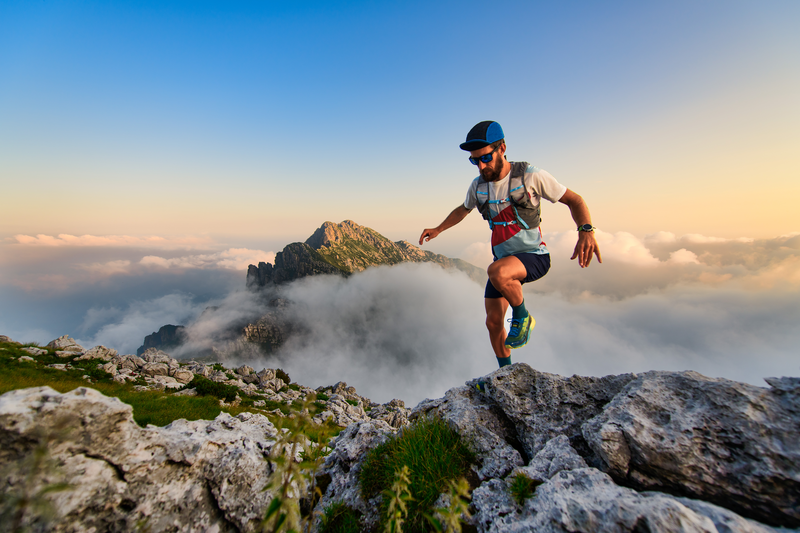
95% of researchers rate our articles as excellent or good
Learn more about the work of our research integrity team to safeguard the quality of each article we publish.
Find out more
REVIEW article
Front. Neurosci. , 05 March 2018
Sec. Neurodegeneration
Volume 12 - 2018 | https://doi.org/10.3389/fnins.2018.00125
Age-related hearing loss (ARHL), presbycusis, is a chronic health condition that affects approximately one-third of the world's population. The peripheral and central hearing alterations associated with age-related hearing loss have a profound impact on perception of verbal and non-verbal auditory stimuli. The high prevalence of hearing loss in the older adults corresponds to the increased frequency of dementia in this population. Therefore, researchers have focused their attention on age-related central effects that occur independent of the peripheral hearing loss as well as central effects of peripheral hearing loss and its association with cognitive decline and dementia. Here we review the current evidence for the age-related changes of the peripheral and central auditory system and the relationship between hearing loss and pathological cognitive decline and dementia. Furthermore, there is a paucity of evidence on the relationship between ARHL and established biomarkers of Alzheimer's disease, as the most common cause of dementia. Such studies are critical to be able to consider any causal relationship between dementia and ARHL. While this narrative review will examine the pathophysiological alterations in both the peripheral and central auditory system and its clinical implications, the question remains unanswered whether hearing loss causes cognitive impairment or vice versa.
Age-related hearing loss (ARHL) or Presbycusis, used interchangeably in this paper, is a common chronic health problem affecting individuals above 65 years old (WHO, 2012). ARHL is defined as a progressive, bilateral, and symmetrical hearing loss primarily observed in the high frequency region (Fetoni et al., 2011). Gates and Mills (2005) defined ARHL as a multifactorial disorder affecting hearing sensitivity varying from mild to substantial, resulting from lifetime insults to the auditory system. This review discusses the ARHL prevalence and risk factors, mechanisms of peripheral and central hearing loss, changes in the peripheral and central hearing pathway, and its consequences for speech understanding and cognition.
Most of the ARHL epidemiological studies have reported hearing loss prevalence data in the 500 Hz−4 kHz (Cruickshanks et al., 1998b; Gopinath et al., 2009), and/or between 4 and 8 kHz regions. However, age-related changes in hearing thresholds occur significantly earlier in the extended high frequencies, i.e., >8 kHz (Matthews et al., 1997; Sakamoto et al., 1998; Lee et al., 2005, 2012). Lee et al. (2012) reported two different aging process that impact the hearing thresholds across the entire frequency range: a slower process in the low frequencies (up to 4 kHz) and a faster process at higher frequencies (6–12.5 kHz) that in combination represent the thresholds spectrum across the entire hearing frequency range. For frequencies at 6, 8, 10, 11.2, and 12.5 kHz, the faster aging process starts at age 51, 47, 46, 36, and 30. In addition, for individuals aged 65–81 years old there was a decline of 0.7 dB per year at 0.25 kHz, increasing to 1.2 dB per year at 8 kHz, and 1.23 dB per year at 12 kHz (Lee et al., 2005). These results imply that by the time the first conventional pure tone audiogram (0.25–8 kHz) demonstrates ARHL, higher frequency loss (i.e., 10–12.5 kHz) could have already occurred. Therefore, preventive measures should be applied much earlier than previously thought, if the subsequent consequences of ARHL is to be minimized.
National Health and Nutritional Examination Survey (NHANES), 2005-2006 data on participants aged > 70 years old revealed that on average, speech frequency (0.5–4 kHz), and high-frequency (3–8 kHz) PTA thresholds of the black participants were better than white participants by −5.8 (95% CI: −8.6 to −3.1), and −11.1 (95% CI: −13.9 to −8.2), respectively (Lin et al., 2011b). Agrawal et al. (2008) found that the odds of hearing loss was 70% lower in black vs. white individuals. Similarly, Helzner et al. (2005a) reported that from a sample of 2,052 participants, white participants were 63% more likely to have a hearing loss compared to black participants. The data from the National Health and Nutritional Examination Survey (NHANES) on 20–59 year old participants revealed that darker-skinned Hispanics showed better speech and high-frequency PTA compared to lighter-skinned Hispanics by an average of −2.5 dB hearing level (HL; 95% CI, −4.8 to −0.2) and −3.1 dB HL (95% CI, −5.3 to −0.8), respectively (Lin et al., 2012). These results indicate that melanocyte function may be protective against hearing loss in darker skin Hispanics (Lin et al., 2012).
For frequencies between 0.5–8 kHz, the prevalence of ARHL is two times higher in men compared to women (Agrawal et al., 2008; Gopinath et al., 2009). For example, bilateral hearing loss across the 60–69 years old age group is reported to be 43% and 20% in American men and women (Agrawal et al., 2008), and 29% and 17% in Australian men and women, (Gopinath et al., 2009), respectively. The onset of ARHL can be detected around the age of 30 years in men across the 0.5–8 kHz frequencies, however, the onset of ARHL in women occurs much later in life and varies across frequencies (Pearson et al., 1995). In addition, the rate of decline in hearing thresholds is much higher in men than women across 4–8 kHz frequencies, especially after the age of 50 years (Pearson et al., 1995). In contrary, hearing thresholds at 6 to 12 kHz frequencies decline at a significantly faster rate in women than men (Lee et al., 2005). Surprisingly, gender effect for extended high frequencies >8 kHz has not been reported for 10–65 year old individuals (Lee et al., 2012) or for 60–79 year age groups (Matthews et al., 1997).
Many attempts have been made to identify possible environmental and genetic risk factors associated with ARHL (Fetoni et al., 2011). Epidemiological studies have indicated that many of these factors are indeed very significant in the trajectory of ARHL. Yamasoba et al. (2013) identified four categories of risk factors associated with ARHL in humans: genetic predisposition, environment, health co-morbidities and cochlear aging. The first three risk factors associated with ARHL are reviewed here and cochlear aging will be discussed in the section on the impact of aging on the peripheral hearing system.
Contribution of the genetic factors to ARHL in humans have been well-documented. Primary evidence comes from epidemiological studies on twin families in Swedish, North American, and Danish cohorts. A heritability of 0.47 for ARHL in Swedish mono-and dizygotic twins aged > 65 years (Karlsson et al., 1997), 0.4 for siblings (worse-ear average high-frequency thresholds) and 0.25 for parents and children (for worse ear middle and low frequency thresholds) have been reported (Framingham study; Gates et al., 1999). The heritability of hearing loss, as assessed using self-reports, for Danish twins aged 75 years old and over was at 0.4 (Christensen et al., 2001).
Genome wide association studies have reported a strong link between ARHL and single nucleotide polymorphisms (SNPs) located in GRM7, a gene encoding metabotropic glutamate receptor type 7 protein (mGluR7) (Friedman et al., 2009; Newman et al., 2012).
A number of genes and mutations responsible for monogenic non-syndromic hearing loss are linked to ARHL, including: (i) SNPs in 13-kb region in the middle of the KCNQ4, a gene which encodes a voltage-gated K-channel found in both outer and inner hair cells of cochlea (Van Eyken et al., 2006); (ii) 35delG mutation of GJB2, a gene that encodes gap junction proteins expressed in the inner ear (Van Eyken et al., 2007c); (iii) GRHL2, a gene that encodes a transcription factor expressed in cells lining cochlear duct (Lin et al., 2011); and (iv) mutation in MYO6, a gene that encodes myosin VI found in inner ear hair cells (Oonk et al., 2013).
In addition, genes that are linked to oxidative stress such as mutant allele (NAT2*6A), an isoform of N-acetyltransferases-encodes metabolism of reactive oxygen species (Ünal et al., 2005; Van Eyken et al., 2007b), and Glutathione S-transferases (GSTs)-encodes synthesis of glutathione antioxidant enzymes (Ateş et al., 2005) and mitochondrial dysfunction such as deletion of mitochondrial DNA 4,977 bp (Bai et al., 1997) and mitochondrial DNA haplogroups U and K are also reported to be associated with ARHL (Manwaring et al., 2007).
Furthermore, several candidate genes including apolipoprotein E (APOE) ε4 allele (O'Grady et al., 2007), variants of Endothelin-1 (EDN1) (Uchida et al., 2009) and polymorphisms of uncoupling proteins UCP2 Ala55Val (Sugiura et al., 2010) that are responsible for age-related diseases or clinical syndromes are found to be linked to ARHL (see also: Bovo et al., 2011).
In animal model studies, mice have been a useful animal model for studying the underlying cellular and genetic mechanism of human ARHL due to their small size, short life span, and genetic standardization (Ohlemiller, 2006). A number of loci that contribute to ARHL have been identified in inbred mice strains (Johnson et al., 2006). In various, inbred mouse strains an Ahl gene (age-related hearing loss) on chromosome 10 in C57BL/6J mice was found to be present (Johnson et al., 1997). Resistant alleles for Ahl 2, 4, and 8 were reported in the same mouse model (Johnson and Zheng, 2002; Johnson et al., 2008) and for Ahl 5 and 6 were found in CAST/Ei mice strains (Drayton and Noben-Trauth, 2006). Few other mouse models have been used to investigate the genetic mutations involved in ARHL (for examples see: Johnson et al., 2008; Sha et al., 2008; Ohlemiller, 2009). Further investigation into genetic bases of ARHL will further our understanding of pathogenesis processes that occur during aging and will also help provide more refined preclinical models of the disease.
A variety of environmental risk factors such as exposure to industrial chemicals (Campo et al., 2013), occupational (Helzner et al., 2005a; Fransen et al., 2008), and recreational noise exposure (Clark, 1991) are reported to be associated with ARHL. Synergistic effects of simultaneous exposure to industrial chemicals and noise has a greater effect on ARHL than the impact of either one of the agents acting on its own (Morata et al., 2002; Sliwinska-Kowalska et al., 2004; Chang et al., 2006; Fuente and McPherson, 2006).
Exposure of mice to loud noises resulted acutely in substantial loss of cochlear afferent terminals and a slower degeneration of spiral ganglion cells, without significant cochlear hair cell loss or pure-tone hearing threshold loss (Kujawa and Liberman, 2009). Further, dysfunction of synaptic ribbons, which are essential for the accurate acoustic transmission between cochlear inner hair cells and post-synaptic afferent neurons (Fuchs et al., 2003) are documented in ARHL (Stamataki et al., 2006) and in noise-induced hearing loss (Kujawa and Liberman, 2009). For a review see Moser et al. (2013). Acoustic over-exposure also results in selective loss of low- and medium-spontaneous rate auditory nerve fibers in albino guinea pigs (Furman et al., 2013). The loss of spiral ganglion neurons (Kujawa and Liberman, 2006, 2009) and selective loss of low and medium spontaneous rate fibers (Furman et al., 2013) leads to poor temporal and frequency discrimination leading to impaired speech discrimination in noise in the absence of any audiometric loss (Plack et al., 2014). Such a hearing loss is known as “hidden hearing loss” (Plack et al., 2014). Kujawa and Liberman (2006) detected an increased vulnerability to primary neural degeneration of cochlea from mice that were exposed to noise at a younger age. Interestingly, genes (Ahl/Ahl) associated with ARHL increase the susceptibility to noise-induced hearing loss at a younger age in mice (Erway et al., 1996). These findings support the interaction between genetic predisposition to ARHL, noise and ARHL and should be taken into account in future preventive clinical trials.
A number of health-related factors are reported to either increase the risk of ARHL or in fact provide protection against its development. Risk factors increasing ARHL include smoking (Helzner et al., 2005a; Nomura et al., 2005; Fransen et al., 2008) cardiovascular disease (Helzner et al., 2011), Type II Diabetes Mellitus (Frisina et al., 2006; Mitchell et al., 2009), and subclinical atherosclerosis (Fischer et al., 2015) are reported to increase the risk of ARHL. A number of oto-toxic medication are associated with irreversible hearing loss (Koegel, 1985; Brummett and Fox, 1989; Kwong et al., 1996). In contrast, moderate alcohol consumption (Popelka et al., 2000; Helzner et al., 2005a; Fransen et al., 2008), high bone mineral density (Helzner et al., 2005b), and dietary restrictions (Seidman et al., 2002) are described as to provide some protective impact against ARHL.
The inner ear is more vulnerable to age-related changes than both the external and middle ear (Schmiedt, 2010). Hence, pathophysiological changes related to cochlea and afferent neurons are discussed here. Based on underlying case history information, audiometric configurations, and temporal bone analyses, Schuknecht (1974) divided ARHL into four distinct classes: sensory (loss of hair cells and supporting cells), neural (loss of afferent neurons), strial (atrophy of cochlear lateral wall and Stria vascularis), and cochlear conductive (cochlear atrophy mainly basilar membrane and organ of Corti). Rapid high-frequency sloping hearing loss, flat hearing loss, poor word discrimination and gradual hearing loss with no pathological evidence are characteristics of sensory, strial, neural, and cochlear conductive presbycusis, respectively (Schuknecht and Gacek, 1993). Later, Schuknecht and Gacek (1993) added two more categories: (i) mixed-consisting of a mixture of pathological characteristics and (ii) indeterminate-consisting of none of the aforementioned pathological characteristics. Contrary to the above-mentioned findings, Allen and Eddins (2010) reported that hearing phenotypes do not naturally form distinct classes of ARHL and rather form a continuum. However, subtypes of ARHL can still be identified if the distribution of data are in the extremes of either flat or sloping loss (Allen and Eddins, 2010).
Evidence for age-related pathological changes were reported in human temporal bone studies as well as in animal models. Histopathological studies have reported progressive loss and degeneration of spiral ganglion cells (Mills et al., 2006b; Hinojosa and Nelson, 2011), loss of nerve fibers in spiral lamina (Belal, 1975; Mills et al., 2006a) and hypertrophy of the internal elastic lamina of the internal auditory artery (Belal, 1975) in aged adults. Progressive outer hair cell loss with no degeneration of the cells have been reported in Stria vascularis (Sha et al., 2008), spiral ganglion cell degeneration (Keithley et al., 2004), and degeneration of organ of Corti and afferent neurons (Ohlemiller and Gagnon, 2004) in mouse models. Loss of outer hair cells and detachment of the stria vascularis from the spiral ligament was seen in aging Fisher rat F344 models (Buckiova et al., 2007). Loss of marginal and intermediate cells of Stria vascularis (Gates and Mills, 2005) and loss of Stria capillaries (Gratton and Schulte, 1995) were noted in aged gerbil models.
Two most prominent physiological changes have been observed in the aging cochlear: decline in cochlear endolymphatic potentials (EP) and increase in action potential (CAP) of the auditory nerve (Gates and Mills, 2005). Recent studies have concluded that metabolic/strial presbycusis or degenerative changes in the lateral wall and stria vascularis as the predominant cause of ARHL (Gates and Mills, 2005; Ohlemiller, 2009). The above mentioned pathological changes of the stria vascularis leads to loss of expression of key ion transport enzymes, such as Na+, K+-ATPase, and the Na+, K+, Cl− co-transporter, impairment in outer hair cell functions and decline in endolymphatic potential (EP) values (Salt et al., 1987; Wangemann, 2002). The EP provides voltage for the cochlear amplifier, thus, a decline in EP results in impairment in cochlear amplifier ultimately resulting in poor hearing thresholds (Schmiedt et al., 2002; Gates and Mills, 2005).
Another physiological impairment observed in aging cochlear is asynchronous firing of the auditory nerve fibers as indicated by increased thresholds of the compound action potential of the auditory nerve (Gates and Mills, 2005). A combination of pathological changes of the spiral ganglion cells and synaptic connections between the inner hair cells and afferents and decline in EP is thought to be one of the underlying causes of the auditory nerve malfunction (Gates and Mills, 2005).
Yamasoba et al. (2013) proposed a model to explain the development of ARHL. According to this model, a number factors (aging, genetic, environmental, health co-morbidity) and their interactions contribute to ARHL. Mitochondrial dysfunction associated with reactive oxygen species (ROS) plays a major role in the age-related cochlear cells degeneration (Cheng et al., 2005; Someya and Prolla, 2010). Noise trauma also generate excess of ROS in the cochlea (Henderson et al., 2006) triggering necrotic or apoptic cell death in cochlea (Henderson et al., 2006). Oxidative stress can be accelerated by hypoxic situations resulting from decline in cochlear blood supply due to noise trauma (Wen et al., 2017) or cardiovascular diseases (Nomiya et al., 2008) and genetic factors (Uchida et al., 2009), Ototoxic medication (Tabuchi et al., 2011), and health co-morbidity (Cruickshanks et al., 1998a) factors. Long-duration mitochondrial function leads to Bak-dependent apoptosis of the cochlear cells leading to increase in hearing thresholds (Someya and Prolla, 2010). Details of ARHL environmental and health-related co-morbid risk factors are described in Environmental Factors and Health Co-morbidities. Genetic investigations have identified a number genes associated with cochlear aging (see section Genetics). In addition, genes that are associated with antioxidant protection, mitochondrial dysfunction (see section Genetics), also contribute to the cochlear hair cell dysfunction.
The age-associated changes reported in cochlear nucleus and the auditory cortex have also been a focus of research. As Figure 1 shows afferent auditory fibers from the cochlea terminate at the cochlear nucleus (CN) complex which includes the anteroventral cochlear nucleus (AVCN), posteroventral cochlear nucleus (PVCN), and dorsal cochlear nucleus (DCN) (Brawer et al., 1974). First stage of centralized auditory processing occurs at the CN complex (Schmiedt, 2010). AVCN and PVCN carry out the spectral analysis of the auditory signal (Rhode and Greenberg, 1994) and AVCN and DCN process the localization cues (May, 2000). Both animal and human models have been used to investigate the underlying patho- physiological changes of the central auditory system associated with aging. The alterations in animal models from the CN to the auditory cortex are discussed first.
Figure 1. Schematic diagram of the auditory pathway. This schematic diagram represents ipsi and contralateral auditory pathways from cochlea to the auditory cortex. Main nuclei of the central auditory pathway, namely Superior Olivary Complex (SOC), Dorsal and Ventral Cochlear Nuclei (DCN & VCN), Lateral lemniscus (LL), Inferior Colliculus (IC), Medial Geniculate body (MGB), and Primary Auditory Cortex (AC) are shown in the figure.
A number of important age-associated changes of the CN has been reported in animal models, briefly including: (i) decline in overall volume, total number, and neuron size of octopus cells in PVCN (C57 and CBA mice; Willott et al., 1992, 1994); (ii) decline in glycine mediated inhibition in DCN reported in Fisher-344 rats (Caspary, 2005); (iii) increase in total number and percentage of calcium binding protein (parvalbumin and calbindin) positive neurons in DCN and PVCN reported in C57BL/6J mice (Idrizbegovic et al., 2004); (iv) increase in the number of parvalbumin and nicotinamide adenine dinucleotide hydrogen phosphate diaphorase (NADPH-d) positive neurons in PVCN (Rhesus Macaque; Gray et al., 2014a); (v) decline in spontaneous miniature excitatory post-synaptic currents (mEPSC) in the high-frequency regions of AVCN [DBA mice] (Wang and Manis, 2005); and finally, (vi) age-related decline in processing of the temporal properties of the complex signals in DCN (e.g., Fischer Brown Norway rats; Schatteman et al., 2008). It is thought that age- associated changes in calcium binding proteins disrupt the calcium homeostasis and could lead to impaired synaptic transmission, reduced neural plasticity, and degeneration of neurons (Mattson, 2007). Spherical and globular bushy cells of the AVCN are involved in processing inter-aural time difference cues which are essential for sound localization in horizontal plane (Carney, 1990), hence, age-related alterations that occur in synaptic transmission in AVCN cells could result in poor sound localization and pitch detection of the acoustic signals. Furthermore, changes in processing in DCN could underlie the temporal deficits observed in older adults (Schatteman et al., 2008).
Only a handful of studies on the effect of aging of the superior olivary complex (SOC) are available. The SOC comprises three main nuclei, namely the medial and lateral superior olive (MSO and LSO, respectively) and medial nucleus of the trapezoid body (MNTB), (Harrison and Warr, 1962). The SOC plays a vital role in sound localization (Masterton, 1992) by processing inter-aural temporal and intensity differences (Grothe, 2000) and conveying the information to higher [lateral lemniscus (LL), inferior colliculi (IC), and medial geniculate nucleus (MGB)] and lower (to the cochlea and CN) centers of the auditory pathway (Oliver, 2000; Thompson and Schofield, 2000). Age-associated axonal and dendritic degeneration and loss of synaptic terminals have been observed in Sprague-Dawley rat medial nucleus of the trapezoid body (Casey and Feldman, 1982, 1985, 1988), however, no neuronal loss has been reported in LOS and MSO of aged Fischer 344 rats (Casey, 1990). Additionally, an age-related increase in parvalbumin and NADPH-d have been reported in MSO of rhesus macaques, but not in LSO and MNTB, suggesting an underlying compensatory mechanism to account for the poor auditory processing ability of the aging non-human primates (Gray et al., 2014b).
The inferior colliculus (IC) is considered one of the major convergence centers for both mono-and-binaural auditory information (Figure 1). It simultaneously processes the acoustic features of complex signals along with the other relay stations of the auditory pathway (Schmiedt, 2010). Age-associated cytochemical changes of the IC include: (i) a marked decline in GABA mediated inhibition (Burianova et al., 2009); (ii) increase in parvalbumin positive neurons (Engle et al., 2014); (iii) decline in the number of calbindin and calretinin positive neurons (Ouda and Syka, 2012); and (iv) decline in number of SMI-32-immunoreactive neurons and levels of non-phosphorylated neuro-filament proteins (Burianová et al., 2015). These changes influence the temporal processing of acoustical stimuli and reported to be contributing to central presbycusis (Ouda and Syka, 2012; Ouda et al., 2012).
Auditory thalamus/medial geniculate body (MGB) neurons transmit auditory stimuli to cortex and other subcortical structures by filtering and enhancing acoustic features of the auditory signal (Figure 1) (Bartlett, 2013). Gamma-aminobutyric acid (GABA) mediated inhibitory inputs are fundamental for auditory processing in the MGB (Cotillon-Williams et al., 2008). This inhibitory neurotransmission is obtained through synaptic and high-affinity GABAA receptors (Richardson et al., 2013). More than 45% reduction in GABAA receptor density and GABAA receptor mediated tonic whole cell Cl− currents was found in aged rat MGB. In addition, an increase in the number of parvalbumin positive neurons have also been reported in aged rhesus macaque MGB (Gray et al., 2013). Ouda et al. (2012) reported a decrease in calbindin positive neurons in Long Evans and -Fischer 344 rat MGB. These results are indicative of significant age-dependent deficits in the inhibitory neurotransmission within the MGB. Burianová et al. (2015) failed to observe age-associated decline in total number of neurons in IC, MGB, or primary auditory cortex (A1) of Long Evans and Fischer F344 rats. Temporal processing speed is affected by the senescent changes that occur in the cortex but not in the auditory thalamus or midbrain (Mendelson and Ricketts, 2001; Lee et al., 2002). Burianová et al. (2015) reported decline in number of neurons containing non-phosphorylated neuro-filaments and their protein levels, which are known for their conductance properties in the central auditory system. Overall, these changes may negatively impact processing of complex auditory signals in older adults (Richardson et al., 2013).
A number of animal experiments have reported age-related changes of A1. These include, (i) decline in the number of calcium binding positive neurons in rat A1 (Ouellet and de Villers-Sidani, 2014); (ii) decrease in the number and firing of V/U-shaped receptive field neurons in A1(Turner, 2005a); (iii) decline in number of neurons containing non- phosphorylated neuro-filaments and proteins (Burianová et al., 2015); and increase in (iv) spontaneous (29%), peak (24%), and steady state (38%) neuronal response rates in A1-layer V (Turner, 2005a); and (v) the number and firing of complex receptor field neurons in the A1-layer V (Turner, 2005a). These findings indicate that age-dependant changes in A1-layer V neurons could be responsible for diminished signal/noise coding (Turner, 2005a). Further, degradation of processing between cortical areas is reported to contribute to cognitive decline associated with the aging process (Juarez-Salinas et al., 2010). Cytochemical findings in C57BL/6J mice suggest that age-related alterations of glial cells in A1 are exacerbated by peripheral hearing loss (Tremblay and Burkhard, 2012). The A1 shows age-related decreases in pre- and post-synaptic GABA neurotransmission (Caspary et al., 2013). A significant age-associated decline in the number and density of GAD65 and 67 immuno-reactive neurons and GAD67 proteins in A1 layers have been observed in aged rats compared to their young adult controls (Ling et al., 2005; Burianova et al., 2009). These changes are accompanied by age-dependant alterations in the expression of GABAA receptor subunits. Together, these findings support the notion that age-related alterations of GABA neurotransmission alter the temporal coding properties of the primary auditory cortex. (Šuta et al., 2011) provided further evidence for age-related decline in temporal processing in Long Evans rats through behavioral and electrophysiological experimental measures. In sum, current animal model studies suggest that temporal processing of acoustical signals is associated with aging of the central auditory system and thus considered a major contributor to the poor speech perception skills seen in older adults (Ling et al., 2005; Caspary et al., 2013).
Finally, a number of animal experiments suggest that age-related pathophysiological changes of the central auditory system are further exacerbated by the peripheral hearing loss. For example, tonotopic reorganization of central auditory system following peripheral hearing loss has been reported in several animal experiments (Willott, 1984, 1986; Willott et al., 1993). Robertson and Irvine (1989) provided convincing evidence for cortical reorganization in guinea pigs 35–81 days following unilateral cochlear lesions. The auditory cortical areas that represented the frequencies of the damaged cochlea were responsive to the sound frequencies adjacent to the damaged frequency range. Response thresholds of the characteristic frequencies of these reorganized cortical neurones were close to their normal thresholds (Mean difference = −3.8 dB). Similar findings have been observed few hours after a cochlear lesion except that the reorganized cortical neurons had higher response thresholds compared to their normal thresholds (mean difference 31.7 dB).
Recent human studies have utilized several different neuroimaging techniques to investigate the age-related changes of the human central nervous system including magnetic resonance imaging (MRI) to measure brain volumes and MRI spectroscopy for delineating neurochemical and metabolic changes (Ouda et al., 2015). Some of studies on the brain-related changes associated with auditory system will be summarized in this section.
Structural MRI studies have shown a reduction in both gray (Raz et al., 1997, 2004; Lemaitre et al., 2012) and white matter volumes (Silver et al., 1997; Raz et al., 2004, 2007) and cortical thinning due to aging (Lemaitre et al., 2012). Hedman et al. (2012) reviewed 56 longitudinal MRI studies to investigate the age-related brain volume changes in healthy adults. Results revealed annual brain volume loss of 0.2% at 35 years old reaching 0.5% by the age of 60 years. Greater than 0.5% annual brain volume loss observed after 60 years. Age-associated marked decline in temporal lobe volume (Scahill et al., 2003), hippocampal volume (Scahill et al., 2003; Raz et al., 2004), and prefrontal cortex (Raz et al., 1997) have been reported in region-specific imaging studies. Alterations in the above mentioned neural networks are implicated in impaired cognitive functions such as verbal recognition memory (Fletcher and Henson, 2001; Henson, 2005), episodic visuospatial memory, learning and association ability (Sweeney et al., 2000), working memory and executive functions (Owen et al., 1995, 1996), and attention switching (Makhani et al., 2015).
MRI spectroscopy has been used to describe age-related neurochemical and metabolic changes of the central nervous system (Gujar et al., 2005; Gruber et al., 2008; Kirov et al., 2008; Reyngoudt et al., 2012; Gao et al., 2013, 2015). Age-related decrease in glutamate and N-acetyl aspartate levels have been observed in elderly participants with mild and expressed ARHL (Profant et al., 2013). An increase in lactate levels has been observed in elderly participants with expressed ARHL, however, no significant changes in GABA concentrations was noted between young and elderly participants(Profant et al., 2013). Contrary to these observations, Gao et al. (2015) reported significantly lower GABA+ concentrations in participants with ARHL as compared to normal hearing controls. Data from participants with ARHL revealed a significantly negative correlation between pure-tone audiometric average across 500–4,000 Hz and GABA+ concentrations (Gao et al., 2015). These results are consistent with the hypothesis of dysfunctional GABAergic neurotransmission in central auditory system in those with ARHL.
Fewer studies have investigated the impact of ARHL on central auditory system using neuroimaging techniques. In a cohort of 126 participants aged between 56 and 86 years old with normal to severe sensorineural hearing loss, ARHL was shown to be independently associated with higher rate of decline in the entire brain volume and right temporal lobe volume (Lin et al., 2014). A summary of MRI studies revealed that the ARHL results in the changes of the central auditory pathway, including (i) decline in gray matter volume observed in superior and middle temporal gyri (Husain et al., 2011), superior and medial frontal gyrus (Husain et al., 2011; Boyen et al., 2013), primary auditory cortex (Peelle et al., 2011; Eckert et al., 2012), occipital lobe (Boyen et al., 2013), and hypothalamus (Boyen et al., 2013); (ii) a decline in both gray and white matter areas near auditory cortex (Husain et al., 2011); (iii) a decrease in fraction anisotropy values in SOC (Chang et al., 2004), LL & IC (Chang et al., 2004; Lin et al., 2008), and auditory cortex (Chang et al., 2004); and (iv) a decrease in fraction anisotropy values in a number of white matter tracks including right superior and inferior longitudinal fasciculi and corticospinal tract (Husain et al., 2011). Collectively, these findings suggest that ARHL not only results in secondary pathophysiological changes in the central auditory pathway, but also in the areas of the brain that are not directly involved in processing auditory stimuli. The underlying mechanism for the association of peripheral hearing loss and cortical tonotopic reorganization secondary to peripheral hearing loss may further compromise listening and comprehension abilities of older adults (Peelle et al., 2010, 2011).
Difficulties expressed by older adults in understanding speech could be due to age-related deficits in peripheral and/or central auditory pathways (Gates and Mills, 2005). Among the peripheral factors that impact speech understanding, poor audibility resulting from cochlear pathology is considered to play a significant role (George et al., 2007). However, part of the problem in recognizing speech in older adults seem to be associated with temporal deficits resulting from aging and ARHL (Gordon-Salant and Fitzgibbons, 1993). Several studies have investigated the impact of aging on central auditory processing of speech stimuli (See Humes et al., 2010 for a review). These studies have used competing speech (Jerger et al., 1991; Gates et al., 2008), temporally-distorted speech (Gordon-Salant and Fitzgibbons, 2001), and/or binaural speech stimuli (Jerger et al., 1991; Gates et al., 2008). A review by Humes and Dubno (2010) concluded that aging significantly impairs all three speech test measures and hearing loss was the major factor on negatively impacting participants' performance on tasks that utilized competing and time compressed speech stimuli. The above mentioned temporal processing deficits could explain some of the difficulties faced by older adults, with or without hearing loss, in perceiving speech in challenging situations-i.e., accented speech (Gordon-Salant et al., 2013) and speech in noise or reverberation (Dubno et al., 1984; Gordon-Salant and Fitzgibbons, 1993). Further, speech comprehension in quiet and in noise also relies on cognitive functions (Pichora-Fuller et al., 1995). These include searched based attention (Pichora-Fuller and Singh, 2006), selective attention (Alain and Arnott, 2000), divided attention (Kemper et al., 2003), working memory (Pichora-Fuller et al., 1995), and processing speed (Pichora-Fuller and Souza, 2003).
There are two key components of the auditory system involved in the processing of incoming auditory stimuli; the peripheral hearing system and the central hearing system (Katz, 2015). Approximately 83% of adults aged 70 years and above suffer from a peripheral hearing loss (Cruickshanks et al., 1998b). Peripheral hearing loss not only affects the auditory processing of speech sounds but also the higher-level cognitive functions required to process linguistically demanding stimuli (Stewart and Wingfield, 2009; Peelle et al., 2011). For example, the hearing impaired individuals show difficulty in attending to working memory (Wingfield et al., 2006) and auditory and visual free recall tasks (Wingfield et al., 2006) and require longer latencies to make accurate perceptual judgments (Tun et al., 2010). Evidence from both cross-sectional (Valentijn et al., 2005; Tay et al., 2006; Jayakody et al., 2018a), and longitudinal (Valentijn et al., 2005; Lin et al., 2011a; Deal et al., 2016) studies found an association between peripheral hearing loss and cognitive impairment in older adults. A meta-analysis study reported an association between hearing loss and cognitive impairment, with the degree of cognitive impairment being significantly associated with the severity of both untreated and treated peripheral hearing loss (Taljaard et al., 2015). Further, an association between ARHL and risk of incident dementia (Lin et al., 2011a; Gallacher et al., 2012; Gurgel et al., 2014; Deal et al., 2016) and risk of incident Alzheimer's disease (AD) (Lin et al., 2011a) has also been reported. Most of the studies reported above have considered verbally loaded cognitive measures to assess the cognitive functions of older adults. Cumulative evidence strongly suggest that hearing loss could result in overestimation of the level of cognitive impairment in hearing impaired participants (Jorgensen, 2012; Dupuis et al., 2015). Therefore, non-verbal cognitive measures could be more appropriate for older adults with potential ARHL. A small number of recent studies that investigated the association between ARHL and cognitive impairment by removing auditory items of the cognitive screening tests (Dupuis et al., 2015) or using non-auditory tests of cognition (Lin et al., 2013, 2017; Wong et al., 2014; Jayakody et al., 2018a) have also reported an association between peripheral hearing loss and cognitive impairment.
In some individuals, difficulties experienced during comprehending speech in noisy backgrounds or competing speakers could be related to central auditory processing (CAP) difficulties rather than peripheral hearing deficits (Panza et al., 2015). Results from a number of longitudinal studies suggest that CAP in the absence of severe peripheral hearing loss is associated with high incidence of cognitive decline and dementia due to AD (Gates et al., 1996, 2002, 2008, 2011; Profant et al., 2015). These studies have demonstrated that individuals with central auditory dysfunction were at a significantly increased risk for incident dementia with hazard ratios ranging from 9.9 (95% confidence interval [CI], 3.6–26.7) to 23.3 (95% CI, 6.6–82.7) (Gates et al., 1996, 2011; Profant et al., 2015). Gates et al. (1996) reported that the relative risk of developing cognitive decline or dementia was twice higher for those with bilateral poor CAP scores as measured by Synthetic Identification-Ipsilateral Competing Message (SSI-ICM) scores compared to those with unilateral poor SSI-ICM scores. Furthermore, impaired central auditory functions have been observed five to ten years prior to an official AD diagnoses (Iliadou et al., 2003; Bateman et al., 2012).
Numerous attempts have been made to provide plausible explanations for the association between ARHL and cognitive decline. Cognitive load on perception hypothesis argues that the decline in cognitive capacity increases the cognitive load resulting in a sensory loss (CHABA, 1988; Lindenberger and Baltes, 1994). Sensory Deprivation hypothesis proposes that hearing loss leads to permanent deterioration in cognitive functions (CHABA, 1988; Lindenberger and Baltes, 1994; Schneider and Pichora-Fuller, 2000; Pichora-fuller, 2003; Lin et al., 2011b, 2013; Humes et al., 2013). Cortical reorganization following ARHL provides substantial evidence to support the sensory deprivation hypothesis (Husain et al., 2011; Peelle et al., 2011; Eckert et al., 2012; Boyen et al., 2013). Findings demonstrating that severe to profound hearing impaired children and young adults with a long duration of hearing impairment performed similar to their hearing peers on tests of intelligence seem to contradict the sensory deprivation hypothesis (Vernon, 2005). Hence, one could argue that hearing is not the sole contributor to cognitive impairment, rather one of the factors. Information degradation hypothesis postulates that hearing loss results in reversible cognitive decline (CHABA, 1988; Schneider and Pichora-Fuller, 2000; Pichora-fuller, 2003). Supportive evidence for the information degradation hypothesis can be found in a large number of existing research studies. In sum, these studies suggest that older adults rely more on cognitive resources to interpret degraded speech signals, resulting from hearing loss thereby placing more demand on executive functions and working memory (Pichora-Fuller et al., 1995; Pichora-fuller, 2003; Pichora-Fuller and Singh, 2006; Amichetti et al., 2013).
Common cause hypothesis argues that a common mechanism underlies age-associated changes observed in cognition, hearing, and other sensory modalities (CHABA, 1988; Lindenberger and Baltes, 1994; Baltes and Lindenberger, 1997). It has been suggested that general age-related neuropathological changes in the brain may actually explain this relationship, but current data do not support this general domain and function specific underlying mechanism theory (Lindenberger and Ghisletta, 2009). For example, when the relationship between hearing loss and memory was examined, a significant relationship was observed even after controlling for the effects of age and the type of task (e.g., verbal vs. visual), confirming hearing loss is an independent factor involved in cognitive decline (Lindenberger and Ghisletta, 2009).
Wayne and Johnsrude (2015) proposed a new framework with a common cause phenomena approach, where age-related changes of the brain result in decline of sensory (e.g., ARHL) and cognitive abilities. According to this hypothesis, the changes in sensory abilities increase the demand on the already strained cognitive system resulting in functional cognitive impairment (Wayne and Johnsrude, 2015).
Recently, a cognitive reserve hypothesis has been proposed to explain how individuals with similar neuropathological conditions differ substantially in their ability to make efficient use of brain reserve during tasks (Stern, 2002). Intelligence (Alexander et al., 1997) and higher education (Amieva et al., 2014), occupational level (Staff et al., 2004), participation in leisure activities (Scarmeas et al., 2001), and social networking (Fratiglioni et al., 2000) are considered to be contributing factors to the cognitive reserve. If sensory or cognitive demands exceeds the cognitive reserve capacity, it could lead to impairment in cognitive abilities (Wayne and Johnsrude, 2015). However, according to cognitive reserve hypothesis, the observed cognitive impairment could be reversed if the perceptual challenges were reduced (i.e., improved speech perception through amplification; Wayne and Johnsrude, 2015). In summary, no single hypothesis has been able to provide a comprehensive inference with regards to the causal relationship between ARHL and cognitive impairment.
Even though, ARHL is still not considered as a major risk factor for AD, it is a modifiable age-associated condition linked to dementia and late-life cognitive decline (Livingston et al., 2017). The Lancet International Commission on Dementia Prevention, Intervention, and Care has estimated that a third of AD diagnoses worldwide could be delayed or prevented, with early intervention and changes to lifestyle and utilizing available public health strategies (Livingston et al., 2017) such as post-lingual hearing loss correction. Furthermore, studies demonstrate that if the prevalence of each risk factor is reduced by only 10–20% per decade, the number of AD diagnoses could reduce by between 8.8 and 16.2 million worldwide by 2050 (Norton et al., 2014). As an example of potential changes in outcome measures following hearing loss correction, we have recently reported that cochlear implant recipients performed significantly better on cognitive function measures compared to similar individuals on a waiting list to receive cochlear implants (Jayakody et al., 2017). Others have also reported similar positive results following hearing loss improvement using hearing aids and cochlear implantation (Castiglione et al., 2016; Sonnet et al., 2017). Whether the correction of ARHL could significantly delay or arrest the late life pathological cognitive decline, dementia, or AD neurodegeneration is yet to be investigated. However, treating ARHL is extremely low risk, has significant health, social, and safety benefits beyond cognition, and there are enough evidence to discuss this option with ARHL patients (Golub, 2017).
ARHL is an important marker in frailty (Panza et al., 2015). Frailty has been defined as a clinical syndrome that contains three or more of the following symptoms: unintentional weight loss (10 lbs in past year), self-reported exhaustion, weakness (grip strength), slow walking speed, and low physical activity (Fried et al., 2001). Frailty increases the risk of detrimental health hazards such as falls (Speechley and Tinetti, 1991), institutionalization (Rockwood et al., 1996), hospitalization (Purser et al., 2006), and death (Ensrud et al., 2007). Interestingly, the risk of frailty in older adults with hearing loss increases by 63% and ARHL appears to be an independent risk factor for frailty with great risk of falls (Kamil et al., 2016). Further, ARHL has been shown to be associated with health-related outcomes linked to frailty, such as low level of physical activity (Gispen et al., 2014), slow gait speed (Li et al., 2013), and incident falls (Lin and Ferrucci, 2012). In addition, frailty is reported to be associated with cognitive impairment (Ávila-Funes et al., 2009; Yassuda et al., 2012), cognitive decline (Samper-Ternent et al., 2008; Auyeung et al., 2011) and incident dementia (Solfrizzi et al., 2013). See Robertson et al. (2013) for a review.
Social isolation and loneliness is considered one of the risk factors for ARHL. Mick and Lin (2013) found a significant association between ARHL and social isolation in the 60–69 year old age group [odds ratio = 2.4, 95% CI = (1.36, 4.23) p = 0.003]. The association was more pronounced in females than in males (Ramage-Morin, 2016). ARHL is significantly associated with late-life depression, anxiety, and stress (Jayakody et al., 2018b). Depression is considered an early manifestation of dementia and AD (Panza et al., 2010). Accumulated evidence suggest that depression accelerates the aging process by increasing the incident risk factors of age-associated diseases such as cardiovascular diseases (Frasure-Smith et al., 1993), metabolic disturbances (McIntyre et al., 2007), and cognitive impairment (Lee et al., 2011; Dawes et al., 2015). Further, a bidirectional association between depression and late-life frailty has also been reported (Mezuk et al., 2012). Freret et al. (2015) proposed that an imbalance between brain reserve, resilience, neuroplasticity, and impaired pathophysiological mechanisms associated with aging, stress, and synaptic plasticity may lead to late-life depression. Although the pathophysiological mechanisms underlying depression, ARHL, and dementia remain to be further elucidated, a putative mechanism underlying both depression and dementia linked to neuro-inflammation and perfusion deficits in older adults (Maes et al., 2009; see Popa-Wagner et al., 2014, for a review).
Based on the above reported findings, there seems to be a bidirectional association between ARHL and cognitive impairment, depression and social isolation, depression, and frailty (Figure 2). As shown in the schematic approach in Figure 2, primary factors including aging, genetic predispositions, environmental factors, and medical and health related conditions accompany cognitive decline, ARHL, and frailty. Furthermore, ARHL increases the risk of depression and frailty, both of which are associated with age-related cognitive decline and future risk of dementia. As ARHL is the primary modifiable risk factor for cognitive decline, depression, and social isolation in this model, its treatment may, to some degree, improve the associated outcomes and change the trajectory of cognitive impairment. The diagram shown in Figure 2 is a simplified account of the relationship between various known factors with the understanding that so many are yet to be determined. However, this model provides a basic rationale to argue that treating ARHL will provide multiple benefits, each with significant implications for future trajectory of cognitive functioning in older adults.
Figure 2. Directional associations between aging, ARHL, depression, social isolation and loneliness, frailty and cognitive impairment. The schematic diagram represents the unidirectional association between aging, age-related hearing loss (ARHL), and cognitive impairment, and unidirectional association between ARHL and social isolation, depression and frailty, and bidirectional association between depression and social isolation and depression and frailty.
A number of limitations can be identified in the existing body of literature on neuro- patho-physiological changes in the peripheral and central auditory systems due to aging. As mentioned in the previous sections, ARHL is influenced by different factors including aging, lifestyle choices, genetics, and environmental factors (Van Eyken et al., 2007a), hence, pathophysiological consequences of ARHL cannot be studied in isolation. Most of the inferences on the aging human auditory system have been drawn from the results of animal experiments or post-mortem human temporal bone studies. Inadequate sample size across lifespan studies, lack of robust experimental designs, and a paucity of comprehensive case history information cannot be underestimated in the available human studies. Another limitation relates to the hearing assessments methods used in previously published ARHL studies. The majority have utilized selective audiometric data to identify the decline in hearing sensitivity. Even though it has been established that the ARHL primarily affects high-frequency hearing thresholds, most of the studies have reported either 3 PTA (i.e., average of pure-tone thresholds at 500 Hz, 1 and 2 kHz) or 4PTA (i.e., average of pure-tone thresholds at 500 Hz, 1, 2, and 4 kHz) data, thereby omitting the vital high-frequency information. Whilst it has been well-documented that older adults have difficulty with perceiving complex sounds (speech or music-in-noise; Kim et al., 2006; Alain et al., 2014), only a handful of studies have incorporated speech-perception-in-noise tests in their designs. Further, no study, to our knowledge, has used vocal or instrumental sounds in the presence of background accompaniment, thus failing to provide an accurate picture of the real-life difficulties faced by elderly participants with ARHL. Finally, hearing thresholds should be taken into consideration while assessing cognitive functions of older adults. Failure to do so could underestimate the level of cognitive functioning in older adults with a hearing (Jayakody et al., 2018a) and/or visual impairment (Dupuis et al., 2015).
In conclusion, aging results in pathological and physiological changes in both peripheral and central auditory systems. A number of genetic, environmental, and health co-morbid factors increase the risk of ARHL. Peripheral hearing loss further exacerbates the changes in the central auditory pathway. In this review, we have described the evidence for the association between ARHL and cognitive impairment, AD, depression, social isolation, and frailty. There is no substaintial evidence of how ARHL and AD-related biomarkers are associated prior to the clinical manifestation of the dementia syndrome. This is a necessary yet expensive research question that will inform our understanding of the statistical associations reported between the two. However, it is clear that ARHL represents a modifiable condition and a useful target for secondary prevention of cognitive impairment, frailty, social isolation, and depression in older adults. Further research is required to comprehensively address underlying mechanisms and determine whether hearing loss treatment could delay or arrest late life cognitive decline or dementia. Specifically, longitudinal studies including randomized clinical trials with representative samples of hearing aid, and hearing implant users and rehabilitation programs could further elucidate the association between ARHL and other components of our proposed model in Figure 2.
All authors listed have made a substantial, direct and intellectual contribution to the work, and approved it for publication.
DJ and PF have no conflicts of interest to declare. RM is the founder and Chief Scientific Officer of the Alzhyme and KaRa Minds Institute. HS has, and continues to receive remuneration from activities with Pfizer and Takeda Pharmaceuticals. Although grants and funds for our research were provided by public and private organizations, they in no way influenced any part of this review, its conclusions or the decision to submit this manuscript for publication to this journal.
Authors would like to thank Dr Wilhelmina (Helmy) H.A.M. Mulders of the School of Anatomy, Physiology and Human Biology, University of Western Australia, for reviewing an earlier version of this manuscript.
Agrawal, Y., Platz, E. A., and Niparko, J. K. (2008). Prevalence of hearing loss and differences by demographic characteristics among US adults: data from the National Health and Nutrition Examination Survey, 1999-2004. Arch. Intern. Med. 168, 1522–1530. doi: 10.1001/archinte.168.14.1522
Alain, C., and Arnott, S. R. (2000). Selectively attending to auditory objects. Front. Biosci. 5, D202–D212.
Alain, C., Zendel, B. R., Hutka, S., and Bidelman, G. M. (2014). Turning down the noise: the benefit of musical training on the aging auditory brain. Hear. Res. 308, 162–173. doi: 10.1016/j.heares.2013.06.008
Alexander, G. E., Furey, M. L., Grady, C. L., Pietrini, P., Brady, D. R., Mentis, M. J., et al. (1997). Association of premorbid intellectual function with cerebral metabolism in Alzheimer's disease: implications for the cognitive reserve hypothesis. Am. J. Psychiatry 154, 165–172. doi: 10.1176/ajp.154.2.165
Allen, P. D., and Eddins, D. A. (2010). Presbycusis phenotypes form a heterogeneous continuum when ordered by degree and configuration of hearing loss. Hear. Res. 264, 10–20. doi: 10.1016/j.heares.2010.02.001
Amichetti, N. M., Stanley, R. S., White, A. G., and Wingfield, A. (2013). Monitoring the capacity of working memory: executive control and effects of listening effort. Mem. Cognit. 41:839. doi: 10.3758/s13421-013-0302-0
Amieva, H., Mokri, H., Le Goff, M., Meillon, C., Jacqmin-Gadda, H., Foubert-Samier, A., et al. (2014). Compensatory mechanisms in higher-educated subjects with Alzheimer's disease: a study of 20 years of cognitive decline. Brain 137, 1167–1175. doi: 10.1093/brain/awu035
Ateş, N. A., Ünal, S. M., Tamer, S. L., Derici, S. E., Karakaş, S. S., Ercan, S. B., et al. (2005). Glutathione S-transferase gene polymorphisms in presbycusis. Otol. Neurotol. 26, 392–397. doi: 10.1097/01.mao.0000169774.23668.f1
Auyeung, T. W., Lee, J. S., Kwok, T., and Woo, J. (2011). Physical frailty predicts future cognitive decline — A four-year prospective study in 2737 cognitively normal older adults. J. Nutr. Health Aging 15, 690–694. doi: 10.1007/s12603-011-0110-9
Ávila-Funes, J. A., Amieva, H., Barberger-Gateau, P., Le Goff, M., Raoux, N., Ritchie, K., et al. (2009). Cognitive impairment improves the predictive validity of the phenotype of frailty for adverse health outcomes: the three-city study. J. Am. Geriatr. Soc. 57, 453–461. doi: 10.1111/j.1532-5415.2008.02136.x
Bai, U., Seidman, M. D., Hinojosa, R., and Quirk, W. S. (1997). Mitochondrial DNA deletions associated with aging and possibly presbycusis: a human archival temporal bone study. Am. J. Otol. 18:449.
Baltes, P. B., and Lindenberger, U. (1997). Emergence of a powerful connection between sensory and cognitive functions across the adult life span: a new window to the study of cognitive aging? Psychol. Aging 12, 12–21.
Bartlett, E. L. (2013). The organization and physiology of the auditory thalamus and its role in processing acoustic features important for speech perception. Brain Lang. 126, 29–48. doi: 10.1016/j.bandl.2013.03.003
Bateman, R. J., Xiong, C., Benzinger, T. L. S., Fagan, A. M., Goate, A., Fox, N. C., et al. (2012). Clinical and Biomarker changes in dominantly inherited Alzheimer's disease. N. Engl. J. Med. 367, 795–804. doi: 10.1056/NEJMoa1202753
Bovo, R., Ciorba, A., and Martini, A. (2011). Environmental and genetic factors in age-related hearing impairment. Aging Clin. Exp. Res. 23, 3–10. doi: 10.1007/BF03324947
Boyen, K., Langers, D. R. M., de Kleine, E., and van Dijk, P. (2013). Gray matter in the brain: differences associated with tinnitus and hearing loss. Hear. Res. 295, 67–78. doi: 10.1016/j.heares.2012.02.010.
Brawer, J. R., Morest, D. K., and Kane, E. C. (1974). The neuronal architecture of the cochlear nucleus of the cat. J. Comp. Neurol. 155, 251–299. doi: 10.1002/cne.901550302.
Brummett, R. E., and Fox, K. E. (1989). Aminoglycoside-induced hearing loss in humans. Antimicrob. Agents Chemother. 33:797. doi: 10.1128/AAC.33.6.797
Buckiova, D., Popelar, J., and Syka, J. (2007). Aging cochleas in the F344 rat: morphological and functional changes. Exp. Gerontol. 42, 629–638. doi: 10.1016/j.exger.2007.02.007
Burianová, J., Ouda, L., and Syka, J. (2015). The influence of aging on the number of neurons and levels of non-phosporylated neurofilament proteins in the central auditory system of rats. Front. Aging Neurosci. 7:27. doi: 10.3389/fnagi.2015.00027
Burianova, J., Ouda, L., Profant, O., and Syka, J. (2009). Age-related changes in GAD levels in the central auditory system of the rat. Exp. Gerontol. 44, 161–169. doi: 10.1016/j.exger.2008.09.012
Campo, P., Morata, T. C., and Hong, O. (2013). Chemical exposure and hearing loss. Dis.Month 59:119. doi: 10.1016/j.disamonth.2013.01.003
Carney, L. H. (1990). Sensitivities of cells in anteroventral cochlear nucleus of cat to spatiotemporal discharge patterns across primary afferents. J. Neurophysiol. 64:437.
Casey, M. A. (1990). The effects of aging on neuron number in the rat superior olivary complex. Neurobiol. Aging 11, 391–394. doi: 10.1016/0197-4580(90)90004-J
Casey, M. A., and Feldman, M. L. (1982). Aging in the rat medial nucleus of the trapezoid body I. Light microscopy. Neurobiol. Aging 3, 187–195. doi: 10.1016/0197-4580(82)90039-2
Casey, M. A., and Feldman, M. L. (1985). Aging in the rat medial nucleus of the trapezoid Body. II. Electron microscopy. J. Comp. Neurol. 232, 401–413. doi: 10.1002/cne.902320311
Casey, M. A., and Feldman, M. L. (1988). Age-related loss of synaptic terminals in the rat medial nucleus of the trapezoid body. Neuroscience 24, 189–194. doi: 10.1016/0306-4522(88)90322-3
Caspary, D. M. (2005). Age-related changes in the inhibitory response properties of dorsal cochlear nucleus output neurons: role of inhibitory inputs. J. Neurosci. 25, 10952–10959. doi: 10.1523/JNEUROSCI.2451-05.2005
Caspary, D. M., Hughes, L. F., and Ling, L. L. (2013). Age-related GABAA receptor changes in rat auditory cortex. Neurobiol. Aging 34, 1486–1496. doi: 10.1016/j.neurobiolaging.2012.11.009
Castiglione, A., Benatti, A., Velardita, C., Favaro, D., Padoan, E., Severi, D., et al. (2016). Aging, cognitive decline and hearing loss: effects of auditory rehabilitation and training with hearing aids and cochlear implants on cognitive function and depression among older adults. Audiol. Neurootol. 21(Suppl. 1), 21–28. doi: 10.1159/000448350
CHABA (1988). Committee on hearing and bioacoustics and biomechanics: speech understanding and aging. J. Acoust. Soc. Am. 83, 859–895.
Chang, S.-J., Chen, C.-J., Lien, C.-H., and Sung, F.-C. (2006). Hearing loss in workers exposed to toluene and noise. (Environmental Medicine). Environ. Health Perspect. 114:1283. doi: 10.1289/ehp.8959
Chang, Y., Lee, S.-H., Lee, Y.-J., Hwang, M.-J., Bae, S.-J., Kim, M.-N., et al. (2004). Auditory neural pathway evaluation on sensorineural hearing loss using diffusion tensor imaging. Neuroreport 15, 1699–1703.
Cheng, A. G., Cunningham, L. L., and Rubel, W. E. (2005). Mechanisms of hair cell death and protection. Curr. Opin. Otolaryngol. Head Neck Surg. 13, 343–348. doi: 10.1097/01.moo.0000186799.45377.63
Christensen, K., Frederiksen, H., and Hoffman, H. J. (2001). Genetic and environmental influences on self-reported reduced hearing in the old and oldest old. J. Am. Geriatr. Soc. 49, 1512–1517. doi: 10.1046/j.1532-5415.2001.4911245.x
Cotillon-Williams, N., Huetz, C., Hennevin, E., and Edeline, J.-M. (2008). Tonotopic control of auditory thalamus frequency tuning by reticular thalamic neurons. J. Neurophysiol. 99:1137. doi: 10.1152/jn.01159.2007
Cruickshanks, K. J., Klein, R., Klein, B. E. K., Wiley, T. L., Nondahl, D. M., and Tweed, T. S. (1998a). Cigarette smoking and hearing loss: the epidemiology of hearing loss study. J. Am. Med. Assoc. 279, 1715–1719.
Cruickshanks, K. J., Wiley, T. L., Tweed, T. S., Klein, B. E. K., Klein, R., Mares-Perlman, J. A., et al. (1998b). Prevalence of hearing loss in older adults in Beaver Dam, Wisconsin. Am. J. Epidemiol. 148:879.
Dawes, P., Emsley, R., Cruickshanks, K. J., Moore, D. R., Fortnum, H., Edmondson-Jones, M., et al. (2015). Hearing loss and cognition: the role of hearing aids, social isolation and depression (hearing loss and cognition). PLoS ONE 10:e0119616. doi: 10.1371/journal.pone.0119616
Deal, J. A., Betz, J., Yaffe, K., Harris, T., Purchase-Helzner, E., Satterfield, S., et al. (2016). Hearing impairment and incident dementia and cognitive decline in older adults: the health ABC study. J. Gerontol. Ser. A Biol. Sci. Med. Sci. 72, 703–709. doi: 10.1093/gerona/glw069
Drayton, M., and Noben-Trauth, K. (2006). Mapping quantitative trait loci for hearing loss in Black Swiss mice. Hear. Res. 212, 128–139. doi: 10.1016/j.heares.2005.11.006
Dubno, J. R., Dirks, D. D., and Morgan, D. E. (1984). Effects of age and mild hearing loss on speech recognition in noise. J. Acoust. Soc. Am. 76:87. doi: 10.1121/1.391011
Dupuis, K., Pichora-Fuller, M. K., Chasteen, A. L., Marchuk, V., Singh, G., and Smith, S. L. (2015). Effects of hearing and vision impairments on the Montreal Cognitive Assessment. Aging Neuropsychol. Cogn. 22, 413–437. doi: 10.1080/13825585.2014.968084
Eckert, M. A., Cute, S., Vaden, K., Kuchinsky, S., and Dubno, J. (2012). Auditory cortex signs of age-related hearing loss. J. Assoc. Res. Otolaryngol. 13, 703–713. doi: 10.1007/s10162-012-0332-5
Engle, J. R., Gray, D. T., Turner, H., Udell, J. B., and Recanzone, G. H. (2014). Age-related neurochemical changes in the rhesus macaque inferior colliculus. Front. Aging Neurosci. 6:73. doi: 10.3389/fnagi.2014.00073
Ensrud, K. E., Ewing, S. K., Taylor, B. C., Fink, H. A., Stone, K. L., Cauley, J. A., et al. (2007). Frailty and risk of falls, fracture, and mortality in older women: the study of osteoporotic fractures. J. Gerontol. Ser. A Biol. Sci. Med. Sci. 62, 744–751.
Erway, L. C., Shiau, Y.-W., Davis, R. R., and Krieg, E. F. (1996). Genetics of age-related hearing loss in mice. III. Susceptibility of inbred and F1 hybrid strains to noise-induced hearing loss. Hear. Res 93, 181–187. doi: 10.1016/0378-5955(95)00226-X
Fetoni, A. R., Picciotti, P. M., Paludetti, G., and Troiani, D. (2011). Pathogenesis of presbycusis in animal models: a review. Exp. Gerontol. 46, 413–425. doi: 10.1016/j.exger.2010.12.003
Fischer, M. E., Schubert, C. R., Nondahl, D. M., Dalton, D. S., Huang, G.-H., Keating, B. J., et al. (2015). Subclinical atherosclerosis and increased risk of hearing impairment. Atherosclerosis 238, 344–349. doi: 10.1016/j.atherosclerosis.2014.12.031
Fransen, E., Topsakal, V., Hendrickx, J.-J., Van Laer, L., Huyghe, J. R., Van Eyken, E., et al. (2008). Occupational noise, smoking, and a high body mass index are risk factors for age-related hearing impairment and moderate alcohol consumption is protective: a european population-based multicenter study. J. Assoc. Res. Otolaryngol. 9, 264–276. doi: 10.1007/s10162-008-0123-1
Frasure-Smith, N., Lespérance, F., and Talajic, M. (1993). Depression following myocardial infarction: impact on 6-month survival. JAMA 270:1819. doi: 10.1001/jama.1993.03510150053029
Fratiglioni, L., Wang, H.-X., Ericsson, K., Maytan, M., and Winblad, B. (2000). Influence of social network on occurrence of dementia: a community-based longitudinal study. Lancet 355, 1315–1319. doi: 10.1016/S0140-6736(00)02113-9
Freret, T., Gaudreau, P., Schumann-Bard, P., Billard, J. M., and Popa-Wagner, A. (2015). Mechanisms underlying the neuroprotective effect of brain reserve against late life depression. J. Neural Transm 122(Suppl. 1), S55–S61. doi: 10.1007/s00702-013-1154-2
Fried, L. P., Tangen, C. M., Walston, J., Newman, A. B., Hirsch, C., Gottdiener, J., et al. (2001). Frailty in older adults: evidence for a phenotype. J. Gerontol. Ser. A Biol. Sci. Med. Sci. 56:M146. doi: 10.1093/gerona/56.3.M146
Friedman, R. A., Van Laer, L., Huentelman, M. J., Sheth, S. S., Van Eyken, E., Corneveaux, J. J., et al. (2009). GRM7 variants confer susceptibility to age-related hearing impairment. Hum. Mol. Genet. 18, 785–796. doi: 10.1093/hmg/ddn402
Frisina, S. T., Mapes, F., Kim, S.-H., and Frisina, R. D. (2006). Characterization of hearing loss in aged type II diabetics. Hear. Res. 211, 103–113. doi: 10.1016/j.heares.2005.09.002
Fuchs, P. A., Glowatzki, E., and Moser, T. (2003). The afferent synapse of cochlear hair cells. Curr. Opin. Neurobiol. 13, 452–458. doi: 10.1016/S0959-4388(03)00098-9
Fuente, A., and McPherson, B. (2006). Organic solvents and hearing loss: the challenge for audiology. Int. J. Audiol. 45:367. doi: 10.1080/14992020600753205
Furman, A. C., Kujawa, S. G., and Liberman, M. C. (2013). Noise-induced cochlear neuropathy is selective for fibers with low spontaneous rates. J. Neurophysiol. 110:577. doi: 10.1152/jn.00164.2013
Gallacher, J., Ilubaera, V., Ben-Shlomo, Y., Bayer, A., Fish, M., Babisch, W., et al. (2012). Auditory threshold, phonologic demand, and incident dementia. Neurology 79:1583. doi: 10.1212/WNL.0b013e31826e263d
Gao, F., Edden, R. A. E., Li, M., Puts, N. A. J., Wang, G., Liu, C., et al. (2013). Edited magnetic resonance spectroscopy detects an age-related decline in brain GABA levels. Neuroimage 78, 75–82. doi: 10.1016/j.neuroimage.2013.04.012
Gao, F., Wang, G., Ma, W., Ren, F., Li, M., Dong, Y., et al. (2015). Decreased auditory GABA + concentrations in presbycusis demonstrated by edited magnetic resonance spectroscopy. Neuroimage 106, 311–316. doi: 10.1016/j.neuroimage.2014.11.023
Gates, G. A., and Mills, J. H. (2005). Presbycusis. Lancet 366, 1111–1120. doi: 10.1016/s0140-6736(05)67423-5
Gates, G. A., Anderson, M. L., Feeney, M. P., McCurry, S. M., and Larson, E. B. (2008). Central auditory dysfunction in older persons with memory impairment or alzheimer dementia. Arch. Otolaryngol. Head Neck Surg. 134, 771–777. doi: 10.1001/archotol.134.7.771
Gates, G. A., Anderson, M. L., McCurry, S. M., Feeney, M. P., and Larson, E. B. (2011). Central auditory dysfunction as a harbinger of alzheimer dementia. (Clinical report). Arch. Otolaryngol. Head Neck Surg. 137:390. doi: 10.1001/archoto.2011.28
Gates, G. A., Beiser, A., Rees, T. S., D'Agostino, R. B., and Wolf, P. A. (2002). Central auditory dysfunction may precede the onset of clinical dementia in people with probable Alzheimer's disease. J. Am. Geriatr. Soc. 50, 482–488. doi: 10.1046/j.1532-5415.2002.50114.x
Gates, G. A., Cobb, J. L., Linn, R. T., Rees, T., Wolf, P. A., and D'Agostino, R. B. (1996). Central auditory dysfunction, cognitive dysfunction, and dementia in older people. Arch. Otolaryngol. Head Neck Surg. 122, 161–167.
Gates, G. A., Couropmitree, N. N., and Myers, R. H. (1999). Genetic associations in age-related hearing thresholds. Arch. Otolaryngol. Head Neck Surg. 125, 654–659. doi: 10.1001/archotol.125.6.654
George, E. L., Zekveld, A. A., Kramer, S. E., Goverts, S. T., Festen, J. M., and Houtgast, T. (2007). Auditory and nonauditory factors affecting speech reception in noise by older listeners. J. Acoust. Soc. Am. 121:2362. doi: 10.1121/1.2642072
Gispen, F. E., Chen, D. S., Genther, D. J., and Lin, F. R. (2014). Association between hearing impairment and lower levels of physical activity in older adults. J. Am. Geriatr. Soc. 62, 1427–1433. doi: 10.1111/jgs.12938
Golub, J. S. (2017). Brain changes associated with age-related hearing loss. Curr. Opin. Otolaryngol. Head Neck Surg. 25:347. doi: 10.1097/MOO.0000000000000387
Gopinath, B., Rochtchina, E., Wang, J. J., Schneider, J., Leeder, S. R., and Mitchell, P. (2009). Prevalence of age-related hearing loss in older adults: blue Mountains Study. Arch. Intern. Med. 169, 415–416. doi: 10.1001/archinternmed.2008.597
Gordon-Salant, S., and Fitzgibbons, P. (1993). Temporal factors and speech recognition performance in young and elderly listeners. J. Speech Hear. Res. 36, 1276–1285. doi: 10.1044/jshr.3606.1276
Gordon-Salant, S., and Fitzgibbons, P. J. (2001). Sources of age-related recognition difficulty for time-compressed speech. J. Speech Lang. Hear. Res. 44:709. doi: 10.1044/1092-4388(2001/056)
Gordon-Salant, S., Yeni-Komshian, G. H., Fitzgibbons, P. J., Cohen, J. I., and Waldroup, C. (2013). Recognition of accented and unaccented speech in different maskers by younger and older listeners. J. Acoust. Soc. Am. 134:618. doi: 10.1121/1.4807817
Gratton, M. A., and Schulte, B. A. (1995). Alterations in microvasculature are associated with atrophy of the stria vascularis in quiet-aged gerbils. Hear. Res. 82, 44–52. doi: 10.1016/0378-5955(94)00161-I
Gray, D. T., Engle, J. R., and Recanzone, G. H. (2014a). Age-related neurochemical changes in the rhesus macaque cochlear nucleus. J. Comp. Neurol. 522, 1527–1541. doi: 10.1002/cne.23479
Gray, D. T., Engle, J. R., Rudolph, M. L., and Recanzone, G. H. (2014b). Regional and age-related differences in GAD67 expression of parvalbumin- and calbindin-expressing neurons in the rhesus macaque auditory midbrain and brainstem. J. Comp. Neurol. 522, 4074–4084. doi: 10.1002/cne.23659
Gray, D. T., Rudolph, M. L., Engle, J. R., and Recanzone, G. H. (2013). Parvalbumin increases in the medial and lateral geniculate nuclei of aged rhesus macaques. Front. Aging Neurosci. 5:69. doi: 10.3389/fnagi.2013.00069
Grothe, B. (2000). The evolution of temporal processing in the medial superior olive, an auditory brainstem structure. Prog. Neurobiol. 61, 581–610. doi: 10.1016/S0301-0082(99)00068-4
Gruber, S., Pinker, K., Riederer, F., Chmelík, M., Stadlbauer, A., Bittšanský, M., et al. (2008). Metabolic changes in the normal ageing brain: consistent findings from short and long echo time proton spectroscopy. Eur. J. Radiol. 68, 320–327. doi: 10.1016/j.ejrad.2007.08.038
Gujar, K. S., Maheshwari, C. S., Björkman-Burtscher, C. I., and Sundgren, C. P. (2005). Magnetic resonance spectroscopy. J. Neuro Ophthalmol. 25, 217–226. doi: 10.1097/01.wno.0000177307.21081.81
Gurgel, R. K., Ward, P. D., Schwartz, S., Norton, M. C., Foster, N. L., and Tschanz, J. T. (2014). Relationship of Hearing loss and dementia: a prospective, population-based study. Otol. Neurotol. 35, 775–781. doi: 10.1097/MAO.0000000000000313
Harrison, J. M., and Warr, W. B. (1962). A study of the cochlear nuclei and ascending auditory pathways of the medulla. J. Comp. Neurol. 119:341.
Hedman, A. M., Van Haren, N. E. M., Schnack, H. G., Kahn, R. S., and Hulshoff Pol, H. E. (2012). Human brain changes across the life span: a review of 56 longitudinal magnetic resonance imaging studies. Hum. Brain Mapp. 33, 1987–2002. doi: 10.1002/hbm.21334.
Helzner, E. P., Cauley, J. A., Pratt, S. R., Wisniewski, S. R., Zmuda, J. M., Talbott, E. O., et al. (2005a). Race and sex differences in age-related hearing loss: the health, aging and body composition study. J. Am. Geriatr. Soc. 53, 2119–2127. doi: 10.1111/j.1532-5415.2005.00525.x
Helzner, E. P., Cauley, J. A., Pratt, S. R., Wisniewski, S. R., Talbott, E. O., Zmuda, J. M., et al. (2005b). Hearing sensitivity and bone mineral density in older adults: the health, aging and body composition study. Osteoporosis Int. 16, 1675–1682. doi: 10.1007/s00198-005-1902-8
Helzner, E., Patel, A., Pratt, S., Sutton-Tyrrell, K., Cauley, J., Talbott, E., et al. (2011). Hearing sensitivity in older adults: associations with cardiovascular risk factors in the health, aging and body composition study. (report). J. Am. Geriatr. Soc. 59:972. doi: 10.1111/j.1532-5415.2011.03444.x
Henderson, D., Bielefeld, E. C., Harris, K. C., and Hu, B. H. (2006). The role of oxidative stress in noise-induced hearing loss. Ear Hear. 27, 1–19. doi: 10.1097/01.aud.0000191942.36672.f3
Henson, R. (2005). What can functional neuroimaging tell the experimental psychologist? Q. J. Exp. Psychol. Sect. A 58, 193–233. doi: 10.1080/02724980443000502
Hinojosa, R., and Nelson, E. G. (2011). Cochlear nucleus neuron analysis in individuals with presbycusis. Laryngoscope 121, 2641–2648. doi: 10.1002/lary.22383
Humes, L. E., and Dubno, J. R. (2010). “Factors affecting speech understanding in older adults,” in The Aging Auditory System: Perceptual Characterization and Neural Bases of Presbycusis, eds S. Gordon-Salant, D. R. Frisina, A. N. Popper, and R. R. Fay (New York, NY: Springer-Verlag), 211–258.
Humes, L. E., Busey, T. A., Craig, J., and Kewley-Port, D. (2013). Are age-related changes in cognitive function driven by age-related changes in sensory processing? Attent. Percept. Psychophys. 75, 508–524. doi: 10.3758/s13414-012-0406-9
Humes, L. E., Kewley-Port, D., Fogerty, D., and Kinney, D. (2010). Measures of hearing threshold and temporal processing across the adult lifespan. Hear. Res. 264, 30–40. doi: 10.1016/j.heares.2009.09.010
Husain, F. T., Medina, R. E., Davis, C. W., Szymko-Bennett, Y., Simonyan, K., Pajor, N. M., et al. (2011). Neuroanatomical changes due to hearing loss and chronic tinnitus: a combined VBM and DTI study. Brain Res. 1369, 74–88. doi: 10.1016/j.brainres.2010.10.095
Idrizbegovic, E., Bogdanovic, N., Willott, J. F., and Canlon, B. (2004). Age-related increases in calcium-binding protein immunoreactivity in the cochlear nucleus of hearing impaired C57BL/6J mice. Neurobiol. Aging 25, 1085–1093. doi: 10.1016/j.neurobiolaging.2003.11.004
Iliadou, V., Kaprinis, S., Iliadou, V., and Kaprinis, S. (2003). Clinical psychoacoustics in Alzheimer's disease central auditory processing disorders and speech deterioration. Ann. Gen. Hosp. Psychiatry 2:12. doi: 10.1186/1475-2832-2-12
Jayakody, D. M. P., Almeida, O. P., Speelman, C. P., Bennett, R. J., Moyle, T. C., Yiannos, J. M., et al. (2018b). Association between speech and high-frequency hearing loss and depression, anxiety and stress in older adults. Maturitas 110, 86–91. doi: 10.1016/j.maturitas.2018.02.002
Jayakody, D. M. P., Friedland, P. L., Eikelboom, R. H., Martins, R. N., and Sohrabi, H. R. (2018a). A novel study on association between untreated hearing loss and cognitive functions of older adults: baseline non-verbal cognitive assessment results. Clin. Otolaryngol. 43, 182–191. doi: 10.1111/coa.12937
Jayakody, D. M. P., Friedland, P. L., Nel, E., Martins, R. N., Atlas, M. D., and Sohrabi, H. R. (2017). Impact of cochlear implantation on cognitive functions of older adults: pilot test results. Otol. Neurotol. 38, e289–e295. doi: 10.1097/mao.0000000000001502
Jerger, J., Jerger, S., and Pirozzolo, F. (1991). Correlational analysis of speech audiometric scores, hearing loss, age, and cognitive abilities in the elderly. Ear Hear. 12, 103–109.
Johnson, K. R., and Zheng, Q. Y. (2002). Ahl2, a second locus affecting age-related hearing loss in mice. Genomics 80:461. doi: 10.1006/geno.2002.6858
Johnson, K. R., Erway, L. C., Cook, S. A., Willott, J. F., and Zheng, Q. Y. (1997). A major gene affecting age-related hearing loss in C57BL/6J mice. Hear. Res. 114, 83–92. doi: 10.1016/S0378-5955(97)00155-X
Johnson, K. R., Longo-Guess, C., Gagnon, L. H., Yu, H., and Zheng, Q. Y. (2008). A locus on distal chromosome 11 (ahl8) and its interaction with Cdh23 ahl underlie the early onset, age-related hearing loss of DBA/2J mice. Genomics 92, 219–225. doi: 10.1016/j.ygeno.2008.06.007
Johnson, K. R., Zheng, Q. Y., and Noben-Trauth, K. (2006). Strain background effects and genetic modifiers of hearing in mice. Brain Res. 1091, 79–88. doi: 10.1016/j.brainres.2006.02.021
Jorgensen, L. (2012). The Potential Impact of Undiagnosed Hearing Loss on the Diagnosis of Dementia. Doctor of Philosophy PhD thesis, University of Pittsburgh, Pittsburgh, PA, Pennsylvania.
Juarez-Salinas, D. L., Engle, J. R., Navarro, X. O., and Recanzone, G. H. (2010). Hierarchical and serial processing in the spatial auditory cortical pathway is degraded by natural aging. J. Neurosci. 30:14795. doi: 10.1523/JNEUROSCI.3393-10.2010
Kamil, R. J., Betz, J., Powers, B. B., Pratt, S., Kritchevsky, S., Ayonayon, H. N., et al. (2016). Association of hearing impairment with incident frailty and falls in older adults. J. Aging Health 28, 644–660. doi: 10.1177/0898264315608730
Karlsson, K. K., Harris, J. R., and Svartengren, M. (1997). Description and primary results from an audiometric study of male twins. Ear Hear. 18:114. doi: 10.1097/00003446-199704000-00003
Katz, J. (2015). Handbook of Clinical Audiology, 7th Edn. Philadelphia, PA: Wolters Kluwer Health; Lippincott Williams & Wilkins.
Keithley, E. M., Canto, C., Zheng, Q. Y., Fischel-Ghodsian, N., and Johnson, K. R. (2004). Age-related hearing loss and the ahl locus in mice. Hear. Res. 188, 21–28. doi: 10.1016/S0378-5955(03)00365-4.
Kemper, S., Herman, R. E., and Lian, C. H. T. (2003). The costs of doing two things at once for young and older adults: talking while walking, finger tapping, and ignoring speech or noise. Psychol. Aging 18, 181–192. doi: 10.1037/0882-7974.18.2.181
Kim, S., Frisina, R. D., Mapes, F. M., Hickman, E. D., and Frisina, D. R. (2006). Effect of age on binaural speech intelligibility in normal hearing adults. Speech Commun. 48, 591–597. doi: 10.1016/j.specom.2005.09.004
Kirov, I. I., Fleysher, L., Fleysher, R., Patil, V., Liu, S., and Gonen, O. (2008). Age dependence of regional proton metabolites T 2 relaxation times in the human brain at 3 T. Magn. Reson. Med. 60, 790–795. doi: 10.1002/mrm.21715
Koegel, L. (1985). Ototoxicity: a contemporary review of aminoglycosides, loop diuretics, acetylsalicylic acid, quinine, erythromycin, and cisplatinum. Am. J. Otol. 6:190.
Kujawa, S. G., and Liberman, M. C. (2006). Acceleration of age-related hearing loss by early noise exposure: evidence of a misspent youth. J. Neurosci. 26, 2115–2123. doi: 10.1523/JNEUROSCI.4985-05.2006
Kujawa, S. G., and Liberman, M. C. (2009). Adding insult to injury: cochlear nerve degeneration after “temporary” noise-induced hearing loss. J. Neurosci. 29, 14077–14085. doi: 10.1523/JNEUROSCI.2845-09.2009
Kwong, D. L., Wei, W. I., Sham, J. S. T., Ho, W. K., Yuen, P. W., Chua, D. T. T., et al. (1996). Sensorineural hearing loss in patients treated for nasopharyngeal carcinoma: a prospective study of the effect of radiation and cisplatin treatment. Int. J. Radiat. Oncol. Biol. Phys. 36, 281–289. doi: 10.1016/S0360-3016(96)00302-1
Lee, F.-S., Matthews, L. J., Dubno, J. R., and Mills, J. H. (2005). Longitudinal study of pure-tone thresholds in older persons. Ear Hear. 26, 1–11.
Lee, H. J., Wallani, T., and Mendelson, J. R. (2002). Temporal processing speed in the inferior colliculus of young and aged rats. Hear. Res. 174, 64–74. doi: 10.1016/S0378-5955(02)00639-1
Lee, J., Dhar, S., Abel, R., Banakis, R., Grolley, E., Lee, J., et al. (2012). Behavioral hearing thresholds between 0.125 and 20 kHz using depth-compensated ear simulator calibration. Ear Hear. 33:315. doi: 10.1097/AUD.0b013e31823d7917
Lee, R. S., Hermens, D. F., Porter, M. A., and Redoblado-Hodge, M. A. (2011). A meta-analysis of cognitive deficits in first-episode Major Depressive Disorder. J. Affect. Disord. 140, 113–124. doi: 10.1016/j.jad.2011.10.023
Lemaitre, H., Goldman, A. L., Sambataro, F., Verchinski, B. A., Meyer-Lindenberg, A., Weinberger, D. R., et al. (2012). Normal age-related brain morphometric changes: nonuniformity across cortical thickness, surface area and gray matter volume? Neurobiol. Aging 33, 617.e1-617.e9. doi: 10.1016/j.neurobiolaging.2010.07.013
Li, L., Simonsick, E. M., Ferrucci, L., and Lin, F. R. (2013). Hearing loss and gait speed among older adults in the United States. Gait Posture 38, 25–29. doi: 10.1016/j.gaitpost.2012.10.006
Lin, F., Maas, P., Chien, W., Carey, J., Ferrucci, L., and Thorpe, R. (2012). Association of skin color, race/ethnicity, and hearing loss among adults in the USA. J. Assoc. Res. Otolaryngol. 13, 109–117. doi: 10.1007/s10162-011-0298-8
Lin, F. R., and Ferrucci, L. (2012). Hearing loss and falls among older adults in the United States. (Research Letters)(Letter to the editor). Arch. Intern. Med. 172:369. doi: 10.1001/archinternmed.2011.728
Lin, F. R., Ferrucci, L., An, Y., Goh, J. O., Doshi, J., Metter, E. J., et al. (2014). Association of hearing impairment with brain volume changes in older adults. Neuroimage 90, 84–92. doi: 10.1016/j.neuroimage.2013.12.059
Lin, F. R., Metter, E. J., O'Brien, R. J., Resnick, S. M., Zonderman, A. B., and Ferrucci, L. (2011a). Hearing loss and incident dementia. Arch. Neurol. 68, 214–220. doi: 10.1001/archneurol.2010.362
Lin, F. R., Thorpe, R., Gordon-Salant, S., and Ferrucci, L. (2011b). Hearing loss prevalence and risk factors among older adults in the United States. J. Gerontol. Ser. A Biomed. Sci. Med. Sci. 66A, 582–590. doi: 10.1093/gerona/glr002
Lin, F. R., Yaffe, K., Xia, J., Xue, Q. L., Harris, T. B., Purchase-Helzner, E., et al. (2013). Hearing loss and cognitive decline in older adults. JAMA Intern. Med. 173, 293–299. doi: 10.1001/jamainternmed.2013.1868
Lin, V. Y., Chung, J., Callahan, B. L., Smith, L., Gritters, N., Chen, J. M., et al. (2017). Development of cognitive screening test for the severely hearing impaired: hearing-impaired MoCA. Laryngoscope 127, S4–S11. doi: 10.1002/lary.26590
Lin, Y., Wang, J., Wu, C., Wai, Y., Yu, J., and Ng, S. (2008). Diffusion tensor imaging of the auditory pathway in sensorineural hearing loss: changes in radial diffusivity and diffusion anisotropy. J. Magn. Reson. Imaging 28, 598–603. doi: 10.1002/jmri.21464
Lin, Y.-H., Wu, C.-C., Hsu, C.-J., Hwang, J.-H., and Liu, T.-C. (2011). The grainyhead-like 2 gene (GRHL2) single nucleotide polymorphism is not associated with age-related hearing impairment in Han Chinese. Laryngoscope 121:1303. doi: 10.1002/lary.21771
Lindenberger, U., and Baltes, P. B. (1994). Sensory functioning and intelligence in old age: a strong connection. Psychol. Aging 9, 339–355.
Lindenberger, U., and Ghisletta, P. (2009). Cognitive and sensory declines in old age: gauging the evidence for a common cause. Psychol. Aging 24, 1–16. doi: 10.1037/a0014986
Ling, L. L., Hughes, L. F., and Caspary, D. M. (2005). Age-related loss of the GABA synthetic enzyme glutamic acid decarboxylase in rat primary auditory cortex. Neuroscience 132, 1103–1113. doi: 10.1016/j.neuroscience.2004.12.043
Livingston, G., Sommerlad, A., Orgeta, V., Costafreda, S. G., Huntley, J., Ames, D., et al. (2017). Dementia prevention, intervention, and care. Lancet 390, 2673–2734. doi: 10.1016/S0140-6736(17)31363-6
Maes, M., Yirmyia, R., Noraberg, J., Brene, S., Hibbeln, J., Perini, G., et al. (2009). The inflammatory & neurodegenerative (I&ND) hypothesis of depression: leads for future research and new drug developments in depression. Metab. Brain Dis. 24, 27–53. doi: 10.1007/s11011-008-9118-1
Makhani, A., Akbaryan, F., and Cernak, I. (2015). Cognitive performance improvement in Canadian Armed Forces personnel during deployment. J. Milit. Vet. Fam. Health 1, 59–67. doi: 10.3138/jmvfh.2014-04
Manwaring, N., Jones, M. M., Wang, J. J., Rochtchina, E., Howard, C., Newall, P., et al. (2007). Mitochondrial DNA Haplogroups and age-related hearing loss. Arch. Otolaryngol. Head Neck Surg. 133, 929–933. doi: 10.1001/archotol.133.9.929
Masterton, R. B. (1992). Role of the central auditory system in hearing: the new direction. Trends Neurosci. 15, 280–285. doi: 10.1016/0166-2236(92)90077-L
Matthews, L., Lee, F.-S., Mills, J., and Dubno, J. (1997). Extended high-frequency thresholds in older adults. J. Speech Lang. Hear. Res. 40, 208–214. doi: 10.1044/jslhr.4001.208
Mattson, M. P. (2007). Calcium and neurodegeneration. Aging Cell 6, 337–350. doi: 10.1111/j.1474-9726.2007.00275.x
May, B. J. (2000). Role of the dorsal cochlear nucleus in the sound localization behavior of cats. Hear. Res. 148, 74–87. doi: 10.1016/S0378-5955(00)00142-8
McIntyre, R. S., Soczynska, J. K., Konarski, J. Z., Woldeyohannes, H. O., Law, C. W. Y., Miranda, A., et al. (2007). Should depressive syndromes be reclassified as “metabolic syndrome type II”? Ann. Clin. Psychiatry 19:257. doi: 10.3109/10401230701653377
Mendelson, J. R., and Ricketts, C. (2001). Age-related temporal processing speed deterioration in auditory cortex. Hear. Res. 158, 84–94. doi: 10.1016/S0378-5955(01)00294-5
Mezuk, B., Edwards, L., Lohman, M., Choi, M., and Lapane, K. (2012). Depression and Frailty in Later Life: A synthetic Review, Vol. 27. Chichester: John Wiley & Sons, Ltd, 879–892.
Mick, P. T., and Lin, F. R. (2013). Social isolation and hearing loss in the elderly. Otolaryngol. Head Neck Surg. 149(2 Suppl.), P110. doi: 10.1177/0194599813495815a228
Mills, J. H., Konrad-Martin, D., Leek, M., and Hood, L. J. (2006a). Roundtable discussion: pathophysiology of the aging auditory system. Semin. Hear. 27, 237–242. doi: 10.1055/s-2006-954850
Mills, J. H., Schmiedt, R. A., Schulte, B. A., and Dubno, J. R. (2006b). Age-related hearing loss: a loss of voltage, not hair cells. Semin. Hear. 27, 228–236. doi: 10.1055/s-2006-954849
Mitchell, P., Gopinath, B., McMahon, C. M., Rochtchina, E., Wang, J. J., Boyages, S. C., et al. (2009). Relationship of Type 2 diabetes to the prevalence, incidence and progression of age-related hearing loss. Diabet. Med. 26, 483–488. doi: 10.1111/j.1464-5491.2009.02710.x
Morata, T. C., Johnson, A. C., Nylen, P., Svensson, E. B., Cheng, J., Krieg, E. F., et al. (2002). Audiometric findings in workers exposed to low levels of styrene and noise. J. Occup. Environ. Med. 44, 806–814.
Moser, T., Predoehl, F., and Starr, A. (2013). Review of hair cell synapse defects in sensorineural hearing impairment. Otol. Neurotol. 34, 995–1004. doi: 10.1097/MAO.0b013e3182814d4a
Newman, D. L., Fisher, L. M., Ohmen, J., Parody, R., Fong, C.-T., Frisina, S. T., et al. (2012). GRM7 variants associated with age-related hearing loss based on auditory perception. Hear. Res. 294, 125–132. doi: 10.1016/j.heares.2012.08.016
Nomiya, R., Nomiya, S., Kariya, S., Okano, M., Morita, N., Cureoglu, S., et al. (2008). Generalized arteriosclerosis and changes of the cochlea in young adults. Otol. Neurotol. 29, 1193–1197. doi: 10.1097/MAO.0b013e31818a0906
Nomura, K., Nakao, M., and Morimoto, T. (2005). Effect of smoking on hearing loss: quality assessment and meta-analysis. Prev. Med. 40, 138–144. doi: 10.1016/j.ypmed.2004.05.011
Norton, S., Matthews, F. E., Barnes, D. E., Yaffe, K., and Brayne, C. (2014). Potential for primary prevention of Alzheimer's disease: an analysis of population-based data. Lancet Neurol. 13, 788–794. doi: 10.1016/S1474-4422(14)70136-X
O'Grady, G., Boyles, A. L., Speer, M., Deruyter, F., Strittmatter, W., and Worley, G. (2007). Apolipoprotein E alleles and sensorineural hearing loss. Int. J. Audiol. 46:183. doi: 10.1080/14992020601145294
Ohlemiller, K. K. (2006). Contributions of mouse models to understanding of age- and noise-related hearing loss. Brain Res. 1091, 89–102. doi: 10.1016/j.brainres.2006.03.017
Ohlemiller, K. K. (2009). Mechanisms and genes in human strial presbycusis from animal models. Brain Res. 1277, 70–83. doi: 10.1016/j.brainres.2009.02.079
Ohlemiller, K. K., and Gagnon, P. M. (2004). Cellular correlates of progressive hearing loss in 129S6/SvEv mice. J. Comparat. Neurol. 469, 377–390. doi: 10.1002/cne.11011
Oliver, D. L. (2000). Ascending efferent projections of the superior olivary complex. Microsc. Res. Tech. 51, 355–363. doi: 10.1002/1097-0029(20001115)51:4<355::AID-JEMT5>3.0.CO;2-J
Oonk, A. M. M., Leijendeckers, J. M., Lammers, E. M., Weegerink, N. J. D., Oostrik, J., Beynon, A. J., et al. (2013). Progressive hereditary hearing impairment caused by aMYO6 mutation resembles presbyacusis. Hear. Res. 299, 88–98. doi: 10.1016/j.heares.2012.12.015
Ouda, L., and Syka, J. (2012). Immunocytochemical profiles of inferior colliculus neurons in the rat and their changes with aging. Front. Neural Circuits 6:68. doi: 10.3389/fncir.2012.00068
Ouda, L., Burianova, J., and Syka, J. (2012). Age-related changes in calbindin and calretinin immunoreactivity in the central auditory system of the rat. Exp. Gerontol. 47, 497–506. doi: 10.1016/j.exger.2012.04.003
Ouda, L., Profant, O., and Syka, J. (2015). Age-related changes in the central auditory system. Cell Tissue Res. 361, 337–358. doi: 10.1007/s00441-014-2107-2
Ouellet, L., and de Villers-Sidani, E. (2014). Trajectory of the main GABAergic interneuron populations from early development to old age in the rat primary auditory cortex. Front. Neuroanat. 8:40. doi: 10.3389/fnana.2014.00040
Owen, A. M., Evans, A. C., and Petrides, M. (1996). Evidence for a two-stage model of spatial working memory processing within the lateral frontal cortex: a positron emission tomography study. Cereb. Cortex 6, 31–38.
Owen, A. M., Sahakian, B. J., Semple, J., Polkey, C. E., and Robbins, T. W. (1995). Visuo-spatial short-term recognition memory and learning after temporal lobe excisions, frontal lobe excisions or amygdalo-hippocampectomy in man. Neuropsychologia 33, 1–24.
Panza, F., Frisardi, V., Capurso, C., D'Introno, A., Colacicco, A. M., Imbimbo, B. P., et al. (2010). Late-life depression, mild cognitive impairment, and dementia: possible continuum? Am. J. Geriatr. Psychiatry 18, 98–116. doi: 10.1097/JGP.0b013e3181b0fa13
Panza, F., Solfrizzi, V., Seripa, D., Imbimbo, B. P., Capozzo, R., Quaranta, N., et al. (2015). Age-related hearing impairment and frailty in Alzheimer's disease: interconnected associations and mechanisms. Front. Aging Neurosci. 7:113. doi: 10.3389/fnagi.2015.00113
Pearson, J. D., Morrell, C. H., Gordon-Salant, S., Brant, L. J., Metter, E. J., Klein, L. L., et al. (1995). Gender differences in a longitudinal study of age-associated hearing loss. J. Acoust. Soc. Am. 97, 1196–1205.
Peelle, J. E., Johnsrude, I., and Davis, M. H. (2010). Commentary: hierarchical processing for speech in human auditory cortex and beyond. Front. Hum. Neurosci. 4:51. doi: 10.3389/fnhum.2010.00051
Peelle, J. E., Troiani, V., Grossman, M., and Wingfield, A. (2011). Hearing loss in older adults affects neural systems supporting speech comprehension. J. Neurosci. 31, 12638–12643. doi: 10.1523/JNEUROSCI.2559-11.2011
Pichora-fuller, M. K. (2003). Cognitive aging and auditory information processing. Int. J. Audiol. 42, 26–32. doi: 10.3109/14992020309074641
Pichora-Fuller, M. K., and Singh, G. (2006). Effects of age on auditory and cognitive processing: implications for hearing aid fitting and audiologic rehabilitation. Trends Amplif. 10:29. doi: 10.1177/108471380601000103
Pichora-Fuller, M. K., and Souza, P. E. (2003). Effects of aging on auditory processing of speech. Int. J. Audiol. 42, 11–16. doi: 10.3109/14992020309074638
Pichora-Fuller, M. K., Schneider, B. A., and Daneman, M. (1995). How young and old adults listen to and remember speech in noise. J. Acoust. Soc. Am. 97, 593–608. doi: 10.1121/1.412282
Plack, C. J., Barker, D., and Prendergast, G. (2014). Perceptual consequences of “Hidden” hearing loss. Trends Hear. 18:2331216514550621. doi: 10.1177/2331216514550621
Popa-Wagner, A., Buga, A. M., Tica, A. A., and Albu, C. V. (2014). Perfusion deficits, inflammation and aging precipitate depressive behaviour. Biogerontology 15, 439–448. doi: 10.1007/s10522-014-9516-1
Popelka, M. M., Cruickshanks, K. J., Wiley, T. L., Tweed, T. S., Klein, B. E., Klein, R., et al. (2000). Moderate alcohol consumption and hearing loss: a protective effect. J. Am. Geriatr. Soc. 48, 1273–1278. doi: 10.1111/j.1532-5415.2000.tb02601.x
Profant, O., Balogová, Z., Dezortová, M., Wagnerová, D., Hájek, M., and Syka, J. (2013). Metabolic changes in the auditory cortex in presbycusis demonstrated by MR spectroscopy. Exp. Gerontol. 48, 795–800. doi: 10.1016/j.exger.2013.04.012
Profant, O., Tintěra, J., Balogová, Z., Ibrahim, I., Jilek, M., and Syka, J. (2015). Functional changes in the human auditory cortex in ageing. PLoS ONE 10:e0116692. doi: 10.1371/journal.pone.0116692
Purser, J. L., Kuchibhatla, M. N., Fillenbaum, G. G., Harding, T., Peterson, E. D., and Alexander, K. P. (2006). Identifying frailty in hospitalized older adults with significant coronary artery disease. J. Am. Geriatr. Soc. 54, 1674–1681. doi: 10.1111/j.1532-5415.2006.00914.x
Ramage-Morin, P. L. (2016). Hearing difficulties and feelings of social isolation among Canadians aged 45 or older. Health Rep. 27, 3–12.
Raz, N., Gunning, F. M., Head, D., Dupuis, J. H., McQuain, J., Briggs, S. D., et al. (1997). Selective aging of the human cerebral cortex observed in vivo: differential vulnerability of the prefrontal gray matter. Cereb. Cortex 7, 268–282.
Raz, N., Rodrigue, K. M., Head, D., Kennedy, K. M., and Acker, J. D. (2004). Differential aging of the medial temporal lobe: a study of a five-year change. Neurology 62, 433–438. doi: 10.1212/01.WNL.0000106466.09835.46
Raz, N., Rodrigue, K. M., Kennedy, K. M., and Acker, J. D. (2007). Vascular Health and longitudinal changes in brain and cognition in middle-aged and older adults. Neuropsychology, 21, 149–157. doi: 10.1037/0894-4105.21.2.149
Reyngoudt, H., Claeys, T., Vlerick, L., Verleden, S., Acou, M., Deblaere, K., et al. (2012). Age-related differences in metabolites in the posterior cingulate cortex and hippocampus of normal ageing brain: a 1H-MRS study. Eur. J. Radiol. 81, e223–e231. doi: 10.1016/j.ejrad.2011.01.106
Rhode, W. S., and Greenberg, S. (1994). Encoding of amplitude modulation in the cochlear nucleus of the cat. J. Neurophysiol. 71:1797. doi: 10.1152/jn.1994.71.5.1797
Richardson, B. D., Ling, L. L., Uteshev, V. V., and Caspary, D. M. (2013). Reduced GABA(A) receptor-mediated tonic inhibition in aged rat auditory thalamus. J. Neurosci. 33:1218. doi: 10.1523/JNEUROSCI.3277-12.2013
Robertson, D. A., Savva, G. M., Kenny, R. A., Robertson, D. A., Savva, G. M., and Kenny, R. A. (2013). Frailty and cognitive impairment—A review of the evidence and causal mechanisms. Ageing Res. Rev. 12, 840–851. doi: 10.1016/j.arr.2013.06.004
Robertson, D., and Irvine, D. R. F. (1989). Plasticity of frequency organization in auditory cortex of guinea pigs with partial unilateral deafness. J. Comp. Neurol. 282, 456–471. doi: 10.1002/cne.902820311
Rockwood, K., Stolee, P., and McDowell, I. (1996). Factors associated with institutionalization of older people in Canada: testing a multifactorial definition of frailty. J. Am. Geriatr. Soc. 44, 578–582.
Sakamoto, M., Sugasawa, M., Kaga, K., and Kamio, T. (1998). Average thresholds in the 8 to 20 kHz range as a function of age. Scand. Audiol. 27:189.
Salt, A. N., Melichar, I., and Thalmann, R. (1987). Mechanisms of endocochlear potential generation by stria vascularis. Laryngoscope 97(8 Pt 1), 984–991.
Samper-Ternent, R., Al Snih, S., Raji, M. A., Markides, K. S., and Ottenbacher, K. J. (2008). Relationship between frailty and cognitive decline in older Mexican Americans. J. Am. Geriatr. Soc. 56, 1845–1852. doi: 10.1111/j.1532-5415.2008.01947.x
Scahill, R. I., Frost, C., Jenkins, R., Whitwell, J. L., Rossor, M. N., and Fox, N. C. (2003). A longitudinal study of brain volume changes in normal aging using serial registered magnetic resonance imaging. Arch. Neurol. 60:989. doi: 10.1001/archneur.60.7.989
Scarmeas, N., Levy, G., Tang, M. X., Manly, J., and Stern, Y. (2001). Influence of leisure activity on the incidence of Alzheimer's Disease. Neurology 57, 2236–2242. doi: 10.1212/WNL.57.12.2236
Schatteman, T. A., Hughes, L. F., and Caspary, D. M. (2008). Aged-related loss of temporal processing: altered responses to amplitude modulated tones in rat dorsal cochlear nucleus. Neuroscience 154, 329–337. doi: 10.1016/j.neuroscience.2008.02.025
Schmiedt, R. A. (2010). “The physiology of the cochlear presbycusis,” in Aging Auditory System, eds S. Gordon-Salant, D. R. Frisina, A. N. Popper and R. R. Fay (New York, NY: Springer).
Schmiedt, R. A., Lang, H., Okamura, H.-O., and Schulte, B. A. (2002). Effects of furosemide applied chronically to the round window: a model of metabolic presbyacusis. J. Neurosci. 22, 9643–9650.
Schneider, B. A., and Pichora-Fuller, M. K. (2000). “Implications of perceptual deterioration for cognitive aging research,” in The Handbook of Aging and Cognition, 2nd Edn., (Mahwah, NJ: Lawrence Erlbaum Associates Publishers), 155–219.
Schuknecht, H. F., and Gacek, M. R. (1993). Cochlear pathology in presbycusis. Ann. Otol. Rhinol. Laryngol. 102(1 Pt 2), 1–16.
Seidman, M. D., Ahmad, N., and Bai, U. (2002). Molecular mechanisms of age-related hearing loss. Ageing Res. Rev. 1, 331–343. doi: 10.1016/S1568-1637(02)00004-1
Sha, S.-H., Kanicki, A., Dootz, G., Talaska, A. E., Halsey, K., Dolan, D., et al. (2008). Age-related auditory pathology in the CBA/J mouse. Hear. Res. 243, 87–94. doi: 10.1016/j.heares.2008.06.001
Silver, N. C., Barker, G. J., MacManus, D. G., Tofts, P. S., and Miller, D. H. (1997). Magnetisation transfer ratio of normal brain white matter: a normative database spanning four decades of life. J. Neurol. Neurosurg. Psychiatry 62:223.
Sliwinska-Kowalska, M., Zamyslowska-Szmytke, E., Szymczak, W., Kotylo, P., Fiszer, M., Wesolowski, W., et al. (2004). Effects of coexposure to noise and mixture of organic solvents on hearing in dockyard workers. J. Occup. Environ. Med. 46, 30–38. doi: 10.1097/01.jom.0000105912.29242.5b
Solfrizzi, V., Scafato, E., Frisardi, V., Seripa, D., Logroscino, G., Maggi, S., et al. (2013). Frailty syndrome and the risk of vascular dementia: the Italian longitudinal study on aging. Alzheimer'sDement. 9, 113–122. doi: 10.1016/j.jalz.2011.09.223
Someya, S., and Prolla, T. A. (2010). Mitochondrial oxidative damage and apoptosis in age-related hearing loss. Mech. Ageing Dev. 131, 480–486. doi: 10.1016/j.mad.2010.04.006
Sonnet, M.-H., Montaut-Verient, B., Niemier, J.-Y., Hoen, M., Ribeyre, L., and Parietti-Winkler, C. (2017). Cognitive abilities and quality of life after cochlear implantation in the elderly. Otol. Neurotol. 38, e296–e301. doi: 10.1097/MAO.0000000000001503
Speechley, M., and Tinetti, M. (1991). Falls and injuries in frail and vigorous community elderly persons. J. Am. Geriatr. Soc. 39, 46–52.
Staff, R. T., Murray, A. D., Deary, I. J., and Whalley, L. J. (2004). What provides cerebral reserve? Brain 127, 1191–1199. doi: 10.1093/brain/awh144
Stamataki, S., Francis, H. W., Lehar, M., May, B. J., and Ryugo, D. K. (2006). Synaptic alterations at inner hair cells precede spiral ganglion cell loss in aging C57BL/6J mice. Hear. Res. 221, 104–118. doi: 10.1016/j.heares.2006.07.014
Stern, Y. (2002). What is cognitive reserve? Theory and research application of the reserve concept. J. Int. Neuropsychol. Soc. 8, 448–460. doi: 10.1017/S1355617702813248
Stewart, R., and Wingfield, A. (2009). Hearing loss and cognitive effort in older adults' report accuracy for verbal materials. J. Am. Acad. Audiol. 20, 147–154.
Sugiura, S., Uchida, Y., Nakashima, T., Ando, F., and Shimokata, H. (2010). The association between gene polymorphisms in uncoupling proteins and hearing impairment in Japanese elderly. Acta Otolaryngol. 130:487. doi: 10.3109/00016480903283758
Šuta, D., Rybalko, N., Pelánová, J., Popelár, J., and Syka, J. (2011). Age-related changes in auditory temporal processing in the rat. Exp. Gerontol. 46, 739–746. doi: 10.1016/j.exger.2011.05.004
Sweeney, J. A., Kmiec, J. A., and Kupfer, D. J. (2000). Neuropsychologic impairments in bipolar and unipolar mood disorders on the CANTAB neurocognitive battery. Biol. Psychiatry 48, 674–684. doi: 10.1016/S0006-3223(00)00910-0
Tabuchi, K., Nishimura, B., Nakamagoe, M., Hayashi, K., Nakayama, M., and Hara, A. (2011). Ototoxicity: mechanisms of cochlear impairment and its prevention. Curr. Med. Chem. 18, 4866–4871. doi: 10.2174/092986711797535254
Taljaard, D. S., Olaithe, M., Brennan-Jones, C. G., Eikelboom, R. H., and Bucks, R. S. (2015). The relationship between hearing impairment and cognitive function: a meta-analysis in adults. Clin. Otolaryngol. 41, 718–729. doi: 10.1111/coa.12607
Tay, T., Kifley, A., Lindley, R., Landau, P., Ingham, N., Mitchell, P., et al. (2006). Are sensory and cognitive declines associated in older persons seeking aged care services? Findings from a pilot study. Ann. Acad. Med. 35, 254–259.
Thompson, A. M., and Schofield, B. R. (2000). Afferent projections of the superior olivary complex. Microsc. Res. Tech. 51, 330–354. doi: 10.1002/1097-0029(20001115)51:4<330::AID-JEMT4>3.0.CO2-X
Tremblay, K. L., and Burkhard, R. F. (2012). Hearing Across the Lifespan - Assessment and Disorders. San Diego, CA: Plural Publishing, Inc.
Tun, P. A., Benichov, J., and Wingfield, A. (2010). Response latencies in auditory sentence comprehension: effects of linguistic versus perceptual challenge. Psychol. Aging 25:730. doi: 10.1037/a0019300
Turner, J. G. (2005a). Affects of aging on receptive fields in rat primary auditory cortex layer V neurons. J. Neurophysiol. 94, 2738–2747. doi: 10.1152/jn.00362.2005
Uchida, Y., Sugiura, S., Nakashima, T., Ando, F., and Shimokata, H. (2009). Endothelin-1 gene polymorphism and hearing impairment in elderly Japanese. Laryngoscope 119, 938–943. doi: 10.1002/lary.20181
Ünal, M., Tamer, L., Dogruer, Z. N., and Yildirim, H., Vayisoglu, Y., Çamdeviren, H. (2005). N-Acetyltransferase 2 gene polymorphism and presbycusis. Laryngoscope 115, 2238–2241. doi: 10.1097/01.mlg.0000183694.10583.12
Valentijn, S. A., Van Boxtel, M. P., Van Hooren, S. A., Bosma, H., Beckers, H. J., Ponds, R. W. H. M., et al. (2005). Change in sensory functioning predicts change in cognitive functioning: results from a 6-year follow-up in the maastricht aging study. J. Am. Geriatr. Soc. 53, 374–380. doi: 10.1111/j.1532-5415.2005.53152.x
Van Eyken, E., Van Camp, G., Fransen, E., Topsakal, V., Hendrickx, J. J., Demeester, K., et al. (2007b). Contribution of the N-acetyltransferase 2 polymorphism NAT2*6A to age-related hearing impairment. J. Med. Genet. 44:570. doi: 10.1136/jmg.2007.049205
Van Eyken, E., Van Camp, G., and Van Laer, L. (2007a). The complexity of age-related hearing impairment: contributing environmental and genetic factors. Audiol. Neurotol. 12, 345–358. doi: 10.1159/000106478
Van Eyken, E., Van Laer, L., Fransen, E., Topsakal, V., Hendrickx, J.-J., Demeester, K., et al. (2007c). The contribution of GJB2 (Connexin 26) 35delG to age-related hearing impairment and noise-induced hearing loss. Otol. Neurotol. 28, 970–975. doi: 10.197/MAO.0b013e3180dca1b9
Van Eyken, E., Van Laer, L., Fransen, E., Topsakal, V., Lemkens, N., Laureys, W., et al. (2006). KCNQ4: a gene for age-related hearing impairment? Hum. Mutat. 27, 1007–1016. doi: 10.1002/humu.20375
Vernon, M. (2005). Fifty years of research on the intelligence of deaf and hard-of-hearing children: a review of literature and discussion of implications. J. Deaf Stud. Deaf Educ. 10, 225–231. doi: 10.1093/deafed/eni024
Wang, Y., and Manis, P. B. (2005). Synaptic transmission at the cochlear nucleus endbulb synapse during age-related hearing loss in mice. J. Neurophysiol. 94:1814. doi: 10.1152/jn.00374.2005
Wangemann, P. (2002). K + cycling and the endocochlear potential. Hear. Res. 165, 1–9. doi: 10.1016/S0378-5955(02)00279-4
Wayne, R. V., and Johnsrude, I. S. (2015). A review of causal mechanisms underlying the link between age-related hearing loss and cognitive decline. Ageing Res. Rev. 23(Pt B), 154–166. doi: 10.1016/j.arr.2015.06.002
Wen, J., Duan, N., Wang, Q., Jing, G. X., and Xiao, Y. (2017). Protective effect of propofol on noise-induced hearing loss. Brain Res. 1657, 95–100. doi: 10.1016/j.brainres.2016.12.005
WHO (2012). WHO Global Estimates on Prevalence of Hearing Loss: Mortality and Burden of Diseases and Prevention of Blindness and Deafness. Available online at: http://www.who.int/pbd/deafness/WHO_GE_HL.pdf. (Accessed August 1,2017).
Willott, J. F. (1984). Changes in frequency representation in the auditory system of mice with age-related hearing impairment. Brain Res. 309, 159–162.
Willott, J. F. (1986). Effects of aging, hearing loss, and anatomical location on thresholds of inferior colliculus neurons in C57BL/6 and CBA mice. J. Neurophysiol. 56:391.
Willott, J. F., Aitkin, L. M., and McFadden, S. L. (1993). Plasticity of auditory cortex associated with sensorineural hearing loss in adult C57BL/6J mice. J. Comp. Neurol. 329, 402–411. doi: 10.1002/cne.903290310
Willott, J. F., Bross, L. S., and McFadden, S. L. (1992). Morphology of the dorsal cochlear nucleus in C57BL/6J and CBA/J mice across the life span. J. Comp. Neurol. 321, 666–678. doi: 10.1002/cne.903210412
Willott, J. F., Bross, L. S., and McFadden, S. L. (1994). Morphology of the cochlear nucleus in CBA/J mice with chronic, severe sensorineural cochlear pathology induced during adulthood. Hear. Res. 74, 1–21.
Wingfield, A., Tun, P. A., McCoy, S. L., Stewart, R. A., and Clarke Cox, L. (2006). Sensory and cognitive constraints in comprehension of spoken language in adult aging. Semin. Hear. 27, 273–283. doi: 10.1055/s-2006-954854
Wong, L. L., Yu, J. K., Chan, S. S., and Tong, M. C. (2014). Screening of cognitive function and hearing impairment in older adults: a preliminary study. Biomed. Res. Int. 2014:867852. doi: 10.1155/2014/867852
Yamasoba, T., Lin, F. R., Someya, S., Kashio, A., Sakamoto, T., and Kondo, K. (2013). Current concepts in age-related hearing loss: epidemiology and mechanistic pathways. Hear. Res. 303, 30–38. doi: 10.1016/j.heares.2013.01.021
Keywords: age-related hearing loss, presbycusis, aging, auditory system, cognitive functions, dementia
Citation: Jayakody DMP, Friedland PL, Martins RN and Sohrabi HR (2018) Impact of Aging on the Auditory System and Related Cognitive Functions: A Narrative Review. Front. Neurosci. 12:125. doi: 10.3389/fnins.2018.00125
Received: 18 September 2017; Accepted: 15 February 2018;
Published: 05 March 2018.
Edited by:
Stefano L. Sensi, Università degli Studi G. d'Annunzio Chieti e Pescara, ItalyReviewed by:
Aurel Popa-Wagner, University of Rostock, GermanyCopyright © 2018 Jayakody, Friedland, Martins and Sohrabi. This is an open-access article distributed under the terms of the Creative Commons Attribution License (CC BY). The use, distribution or reproduction in other forums is permitted, provided the original author(s) and the copyright owner are credited and that the original publication in this journal is cited, in accordance with accepted academic practice. No use, distribution or reproduction is permitted which does not comply with these terms.
*Correspondence: Hamid R. Sohrabi, aC5zb2hyYWJpQGVjdS5lZHUuYXU=
Disclaimer: All claims expressed in this article are solely those of the authors and do not necessarily represent those of their affiliated organizations, or those of the publisher, the editors and the reviewers. Any product that may be evaluated in this article or claim that may be made by its manufacturer is not guaranteed or endorsed by the publisher.
Research integrity at Frontiers
Learn more about the work of our research integrity team to safeguard the quality of each article we publish.