Determining the Nature of Prefrontal Cortex Recruitment After Traumatic Brain Injury: A Response to Turner
- Department of Neurosurgery, Yi-Ji Shan Hospital of Wannan Medical College, Wuhu, China
Traumatic brain injury (TBI) is a primary cause of disability and mortality. Ghrelin, a gastrointestinal hormone, has been found to have protective effects for the brain, but the molecular mechanism of these neuroprotective effects of ghrelin remains unclear. In this study, an electronic cortical contusion impactor was used to establish a rat TBI model and we investigated the effect of ghrelin on brain repair by neurological severity score and histological examination. An antibody array was employed to uncover the molecular mechanism of ghrelin’s neuroprotective effects by determining the alterations of multiple proteins in the brain cortex. As a result, ghrelin attenuated brain injury and promoted brain functional recovery. After TBI, 13 proteins were up-regulated in the brain cortex, while basic fibroblast growth factor (bFGF) and fibroblast growth factor-binding protein (FGF-BP) were down-regulated after ghrelin treatment. It is known that bFGF can induce angiogenesis in the brain and accelerate wound healing, which can be further enhanced by FGF-BP. Based on the previous studies, it is hypothesized that the exogenous ghrelin curing TBI might cause the closure of bFGF and FGF-BP functions on wound healing, or ghrelin might exert the neuroprotective effects by competitively inhibiting bFGF/FGF-BP-induced neovascularization. Whether the combinational administration of ghrelin and bFGF/FGF-BP can enhance or weaken the therapeutic effect on TBI requires further research.
Introduction
Traumatic brain injury (TBI) is a major public health concern and carries a high morbidity and mortality (Aarabi and Simard, 2009). Globally, approximately 10 million TBI cases are reported each year. Patients with TBI typically show various symptoms including headaches, dizziness, imbalance, vertigo, fatigue, changes in sleep pattern, neuropsychiatric symptoms, cognitive impairments, and even death (McCauley et al., 2005). Although advances in research and improved neurological intensive care have been achieved in recent years, the clinical outcome of patients with severe TBI remains poor, emphasizing the need for further research and improvement in care.
Ghrelin, a 28-amino acid peptide secreted mainly in the stomach, is a neuroendocrine hormone and an endogenous ligand for growth hormone secretagogue receptor (GHS-R) (Kojima et al., 1999). Additionally, ghrelin is slightly produced in the central nervous system, such as in the arcuate nucleus of the hypothalamus, ependymal layer of the third ventricle, dorsomedial nucleus, paraventricular nucleus, ventromedial nucleus and the ventral tegmental area (Abizaid, 2009). It has been suggested that ghrelin is protective against TBI (Bansal et al., 2010; Wei et al., 2015). Ghrelin-treated mice show significant neuroprotection following TBI including preservation of neurons, inhibition of neuronal apoptosis, and prevention of blood–brain barrier breakdown (Lopez et al., 2011, 2012). However, the molecular mechanism behind the protective role of ghrelin against TBI is unclear.
A protein plays its role in organisms via affecting other proteins, which forms a protein–protein interaction network. In the present study, in order to reveal the molecular mechanism of ghrelin against TBI, we used an advanced antibody array technology that has the advantages of being amenable to high-throughput screening and rapid parallel detection of multiple proteins.
Materials and Methods
Animals
Sprague-Dawley rats weighing 300–350 g were provided by the Chinese People’s Liberation Army Medical Center Experimental Animal Center [SCXK- (Army) -2007-004]. Experimental procedures were approved by the Animal Ethics Committee of the Academy of Military Medical Sciences.
TBI Model and Ghrelin Treatment
Sixty rats were anesthetized with 50% chloral hydrate, vertical incisions were made over the cranium and the periosteums were stripped off. Using a surgical drill, a burr hole, 5 mm in diameter, at the junction 5 mm posterior to the coronal suture and 5 mm to the right of the sagittal suture, was created to expose the dura mater in each rat. Random 30 rats were fixed in the electron cortical contusion impactor (eCCI 6.3, Custom Design and Fabrication, Richmond, VA, United States). A strike was made onto the dura mater at a 3 mm depth and 5 m/s rate. The incisions in all rats were sutured. Among 30 stricken and the other 30 not stricken rats, 15 individuals were randomly chosen from each group to be treated with intravenous ghrelin (Phoenix Pharmaceuticals, Burlingame, CA, United States) at 20 μg/kg dose 30 min after sutured.
Evaluation of Neurological Severity Scale
At 6, 24, 48 and 72 h after TBI, neurological functional deficits of all animals were evaluated according to the Neurological Severity Scale (NSS). The neurological scoring method was used based on the literature (Huang et al., 2005). This NSS includes motor function, sensory function, balance capacity and reflexes (details are included in Table 1). The maximum neurological score was 18 points. A score of 13–18 points denoted severe injury, 7–12 points showed moderate injury, and 1–6 points indicated mild injury. Neurological scores were expressed as mean ± standard deviation (SD).
Histological Examination
At 72 h after TBI, the animals were abdominally anesthetized, and were perfused with cold normal saline. After removal from the cranial cavity, the cortices were isolated from the brain, and were cut into two parts. One part was fixed in 4% paraformaldehyde. The cortex samples were embedded in paraffin, then were cut into 4 μm sections and stained with hematoxylin and eosin (HE). Histology was observed in a blinded manner using a BX53 light microscope (Olympus Corporation, Japan) at 400× magnification and documented with photographs.
Antibody Array Assay
The second part of the cortices was ground to obtain protein extracts for measurement using Rat Cytokine Antibody Arrays (RayBio Rat Cytokine Antibody Array 3 and 4, glass series; RayBio Growth Factor Antibody Array, membrane series. RayBiotech, Norcross, GA, United States), which simultaneously detect 75 cytokines (details in Supplementary Table S1), according to the manufacturer’s instructions. Briefly, protein extracts was diluted to 500 μg/ml with blocking buffer and added to the array pools printed with 75 corresponding anti-cytokine antibodies for overnight incubation. After washing, a biotin-conjugated anti-cytokine mix was incubated with the pools for 2 h. Finally, the glass series arrays were performed with Cy3-conjugated streptavidin, while the membrane series arrays were incubated with HRP-conjugated streptavidin for a further 2 h. After the experimental procedure, the glass slides were scanned to detect the fluorescent signals of microarrays using an InnoScan 300 Microarray Scanner (Innopsys, France). The membrane arrays were exposed after HRP catalyzing chemiluminescent solution using an ImageQuant LAS4000 Scanner (GE Healthcare, Waukesha, WI, United States). The signal values were read and normalized, using an internal positive control, by the RayBiotech analysis tool, which is specifically designed to analyze the data of the Rat Cytokine Antibody Array 3 and 4 and Growth Factor Antibody Array.
ELISA Identification
In order to validate the results from the antibody array, ELISA kits (RayBiotech, Norcross, GA, United States) were used to measure significant cytokines, according to the manufacturer’s instructions. Briefly, protein extracts of cortex samples were incubated in plates coated with capture antibody overnight at 4°C. The plates were washed and a biotin-conjugated detection antibody was added into the plates for incubation (2 h, room temperature) to combine with corresponding proteins. HRP-conjugated streptavidin was added to the plates and allowed to incubate for 45 min. TMB reagent was added and allowed to incubate for 30 min before the reaction was stopped with sulfuric acid. Immediately, the optical density was measured via an ELx800NB microplate reader (BioTek, Winooski, CT, United States) at 450 nm wave length.
Statistical Analysis
All data were statistically analyzed by Student’s t-test using SPSS v.17.0 (SPSS, Inc., Chicago, IL, United States) and are presented as mean ± SD. Differences were considered significant if two-sided p-values were less than 0.05 (p < 0.05). In addition, fold change values were given to indicate the relative expression levels of cytokines. The sample size were calculated basing on the proteins data of antibody array altered in TBI and ghrelin groups from the pilot study using EmpowerStats software with two-tailed test. With a power of 80% and a significance level of 5%, 15 rats per group were needed.
Results
Post-TBI Effect of Ghrelin on the NSS
The effect of ghrelin on improved nerve function was evaluated according to the NSS. As shown in Figure 1, NSS scores were significantly increased after TBI at different time points, indicating the successful establishment of the TBI model. However, ghrelin treatment markedly decreased NSS scores at 24, 48, and 72 h. For example, symptoms including contralateral forelimb flexion, tilting to the contralateral side and other neurobehavioral changes caused by TBI were significantly alleviated by ghrelin treatment.
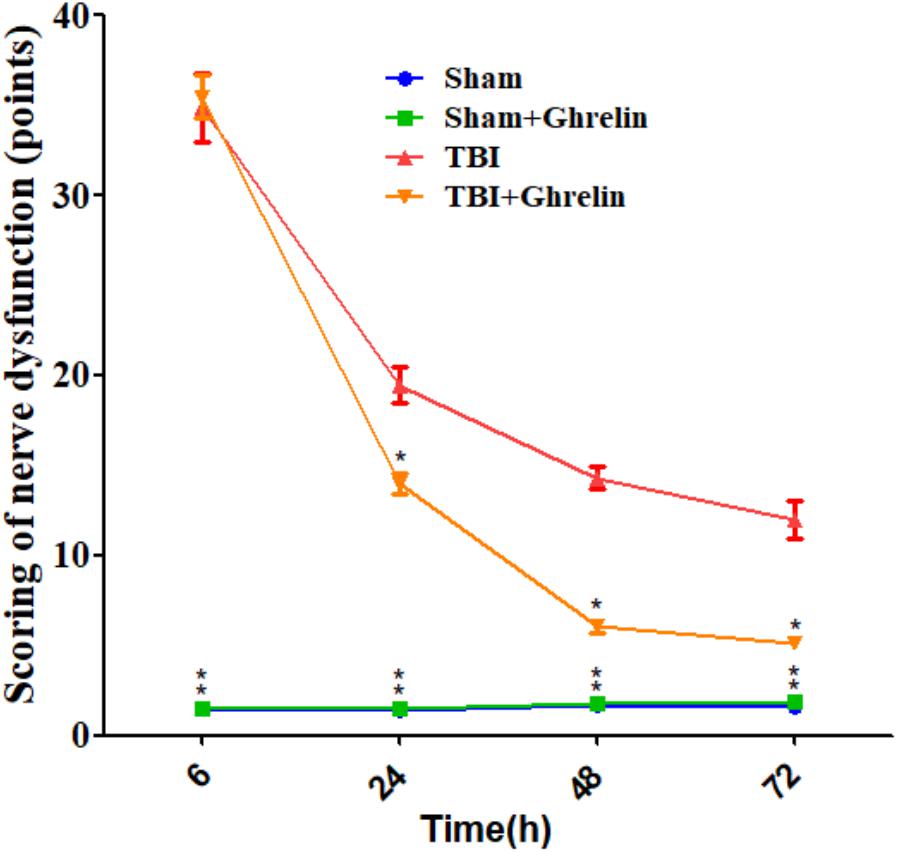
FIGURE 1. The NSS results of nerve dysfunction in rats. The NSS were performed at 6, 24, 48, and 72 h after TBI. Data are presented as means ± SD. ∗p < 0.05 versus TBI group at the same time point. n = 15 in each group.
Effect of Ghrelin on Histological Improvement
Hematoxylin and eosin staining of brain cortices at 72 h after TBI showed extensive edema, neuronal necrosis, karyolysis and vacuolar changes, and widened intercellular gaps. In the ghrelin group, ghrelin treatment obviously reduced damage, including moderating neuronal degeneration, reducing the extent of dark nuclear staining, a lack of shrunken cells, no significant formation of intercellular gaps, and less edema (Figure 2).
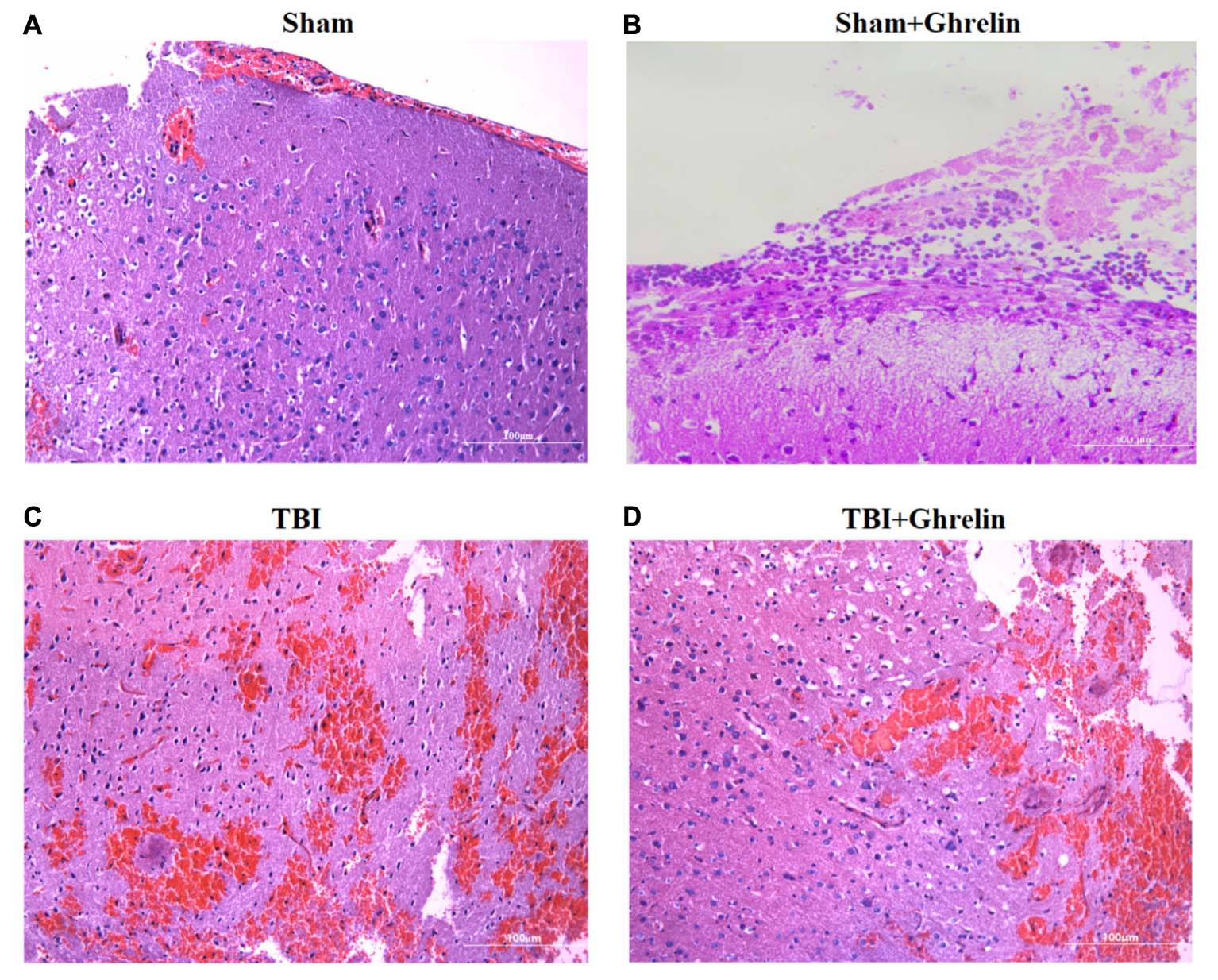
FIGURE 2. Ghrelin-induced morphological improvement of the cortex after TBI. HE staining of brain cortices 72 h after TBI was performed. (A) HE staining (×400) of the sham-operation area of the sham group. (B) HE staining (×400) of the sham-operation area of of the sham + ghrelin group. (C) HE staining (×400) of the injured brain area of the TBI group. (D) HE staining (×400) of the injured brain area of the TBI + ghrelin group.
Altered Cytokine Levels in the TBI Group
Statistical analysis results showed that, compared to sham group, IL-17F, IL-1 ra, RANTES, Galectin-3, ICAM-1, basic fibroblast growth factor (bFGF), HGF, IL-2 Ra, FLT-3 Ligand, CTACK, fibroblast growth factor-binding protein (FGF-BP), bNGF, and Neuropilin-2 were significantly up-regulated in the TBI group (Figure 3, p < 0.05, detailed data in Supplementary Data Sheet S1).
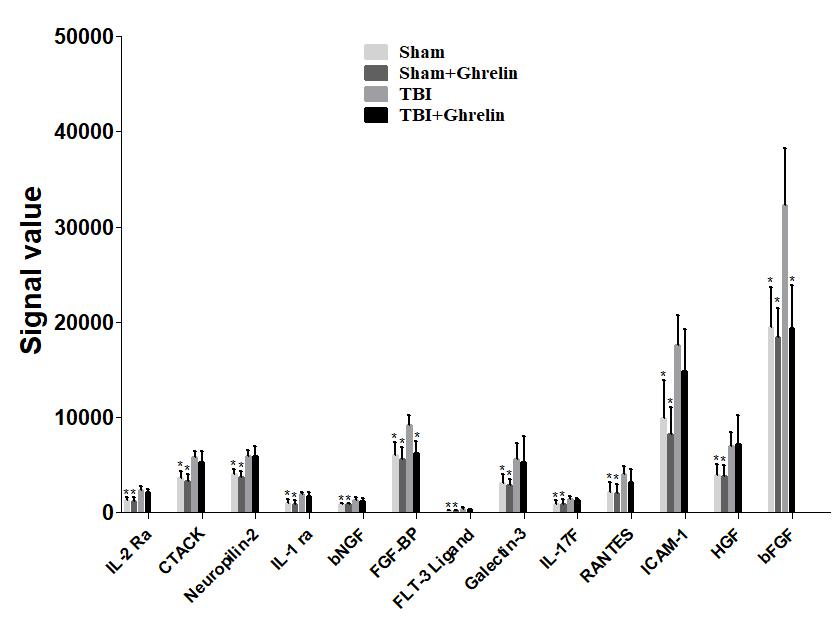
FIGURE 3. Altered factors after TBI. Thirteen proteins were found to be significantly increased after TBI, but only FGF-BP and bFGF were decreased after ghrelin treating TBI. The signal values from 13 proteins are shown by histograms to exhibit their expression levels. ∗p < 0.05 versus TBI group. Data are presented as means ± SD. n = 15 in each group.
Effect of Ghrelin on Protein Expression After TBI
To reveal the molecular mechanism of ghrelin treating TBI, the proteins expressed differentially between the TBI and ghrelin groups were statistically analyzed. As a result, among the cytokines increased in the TBI group, bFGF and FGF-BP were obviously decreased after treatment with ghrelin. As shown in Figure 4, the gray signal spots of bFGF in the Growth Factor Antibody Array in the TBI group were larger than in the sham group. Similarly, as shown in Figure 5, FGF-BP, in the Cytokine Antibody Array 4, displayed brighter fluorescent signals. These results represented bFGF and FGF-BP levels were increased in the TBI group, and were decreased in ghrelin group.
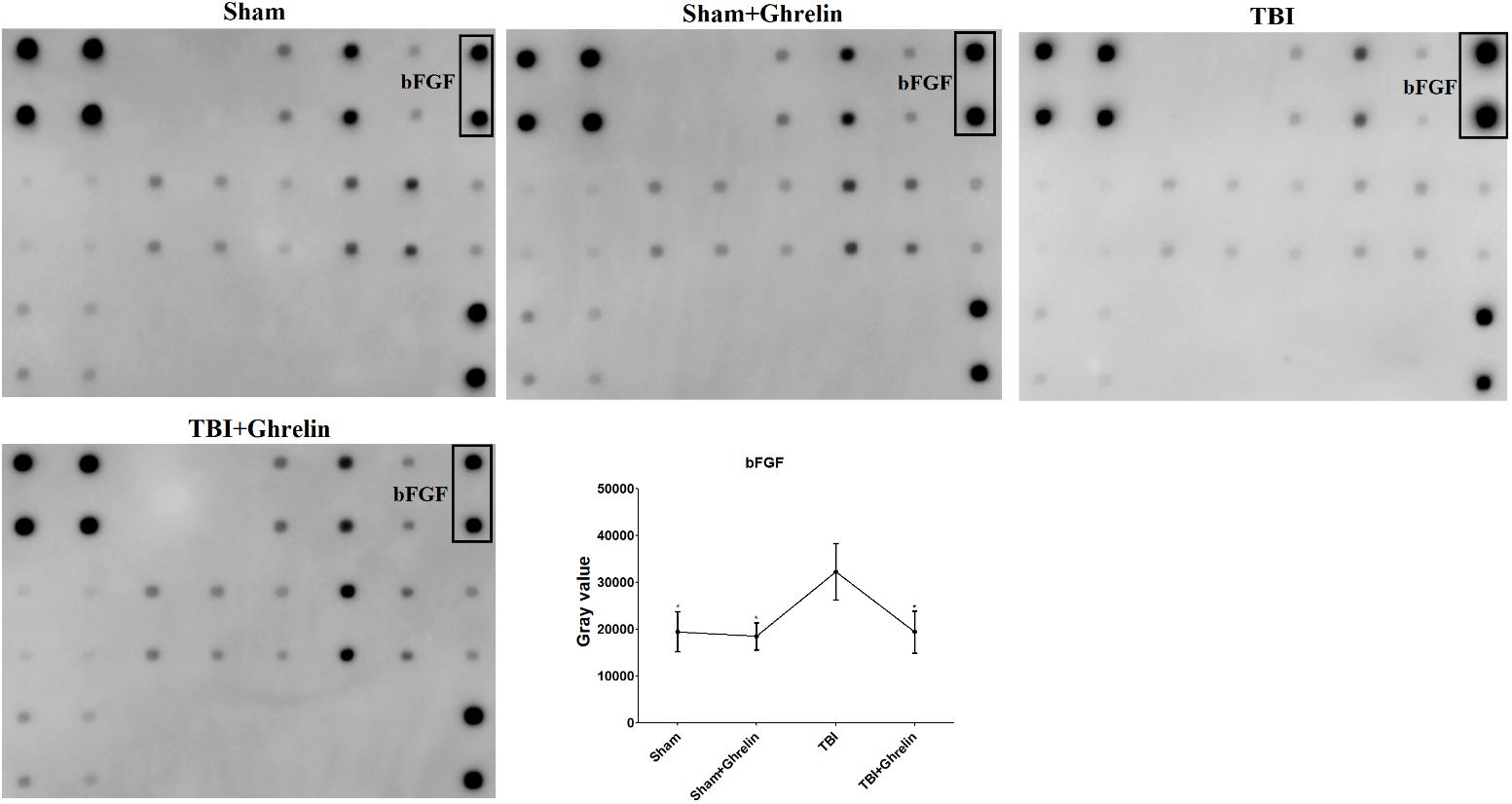
FIGURE 4. Antibody array profiles and expression levels of bFGF among the four groups. bFGF was detected by a RayBio Growth Factor Antibody Array, which is a membrane series array. bFGF location in array is encircled by black boxes. The signal values of bFGF among the four groups is shown by line graphs. ∗p < 0.05 versus TBI group. Data are presented as means ± SD. n = 15 in each group.
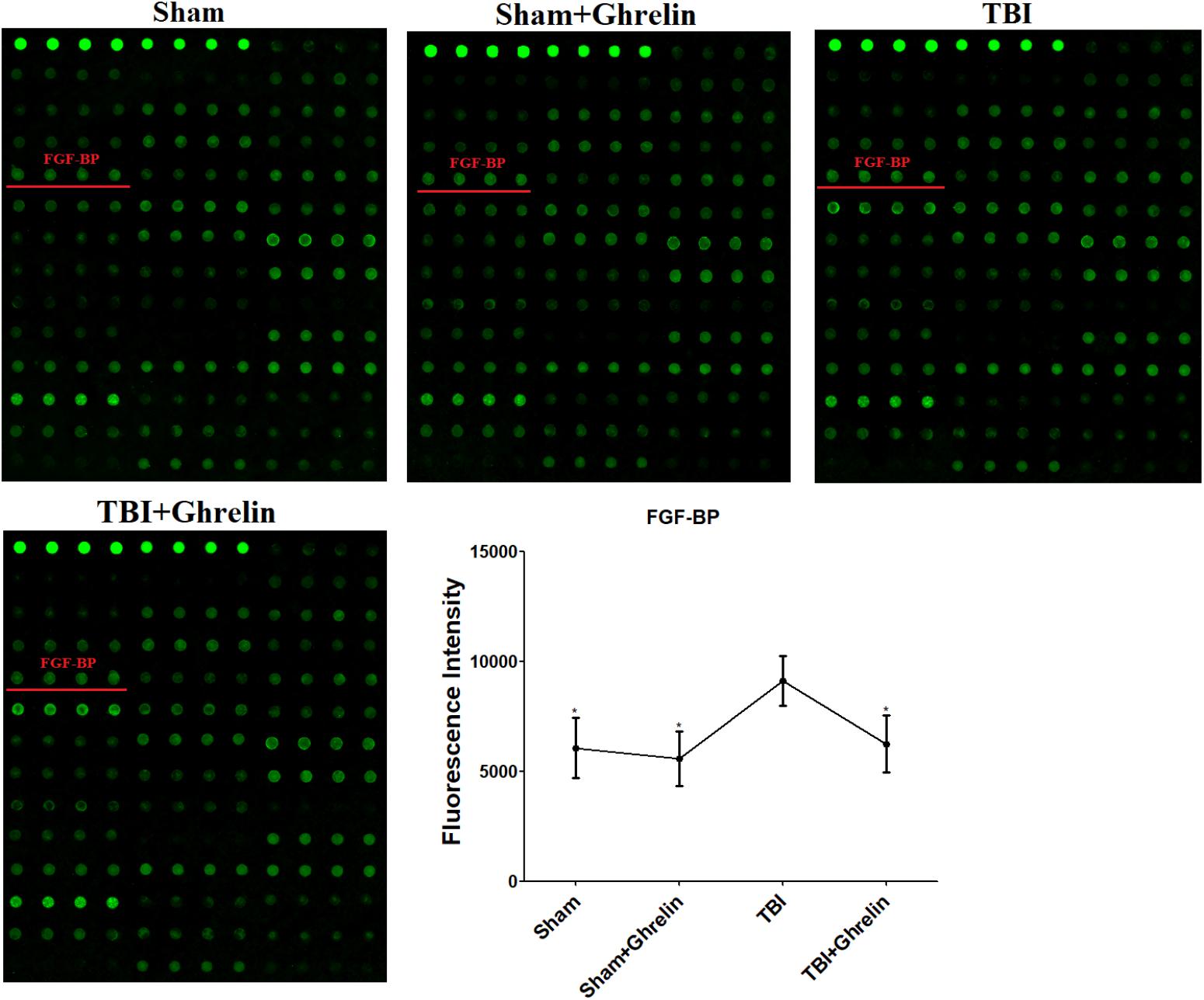
FIGURE 5. Antibody array profiles and expression levels of FGF-BP among the four groups. FGF-BP was detected with a RayBio Rat Cytokine Antibody Array 4, a glass series array. FGF-BP location in the array is labeled by red underscores. The signal values of FGF-BP among the four groups is shown by line graphs. ∗p < 0.05 versus TBI group. Data are presented as means ± SD. n = 15 in each group.
ELISA Validation Results
In order to validate the altered protein expression affected by ghrelin, ELISA was performed for bFGF and FGF-BP. Consistently, the ELISA results showed that bFGF and FGF-BP were up-regulated after TBI, and were down-regulated in the ghrelin treatment group (Figure 6). These results were in accordance with the results of the antibody array, and certify further the effect of ghrelin on bFGF and FGF-BP expression.
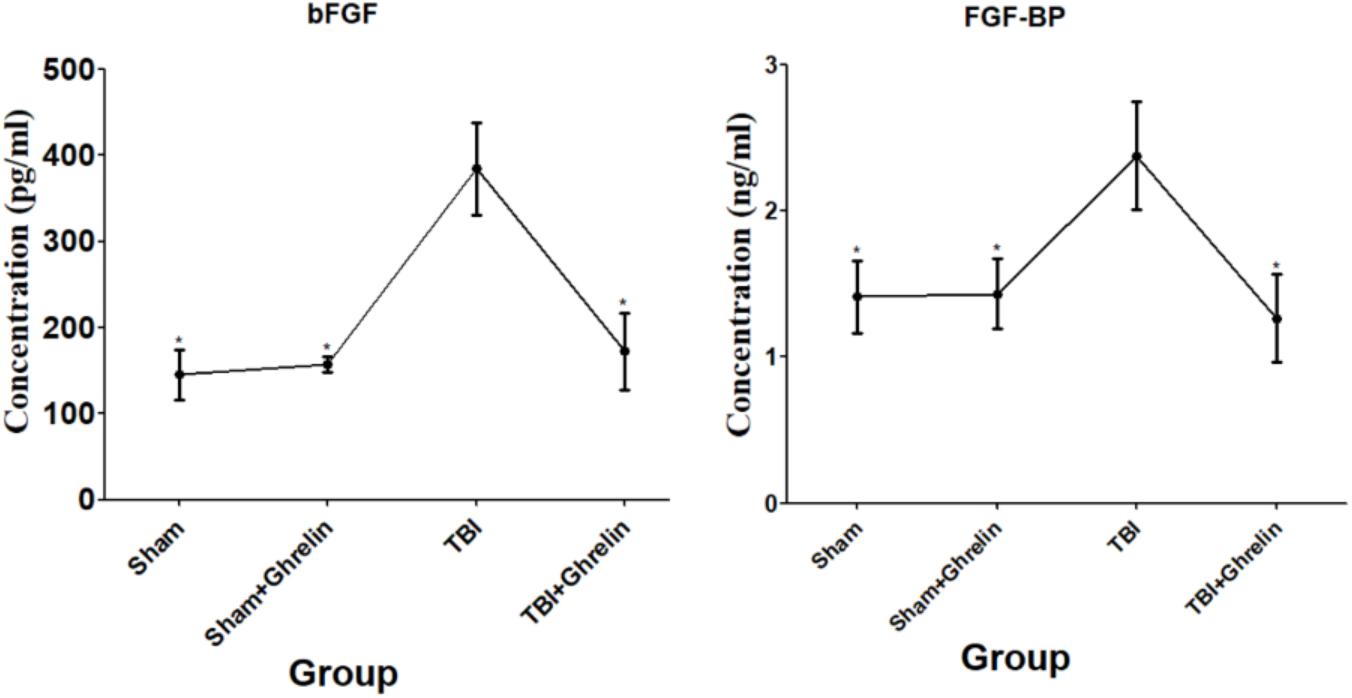
FIGURE 6. ELISA validation for bFGF and FGF-BP. The proteins participating in the molecular mechanism of ghrelin against TBI bFGF and FGF-BP were chosen for validation by ELISA. The concentrations of bFGF and FGF-BP obtained from ELISA are shown by line graphs. ∗p < 0.05 versus TBI group. Data are presented as means ± SD. n = 15 in each group.
Discussion
Traumatic brain injury with neurological functional impairment has high rates of mortality and morbidity, can cause complicated metabolic, physiologic, and cellular responses, and, in particular, a marked inflammatory response. In the present study, we utilized traditional methods such as NSS evaluation and histological examination to authenticate a TBI model. Additionally, antibody array technology revealed that TBI could result in elevated expressions of IL-17F, IL-1 ra, RANTES, Galectin-3, ICAM-1, bFGF, HGF, IL-2 Ra, FLT-3 Ligand, CTACK, FGF-BP, bNGF and Neuropilin-2. As reported previously, among these biomarkers, IL-17F, IL-1 ra, RANTES, Galectin-3, ICAM-1,bFGF,HGF, IL-2 Ra, bNGF and Neuropilin-2 had been found to exhibit raised levels in brain injury (Kumon et al., 1993; Isaksson et al., 1997; Gabellec et al., 1999; Lenzlinger et al., 2001; Shimamura et al., 2007; Svetlov et al., 2012; Zhuang and Li, 2013; Shen et al., 2016; Albert et al., 2017; Li et al., 2017), which further demonstrated the successful establishment of our TBI model and revealed the molecular mechanism promoting TBI. Moreover, FLT-3 Ligand, CTACK and FGF-BP were found to be increased after TBI, implying the complexity of TBI pathogenesis. Bioinformatics analysis of these factors showed the most enriched Gene Ontology (GO) terms were “response to external stimulus,” “cellular response to stimulus,” “response to stimulus.” TBI occurs from external trauma, which will activate these proteins in order to regulate the cellular response to this stimulus.
More importantly, in the present study, ghrelin treatment exhibited evident improvement of TBI symptoms. The improvement was corroborated by the results of NSS evaluation and histological examination. Ghrelin is a 28-amino acid orexigenic peptide, and has been found to have neuroprotective effects in several models of neurological diseases. Relevant to the neuroprotective mechanism, Sun et al. (2016) discovered ghrelin attenuates brain injury in septic mice by increasing the expression of p-Akt and Bcl-2 and decreasing Bax expression. Previous study (Qi et al., 2014) reported that ghrelin protects rats against traumatic brain injury through up-regulation of UCP2. However, the mechanism by which ghrelin acts against TBI may be complex due to the interplay of proteins. The present study is the first to investigate comprehensively the mechanism of ghrelin against TBI by employing an antibody array. As a result, we found 13 factors that were up-regulated in the brain cortex after TBI, and, among these factors regulating TBI progression, bFGF and FGF-BP were obviously decreased after ghrelin treatment.
Basic fibroblast growth factor, as one of growth factors which are important mediators for neurogenesis, is a potent mitogenic factor for neural stem cells. Some studies have shown that administration of bFGF could reduce focal ischemia-induced infarct size, improve sensorimotor function, and promote functional recovery of rats who were subjected to motor cortex lesions (Li and Stephenson, 2002; Monfils et al., 2005). Moreover, elevated bFGF expression levels have been found during brain development and various forms of brain insult, suggesting that elevation of bFGF may play a key role in initiating nerve regeneration and scar production to respond the cellular wounding (Logan et al., 1992; Shetty et al., 2005). FGF-BP is a secreted protein that reversibly and non-covalently binds these FGFs to protect them from degradation and to enhance their biological activity. Some studies have reported that FGF-BP is up-regulated during the initial healing phase, such as a FGF-BP upregulation after surgical wounding of human skin grafts or of mouse skin within a few hours, but a return to control levels after 2 days with wound closure, and exogenous FGF-BP increased the activity of bFGF to stimulate neurite outgrowth and accelerated wound healing by enhancing bFGF-mediated angiogenesis (Kurtz et al., 2004; Beer et al., 2005; Tassi et al., 2007). Similarly, the present study showed the levels of bFGF and FGF-BP were elevated during TBI, implying bFGF and FGF-BP secretion might be activated by the organism to promote wound healing and resist TBI. Then, after ghrelin treatment for 72 h, the levels of bFGF and FGF-BP were reduced, suggesting that ghrelin might effectively treat TBI, causing the closure of bFGF and FGF-BP effects on wound healing by down-regulating bFGF and FGF-BP. As known, bFGF and FGF-BP involve into the wound healing process by neovascularization of wounds. However, Baiguera et al. (2004) found that ghrelin could inhibit bFGF-mediated angiogenesis of rat brain microvascular endothelial cells, hinting exogenous ghrelin probably promotes the repair of injured brain tissues by another pathway and competitively inhibiting bFGF/FGF-BP-induced neovascularization. Therefore, it is unclear whether the relationship of Ghrelin with bFGF and FGF-BP is causative or competitive, which might need further identification.
Conclusion
We show that ghrelin attenuated brain injury and promoted brain functional recovery by reducing the levels of bFGF and FGF-BP. However, it has not been identified whether the relationship of Ghrelin with bFGF and FGF-BP on TBI treatment is causative or competitive, which will be researched soon.
Moreover, if the combinational administration of ghrelin and bFGF/FGF-BP can enhance the therapeutic effects in TBI, this would be a great stride in the treatment of TBI.
Author Contributions
XS and XJ conceived and designed the experiments and analyzed the data. XS, QH, SC, QW, PX, and XJ performed the experiments. XS wrote the paper.
Funding
This work was supported by the priority of research funds of Wannan Medical College (Grant No. WK2017ZF04) and Collegiate Major Natural Science Research Projects (Grant No. KJ2018ZD027), Anhui Province, China.
Conflict of Interest Statement
The authors declare that the research was conducted in the absence of any commercial or financial relationships that could be construed as a potential conflict of interest.
Supplementary Material
The Supplementary Material for this article can be found online at: https://www.frontiersin.org/articles/10.3389/fnins.2018.00445/full#supplementary-material
TABLE S1 | Maps of RayBio antibody arrays.
DATA SHEET S1 | Antibody array data of differential proteins.
References
Aarabi, B., and Simard, J. M. (2009). Traumatic brain injury. Curr. Opin. Crit. Care 15, 548–553. doi: 10.1097/MCC.0b013e32833190da
Abizaid, A. (2009). Ghrelin and dopamine: new insights on the peripheral regulation of appetite. J. Neuroendocrinol. 21, 787–793. doi: 10.1111/j.1365-2826.2009.01896.x
Albert, V., Subramanian, A., Agrawal, D., Bhoi, S. K., Pallavi, P., and Mukhopadhayay, A. K. (2017). RANTES levels in peripheral blood, CSF and contused brain tissue as a marker for outcome in traumatic brain injury (TBI) patients. BMC Res. Notes 10:139. doi: 10.1186/s13104-017-2459-2
Baiguera, S., Conconi, M. T., Guidolin, D., Mazzocchi, G., Malendowicz, L. K., Parnigotto, P. P., et al. (2004). Ghrelin inhibits in vitro angiogenic activity of rat brain microvascular endothelial cells. Int. J. Mol. Med. 14, 849–854. doi: 10.3892/ijmm.14.5.849
Beer, H. D., Bittner, M., Niklaus, G., Munding, C., Max, N., Goppelt, A., et al. (2005). The fibroblast growth factor binding protein is a novel interaction partner of FGF-7, FGF-10 and FGF-22 and regulates FGF activity: implications for epithelial repair. Oncogene 24, 5269–5277. doi: 10.1038/sj.onc.1208560
Bansal, V., Ryu, S. Y., Blow, C., Costantini, T., Loomis, W., Eliceiri, B., et al. (2010). The hormone ghrelin prevents traumatic brain injury induced intestinal dysfunction. J. Neurotrauma 27, 2255–2260. doi: 10.1089/neu.2010.1372
Gabellec, M. M., Crumeyrolle-Arias, M., Le Saux, F., Auriou, N., Jacque, C., and Haour, F. (1999). Expression of interleukin-1 genes and interleukin-1 receptors in the mouse brain after hippocampal injury. Neurosci. Res. 33, 251–260. doi: 10.1016/S0168-0102(99)00014-0
Huang, W. D., Fei, Z., and Zhang, X. (2005). Traumatic injury induced homer-la gene expression in cultured cortical neurons of rat. Neurosci. Lett. 389, 46–50. doi: 10.1016/j.neulet.2005.07.014
Isaksson, J., Lewén, A., Hillered, L., and Olsson, Y. (1997). Up-regulation of intercellular adhesion molecule 1 in cerebral microvessels after cortical contusion trauma in a rat model. Acta Neuropathol. 94, 16–20. doi: 10.1007/s004010050666
Kojima, M., Hosoda, H., Date, Y., Nakazato, M., Matsuo, H., and Kangawa, K. (1999). Ghrelin is a growth-hormone-releasing acylated peptide from stomach. Nature 402, 656–660. doi: 10.1038/45230
Kumon, Y., Sakaki, S., Kadota, O., Matsuda, S., Fujita, H., Yoshimura, H., et al. (1993). Transient increase in endogenous basic fibroblast growth factor in neurons of ischemic rat brains. Brain Res. 605, 169–174. doi: 10.1016/0006-8993(93)91369-4
Kurtz, A., Aigner, A., Cabal-Manzano, R. H., Butler, R. E., Hood, D. R., Sessions, R. B., et al. (2004). Differential regulation of a fibroblast growth factor-binding protein during skin carcinogenesis and wound healing. Neoplasia 6, 595–602. doi: 10.1593/neo.04214
Lenzlinger, P. M., Hans, V. H., Jöller-Jemelka, H. I., Trentz, O., Morganti-Kossmann, M. C., and Kossmann, T. (2001). Markers for cell-mediated immune response are elevated in cerebrospinal fluid and serum after severe traumatic brain injury in humans. J. Neurotrauma 18, 479–489. doi: 10.1089/089771501300227288
Li, Q., and Stephenson, D. (2002). Postischemic administration of basic fibroblast growth factor improves sensorimotor function and reduces infarct size following permanent focal cerebral ischemia in the rat. Exp. Neurol. 177, 531–537. doi: 10.1006/exnr.2002.7994
Li, T., Zhang, Y. M., Han, D., Hua, R., Guo, B. N., Hu, S. Q., et al. (2017). Involvement of IL-17 in secondary brain injury after a traumatic brain injury in rats. Neuromolecular Med. 19, 541–554. doi: 10.1007/s12017-017-8468-4
Logan, A., Frautschy, S. A., Gonzalez, A. M., and Baird, A. (1992). A time course for the focal elevation of synthesis of basic fibroblast growth factor and one of its high-affinity receptors (flg) following a localized cortical brain injury. J. Neurosci. 12, 3828–3837. doi: 10.1523/JNEUROSCI.12-10-03828.1992
Lopez, N. E., Gaston, L., Lopez, K. R., Coimbra, R. C., Hageny, A., Putnam, J., et al. (2012). Early ghrelin treatment attenuates disruption of the blood brain barrier and apoptosis after traumatic brain injury through a UCP-2 mechanism. Brain Res. 1489, 140–148. doi: 10.1016/j.brainres.2012.10.031
Lopez, N. E., Krzyzaniak, M. J., Blow, C., Putnam, J., Ortiz-Pomales, Y., Hageny, A. M., et al. (2011). Ghrelin prevents disruption of the blood-brain barrier after traumatic brain injury. J. Neurotrauma 29, 385–393. doi: 10.1089/neu.2011.2053
McCauley, S. R., Boake, C., Pedroza, C., Brown, S. A., Levin, H. S., Goodman, H. S., et al. (2005). Postconcussional disorder: are the DSM-IV criteria an improvement over the ICD-10? J. Nerv. Ment. Dis. 193, 540–550. doi: 10.1097/01.nmd.0000172592.05801.71
Monfils, M. H., Driscoll, I., Vandenberg, P. M., Thomas, N. J., Danka, D., Kleim, J. A., et al. (2005). Basic fibroblast growth factor stimulates functional recovery after neonatal lesions of motor cortex in rats. Neuroscience 134, 1–8. doi: 10.1016/j.neuroscience.2005.04.006
Qi, L., Cui, X. X., Dong, W. F., Barrera, R., Coppa, G. F., Wang, P., et al. (2014). Ghrelin protects rats against traumatic brain injury and hemorrhagic shock through upregulation of UCP2. Ann. Surg. 260, 169–178. doi: 10.1097/SLA.0000000000000328
Shen, Y. F., Yu, W. H., Dong, X. Q., Du, Q., Yang, D. B., Wu, G. Q., et al. (2016). The change of plasma galectin-3 concentrations after traumatic brain injury. Clin. Chim. Acta 456, 75–80. doi: 10.1016/j.cca.2016.02.029
Shetty, A. K., Hattiangady, B., and Shetty, G. A. (2005). Stem/progenitor cell proliferation factors FGF-2, IGF-1, and VEGF exhibit early decline during the course of aging in the hippocampus: role of astrocytes. Glia 51, 173–186. doi: 10.1002/glia.20187
Shimamura, M., Sato, N., Sata, M., Wakayama, K., Ogihara, T., and Morishita, R. (2007). Expression of hepatocyte growth factor and c-Met after spinal cord injury in rats. Brain Res. 1151, 188–194. doi: 10.1016/j.brainres.2007.03.022
Sun, N., Wang, H., Ma, L., Lei, P., and Zhang, Q. (2016). Ghrelin attenuates brain injury in septic mice via PI3K/Akt signaling activation. Brain Res. Bull. 124, 278–285. doi: 10.1016/j.brainresbull.2016.06.002
Svetlov, S. I., Prima, V., Glushakova, O., Svetlov, A., Kirk, D. R., Gutierrez, H., et al. (2012). Neuro-glial and systemic mechanisms of pathological responses in rat models of primary blast overpressure compared to “composite” blast. Front. Neurol. 3:15. doi: 10.3389/fneur.2012.00015
Tassi, E., Walter, S., Aigner, A., Cabal-Manzano, R. H., Ray, R. J., Reier, P. J., et al. (2007). Effects on neurite outgrowth and cell survival of a secreted fibroblast growth factor binding protein upregulated during spinal cord injury. Am. J. Physiol. Regul. Integr. Comp. Physiol. 293, R775–R783. doi: 10.1152/ajpregu.00737.2006
Wei, H., Cao, X., Zeng, Q., Zhang, F., Xue, Q., Luo, Y., et al. (2015). Ghrelin inhibits proinflammatory responses and prevents cognitive impairment in septic rats. Crit. Care Med. 43, 43–150. doi: 10.1097/CCM.0000000000000930
Keywords: ghrelin, traumatic brain injury, neuroprotective effects, bFGF, FGF-BP
Citation: Shao X, Hu Q, Chen S, Wang Q, Xu P and Jiang X (2018) Ghrelin Ameliorates Traumatic Brain Injury by Down-Regulating bFGF and FGF-BP. Front. Neurosci. 12:445. doi: 10.3389/fnins.2018.00445
Received: 13 April 2018; Accepted: 12 June 2018;
Published: 05 July 2018.
Edited by:
Hector J. Caruncho, University of Victoria, CanadaReviewed by:
Changiz Taghibiglou, University of Saskatchewan, CanadaCarina Rodrigues Boeck, Centro Universitário Franciscano, Brazil
Copyright © 2018 Shao, Hu, Chen, Wang, Xu and Jiang. This is an open-access article distributed under the terms of the Creative Commons Attribution License (CC BY). The use, distribution or reproduction in other forums is permitted, provided the original author(s) and the copyright owner(s) are credited and that the original publication in this journal is cited, in accordance with accepted academic practice. No use, distribution or reproduction is permitted which does not comply with these terms.
*Correspondence: Xiaochun Jiang, amlhbmd4aWFvY2h1bjIwMDFAaG90bWFpbC5jb20=