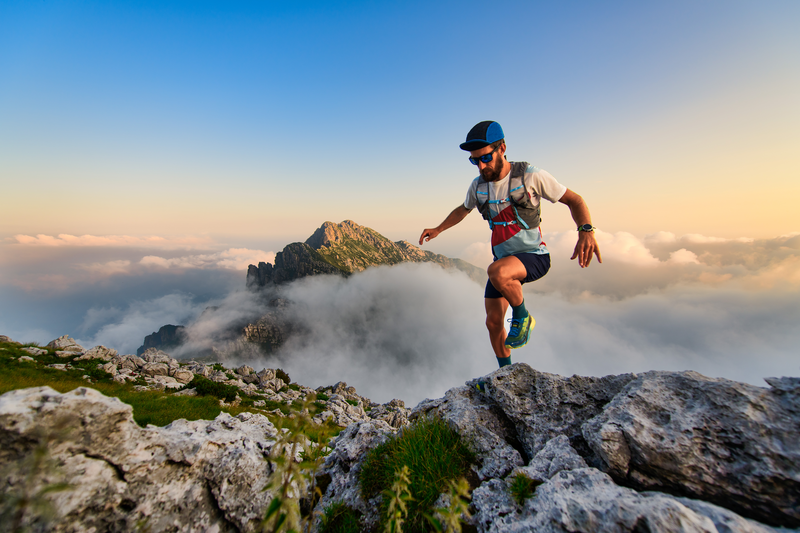
94% of researchers rate our articles as excellent or good
Learn more about the work of our research integrity team to safeguard the quality of each article we publish.
Find out more
SYSTEMATIC REVIEW article
Front. Neurosci. , 10 January 2019
Sec. Perception Science
Volume 12 - 2018 | https://doi.org/10.3389/fnins.2018.01021
Background: Spatial ability is vital for human survival and development. However, the relationship between large-scale and small-scale spatial ability remains poorly understood. To address this issue from a novel perspective, we performed an activation likelihood estimation (ALE) meta-analysis of neuroimaging studies to determine the shared and distinct neural bases of these two forms of spatial ability.
Methods: We searched Web of Science, PubMed, PsycINFO, and Google Scholar for studies regarding “spatial ability” published within the last 20 years (January 1988 through June 2018). A final total of 103 studies (Table 1) involving 2,085 participants (male = 1,116) and 2,586 foci were incorporated into the meta-analysis.
Results: Large-scale spatial ability was associated with activation in the limbic lobe, posterior lobe, occipital lobe, parietal lobe, right anterior lobe, frontal lobe, and right sub-lobar area. Small-scale spatial ability was associated with activation in the parietal lobe, occipital lobe, frontal lobe, right posterior lobe, and left sub-lobar area. Furthermore, conjunction analysis revealed overlapping regions in the sub-gyrus, right superior frontal gyrus, right superior parietal lobule, right middle occipital gyrus, right superior occipital gyrus, left inferior occipital gyrus, and precuneus. The contrast analysis demonstrated that the parahippocampal gyrus, left lingual gyrus, culmen, right middle temporal gyrus, left declive, left superior occipital gyrus, and right lentiform nucleus were more strongly activated during large-scale spatial tasks. In contrast, the precuneus, right inferior frontal gyrus, right precentral gyrus, left inferior parietal lobule, left supramarginal gyrus, left superior parietal lobule, right inferior occipital gyrus, and left middle frontal gyrus were more strongly activated during small-scale spatial tasks. Our results further indicated that there is no absolute difference in the cognitive strategies associated with the two forms of spatial ability (egocentric/allocentric).
Conclusion: The results of the present study verify and expand upon the theoretical model of spatial ability proposed by Hegarty et al. Our analysis revealed a shared neural basis between large- and small-scale spatial abilities, as well as specific yet independent neural bases underlying each. Based on these findings, we proposed a more comprehensive version of the behavioral model.
Spatial ability is a core cognitive function that plays a significant role in individual intelligence. In general, spatial ability has been defined as the ability to understand the relationships among different positions in space or imagined movements of two- and three-dimensional objects (Clements, 1999; Wang et al., 2014). Specifically, spatial ability can be divided into two major categories: large-scale spatial ability and small-scale spatial ability. Large-scale spatial ability refers to the ability of individuals to carry out cognitive processing of spatial information in the large-scale environment. During this process, the viewer's perspective changes with respect to the larger environment, but the spatial relationships among individual objects remain the same (Hegarty and Waller, 2004; Wang et al., 2014). Representative examples of large-scale spatial ability include navigation and spatial orientation abilities (Jansen, 2009; Höffler, 2010; Wang et al., 2014). Navigation ability refers to the ability to navigate within a large-scale environment in which the spatial relationships among landmarks cannot be fully apprehended from a single vantage point (Wang and Carr, 2014), while spatial orientation refers to the ability to imagine objects from different perspectives (Yilmaz, 2009; Turgut, 2015).
Small-scale spatial ability can be defined as the ability to mentally represent and transform two- and three-dimensional images that can typically be apprehended from a single vantage point (Wang and Carr, 2014). Small-scale spatial ability mainly refers to spatial visualization and spatial relations capabilities (Jansen, 2009; Höffler, 2010). Spatial visualization is defined as the ability to manipulate complex spatial information involving the configurations of shapes (e.g., image folding or movement) or to mentally transform a two-dimensional object into three-dimensional object (Linn and Petersen, 1985; Yang and Chen, 2010). Spatial relations ability refers to the ability to recognize the relationships among the visual components of an object (Bosnyak and Nagy-Kondor, 2008; Turgut, 2015).
Currently, the relationship between large- and small-scale spatial ability can be explained using four models: (1) The “unitary model” assumes that spatial abilities along the two scales exhibit complete overlap, (2) while the “partial dissociation model” proposes that the two types of spatial ability exhibit similarities and differences. (3) In contrast, the “total dissociation model” assumes that the two sets of abilities are distinct. Lastly, (4) the “mediation model” assumes that small- and large-scale spatial abilities can be dissociated, but are determined by a third variable (Hegarty et al., 2006; Jansen, 2009). That is, while Models 1 and 2 propose that the two forms of spatial ability are related, Models 3, and 4 propose that they are not.
Each of the aforementioned models has been verified to some extent in previous studies. For instance, Hegarty and Waller (2004) observed a dissociative relationship between mental rotation and perspective-taking spatial abilities. A meta-analysis by Wang et al. (2014) further suggested that small- and large-scale spatial abilities are best characterized as separate entities. However, other studies have yielded conflicting results. Kozhevnikov et al. (2006) demonstrated that spatial navigation performance can be predicted based on mental rotation ability. In addition, Malinowski (2001) reported that mental rotation skills are significantly correlated with wayfinding performance during an orienteering task.
Notably, in a study that examined spatial abilities, spatial updating, verbal abilities, and working memory in 221 participants, Hegarty et al. (2006) reported results that were consistent with the partial dissociation model only. Moreover, they specified the degree of overlap between small-scale and large-scale abilities, providing new insights regarding the similarities, and differences between these abilities (see Figure 1). Although their work is of great significance, the partial dissociation model remains problematic for two reasons: The model is currently incomplete, and evidence for the neural basis of such a model requires further and more comprehensive verification.
Figure 1. Model characterizing the relationship between large- and small-scale spatial abilities, as proposed by Hegarty et al. (2006).
The rapid development of neuroimaging techniques in recent years has prompted extensive investigation of large- and small-scale spatial abilities using functional magnetic resonance imaging (FMRI) and positron emission tomography (PET). In an effort to verify and expand the model of spatial ability proposed by Hegarty et al. (2006), the present study aimed to elucidate the neural basis of large- and small-scale spatial abilities via activation likelihood estimation (ALE) analysis (Eickhoff et al., 2012).
We searched Web of Science, PubMed, PsycINFO, and Google Scholar for studies regarding “spatial ability” published within the last 20 years (January 1988 through June 2018). In order to retrieve the maximum number of relevant articles, we classified the search keywords into the following four series based on the type of spatial ability, yielding a total of 76 groups:
(1) Spatial ability/Spatial cognition/Spatial perception/Spatial information processing + FMRI/PET.
(2) Large-scale Spatial ability/Small-scale Spatial ability + FMRI/PET.
(3) Navigation/Spatial orientation/Spatial visualization/ Spatial relations + FMRI/PET.
(4) Navigation task/test + FMRI/PET; Draw maps task/test + FMRI/PET; Way-finding task/test + FMRI/PET; Map learning task/test + FMRI/PET; Spatial orientation task/test + FMRI/PET; Perspective taking task/test + FMRI/PET; Spatial visualization task/test + FMRI/PET; Mental rotations task/test + FMRI/PET; Paper folding task/test + FMRI/PET; Spatial relations task/test + FMRI/PET; Water level task/test + FMRI/PET; Card rotation task/test + FMRI/PET; Figures task/test + FMRI/PET; Differential aptitude task/test + FMRI/PET.
After four rounds of the above search process, a total of 826 documents were identified. We then examined each of the documents in full, incorporating those with the following characteristics into our meta-analysis:
(1) All participants were healthy individuals.
(2) The study must have included whole-brain rather than region-of-interest analyses, and the data reported must have been standardized (Montreal Neurological Institute (MNI) or Talairach space).
(3) The study must have utilized behavioral experimental methods, and the articles must have included brain imaging data obtained from individuals following independent experimental tasks.
(4) If an experimental result was reported in multiple papers, only one instance was utilized for the meta-analysis.
Following this screening process, a total of 103 studies (Table 1 and Figure 2; see Supplementary Table 1 for PRISMA Checklist) involving 2,085 participants (male = 1,116) and 2,586 foci were incorporated into the meta-analysis (see Supplementary Table 2). Among these foci, 1,372 were associated with large-scale spatial ability, while 1,214 were associated with small-scale spatial ability.
ALE, which treats activation foci reported in neuroimaging studies not as single points but as spatial probability distributions centered at the given coordinates, is the most common algorithm for coordinate-based meta-analysis (Eickhoff et al., 2012). In the present study, we utilized the revised algorithm for ALE analysis proposed by Eickhoff et al. (2009). This algorithm models the spatial uncertainty—and thus the probability distribution—of each focus using an estimation of the inter-individual and inter-laboratory variability typically observed in neuroimaging experiments, rather than using a pre-specified full-width at half maximum (FWHM) for all experiments as originally proposed. The modified permutation procedure reflects the null-distribution of a random spatial association between studies (i.e., random-effects analysis), rather than between foci (i.e., fixed-effects analysis; Eickhoff et al., 2016, 2017). The “modeled activation” (MA) map is computed by the following procedure. First, all foci reported for a given study are modeled as Gaussian probability distributions. The information provided by the foci of a given study is then merged into a single 3D-volume. To this end, the modeled probabilities are combined over all foci reported in that experiment by taking the voxel-wise union of their probability values (Eickhoff et al., 2009). The respective activation probabilities (the values of the MA maps) are then recorded, yielding as many values as there had been studies included in the current meta-analysis. These values correspond to MA values, that were sampled from random, spatially independent locations. The union of these activation probabilities is then computed in the same manner as done for the meta-analysis itself in order to yield an ALE score under the null-hypothesis of spatial independence (Eickhoff et al., 2009, 2012).
The ALE method is advantageous in that it may help to resolve several issues in current brain imaging research. First, the number of participants involved in single brain imaging studies is generally low, often providing unstable results. Second, single-brain imaging results are likely influenced by certain experimental conditions (e.g., scan parameters). Third, interpretations regarding the function of a given brain region derived from a single study are often based on a small number of experimental tasks.
Ginger ALE software (version 2.3; http://www.brainmap.org/ale) was used to conduct the current meta-analysis, including the conversion of Talairach coordinates into MNI space. Loci in both cerebral and cerebellar regions were all focused by the current research. The resulting p-value maps were threshold using cluster-level family-wise error (FWE) correction at p < 0.05, with a cluster-defining threshold of p < 0.005 and 5,000 permutations (Eickhoff et al., 2012). The results were overlaid onto an anatomical template (Colin27 T1 seg MNI.nii; http://www.brainmap.org/ale) and displayed using Mango software (http://rii.uthscsa.edu/mango).
Our ALE analysis identified 16 clusters of consistent activation related to large-scale spatial ability. The largest two clusters of activation in the bilateral limbic lobe were centered in the bilateral parahippocampal gyrus. Other clusters were primarily located in the bilateral posterior lobe, bilateral occipital lobe, bilateral parietal lobe, right anterior lobe, bilateral frontal lobe, and right sub-lobar area (Table 2, Figure 3). Clusters exhibiting consistent activation in the analysis of small-scale spatial ability were concentrated mainly within the bilateral parietal lobe, bilateral occipital lobe, bilateral frontal lobe, right posterior lobe, and left sub-lobar area (Table 3 and Figure 3).
Figure 3. ALE meta-analysis of neuroimaging studies regarding large-scale spatial ability (A) and small-scale spatial ability (B). Coordinates are presented in millimeters (mm). ALE, activation likelihood estimation.
We then compared the ALE results for large- and small-scale spatial abilities to identify common and distinct neural regions associated with each ability. Conjunction analysis revealed that bilateral sub-gyrus, right superior frontal gyrus (SFG), right superior parietal lobule (SPL), right middle occipital gyrus (MOG), right superior occipital gyrus (SOG), left inferior occipital gyrus (IOG), and bilateral precuneus were activated for both large- and small-scale spatial abilities (Table 4 and Figure 4). In addition, large- and small-scale spatial ability contrasts demonstrated correspondence in the bilateral parahippocampal gyrus, left lingual gyrus, bilateral culmen, right middle temporal gyrus (MTG), left declive, left SOG, and right lentiform nucleus (Table 5 and Figure 4). Conversely, small- and large-scale spatial ability contrasts converged in the bilateral precuneus, right inferior frontal gyrus (IFG), right precentral gyrus, left inferior parietal lobule (IPL), left supramarginal gyrus, left SPL, right IOG, and left middle frontal gyrus (MFG; Table 5 and Figure 4).
Figure 4. Results of conjunction and contrast analyses. (A) The common regions associated with large- and small-scale spatial ability. (B) Brain regions exhibiting greater activation for large-scale spatial ability than for small-scale spatial ability. (C) Brain regions exhibiting greater activation for small-scale spatial ability than for large-scale spatial ability. Coordinates are presented in millimeters (mm).
In the present study, we conducted an ALE meta-analysis to elucidate the neural basis of large- and small-scale spatial abilities. Our results indicated that large-scale spatial tasks were associated with increased activation in the parahippocampal gyrus, SOG, MOG, precuneus, posterior cingulate, lingual gyrus, sub-gyrus, right SFG, left cuneus, and left SPL. This result is consistent with the findings of Boccia et al. (2014) and Kühn and Gallinat (2014) regarding individual navigational capabilities. Furthermore, cognitive processing of individual large-scale spatial information was associated with activation in the declive, culmen, left IOG, right lentiform nucleus, and right claustrum. These findings are in accordance with those of several previous studies, which have also suggested that the parahippocampal gyrus plays a key role in large-scale spatial ability (Connor and Knierim, 2017; Cullen and Taube, 2017; Epstein et al., 2017; Lisman et al., 2017; Moser et al., 2017).
Our analysis also revealed that small-scale spatial tasks were associated with higher activation in the IPL, MOG, left SPL, left IOG, MFG, and IFG. These results are consistent with the findings of Tomasino and Gremese (2016) regarding individual mental rotation capabilities. Furthermore, cognitive processing of individual small-scale spatial information was also associated with activation in the precuneus, right MFG, right tuber, right declive, and left insula.
Notably, our ALE conjunction analysis revealed that the sub-gyrus, right SFG, right SPL, right MOG, right SOG, left IOG, and precuneus participate in the cognitive processing of both large- and small-scale spatial information. These results are in accordance with the model proposed by Hegarty et al. (2006), which argues that there is a partial intersection/association between individual large-scale and small-scale spatial abilities. Hegarty et al. (2006) speculated that this association arises from the fact that spatial ability is not a completely independent cognitive function, as both large- and small-scale spatial ability involve cognitive processes such as visual processing and working memory. Indeed, our results support this notion, suggesting a shared neural basis for large- and small-scale spatial ability. Extensive research has demonstrated that the sub-gyrus, right SPL, right MOG, right SOG, left IOG, and precuneus are associated with the processing of visual spatial information (Fink et al., 2003; Price and Devlin, 2003; Bristow et al., 2005; Konen et al., 2005; Slotnick and Moo, 2006; Deutschländer et al., 2008; Choi et al., 2010; Seo et al., 2012). Additional studies have indicated that the sub-gyrus, right SFG, and precuneus are significantly associated with working memory tasks (Mitchell, 2007; Chein et al., 2011; Fassbender et al., 2011; Ma et al., 2012; Opitz et al., 2014). Furthermore, the right sub-gyrus, right SFG, right MOG, and precuneus have been associated with attention (Wu et al., 2007; Kelley et al., 2008; Thakral and Slotnick, 2009; Li et al., 2012; Majerus et al., 2012), while the sub-gyrus and left precuneus have been associated with the planning process (Wagner et al., 2006; Jankowski et al., 2009; Crescentini et al., 2012). The sub-gyrus has also been implicated in executive function (Kerstin et al., 2005; Schubotz and von Cramon, 2009; Jin et al., 2012; Dambacher et al., 2014).
Taken together, these findings suggest that both large- and small-scale spatial ability involve visual processing and working memory of spatial information, as well as cognitive processes such as attention, planning, and executive control. These processes may represent vital cognitive and behavioral foundations for the overlap and interaction between large- and small-scale spatial ability. In this context, an individual first relies on attention to capture visual spatial information, following which working memory aids in the preparation and processing of the input. The individual then develops a detailed plan to finish the current spatial cognitive task. The planning process is often embodied in the corresponding task execution strategy for large-scale spatial tasks. For small-scale spatial tasks, it is often embodied in the operational scheme developed by the participant according to his/her own operational capabilities. Execution of the plan and behavioral responses during the experimental task are under the control of executive function.
We further analyzed the specific neural activity involved in large- and small-scale spatial abilities. Our analysis indicated that large-scale spatial tasks were associated with more intense activation of the parahippocampal gyrus, left lingual gyrus, culmen, right MTG, left declive, left SOG, and right lentiform nucleus. Indeed, previous studies have indicated that the parahippocampal gyrus, left lingual gyrus, culmen, and right MTG are significantly associated with the processing of information related to virtual environments (Weniger et al., 2009, 2013; Mellet et al., 2010; Nieuwland, 2012; Fairhurst et al., 2013). Additional studies have demonstrated that the parahippocampal gyrus, left lingual gyrus, culmen, and left SOG are associated with the processing of information related to construction, scene, and place (Suzuki et al., 2005; Henderson et al., 2008; Mullally et al., 2012; Rémy, 2014; Sulpizio et al., 2014; Zeidman et al., 2015). The parahippocampal gyrus, left lingual gyrus, and culmen have also been implicated in autobiographical memory (Maguire and Dhassabis, 2010; Whalley et al., 2012; Wilbers et al., 2012). Several studies have further reported that the parahippocampal gyrus, culmen, and left SOG are associated with episodic memory (Suzuki et al., 2009; Reggev et al., 2011; Demaster and Ghetti, 2013), while the right parahippocampal gyrus, left lingual gyrus, left culmen, and left declive are associated with semantic memory (Grossman et al., 2003; Assaf et al., 2005; Kodama et al., 2015). Recent research has also demonstrated that the right lentiform nucleus is associated with emotional processing (Goldin et al., 2008; Telzer et al., 2014; Wardle et al., 2014; Touroutoglou et al., 2015). Taken together, these findings suggest that, relative to small-scale spatial ability, large-scale spatial ability relies more heavily on the cognitive processing of spatial information related to aspects of the virtual environment (e.g., construction, scene, place, etc.), and on the autobiographical, episodic, and semantic aspects of memory. Our findings further suggest that large-scale spatial ability is associated with greater emotional responses.
In contrast, small-scale spatial tasks were associated with more intense activation in the precuneus, right IFG, right precentral gyrus, left IPL, left supramarginal gyrus, left SPL, right IOG, and left MFG. Previous studies have revealed that the precuneus, right IFG, right precentral gyrus, left IPL, left SPL, right IOG, and left MFG are associated with motor imagery (Kaladjian et al., 2007; De Lange, 2008; Chen et al., 2009; Thompson and Baccus, 2012; Chinier et al., 2014; Lissek et al., 2014). Additional studies have demonstrated that the precuneus, right IFG, right precentral gyrus, left supramarginal gyrus, left IPL, and left MFG are associated with control of the finger and hand, particular for grasping and tapping movements (Culham et al., 2003; Vandermeeren et al., 2003; Müller et al., 2011; Schweisfurth et al., 2011; Akhlaghi et al., 2012; Lavrysen et al., 2012; Stefanics and Czigler, 2012). Moreover, the right precentral gyrus, left IPL, and left supramarginal gyrus have been associated with working memory (Mitchell, 2007; Burgess and Braver, 2010; Chein et al., 2011; Fassbender et al., 2011). In addition, several studies have indicated that the precuneus, right IFG, right precentral gyrus, left IPL, and left supramarginal gyrus are involved in arithmetic and calculation (Gruber et al., 2001; Fehr et al., 2007; Benn et al., 2013). Some studies have also reported that the right precentral gyrus and right IFG are assocaited with matching activities (Darcy Burgund et al., 2005; Soulières et al., 2009). Taken together, these findings indicate that, relative to large-scale spatial ability, small-scale spatial ability relies more heavily on motor imagery, mental processes associated with finger and hand movements, and cognitive processes such as working memory, calculation, and matching.
However, it is noteworthy that although there are brain regions related to individuals' working memory in the common part of large- and small-scale spatial ability and in the unique part of the small-scale spatial ability, these brain regions are still different with respect to the cognitive process and the functions they are specifically involved in. First of all, the cognitive process shows that the working memory includes such three processes as encoding, maintenance, and retrieval; however, the sub-gyrus in the common part is involved in the encoding process, precuneus is involved in the maintenance process, and SFG is involved in the retrieval process; whereas the precentral gyrus in the unique part is involved in the encoding and maintenance processes, IPL is involved in the retrieval process, and supramarginal gyrus is involved in the encoding and retrieval processes (Langel et al., 2014). Then, the function of the working memory shows that these brain regions serve as the significant neural foundations of the central executive system in individuals' working memory. The central execution system, as the core component of the working memory, has such important functions as selection, inhibition, updating, and conversion (Collette and Linden, 2002). In the common part, however, SFG is mainly responsible for updating in the central executive system, and precuneus is mainly responsible for selection. In addition, IPL and supramarginal gyrus in the unique part are also crucial brain regions for updating (Bledowski et al., 2009; Borst and Anderson, 2013). To sum up, we believe that although both large- and small-scale spatial ability involves the cognitive process of encoding, maintenance, and retrieval in individuals' working memory, small-scale spatial ability also involves working memory processes that more precuneus, IPL and supramarginal gyrus are involved in. It can also be understood this way: although working memory is involved in the cognitive processing of large- and small-scale spatial information of individuals at the same time, the involvement in the latter is wider and deeper. On the other hand, the selection and updating functions of the central executive system in the working memory also constitute the common basis of individuals' large- and small-scale spatial ability, but the small-scale spatial ability, compared with the large-scale spatial ability, is more dependent on the updating function. Therefore, the result that this working memory is present in the common part of the large- and small-scale spatial ability and in the unique part of the small-scale spatial ability is not contradictory. Moreover, this result, to a certain extent, also mutually supports the phenomenon that the working memory training as found by many researchers in the domain of cognitive training can lead to a significant transfer influence on individuals' spatial ability (Alloway et al., 2013; Borella et al., 2017; Nilsson et al., 2017).
Although large- and small-scale spatial ability are to some extent associated at both the neural and behavioral levels, they are profoundly different in many ways. Large-scale spatial ability reflects more of the individual's ability to process spatial information related to actual or virtual situations. In such cases, regions associated with the cognitive processing of environmental information (e.g., construction, scene, place, etc.) will be activated more intensely, following which brain regions corresponding to autobiographical and episodic memory will be engaged. In addition, as large-scale spatial tasks often require more detailed explanation, semantic memory load will be greater for such tasks than for those associated with small-scale spatial ability. Interestingly, we also observed activation of emotion-related regions during large-scale spatial tasks. One explanation for this finding was that experimental tasks in general might elicit emotional responses. Individuals are more prone to such negative emotions as anxiety and fear when completing large-scale spatial tasks. For instance, Lawton (1994) and Malinowski and Gillespie (2001) found that wayfinding performance was negatively correlated with anxiety. Lawton and Kallai (2002) further discovered that women showed greater anxiety than men in large-scale spatial tasks. In addition, many scholars focus on the stress responses of individuals in navigation tasks (Duncko et al., 2007; Schwabe et al., 2007, 2009). Therefore, when compared with small-scale spatial ability, the large-scale spatial ability of individuals is more often studied by being linked to emotional factors. Consistent with these studies, our findings also showed that individuals have actually activated the emotion-related brain regions when completing large-scale spatial tasks. Alternatively, experimental materials depicting beautiful scenery, architecture, or familiar scenes may evoke various emotional responses among individual participants. Such task elements as the stimulus materials and the experimental scenarios for the large-scale spatial tasks at this stage are much more plentiful than smaller-scale space tasks. It is for sure that this also prompts them to have more elements affecting individual emotions potentially. So, can the improved and enriched small-scale spatial tasks or research paradigms affect individuals' activities and emotion-related brain regions? We believe that this question still warrants validation of more studies.
In contrast, our findings indicated that small-scale spatial ability was more strongly associated with brain regions involved in motor imagery, grasping, calculation, and matching, suggesting that this form of spatial ability reflects an individual's ability to process spatial information for specific objects. In this case, judgments are made based on imagination and mental representations. Thus, grasping, calculation, and matching functions may aid individuals in operating upon mental representations. Because imagination and operation abilities reflect working memory function, our findings suggest that small-scale spatial ability relies more heavily on working memory than other aspects of memory function. Given these findings, we propose a new model, as shown in Figure 5.
Figure 5. Model of the relationship between large- and small-scale spatial ability based on the current findings.
The current model was proposed on basis of Hegarty et al. (2006)'s model. We validated and supplemented their model from the neuroimaging level. We know that each region of our brain has many corresponding functions, and we proposed the current model mainly based on the following three considerations: first, based on our own literature accumulation and experience and taking into account the functional intelligence of the relevant brain regions from such database as Neurosynth (http://neurosynth.org/) and Brainnetome (http://atlas.brainnetome.org/bnatlas.html), we determined the aspects of cognitive function that brain regions mainly involve in the current ALE results; then, we examined whether these functions were consistent or directly associated with the cognitive activity components of the various components of Hegarty et al. (2006)'s model, thereby determining whether our findings can support Hegarty et al. (2006)'s model; lastly, by summarizing the work in the previous two steps, findings of previous studies, and our understanding of the individual spatial ability, we proposed the current model that would supplement and deepen Hegarty et al. (2006)'s model finally.
In general, the findings of the present research supported and extended the model proposed by Hegarty et al. (2006). With regard to the large-scale spatial ability, first, Hegarty et al. summed up the cognitive components specific to individuals' large-scale spatial ability based on his own studies, including addressing and spatial learning based on sensing of body motions. In fact, both “updating” and “learning” refer to the process of encoding the spatial stimulus information by individuals, and this spatial stimulus information is on basis of sensing of body motions. According to Hegarty et al. (2006), on the one hand, these stimuli were derived from the real environment; on the other hand, these stimulus messages also contained information regarding the “self.” Our model based on the current ALE results also supported such a view. According to our model, individuals' large-scale spatial ability not only involves individuals' processing of such real-world spatial stimulus information as constructions, scenes and places, but also contains individuals' cognitive processing of the virtual context space stimulus information. Moreover, we have also found that the brain regions associated with individuals' autobiographical memory, episodic memory, semantic memory and emotional responses are also deeply involved in individuals' large-scale spatial cognition, among which the first two items are associated with individuals' “self” information. Secondly, Hegarty et al. (2006) also thought that individuals' large- and small-scale spatial ability involves individuals' encoding of the visual spatial stimulus information, maintenance of spatial representations in the working memory and process of inference on the basis of these spatial representations. Not only does our model support the above conclusions fully, but also we further propose that all the processes of encoding, maintenance and retrieval, and the core central execution system components in the working memory are important for individuals' spatial ability. In addition, we believe that the final inference process involves mainly the ability of individuals' planning and executive control. Finally, as mentioned earlier (also as Figure 5), another important addition and extension of the current research to Hegarty et al. (2006)'s model was that we attempted to present the cognitive ability base specific to individuals' small-scale spatial ability.
Notably, we observed no distinct differences in the cognitive processing strategies utilized during large- and small-scale spatial tasks. Currently, strategies for processing spatial information are divided into three main categories: (1) egocentric-allocentric (Galati et al., 2000), (2) whole-part (Li and O'Boyle, 2011; Janssen and Geiser, 2012), and (3) motor imagination-visual processing (Tomasino and Gremese, 2016). However, the basis and theory of these three categories are quite similar, and we speculate that the latter two represent variations upon the first category. Whole-part strategies are likely to be utilized during the processing of large-scale spatial information, while motor imagination-visual processing strategies are likely to be utilized during the processing of small-scale spatial information. Some researchers have hypothesized that large-scale spatial tasks primarily require egocentric strategies, while small-scale tasks primarily require allocentric strategies (Malinowski, 2001; Zacks et al., 2001; Peña et al., 2008).
A meta-analysis by Boccia et al. (2014) revealed that navigation tasks were associated with greater activation of the right SOG, right angular gyrus, and right precuneus when egocentric rather than allocentric strategies are adopted. A subsequent meta-analysis by Tomasino and Gremese (2016) revealed that small-scale spatial tasks were associated with greater activation in the right postcentral gyrus, left IPL, left postcentral gyrus, left SPL, and right angular gyrus when egocentric strategies are adopted. In contrast, allocentric strategies were associated with greater activation of the right precuneus, right SFG, right SOG, left MOG, left SPL, left inferior temporal gyrus (ITG), left IOG, left MOG, and right posterior medial frontal gyrus. Interestingly, the right precuneus, right SOG, and left SPL reflect opposing processing strategies for large- and small-scale spatial tasks. Taken together, these findings suggest that there is no absolute difference in cognitive processing strategies between large- and small-scale spatial ability, and that either egocentric or allocentric strategies may be utilized in both task types. Differences in strategy selection may be related to individual differences such as the interaction between gender and verbal working memory (VWM)/visual spatial working memory (VSWM) ratio (Wang and Carr, 2014).
The present study possesses several limitations of note. First, unlike meta-analyses performed in other fields of research, ALE calculations based on neuroimaging do not consider the size of an effect; consequently, they cannot include evidence for the absence of an effect (i.e., so-called null results). Furthermore, ALE analyses cannot illuminate the temporal dynamics of cognitive processes (Winlove et al., 2018). Secondly, as most participants in the included studies were relatively young, we were unable to explore the effect of life-long development on the relationship between large- and small-scale spatial ability. Finally, we investigated spatial ability in healthy individuals only. Further studies are required to determine whether similar results can be obtained in patients with major depressive disorder, schizophrenia, attention deficit hyperactivity disorder, autism, and Alzheimer's disease.
The results of the present study verify and expand upon the theoretical model of spatial ability proposed by Hegarty et al. (2006). Our analysis revealed a shared neural basis between large- and small-scale spatial abilities, as well as specific yet independent neural bases underlying each. Based on these findings, we proposed a more comprehensive version of the behavioral model (Figure 5). Our results further suggest that there is no absolute difference in cognitive strategies adopted during large- and small-scale spatial tasks.
This study was approved by the Human Research Ethics Committee of Shaanxi Normal University.
YL, FK, MJ, YL, JL, and XY design of the study. YL, FK, MJ, and YL data collection, coding, and data analysis. YL, FK, MJ, and XY paper writing and revising.
The authors declare that the research was conducted in the absence of any commercial or financial relationships that could be construed as a potential conflict of interest.
This research was supported by the Fundamental Research Funds for the Central Universities (2016CBZ010; 2017TS115), Key research and development programs in Shaanxi (2017SF-271) and the Major Project of Medicine Science and Technology of PLA (AWS17J012).
The Supplementary Material for this article can be found online at: https://www.frontiersin.org/articles/10.3389/fnins.2018.01021/full#supplementary-material
Supplementary Table 1. PRISMA 2009 Checklist.
Supplementary Table 2. Samples of coordinate.
* ^Papers with asterisk were included in the meta-analysis.
Akhlaghi, H., Corben, L., Georgioukaristianis, N., Bradshaw, J., Delatycki, M. B., Storey, E., et al. (2012). A functional mri study of motor dysfunction in friedreich's ataxia. Brain Res. 1471, 138–154. doi: 10.1016/j.brainres.2012.06.035
Alloway, T. P., Bibile, V., and Lau, G. (2013). Computerized working memory training: Can it lead to gains in cognitive skills in students? Comput. Hum. Behav. 29, 632–638. doi: 10.1016/j.chb.2012.10.023
Assaf, M., Rivkin, P. R., Kuzu, C. H., Calhoun, V. D., Kraut, M. A., Groth, K. M., et al. (2005). Abnormal object recall and anterior cingulate overactivation correlate with formal thought disorder in schizophrenia. Biol. Psychiatr. 59, 452–459. doi: 10.1016/j.biopsych.2005.07.039
*Baumann, O., Chan, E., and Mattingley, J. B. (2010). Dissociable neural circuits for encoding and retrieval of object locations during active navigation in humans. Neuroimage 49, 2816–2825. doi: 10.1016/j.neuroimage.2009.10.021
*Baumann, O., Chan, E., and Mattingley, J. B. (2012). Distinct neural networks underlie encoding of categorical versus coordinate spatial relations during active navigation. Neuroimage 60, 1630–1637. doi: 10.1016/j.neuroimage.2012.01.089
Benn, Y., Wilkinson, I. D., Zheng, Y., Cohen, K. K., Romanowski, C. A., Siegal, M., et al. (2013). Differentiating core and co-opted mechanisms in calculation: the neuroimaging of calculation in aphasia. Brain Cogn. 82, 254–264. doi: 10.1016/j.bandc.2013.04.012
*Blacker, K. J., and Courtney, S. M. (2016). Distinct neural substrates for maintaining locations and spatial relations in working memory. Front. Hum. Neurosci. 10:594. doi: 10.3389/fnhum.2016.00594
Bledowski, C., Rahm, B., and Rowe, J. B. (2009). What “works” in working memory? separate systems for selection and updating of critical information. J. Neurosci. 29, 13735–13741. doi: 10.1523/JNEUROSCI.2547-09.2009
Boccia, M., Nemmi, F., and Guariglia, C. (2014). Neuropsychology of environmental navigation in humans: review and meta-analysis of fmri studies in healthy participants. Neuropsychol. Rev. 24, 236–251. doi: 10.1007/s11065-014-9247-8
*Bodin, K., Elgh, E., Eriksson, J., Janlert, L. E., and Nyberg, L. (2010). “Effects of interactivity and 3D-motion on mental rotation brain activity in an immersive virtual environment,” Sigchi Conference on Human Factors in Computing Systems (Atlanta, GA: ACM), 869–878.
Borella, E., Carretti, B., Meneghetti, C., Carbone, E., Vincenzi, M., and Madonna, J. C. (2017). Is working memory training in older adults sensitive to music? Psychol. Res. doi: 10.1007/s00426-017-0961-8. [Epub ahead of print].
Borst, J. P., and Anderson, J. R. (2013). Using model-based functional mri to locate working memory updates and declarative memory retrievals in the fronto-parietal network [neuroscience]. Proc. Natl. Acad. Sci. U.S.A. 110, 1628–1633. doi: 10.1073/pnas.1221572110
Bosnyak, A., and Nagy-Kondor, R. (2008). The spatial ability and spatial geometrical knowledge of university students majored in mathematics. Acta Didactica Universitatis Univer. Comenianae 1–25. Available online at: https://www.ddm.fmph.uniba.sk/ADUC/files/Issue8/01Bosnyak-Nagy.pdf
Bristow, D., Frith, C., and Rees, G. (2005). Two distinct neural effects of blinking on human visual processing. Neuroimage 27, 136–145. doi: 10.1016/j.neuroimage.2005.03.037
*Brown, T. I., Ross, R. S., Keller, J. B., Hasselmo, M. E., and Stern, C. E. (2010). Which way was i going? contextual retrieval supports the disambiguation of well-learned overlapping navigational routes. J. Neurosci. Offi. 30, 7414–7422. doi: 10.1523/JNEUROSCI.6021-09.2010
Burgess, G. C., and Braver, T. S. (2010). Neural mechanisms of interference control in working memory: effects of interference expectancy and fluid intelligence. PLoS ONE 5:e12861. doi: 10.1371/journal.pone.0012861
*Carrillo, B., Gómez-Gil, E., Rametti, G., Junque, C., Gomez, A., Karadi, K., et al. (2010). Cortical activation during mental rotation in male-to-female and female-to-male transsexuals under hormonal treatment. Psychoneuroendocrinology 35, 1213–1222. doi: 10.1016/j.psyneuen.2010.02.010
Chein, J. M., Moore, A. B., and Conway, A. R. (2011). Domain-general mechanisms of complex working memory span. Neuroimage 54, 550–559. doi: 10.1016/j.neuroimage.2010.07.067
Chen, H., Yang, Q., Liao, W., Gong, Q., and Shen, S. (2009). Evaluation of the effective connectivity of supplementary motor areas during motor imagery using granger causality mapping. Neuroimage 47, 1844–1853. doi: 10.1016/j.neuroimage.2009.06.026
Chinier, E., N'Guyen, S., Lignon, G., Ter, M. A., Richard, I., and Dinomais, M. (2014). Effect of motor imagery in children with unilateral cerebral palsy: fmri study. Ann. Phys. Rehabil. Med. 57:e339. doi: 10.1016/j.rehab.2014.03.1242
Choi, M. H., Lee, S. J., Yang, J. W., Choi, J. S., Kim, H. S., Kim, H. J., et al. (2010). Activation of the limbic system under 30% oxygen during a visuospatial task: an fmri study. Neurosci. Lett. 471, 70–73. doi: 10.1016/j.neulet.2010.01.013
*Clemente, M., Rey, B., Rodríguezpujadas, A., Barrosloscertales, A., Baños, R. M., Botella, C., et al. (2013). An fmri study to analyze neural correlates of presence during virtual reality experiences. Interact. Comput. 26, 269–284. doi: 10.1093/iwc/iwt037
Clements, D. H. (1999). “Geometric and spatial thinking in young children,” in Mathematics in the Early Years, ed J. V. Copley (Reston, VA: National Council of Teachers of Mathematics), 27–29.
Collette, F., and Linden, M. V. D. (2002). Brain imaging of the central executive component of working memory. Neurosci. Biobehav. Rev. 26, 105–125. doi: 10.1016/S0149-7634(01)00063-X
Connor, C. E., and Knierim, J. J. (2017). Integration of objects and space in perception and memory. Nat. Neurosci. 20, 1493–1503. doi: 10.1038/nn.4657
*Corradi-Dell'Acqua, C., Tomasino, B., and Fink, G. R. (2009). What is the position of an arm relative to the body? neural correlates of body schema and body structural description. J. Neurosci. Offi. 29, 4162–4171. doi: 10.1523/JNEUROSCI.4861-08.2009
*Creem, S. H., Downs, T. H., Wraga, M., Harrington, G. S., Proffitt, D. R., and Downs, J. H. (2001). An fmri study of imagined self-rotation. Cogn. Affect. Behav. Neurosci. 1, 239–249. doi: 10.3758/CABN.1.3.239
*Creem-Regehr, S., Neil, J., and Yeh, H. (2007). Neural correlates of two imagined egocentric transformations. Neuroimage 35, 916–927. doi: 10.1016/j.neuroimage.2006.11.057
Crescentini, C., Seyed-Allaei, S., Vallesi, A., and Shallice, T. (2012). Two networks involved in producing and realizing plans. Neuropsychologia 50, 1521–1535. doi: 10.1016/j.neuropsychologia.2012.03.005
Culham, J. C., Danckert, S. L., Souza, J. F. X. D., Gati, J. S., Menon, R. S., and Goodale, M. A. (2003). Visually guided grasping produces fmri activation in dorsal but not ventral stream brain areas. Exp. Brain Res. 153, 180–189. doi: 10.1007/s00221-003-1591-5
Cullen, K. E., and Taube, J. S. (2017). Our sense of direction: progress, controversies and challenges. Nat. Neurosci. 20, 1465–1473. doi: 10.1038/nn.4658
Dambacher, F., Sack, A. T., Lobbestael, J., Arntz, A., Brugman, S., and Schuhmann, T. (2014). A network approach to response inhibition: dissociating functional connectivity of neural components involved in action restraint and action cancellation. Eur. J. Neurosci. 39, 821–831. doi: 10.1111/ejn.12425
Darcy Burgund, E., Lugar, H. M., Schlaggar, B. L., and Petersen, S. E. (2005). Task demands modulate sustained and transient neural activity during visual-matching tasks. Neuroimage 25, 511–519. doi: 10.1016/j.neuroimage.2004.12.039
De Lange, F. P. (2008). Motor imagery: a window into the mechanisms and alterations of the motor system. Cortex 44, 494–506. doi: 10.1016/j.cortex.2007.09.002
*de Lange, F. P., Hagoort, P., and Toni, I. (2005). Neural topography and content of movement representations. J. Cogn. Neurosci. 17, 97–112. doi: 10.1162/0898929052880039
*De Lange, F. P., Helmich, R. C., and Toni, I. (2006). Posture influences motor imagery: an fmri study. Neuroimage 33, 609–617. doi: 10.1016/j.neuroimage.2006.07.017
Demaster, D. M., and Ghetti, S. (2013). Developmental differences in hippocampal and cortical contributions to episodic retrieval. Cortex 49, 1482–1493. doi: 10.1016/j.cortex.2012.08.004
Deutschländer, A., Hüfner, K., Kalla, R., Stephan, T., Dera, T., Glasauer, S., et al. (2008). Unilateral vestibular failure suppresses cortical visual motion processing. Brain 131, 1025–1034. doi: 10.1093/brain/awn035
Duncko, R., Cornwell, B., Cui, L., Merikangas, K. R., and Grillon, C. (2007). Acute exposure to stress improves performance in trace eyeblink conditioning and spatial learning tasks in healthy men. Learn. Memory 14, 329–335. doi: 10.1101/lm.483807
*Ebisch, S. J., Perrucci, M. G., Mercuri, P., Romanelli, R., Mantini, D., Romani, G. L., et al. (2012). Common and unique neuro-functional basis of induction, visualization, and spatial relationships as cognitive components of fluid intelligence. Neuroimage 62, 331–342. doi: 10.1016/j.neuroimage.2012.04.053
*Ecker, C., Brammer, M. J., David, A. S., and Williams, S. C. (2006). Time-resolved fmri of mental rotation revisited-dissociating visual perception from mental rotation in female subjects. Neuroimage 32, 432–444. doi: 10.1016/j.neuroimage.2006.03.031
Eickhoff, S. B., Bzdok, D., Laird, A. R., Kurth, F., and Fox, P. T. (2012). Activation likelihood estimation meta-analysis revisited. Neuroimage 59, 2349–2361. doi: 10.1016/j.neuroimage.2011.09.017
Eickhoff, S. B., Laird, A. R., Fox, P. M., Lancaster, J. L., and Fox, P. T. (2017). Implementation errors in the gingerale software: description and recommendations. Hum. Brain Mapp. 38, 7–11. doi: 10.1002/hbm.23342
Eickhoff, S. B., Laird, A. R., Grefkes, C., Wang, L. E., Zilles, K., and Fox, P. T. (2009). Coordinate-based activation likelihood estimation meta-analysis of neuroimaging data: a random-effects approach based on empirical estimates of spatial uncertainty. Hum. Brain Mapp. 30, 2907–2926. doi: 10.1002/hbm.20718
Eickhoff, S. B., Nichols, T. E., Laird, A. R., Hoffstaedter, F., Amunts, K., Fox, P. T., et al. (2016). Behavior, sensitivity, and power of activation likelihood estimation characterized by massive empirical simulation. Neuroimage 137, 70–85. doi: 10.1016/j.neuroimage.2016.04.072
*Elizabeth, W., and Paul, D. (2011). Activation in a frontoparietal cortical network underlies individual differences in the performance of an embedded figures task. PLoS ONE 6:e20742. doi: 10.1371/journal.pone.0020742
Epstein, R. A., Patai, E. Z., Julian, J. B., and Spiers, H. J. (2017). The cognitive map in humans: spatial navigation and beyond. Nat. Neurosci. 20, 1504–1513. doi: 10.1038/nn.4656
*Etchamendy, N., Konishi, K., Pike, G. B., Marighetto, A., and Bohbot, V. D. (2012). Evidence for a virtual human analog of a rodent relational memory task: a study of aging and fmri in young adults. Hippocampus 22, 869–880. doi: 10.1002/hipo.20948
Fairhurst, M. T., Janata, P., and Keller, P. E. (2013). Being and feeling in sync with an adaptive virtual partner: brain mechanisms underlying dynamic cooperativity. Cereb. Cortex 23, 2592–2600. doi: 10.1093/cercor/bhs243
Fassbender, C., Schweitzer, J. B., Cortes, C. R., Tagamets, M. A., Windsor, T. A., Reeves, G. M., et al. (2011). Working memory in attention deficit/hyperactivity disorder is characterized by a lack of specialization of brain function. PLoS ONE 6:e27240. doi: 10.1371/journal.pone.0027240
Fehr, T., Code, C., and Herrmann, M. (2007). Common brain regions underlying different arithmetic operations as revealed by conjunct fmri-bold activation. Brain Res. 1172, 93–102. doi: 10.1016/j.brainres.2007.07.043
*Ferri, F., Frassinetti, F., Ardizzi, M., Costantini, M., and Gallese, V. (2014). A sensorimotor network for the bodily self. J. Cogn. Neurosci. 24, 1584–1595. doi: 10.1162/jocn_a_00230
Fink, G. R., Marshall, J. C., Weiss, P. H., Stephan, T., Grefkes, C., Shah, N. J., et al. (2003). Performing allocentric visuospatial judgments with induced distortion of the egocentric reference frame: an fmri study with clinical implications. Neuroimage 20, 1505–1517. doi: 10.1016/j.neuroimage.2003.07.006
Galati, G., Lobel, E., Vallar, G., Berthoz, A., Pizzamiglio, L., and Bihan, D. L. (2000). The neural basis of egocentric and allocentric coding of space in humans: a functional magnetic resonance study. Exp. Brain Res. 133, 156–164. doi: 10.1007/s002210000375
*Ganesh, S., Schie, H. T. V., Cross, E. S., Lange, F. P. D., and Wigboldus, D. H. J. (2015). Disentangling neural processes of egocentric and allocentric mental spatial transformations using whole-body photos of self and other. Neuroimage 116, 30–39. doi: 10.1016/j.neuroimage.2015.05.003
*Gao, M., Zhang, D., Wang, Z., Liang, B., Cai, Y., Gao, Z., et al. (2017). Mental rotation task specifically modulates functional connectivity strength of intrinsic brain activity in low frequency domains: a maximum uncertainty linear discriminant analysis. Behav. Brain Res. 320, 233–243. doi: 10.1016/j.bbr.2016.12.017
*Gogos, A., Gavrilescu, M., Davison, S., Searle, K., Adams, J., Rossell, S. L., et al. (2010). Greater superior than inferior parietal lobule activation with increasing rotation angle during mental rotation: an fmri study. Neuropsychologia 48, 529–535. doi: 10.1016/j.neuropsychologia.2009.10.013
*Goh, J. O., Hebrank, A. C., Sutton, B. P., Chee, M. W. L., Sim, S. K. Y., and Park, D. C. (2013). Culture-related differences in default network activity during visuo-spatial judgments. Soc. Cogn. Affect. Neurosci. 8, 134–142. doi: 10.1093/scan/nsr077
Goldin, P., McRae, R., Ramel, K., Wiveka, W., and Gross, J. J. (2008). The neural bases of emotion regulation: reappraisal and suppression of negative emotion. Biol. Psychiatr. 63, 577–586. doi: 10.1016/j.biopsych.2007.05.031
*Grön, G., Wunderlich, A. P., Spitzer, M., Tomczak, R., and Riepe, M. W. (2000). Brain activation during human navigation: gender-different neural networks as substrate of performance. Nat. Neurosci. 3, 404–408. doi: 10.1038/73980
Grossman, M., Koenig, P., Glosser, G., Devita, C., Moore, P., Rhee, J., et al. (2003). Neural basis for semantic memory difficulty in alzheimer's disease: an fmri study. Brain J. Neurol. 126, 292–311. doi: 10.1093/brain/awg027
Gruber, O., Indefrey, P., and Steinmetz, H. (2001). Dissociating neural correlates of cognitive components in mental calculation. Cereb. Cortex 11, 350–359. doi: 10.1093/cercor/11.4.350
*Halari, R., Sharma, T., Hines, M., Andrew, C., Simmons, A., and Kumari, V. (2006). Comparable fmri activity with differential behavioural performance on mental rotation and overt verbal fluency tasks in healthy men and women. Exp. Brain Res. 169, 1–14. doi: 10.1007/s00221-005-0118-7
*Hartley, T., and Maguire, E. A. (2003). The well-worn route and the path less traveled: distinct neural bases of route following and wayfinding in humans. Neuron 37, 877–888. doi: 10.1016/S0896-6273(03)00095-3
Hegarty, M., Montello, D. R., Richardson, A. E., Ishikawa, T., and Lovelace, K. (2006). Spatial abilities at different scales: individual differences in aptitude-test performance and spatial-layout learning. Intelligence 34, 151–176. doi: 10.1016/j.intell.2005.09.005
Hegarty, M., and Waller, D. (2004). A dissociation between mental rotation and perspective-taking spatial abilities. Intelligence 32, 175–191. doi: 10.1016/j.intell.2003.12.001
Henderson, J. M., Larson, C. L., and Zhu, D. C. (2008). Full scenes produce more activation than close-up scenes and scene-diagnostic objects in parahippocampal and retrosplenial cortex: an fmri study. Brain Cogn. 66, 40–49. doi: 10.1016/j.bandc.2007.05.001
Hirshhorn, M., Grady, C., Rosenbaum, R. S., Winocur, G., and Moscovitch, M. (2011). The hippocampus is involved in mental navigation for a recently learned, but not a highly familiar environment: a longitudinal fmri study. Hippocampus 22, 842–852. doi: 10.1002/hipo.20944
Hirshhorn, M., Grady, C., Rosenbaum, R. S., Winocur, G., and Moscovitch, M. (2012). Brain regions involved in the retrieval of spatial and episodic details associated with a familiar environment: an fmri study. Neuropsychologia 50, 3094–3106. doi: 10.1016/j.neuropsychologia.2012.08.008
Höffler, T. N. (2010). Spatial ability: its influence on learning with visualizations—a meta-analytic review. Educ. Psychol. Rev. 22, 245–269. doi: 10.1007/s10648-010-9126-7
*Hugdahl, K., Thomsen, T., and Ersland, L. (2006). Sex differences in visuo-spatial processing: an fmri study of mental rotation. Neuropsychologia 44, 1575–1583. doi: 10.1016/j.neuropsychologia.2006.01.026
*Iaria, G., Chen, J. K., Guariglia, C., Ptito, A., and Petrides, M. (2007). Retrosplenial and hippocampal brain regions in human navigation: complementary functional contributions to the formation and use of cognitive maps. Eur. J. Neurosci. 25, 890–899. doi: 10.1111/j.1460-9568.2007.05371.x
*Iaria, G., Fox, C. J., Chen, J. K., Petrides, M., and Barton, J. J. (2008). Detection of unexpected events during spatial navigation in humans: bottom-up attentional system and neural mechanisms. Eur. J. Neurosci. 27, 1017–1025. doi: 10.1111/j.1460-9568.2008.06060.x
*Iaria, G., Petrides, M., Dagher, A., Pike, B., and Bohbot, V. D. (2003). Cognitive strategies dependent on the hippocampus and caudate nucleus in human navigation: variability and change with practice. J. Neurosci. Off. 23, 5945–5952. doi: 10.1523/JNEUROSCI.23-13-05945.2003
*IglóiI, K., Doeller, C. F., Berthoz, A., Rondi-Reig, L., and Burgess, N. (2010). Lateralized human hippocampal activity predicts navigation based on sequence or place memory. Proc. Natl. Acad. Sci. U.S.A. 107, 14466–14471. doi: 10.1073/pnas.1004243107
*Ino, T., Doi, T., Hirose, S., Kimura, T., Jin, I., and Fukuyama, H. (2007). Directional disorientation following left retrosplenial hemorrhage: a case report with fmri studies. Cortex 43, 248–254. doi: 10.1016/S0010-9452(08)70479-9
*Ino, T., Inoue, Y., Kage, M., Hirose, S., Kimura, T., and Fukuyama, H. (2002). Mental navigation in humans is processed in the anterior bank of the parieto-occipital sulcus. Neurosci. Lett. 322, 182–186. doi: 10.1016/S0304-3940(02)00019-8
*Jandl, N. M., Sprenger, A., Wojak, J. F., Göttlich, M., Münte, T. F., Krämer, U. M., et al. (2015). Dissociable cerebellar activity during spatial navigation and visual memory in bilateral vestibular failure. Neuroscience 305, 257–267. doi: 10.1016/j.neuroscience.2015.07.089
Jankowski, J., Scheef, L., Hüppe, C., and Boecker, H. (2009). Distinct striatal regions for planning and executing novel and automated movement sequences. Neuroimage 44, 1369–1379. doi: 10.1016/j.neuroimage.2008.10.059
Jansen, P. (2009). The dissociation of small- and large-scale spatial abilities in school-age children. Percept. Mot. Skills 109, 357–361. doi: 10.2466/pms.109.2.357-361
Janssen, A. B., and Geiser, C. (2012). Cross-cultural differences in spatial abilities and solution strategies— an investigation in cambodia and germany. J. Cross Cult. Psychol. 43, 533–557. doi: 10.1177/0022022111399646
*Janzen, G., and Jansen, C. (2010). A neural wayfinding mechanism adjusts for ambiguous landmark information. Neuroimage 52, 364–370. doi: 10.1016/j.neuroimage.2010.03.083
*Janzen, G., and Weststeijn, C. G. (2007). Neural representation of object location and route direction: an event-related fmri study. Brain Res. 1165, 116–125. doi: 10.1016/j.brainres.2007.05.074
Jin, F., Silvia, B., Dam, N. T., Evdokia, A., Gu, X., Laura, M., et al. (2012). Functional deficits of the attentional networks in autism. Brain Behav. 2, 647–660. doi: 10.1002/brb3.90
*Johnston, S., Leek, E. C., Atherton, C., Thacker, N., and Jackson, A. (2004). Functional contribution of medial premotor cortex to visuo-spatial transformation in humans. Neurosci. Lett. 355, 209–212. doi: 10.1016/j.neulet.2003.11.011
*Jordan, K., Heinze, H. J., Lutz, K., Kanowski, M., and JãNcke, L. (2001). Cortical activations during the mental rotation of different visual objects. Neuroimage 13, 143–152. doi: 10.1006/nimg.2000.0677
*Jordan, K., Wüstenberg, T., Heinze, H. J., Peters, M., and Jäncke, L. (2002). Women and men exhibit different cortical activation patterns during mental rotation tasks. Neuropsychologia 40, 2397–2408. doi: 10.1016/S0028-3932(02)00076-3
*Kaiser, S., Walther, S., Nennig, E., Kronmüller, K., Mundt, C., Weisbrod, M., et al. (2008). Gender-specific strategy use and neural correlates in a spatial perspective taking task. Neuropsychologia 46, 2524–2531. doi: 10.1016/j.neuropsychologia.2008.04.013
Kaladjian, A., Jeanningros, R., Azorin, J. M., Grimault, S., Anton, J. L., and Mazzola-Pomietto, P. (2007). Blunted activation in right ventrolateral prefrontal cortex during motor response inhibition in schizophrenia. Schizophr. Res. 97, 184–193. doi: 10.1016/j.schres.2007.07.033
*Kawamichi, H., Kikuchi, Y., Noriuchi, M., Senoo, A., and Ueno, S. (2007). Distinct neural correlates underlying two- and three-dimensional mental rotations using three-dimensional objects. Brain Res. 1144, 117–126. doi: 10.1016/j.brainres.2007.01.082
*Keehner, M., Guerin, S. A., Miller, M. B., Turk, D. J., and Hegarty, M. (2006). Modulation of neural activity by angle of rotation during imagined spatial transformations. Neuroimage 33, 391–398. doi: 10.1016/j.neuroimage.2006.06.043
Kelley, T. A., Serences, J. T., Giesbrecht, B., and Yantis, S. (2008). Cortical mechanisms for shifting and holding visuospatial attention. Cereb. Cortex 18, 114–125. doi: 10.1093/cercor/bhm036
Kerstin, K., Susanne, N., Thiel, C. M., Karsten, S., Charlotte, H., Jin, F., et al. (2005). Development of attentional networks: an fmri study with children and adults. Neuroimage 28, 429–439. doi: 10.1016/j.neuroimage.2005.06.065
*Kesler, S. R., Haberecht, M. F., Menon, V., Warsofsky, I. S., Dyerfriedman, J., Neely, E. K., et al. (2004). Functional neuroanatomy of spatial orientation processing in turner syndrome. Cereb. Cortex 14, 174–180. doi: 10.1093/cercor/bhg116
Kodama, K., Terao, T., Hatano, K., Kohno, K., Makino, M., Mizokami, Y., et al. (2015). Identification of the neural correlates of cyclothymic temperament using a working memory task in fmri. J. Affect. Disord. 171, 1–5. doi: 10.1016/j.jad.2014.09.009
Konen, C. S., Kleiser, R., Seitz, R. J., and Bremmer, F. (2005). An fmri study of optokinetic nystagmus and smooth-pursuit eye movements in humans. Exp. Brain Res. 165, 203–216. doi: 10.1007/s00221-005-2289-7
Kozhevnikov, M., Motes, M. A., Rasch, B., and Blajenkova, O. (2006). Perspective-taking vs. mental rotation transformations and how they predict spatial navigation performance. Appl. Cogn. Psychol. 20, 397–417. doi: 10.1002/acp.1192
*Kucian, K., Loenneker, T. H., Dietrich, T. H., and Martin, E. (2005). Gender differences in brain activation patterns during mental rotation and number related cognitive tasks. Psychol. Sci. 4, 112–131.
*Kucian, K., Von, A. M., Loenneker, T., Dietrich, T., Mast, F. W., and Martin, E. (2006). Brain activation during mental rotation in school children and adults. J. Neural. Transm. 114:675. doi: 10.1007/s00702-006-0604-5
Kühn, S., and Gallinat, J. (2014). Segregating cognitive functions within hippocampal formation: a quantitative meta-analysis on spatial navigation and episodic memory. Hum. Brain Mapp. 35, 1129–1142. doi: 10.1002/hbm.22239
*Lambrey, S., Doeller, C., Berthoz, A., and Burgess, N. (2012). Imagining being somewhere else: neural basis of changing perspective in space. Cereb. Cortex 22, 166–174. doi: 10.1093/cercor/bhr101
*Lamm, C., Windischberger, C., Leodolter, U., Moser, E., and Bauer, H. (2001). Evidence for premotor cortex activity during dynamic visuospatial imagery from single-trial functional magnetic resonance imaging and event-related slow cortical potentials. Neuroimage 14, 268–283. doi: 10.1006/nimg.2001.0850
*Lamm, C., Windischberger, C., Moser, E., and Bauer, H. (2007). The functional role of dorso-lateral premotor cortex during mental rotation: an event-related fmri study separating cognitive processing steps using a novel task paradigm. Neuroimage 36, 1374–1386. doi: 10.1016/j.neuroimage.2007.04.012
Langel, J., Hakun, J., Zhu, D. C., and Ravizza, S. M. (2014). Functional specialization of the left ventral parietal cortex in working memory. Front. Hum. Neurosci. 8:440. doi: 10.3389/fnhum.2014.00440
*Latini-Corazzini, L., Nesa, M. P., Ceccaldi, M., Guedj, E., Thinus-Blanc, C., Cauda, F., et al. (2010). Route and survey processing of topographical memory during navigation. Psychol. Res. 74, 545–559. doi: 10.1007/s00426-010-0276-5
Lavrysen, A., Heremans, E., Peeters, R., Wenderoth, N., Feys, P., Swinnen, S. P., et al. (2012). Hemispheric asymmetries in goal-directed hand movements are independent of hand preference. Neuroimage 62, 1815–1824. doi: 10.1016/j.neuroimage.2012.05.033
Lawton, C. A. (1994). Gender differences in way-finding strategies: relationship to spatial ability and spatial anxiety. Sex Roles 30, 765–779.
Lawton, C. A., and Kallai, J. (2002). Gender differences in wayfinding strategies and anxiety about wayfinding: a cross-cultural comparison. Sex Roles 47, 389–401. doi: 10.1023/A:1021668724970
*Ledoux, A. A., Phillips, J. L., Labelle, A., Smith, A., Bohbot, V. D., and Boyer, P. (2013). Decreased fmri activity in the hippocampus of patients with schizophrenia compared to healthy control participants, tested on a wayfinding task in a virtual town. Psychiatr. Res. Neuroimag. 211, 47–56. doi: 10.1016/j.pscychresns.2012.10.005
*Lee, C., Liu, A., Ng, Y., Mak, A., Gao, J., and Lee, T. (2005). The cerebellum's involvement in the judgement of spatial orientation. Neuropsychologia 43, 1870–1877. doi: 10.1016/j.neuropsychologia.2005.03.025
*Levin, S. L., Mohamed, F. B., and Platek, S. M. (2005). Common ground for spatial cognition? a behavioral and fmri study of sex differences in mental rotation and spatial working memory. Evol. Psychol. 3, 227–254. doi: 10.1177/147470490500300116
Li, C., Chen, K., Han, H., Chui, D., and Wu, J. (2012). An fmri study of the neural systems involved in visually cued auditory top-down spatial and temporal attention. Neurosci. Biomed. Eng. 2:e49948. doi: 10.1371/journal.pone.0049948
Li, Y., and O'Boyle, M. W. (2011). Differences in mental rotation strategies for native speakers of chinese and english and how they vary as a function of sex and college major. Psychol. Record 61, 2–19. doi: 10.1007/BF03395743
Linn, M. C., and Petersen, A. C. (1985). Emergence and characterization of sex differences in spatial ability: a meta-analysis. Child Dev. 56, 1479–1498. doi: 10.2307/1130467
Lisman, J., Buzs, Ã K., Eichenbaum, H., Nadel, L., Ranganath, C., and Redish, A. D. (2017). Publisher correction: viewpoints: how the hippocampus contributes to memory, navigation and cognition. Nat. Neurosci. 20:14341018. doi: 10.1038/s41593-017-0034-8
Lissek, S., Vallana, G. S., Schlaffke, L., Lenz, M., Dinse, H. R., and Tegenthoff, M. (2014). Opposing effects of dopamine antagonism in a motor sequence task-tiapride increases cortical excitability and impairs motor learning. Front. Behav. Neurosci. 8:201. doi: 10.3389/fnbeh.2014.00201
*Logie, R. H., Pernet, C. R., Buonocore, A., and Della, S. S. (2011). Low and high imagers activate networks differentially in mental rotation. Neuropsychologia 49, 3071–3077. doi: 10.1016/j.neuropsychologia.2011.07.011
*Lux, S., Marshall, J. C., Ritzl, A., Zilles, K., and Fink, G. R. (2003). Neural mechanisms associated with attention to temporal synchrony versus spatial orientation: an fmri study. Neuroimage 20, 58–65. doi: 10.1016/j.neuroimage.2003.09.009
Ma, L., Steinberg, J. L., Hasan, K. M., Narayana, P. A., Kramer, L. A., and Moeller, F. G. (2012). Working memory load modulation of parieto-frontal connections: evidence from dynamic causal modeling. Hum. Brain Mapp. 33, 1850–1867. doi: 10.1002/hbm.21329
Maguire, E. A., and Dhassabis, K. (2010). Autobiographical memory in semantic dementia: a longitudinal fmri study. Neuropsychologia 48, 123–136. doi: 10.1016/j.neuropsychologia.2009.08.020
*Maguire, E. A., Frackowiak, R. S. J., and Frith, C. D. (1997). Recalling routes around london: activation of the right hippocampus in taxi drivers. J. Neurosci. 17, 7103–7110. doi: 10.1523/JNEUROSCI.17-18-07103.1997
Majerus, S., Attout, L., D'Argembeau, A., Degueldre, C., Fias, W., Maquet, P., et al. (2012). Attention supports verbal short-term memory via competition between dorsal and ventral attention networks. Cereb. Cortex 22, 1086–1097. doi: 10.1093/cercor/bhr174
Malinowski, J. C. (2001). Mental rotation and real-world wayfinding. Percept. Mot. Skills 92, 19–30. doi: 10.2466/pms.2001.92.1.19
Malinowski, J. C., and Gillespie, W. T. (2001). Individual differences in performance on a large-scale, real-world wayfinding task. J. Environ. Psychol. 21, 73–82. doi: 10.1006/jevp.2000.0183
*Marsh, R., Hao, X., Xu, D., Wang, Z., Duan, Y., Liu, J., et al. (2010). A virtual reality-based fmri study of reward-based spatial learning. Neuropsychologia 48, 2912–2921. doi: 10.1016/j.neuropsychologia.2010.05.033
*Mazzarella, E., Ramsey, R., Conson, M., and Hamilton, A. (2013). Brain systems for visual perspective taking and action perception. Soc. Neurosci. 8, 248–267. doi: 10.1080/17470919.2012.761160
Mellet, E., Laou, L., Petit, L., Zago, L., Mazoyer, B., and Tzourio-Mazoyer, N. (2010). Impact of the virtual reality on the neural representation of an environment. Hum. Brain Mapp. 31, 1065–1075. doi: 10.1002/hbm.20917
*Milivojevic, B., Hamm, J. P., and Corballis, M. C. (2008). Functional neuroanatomy of mental rotation. J. Cogn. Neurosci. 21, 945–959. doi: 10.1162/jocn.2009.21085
Mitchell, R. L. (2007). Fmri delineation of working memory for emotional prosody in the brain: commonalities with the lexico-semantic emotion network. Neuroimage 36, 1015–1025. doi: 10.1016/j.neuroimage.2007.03.016
*Moffat, S. D., Elkins, W., and Resnick, S. M. (2006). Age differences in the neural systems supporting human allocentric spatial navigation. Neurobiol. Aging 27, 965–972. doi: 10.1016/j.neurobiolaging.2005.05.011
Moser, E. I., Moser, M. B., and Mcnaughton, B. L. (2017). Spatial representation in the hippocampal formation: a history. Nat. Neurosci. 20, 1448–1464. doi: 10.1038/nn.4653
Mullally, S. L., Hassabis, D., and Maguire, E. A. (2012). Scene construction in amnesia: an fmri study. J. Soc. Neurosci. 32, 5646–5653. doi: 10.1523/JNEUROSCI.5522-11.2012
Müller, K., Kleiser, R., Mechsner, F., and Seitz, R. J. (2011). Involvement of area mt in bimanual finger movements in left-handers: an fmri study. Eur. J. Neurosci. 34, 1301–1309. doi: 10.1111/j.1460-9568.2011.07850.x
Nemmi, F., Piras, F., Péran, P., Incoccia, C., Sabatini, U., and Guariglia, C. (2013). Landmark sequencing and route knowledge: an fmri study. Cortex 49, 507–519. doi: 10.1016/j.cortex.2011.11.016
*Newman, S. D., Hansen, M. T., and Gutierrez, A. (2016). An fmri study of the impact of block building and board games on spatial ability. Front. Psychol. 7:1278. doi: 10.3389/fpsyg.2016.01278
*Ng, V. W., Bullmore, E. T., de Zubicaray, G. I., Cooper, A., Suckling, J., and Williams, S. C. (2001). Identifying rate-limiting nodes in large-scale cortical networks for visuospatial processing: an illustration using fmri. J. Cogn. Neurosci. 13, 537–545. doi: 10.1162/08989290152001943
Nieuwland, M. S. (2012). Establishing propositional truth-value in counterfactual and real-world contexts during sentence comprehension: differential sensitivity of the left and right inferior frontal gyri. Neuroimage 59, 3433–3440. doi: 10.1016/j.neuroimage.2011.11.018
Nilsson, J., Lebedev, A. V., Rydström, A., and Lövdén, M. (2017). Direct-current stimulation does little to improve the outcome of working memory training in older adults. Psychol. Sci. 28, 907–920. doi: 10.1177/0956797617698139
*O'Boyle, M. W., Cunnington, R., Silk, T. J., Vaughan, D., Jackson, G., Syngeniotis, A., et al. (2005). Mathematically gifted male adolescents activate a unique brain network during mental rotation. Cogn. Brain Res. 25, 583–587. doi: 10.1016/j.cogbrainres.2005.08.004
*Ohnishi, T., Matsuda, H., Hirakata, M., and Ugawa, Y. (2006). Navigation ability dependent neural activation in the human brain: an fmri study. Neurosci. Res. 55, 361–369. doi: 10.1016/j.neures.2006.04.009
Opitz, B., Schneiders, J. A., Krick, C. M., and Mecklinger, A. (2014). Selective transfer of visual working memory training on chinese character learning. Neuropsychologia 53, 1–11. doi: 10.1016/j.neuropsychologia.2013.10.017
*Orban, P., Rauchs, G., Balteau, E., Degueldre, C., Luxen, A., Maquet, P., et al. (2006). Sleep after spatial learning promotes covert reorganization of brain activity. Proc. Natl. Acad. Sci. U.S.A. 103, 7124–7129. doi: 10.1073/pnas.0510198103
*Papeo, L., Rumiati, R. I., Cecchetto, C., and Tomasino, B. (2012). On-line changing of thinking about words: the effect of cognitive context on neural responses to verb reading. J. Cogn. Neurosci. 24, 2348–2362. doi: 10.1162/jocn_a_00291
*Paschke, K., Jordan, K., and Wüstenberg, T. (2012). Mirrored or identical–is the role of visual perception underestimated in the mental rotation process of 3d-objects? a combined fmri-eye tracking-study. Neuropsychologia 50, 1844–1851. doi: 10.1016/j.neuropsychologia.2012.04.010
Peña, D., Contreras, M. J., Shih, P. C., and Santacreu, J. (2008). Solution strategies as possible explanations of individual and sex differences in a dynamic spatial task.Solution strategies as possible explanations of individual and sex differences in a dynamic spatial task. Acta Psychol. 128, 1–14. doi: 10.1016/j.actpsy.2007.09.005
*Pine, D. S., Grun, J., Maguire, E. A., Burgess, N., Zarahn, E., Koda, V., et al. (2002). Neurodevelopmental aspects of spatial navigation: a virtual reality fmri study. Neuroimage 15, 396–406. doi: 10.1006/nimg.2001.0988
*Pintzka, C. W., Evensmoen, H. R., Lehn, H., and Håberg, A. K. (2016). Changes in spatial cognition and brain activity after a single dose of testosterone in healthy women. Behav. Brain Res. 298(Pt B), 78–90. doi: 10.1016/j.bbr.2015.10.056
*Podzebenko, K., Egan, G. F., and Watson, J. D. G. (2002). Widespread dorsal stream activation during a parametric mental rotation task, revealed with functional magnetic resonance imaging. Neuroimage 15, 547–558. doi: 10.1006/nimg.2001.0999
*Powell, J. L., Kemp, G. J., and Garcíafinaña, M. (2012). Association between language and spatial laterality and cognitive ability: an fmri study. Neuroimage 59, 1818–1829. doi: 10.1016/j.neuroimage.2011.08.040
*Prescott, J., Gavrilescu, M., Cunnington, R., O'Boyle, M. W., and Egan, G. F. (2010). Enhanced brain connectivity in math-gifted adolescents: an fmri study using mental rotation. Cogn. Neurosci. 1, 277–288. doi: 10.1080/17588928.2010.506951
Price, C. J., and Devlin, J. T. (2003). The myth of the visual word form area. Neuroimage 19, 473–481. doi: 10.1016/S1053-8119(03)00084-3
*Rauchs, G., Orban, P., Balteau, E., Schmidt, C., Degueldre, C., Luxen, A., et al. (2008). Partially segregated neural networks for spatial and contextual memory in virtual navigation. Hippocampus 18, 503–518. doi: 10.1002/hipo.20411
Reggev, N., Zuckerman, M., and Maril, A. (2011). Are all judgments created equal? an fmri study of semantic and episodic metamemory predictions. Neuropsychologia 49, 1332–1342. doi: 10.1016/j.neuropsychologia.2011.01.013
Rémy, F. (2014). Incongruent object/context relationship in visual scenes: where are they processed in the brain? Brain Cogn. 84, 34–43. doi: 10.1016/j.bandc.2013.10.008
*Rodriguez, P. F. (2010). Human navigation that requires calculating heading vectors recruits parietal cortex in a virtual and visually sparse water maze task in fmri. Behav. Neurosci. 124, 532–540. doi: 10.1037/a0020231
*Rosenbaum, R. S., Winocur, G., Grady, C. L., Ziegler, M., and Moscovitch, M. (2007). Memory for familiar environments learned in the remote past: fmri studies of healthy people and an amnesic person with extensive bilateral hippocampal lesions. Hippocampus 17, 1241–1251. doi: 10.1002/hipo.20354
*Rosenbaum, R. S., Ziegler, M., Winocur, G., Grady, C. L., and Moscovitch, M. (2004). “I have often walked down this street before”: fmri studies on the hippocampus and other structures during mental navigation of an old environment. Hippocampus 14, 826–835. doi: 10.1002/hipo.10218
*Schendan, H. E., and Stern, C. E. (2007). Mental rotation and object categorization share a common network of prefrontal and dorsal and ventral regions of posterior cortex. Neuroimage 35, 1264–1277. doi: 10.1016/j.neuroimage.2007.01.012
*Schinazi, V. R., and Epstein, R. A. (2010). Neural correlates of real-world route learning. Neuroimage 53, 725–735. doi: 10.1016/j.neuroimage.2010.06.065
*Schöning, S., Engelien, A., Kugel, H., Schäfer, S., Schiffbauer, H., Zwitserlood, P., et al. (2007). Functional anatomy of visuo-spatial working memory during mental rotation is influenced by sex, menstrual cycle, and sex steroid hormones. Neuropsychologia 45, 3203–3214. doi: 10.1016/j.neuropsychologia.2007.06.011
Schubotz, R. I., and von Cramon, D. Y. (2009). The case of pretense: observing actions and inferring goals. Cogn. Neurosci. 21, 642–653. doi: 10.1162/jocn.2009.21049
Schwabe, L., Oitzl, M. S., Philippsen, C., Richter, S., Bohringer, A., and Wippich, W. (2007). Stress modulates the use of spatial versus stimulus-response learning strategies in humans. Learn. Memory 14, 109–116. doi: 10.1101/lm.435807
Schwabe, L., Oitzl, M. S., Richter, S., and SchChinger, H. (2009). Modulation of spatial and stimulus–response learning strategies by exogenous cortisol in healthy young women. Psychoneuroendocrinology 34, 358–366. doi: 10.1016/j.psyneuen.2008.09.018
Schweisfurth, M. A., Schweizer, R., and Frahm, J. (2011). Functional mri indicates consistent intra-digit topographic maps in the little but not the index finger within the human primary somatosensory cortex. Neuroimage 56, 2138–2143. doi: 10.1016/j.neuroimage.2011.03.038
Seo, J., Kim, Y. T., Song, H. J., Lee, H. J., Lee, J., Jung, T. D., et al. (2012). Stronger activation and deactivation in archery experts for differential cognitive strategy in visuospatial working memory processing. Behav. Brain Res. 229, 185–193. doi: 10.1016/j.bbr.2012.01.019
*Seurinck, R., de Lange, F. P., Achten, E., and Vingerhoets, G. (2011). Mental rotation meets the motion aftereffect: the role of hv5/mt+ in visual mental imagery. J. Cogn. Neurosci. 23, 1395–1404. doi: 10.1162/jocn.2010.21525
*Seurinck, R., Vingerhoets, G., de Lange, F. P., and Achten, E. (2004). Does egocentric mental rotation elicit sex differences? Neuroimage 23, 1440–1449. doi: 10.1016/j.neuroimage.2004.08.010
*Seurinck, R., Vingerhoets, G., Vandemaele, P., Deblaere, K., and Achten, E. (2005). Trial pacing in mental rotation tasks. Neuroimage 25, 1187–1196. doi: 10.1016/j.neuroimage.2005.01.010
*Shelton, A. L., and Gabrieli, J. D. (2002). Neural correlates of encoding space from route and survey perspectives. J. Neurosci. Off. 22, 2711–2717. doi: 10.1523/JNEUROSCI.22-07-02711.2002
Slotnick, S. D., and Moo, L. R. (2006). Prefrontal cortex hemispheric specialization for categorical and coordinate visual spatial memory. Neuropsychologia 44, 1560–1568. doi: 10.1016/j.neuropsychologia.2006.01.018
*Sluming, V., Brooks, J., Howard, M., Downes, J. J., and Roberts, N. (2007). Broca's area supports enhanced visuospatial cognition in orchestral musicians. J. Neurosci. 27, 3799–3806. doi: 10.1523/JNEUROSCI.0147-07.2007
Soulières, I., Dawson, M., Samson, F., Barbeau, E. B., Sahyoun, C., Strangman, G. E., et al. (2009). Enhanced visual processing contributes to matrix reasoning in autism. Hum. Brain Mapp. 30, 4082–4107. doi: 10.1002/hbm.20831
Stefanics, G., and Czigler, I. (2012). Automatic prediction error responses to hands with unexpected laterality: an electrophysiological study. Neuroimage 63, 253–261. doi: 10.1016/j.neuroimage.2012.06.068
*Stoodley, C. J., Valera, E. M., and Schmahmann, J. D. (2012). Functional topography of the cerebellum for motor and cognitive tasks: an fmri study. Neuroimage 59, 1560–1570. doi: 10.1016/j.neuroimage.2011.08.065
*Suchan, B., Botko, R., Gizewski, E., Forsting, M., and Daum, I. (2006). Neural substrates of manipulation in visuospatial working memory. Neuroscience 139, 351–357. doi: 10.1016/j.neuroscience.2005.08.020
*Suchan, B., Yaguez, L. G., Canavan, A. G., Herzog, H., Tellmann, L., Homberg, V., et al. (2002). Hemispheric dissociation of visual-pattern processing and visual rotation. Behav. Brain Res. 136, 533–544. doi: 10.1016/S0166-4328(02)00204-8
Sulpizio, V., Committeri, G., and Galati, G. (2014). Distributed cognitive maps reflecting real distances between places and views in the human brain. Front. Hum. Neurosci. 8:716. doi: 10.3389/fnhum.2014.00716
Suzuki, C., Tsukiura, T., Mochizuki-Kawai, H., Shigemune, Y., and Iijima, T. (2009). Prefrontal and medial temporal contributions to episodic memory-based reasoning. Neurosci. Res. 63, 177–183. doi: 10.1016/j.neures.2008.11.010
Suzuki, M., Tsukiura, T., Matsue, Y., Yamadori, A., and Fujii, T. (2005). Dissociable brain activations during the retrieval of different kinds of spatial context memory. Neuroimage 25, 993–1001. doi: 10.1016/j.neuroimage.2004.12.021
Telzer, E. H., Qu, Y., Goldenberg, D., Fuligni, A. J., Galván, A., and Lieberman, M. D. (2014). Adolescents' emotional competence is associated with parents' neural sensitivity to emotions. Front. Hum. Neurosci. 8:558. doi: 10.3389/fnhum.2014.00558
Thakral, P. P., and Slotnick, S. D. (2009). The role of parietal cortex during sustained visual spatial attention. Brain Res. 1302, 157–166. doi: 10.1016/j.brainres.2009.09.031
Thompson, J. C., and Baccus, W. (2012). Form and motion make independent contributions to the response to biological motion in occipitotemporal cortex. Neuroimage 59, 625–634. doi: 10.1016/j.neuroimage.2011.07.051
*Thomsen, T., Hugdahl, K., Ersland, L., Barndon, R., Lundervold, A., Smievoll, A. I., et al. (2000). Functional magnetic resonance imaging (fmri) study of sex differences in a mental rotation task. Med. Sci. Monit. 6, 1186–1196.
Tomasino, B., and Gremese, M. (2016). Effects of stimulus type and strategy on mental rotation network: an activation likelihood estimation meta-analysis. Front. Hum. Neurosci. 9(Pt 1):693. doi: 10.3389/fnhum.2015.00693
Touroutoglou, A., Lindquist, A. K., Dickerson, C. B., and Barrett, F. L. (2015). Intrinsic connectivity in the human brain does not reveal networks for 'basic' emotions. Soc. Cogn. Affect. Neurosci. 10, 1257–1265. doi: 10.1093/scan/nsv013
Turgut, M. (2015). Development of the spatial ability self-report scale (sasrs): reliability and validity studies. Qual. Quant. 49, 1997–2014. doi: 10.1007/s11135-014-0086-8
Vandermeeren, Y., Sébire, G., Grandin, C. B., Thonnard, J. L., Schlögel, X., and Volder, A. G. D. (2003). Functional reorganization of brain in children affected with congenital hemiplegia: fmri study. Neuroimage 20, 289–301. doi: 10.1016/S1053-8119(03)00262-3
*Vanrie, J., Béatse, E., Wagemans, J., Sunaert, S., and Van Hecke, P. (2002). Mental rotation versus invariant features in object perception from different viewpoints: an fmri study. Neuropsychologia 40, 917–930. doi: 10.1016/S0028-3932(01)00161-0
*Vingerhoets, G., De, F. L., Vandemaele, P., Deblaere, K., and Achten, E. (2002). Motor imagery in mental rotation: an fmri study. Neuroimage 17, 1623–1633. doi: 10.1006/nimg.2002.1290
*Vingerhoets, G., Santens, P., Van, L. K., Lahorte, P., Dierckx, R. A., and De, R. J. (2001). Regional brain activity during different paradigms of mental rotation in healthy volunteers: a positron emission tomography study. Neuroimage 13, 381–391. doi: 10.1006/nimg.2000.0690
Wagner, G., Koch, K., Reichenbach, J. R., Sauer, H., and Schlösser, R. G. (2006). The special involvement of the rostrolateral prefrontal cortex in planning abilities: an event-related fmri study with the tower of london paradigm. Neuropsychologia 44, 2337–2347. doi: 10.1016/j.neuropsychologia.2006.05.014
Wang, L., and Carr, M. (2014). Working memory and strategy use contribute to gender differences in spatial ability. Educ. Psychol. 49, 261–282. doi: 10.1080/00461520.2014.960568
Wang, L., Cohen, A. S., and Carr, M. (2014). Spatial ability at two scales of representation: a meta-analysis. Learn. Individ. Differ. 36, 140–144. doi: 10.1016/j.lindif.2014.10.006
Wardle, M. C., Fitzgerald, D. A., Angstadt, M., Rabinak, C. A., De, W. H., and Phan, K. L. (2014). Effects of oxycodone on brain responses to emotional images. Psychopharmacology 231, 4403–4415. doi: 10.1007/s00213-014-3592-4
*Weiss, M. M., Wolbers, T. M., Witt, K., Marshall, L., Buchel, C., and Siebner, H. (2009). Rotated alphanumeric characters do not automatically activate frontoparietal areas subserving mental rotation. Neuroimage 44, 1063–1073. doi: 10.1016/j.neuroimage.2008.09.042
Weniger, G., Ruhleder, M., Wolf, S., Lange, C., and Irle, E. (2009). Egocentric memory impaired and allocentric memory intact as assessed by virtual reality in subjects with unilateral parietal cortex lesions. Neuropsychologia 47, 59–69. doi: 10.1016/j.neuropsychologia.2008.08.018
Weniger, G., Siemerkus, J., Barke, A., Lange, C., Ruhleder, M., Sachsse, U., et al. (2013). Egocentric virtual maze learning in adult survivors of childhood abuse with dissociative disorders: evidence from functional magnetic resonance imaging. Psychiatr. Res. Neuroimag. 212, 116–124. doi: 10.1016/j.pscychresns.2012.11.004
*Weniger, G., Siemerkus, J., Schmidtsamoa, C., Mehlitz, M., Baudewig, J., Dechent, P., et al. (2010). The human parahippocampal cortex subserves egocentric spatial learning during navigation in a virtual maze. Neurobiol. Learn. Mem. 93, 46–55. doi: 10.1016/j.nlm.2009.08.003
Whalley, M. G., Rugg, M. D., and Brewin, C. R. (2012). Autobiographical memory in depression: an fmri study. Psychiatr. Res. Neuroimag. 201, 98–106. doi: 10.1016/j.pscychresns.2011.08.008
*Whittingstall, K., Bernier, M., Houde, J. C., Fortin, D., and Descoteaux, M. (2014). Structural network underlying visuospatial imagery in humans. Cortex 56, 85–98. doi: 10.1016/j.cortex.2013.02.004
Wilbers, L., Deuker, L., Fell, J., and Axmacher, N. (2012). Are autobiographical memories inherently social? evidence from an fmri study. PLoS ONE 7:e45089. doi: 10.1371/journal.pone.0045089
*Wilson, K. D., and Farah, M. J. (2006). Distinct patterns of viewpoint-dependent bold activity during common-object recognition and mental rotation. Perception 35, 1351–1366. doi: 10.1068/p5571
Winlove, C. I. P., Milton, F., Ranson, J., Fulford, J., Mackisack, M., Macpherson, F., et al. (2018). The neural correlates of visual imagery: a co-ordinate-based meta-analysis. Cortex 105, 4–25. doi: 10.1016/j.cortex.2017.12.014
*Wolbers, T., and Büchel, C. (2005). Dissociable retrosplenial and hippocampal contributions to successful formation of survey representations. J. Neurosci. 25, 3333–3340. doi: 10.1523/JNEUROSCI.4705-04.2005
*Wolbers, T., Schoell, E. D., and Büchel, C. (2006). The predictive value of white matter organization in posterior parietal cortex for spatial visualization ability. Neuroimage 32, 1450–1455. doi: 10.1016/j.neuroimage.2006.05.011
*Wolbers, T., Weiller, C., and Büchel, C. (2003). Contralateral coding of imagined body parts in the superior parietal lobe. Cereb. Cortex 13, 392–399. doi: 10.1093/cercor/13.4.392
*Wolbers, T., Wiener, J. M., Mallot, H. A., and Büchel, C. (2007). Differential recruitment of the hippocampus, medial prefrontal cortex, and the human motion complex during path integration in humans. J. Neurosci. 27, 9408–9416. doi: 10.1523/JNEUROSCI.2146-07.2007
*Wraga, M., Boyle, H. K., and Flynn, C. M. (2010). Role of motor processes in extrinsically encoding mental transformations. Brain Cogn. 74, 193–202. doi: 10.1016/j.bandc.2010.07.005
*Wraga, M., Shephard, J. M., Church, J. A., Inati, S., and Kosslyn, S. M. (2005). Imagined rotations of self versus objects: an fmri study. Neuropsychologia 43, 1351–1361. doi: 10.1016/j.neuropsychologia.2004.11.028
Wu, C. T., Weissman, D. H., Roberts, K. C., and Woldorff, M. G. (2007). The neural circuitry underlying the executive control of auditory spatial attention. Brain Res. 1134, 187–198. doi: 10.1016/j.brainres.2006.11.088
*Xu, J., Evensmoen, H. R., Lehn, H., Pintzka, C. W., and Håberg, A. K. (2010). Persistent posterior and transient anterior medial temporal lobe activity during navigation. Neuroimage 52, 1654–1666. doi: 10.1016/j.neuroimage.2010.05.074
Yang, J. C., and Chen, S. Y. (2010). Effects of gender differences and spatial abilities within a digital pentominoes game. Comput. Educ. 55, 1220–1233. doi: 10.1016/j.compedu.2010.05.019
Yilmaz, H. B. (2009). On the development and measurement of spatial ability. Int. Electr. J. Element. Educ. 1, 83–96. Available online at: https://www.iejee.com/index.php/IEJEE/article/download/279/302
Zacks, J. M., Mires, J., Tversky, B., and Hazeltine, E. (2001). Mental spatial transformations of objects and perspective. Spat. Cogn. Comput. 2, 315–332. doi: 10.1023/A:1015584100204
*Zacks, J. M., Ollinger, J. M, Sheridan, M. A., and Tversky, B. (2002). A parametric study of mental spatial transformations of bodies. Neuroimage 16, 857–872. doi: 10.1006/nimg.2002.1129
Keywords: large-scale spatial ability, small-scale spatial ability, activation likelihood estimation, meta-analysis, behavioral model
Citation: Li Y, Kong F, Ji M, Luo Y, Lan J and You X (2019) Shared and Distinct Neural Bases of Large- and Small-Scale Spatial Ability: A Coordinate-Based Activation Likelihood Estimation Meta-Analysis. Front. Neurosci. 12:1021. doi: 10.3389/fnins.2018.01021
Received: 04 September 2018; Accepted: 18 December 2018;
Published: 10 January 2019.
Edited by:
Rufin VanRullen, Center for the National Scientific Research (CNRS), FranceReviewed by:
Xiang-Zhen Kong, Max-Planck-Institut für Psycholinguistik, NetherlandsCopyright © 2019 Li, Kong, Ji, Luo, Lan and You. This is an open-access article distributed under the terms of the Creative Commons Attribution License (CC BY). The use, distribution or reproduction in other forums is permitted, provided the original author(s) and the copyright owner(s) are credited and that the original publication in this journal is cited, in accordance with accepted academic practice. No use, distribution or reproduction is permitted which does not comply with these terms.
*Correspondence: Yuan Li, cHN5bGl5dWFuQDE2My5jb20=
Xuqun You, eW91eHVxdW4xOTYzQDE2My5jb20=
Disclaimer: All claims expressed in this article are solely those of the authors and do not necessarily represent those of their affiliated organizations, or those of the publisher, the editors and the reviewers. Any product that may be evaluated in this article or claim that may be made by its manufacturer is not guaranteed or endorsed by the publisher.
Research integrity at Frontiers
Learn more about the work of our research integrity team to safeguard the quality of each article we publish.