- 1Department of Physical Education, Xiamen University of Technology, Xiamen, China
- 2School of Kinesiology, Beijing Sport University, Beijing, China
- 3School of Athletic Performance, Shanghai University of Sport, Shanghai, China
Background: Conventional research has asserted that cognitive function, particularly, response inhibition, is closely related to the inferior frontal cortex (IFC), dorsolateral prefrontal cortex (DLPFC), or orbital frontal cortex (OFC), which belong to the prefrontal cortex (PFC). Different targets of anodal or cathodal transcranial direct current stimulation (a-tDCS or c-tDCS) would affect the experimental results, but the stimulation of the same brain target would produce inconsistent findings.
Purpose: This study aimed to investigate the effects of a-tDCS and c-tDCS applied over the PFC for healthy populations on reactive and proactive control process compared with sham or no tDCS conditions, as assessed using the Stop-signal task (SST) and Go/NoGo (GNG) task performance.
Methods: This systematic review was performed following the Preferred Reporting Items for Systematic Reviews and Meta-Analysis guidelines. Search was conducted on Web of Science, Google Scholar, PubMed, Elsevier, Scopus, and Science Direct until March 2024. Studies that assessed the inhibitory control in SST or/and GNG tasks were included to achieve a homogenous sample.
Results: Fourteen studies were included for meta-analyses, which were performed for two outcome measures, namely, stop-signal reaction time (SSRT) and commission error (CE) rate. A-tDCS and c-tDCS over the PFC had significant ergogenic effects on SST performance (mean difference = −17.03, 95% CI [−24.62, −9.43], p < 0.0001; mean difference = −15.19, 95% CI [−19.82, −10.55], p < 0.00001), and that of a-tDCS had a positive effect on GNG task performance (mean difference = −1.42, 95% CI [−2.71, −0.14], p = 0.03).
Conclusion: This review confirmed the engagement of PFC tDCS in reactive and proactive inhibitory processes. Future research should increase sample size and implement personalized stimulus protocols.
1 Introduction
Response inhibition is an innate capability of human beings to suppress certain already initiated or planned actions as circumstances demand; this cognitive control process was mediated by a distributed neural network. As a brain mechanism in the context of cognitive process, executive functions play a role in optimizing and using cognitive resources to achieve a given goal (Fehring et al., 2019; Mansouri et al., 2009). Given their significance, enhancing executive functions through neuromodulation techniques has gained interest. Transcranial direct current stimulation (tDCS) is a non-invasive and easy-to-use neuromodulation technology; it has polar characteristics and immediate effects, which can induce facilitatory or inhibitory effects on the cerebral cortex by applying low-intensity direct current to a stimulated cortical region (Filmer et al., 2014). A recent study indicated that tDCS is considered a promising adjuvant therapeutic modality for human cognitive functions, such as response inhibition and impulse control (Li et al., 2019a). However, the equivocal and even contradictory cognitive outcome of tDCS in many neuropsychological disorders hampers the progress (Hoy and Fitzgerald, 2010).
Response inhibition was thought to be achieved by reducing the excitability of specific active brain regions and increasing the activity of inhibitory brain regions (Coxon et al., 2006). The ergogenic effects of tDCS on brain-related functioning have received widespread attention in healthy and clinical populations. Intending to effectively improve response inhibition capability, previous studies based on the anode-excitatory cathode-inhibitory physiological model noted that anodal tDCS (a-tDCS) over targeted areas of the brain, such as the dorsolateral prefrontal cortex (DLPFC), supplementary motor area (SMA), and inferior frontal cortex (IFC), can result in a sustained neural drive during manual inhibitory control task (Chen et al., 2021; Gabrielle et al., 2013; Kwon and Kwon, 2013; Sankarasubramanian et al., 2017; Swann et al., 2012; Cunillera et al., 2014). In the present study, cathodal tDCS (c-tDCS) was observed to have an ergogenic effect on cognitive performance (Chen et al., 2021; Schroeder and Plewnia, 2016; Weidacker et al., 2016; Wynn et al., 2019). More than five neuroimaging studies confirmed the neural correlates of response inhibition when using a-tDCS or c-tDCS over stimulation regions (Li et al., 2019b; Sehm et al., 2012; Amadi et al., 2014; Callan et al., 2016; Sankarasubramanian et al., 2017); the results consistently point out changes in functional connectivity and activation beyond the brain networks subserving cognitive control. Nevertheless, the rationale behind utilizing tDCS as a tool in many fields is that subliminal a-tDCS over targeted areas increases the excitability of the cerebral cortex by depolarizing the cell membrane then increasing firing rates, while that of c-tDCS induces the opposite effect by hyperpolarization then decreasing firing rates (Nitsche et al., 2009). Thus, the application of c-tDCS in the context of cognitive control tasks such as Go/NoGo (GNG) task and Stop-signal task (SST), has been questioned due to variable and even contradictory results (Stramaccia et al., 2015; Friehs and Frings, 2019; Dumont et al., 2018). These equivocal issues have appeared in a number of studies utilizing a-tDCS (Perrotta et al., 2021; Friehs and Frings, 2019; Stramaccia et al., 2015). Horvath et al. (2014) proposed that fundamental methodological heterogeneity in the application of tDCS may hinder its internal validity in increasing number of research. The interval time between experimental condition (a-tDCS or/and c-tDCS) and control condition (sham tDCS or no tDCS) is too arbitrary, ranging from a few days to several weeks (Dedoncker et al., 2016). Bell et al. (2020) argued that the differences in individualization and neurochemistry may be related to the variable results. In particular, Li et al. (2006) found gender differences in the effect of tDCS on a cognitive-motor task, and the cerebral cortex of the male showed greater activation compared with the female. Therefore, further optimization of the tDCS testing protocol should consistently reflect the ergogenic effects of tDCS in future studies.
As a probe of response inhibition, GNG and SST in clinical and basic neuroimaging research are increasingly used. GNG is an effective means of evaluating simple reaction-time (RT) tasks, the inhibition of motor response is driven internally (vs. the externally driven in the SST) in this task with a proactive control to a specific target in the inhibitory process (Cunillera et al., 2014; Molero-Chamizo et al., 2018; Weidacker et al., 2016). Participants must respond to frequently presented “Go” signals and withhold the response to “No-go” signals (Zheng et al., 2008), which requires adjusting the response threshold of the former stimulus to balance the go and stop processes. In general, low commission error (CE) rates in “No-go” signals have been interpreted as improved inhibitory control in GNG (Perrotta et al., 2021). In addition, SST is a classic behavioral response inhibition task based on the horse-race model, which is regarded as an elaboration of GNG (Band and Boxtel, 1999). As a typical choice RT task, SST mainly differs from GNG in terms of the time of reactive inhibitory process (Zheng et al., 2008). In SST, participants are required to withhold a response in the “stop process” (e.g., a sound or coloration of the arrow) that has already been triggered in the independent “go process” (e.g., left/right or up/down-pointing arrow) based on a stochastic model. The standard index for reflecting a more efficient inhibitory capacity in SST is the shorter value of the stop-signal reaction time (SSRT) (Logan et al., 1984). All the aforementioned studies report that both tasks can be used to evaluate the effect of tDCS on response inhibition.
This systematic review and meta-analysis has a twofold purpose. The primary aim is to evaluate the effect of a-tDCS and c-tDCS compared with sham-tDCS or no-tDCS on modulating executive functions in healthy populations, respectively. SST and GNG were recruited to sketch the framework by discussing and analyzing cognitive outcome measures considering offline effects. The secondary aim is to determine whether the response inhibition correlates of task performance in SST and GNG can be dissociated.
2 Materials and methods
2.1 Protocol and registration
This study was performed following the guidelines of the Preferred Reporting Items for Systematic Reviews and Meta-Analysis (PRISMA) for protocols (Moher et al., 2009), and the registration number in PROSPERO is CRD42024556058.
2.2 Study selection
The inclusion criteria of this review were as follows: (1) participants must be healthy adults without any psychiatric disorders or musculoskeletal injury; (2) the intervention consisted of at least one session of a-tDCS or/and c-tDCS, and the control group had to be sham-tDCS or no-tDCS; (3) unilateral anodal stimulation, single- or double-blind, randomized experimental design; (4) tDCS overed the prefrontal cortex of the brain; (5) implementation of behavioral tasks, such as SST or/and GNG; (6) measurement of “offline” behavioral changes; (7) English full-text studies published in peer-reviewed journals; and (8) outcome measures should include pre and post stimulation.
Web of Science, Google Scholar, PubMed, Elsevier, Scopus, and Science Direct were searched for appropriate studies published before March 2024. The key words included the following terms: “tDCS” OR “transcranial direct current stimulation” AND “Stop-signal task” OR “Go/no-go task” OR “response inhibition” OR “inhibitory control.” Further relevant studies were manually added by screening the reference sections of retrieved studies, conference papers, and previous reviews.
2.3 Data extraction
For each included study, the following data were extracted: (1) study design; (2) sample size and characteristics (gender, age, stimulation interval time); (3) intervention characteristics (duration, current density, electrode size and location); (4) number of sessions; (5) outcome measures (SSRT in the SST, CE rates in GNG); and (6) main results.
2.4 Quality assessment
The Physiotherapy Evidence Database (PEDro scale) was utilized to assess study quality because of its proven reliability and validity in evaluating randomized controlled trials and ensure a rigorous analysis of evidence in our systematic review and meta-analysis. Two authors (G.Y. and X.P.) independently rated each included study to assess the quality and risk of bias by using the checklist of the PEDro scale (de Morton, 2009); in case the PEDro scale of a certain study was inconsistent, the third experienced author was consulted to reach a consensus. The original scale contains 11 items, which can be used to determine the external (the first item) and internal (the subsequent criteria) validity of the included studies. The results of the PEDro scale evaluation are shown in Table 1. In the present study, we ignored the first question in the study, because it does not match the present purpose. The PEDro score (0–10) of each item was evaluated as poor (< 4), fair (≥ 4 and ≤ 5), good (≥ 6 and ≤ 8), excellent (≥ 9 and ≤ 10) (de Morton, 2009). The total score of each included study was collected for subsequent statistical analysis.
2.5 Statistical analysis
A separate meta-analysis was conducted considering a-tDCS or c-tDCS over the PFC and different tasks for investigating response inhibition by using Review Manager 5.4.1 (Copenhagen: The Nordic Cochrane Centre). Given that some studies used a multi-period stimulation protocol, we decided to extract only the data after the first stimulation. For all included studies requiring quantitative analyses, we entered the three values of mean, SD, and total to calculate the mean difference (MD) and 95% confidence interval (95% CI), which were weighted by inverse variance method using a random-effects model. Study heterogeneity was appraised through the Chi-squared test. The magnitude of the heterogeneity was also quantified using the I2 statistic, which was estimated as low (< 30%), moderate (≥ 30% and < 50%), substantial (≥ 50% and < 75%), and considerable (≥ 75% and ≤ 100%) heterogeneity (Higgins et al., 2024). In the case of uncertainty in the information and results provided by the authors and when the data could not be obtained from the figures, tables, and results section, a message would be sent to the first author or corresponding author(s) through e-mail or Research gate to request the mean ± SD of the expected result measurement. If the standard error (SE) was reported instead of SD in the studies, we would use a formula ( , where n is the number of participants in each group) to estimate it (Higgins et al., 2024).
3 Results
3.1 Overall
The electronic search yielded a total of 3,182 studies, of which 24 were included in the review. As shown in Figure 1, the selection process is visualized in the PRISMA flow diagram. After the removal of duplicates, 2,430 studies were manually reviewed. A total of 2,267 studies were excluded after assessing titles and abstracts. The full-text articles of the remaining 163 studies were screened based on the eligibility criteria. The 24 included studies comprised a total of 1,228 participants, which were used for qualitative synthesis. Fourteen studies (58.33%) enrolled 714 participants (58.14%) in quantitative synthesis. In the following sections, the effect of a-tDCS and c-tDCS on response inhibition compared with control group would be discussed in each task. The effect of c-tDCS on response inhibition was not used in the meta-analysis because only one eligible study was identified. In addition, the PEDro score of all included studies was between 7 and 9 range, indicating a quality above good level (Table 1).
3.2 Study characteristics
Four included studies (16.67%) had a total of 3 (6.67%) (Friehs and Frings, 2019), 5 (10%) (Friehs et al., 2021), 5 (12.5%) (Campanella et al., 2018), and 11 (14.10%) (Schroeder et al., 2022) dropouts. The actual sample sizes per study ranged from 11 to 124 participants (51.17 ± 32.71) aged from 18 to 35 years. The study included 484 males and 620 females, only one study lacked report on the gender of the participants (Bell et al., 2020). Three studies (10.53%) only recruited male participants (Campanella et al., 2017; Campanella et al., 2018; Dai et al., 2022), and one study only recruited female participants (Ghayebzadeh et al., 2023).
As shown in Table 2, a randomized design was performed, 16 (66.67%) were parallel and eight (33.33%) were crossover in all included studies. Twenty-three studies (95.83%) had a sham group as a comparator, and only one study (4.17%) had a no stimulation group as a comparator. The current intensity ranged from 0.5 mA to 2 mA, with a stimulation duration of 20.13 ± 5.82 min (ranging from 10–30 min). In terms of electrode placement, the right PFC was selected as the target area for a-tDCS in all but four studies (Fehring et al., 2019; Mansouri et al., 2016; Dai et al., 2022; Schroeder et al., 2022), which selected the left PFC as the stimulation area. Most c-tDCS was placed over the contralateral supraorbital area.
3.3 Effect of a-tDCS on response inhibition in SST and GNG tasks
The pooled analysis showed significant changes in response inhibition after single-session a-tDCS compared with the control group in SST and GNG tasks. Figure 2 summarizes the pooled data (size of SSRT) for SST extracted from nine studies on healthy populations. A significant effect in favor of a-tDCS (MD = −17.03 ms; 95%CI = −24.62, −9.43) was found from the random effects model (p < 0.0001), with a moderate heterogeneity (Chi2 = 24.83, p = 0.02 and I2 = 48%).
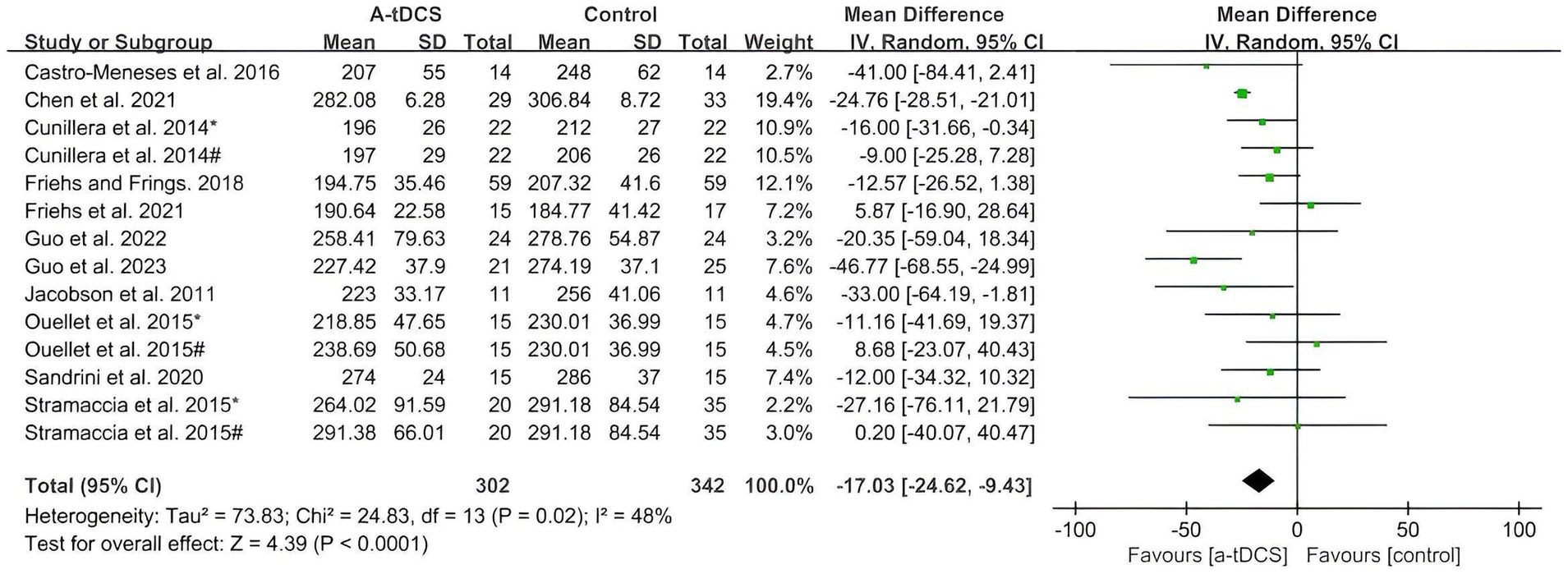
Figure 2. Meta-analysis of a-tDCS on response inhibition in SST task. Cunillera et al. (2014)*, easy discrimination condition; Cunillera et al. (2014)#, hard discrimination condition; Ouellet et al. (2015)*, left OFC group; Ouellet et al. (2015)#, right OFC group; Stramaccia et al. (2015)*, right IFC group; Stramaccia et al. (2015)#, right DLPFC group.
As shown in Figure 3, only four studies that analyzed the effect of a-tDCS on response inhibition were found in the GNG task. The pooled MD using the random effects model after a-tDCS was −1.42 (95%CI: −2.71, −0.14, p = 0.03), which indicated that the effects were significant with a low heterogeneity (Chi2 = 0.22, p = 0.97 and I2 = 0%).
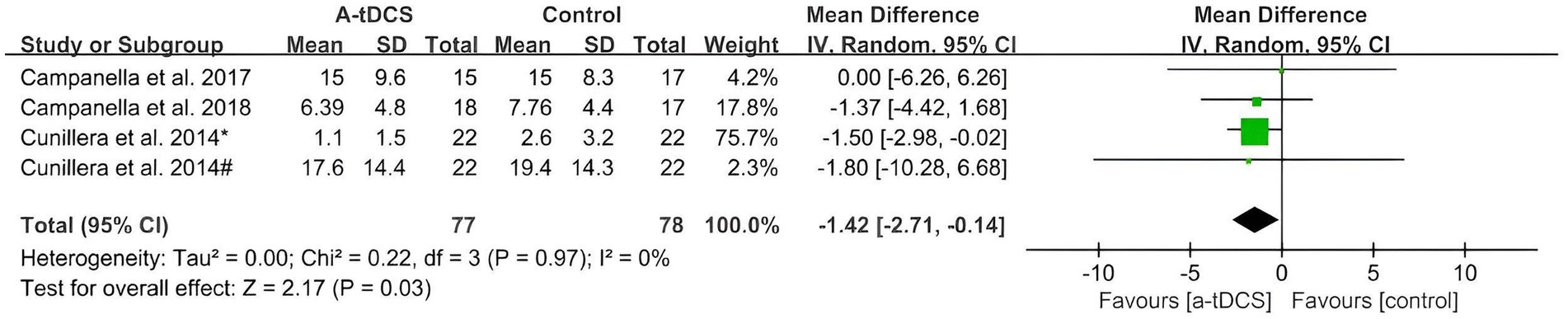
Figure 3. Meta-analysis of a-tDCS on response inhibition in GNG task. Ouellet et al. (2015)*, left OFC group; Ouellet et al. (2015)#.
3.4 Effect of c-tDCS on response inhibition in SST
The application of single-session c-tDCS over the PFC led to a significant effect at post-intervention compared with control group (Figure 4). The MD using the random effects model was −15.19 (95%CI: −19.82, −10.55, p < 0.00001) at post intervention with a low heterogeneity (Chi2 = 5.18, p = 0.39 and I2 = 4%).
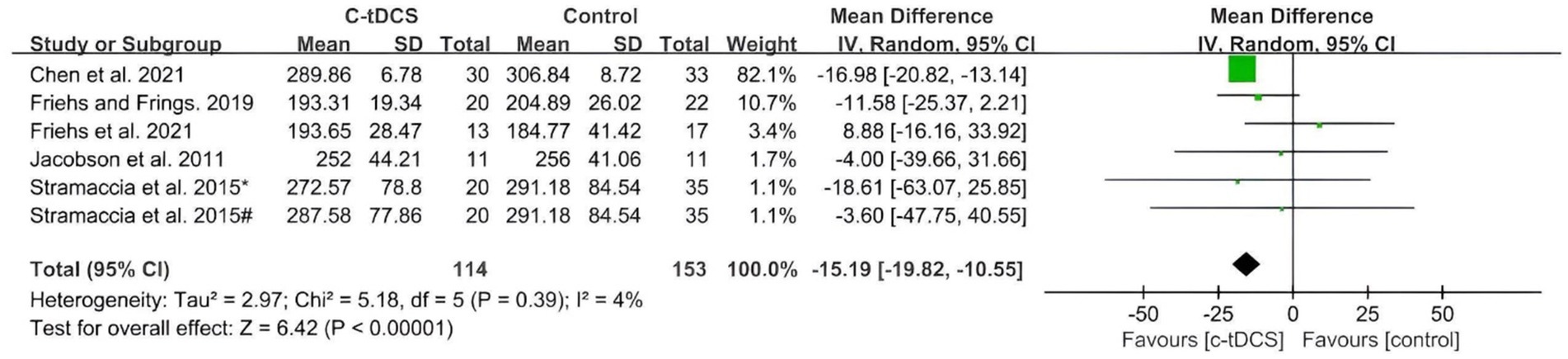
Figure 4. Meta-analysis of c-tDCS on response inhibition in SST task. Stramaccia et al. (2015)*, right IFC group; Stramaccia et al. (2015)#, right DLPFC group.
4 Discussion
This systematic review and meta-analysis aimed to determine the efficacy of a-tDCS and c-tDCS over the PFC regions for healthy populations on two common response inhibition tasks. Significant ergogenic effects were detected using tDCS compared with control groups (s-tDCS or no-tDCS) in SST and GNG tasks.
4.1 A-tDCS over the PFC
The promising outcomes of a-tDCS on modulation executive functioning tasks have recently attracted attention because of its ergogenic potential. As described previously, the right DLPFC is essential for goal-oriented cognitive control tasks (e.g., SST); related studies reported that a-tDCS over the right DLPFC may effectively improve cognitive inhibition processes (Chen et al., 2021; Friehs and Frings, 2018). A growing amount of evidence-based on fMRI studies has indicated that response inhibition is associated with the continuous activation of the right DLPFC that supports the stop process, especially in SST performance (Hughes et al., 2013; Hughes et al., 2014). Interestingly, a-tDCS over the left DLPFC has been confirmed to have a significant ergogenic effect on performing the cognitive task by comparing within (Fehring et al., 2019) and between groups (Mansouri et al., 2016). These findings proposed that a-tDCS can be used to change the susceptibility of the neurocircuitry to regulate cognitive behavior (Fehring et al., 2019). Additionally, accumulating evidence has shown that the right IFC plays a key role in the SST, and a-tDCS over this region can modulate brain activity and enhance functional connectivity to facilitate response inhibition (Cunillera et al., 2014; Jacobson et al., 2011; Sandrini et al., 2020). Functional neuroimaging studies also demonstrated that the right IFC has a large coincidence with a clear overlapped network for inhibitory processes (Chikazoe et al., 2009). Importantly, a-tDCS over the right IFC combined with cognitive training had a more substantial effect on response inhibition (Ditye et al., 2012). The combination of tDCS and cognitive training may emerge as a new method for enhancing cognitive function. However, in disagreement with the present findings, evidence of a previous meta-analysis showed a null effect on inhibitory control while performing a-tDCS over the right DLPFC (Schroeder et al., 2020). This discrepancy may arise from the fact that the previous study included not only healthy participants but also clinical populations. Additionally, the study found that electrode placement, particularly the position of return electrodes, exhibits distance-dependent effects that influence cortical excitability induction. A study has pointed out that the reverse effect of a-tDCS of PFC was mainly because of the differences in neurochemistry and personality for participants (Bell et al., 2020). On a practical and behavioral level, a study documented that the right IFC a-tDCS had a significantly better effect on response inhibition in a single design compared with the right DLPFC; thus, the right IFC is the most reliable brain stimulation target in SST (Stramaccia et al., 2015). The present meta-analysis demonstrated that in the response domain (e.g., SST), the effects of a-tDCS over the PFC studies were significantly larger than sham/no-tDCS groups with moderate heterogeneity.
As described previously, the effects of unilateral a-tDCS of the right IFC on proactive control were significantly increased compared with sham stimulation by testing the GNG task (Cunillera et al., 2014; Campanella et al., 2017; Leite et al., 2018), which may be due to the fact that the inhibition of the promotion response caused by tDCS was related to the decrease in theta band activity (Jacobson et al., 2012; Campanella et al., 2018). However, the anodal electrode was placed on the right IFC combining with left cathodal IFC tDCS, and its ergogenic effects disappeared. A previous study (Leite et al., 2018) speculated that the left IFC was also a particularly critical region in inhibitory control, and the cathodal electrode would weaken the activity of this region and affect inhibition response. The meta-analysis revealed that the application of a-tDCS over the right IFC for clinical populations (e.g., ADHD, major depression, and obesity) can significantly improve proactive inhibition.
To our knowledge, PFC plays an important role in the cognitive control of behavior. The main contribution of the present study is to show the ergogenic response inhibition effects of PFC a-tDCS on reactive and proactive inhibitory processes by means of the SST and GNG tasks, respectively.
4.2 C-tDCS over the PFC
Based on the neurophysiological evidence, cathodal offline tDCS can reduce the glutaminergic neuronal activity and the postsynaptic depolarization is weakened so that the cortex produces a long-term inhibitory effect (Stagg and Nitsche, 2011; Cooke and Bliss, 2006). Compared with a-tDCS in response inhibition, a previous study reported that the effect of c-tDCS was smaller in size (Friehs and Frings, 2018). A study by Friehs and Frings (Friehs and Frings, 2019) further showed that c-tDCS over the right DLPFC can diminish the inhibitory effects. By contrast, another study proved that the a-tDCS and c-tDCS of the right DLPFC had a decrease in SSRT for healthy individuals by testing the SST, which suggested that both were effective for response inhibition (Chen et al., 2021). The former pointed out that the inconsistent results could be due to the difference in study procedures (e.g., electrode size and placement, current density and time) and methodology (pre-post or group-between design) (Friehs and Frings, 2019). The possibility of the latter being able to produce an ergogenic result may be the use of a modified SST. Additionally, the latter believed that the significantly decreased SSRT after c-tDCS was due to its promotion of supraorbital activity and indirect adjustment of related frontal cortex through anatomical connections, such as DLPFC and ACC (Chen et al., 2021). Collectively, studies found that compared with sham stimulation, unilateral c-tDCS over the right IFC can reduce SSRT but did not generate a significant effect in SST (Jacobson et al., 2011; Stramaccia et al., 2015). In the included study, only one determined the positive effect of c-tDCS of the right PFC on inhibition response by testing the GNG task (Weidacker et al., 2016). This study concluded that c-tDCS may contribute to regulating the shift of excitatory glutamate to appropriate levels (Weidacker et al., 2016). According to increased shunting over the scalp, the response inhibition network is mainly contained in the right hemisphere (Menon, 2011; Bari and Robbins, 2013). Combined with the present meta-analysis findings, c-tDCS applied over the right PFC can effectively inhibit executive functions with a low heterogeneity under the premise of increasing the sample size. The findings suggest that c-tDCS over the right PFC may be beneficial for clinical populations with inhibitory control deficits. By reducing glutamatergic activity, c-tDCS could enhance response inhibition and could be a potential therapeutic approach for conditions characterized by impaired cognitive control.
4.3 Limitations
Several limitations of this study should be noted. First of all, the participants included in the studies were all healthy populations. Second, the meta-analysis did not perform subgroup analysis in specific regions of PFC. Third, this study did not make a detailed comparative discussion and analysis of stimulation parameters (e.g., current density, duration) and potential moderators (e.g., age, gender). Future studies should investigate the optimal interval time between experimental and control conditions to determine its effect on modulation effectiveness. Finally, the extracted output data were all unilateral offline tDCS after a single session. Future works should use bilateral online intervention to explore the long-term effects of tDCS.
5 Conclusion
This systematic review and meta-analysis can reveal the effects of tDCS on modulating executive functions in healthy populations. A-tDCS and c-tDCS can have significant ergogenic effects on SST and GNG tasks, with low to moderate heterogeneity. Specifically, this study affirmed the potentially reactive inhibitory effect of a/c-tDCS in the PFC by evaluating SSRT in SST, and the proactive inhibitory effect of a-tDCS by evaluating CE in GNG.
Data availability statement
The original contributions presented in the study are included in the article/supplementary material, further inquiries can be directed to the corresponding author.
Author contributions
GY: Conceptualization, Data curation, Investigation, Methodology, Software, Supervision, Validation, Writing – original draft, Writing – review & editing. XP: Formal analysis, Investigation, Methodology, Resources, Software, Supervision, Validation, Writing – original draft, Writing – review & editing. JL: Resources, Supervision, Validation, Visualization, Writing – original draft, Writing – review & editing. SZ: Funding acquisition, Project administration, Resources, Supervision, Writing – original draft, Writing – review & editing.
Funding
The author(s) declare that financial support was received for the research, authorship, and/or publication of this article. This study was funded by the national social science fund of China (no. 22ATY002).
Conflict of interest
The authors declare that the research was conducted in the absence of any commercial or financial relationships that could be construed as a potential conflict of interest.
Publisher’s note
All claims expressed in this article are solely those of the authors and do not necessarily represent those of their affiliated organizations, or those of the publisher, the editors and the reviewers. Any product that may be evaluated in this article, or claim that may be made by its manufacturer, is not guaranteed or endorsed by the publisher.
References
Amadi, U., Ilie, A., Johansen-Berg, H., and Stagg, C. J. (2014). Polarity-specific effects of motor transcranial direct current stimulation on fMRI resting state networks. NeuroImage 88, 155–161. doi: 10.1016/j.neuroimage.2013.11.037
Band, G., and Boxtel, G. (1999). Inhibitory motor control in stop paradigms: review and reinterpretation of neural mechanisms. Acta Psychol. 101, 179–211. doi: 10.1016/s0001-6918(99)00005-0
Bari, A., and Robbins, T. W. (2013). Inhibition and impulsivity: behavioral and neural basis of response control. Prog. Neurobiol. 108, 44–79. doi: 10.1016/j.pneurobio.2013.06.005
Bell, S. B., Turner, B., Sawaki, L., and DeWall, N. (2020). When brain stimulation backfires: the effects of prefrontal cortex stimulation on impulsivity. Soc. Cogn. Affect. Neurosci. 17, 101–108. doi: 10.1093/scan/nsaa049
Callan, D. E., Brian, F., Atsushi, W., and Raja, P. (2016). Simultaneous tDCS-fMRI identifies resting state networks correlated with visual search enhancement. Front. Hum. Neurosci. 10:72. doi: 10.3389/fnhum.2016.00072
Campanella, S., Schroder, E., Monnart, A., Vanderhasselt, M. A., Duprat, R., Rabijns, M., et al. (2017). Transcranial direct current stimulation over the right frontal inferior cortex decreases neural activity needed to achieve inhibition: a double-blind ERP study in a male population. Clin. EEG Neurosci. 48, 176–188. doi: 10.1177/1550059416645977
Campanella, S., Schroder, E., Vanderhasselt, M. A., Baeken, C., Kornreich, C., Verbanck, P., et al. (2018). Short-term impact of tDCS over the right inferior frontal cortex on impulsive responses in a go/no-go task. Clin. EEG Neurosci. 49, 398–406. doi: 10.1177/1550059418777404
Castro-Meneses, LJ, Johnson, BW, and Sowman, PF. (2016). Vocal response inhibition is enhanced by anodal tDCS over the right prefrontal cortex. Exp Brain Res. 234, 185–195. doi: 10.1007/s00221-015-4452-0
Chen, T., Wang, H., Wang, X., Zhu, C., Zhang, L., Wang, K., et al. (2021). Transcranial direct current stimulation of the right dorsolateral prefrontal cortex improves response inhibition. Int. J. Psychophysiol. 162, 34–39. doi: 10.1016/j.ijpsycho.2021.01.014
Chikazoe, J., Jimura, K., Hirose, S., Yamashita, K. I., Miyashita, Y., and Konishi, S. (2009). Preparation to inhibit a response complements response inhibition during performance of a stop-signal task. J. Neurosci. 29, 15870–15877. doi: 10.1523/JNEUROSCI.3645-09.2009
Cooke, S. F., and Bliss, T. V. (2006). Plasticity in the human central nervous system. Brain 129, 1659–1673. doi: 10.1093/brain/awl082
Coxon, J. P., Stinear, C. M., and Byblow, W. D. (2006). Intracortical inhibition during volitional inhibition of prepared action. J. Neurophysiol. 95, 3371–3383. doi: 10.1152/jn.01334.2005
Cunillera, T., Fuentemilla, L., Brignani, D., Cucurell, D., and Miniussi, C. (2014). A simultaneous modulation of reactive and proactive inhibition processes by anodal tdcs on the right inferior frontal cortex. PLoS One 9:e113537. doi: 10.1371/journal.pone.0113537
Dai, J., Wang, H., Yang, L., Wang, C., Cheng, S., Zhang, T., et al. (2022). The neuroelectrophysiological and behavioral effects of transcranial direct current stimulation on executive vigilance under a continuous monotonous condition. Front. Neurosci. 16:910457. doi: 10.3389/fnins.2022.910457
de Morton, N. A. (2009). The PEDro scale is a valid measure of the methodological quality of clinical trials: a demographic study. Aust. J. Physiother. 55, 129–133. doi: 10.1016/s0004-9514(09)70043-1
Dedoncker, J., Brunoni, A. R., Baeken, C., and Vanderhasselt, M. A. (2016). The effect of the interval-between-sessions on prefrontal transcranial direct current stimulation (tDCS) on cognitive outcomes: a systematic review and meta-analysis. J. Neural Transm. (Vienna) 123, 1159–1172. doi: 10.1007/s00702-016-1558-x
Ditye, T., Jacobson, L., Walsh, V., and Lavidor, M. (2012). Modulating behavioral inhibition by tDCS combined with cognitive training. Exp. Brain Res. 219, 363–368. doi: 10.1007/s00221-012-3098-4
Dumont, L., El Mouderrib, S., and Théoret, H. (2018). Randomized, crossover, sham-controlled, double-blind study of transcranial direct current stimulation of left DLPFC on executive functions. Restor. Neurol. Neurosci. 36, 755–766. doi: 10.3233/RNN-180872
Fehring, D. J., Illipparampil, R., Acevedo, N., Jaberzadeh, S., and Mansouri, F. A. (2019). Interaction of task-related learning and transcranial direct current stimulation of the prefrontal cortex in modulating executive functions. Neuropsychologia 131, 148–159. doi: 10.1016/j.neuropsychologia.2019.05.011
Filmer, H. L., Dux, P. E., and Mattingley, J. B. (2014). Applications of transcranial direct current stimulation for understanding brain function. Trends Neurosci. 37, 742–753. doi: 10.1016/j.tins.2014.08.003
Friehs, M. A., Brauner, L., and Frings, C. (2021). Dual-tDCS over the right prefrontal cortex does not modulate stop-signal task performance. Exp. Brain Res. 239, 811–820. doi: 10.1007/s00221-020-05995-5
Friehs, M. A., and Frings, C. (2018). Pimping inhibition: anodal tDCS enhances stop-signal reaction time. J. Exp. Psychol. Hum. Percept. Perform. 44, 1933–1945. doi: 10.1037/xhp0000579
Friehs, M. A., and Frings, C. (2019). Cathodal tDCS increases stop-signal reaction time. Cogn. Affect. Behav. Neurosci. 19, 1129–1142. doi: 10.3758/s13415-019-00740-0
Gabrielle, H. C., Drummond, N. M., and Carlsen, A. N. (2013). Anodal tDCS over SMA decreases the probability of withholding an anticipated action. Behav. Brain Res. 257, 208–214. doi: 10.1016/j.bbr.2013.09.030
Ghayebzadeh, S., Zardoshtian, S., Amiri, E., Giboin, L. S., and Machado, D. G. D. S. (2023). Anodal transcranial direct current stimulation over the right dorsolateral prefrontal cortex boosts decision making and functional impulsivityi n female sports referees. Life 13:1131. doi: 10.3390/life13051131
Guo, Z, Gong, Y, Lu, H, Qiu, R, Wang, X, Zhu, X, et al. (2022). Multitarget high-definition transcranial direct current stimulation improves response inhibition more than single-target high-definition transcranial direct current stimulation in healthy participants. Front Neurosci. 16:905247. doi: 10.3389/fnins.2022.905247
Guo, Z., Qiu, R., Qiu, H., Lu, H., and Zhu, X. (2023). Long-term effects of repeated multitarget high-definition transcranial direct current stimulation combined with cognitive training on response inhibition gains. Front Neurosci. 17:1107116. doi: 10.3389/fnins.2023.1107116v
Higgins, JPT, Thomas, J, Chandler, J, Cumpston, M, Li, T, Page, MJ, et al. (editors). Cochrane Handbook for Systematic Reviews of Interventions version 6.5 (updated August 2024). Cochrane, (2024). Available at: https://training.cochrane.org/handbook
Horvath, J. C., Olivia, C., and Forte, J. D. (2014). Transcranial direct current stimulation: five important issues we aren't discussing (but probably should be). Front. Syst. Neurosci. 8:2. doi: 10.3389/fnsys.2014.00002
Hoy, K. E., and Fitzgerald, P. B. (2010). Brain stimulation in psychiatry and its effects on cognition. Nat. Rev. Neurol. 6, 267–275. doi: 10.1038/nrneurol.2010.30
Hughes, M. E., Budd, T. W., Fulham, W. R., Lancaster, S., Woods, W., Rossell, S. L., et al. (2014). Sustained brain activation supporting stop-signal task performance. Eur. J. Neurosci. 39, 1363–1369. doi: 10.1111/ejn.12497
Hughes, M. E., Johnston, P. J., Fulham, W. R., Budd, T. W., and Michie, P. T. (2013). Stop-signal task difficulty and the right inferior frontal gyrus. Behav. Brain Res. 256, 205–213. doi: 10.1016/j.bbr.2013.08.026
Jacobson, L., Ezra, A., Berger, U., and Lavidor, M. (2012). Modulating oscillatory brain activity correlates of behavioral inhibition using transcranial direct current stimulation. Clin. Neurophysiol. 123, 979–984. doi: 10.1016/j.clinph.2011.09.016
Jacobson, L., Javitt, D. C., and Lavidor, M. (2011). Activation of inhibition: diminishing impulsive behavior by direct current stimulation over the inferior frontal gyrus. J. Cogn. Neurosci. 23, 3380–3387. doi: 10.1162/jocn_a_00020
Kwon, Y. H., and Kwon, J. W. (2013). Is transcranial direct current stimulation a potential method for improving response inhibition? Neural Regen. Res. 8, 1048–1054. doi: 10.3969/j.issn.1673-5374.2013.11.01
Leite, J., Gonçalves, Ó. F., Pereira, P., Khadka, N., Bikson, M., Fregni, F., et al. (2018). The differential effects of unihemispheric and bihemispheric tDCS over the inferior frontal gyrus on proactive control. Neurosci. Res. 130, 39–46. doi: 10.1016/j.neures.2017.08.005
León, J. J., Sánchez-Kuhn, A, Fernández-Martín, P, Páez-Pérez, M. A., Thomas, C, Datta, A, et al. (2020). Transcranial direct current stimulation improves risky decision making in women but not in men: A sham-controlled study. Behav Brain Res. 382:112485. doi: 10.1016/j.bbr.2020.112485
Li, C. S., Huang, C., Constable, R. T., and Sinha, R. (2006). Gender differences in the neural correlates of response inhibition during a stop signal task. NeuroImage 32, 1918–1929. doi: 10.1016/j.neuroimage.2006.05.017
Li, L. M., Violante, I. R., Leech, R., Hampshire, A., Opitz, A., McArthur, D., et al. (2019a). Cognitive enhancement with salience network electrical stimulation is influenced by network structural connectivity. NeuroImage 185, 425–433. doi: 10.1016/j.neuroimage.2018.10.069
Li, L. M., Violante, I. R., Zimmerman, K., Leech, R., and Sharp, D. J. (2019b). Traumatic axonal injury influences the cognitive effect of non-invasive brain stimulation. Brain 142, 3280–3293. doi: 10.1093/brain/awz252
Logan, G. D., Van Zandt, T., Verbruggen, F., and Wagenmakers, E. J. (1984). On the ability to inhibit thought and action: a theory of an act of control. Psychol. Rev. 91, 295–327. doi: 10.1037/0033-295X.91.3.295
Mansouri, F. A., Fehring, D. J., Feizpour, A., Gaillard, A., Rosa, M. G., Rajan, R., et al. (2016). Direct current stimulation of prefrontal cortex modulates error-induced behavioral adjustments. Eur. J. Neurosci. 44, 1856–1869. doi: 10.1111/ejn.13281
Mansouri, F. A., Tanaka, K., and Buckley, M. J. (2009). Conflict-induced behavioural adjustment: a clue to the executive functions of the prefrontal cortex. Nat. Rev. Neurosci. 10, 141–152. doi: 10.1038/nrn2538
Menon, V. (2011). Large-scale brain networks and psychopathology: a unifying triple network model. Trends Cogn. Sci. 15, 483–506. doi: 10.1016/j.tics.2011.08.003
Moher, D., Liberati, A., Tetzlaff, J., and Altman, D. G. (2009). Preferred reporting items for systematic reviews and meta-analyses: the PRISMA statement. PLoS Med. 6:e1000097. doi: 10.1371/journal.pmed.1000097
Molero-Chamizo, A., Alameda Bailén, J. R., Garrido Béjar, T., García López, M., Jaén Rodríguez, I., Gutiérrez Lérida, C., et al. (2018). Poststimulation time interval-dependent effects of motor cortex anodal tDCS on reaction-time task performance. Cogn. Affect. Behav. Neurosci. 18, 167–175. doi: 10.3758/s13415-018-0561-0
Nitsche, M. A., Boggio, P. S., Fregni, F., and Pascual-Leone, A. (2009). Treatment of depression with transcranial direct current stimulation (tDCS): a review. Exp. Neurol. 219, 14–19. doi: 10.1016/j.expneurol.2009.03.038
Ouellet, J, McGirr, A, Van den Eynde, F, Jollant, F, Lepage, M, and Berlim, MT. (2015). Enhancing decision-making and cognitive impulse control with transcranial direct current stimulation (tDCS) applied over the orbitofrontal cortex (OFC): A randomized and sham-controlled exploratory study. J Psychiatr Res. 69, 27–34. doi: 10.1016/j.jpsychires.2015.07.018
Perrotta, D., Bianco, V., Berchicci, M., Quinzi, F., and Perri, R. L. (2021). Anodal tDCS over the dorsolateral prefrontal cortex reduces Stroop errors. A comparison of different tasks and designs. Behav. Brain Res. 405:113215. doi: 10.1016/j.bbr.2021.113215
Sandrini, M., Xu, B., Volochayev, R., Awosika, O., Wang, W. T., Butman, L. G., et al. (2020). Transcranial direct current stimulation facilitates response inhibition through dynamic modulation of the fronto-basal ganglia network. Brain Stimul. 13, 96–104. doi: 10.1016/j.brs.2019.08.004
Sankarasubramanian, V., Cunningham, D. A., Potter-Baker, K. A., Beall, E. B., Roelle, S. M., Varnerin, N. M., et al. (2017). Transcranial direct current stimulation targeting primary motor versus dorsolateral prefrontal cortices: proof-of-concept study investigating functional connectivity of thalamocortical networks specific to sensory-affective information processing. Brain Connect. 7, 182–196. doi: 10.1089/brain.2016.0440
Schroeder, P. A., and Plewnia, C. (2016). Beneficial effects of cathodal transcranial direct current stimulation (tDCS) on cognitive performance. J Cogn Enhance 1, 5–9. doi: 10.31234/osf.io/xakd4
Schroeder, P. A., Schwippel, T., Wolz, I., and Svaldi, J. (2020). Meta-analysis of the effects of transcranial direct current stimulation on inhibitory control. Brain Stimul. 13, 1159–1167. doi: 10.1016/j.brs.2020.05.006
Schroeder, P. A., Seewald, A., and Svaldi, J. (2022). Spotlight on the left frontal cortex: no evidence for response inhibition from cathodal high-definition tDCS over left IFG or left DLPFC. J. Cogn. Neurosci. 34, 1090–1102. doi: 10.1162/jocn_a_01849
Sehm, B., Schafer, A., Kipping, J., Margulies, D., Conde, V., Taubert, M., et al. (2012). Dynamic modulation of intrinsic functional connectivity by transcranial direct current stimulation. J. Neurophysiol. 108, 3253–3263. doi: 10.1152/jn.00606.2012
Stagg, C. J., and Nitsche, M. A. (2011). Physiological basis of transcranial direct current stimulation. Neuroscientist 17, 37–53. doi: 10.1177/1073858410386614
Stramaccia, D. F., Penolazzi, B., Sartori, G., Braga, M., Mondini, S., and Galfano, G. (2015). Assessing the effects of tDCS over a delayed response inhibition task by targeting the right inferior frontal gyrus and right dorsolateral prefrontal cortex. Exp. Brain Res. 233, 2283–2290. doi: 10.1007/s00221-015-4297-6
Swann, N. C., Cai, W., Conner, C. R., Pieters, T. A., Claffey, M. P., George, J. S., et al. (2012). Roles for the pre-supplementary motor area and the right inferior frontal gyrus in stopping action: electrophysiological responses and functional and structural connectivity. NeuroImage 59, 2860–2870. doi: 10.1016/j.neuroimage.2011.09.049
Weidacker, K., Weidemann, C. T., Boy, F., and Johnston, S. J. (2016). Cathodal tDCS improves task performance in participants high in Coldheartedness. Clin. Neurophysiol. 127, 3102–3109. doi: 10.1016/j.clinph.2016.05.274
Wynn, S., Driessen, J. M. A., Glennon, J. C., Brazil, I. A., and Schutter, D. J. L. G. (2019). Cerebellar transcranial direct current stimulation improves reactive response inhibition in healthy volunteers. Cerebellum 18, 983–988. doi: 10.1007/s12311-019-01047-z
Keywords: response inhibition, prefrontal cortex, neural activity, stop-signal task, go/nogo task
Citation: You G, Pan X, Li J and Zhao S (2024) Effects of transcranial direct current stimulation on modulating executive functions in healthy populations: a systematic review and meta-analysis. Front. Hum. Neurosci. 18:1485037. doi: 10.3389/fnhum.2024.1485037
Edited by:
Mengze Xu, Beijing Normal University, ChinaReviewed by:
Shi-Yang Xu, Shanxi Normal University, ChinaXinglin Zeng, University of Maryland, United States
Copyright © 2024 You, Pan, Li and Zhao. This is an open-access article distributed under the terms of the Creative Commons Attribution License (CC BY). The use, distribution or reproduction in other forums is permitted, provided the original author(s) and the copyright owner(s) are credited and that the original publication in this journal is cited, in accordance with accepted academic practice. No use, distribution or reproduction is permitted which does not comply with these terms.
*Correspondence: Shaocong Zhao, c2hjemhhb0B4bXV0LmVkdS5jbg==