- 1Laboratory of Endocrinology, Department of Clinical Chemistry and Department of Endocrinology and Metabolism, Academic Medical Center, University of Amsterdam, Amsterdam, Netherlands
- 2Clincal Genetics, VU Medical Center, Amsterdam, Netherlands
- 3Metabolism and Reward Group, Netherlands Institute for Neuroscience, Amsterdam, Netherlands
Protein-folding stress at the Endoplasmic Reticulum (ER) occurs in the hypothalamus during diet-induced obesity (DIO) and is linked to metabolic disease development. ER stress is buffered by the activation of the unfolded protein response (UPR), a controlled network of pathways inducing a set of genes that recovers ER function. However, it is unclear whether hypothalamic ER stress during DIO results from obesity related changes or from direct nutrient effects in the brain. We here investigated mRNA expression of UPR markers in the hypothalamus of rats that were exposed to a free choice high-fat high-sugar (fcHFHS) diet for 1 week and then overnight fed ad libitum, or fasted, or fat/sugar deprived (i.e., switched from obesogenic diet to chow). In addition, we determined the direct effects of fat/sugar on mRNA expression of hypothalamus UPR markers by intracarotic infusions of intralipids and/or glucose in chow-fed rats that were fasted overnight. Short term (1 week) exposure to fcHFHS diet increased adiposity compared to chow-feeding. Short term exposure to a fcHFHS diet, followed by mild food restriction overnight, induced hypothalamic ER stress in rats as characterized by an increase in spliced to unspliced X-box binding protein 1 mRNA ratio in hypothalamus of fcHFHS fed rats compared to chow fed rats. Moreover, infused lipids toward the brain of overnight fasted rats, were able to induce a similar response. Non-restricted ad libitum fcHFHS-diet fed or totally fasted rats did not show altered ratios. We also observed a clear increase in hypothalamic activating transcription factor 4 mRNA in rats on the fcHFHS diet while being ad libitum fed or when infused with intralipid via the carotic artery compared to vehicle infusions. However, we did not observe induction of downstream targets implying that this effect is a more general stress response and not related to ER stress. Overall, we conclude that the hypothalamic stress response might be a sensitive sensor of fat and energy status.
Introduction
Obesity is the result of a mismatch between energy intake and energy expenditure. Although a sedentary lifestyle can contribute to obesity development, the consumption of sugar-sweetened beverages and high amounts of saturated fat in foods has been linked to the risk to become obese and develop metabolic disorders (Hall et al., 2012). Over the last decades, the intake of sugar-based beverages has clearly increased worldwide, and from recent surveys it has become clear that consumption of both sugar-sweetened beverages and saturated fats (especially from snack foods) exceeds recommended daily levels (Popkin et al., 2012). It is, therefore, of utmost importance to understand how fat and sugar affect energy balance.
The hypothalamus is an important brain area in regulating energy balance (Elmquist and Flier, 2004; Schwartz and Porte, 2005). Under normal conditions the hypothalamus senses whole body energy demands via nutrient, neuronal and hormonal signaling and adjusts feeding behavior and energy expenditure via the production of orexigenic and anorexigenic peptides. For example leptin, the hormone secreted by adipose tissue in proportion to fat mass, activates the release of α-melanocyte stimulating hormone [α-MSH; derived from pro-opiomelanocortin (POMC)] and inhibits the release of the orexigenic neuropeptide Y (NPY) and agouti related peptide (AgRP) (Sahu, 2011). During obesity, however, the function of intracellular organelles in AGRP/NPY or POMC neurons like the endoplasmic reticulum (ER) was shown to be impaired leading to disturbed leptin signaling and energy imbalance (Hosoi et al., 2008; Zhang et al., 2008; Ozcan et al., 2009; Cakir et al., 2013; Ramírez and Claret, 2015).
The ER is a complex membrane network responsible for the synthesis and folding of various transmembrane and secreted proteins (Westrate et al., 2015). Accumulation of toxic, misfolded proteins in the ER leads to ER stress and activation of the unfolded protein response (UPR). The UPR is a network of pathways controlled by three sensors; PKR-like ER kinase (PERK), the activating transcription factor 6 (ATF6) and the inositol-requiring protein-1 alpha (IREα). Activation of these pathways induces the expression of genes that lead to the expansion of the ER, reduction of protein translation and increase of protein folding capacity promoting cell survival or induces apoptosis (Walter and Ron, 2011; Lee and Ozcan, 2014). Upon PERK activation, activating transcription factor 4 (ATF4) mRNA is translated which increases the transcription of specific UPR target genes, including C/EBP homologous protein (CHOP) (Harding et al., 2000; Han et al., 2013). Immunoglobulin-heavy-chain-binding protein (BiP) mRNA is increased upon ATF6 activation and splicing of unspliced X box binding protein 1 to spliced XBP1 (sXBP1/usXBP1) occurs upon IREα activation (Yoshida et al., 2001). Both usXBP1 and sXBP1 were related to cell viability under ER stress conditions. Under severe ER stress, IREα activation can also lead to degradation of mRNAs and miRNAs and cell apoptosis via the c-Jun N-terminal kinase (JNK) pathway (Todd et al., 2008). DP5 (Death protein 5/harakiri) and FasL (Fas ligand) are genes expressed downstream of JNK indicating activation of the IREα—apoptotic pathway (Schenkel, 2004; Guan et al., 2006; Ma et al., 2007).
Long term high fat diet (HFD) feeding, resulting in profound body weight gain, induces hypothalamic ER stress characterized by increased protein levels of UPR markers like p-PERK, p-IREα, and phospho-eukaryotic initiation factor 2 alpha (p-eIF2α) (Ozcan et al., 2009; Cakir et al., 2013). Activation of UPR pathways was also reported when fatty acids (FA) [arachidic acid, palmitic acid, or ceramide] were directly supplied to the brain via intracerebroventricular (ICV) infusion in rodents (Milanski et al., 2009; Contreras et al., 2014) or when administered to murine neuronal cell lines (Choi et al., 2010) implying a more direct role for FA in hypothalamic ER stress induction. Although many of the HFD used also contain considerable amounts of sugar, the effects of sugar alone on the ER stress induction has not been studied so far.
The fact that long term HFD feeding, but also ICV infusions of FA, induce hypothalamic ER stress marker expression points to the idea that nutrients like FA can induce hypothalamic ER stress. However, it is not clear whether other nutrients or the increased adiposity reflecting a positive energy status or a combination of nutrients and energy status affects hypothalamic ER stress. We therefore determined whether overfeeding/fasting status and its interaction with diet-induced obesity affected genetic markers for ER stress in the hypothalamus. We exposed male Wistar rats to a free choice high-fat high-sugar (fcHFHS) diet or chow for 1 week followed by overnight ad libitum feeding, fat/sugar deprivation or fasting. In addition, we investigated if fat and sugar have a direct effect on the ER stress markers in the hypothalamus by intracarotic infusions of Intralipids (IL), IL and glucose, or glucose to the brain of overnight fasted lean rats. For both experiments, mRNA of different UPR markers was measured using RT-PCR as an indication for the induction of hypothalamic ER stress.
Materials and Methods
Animals
Adult male Wistar rats (250–280 g, Charles River, Germany) were individually housed in a temperature controlled room (19 ± 1°C) on a 12 h light/ 12 h dark cycle (lights on at 7:00 a.m.). During the experiments animals had ad libitum access to water and standard laboratory chow (SDS, UK) unless stated differently. All the studies were approved by and performed according to the regulations of the Committee for Animal Experimentation of the Academic Medical Centre of the University of Amsterdam, Netherlands.
fcHFHS Diet Experiments
Three experiments were performed to study the effect of nutrient availability on the hypothalamic ER stress response and its interaction with obesity. In all experiments rats were on a fcHFHS diet or chow for 1 week. The fcHFHS diet consisted of ad libitum access to chow, tap water, 30% sugar water (1.0 M sucrose mixed from commercial grade sugar and water) and a dish of pure animal fat (beef tallow; Ossewit/Blanc de Boeuf, Vandermoortele, Belgium) (composition: 34% oleic acid, 25% plamitc, and 22% stearic acid and 4% linoleic acid). After this period the three experiments differed in feeding regime the night before the end of the experiment (4:00 p.m. day 7–9:00 a.m. day 8).
• experiment 1: rats had ad libitum access to the control chow diet or to the fcHFHS diet.
• experiment 2: all rats received 10 g chow overnight (fat/sugar deprivation for rats on fcHFHS).
• experiment 3: all rats were fasted overnight.
Fat/sugar deprivation in our diet model is characterized by removing the fat/sugar components of the diet (i.e., saturated fat and sugar water) overnight. We previously showed that removing fat and sugar from the fcHFHS diet results in consumption of 10–15 g chow spontaneously without caloric compensation for the fat/sugar components of the diet (Pandit, 2015). To ensure equal intake overnight between fcHFHS and chow-fed rats we provided all animals with 10 g of chow. Food components of the diet were weighed 5 times a week and the amounts of components eaten were multiplied with the caloric value of each component to determine energy intake in kcals.
At the end of the experiment, rats were anesthetized via 30% CO2/70% O2 and decapitated between 9:00 a.m. and 11:00 a.m. Epididymal, mesenteric, subcutaneous and peritoneal fat were dissected and weighed, trunk blood collected and brains were removed and stored at −80°C until further analysis. Plasma leptin concentrations were determined by radioimmunoassay (Linco Research, Inc., St. Charles, MO, USA). Samples were assayed in duplicate. Amounts of sample, standards, label, antibody and precipitating reagent as described in the procedures of the assay were divided by 4. The detection limit was 0.5 ng/ml and the inter- and intra-assay coefficients were 8% or less.
Intracarotic Infusion Experiment
Rats (n = 6–9 per group) underwent surgery under anesthesia induced with an i.p. injection of 80 mg/kg Ketamin (Eurovet Animal Health, Netherlands), 8 mg/kg Xylazin (Bayer Health Care) and 0.1 mg/kg Atropin (Pharmachemie, Netherlands). A silicon catheter was inserted in the carotid artery and directed toward the brain (according to the method of Steffens, 1969). The catheter was externalized at the vertex of the head and the animals were allowed to recover for 7 days.
To study the direct effect of fat and/or glucose on hypothalamic ER stress markers, NaCl (control), 20% IL [(Fresenuis Kabi); composition of IL is displayed in Table 1] or IL + 1% glucose (G) were infused via the carotid artery toward the brain (experiment 4). Another infusion experiment was performed to study the effect of glucose on hypothalamic ER stress response using glucose (1% in NaCl) or NaCl (experiment 5). All solutions were heparinized (0.04%) and Infusion rate was 5 μl/min for 2 h. One hour after the end of infusion the animals received a single shot of pentobarbital via the carotid artery (100–150 mg/kg BW) and were decapitated. Brains were removed and stored at −80°C for further analysis.
Brain Harvesting—Isolation of the Hypothalamus
Coronal brain slices of 250 μm were obtained from −0.96 to −4.36 mm Bregma (Rat brain atlas; Paxinos and Watson, 2007) and directly put in RNAlater solution (Ambion Life Technologies). The hypothalamic part in each section was isolated using syringe needles (0.4 × 19 mm, BD Microlance) and used for RT-PCR.
RNA Isolation—RT-PCR
One half of the hypothalamus was homogenized in lysis buffer provided with the “High Pure RNA isolation kit” (Roche Molecular Biochemicals, Manheim, Germany) and total RNA was isolated according to the manufacturer's instructions. RNA was quantified by spectrophotometry at 260 nm (Nanodrop 1000, Willmington, Delaware, USA) and cDNA synthesis was performed using the “Transcriptor First Strand cDNA synthesis kit” for RT PCR with oligo(dT) primers (Roche Molecular Biochemicals, Manheim, Germany). The mRNA levels of ER stress markers as well as Hypoxanthine-guanine phosphoribosyltransferase (Hprt), Cyclophilin A and β-actin were determined by RT-PCR using SensiFAST SYBR No-Rox mix (Bioline, Luckenwalde, Germany) at the Lightcycler 480 apparatus (Roche Molecular Biochemicals, Manheim, Germany). The primers were designed using “Primer Blast” (Table 2). Samples were baseline corrected and individually checked for their PCR efficiency using the “LC480 Conversion” and “LinRegPCR” software. Median efficiency was calculated for each assay and samples that differed more than 0.05 from the mean efficiency were excluded from statistical analysis. Specific gene expression was normalized to the geometric mean of three housekeeping genes; (Hprt × β-actin × Cyclophilin A)1/3.
Statistical Analysis
Data are presented as Mean ± SEM. Outliers were detected using “Dixon's Q test” and excluded. Differences between diet groups were evaluated using Student's t-test [experiments 1–4, 5 (NaCl vs. G)] or ANOVA followed by post-hoc Tukey test (experiment 5, NaCl vs. IL vs. IL+G). Difference between groups was considered significant when p < 0.05. In order to determine the effects of low vs. high fat intake and low vs. high sugar intake a median split was performed whereby the median was calculated for fat or for sugar intake and the animals that consumed more than the median were depicted as high consumers and those that consumed lower as low consumers. All tests were performed using Graphpad Prism 6 (Graphpad software Inc., la Jolla, CA, USA).
Results
One Week of fcHFHS Diet Does Not Induce Hypothalamic ER Stress Markers
Rats exposed to the fcHFHS diet for 1 week were hyperphagic as shown by increased caloric intake compared to those on chow (Table 3). As a result, % WAT/BW and plasma leptin levels were significantly higher in rats on the fcHFHS diet compared to rats on chow whereas ΔBW did not differ between the groups (Table 3).
One week of fcHFHS diet exposure significantly increased ATF4 mRNA expression (Figure 1A). However, mRNA expression of CHOP, a target gene of ATF4, and BiP, a target gene of ATF6, was not significantly changed in hypothalami of fcHFHS-fed rats compared to chow-fed controls (Figures 1B,C). Splicing of usXBP1 to sXBP1 as shown by the ratio sXBP1/usXBP1 (Figure 1D), as well as DP5 and FasL mRNA expression did not differ between the groups.
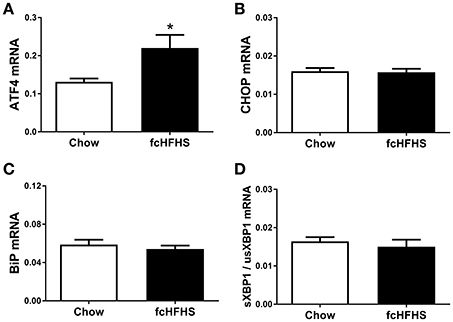
Figure 1. ER stress markers in rat hypothalamus after 1 week ad libitum fcHFHS diet or chow. (A) ATF4 and (B) CHOP (C) BiP, and (D) sXBP1/usXBP1 mRNA expression. mRNA expression of specific genes was normalized to the geometric mean of three housekeeping genes. Significant differences between the fcHFHS and control group: *p < 0.05.
Overnight Fat/Sugar Deprivation Induces ER Stress in Animals Exposed to the fcHFHS Diet for 1 Week
ΔBW, % WAT/BW and leptin were still increased in fcHFHS-fed rats compared to chow-fed rats when overnight deprived of fat and sugar (Table 3), but no changes were observed in hypothalamic ATF4, CHOP, and BiP mRNA expression between the groups (Figures 2A–C; left column). However, sXBP1/usXBP1 mRNA was higher and DP5 mRNA was lower in the fcHFHS-fed group compared to the chow controls (Figures 2D,E; left column).
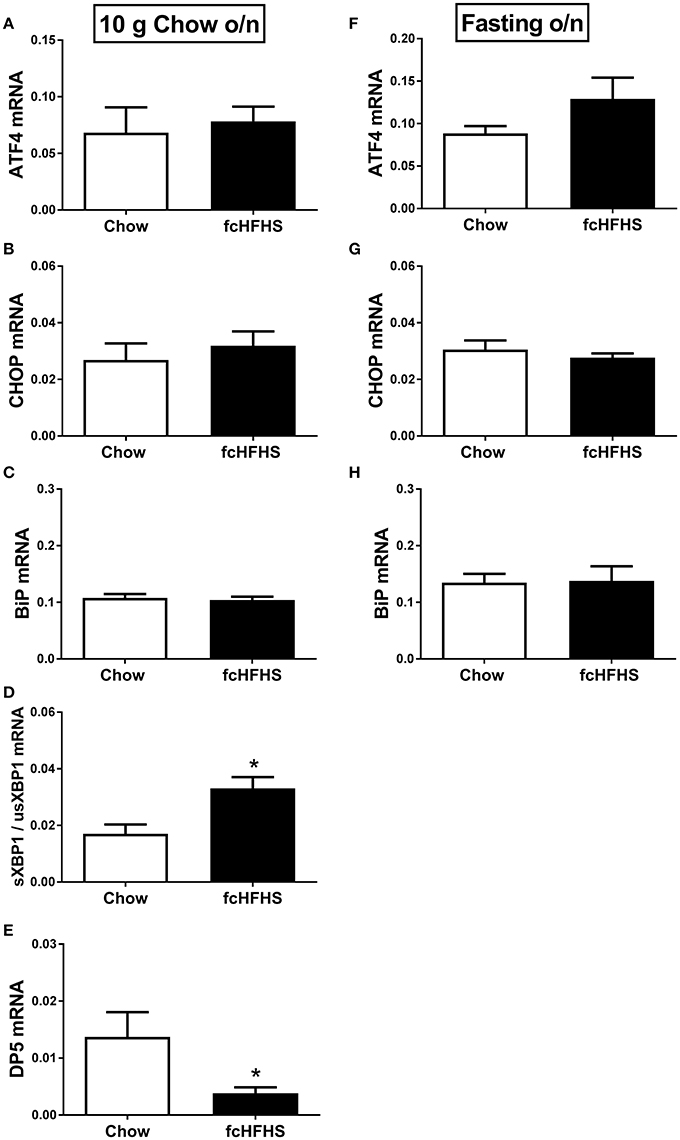
Figure 2. The effect of overnight fat/sugar deprivation (10 g chow; left column) or fasting (right column) after 1 week fcHFHS diet or chow on the mRNA expression of hypothalamic (A,F) ATF4, (B,G) CHOP, (C,H) BiP, (D) sXBP1/usXBP1, and (E) DP5. mRNA expression of specific genes was normalized to the geometric mean of three housekeeping genes. Significant differences between the fcHFHS and control groups: *p < 0.05.
No change in FasL mRNA expression was observed between the groups after removing fat and sugar overnight (not shown). Similarly, no differences in ATF4, CHOP, BiP, and FasL mRNA expression were observed between the groups after overnight fasting (Figure 2, right column). sXBP1/usXBP1 and DP5 mRNA expression were not detectable in the overnight fasted groups.
Fat Consumption Leads the Changes Induced in Stress Gene Markers
To determine to what extent fat or sugar contributed to the observed differences observed in hypothalamic mRNA, we divided the fcHFHS group according to fat and sugar intake using a median split forming either low or high fat consumers (LF or HF) or low or high sugar consumers (LS or HS). The median fat consumption was 10% out of total caloric intake. Rats that consumed <10% fat were assigned as LF consumers and those that consumed >10% as HF consumers. LF and HF consumers had similar BW, total caloric intake and chow intake, but fat consumption in HF group was significantly higher and sugar intake significantly lower compared to the LF group (Table 4). ATF4 mRNA expression tended to be higher (p = 0.07), in the hypothalamus of the HF consumers compared to the LF consumers, whereas sXBP1/usXBP1, DP5 and FasL mRNA expression was not different between the groups (data not shown). Similar analysis was performed for consumption of sugar over a week of fcHFHS exposure and median sugar consumption out of total caloric intake was 38%. No differences in ATF4, usXBP1, sXBP1/usXBP1, DP5, or FasL mRNA expression were observed between HS and LS consumers (data not shown).
To further investigate the direct effects of fat and sugar in the brain on hypothalamic ER stress markers, we infused IL, IL + glucose (ILG), glucose or saline (control) via the carotid artery directly to the brain of overnight fasted rats. IL and ILG increased hypothalamic ATF4 mRNA expression compared to saline but did not affect CHOP, DP5, and FasL mRNA (Figures 3A–D). In addition, IL infusion resulted in increased sXBP1/usXBP1 mRNA expression, and tended to increase BiP mRNA expression (Figures 3E,F). Glucose infusion did not have an effect on AFT4, sXBP1/usXBP1, and DP5 mRNA expression (Figures 3G–I,K), but reduced BiP and FasL mRNA levels compared to saline infusion (Figures 3J,L).
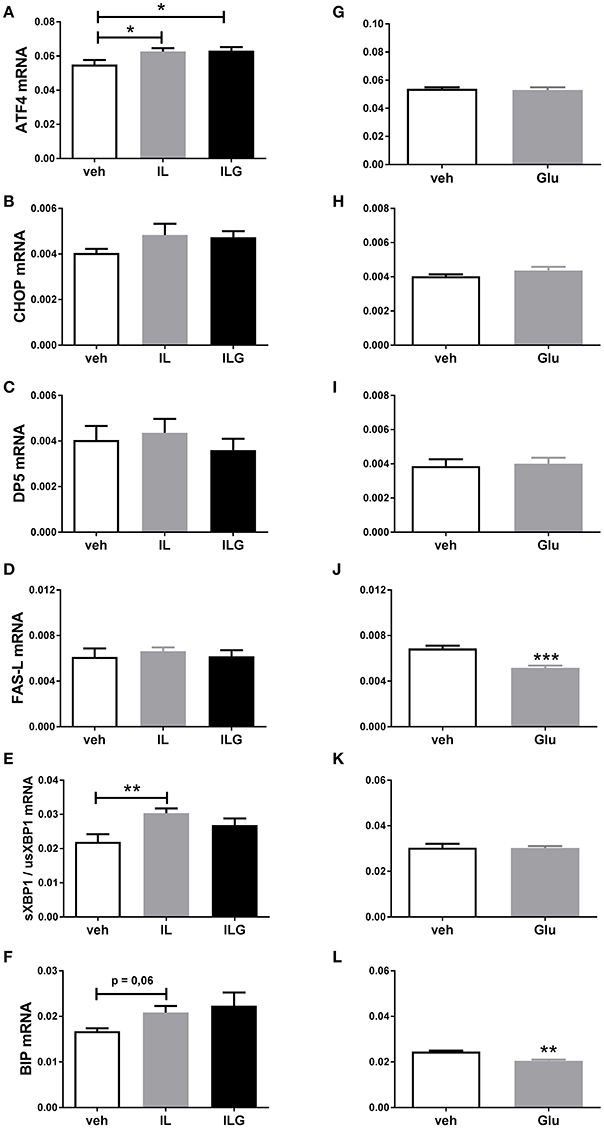
Figure 3. The effect of central infusion of Intralipid (IL), Intralipid and Glucose (ILG) or Glucose on hypothalamic (A,G) ATF4, (B,H) CHOP (C), (C,I) DP5, (D,J) FasL, (E,K) sXBP1/usXBP1, (F,L) Bip mRNA expression in chow rats. mRNA expression of specific genes was normalized to the geometric mean of three housekeeping genes. Significant differences between groups: *p < 0.05, **p ≤ 0.01; ***p < 0.001.
Discussion
We showed that short term exposure to a fcHFHS diet, followed by mild food restriction overnight induces hypothalamic ER stress in rats as characterized by an increase in sXPB1/usXBP1 mRNA ratio in hypothalamus of fcHFHS fed rats compared to chow fed rats. Moreover, we showed that lipids, directly infused towards the brain of overnight fasted rats, are able to induce a similar response. As non-restricted ad libitum fcHFHS-diet fed or totally fasted rats do not show altered ratios, these data point to an interaction of lipid exposure to the brain and a negative energy balance in ER stress induction. In addition, we observed an increase in ATF4 mRNA when animals were ad libitum fed the fcHFHS diet which is abolished when rats are either provided with 10 g of chow overnight or totally fasted. In addition, high fat consumers on the fcHFHS diet have higher ATF4 mRNA, pointing to a direct role for fat intake in the increase in hypothalamic ATF4 mRNA. Indeed, ATF4 mRNA is also increased when animals are directly infused with lipids or lipids and glucose towards the brain, but not when glucose is infused alone.
An increase in sXPB1/usXBP1mRNA ratio implies activation of the IREα pathway (Yoshida et al., 2001; Calfon et al., 2002). The activation of IREα pathway due to HFD-feeding has been reported earlier. However, this was after long-term (8 or 20 weeks) exposure (Ozcan et al., 2009; Won et al., 2009). Here we showed for the first time that short term exposure to a fcHFHS diet is enough to induce activation of this pathway, but only under mild food restriction.
It is unclear why animals on a fcHFHS diet display this response only when food restricted while absent when animals are ad libitum fed overnight. One possibility might be that ER stress was induced via anabolic processes as illustrated by the observation that overnight fasting and subsequent refeeding increases sXBP1 mRNA and protein in the liver (Deng et al., 2013) and in the hypothalamus of mice (Williams et al., 2014). It is also possible that protective mechanisms play a role under ad libitum conditions. For example, we observed recently that 1 week fcHFHS diet increases beta- oxidation genes in the hypothalamus (Rijnsburger et al., 2016), and since enhanced beta-oxidation has been reported to counter palmitate-induced ER stress in in vitro models (McFadden et al., 2014), it is possible that we do not observe increased splicing of XPB1 under ad libitum feeding because of counter-active regulatory fatty acid oxidation. In line, we observed no changes in fatty acid oxidation genes after lipid infusion directly to the brain while it does induce XBP1 splicing (M. Rijnsburger, unpublished data). Further research is needed to determine the exact role of energy status and nutrient sensing on hypothalamic ER stress induction.
We observed a clear increase in hypothalamic ATF4 mRNA in rats on the fcHFHS diet while being ad libitum fed or when infused with intralipids via the carotic artery compared to vehicle infusions. However, we did not observe induction of downstream targets. It might be possible that increased ATF4 mRNA expression in our models is associated with other general stress related events within a cell i.e., amino-acid or glucose deprivation (Siu et al., 2002) or less protein availability (which has been shown in muscle to result in UPR activation; Deldicque et al., 2010). An additional effect of the fcHFHS diet is reduced chow—and thus protein—intake compared to chow controls, it is well possible that this might be the cause for the changes in ATF4 mRNA expression observed in our study. It is unclear at this point whether this increase in ATF4 mRNA will result in increased ATF4 protein. Interestingly, overexpression and inhibition of ATF4 in the hypothalamus induces hepatic insulin resistance and improves hepatic insulin sensitivity, respectively (Zhang et al., 2013). In addition, a recent study showed that ATF4 deletion in AGRP neurons of the hypothalamus specifically protects against high fat diet induced weight gain and insulin resistance (Deng et al., 2016). Together, this suggests an important role for hypothalamic ATF4 in the regulation of energy metabolism. Interestingly, we previously reported hepatic insulin resistance in rats on a fcHFHS diet (Diepenbroek et al., 2017), which could well be related to the observed increase in hypothalamic ATF4 mRNA.
Under severe ER stress, IREα activation can also lead to cell apoptosis via the JNK pathway (Todd et al., 2008) and to activation of apoptotic genes (Urano et al., 2000; Guan et al., 2006; Kim et al., 2006; Ma et al., 2007). We therefore measured DP5 and FasL mRNA, target genes of JNK pathway (Schenkel, 2004; Guan et al., 2006; Ma et al., 2007). However, 1 week fcHFHS diet did not result in activation of apoptotic gene expression. It could well be that this is due to the duration of the diet, as exposure to the diet for a longer period induces apoptosis accompanied by obesity and other metabolic disturbances (Ozcan et al., 2009; Won et al., 2009).
Interestingly a decrease in DP5 mRNA expression was observed in the fcHFHS group after overnight mild food restriction (10 g chow intake). That might be related to the increase in sXBP1 mRNA under the same conditions. Since both are regulated by IREα activation, the observed alterations suggest a shift from cell death related pathways to the induction of cell survival mechanisms. Like DP5, FasL mRNA can be also induced upon IREα/JNK activation during neuronal apoptosis (Le-Niculescu et al., 1999; Schenkel, 2004; Chen et al., 2015). However, no change was observed in FasL expression indicating that FasL does not play a major role in our experimental setting. In addition, a glucose infusion directly to the brain lowered FasL mRNA expression when compared to a vehicle control infusion, however what this means physiologically remains to be determined.
In summary, a fcHFHS diet and overnight fat and sugar availability affects the mRNA expression of hypothalamic ER stress related UPR markers. Overall, the UPR markers seemed to be a sensitive sensor of fatty acid availability as well as nutrient load. More studies are necessary to define the exact role of nutrients in induction of UPR intermediates that play a role in cellular metabolism and viability.
Author Contributions
EB, AB, and Sl designed experiments. EB, MR, LE, UU, and Sl performed experiments. EB, AB, and Sl prepared the manuscript. WS, MR, LE, and UU edited the manuscript. The entire study was supervised by AB and Sl.
Conflict of Interest Statement
The authors declare that the research was conducted in the absence of any commercial or financial relationships that could be construed as a potential conflict of interest.
Acknowledgments
This research was supported by the Dutch Technology Foundation STW (grant 12264), which is part of the Netherlands Organization for Scientific Research (NWO), and which is partly funded by the Ministry of Economic Affairs.
References
Cakir, I., Cyr, N. E., Perello, M., Litvinov, B. P., Romero, A., Stuart, R. C., et al. (2013). Obesity induces hypothalamic endoplasmic reticulum stress and impairs proopiomelanocortin (POMC) post-translational processing. J. Biol. Chem. 288, 17675–17688. doi: 10.1074/jbc.M113.475343
Calfon, M., Zeng, H., Urano, F., Till, J. H., Hubbard, S. R., Harding, H. P., et al. (2002). IRE1 couples endoplasmic reticulum load to secretory capacity by processing the XBP-1 mRNA. Nature 415, 92–96. doi: 10.1038/415092a
Chen, X., Shen, J., Wang, Y., Chen, X., Yu, S., Shi, H., et al. (2015). Up-regulation of c-Fos associated with neuronal apoptosis following intracerebral hemorrhage. Cell. Mol. Neurobiol. 35, 363–376. doi: 10.1007/s10571-014-0132-z
Choi, S. J., Kim, F., Schwartz, M. W., and Wisse, B. E. (2010). Cultured hypothalamic neurons are resistant to inflammation and insulin resistance induced by saturated fatty acids. Am. J. Physiol. Endocrinol. Metab. 298, E1122–E1130. doi: 10.1152/ajpendo.00006.2010
Contreras, C., González-García, I., Martínez-Sánchez, N., Seoane-Collazo, P., Jacas, J., Morgan, D. A., et al. (2014). Central ceramide-induced hypothalamic lipotoxicity and ER stress regulate energy balance. Cell Rep. 9, 366–377. doi: 10.1016/j.celrep.2014.08.057
Deldicque, L., Cani, P. D., Philp, A., Raymackers, J. M., Meakin, P. J., Ashford, M. L., et al. (2010). The unfolded protein response is activated in skeletal muscle by high-fat feeding: potential role in the downregulation of protein synthesis. Am. J. Physiol. Endocrinol. Metab. 299, E695–E705. doi: 10.1152/ajpendo.00038.2010
Deng, J., Yuan, F., Guo, Y., Xiao, Y., Niu, Y., Deng, Y., et al. (2016). Deletion of ATF4 in AgRP neurons promotes fat loss mainly via increasing energy expenditure. Diabetes 66, 640–650. doi: 10.2337/db16-0954
Deng, Y., Wang, Z. V., Tao, C., Gao, N., Holland, W. L., Ferdous, A., et al. (2013). The Xbp1s/GalE axis links ER stress to postprandial hepatic metabolism. J. Clin. Invest. 123, 455–468. doi: 10.1172/JCI62819
Diepenbroek, C., Eggels, L., Ackermans, M. T., Fliers, E., Kalsbeek, A., Serlie, M. J., et al. (2017). Differential effects of hypercaloric choice diets on insulin sensitivity in rats. J. Endocrinol. 232, 49–57. doi: 10.1530/JOE-16-0265
Elmquist, J. K., and Flier, J. S. (2004). Neuroscience. The fat-brain axis enters a new dimension. Science 304, 63–64. doi: 10.1126/science.1096746
Guan, Q. H., Pei, D. S., Xu, T. L., and Zhang, G. Y. (2006). Brain ischemia/reperfusion-induced expression of DP5 and its interaction with Bcl-2, thus freeing Bax from Bcl-2/Bax dimmers are mediated by c-Jun N-terminal kinase (JNK) pathway. Neurosci. Lett. 393, 226–230. doi: 10.1016/j.neulet.2005.09.075
Hall, K. D., Heymsfield, S. B., Kemnitz, J. W., Klein, S., Schoeller, D. A., and Speakman, J. R. (2012). Energy balance and its components: implications for body weight regulation. Am. J. Clin. Nutr. 95, 989–994. doi: 10.3945/ajcn.112.036350
Han, J., Back, S. H., Hur, J., Lin, Y. H., Gildersleeve, R., Shan, J., et al. (2013). ER-stress-induced transcriptional regulation increases protein synthesis leading to cell death. Nat. Cell Biol. 15, 481–490. doi: 10.1038/ncb2738
Harding, H. P., Novoa, I., Zhang, Y., Zeng, H., Wek, R., Schapira, M., et al. (2000). Regulated translation initiation controls stress-induced gene expression in mammalian cells. Mol. Cell 6, 1099–1108. doi: 10.1016/S1097-2765(00)00108-8
Hosoi, T., Sasaki, M., Miyahara, T., Hashimoto, C., Matsuo, S., Yoshii, M., et al. (2008). Endoplasmic reticulum stress induces leptin resistance. Mol. Pharmacol. 74, 1610–1619. doi: 10.1124/mol.108.050070
Kim, B. J., Ryu, S. W., and Song, B. J. (2006). JNK- and p38 kinase-mediated phosphorylation of Bax leads to its activation and mitochondrial translocation and to apoptosis of human hepatoma HepG2 cells. J. Biol. Chem. 281, 21256–21265. doi: 10.1074/jbc.M510644200
Lee, J., and Ozcan, U. (2014). Unfolded protein response signaling and metabolic diseases. J. Biol. Chem. 289, 1203–1211. doi: 10.1074/jbc.R113.534743
Le-Niculescu, H., Bonfoco, E., Kasuya, Y., Claret, F. X., Green, D. R., and Karin, M. (1999). Withdrawal of survival factors results in activation of the JNK pathway in neuronal cells leading to Fas ligand induction and cell death. Mol. Cell. Biol. 19, 751–763. doi: 10.1128/MCB.19.1.751
Ma, C., Ying, C., Yuan, Z., Song, B., Li, D., Liu, Y., et al. (2007). dp5/HRK is a c-Jun target gene and required for apoptosis induced by potassium deprivation in cerebellar granule neurons. J. Biol. Chem. 282, 30901–30909. doi: 10.1074/jbc.M608694200
McFadden, J. W., Aja, S., Li, Q., Bandaru, V. V., Kim, E. K., Haughey, N. J., et al. (2014). Increasing fatty acid oxidation remodels the hypothalamic neurometabolome to mitigate stress and inflammation. PLoS ONE 9:e115642. doi: 10.1371/journal.pone.0115642
Milanski, M., Degasperi, G., Coope, A., Morari, J., Denis, R., Cintra, D. E., et al. (2009). Saturated fatty acids produce an inflammatory response predominantly through the activation of TLR4 signaling in hypothalamus: implications for the pathogenesis of obesity. J. Neurosci. 29, 359–370. doi: 10.1523/JNEUROSCI.2760-08.2009
Oslowski, C. M., and Urano, F. (2011). Measuring ER stress and the unfolded protein response using mammalian tissue culture system. Meth. Enzymol. 490, 71–92. doi: 10.1016/B978-0-12-385114-7.00004-0
Ozcan, L., Ergin, A. S., Lu, A., Chung, J., Sarkar, S., Nie, D., et al. (2009). Endoplasmic reticulum stress plays a central role in development of leptin resistance. Cell Metab. 9, 35–51. doi: 10.1016/j.cmet.2008.12.004
Paxinos, G., and Watson, C. (2007). The Rat Brain in Stereotaxic Coordinates, 6th Edn. San Diego, CA: Academic Press; Elsevier.
Popkin, B. M., Adair, L. S., and Ng, S. W. (2012). Global nutrition transition and the pandemic of obesity in developing countries. Nutr. Rev. 70, 3–21. doi: 10.1111/j.1753-4887.2011.00456.x
Ramírez, S., and Claret, M. (2015). Hypothalamic ER stress: a bridge between leptin resistance and obesity. FEBS Lett. 589, 1678–1687. doi: 10.1016/j.febslet.2015.04.025
Rijnsburger, M., Belegri, E., Eggels, L., Unmehopa, U. A., Boelen, A., Serlie, M. J., et al. (2016). The effect of diet interventions on hypothalamic nutrient sensing pathways in rodents. Physiol. Behav. 162, 61–68. doi: 10.1016/j.physbeh.2016.04.011
Sahu, A. (2011). Intracellular leptin-signaling pathways in hypothalamic neurons: the emerging role of phosphatidylinositol-3 kinase-phosphodiesterase-3B-cAMP pathway. Neuroendocrinology 93, 201–210. doi: 10.1159/000326785
Schenkel, J. (2004). Activation of the c-Jun transcription factor following neurodegeneration in vivo. Neurosci. Lett. 361, 36–39. doi: 10.1016/j.neulet.2003.12.011
Schwartz, M. W., and Porte, D. Jr. (2005). Diabetes, obesity, and the brain. Science 307, 375–379. doi: 10.1126/science.1104344
Siu, F., Bain, P. J., LeBlanc-Chaffin, R., Chen, H., and Kilberg, M. S. (2002). ATF4 is a mediator of the nutrient-sensing response pathway that activates the human asparagine synthetase gene. J. Biol. Chem. 277, 24120–24127. doi: 10.1074/jbc.M201959200
Steffens, A. B. (1969). Blood glucose and FFA levels in relation to the meal pattern in the normal rat and the ventromedial hypothalamic lesioned rat. Physiol. Behav. 4, 215–216, IN11–IN12, 217–225. doi: 10.1016/0031-9384(69)90083-3
Todd, D. J., Lee, A. H., and Glimcher, L. H. (2008). The endoplasmic reticulum stress response in immunity and autoimmunity. Nat. Rev. Immunol. 8, 663–674. doi: 10.1038/nri2359
Urano, F., Wang, X., Bertolotti, A., Zhang, Y., Chung, P., Harding, H. P., et al. (2000). Coupling of stress in the ER to activation of JNK protein kinases by transmembrane protein kinase IRE1. Science 287, 664–666. doi: 10.1126/science.287.5453.664
Walter, P., and Ron, D. (2011). The unfolded protein response: from stress pathway to homeostatic regulation. Science 334, 1081–1086. doi: 10.1126/science.1209038
Westrate, L. M., Lee, J. E., Prinz, W. A., and Voeltz, G. K. (2015). Form follows function: the importance of endoplasmic reticulum shape. Annu. Rev. Biochem. 84, 791–811. doi: 10.1146/annurev-biochem-072711-163501
Williams, K. W., Liu, T., Kong, X., Fukuda, M., Deng, Y., Berglund, E. D., et al. (2014). Xbp1s in Pomc neurons connects ER stress with energy balance and glucose homeostasis. Cell Metab. 20, 471–482. doi: 10.1016/j.cmet.2014.06.002
Won, J. C., Jang, P. G., Namkoong, C., Koh, E. H., Kim, S. K., Park, J. Y., et al. (2009). Central administration of an endoplasmic reticulum stress inducer inhibits the anorexigenic effects of leptin and insulin. Obesity (Silver. Spring). 17, 1861–1865. doi: 10.1038/oby.2009.194
Yoshida, H., Matsui, T., Yamamoto, A., Okada, T., and Mori, K. (2001). XBP1 mRNA is induced by ATF6 and spliced by IRE1 in response to ER stress to produce a highly active transcription factor. Cell 107, 881–891. doi: 10.1016/S0092-8674(01)00611-0
Zhang, Q., Yu, J., Liu, B., Lv, Z., Xia, T., Xiao, F., et al. (2013). Central activating transcription factor 4 (ATF4) regulates hepatic insulin resistance in mice via S6K1 signaling and the vagus nerve. Diabetes 62, 2230–2239. doi: 10.2337/db12-1050
Keywords: ER stress response, hypothalamus, food restriction, fatty acids, sugar
Citation: Belegri E, Rijnsburger M, Eggels L, Unmehopa U, Scheper W, Boelen A and la Fleur SE (2017) Effects of Fat and Sugar, Either Consumed or Infused toward the Brain, on Hypothalamic ER Stress Markers. Front. Neurosci. 11:270. doi: 10.3389/fnins.2017.00270
Received: 15 February 2017; Accepted: 26 April 2017;
Published: 15 May 2017.
Edited by:
Hubert Vaudry, University of Rouen, FranceReviewed by:
Denis Richard, Laval University, CanadaJulie A. Chowen, Hospital Infantil Universitario Niño Jesús, Spain
Copyright © 2017 Belegri, Rijnsburger, Eggels, Unmehopa, Scheper, Boelen and la Fleur. This is an open-access article distributed under the terms of the Creative Commons Attribution License (CC BY). The use, distribution or reproduction in other forums is permitted, provided the original author(s) or licensor are credited and that the original publication in this journal is cited, in accordance with accepted academic practice. No use, distribution or reproduction is permitted which does not comply with these terms.
*Correspondence: Susanne E. la Fleur, s.e.lafleur@amc.uva.nl
†These authors have contributed equally to this work.