c.v.). NaHS was dissolved in Phosphate Buffered Saline (PBS), and Sirtinol (5 mg) was diluted in sterilized artificial CSF (ACSF)/0.5% DMSO. All rats were killed, and the hippocampi were rapidly collected and stored at −80°C for analysis.
Intracerebroventricular Injection
Rats were deeply anesthetized with 1% sodium pentobarbital (45 mg/kg, i.p.) and were then fixed on a stereotaxic apparatus for operation. The area around the incision was clipped by sterile surgical scissors. Sirtinol (10 nmol) was injected into the unilateral lateral ventricle using coordinates—AP: 1.0 mm, R or L: 1.5 mm—with an injection rate of 0.75 μL/min using a 10-μL Hamilton syringe. In order to guarantee the entire injection had been completely delivered, the needle was gradually pulled out halfway and kept in cannula for an extra 2 min before being removed. After the surgery, all rats were injected subcutaneously with administered penicillin (q.d.) for three consecutive days.
Biochemical Analysis for MDA, GSH, Caspase-3, and SOD
The hippocampus tissues were homogenized (10% w/v) with 0.1 mol/L of PBS and then centrifuged at 12,000 × g for 10 min. The supernatants were collected, and total protein concentrations were quantified using a BCA Protein Assay. The levels of MDA, GSH, and Caspase-3 were analyzed by ELISA kits. The activity of SOD was measured by the NBT assay kit. Specific steps were laid out according to the manufacturer’s instruction on the reagent kits.
Western Blot Detection for the Expressions of CPR78, CHOP, Cleaved Caspase-12, Bax, Bcl-2, and Sirt1 in the Hippocampus Tissue
Hippocampal tissue was removed and homogenized in an ice-cold homogenizing buffer (20 mM Tris-Cl, pH 7.4, 150 mM NaCl, 1 mM EDTA, 1% Triton X-100, 1 mM PMSF). After centrifugation at 12,000 × g for 30 min at 4°C, the supernatant was collected, and the protein content was subsequently assayed by using a BCA Protein Assay Kit (Beyotime, Shanghai, China). The protein was then diluted by PBS to the same concentration. The protein extract with an equivalent volume for each sample was run on sodium dodecyl sulfate-polyacrylamide gel electrophoresis. After that, the protein was transferred to a PVDF membrane using a wet transfer system and blocked with TBST (50 mmol/L Tris–HCl, pH 7.5,150 mmol/L NaCl, 0.1% Tween-20) for 2 h at room temperature. Then the PVDF membranes were irrespectively incubated with primary antibodies against GPR78, CHOP, Cleaved Caspase-12, Bcl-2, Bax, Sirt1 (1:1000), and β-actin (1:2000) overnight at 4°C. Next day, the membrane was washed three times with TBST (50 mmol/L Tris–HCl, pH 7.5,150 mmol/L NaCl, 0.1% Tween-20) for 15 min then incubated with secondary antibody (1:5000) for 2 h. Finally, Protein bands were analyzed through a developing system equipped with a software BIO-ID (Vilber Lourmat, France).
Tunel Staining
TUNEL staining was put into effect using an Apoptag Peroxidase In Situ Apoptosis Detection Kit S7100 (EMD Millipore, Billerica, MA, United States) on the basis of the manufacturer’s instructions. The cerebral sections of the CA3 area of hippocampus were incubated with the TUNEL reaction mixture at 37°C for 60 min, and they were also incubated with a proteinase K solution for 15 min at 37°C to enhance permeability, and then the sections were put into the 3% hydrogen peroxide solution prepared with methanol to block the endoperoxidase. Finally, they were treated with a DAB-substrate solution. Five hippocampus sections of each rat were selected. The sections of the CA3 area images were acquired using a fluorescent microscope (Nikon, Japan), and the number of positive TUNEL cells were counted with Image J and Image-Pro Plus 6.0.
Statistical Analysis
The statistical analysis of all data was carried out with SPSS 20.0 software. The experimental data were displayed as the mean ± standard error of the mean. The significance of intergroup differences was analyzed by one-way ANOVA and the least significant difference (LSD) post hoc test. The level of significance was considered at P < 0.05.
Results
H2S Inhibites SD-Generated Hippocampal Oxidative Stress
To investigate whether H2S inhibits SD-generated hippocampal oxidative stress, we explored the effects of H2S on the generation of MDA and GSH as well as the activity of SOD in the hippocampus of rats exposed to SD. After exposure with SD, the content of MDA (Figure 1A) in the hippocampus was significantly increased, while the level of GSH (Figure 1B) and the activity of SOD (Figure 1C) in the hippocampus were significantly decreased. These data indicated that SD induced hippocampal oxidative stress. However, treatment with NaHS (30 and 100 μmol/kg) significantly decreased the content of MDA (Figure 1A) as well as markedly increased the level of GSH (Figure 1B) and the activity of SOD (Figure 1C) in the hippocampus of SD-treated rats. NaHS (100 μmol/kg) alone had no effect on the content of MDA, the level of GSH, and the activity of SOD. Taken together, these results indicated that H2S prevents SD-induced hippocampal oxidative stress.
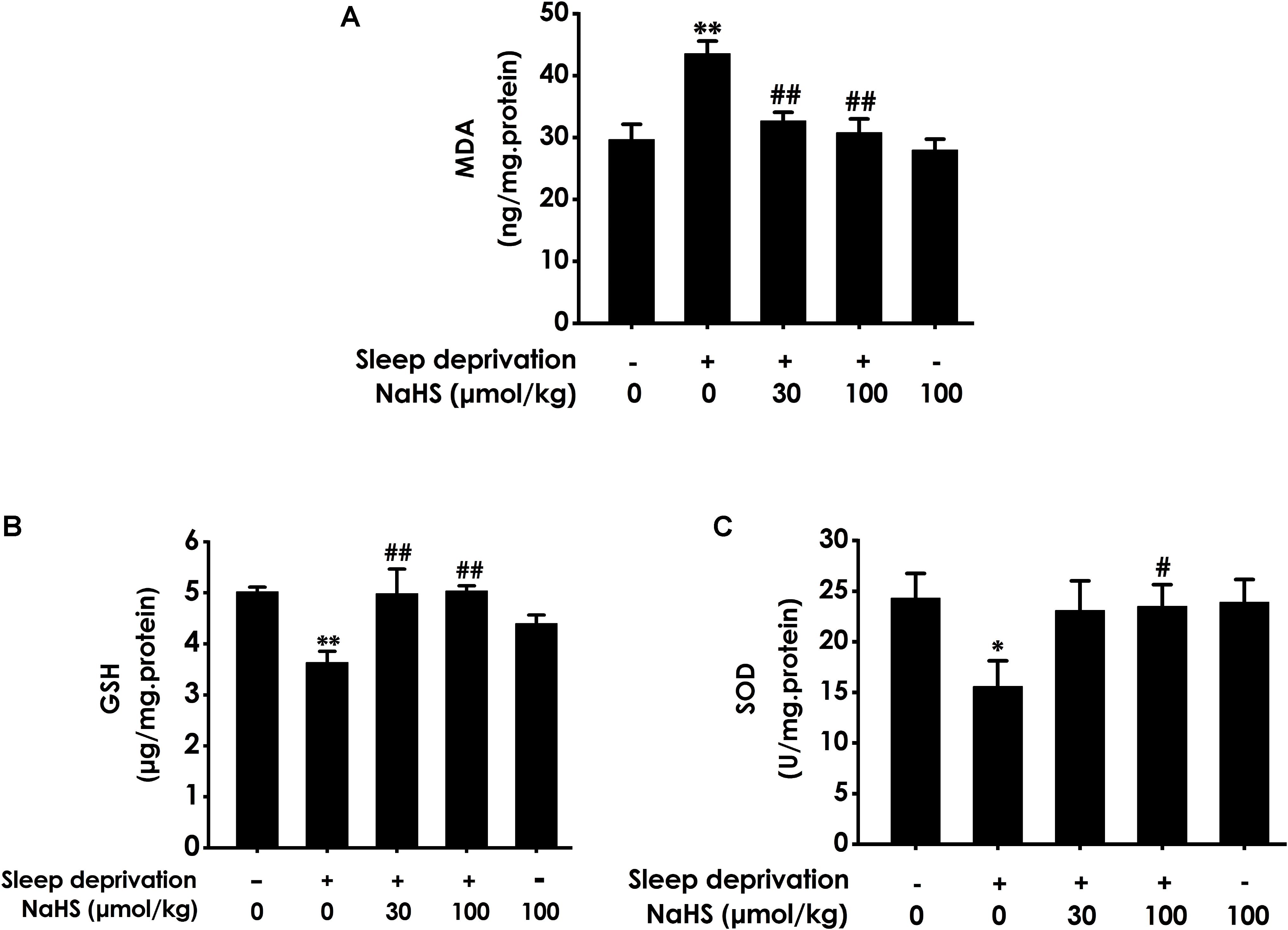
Figure 1. Effect of hydrogen sulfide on sleep deprivation-exerted hippocampal oxidative stress in rats. Rats were pretreated with NaHS (30 and 100 μmol/kg/d, ip) for 7 days and then cotreated with SD for 72 h. The level of MDA (A) and the content of GSH (B) in hippocampus were detected by ELISA kit. The activity of SOD (C) was measured by the NBT assay kit. Values are the means ± SEM (n = 3). ∗P < 0.05, ∗∗P < 0.01, vs. control; #P < 0.05, ##P < 0.01, vs. SD-treated alone group.
H2S Prevents SD-Induced Hippocampal ER Stress
To investigate whether H2S prevents SD-induced hippocampal ER stress, we explored the effect of H2S on the expressions of GPR78, CHOP, and cleaved caspase-12 in the hippocampus of SD-exposed rats. The expressions of GRP78 (Figure 2A), CHOP (Figure 2B), and cleaved caspase-12 (Figure 2C) were significantly increased in the hippocampus of SD-treated rats, which indicated that SD induces hippocampal ER stress. However, treatment with NaHS (30 and 100 μmol/kg) observably decreased the expressions of GRP78 (Figure 2A), CHOP (Figure 2B), and cleaved caspase-12 (Figure 2C) in the hippocampus of SD-exposed rats. NaHS (100 μmol/kg) alone had no effect on the expressions of GRP78, CHOP, and cleaved caspase-12 proteins. These results indicated that H2S prevents SD-induced hippocampal ER stress.
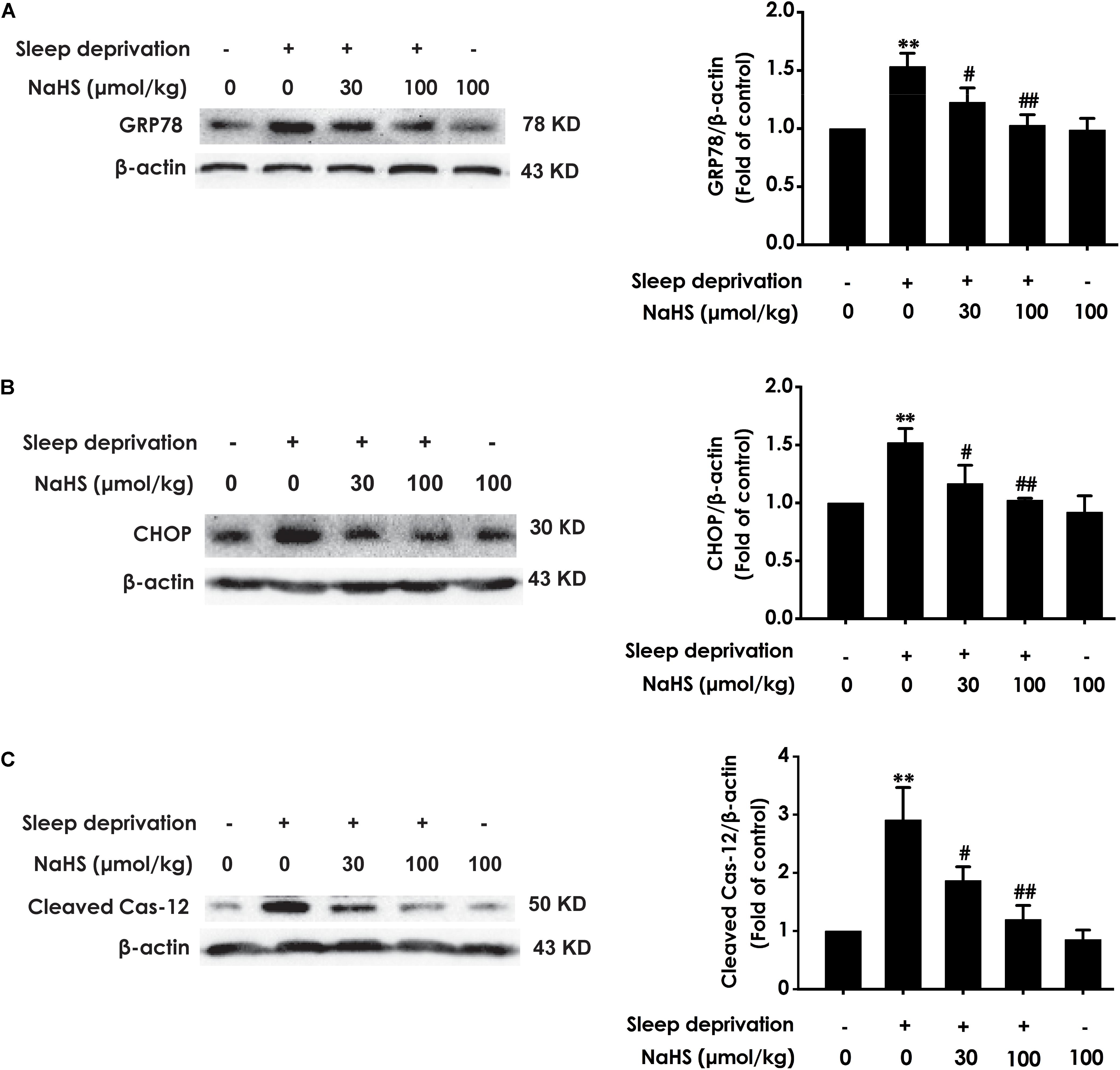
Figure 2. Effect of hydrogen sulfide on sleep deprivation-induced hippocampal ER stress in rats. Rats were pretreated with NaHS (30 and 100 μmol/kg/d, ip) for 7 days and then cotreated with SD for 72 h. The expression of CPR78 (A), CHOP (B), and cleaved caspase-12 (C) were detected by western blotting. In all blots, β-actin was used to determine control. Values are the means ± SEM (n = 3). ∗∗P < 0.01, vs. control; #P < 0.05,##P < 0.01, vs. SD-treated alone group.
H2S Attenuates SD-Exerted Hippocampal Apoptosis
Next, we also investigated whether H2S inhibits SD-induced hippocampal apoptosis. The number of apoptotic cells (Figure 3A) in the hippocampal CA3 region of SD-exposed rats was markedly increased. In addition, in the hippocampus of SD-exposed rats, the activity of caspase-3 was enhanced (Figure 3B), and the expression of Bax (Figure 3C) was significantly upregulated, while the expression of Bcl-2 (Figure 3D) was downregulated. These data indicated that SD induces hippocampal apoptosis. However, treatment with NaHS (30 and 100 μmol/kg) significantly decreased the number of apoptotic cells in the hippocampal CA3 region (Figure 3A) of SD-exposed rats and reversed SD-exerted activation of caspase-3 (Figure 3B), upregulation of Bax expression (Figure 3C), and downregulation of Bcl-2 expression (Figure 3D) in the hippocampus. NaHS (100 μmol/kg) alone had no effect on the number of apoptotic cells in the hippocampal CA3 region, the activity of caspase-3, and the expressions of Bax and Bcl-2. These results indicated the protection of H2S against SD-induced hippocampal apoptosis.
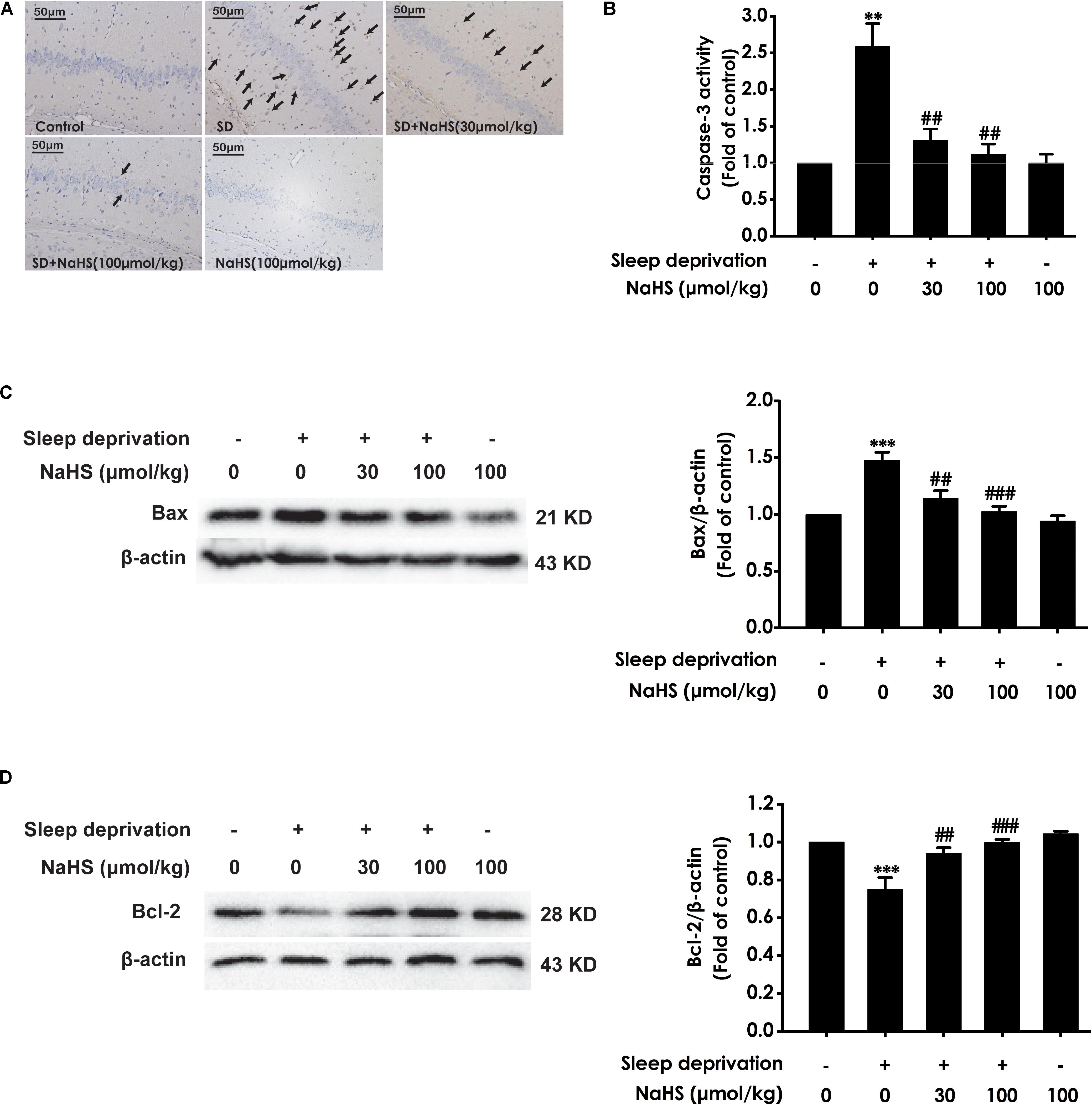
Figure 3. Effect of hydrogen sulfide on sleep deprivation-induced hippocampal apoptosis in rats. Rats were pretreated with NaHS (30 and 100 μmol/kg/d, ip) for 7 days and then cotreated with SD for 72 h. The apoptotic cells in the CA3 area of hippocampus (A) were assessed using Tunel staining, and the arrows indicate the apoptotic cells. The activity of caspase-3 (B) in the hippocampus was detected by ELISA kits. The expressions of Bax (C) and Bcl-2 (D) in the hippocampus were detected by western blot using anti-Bax antibody and anti-Bcl-2 antibody, respectively. In all blots, β-actin was used to determine control. Values are the mean ± S.E.M. (n = 3–4), ∗∗P < 0.01, ∗∗∗P < 0.001 vs. control group. ##P < 0.01, ###P < 0.001, vs. SD-treated alone group.
H2S Upregulates Hippocampal Sirt1 Expression in SD-Exposed Rats
To determine whether Sirt1 mediates the protective effect of H2S against SD-induced hippocampal damage, we explored the effect of H2S on the expression of Sirt1 protein in the hippocampus of SD-exposed rats. After exposure with SD, the expression of Sirt-1 in the hippocampus of rats was markedly decreased (Figure 4). However, treatment with NaHS (30 and 100 μmol/kg) observably increased the expression of Sirt1 in hippocampus of SD-exposed rats (Figure 4). These results indicated that H2S upregulates the expression of hippocampal Sirt1 in SD-exposed rats.
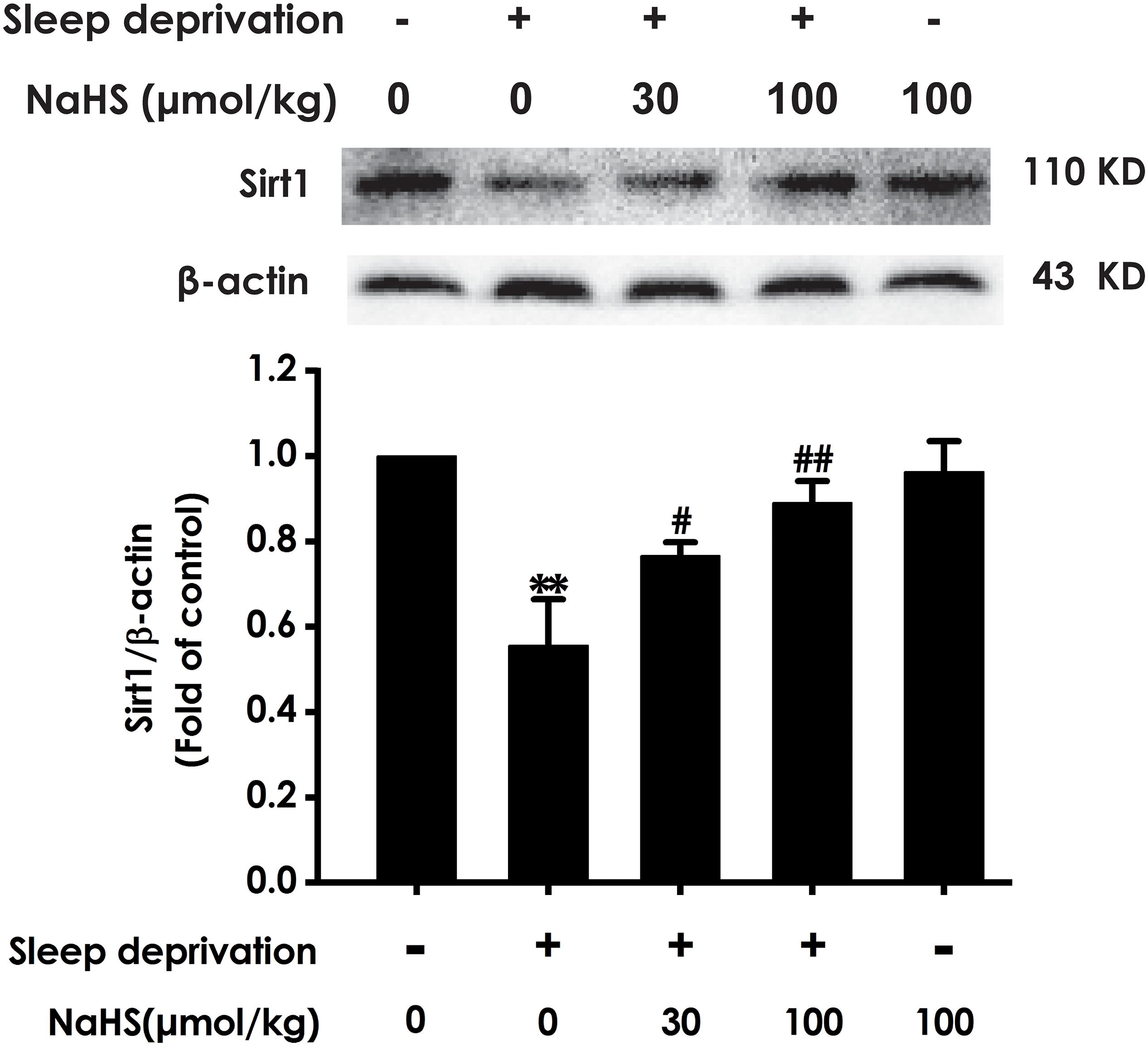
Figure 4. Effect of hydrogen sulfide on the level of hippocampal Sirt1 in sleep deprivation-exposed rats. Rats were pretreated with NaHS (30 and 100 μmol/kg/d, ip) for 7 days and then cotreated with SD for 72 h. The expression of hippocampal Sirt1 was measured by western blotting. Values are the means ± SEM (n = 3–4). ∗∗P < 0.01, vs. control; #P < 0.05, ##P < 0.01, vs. SD-treated alone group.
Inhibition of Sirt-1 by Sirtinol Attenuates the Protection of H2S Against SD-Exerted Hippocampal Oxidative Stress
To confirm the mediatory role of Sirt1 in the protection of H2S against SD-induced hippocampal damage, we first explored whether Sirtinol, a specific Sirt1 inhibitor, blocks the protection of H2S against SD-induced hippocampal oxidative stress. Sirtinol (10 nmol × 1w, i.c.v.) increased the hippocampal MDA level (Figure 5A) and decreased the hippocampal GSH content (Figure 5B) as well as SOD activity (Figure 5C) in the rats cotreated with SD and NaHS (100 μmol/kg/d, ip), which indicated that Sirtinol reverses the antagonistic effect of H2S on SD-induced hippocampal oxidative stress in rats.
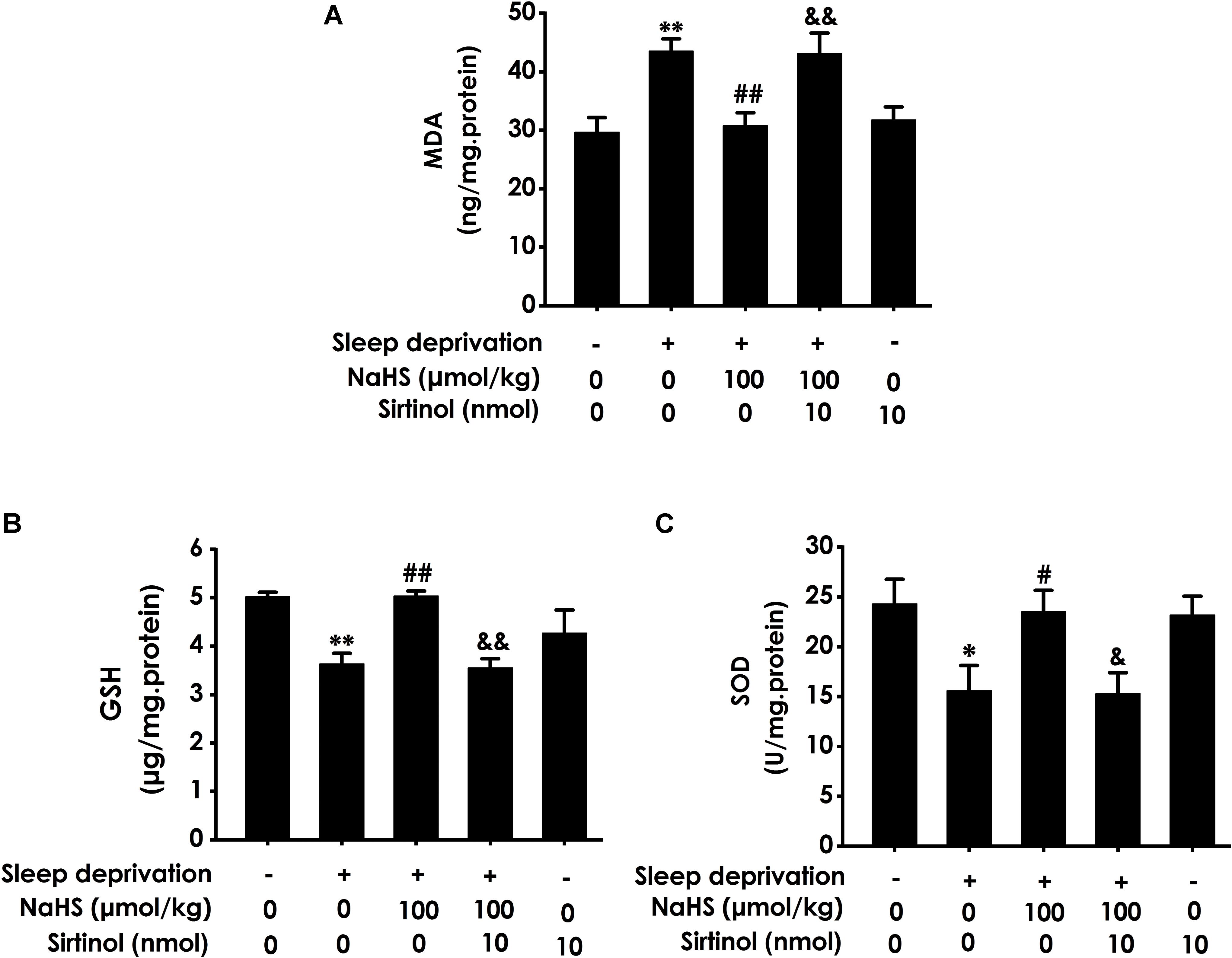
Figure 5. Effect of Sirtinol on hydrogen sulfide ameliorated hippocampal oxidative stress in sleep deprivation-exposed rats. Rats were pretreated with NaHS (100 μmol/kg/d, ip) for 7 days and then cotreated with SD and Sirtinol (10 nmol/d, icv) for 72 h. The levels of MDA (A) and GSH (B) in the hippocampus were detected by ELISA kit. The activity of SOD (C) in the hippocampus was measured by the NBT assay kit. Values are the means ± SEM (n = 3). ∗P < 0.05, ∗∗P < 0.01, vs. control; #P < 0.05, ##P < 0.01, vs. SD-treated alone group; &P < 0.05, &&P < 0.01, vs. cotreated with SD and NaHS (100 μmol/kg/d, i.p.) group.
Inhibition of Sirt-1 by Sirtinol Reverses the Meliorating Effect of H2S on SD-Exerted Hippocampal ER Stress
Next, we investigated whether Sirtinol reverses the protective role of H2S on SD-induced ER stress. Sirtinol (10 nmol, i.c.v.) significantly increased the expressions of GPR78 (Figure 6A), CHOP (Figure 6B), and cleaved caspase-12 (Figure 6C) protein in the hippocampus of rats cotreated with SD and NaHS (100 μmol/kg/d, i.p.), which indicated that Sirtinol abrogates the protective action of H2S on SD-induced hippocampal ER stress.
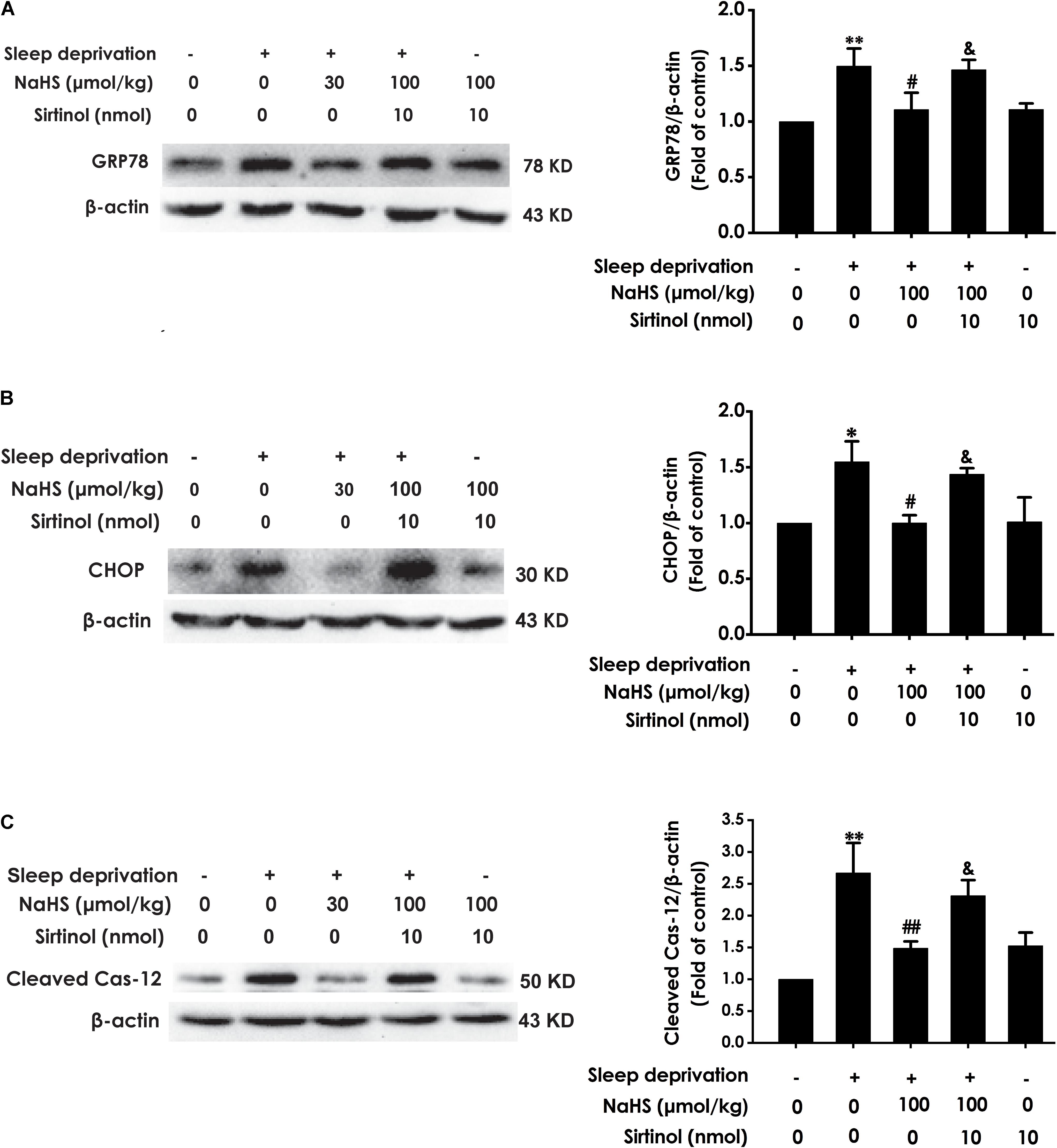
Figure 6. Effect of Sirtinol on hydrogen sulfide ameliorated hippocampal ER stress in sleep deprivation-exposed rats. Rats were pretreated with NaHS (100 μmol/kg/d, ip) for 7 days and then cotreated with SD and Sirtinol (10 nmol/d, icv) for 72 h. The expression of CPR78 (A), CHOP (B), and cleaved caspase-12 (C) in the hippocampus were detected by western blotting. In all blots, β-actin was used to determine control. Values are the means ± SEM (n = 3). ∗P < 0.05, ∗∗P < 0.01, vs. control; #P < 0.05, ##P < 0.01, vs. SD-treated alone group; &P < 0.05, vs. cotreated with NaHS (100 μmol/kg/d, i.p.) and SD treated group.
Inhibition of Sirt-1 by Sirtinol Antagonizes H2S-Ameliorated Hippocampal Apoptosis in SD-Exposed Rats
We also detected whether Sirtinol blocks the protective role of H2S in SD-induced hippocampal apoptosis. Sirtinol (10 nmol, i.c.v.) remarkably increased the amount of apoptotic cells in the hippocampal CA3 region (Figure 7A), enhanced the activity of caspase-3 (Figure 7B), and upregulated the expression of Bax (Figure 7C), while it also significantly downregulated the expression of Bcl-2 (Figure 7D) in the hippocampus of rats cotreated with SD and NaHS (100 μmol/kg/d, i.p.), which indicated that Sirtinol abrogates the protective action of H2S in SD-induced hippocampal apoptosis.
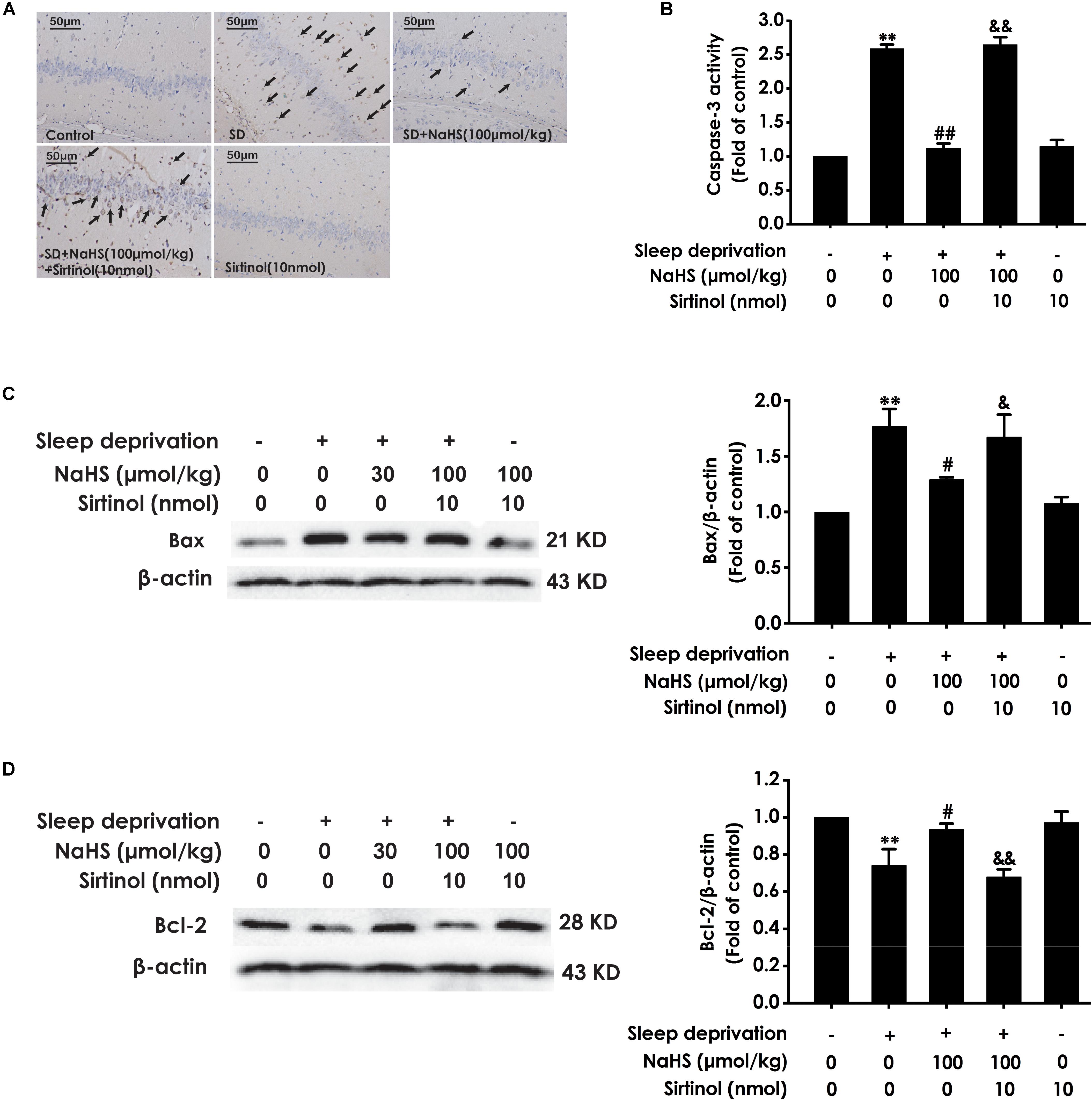
Figure 7. Effect of Sirtinol on hydrogen sulfide ameliorated hippocampal apoptosis in sleep deprivation-induced rats. Rats were pretreated with NaHS (100 μmol/kg/d, ip) for 7 days and then cotreated with SD and Sirtinol (10 nmol/d, icv) for 72 h. The apoptotic cells in the CA3 area of hippocampus (A) were assessed using Tunel staining and the arrow indicated the apoptotic cell. The activity of caspase-3 (B) in the hippocampus was detected by ELISA kits. The expressions of Bax (C) and Bcl-2 (D) in the hippocampus were measured by western blotting. In all blots, β-actin was used to determine control. Values are the mean ± S.E.M. (n = 3). ∗∗P < 0.01, vs. control group; #P < 0.05, ##P < 0.01, vs. SD-treated alone group; &P < 0.05, &&P < 0.01, vs. cotreated with NaHS (100 μmol/kg/d, i.p.) and SD treated group.
Discussion
In the present work, we investigated whether H2S ameliorates SD-induced hippocampal damage and whether Sirt1 mediates this role of H2S. There were several major findings: (1) NaHS (a donor of H2S) mitigates SD-induced hippocampal oxidative stress, ER stress, and apoptosis in SD-exposed rats; (2) NaHS upregulates the expression of Sirt1 in the hippocampus of SD-generated rats; and (3) Sirtinol, an inhibitor of Sirt1, abolishes the protection of H2S against hippocampal oxidative stress, ER stress, and apoptosis in SD-induced rats. Taken together, these data indicated that H2S alleviates SD-induced hippocampal damage, which is via upregulation of hippocampal Sirt1.
Sleep deprivation is an increasingly serious disease in the world, which detrimentally effects on cognitive function (Basner et al., 2007). Increasing evidence in SD animal models also demonstrated that SD induces cognitive impairment (Zhao et al., 2017). In addition, it has been shown that SD results in hippocampal damage (Havekes and Abel, 2017), which is the key link of cognitive dysfunction (Jia et al., 2015). Therefore, antagonizing hippocampal damage may be a therapeutic approach for SD-induced cognitive dysfunction. H2S, as an important neuromodulator and neuroprotectant (Kimura, 2002; Hu et al., 2011; Kimura et al., 2012), plays an essential role in alleviating hippocampal damage (Alzoubi et al., 2018). It has been demonstrated that exogenous H2S alleviates oxidative stress-induced rat hippocampal damage (Jiang et al., 2011) and that H2S prevents cognitive dysfunction via ameliorating hippocampal damage (Zou et al., 2017). Therefore, it is necessary to explore the potential effect of H2S on SD-induced hippocampal damage.
In the present work, rats were pretreated with NaHS (a donor of H2S) for 7 days cotreated with SD for 72 h and the levels of oxidative stress, ER stress and apoptosis in hippocampal were detected. We found that NaHS decreased the level of MDA, increased the activation of SOD, and raised the level of GSH in the hippocampus of SD-exposed rats, which indicated that H2S protects against SD-generated hippocampal oxidative stress. Meanwhile, these data are consistent with the previous study that H2S decreased the level of MDA, increased the activation of SOD, and raised the level of GSH in the hippocampus of rats undergoing heroin withdrawal (Jiang et al., 2011), Moreover, we found that H2S downregulated the expressions of ER stress-related proteins, including GRP78, CHOP and cleaved-caspase-12, in the hippocampus of SD-induced rats. GRP78, CHOP, and caspase-12 are three important markers of ER stress (Hetz, 2012). Our results indicated the protective effects of H2S against SD-induced hippocampal ER stress. This finding was consistent with the previous observations that H2S prevents the hippocampal ER Stress in chronic unpredictable mild stress-exposed rats (Liu et al., 2017). It has been found that H2S attenuates isoflurane-induced hippocampal apoptosis (Hu et al., 2017). Therefore, we investigated whether H2S attenuates SD-induced apoptosis in hippocampus. Bax is a apoptotic protein, Bcl-2 is an anti-apoptotic protein, and Caspase-3 is a critical executioner of apoptosis (Konstantinidou et al., 2007; Jiang et al., 2011). Notably, we also found that NaHS decreased the number of apoptotic cells in the hippocampal CA3 region, suppressed the activity of caspase-3, downregulated the expression of Bax, and upregulated the expression of Bcl2 in the hippocampus of SD-exposed rats, which indicated that H2S attenuates the hippocampal apoptosis in SD-induced rats. Similarity, it has been found that the activity of caspase-3 was decreased, the expression of Bax protein was upregulated, and that the expression of Bcl-2 protein was downregulated in the hippocampus of rats undergoing heroin withdrawal, which were reversed by treatment with exogenous H2S (Jiang et al., 2011). Taken together, these data suggested that H2S prevents hippocampal damage evoked by SD. It has been confirmed that the protection of H2S on recurrent febrile seizures-induced hippocampal damage (Han et al., 2005), which offered a reasonable explanation for our present results.
Next, we further explored the underlying mechanisms for the protection of H2S against SD-induced hippocampal damage. Sirt1, is a member of the sirtuin family of nuclear and cytoplasmic class III histone deacetylases (Sauve et al., 2006), which are expressed in the hippocampus (Michan et al., 2010). Accumulating evidence demonstrated that Sirt1 plays an important role for neuroprotection in several models of neurodegenerative diseases, such as Alzheimer’s disease (AD) (Fujita and Yamashita, 2018), while the level of hippocampal Sirt1 were significantly decreased in patients with AD (Cao et al., 2018). Meanwhile, it has been demonstrated that oxymatrine attenuated hippocampus ischemia/reperfusion injury through upregulation of Sirt1 (Zhou et al., 2018), indicating that Sirt1 acts as a key factor in hippocampal damage. In addition, SD decreases the expression of Sirt1 in hippocampus (Chang et al., 2009). Does Sirt1 mediate the protection of H2S against SD-induced hippocampal damage? Interestingly, our previous study testified that H2S upregulates the expression of Sirt1 in hippocampus (Tang et al., 2018) and that Sirt1 mediates the protection of H2S against chronic restrain stress-induced hippocampal damage in rats (Li et al., 2017). Therefore, the speculation that Sirt1 mediates the protection of H2S against SD-induced hippocampus damage is reasonable. Our present work showed that SD caused decreases in the expression of Sirt1 in hippocampus and that H2S observably increased the expression of Sirt1 in the hippocampus of the SD-generated damage. Moreover, Sirtinol, the Sirt1 inhibitor, blocked the protective effect of H2S on SD-induced hippocampal oxidative stress, ER stress, and apoptosis in rats. This finding is consistent with the previous report that Sirt1 reverses the protective effect of H2S on hippocampal damage in chronic restrain stress-induced rats (Li et al., 2017). These data indicated that upregulating the expression of Sirt1 in hippocampus mediates the protective role of H2S in SD-induced hippocampal damage.
In summary, the present study elucidated the protective effect of H2S on SD-induced hippocampal damage, which is mediated by the upregulation of Sirt1 in hippocampus. Our finding suggested that H2S may act a novel potential therapeutic agent for SD-induced hippocampal damage, which opens a new perspective for the prevention and treatment of cognitive dysfunction after SD.
Data Availability Statement
All datasets generated for this study are included in the article/supplementary material.
Ethics Statement
The animal study was reviewed and approved by the Animal Use and Protection Committee of University of South China.
Author Contributions
X-QT and WZ contributed conception and design of the study. X-QT, PZ, and C-YW guided and supervised the study. J-XZ, ML, LJ, FL, Y-YT, and XK performed the experiments. J-XZ, ML, and LJ analyzed the data of the experiments and wrote the manuscript. All authors contributed to manuscript revision and read and approved the submitted version.
Funding
This study was supported by the National Natural Science Foundation of China (81671057), the Major Research Topics of the Health and Family Planning Commission of Hunan province (A2017020), and the Natural Science Foundation of Hunan Province, China (2019JJ80546).
Conflict of Interest
The authors declare that the research was conducted in the absence of any commercial or financial relationships that could be construed as a potential conflict of interest.
References
Alzoubi, K. H., Malkawi, B. S., Khabour, O. F., El-Elimat, T., and Alali, F. Q. (2018). Arbutus andrachne L. reverses sleep deprivation-induced memory impairments in rats. Mol. Neurobiol. 55, 1150–1156. doi: 10.1007/s12035-017-0387-8
Basner, M., Fomberstein, K. M., Razavi, F. M., Banks, S., William, J. H., Rosa, R. R., et al. (2007). American time use survey: sleep time and its relationship to waking activities. Sleep 30, 1085–1095. doi: 10.1093/sleep/30.9.1085
Cao, K., Dong, Y. T., Xiang, J., Xu, Y., Hong, W., Song, H., et al. (2018). Reduced expression of SIRT1 and SOD-1 and the correlation between these levels in various regions of the brains of patients with Alzheimer’s disease. J. Clin. Pathol. 71, 1090–1099. doi: 10.1136/jclinpath-2018-205320
Centers for Disease and Prevention (2011). Effect of short sleep duration on daily activities–United States, 2005-2008. MMWR Morb. Mortal. Wkly. Rep. 60, 239–242.
Chang, H. M., Wu, U. I., and Lan, C. T. (2009). Melatonin preserves longevity protein (sirtuin 1) expression in the hippocampus of total sleep-deprived rats. J. Pineal Res. 47, 211–220. doi: 10.1111/j.1600-079X.2009.00704.x
Cohen, H. Y., Miller, C., Bitterman, K. J., Wall, N. R., Hekking, B., Kessler, B., et al. (2004). Calorie restriction promotes mammalian cell survival by inducing the SIRT1 deacetylase. Science 305, 390–392. doi: 10.1126/science.1099196
Dai, X. J., Gong, H. H., Wang, Y. X., Zhou, F. Q., Min, Y. J., Zhao, F., et al. (2012). Gender differences in brain regional homogeneity of healthy subjects after normal sleep and after sleep deprivation: a resting-state fMRI study. Sleep Med. 13, 720–727. doi: 10.1016/j.sleep.2011.09.019
Donmez, G. (2012). The neurobiology of sirtuins and their role in neurodegeneration. Trends Pharmacol. Sci. 33, 494–501. doi: 10.1016/j.tips.2012.05.007
Eichenbaum, H. (2000). A cortical-hippocampal system for declarative memory. Nat. Rev. Neurosci. 1, 41–50. doi: 10.1038/35036213
Elbassuoni, E. A., and Nazmy, W. H. (2018). Novel neuroprotective role of hydrogen sulfide in a rat model of stress brain injury. Gen. Physiol. Biophys. 37, 233–241. doi: 10.4149/gpb_2017029
Fujita, Y., and Yamashita, T. (2018). Sirtuins in neuroendocrine regulation and neurological diseases. Front. Neurosci. 12:778. doi: 10.3389/fnins.2018.00778
Hakim, F., Wang, Y., Carreras, A., Hirotsu, C., Zhang, J., Peris, E., et al. (2015). Chronic sleep fragmentation during the sleep period induces hypothalamic endoplasmic reticulum stress and PTP1b-mediated leptin resistance in male mice. Sleep 38, 31–40. doi: 10.5665/sleep.4320
Han, S. H. (2009). Potential role of sirtuin as a therapeutic target for neurodegenerative diseases. J. Clin. Neurol. 5, 120–125.
Han, Y., Qin, J., Chang, X., Yang, Z., Tang, X., and Du, J. (2005). Hydrogen sulfide may improve the hippocampal damage induced by recurrent febrile seizures in rats. Biochem. Biophys. Res. Commun. 327, 431–436. doi: 10.1016/j.bbrc.2004.12.028
Havekes, R., and Abel, T. (2017). The tired hippocampus: the molecular impact of sleep deprivation on hippocampal function. Curr. Opin. Neurobiol. 44, 13–19. doi: 10.1016/j.conb.2017.02.005
Hetz, C. (2012). The unfolded protein response: controlling cell fate decisions under ER stress and beyond. Nat. Rev. Mol. Cell Biol. 13, 89–102. doi: 10.1038/nrm3270
Hu, L. F., Lu, M., Hon Wong, P. T., and Bian, J. S. (2011). Hydrogen sulfide: neurophysiology and neuropathology. Antioxid. Redox Signal. 15, 405–419. doi: 10.1089/ars.2010.3517
Hu, M., Zou, W., Wang, C. Y., Chen, X., Tan, H. Y., Zeng, H. Y., et al. (2016). Hydrogen sulfide protects against chronic unpredictable mild stress-induced oxidative stress in hippocampus by upregulation of BDNF-TrkB pathway. Oxid. Med. Cell. Longev. 2016:2153745. doi: 10.1155/2016/2153745
Hu, X., Luan, L., Guan, W., Zhang, S., Li, B., Ji, W., et al. (2017). Hydrogen sulfide attenuates isoflurane-induced neuroapoptosis and cognitive impairment in the developing rat brain. BMC Anesthesiol. 17:123. doi: 10.1186/s12871-017-0419-y
Imai, S., Armstrong, C. M., Kaeberlein, M., and Guarente, L. (2000). Transcriptional silencing and longevity protein Sir2 is an NAD-dependent histone deacetylase. Nature 403, 795–800. doi: 10.1038/35001622
Jia, Z., Geng, L., Xie, G., Chu, Q., and Zhang, W. (2015). Sevoflurane impairs acquisition learning and memory function in transgenic mice model of Alzheimer’s disease by induction of hippocampal neuron apoptosis. Int. J. Clin. Exp. Med. 8, 15490–15497.
Jiang, L.-H., Luo, X., He, W., Huang, X.-X., and Cheng, T.-T. (2011). Effects of exogenous hydrogen sulfide on apoptosis proteins and oxidative stress in the hippocampus of rats undergoing heroin withdrawal. Arch. Pharm. Res. 34, 2155–2162. doi: 10.1007/s12272-011-1220-y
Kaliyaperumal, D., Elango, Y., Alagesan, M., and Santhanakrishanan, I. (2017). Effects of sleep deprivation on the cognitive performance of nurses working in shift. J. Clin. Diagn. Res. 11, CC01–CC03.
Killgore, W. D. (2010). Effects of sleep deprivation on cognition. Prog. Brain Res. 185, 105–129. doi: 10.1016/B978-0-444-53702-7.00007-5
Kim, K. E., Jeong, E. A., Lee, J. Y., Yi, C. O., Park, K. A., Jin, Z., et al. (2018). Myeloid sirtuin1 deficiency aggravates hippocampal inflammation in mice fed high-fat diets. Biochem. Biophys. Res. Commun. 499, 1025–1031. doi: 10.1016/j.bbrc.2018.04.044
Kimura, H., Shibuya, N., and Kimura, Y. (2012). Hydrogen sulfide is a signaling molecule and a cytoprotectant. Antioxid. Redox Signal. 17, 45–57. doi: 10.1089/ars.2011.4345
Konstantinidou, A. E., Givalos, N., Gakiopoulou, H., Korkolopoulou, P., Kotsiakis, X., Boviatsis, E., et al. (2007). Caspase-3 immunohistochemical expression is a marker of apoptosis, increased grade and early recurrence in intracranial meningiomas. Apoptosis 12, 695–705. doi: 10.1007/s10495-006-0001-4
Li, M. H., Tang, J. P., Zhang, P., Li, X., Wang, C. Y., Wei, H. J., et al. (2014). Disturbance of endogenous hydrogen sulfide generation and endoplasmic reticulum stress in hippocampus are involved in homocysteine-induced defect in learning and memory of rats. Behav. Brain Res. 262, 35–41. doi: 10.1016/j.bbr.2014.01.001
Li, X. N., Chen, L., Luo, B., Li, X., Wang, C. Y., Zou, W., et al. (2017). Hydrogen sulfide attenuates chronic restrain stress-induced cognitive impairment by upreglulation of Sirt1 in hippocampus. Oncotarget 8, 100396–100410. doi: 10.18632/oncotarget.22237
Liu, S. Y., Li, D., Zeng, H. Y., Kan, L. Y., Zou, W., Zhang, P., et al. (2017). Hydrogen sulfide inhibits chronic unpredictable mild stress-induced depressive-like behavior by upregulation of Sirt-1: involvement in suppression of hippocampal endoplasmic reticulum stress. Int. J. Neuropsychopharmacol. 20, 867–876. doi: 10.1093/ijnp/pyx030
Machado, R. B., Hipolide, D. C., Benedito-Silva, A. A., and Tufik, S. (2004). Sleep deprivation induced by the modified multiple platform technique: quantification of sleep loss and recovery. Brain Res. 1004, 45–51. doi: 10.1016/j.brainres.2004.01.019
McDermott, C. M., Lahoste, G. J., Chen, C., Musto, A., Bazan, N. G., and Magee, J. C. (2003). Sleep deprivation causes behavioral, synaptic, and membrane excitability alterations in hippocampal neurons. J. Neurosci. 23, 9687–9695. doi: 10.1523/jneurosci.23-29-09687.2003
Meng, Z., Li, J., Zhao, H., Liu, H., Zhang, G., Wang, L., et al. (2015). Resveratrol relieves ischemia-induced oxidative stress in the hippocampus by activating SIRT1. Exp. Ther. Med. 10, 525–530.
Michan, S., Li, Y., Chou, M. M., Parrella, E., Ge, H., Long, J. M., et al. (2010). SIRT1 is essential for normal cognitive function and synaptic plasticity. J. Neurosci. 30, 9695–9707. doi: 10.1523/JNEUROSCI.0027-10.2010
Nabaee, E., Kesmati, M., Shahriari, A., Khajehpour, L., and Torabi, M. (2018). Cognitive and hippocampus biochemical changes following sleep deprivation in the adult male rat. Biomed. Pharmacother. 104, 69–76. doi: 10.1016/j.biopha.2018.04.197
Nasehi, M., Mosavi-Nezhad, S. M., Khakpai, F., and Zarrindast, M. R. (2018). The role of omega-3 on modulation of cognitive deficiency induced by REM sleep deprivation in rats. Behav. Brain Res. 351, 152–160. doi: 10.1016/j.bbr.2018.06.002
Nie, L., Hu, Y., Yan, X., Li, M., Chen, L., Li, H., et al. (2013). The anti-apoptotic effect of hydrogen sulfide attenuates injuries to the medullary respiratory centers of neonatal rats subjected to in utero cigarette smoke exposure. Respir. Physiol. Neurobiol. 188, 29–38. doi: 10.1016/j.resp.2013.05.001
Ohayon, M. M., Smolensky, M. H., and Roth, T. (2010). Consequences of shiftworking on sleep duration, sleepiness, and sleep attacks. Chronobiol. Int. 27, 575–589. doi: 10.3109/07420521003749956
Panthi, S., Chung, H. J., Jung, J., and Jeong, N. Y. (2016). Physiological importance of hydrogen sulfide: emerging potent neuroprotector and neuromodulator. Oxid. Med. Cell. Longev. 2016:9049782. doi: 10.1155/2016/9049782
Qiu, H., Zhong, R., Liu, H., Zhang, F., Li, S., and Le, W. (2016). Chronic sleep deprivation exacerbates learning-memory disability and Alzheimer’s disease-like pathologies in AbetaPP(swe)/PS1(DeltaE9) mice. J. Alzheimers Dis. 50, 669–685. doi: 10.3233/JAD-150774
Sauve, A. A., Wolberger, C., Schramm, V. L., and Boeke, J. D. (2006). The biochemistry of sirtuins. Annu. Rev. Biochem. 75, 435–465. doi: 10.1146/annurev.biochem.74.082803.133500
Shohamy, D., and Turk-Browne, N. B. (2013). Mechanisms for widespread hippocampal involvement in cognition. J. Exp. Psychol. Gen. 142, 1159–1170. doi: 10.1037/a0034461
Singh, R., Kiloung, J., Singh, S., and Sharma, D. (2008). Effect of paradoxical sleep deprivation on oxidative stress parameters in brain regions of adult and old rats. Biogerontology 9, 153–162. doi: 10.1007/s10522-008-9124-z
Smith, J. S., Brachmann, C. B., Celic, I., Kenna, M. A., Muhammad, S., Starai, V. J., et al. (2000). A phylogenetically conserved NAD+-dependent protein deacetylase activity in the Sir2 protein family. Proc. Natl. Acad. Sci. U.S.A. 97, 6658–6663. doi: 10.1073/pnas.97.12.6658
Squire, L. R., and Zola, S. M. (1996). Structure and function of declarative and nondeclarative memory systems. Proc. Natl. Acad. Sci. U.S.A. 93, 13515–13522. doi: 10.1073/pnas.93.24.13515
Suchecki, D., and Tufik, S. (2000). Social stability attenuates the stress in the modified multiple platform method for paradoxical sleep deprivation in the rat. Physiol. Behav. 68, 309–316. doi: 10.1016/s0031-9384(99)00181-x
Tang, Y. Y., Wang, A. P., Wei, H. J., Li, M. H., Zou, W., Li, X., et al. (2018). Role of silent information regulator 1 in the protective effect of hydrogen sulfide on homocysteine-induced cognitive dysfunction: involving reduction of hippocampal ER stress. Behav. Brain Res. 342, 35–42. doi: 10.1016/j.bbr.2017.12.040
Walker, M. P., and Stickgold, R. (2006). Sleep, memory, and plasticity. Annu. Rev. Psychol. 57, 139–166.
Wang, R. (2010). Hydrogen sulfide: the third gasotransmitter in biology and medicine. Antioxid. Redox Signal. 12, 1061–1064. doi: 10.1089/ars.2009.2938
Wei, H. J., Xu, J. H., Li, M. H., Tang, J. P., Zou, W., Zhang, P., et al. (2014). Hydrogen sulfide inhibits homocysteine-induced endoplasmic reticulum stress and neuronal apoptosis in rat hippocampus via upregulation of the BDNF-TrkB pathway. Acta Pharmacol. Sin. 35, 707–715. doi: 10.1038/aps.2013.197
Wu, H., Wang, H., Zhang, W., Wei, X., Zhao, J., Yan, P., et al. (2015). rhEPO affects apoptosis in hippocampus of aging rats by upregulating SIRT1. Int. J. Clin. Exp. Pathol. 8, 6870–6880.
Zhang, F., Wang, S., Gan, L., Vosler, P. S., Gao, Y., Zigmond, M. J., et al. (2011). Protective effects and mechanisms of sirtuins in the nervous system. Prog. Neurobiol. 95, 373–395. doi: 10.1016/j.pneurobio.2011.09.001
Zhang, Q., Yuan, L., Liu, D., Wang, J., Wang, S., Zhang, Q., et al. (2014). Hydrogen sulfide attenuates hypoxia-induced neurotoxicity through inhibiting microglial activation. Pharmacol. Res. 84, 32–44. doi: 10.1016/j.phrs.2014.04.009
Zhang, X., and Bian, J. S. (2014). Hydrogen sulfide: a neuromodulator and neuroprotectant in the central nervous system. ACS Chem. Neurosci. 5, 876–883. doi: 10.1021/cn500185g
Zhao, H. Y., Wu, H. J., He, J. L., Zhuang, J. H., Liu, Z. Y., Huang, L. Q., et al. (2017). Chronic sleep restriction induces cognitive deficits and cortical beta-amyloid deposition in mice via BACE1-antisense activation. CNS Neurosci. Ther. 23, 233–240. doi: 10.1111/cns.12667
Zhou, S., Qiao, B., Chu, X., and Kong, Q. (2018). Oxymatrine attenuates cognitive deficits through SIRT1-mediated autophagy in ischemic stroke. J. Neuroimmunol. 323, 136–142. doi: 10.1016/j.jneuroim.2018.06.018
Keywords: sleep deprivation, hydrogen sulfide, hippocampal damage, silence information regulating factor 1, oxidative stress
Citation: Zuo J-X, Li M, Jiang L, Lan F, Tang Y-Y, Kang X, Zou W, Wang C-Y, Zhang P and Tang X-Q (2020) Hydrogen Sulfide Prevents Sleep Deprivation-Induced Hippocampal Damage by Upregulation of Sirt1 in the Hippocampus. Front. Neurosci. 14:169. doi: 10.3389/fnins.2020.00169
Received: 15 November 2019; Accepted: 14 February 2020;
Published: 11 March 2020.
Edited by:
Patrizia Longone, Santa Lucia Foundation (IRCCS), ItalyReviewed by:
Xiangping Chu, University of Missouri–Kansas City, United StatesHua Zhang, Hefei University of Technology, China
Copyright © 2020 Zuo, Li, Jiang, Lan, Tang, Kang, Zou, Wang, Zhang and Tang. This is an open-access article distributed under the terms of the Creative Commons Attribution License (CC BY). The use, distribution or reproduction in other forums is permitted, provided the original author(s) and the copyright owner(s) are credited and that the original publication in this journal is cited, in accordance with accepted academic practice. No use, distribution or reproduction is permitted which does not comply with these terms.
*Correspondence: Wei Zou, em91dzQxNUAxNjMuY29t; em91dzQxNUB1c2MuZWR1LmNu; Xiao-Qing Tang, dGFuZ3hxLXVzY0B1c2MuZWR1LmNu; dGFuZ3hxLXVzY0BxcS5jb20=
†These authors have contributed equally to this work