- Department of Neurophysiology, Beritashvili Institute of Physiology, Tbilisi, Georgia
Repeated injection of opioid analgesics can lead to a progressive loss of effect. This phenomenon is known as tolerance. Several lines of investigations have shown that systemic, intraperitoneal administration or the microinjection of non-opioid analgesics, non-steroidal anti-inflammatory drugs (NSAIDs) into the midbrain periaqueductal gray matter induces antinociception with some effects of tolerance. Our recent study has revealed that microinjection of three drugs analgin, ketorolac, and xefocam into the central nucleus of amygdala produce tolerance to them and cross-tolerance to morphine. Here we report that repeated administrations of these NSAIDs into the nucleus raphe magnus (NRM) in the following 4 days result in progressively less antinociception compare to the saline control, i.e., tolerance develops to these drugs in male rats. Special control experiments showed that post-treatment with the μ-opioid antagonist naloxone into the NRM significantly decreased antinociceptive effects of NSAIDs on the first day of testing in the tail-flick (TF) reflex and hot plate (HP) latency tests. On the second day, naloxone generally had trend effects in both TF and HP tests and impeded the development of tolerance to the antinociceptive effect of non-opioid analgesics. These findings strongly support the suggestion of endogenous opioid involvement in NSAIDs antinociception and tolerance in the descending pain-control system. Moreover, repeated injections of NSAIDs progressively lead to tolerance to them, cross-tolerance to morphine, and the risk of a withdrawal syndrome. Therefore, these results are important for human medicine too.
Introduction
It has been established that the pain-control system includes the midbrain periaqueductal gray matter (PAG) and rostral ventro-medial medulla (RVM). The RVM involves the midline nucleus raphe magnus (NRM) and adjacent reticular formation. The medullar NRM is one of the important parts of the CNS circuit that controls nociceptive transmission at the level of the spinal cord. It is functionally involved in descending pain modulation, and mainly consists of serotoninergic neurons (Fields, 2004; Fields et al., 2006; Ren and Dubner, 2009). The PAG controls nociceptive transmission mainly through the RVM. The PAG–RVM system is the central substrate for the actions of opioid analgesic drugs. Endogenous opioid peptides are present in neural somata and/or terminal fields in several components of this network. In animals, electrical stimulation of the PAG inhibits simple noxious-stimulus reflexes, such as seen in the tail-flick (TF) and/or hot plate (HP) paw withdrawal tests. Furthermore, this circuit contributes to opiate analgesia and opioid dependence (Henricher and Ingram, 2009; Heinricher et al., 2009; Ren and Dubner, 2009).
Several lines of investigations have shown that in the PAG and RVM, the microinjection of non-opioid analgesics, and non-steroidal anti-inflammatory drugs (NSAIDs) induces antinociception but with some evidence of tolerance (Jones, 1996; Tortorici and Vanegas, 2000; Pernia-Andrade et al., 2004; Tortorici et al., 2004, 2009). Specifically, microinjections of dipyrone (metamizol) into the medullary NRM of lightly pentobarbital-anesthetized rats dose-dependently inhibited the nociceptive TF reflex (Jones, 1996). Moreover, repeated microinjections of dipyrone and lysine-acetylsalicylate (LASA) into the PAG produced opioid like tolerance in rats, together with a withdrawal syndrome (Tortorici and Vanegas, 2000; Pernia-Andrade et al., 2004; Tortorici et al., 2004, 2009). Our recent findings also have shown the same effects of tolerance following intraperitoneal (i.p.) injections of analgin (metamizol), ketorolac, and xefocam (Tsagareli et al., 2005; Tsiklauri and Tsagareli, 2006; Tsiklauri et al., 2006, 2008; Tsiklauri et al., 2010). Taken together these studies support the notion that contribution of the downstream pain-control system to the tolerance effects of the above-mentioned NSAIDs involves endogenous opioidergic mechanisms (Hernández-Delgadillo and Cruz, 2006; Henricher and Ingram, 2009; Vanegas et al., 2010). Just recently, we have shown that microinjection of three NSAIDs analgin, ketorolac, and xefocam into the central nucleus of amygdala (CeA) produced tolerance to these drugs and cross-tolerance to morphine (Tsagareli et al., 2009, 2010).
In the present study, we have examined whether microinjection of analgin (metamizol), clodifen, ketorolac, and xefocam into the NRM leads to the development of tolerance and whether their action in the NRM is opioid sensitive.
Materials and Methods
Animals
The experiments were carried out on male Wistar rats (200–250 g) bred at the Beritashvili Institute of Physiology. The experimental protocol was approved by the local Bioethic Committee of the Institute and Guidelines of the International Association for the Study of Pain regarding animal experimentation were followed throughout (Zimmermann, 1983).
Surgical Procedures
Under anesthesia with thiopental (55 mg/kg, i.p. “Kievmed” Ukraine) a 12-mm-long stainless steel guide cannula (Small Parts, Inc., USA) was stereotaxically implanted (AP: −9.16; L: +1; H: 9.8) according to the coordinates in the atlas of Paxinos and Watson (1997) siting the tip 2 mm above the NRM. The guides were anchored to the cranium by dental cement. The guide cannula was plugged with a stainless steel stylet. Thereafter, the rats were handled every day for 3 days for 15 min to get familiar with the testing protocol and experimental environment. During this time, the stylet was removed and 14 mm-long stainless steel microinjection cannula was inserted into the guide cannula to reach the NRM, but no drug was injected. This helped to habituate the rats to the injection procedure and to reduce artifacts arising from mechanical manipulation during the test days. Five days after surgery the microinjection cannula, attached to a 5-μl Hamilton syringe (Hamilton, Inc., USA), was joined to the guide cannula, and the drug was introduced through it while the rat was gently restrained.
Drugs
Analgin 150 μg/0.5 μl (metamizol sodium, “Sanitas,” Ltd., Lithuania), clodifen (diclofenac sodium, 75 μg/0.5 μl, “E.I.P.I.” Comp., Egypt), ketorolac (ketorolac tromethamine, 90 μg/0.5 μl, “Zee Drugs,” India), xefocam (lornoxicam, 12 μg/0.5 μl, “Nycomed,” GmbH, Austria) were then injected through the microinjection cannula; then the guide cannula was plugged with a stainless steel stylet. Saline was injected in the same volume (0.5 μl; “GalichPharm” Ltd., Ukraine) and manner in a separate group of rats for controls.
Behavioral Testing
Twenty minutes post microinjection, i.e., 10-min before the peak of the drugs’ effect is normally reached, animals were tested with TF or HP. For the TF test, the distal part of the tail was stimulated with a light beam (IITC #33, IITC Life science, Inc., Woodland Hills, CA, USA) and the latency measured until the tail was reflexively flicked away from the beam. For the HP test, the rat was placed on a 55°C HP (IITC #39) and the latency to the first lick of the hindpaw or jumping was measured. The cut-off time was 20 s for both TF and HP latencies. Each rat was tested for both TF and HP latencies in the same session. A similar procedure was followed for the repeated microinjection of analgine, clodifen, ketorolac, xefocam, or saline for five consecutive days.
In a separate set of experiments, 20 min following NSAIDs administration we tested whether post-treatment with the μ-opioid antagonist naloxone (0.5 μl) in the NRM diminishes NSAID-induced antinociception on the first and second experimental days.
Histology
At the end of each experiment, after the fifth day and the second day of the second series of experiments the microinjection site was marked with 2 μl of a saturated solution of Pontamine Sky Blue (Sigma Chemical, Co.), and the animal was killed with ester by an overdose of an anesthetic. After fixation by immersion in 10% formalin the brain was sectioned and the microinjection site was identified with the aid of Paxinos and Watson (1997) stereotaxic atlas coordinates.
Statistical Analysis
All data are presented as mean ± SEM. Analysis of variance (ANOVA) with post hoc Tukey–Kramer Multiple Compayon Tests were used for statistical evaluations of comparisons between treated and saline groups, and treated and naloxone groups respectively. The Kolmogorov–Smirnov test was applied to verify normality. The statistical software utilized was InStat 3.05 (GraphPad Software, Inc., USA). Statistical significance between vehicle control and treated groups, and naloxone and treated groups of rats was acknowledged if P < 0.05.
Results
Histology Control
Only rats with microinjections located in the NRM were included for data analysis. Histological location of microinjection sites is shown in a simplified drawing section from the Paxinos and Watson (1997) atlas. All microinjections of NSAIDs or saline were histologically located in the NRM (Figure 1).
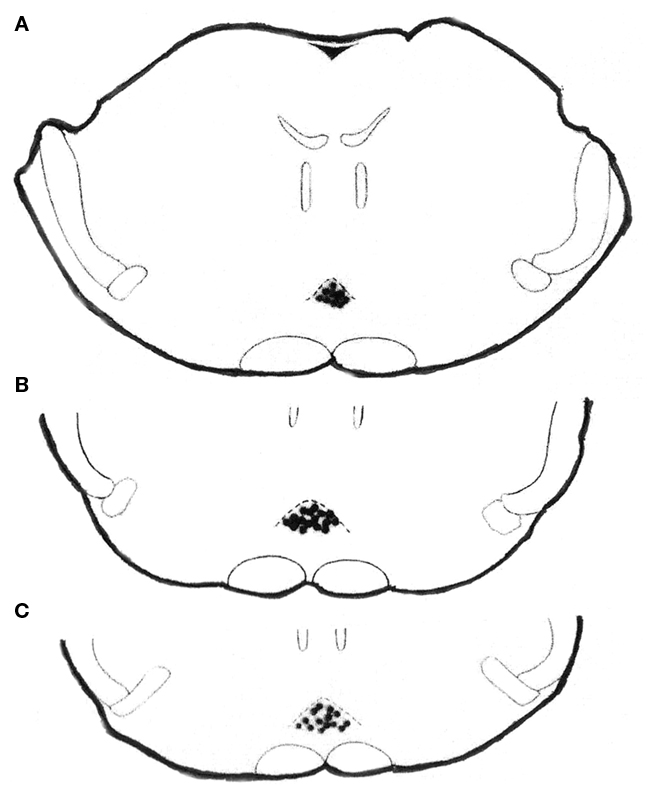
Figure 1. Histologically verified microinjection sites for analgin, clodifen, ketorolac, xefocam, or saline in the NRM, in transverse sections simplified from Paxinos and Watson (1997) atlas. Rats were microinjected with NSAIDs or saline for 5 days to induce tolerance. The distance from the bregma is −9.16 mm (A), −9.68 (B), and −9.8 (C), respectively.
Tolerance to NSAIDs
Our investigation showed that microinjection of NSAIDs into the NRM produced antinociception as revealed by a latency increase in TF and HP responses compared to the saline control microinjected into the same nucleus. The TF latency significantly increased for analgin [ANOVA: F(5,44) = 53.797, (P < 0.0001)], clodifen [ANOVA: F(5,39) = 43.233, (P < 0.0001)], ketorolac [ANOVA: F(5,44) = 39.952, (P < 0.0001)], and xefocam [ANOVA: F(5,39) = 41.904, (P < 0.0001)]. On the first experimental day, the differences between treated and control groups were significant for analgin (t = 11.854, P < 0.001), for xefocam (t = 11.096, P < 0.001), for ketorolac (t = 9.652, P < 0.001), and clodifen (t = 8.813, P < 0.001) respectively (Figure 2A).
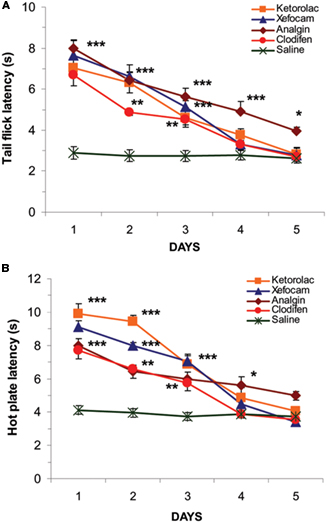
Figure 2. Microinjections of NSAIDs into the NRM for five consecutive days result in progressively decrease in TF (A) and HP (B) latencies as compared to vehicle saline control (*P < 0. 05, **P < 0.01, ***P < 0.001).
We revealed similar significant differences in the HP latencies for analgin [ANOVA: F(5,44) = 79.984, (P < 0.0001)], clodifen [ANOVA: F(5,39) = 33.024, (P < 0.0001)], ketorolac [ANOVA: F(5,44) = 68.839, (P < 0.0001)], and xefocam [ANOVA: F(5,39) = 67.945, (P < 0.0001)] respectively. On the first treatment day antinociception was maximal for each drugs for ketorolac (t = 15.172, P < 0.001), for xefocam (t = 13.047, P < 0.001), for analgin (t = 10.135, P < 0.001), and clodifen (t = 9.432, P < 0.001) respectively (Figure 2B). However, subsequent NSAIDs microinjections caused progressively less antinociception, so by day 5 there was no effect, i.e., we observed a similar effect to the vehicle control of saline microinjections for both the TF (Figure 2A) and the HP (Figure 2B) tests, except analgin in TF latency. The latter did not show complete tolerance even at the fifth experimental day (t = 5.2, P < 0.05; Figure 2A).
Opioid Sensitivity
Control experiments
Control experiments with saline microinjections into the NRM followed by the μ-opioid antagonist naloxone statistically did not change the latency to respond in the TF and HP tests for the first and second experimental days (P > 0.05; Figures 3A,B).
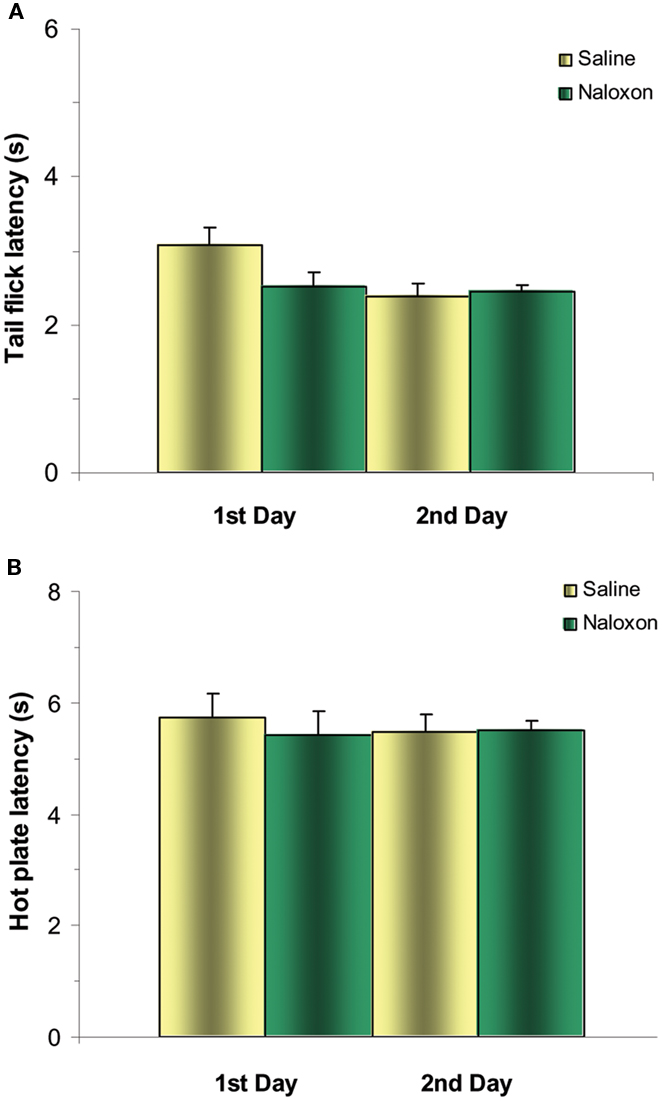
Figure 3. Control experiments of post-treatment with naloxone after microinjections of saline into NRM does not significantly change TF (A) and HP (B) latencies either for the first or second days. N = 4 per group.
Opioid sensitivity of NSAIDs
To examine in detail an opioid sensitivity of NSAIDs action we tested whether post-treatment with the μ-opioid antagonist naloxone into the NRM diminishes NSAID-induced antinociception on the first and second experimental days. Twenty minutes after NSAIDs testing, microinjection of naloxone into the NRM significantly decreased antinociceptive effects of NSAIDs on the first day in the TF for analgin (t = 5.432, P < 0.05), clodifen (t = 6.258, P < 0.01), ketorolac (t = 10.847, P < 0.001), and xefocam (t = 8.709, P < 0.001), respectively (Figure 4). The same effects we discovered in the HP test for analgin (t = 5.701, P < 0.05), clodifen (t = 5.287, P < 0.05), ketorolac (t = 7.303, P < 0.001), and xefocam (t = 5.64, P < 0.05), respectively (Figure 5). On the second day, naloxone showed trend effects in both TF and HP tests (Figures 4 and 5).
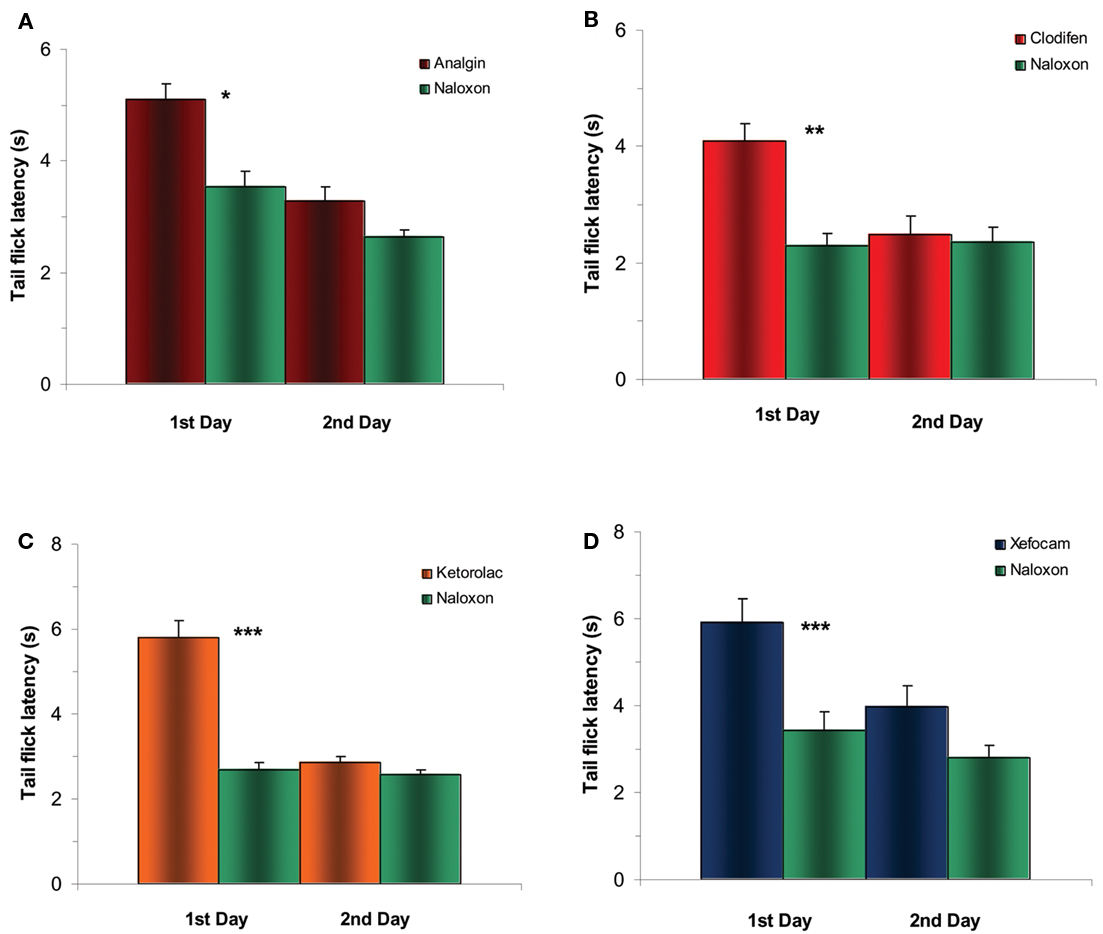
Figure 4. Experiments of post treatment with naloxone after microinjection of NSAIDs into NRM results in a significant decrease in TF latency for the first day for analgin (A), clodifen (B), ketorolac (C), and xefocam (D), respectively. On the second day, there are trend effects for all four non-opioid analgesics. N=4 per group. Here and in the next figure *P < 0.05, **P < 0.01, ***P < 0.001.
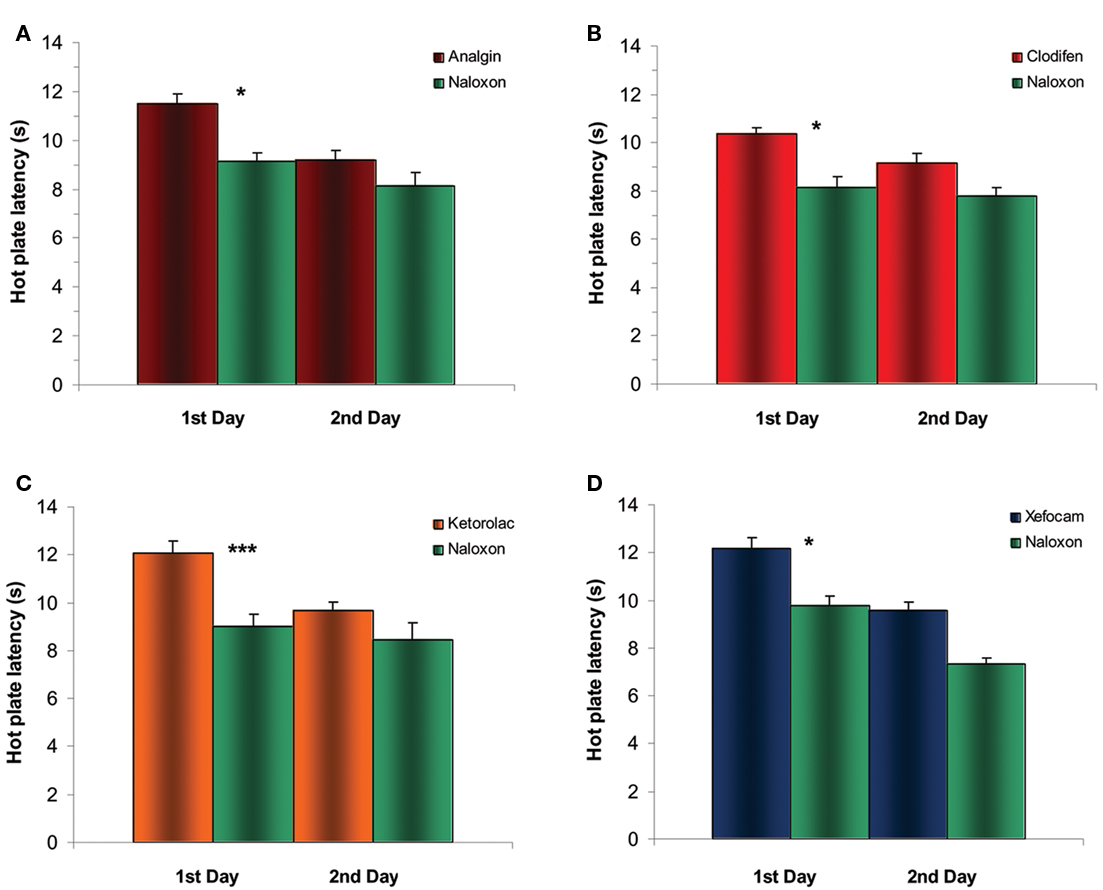
Figure 5. Experiments of post treatment with naloxone after microinjection of NSAIDs into NRM results in a significant decrease in HP latency for the first day for analgin (A), clodifen (B), ketorolac (C), and xefocam (D), respectively. On the second day, there are trend effects for all four non-opioid analgesics. N=4 per group.
Discussion
The obtained data revealed for the first time non-opioid tolerance to NSAIDs in the NRM. These results add evidence to the hypothesis that, like opioids, non-opioid analgesics, particularly NSAIDs analgin, clodifen, ketorolac, and xefocam induce tolerance, and also by mechanisms strongly related to the PAG (Tortorici and Vanegas, 2000; Tortorici et al., 2004, 2009). It has been shown that other non-opioid analgesics, e.g., aspirin also induce tolerance after repeated microinjections into the PAG (Vanegas and Tortorici, 2002; Pernia-Andrade et al., 2004). The PAG and its descending projections to the NRM are principal components of the descending antinociceptive pain-control system (Fields et al., 2006; Henricher and Ingram, 2009; Heinricher et al., 2009; Ren and Dubner, 2009). In naive rats, microinjection of morphine and dipyrone into the PAG decrease the activity of pain-facilitating “on-cells” and increase the activity of pain inhibiting “off-cells” thus giving rise to antinociception (Tortorici et al., 2001, 2009; Fields, 2004).
Although similar effects of antinociception can be produced by direct action of morphine upon the RVM (Henricher et al., 1992), tolerance to morphine was not remarkably obtained by repeated microinjection into the RVM (Morgan et al., 2005). Furthermore, inactivation of the RVM did not prevent the development of tolerance to repeated morphine microinjections into the PAG, and tolerance to systemic morphine did not develop if opioid receptors were blocked in the PAG even if the RVM remained intact (Lane et al., 2005). The PAG is thus crucial for tolerance to morphine as well as non-opioid NSAIDs such as metamizol, clodifen, ketorolac, aspirin, and xefocam (Vanegas and Tortorici, 2002; Tortorici et al., 2003, 2004, 2009). On the other hand, however, in this study we revealed for the first time tolerance induction to NSAIDs microinjected into NRM.
The mechanism producing tolerance to NSAIDs can be due to the participation of endogenous opioids, endorphins (Hernández-Delgadillo and Cruz, 2006; Tsiklauri et al., 2006; Henricher and Ingram, 2009; Vanegas et al., 2010). Herein we clearly showed that the μ-opioid antagonist naloxone diminishes NSAID-induced antinociception on the first and second experimental days and impedes the development of tolerance to the antinociceptive effect of NSAIDs. Our findings affirm the results of other investigators that microinjection of dipyrone and aspirin, and systemic dipyrone are abolished by systemically injected and/or microinjections of the selective μ-opioid antagonists, naloxone, and CTOP (D-phe-Cys-Tyr-D-trp-Orn-thr-Pen-thr-NH2; Pernia-Andrade et al., 2004; Hernández-Delgadillo and Cruz, 2006; Tortorici et al., 2009). The latter is a cyclic analog of the neuropeptide somatostatin and is known to block the analgesic effect of morphine (Tortorici et al., 2009). Moreover, endogenous opioids are involved in the potentiation of analgesia observed with the combination of morphine plus dipyrone (metamizol). The release of endogenous opioids by dipyrone could enhance exogenous opiate effects, explaining the need for the need for more naloxone to counteract the antinociception produced by morphine plus dipyrone (Hernández-Delgadillo and Cruz, 2006).
The mechanisms whereby NSAIDs, in general, engage endogenous opioids are not completely understood. There is a proposed model for the PAG where γ-amino-butyric acid (GABA) containing synapses act as the plausible site where NSAIDs could converge with endogenous opioids. The PAG output neurons that drive antinociception via downstream relays, like the NRM and RVM, are tonically inhibited by local GABA-ergic synapses (Henricher and Ingram, 2009; Vanegas et al., 2010). Endogenous opioids reduce presynaptic release of GABA in the PAG. Furthermore, activation of μ-opioid receptors in the PAG brings about an elevation of the intracellular concentration of arachidonic acid metabolites. One of the pathways leads to the formation of hepoxolins, which increase potassium conductance. This is turn hyperpolarizes the presynaptic GABA-ergic terminals and decreases GABA release (Vaugan, 1998). Disinhibition of PAG output neurons would thus increase and drive antinociception in the downstream loop of the PAG–RVM–spinal dorsal horn (Wessendorf et al., 2006; Morgan et al., 2008). For this pathway to function, however, an activation of μ-opioid receptors seems to be necessary, because naloxone or CTOP block the effect of PAG-microinjected metamizol or aspirin (Pernia-Andrade et al., 2004; Tortorici et al., 2009).
As mentioned above, the action of either opioid or non-opioid analgesics in the PAG leads to an excitation of PAG output neurons and this causes an activation of RVM off-cells and an inhibition of RVM on-cells, thus leading to antinociception (analgesia). When tolerance develops, PAG microinjections of morphine (Tortorici et al., 2001), or metamizol (Tortorici et al., 2009) are no longer capable of affecting RVM neurons and inducing analgesia. These results show further neuronal relationships between opioid and non-opioid analgesics as regards the descending pain-control and modulation system (Vanegas et al., 2010).
Conflict of Interest Statement
The authors declare that the research was conducted in the absence of any commercial or financial relationships that could be construed as a potential conflict of interest.
Acknowledgments
This research was supported by the grant from Georgian National Science Foundation (GNSF/ST07/6-234).
References
Fields, H. L., Basbaum, A. I., and Heinricher, M. M. (2006). “Central nervous system mechanisms of pain modulation,” in Wall and Mellzack’s Textbook of Pain, 5th Edn, eds S. B. McMahon and M. Koltzenburg (London: Elsevier), 125–143.
Henricher, M. M., and Ingram, S. L. (2009). “The brainstem and nociceptive modulation,” in Science of Pain, eds A. I. Basbaum and M. C. Bushnell (San Diego: Elsevier), 593–626.
Henricher, M. M., Morgan, M. M., and Fields, H. L. (1992). Direct and indirect actions of morphine on medullary neurons that modulate nociception. Neuroscience 48, 533–543.
Heinricher, M. M., Travares, I., Leith, J. L., and Lumb, B. M. (2009). Descending control of nociception: specificity, recruitment and plasticity. Brain Res. Rev. 60, 214–225.
Hernández-Delgadillo, G. P., and Cruz, S. L. (2006). Endogenous opioids are involved in morphine and dipyrone analgesic potentiation in the tail flick test in rats. Eur. J. Pharmacol. 546, 54–59.
Jones, S. L. (1996). Dipyrone into the nucleus raphe magnus inhibits the rat nociceptive tail flick reflex. Eur. J. Pharmacol. 318, 37–40.
Lane, D. A., Patel, P. A., and Morgan, M. M. (2005). Evidence for an intrinsic mechanism of anti-nociceptive tolerance within the ventrolateral periaqueductal gray of rats. Neuroscience 135, 227–234.
Morgan, M. M., Clayton, C. C., and Boyer-Quick, J. S. (2005). Differential susceptibility of the PAG and RVM to tolerance to the antinociceptive effect of morphine in the rat. Pain 113, 91–98.
Morgan, M. M., Whittier, K. L., Hegarty, D. M., and Aicher, S. A. (2008). Periaqueductal gray neurons project to spinally projecting GABAergic neurons in the rostral ventromedial medulla. Pain 140, 376–386.
Paxinos, G., and Watson, C. (1997). The Rat Brain in Stereotaxic Coordinates. San Diego: Academic Press.
Pernia-Andrade, A. J., Tortorici, V., and Venegas, H. (2004). Induction of opioid tolerance by lysine-acetylsalicylate in rats. Pain 111, 191–200.
Ren, K., and Dubner, R. (2009). “Descending control mechanisms,” in Science of Pain, eds A. I. Basbaum and M. C. Bushnell (San Diego: Elsevier), 723–762.
Tortorici, V., Aponte, Y., Acevedo, H., Nogueira, L., and Vanegas, H. (2009). Tolerance to non-opioid analgesics in PAG involves unresponsiveness of medullary pain-modulating neurons in male rats. Eur. J. Neurosci. 29, 1188–1196.
Tortorici, V., Morgan, M. M., and Vanegas, H. (2001). Tolerance to repeated microinjection of morphine into the periaqueductal gray is associated with changes in the behavior of off- and on-cells in the rostral ventromedial medulla. Pain 89, 237–244.
Tortorici, V., Nogueira, L., Aponte, Y., and Vanegas, H. (2004). Involvement of cholecystokinin in the opioid tolerance induced by dipyrone (metamizol) microinjections into the periaqueductal gray matter of rats. Pain 112, 113–120.
Tortorici, V., Nogueira, L., Salas, R., and Venegas, H. (2003). Involvement of local cholecystokinin in the tolerance induced by morphine microinjections into the periaqueductal gray of rats. Pain 102, 9–16.
Tortorici, V., and Vanegas, H. (2000). Opioid tolerance induced by metamizol (dipyrone) microinjections into the periaqueductal gray of rats. Eur. J. Neurosci. 12, 4074–4080.
Tsagareli, M. G., Tsiklauri, N., Gurtskaia, G., Nozadze, I., and Abzianidze, E. (2010). The central nucleus of amygdala is involved in tolerance to the antinociceptive effect of NSAIDs. Health 2, 64–68.
Tsagareli, M. G., Tsiklauri, N., Gurtskaia, G., Nozadze, I., Kandelaki, R., and Abzianidze, E. (2009). Tolerance effects induced by NSAIDs microinjections into the central nucleus of the amygdala in rats. Neurophysiology 41, 404–408.
Tsagareli, M. G., Tsiklauri, N., Lagidze, T., Gurtskaia, G., Berishvili, V., and Abzianidze, E. (2005). Tolerance induction by non-opioid analgesics in rats. Proc. Georgian Acad. Sci. Biol. Ser. A 31, 903–909.
Tsiklauri, N., Gurtskaia, G., and Tsagareli, M. G. (2006). Is endogenous opioid system involved in non-opioid analgesics tolerance? Georgian Med. News 8, 121–125.
Tsiklauri, N., Gurtskaia, G., and Tsagareli, M. G. (2008). Study of non-opioid analgesics tolerance in young and adult rats. Georgian Med. News 5, 40–44.
Tsiklauri, N., and Tsagareli, M. G. (2006). Non-opioid-induced tolerance in rats. Neurophysiology 38, 370–373.
Tsiklauri, N., Viatchenko-Karpinski, V., Voitenko, N., and Tsagareli, M. G. (2010). Non-opioid tolerance in juvenile and adult rats. Eur. J. Pharmacol. 629, 68–72.
Vanegas, H., and Tortorici, V. (2002). Opioidergic effects of non-opioid analgesics on the central nervous system. Cell. Mol. Neurobiol. 22, 655–661.
Vanegas, H., Vazquez, E., and Tortorici, V. N. (2010). NSAIDs, opioids, cannabinoids and the control of pain by the central nervous system. Pharmaceuticals 3, 1335–1347.
Vaugan, C. W. (1998). Enhancement of opioid inhibition of GABAergic synaptic transmission by cyclo-oxygenase inhibitors in rat periaqueductal gray neurons. Br. J. Pharmacol. 123, 1479–1481.
Wessendorf, M. W., Vaughan, C. W., and Vanegas, H. (2006). “Rethinking the PAG and RVM: supraspinal modulation of nociception by opioids and non-opioids,” in Proceeding 11th World Congress Pain (Seattle: IASP Press), 311–320.
Keywords: antinociception, hot plate test, non-opioid tolerance, non-steroidal anti-inflammatory drugs, tail-flick reflex
Citation: Tsagareli MG, Nozadze I, Tsiklauri N and Gurtskaia G (2011) Tolerance to non-opioid analgesics is opioid sensitive in the nucleus raphe magnus. Front. Neurosci. 5:92. doi: 10.3389/fnins.2011.00092
Received: 27 May 2011;
Accepted: 12 July 2011;
Published online: 26 July 2011.
Edited by:
Nick Andrews, Pfizer, UKCopyright: © 2011 Tsagareli, Nozadze, Tsiklauri and Gurtskaia. This is an open-access article subject to a non-exclusive license between the authors and Frontiers Media SA, which permits use, distribution and reproduction in other forums, provided the original authors and source are credited and other Frontiers conditions are complied with.
*Correspondence: Merab G. Tsagareli, Laboratory of Pain Research, Department of Neurophysiology, Beritashvili Institute of Physiology, Gotua Street 14, 0160 Tbilisi, Georgia. e-mail:dHNhZ2FyZWxpQGJpcGh5c2lvbC5nZQ==