- VIE, Inc., Kamakura, Japan
A frequency range exceeding approximately 30 Hz, denoted as the gamma frequency range, is associated with various cognitive functions, consciousness, sensory integration, short-term memory, working memory, encoding and maintenance of episodic memory, and retrieval processes. In this study, we proposed a new form of gamma stimulation, called gamma music, combining 40 Hz auditory stimuli and music. This gamma music consists of drums, bass, and keyboard sounds, each containing a 40 Hz frequency oscillation. Since 40 Hz stimuli are known to induce an auditory steady-state response (ASSR), we used the 40 Hz power and phase locking index (PLI) as indices of neural activity during sound stimulation. We also recorded subjective ratings of each sound through a questionnaire using a visual analog scale. The gamma music, gamma drums, gamma bass, and gamma keyboard sounds showed significantly higher values in 40 Hz power and PLI compared to the control music without a 40 Hz oscillation. Particularly, the gamma keyboard sound showed a potential to induce strong ASSR, showing high values in these indices. In the subjective ratings, the gamma music, especially the gamma keyboard sound, received more relaxed, comfortable, preferred, pleasant, and natural impressions compared to the control music with conventional gamma stimulation. These results indicate that our proposed gamma music has potential as a new method for inducing ASSR. Particularly, the gamma keyboard sound proved to be an effective acoustic source for inducing a strong ASSR while preserving the comfortable and pleasant sensation of listening to music. Our developed gamma music, characterized by its pleasantness to the human ear, offers a significant advantage for the long-term use of gamma stimulation. The utilization of this music could potentially reduce the physical and psychological burden on participants compared to conventional 40 Hz stimuli. This music is not only expected to contribute to fundamental neuroscience research utilizing ASSR but also to facilitate the implementation of gamma music-based interventions aimed at enhancing human cognitive functions in everyday life.
1 Introduction
Human electroencephalogram (EEG) signals are designated as discrete frequency ranges, each correlating with distinct neural activity. Notably, a frequency range exceeding approximately 30 Hz, denoted as the gamma frequency range, is associated with various cognitive functions, consciousness, sensory integration, short-term memory, working memory, encoding and maintenance of episodic memory, and retrieval processes (Tallon-Baudry et al., 1998; Gruber et al., 2004; Herrmann et al., 2004; Jensen and Lisman, 2005; Mormann et al., 2005; Osipova et al., 2006; Fries et al., 2007; Lisman, 2010; Shirvalkar et al., 2010; Kucewicz et al., 2017; Griffiths et al., 2019). For instance, the amplitude of gamma frequency activity increases during the encoding of successfully memorized words compared to words that cannot be memorized (Gruber et al., 2004). In alternative instances, gamma frequency activity is detected during the preservation of visual stimuli in a short-term memory task (Tallon-Baudry et al., 1998), and the magnitude of gamma frequency activity is augmented commensurately with the number of items maintained in working memory (Howard et al., 2003; Van Vugt et al., 2010; Roux et al., 2012). Modulation of gamma frequency activity also has been reported in various neuropsychiatric disorders, including Alzheimer’s disease and schizophrenia (Herrmann and Demiralp, 2005; Mathalon and Sohal, 2015). Patients with Alzheimer’s disease exhibit reduced functional synchronization in gamma frequency activity during the resting state (Stam et al., 2002; Koenig et al., 2005) and protracted latencies in gamma frequency activity compared with healthy subjects (Başar et al., 2016). Similarly, during a series of cognitive tasks, including attention, memory, and object representation, gamma frequency activity in patients with schizophrenia is attenuated compared with healthy subjects (Shin et al., 2011). For instance, when presented with perceptually ambiguous images, also known as Mooney’s faces, gamma frequency activity in patients with schizophrenia is more attenuated than that in healthy subjects (Uhlhaas et al., 2006).
Numerous studies have reported that modulating neural activity within the brain during cognitive tasks can be observed as oscillations in the gamma frequency range. Conversely, in recent years, it has been reported that the presentation of stimuli possessing gamma frequency power can modulate cognitive processes within the brain. In a study involving mice, visual gamma frequency stimulation altered synaptic signaling and enhanced spatial learning and memory capabilities (Adaikkan et al., 2019). Similarly, visual and auditory gamma frequency stimulation has demonstrated the potential to improve Alzheimer’s disease biomarkers in mice, suggesting a possible alleviation of disease severity (Iaccarino et al., 2016; Martorell et al., 2019).
Presentation of periodic sensory stimuli amplifies the oscillatory activity corresponding to the stimulus frequency; this component is generally referred to as a steady-state evoked potential. The steady-state evoked potentials induced by auditory stimuli are commonly known as auditory steady-state responses (ASSR). ASSR is reportedly strongly evoked by a 40 Hz auditory stimulus (Galambos et al., 1981; Ross et al., 2000). The 40 Hz ASSR is modulated by various cognitive functions, such as human arousal levels (Griskova et al., 2007; Górska and Binder, 2019), attention levels (Skosnik et al., 2007; Gander et al., 2010; Voicikas et al., 2016), and workload (Yokota and Naruse, 2015; Yokota et al., 2017), as well as pathological symptoms such as schizophrenia (Kwon et al., 1999; O’Donnell et al., 2013; Thuné et al., 2016). Considering its impact on various cognitive functions, the magnitude of the ASSR elicited by the presentation of 40 Hz auditory stimuli holds potential as a biomarker for evaluating the degree of human cognitive function. The potential of 40 Hz stimulation to alleviate Alzheimer’s symptoms has been demonstrated previously. A 40 Hz amalgamated visual and auditory stimulation demonstrated diminished brain atrophy, attenuated loss of functional connectivity, and improved performance on an associative memory task (Chan et al., 2022). Since humans generally perceive simple 40 Hz auditory stimuli as unpleasant sounds (Fastl, 1990), this presents a considerable impediment to the social application of technologies that employ 40 Hz auditory stimuli. One potential approach to mitigate the unpleasantness associated with 40 Hz stimulation and enable humans to endure prolonged exposure is the integration of 40 Hz stimulation with music.
Listening to music is an emotive experience for many individuals, and it inherently functions as an enticing sensory stimulus (Zatorre and Salimpoor, 2013; Koelsch, 2014). One study reported that music-based interventions in patients with dementia improved their quality of life and emotional wellbeing (van der Steen et al., 2018). The integration of gamma stimulation technologies with music has been proposed and referred to as Gamma-MBI (music-based interventions) by Tichko et al., 2022. Tichko et al. (2022) argued that incorporating 40 Hz gamma frequency sounds into existing music presents challenges from the perspective of musical harmony because adding 40 Hz auditory stimuli to existing music can create an uncomfortable and unpleasant listening experience (see also Fastl, 1990). For example, in a study by Martorell et al. (2019), a sequence of tones repeating at 40 Hz (i.e., 1 ms long, 10 kHz tones played every 25 ms inter-tone intervals) was used as gamma auditory stimulus to ameliorate Alzheimer’s-associated pathology and enhance cognitive function. Since conventional gamma auditory stimulation is typically perceived as rough and unpleasant by listeners, it has been challenging to incorporate this stimulation into music while preserving the comfortable and pleasant sensation of listening (Tichko et al., 2022).
We conjectured that synthesizing the timbre of musical instruments, such as drum-kit sounds from 40 Hz oscillations—and modifying the amplitude envelope of musical instrument sounds, such as bass and keyboard sounds, into the gamma frequency range could solve this issue. Music can be divided into three fundamental elements: rhythm, melody, and harmony. Each of these elements has been reported to be associated with human perception, prediction, and emotional functioning (Vuust et al., 2022). We hypothesized that 40 Hz gamma auditory stimulation could be effectively integrated with these elements by creating a timbre of drum-kit sounds from gamma frequency tones and modifying the amplitude envelope of bass and keyboard sounds into the gamma frequency range. Accordingly, the gamma music used in this study was therefore composed of three musical instruments: a drum-kit, a bass guitar, and a keyboard, each representing the rhythm, melody, and harmony of music, respectively. The drum-kit sounds consisted of a kick drum, a snare drum, and a hi-hat sound. We synthesized each drum-kit sound from the gamma frequency tones, and termed them “gamma drums.” These three drum sounds were originally designed for this specific study by synthesizing them from a 40 Hz oscillatory component, with controlled envelopes to reproduce traditional drum sounds, along with noise sounds to enhance the percussive quality of the sounds (see section “2 Materials and methods” for details). The bass guitar and keyboard sounds provide melody in the lower registers and harmony in the higher registers, respectively. We supplemented a 40 Hz frequency component into the bass and keyboard sounds by using a Low-Frequency Oscillator (LFO) and termed these sounds “gamma bass” and “gamma keyboard,” respectively. As a control condition for gamma music, we used musical stimuli termed “control music” composed of drums, bass, and keyboard sounds that did not include a strong power at or around the 40 Hz frequency range. Additionally, as a third condition, we used the conventional gamma stimulation used by Martorell et al. (2019) and added it to the control music, terming it the “control music with conventional gamma stimulation (Conv. Gamma).”
We hypothesized that ASSR is elicited during the presentation of gamma music, gamma drums, gamma bass, gamma keyboard, and Conv. Gamma but not during the presentation of control music. We also hypothesized that the subjective ratings of gamma music, gamma drums, gamma bass, gamma keyboard, and control music are more comfortable and pleasant than those of Conv. Gamma. In assessing the activity of the ASSR, commonly used indicators are power response, representing amplitude values, and phase synchronization across trials (measured through the phase-locking index, PLI), representing phase values. We used the power response and PLI for 40 Hz as indicators of ASSR. Most prior research has employed either one or both of these indicators (Parciauskaite et al., 2021). Additionally, we collected subjective ratings of the auditory stimuli from the participants using a Visual Analog Scale (VAS). Using these measurements of 40 Hz ASSR power, PLI, and subjective rating scales, we assessed the effectiveness of gamma music.
2 Materials and methods
2.1 Participants
Seventeen individuals (nine men and eight women; age range: 20–41 years) participated in this study. All the participants had normal hearing. Prior to the experiment, the participants received a thorough explanation of the procedural details and provided written informed consent. This study was approved by the Shiba Palace Clinic Ethics Review Committee (Approval number: 152353_rn-35200) and conducted in accordance with the ethical principles outlined in the Declaration of Helsinki.
2.2 Stimuli
One of the authors (KT) composed an original piece 196 s duration for this study using Ableton Live software (Ableton AG, Berlin, Germany). The piece was composed of MIDI signals and consisted of three musical-instrument tracks: a drum-kit, a bass guitar, and a keyboard. We utilized the “505 Core Kit,” “Basic Fine Line Bass,” and “Drifting Ambient Pad” preset sounds in Ableton Live to play each of the drums, bass, and keyboard MIDI tracks, respectively. Each audio track was output from Ableton Live and saved as a WAV audio file at a sampling frequency of 44,100 Hz. We referred to these output audio files as the “control drums,” “control bass,” and “control keyboard,” respectively. The “control music” was created by mixing these control drums, bass, and keyboard sounds.
Next, the control bass and keyboard sounds were loaded using MATLAB 2023 a software (MathWorks, Inc., Natick, MA, United States). We modified the amplitude envelope of each sound to 40 Hz using a custom-written program in MATLAB. We referred to these modified sounds as the “gamma bass” and “gamma keyboard,” respectively. As for the “gamma drums,” we created the bass drum, snare drum, and high-hat sounds from scratch using MATLAB. The bass drum sound had a carrier frequency of 40 Hz. The amplitude underwent decay and was supplemented with high-frequency noise during the attack phase to create a kick sound timbre. The snare sound has a carrier frequency of 40 Hz, and the amplitude underwent decay with the addition of bandpass noise to create a snare sound timbre. The high-hat sound was created by modifying the conventional gamma stimuli used by Martorell et al. (2019). The amplitude envelope of a train of gamma tones was decayed to create a high-hat timbre. The bass drum, snare drum, and high-hat sounds were saved as WAV audio files at a sampling frequency of 44,100 Hz in MATLAB and then loaded into Ableton Live to play the drum MIDI track. The output from Ableton Live was subsequently saved as a WAV audio file at a sampling frequency of 44,100 Hz. This drum-kit sound was referred to as the “gamma drums.” The “gamma music” was created by mixing these gamma drums, bass, and keyboard sounds. We also created conventional gamma tones using MATLAB software, based on the report by Martorell et al. (2019). We created a 1 ms long, 10 kHz tone played every 25 ms inter-tone interval, with a total duration of 196 s, and a sampling frequency of 44,100 Hz. The Conv. Gamma was created by mixing gamma tones with control music.
A total of six auditory stimuli were created: (1) gamma music, (2) gamma drums, (3) gamma bass, (4) gamma keyboard, (5) control music, and (6) Conv. Gamma. Perceptual loudness of all six stimuli was adjusted using the integratedLoudness function in MATLAB, performing loudness normalization in accordance with the EBU R 128 Standard with a target loudness level of—23 LUFS. All stimuli were configured to fade in and out over the first and final 5 s. The waveforms and frequency characteristics of all the stimuli are shown in Figure 1. The envelope characteristics of the stimulus were determined by first performing a Hilbert transformation on the signal, then calculating its absolute value, followed by a Fourier transformation. The music data are presented in Supplementary Data 1. During the experiment, all the stimuli were played as monaural sound sources in the first channel of the audio file. Additionally, an impulse signal synchronized with the gamma oscillation of the sound source was included every second as trigger information in the second channel. While the control music did not include a strong power at or around the 40 Hz frequency range, it was provided with trigger information at the same temporal timing as with the gamma music. This method was used for the PLI analysis, as described later in the study. The monaural sound source in the first channel was transformed into stereo and then delivered through headphones to both ears.
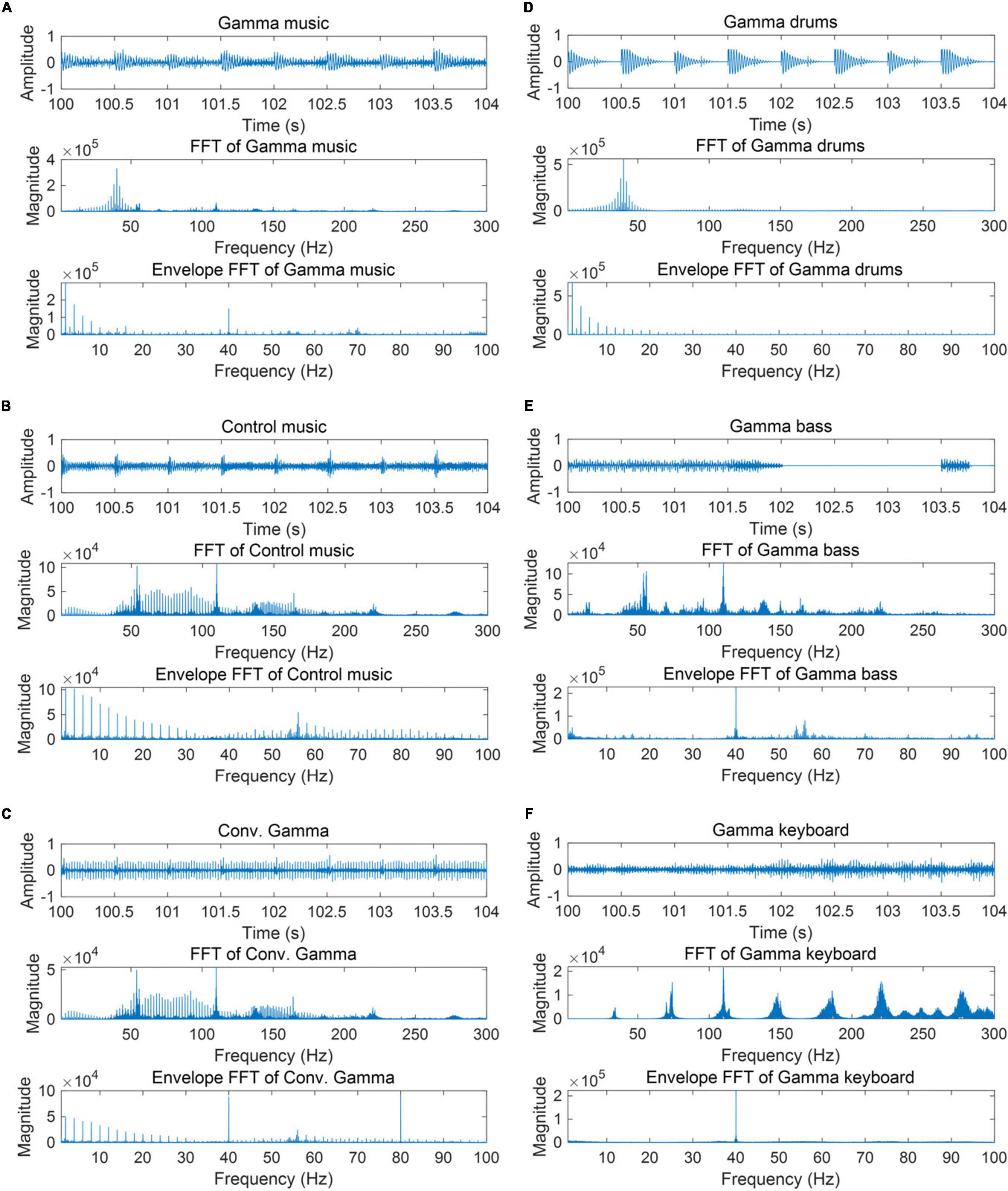
Figure 1. Partial waveform of each stimulus, the frequency response, and the frequency response of the auditory envelope. (A) Gamma music (B) control music (C) control music with conventional gamma stimulation (Conv. Gamma) (D) gamma drums (E) gamma bass (F) gamma keyboard.
2.3 Experimental procedure
We presented six stimuli to the participants in random order. Participants were asked to relax their bodies and listen to music without any body movements. Participants were instructed to look at a fixation point displayed on the monitor in front of them to avoid unnecessary eye movements during the music presentation. After each music presentation, the participants self-assessed their mental relaxation and absorption levels (Rainvilles et al., 2002; Orozco Perez et al., 2020), as well as their valence and arousal levels (Russell, 1980). Furthermore, participants responded to several subjective rating items regarding the previously presented music. Table 1 presents all questionnaire items. All responses were measured using a VAS. After all recordings, the participants completed a self-report inventory using the Goldsmiths Musical Sophistication Index (GMSI) to assess individual differences in musical sophistication (Müllensiefen et al., 2014; Sadakata et al., 2022) and the Barcelona Music Reward Questionnaire (BMRQ) to assess the global sensitivity of the participants to music rewards (Mas-Herrero et al., 2013). All the subjective evaluations were conducted using the PsychoPy package (Peirce et al., 2019).
2.4 Experimental devices
Electroencephalogram responses were recorded using a portable EEG device (Miyuki Giken, Bunkyô City, Tokyo, Japan; Polymate Mini AP108) with active electrodes placed at the forehead, FC3, FCz, and FC4, in accordance with the International 10–20 system. Generally, ASSR is strongly observed through electrodes spanning from the frontal to the central regions (Picton et al., 2003; Griskova et al., 2007). Additionally, Yokota et al. (2017) have reported that variations in ASSR activity can be captured even with a few electrodes in the fronto-central regions. To obtain electrooculograms (EOGs), two electrodes were affixed to the superior and lateral aspects of each participant’s right eye. Moreover, to verify whether the components of the electrocardiogram (ECG) intermixed with those from the EEG electrodes, an electrode was affixed to the participant’s chest for ECG recording. All captured signals were referenced to the right mastoid, and the ground electrode was placed on the left. All electrode impedances were reduced to less than 50 kΩ. All the signals were sampled at 500 Hz.
All acoustic sources were produced via a USB audio interface (TASCAM, Santa Fe Springs, CA, USA; US-4 × 4HR) and transmitted to the participants using headphones (Sony Corporation, Minato City, Tokyo, Japan; MDR-CD900ST). The delivery of all sounds was facilitated using a VLC media player (VideoLAN Organization, Paris, France).
2.5 Data analysis
Data analyses were conducted using MATLAB 2023 a and R Studio version 2023.03.1. Build 446 (R Foundation for Statistical Computing, Vienna, Austria). Continuous EEG, EOGs, and ECG signals were digitally filtered using a finite-impulse response bandpass filter (1–50 Hz, order: 1500). Artifacts associated with eye movements were removed from the EEG data using independent component analysis.
We conducted a Fourier analysis of the continuous EEG data to convert it into the frequency domain for power analysis. Therefore, in the power analysis, continuous EEG data was not segmented into epochs based on auditory triggers. To improve the performance of fft function, power was calculated as the square of the amplitude normalized using a factor of N, where N is the next power of two from the continuous EEG data. In this study, N was 131072 for all the experimental data. Subsequently, the power was transformed into decibel units using the calculation formula 20log10(power). Power was computed individually for each participant at each electrode and subsequently averaged across the forehead and fronto-central (FC3, FCz, and FC4) channel locations. Finally, the grand-average power was calculated by averaging the power values of the participants.
For PLI analysis, the continuous EEG data for each channel were segmented into 1000 ms epochs based on the auditory trigger that occurred every second in the continuous external input data. Therefore, there was no overlap in the data segmentation. Epochs exceeding ± 40 μV on the forehead, FC3, FCz, and FC4 channels were removed from the analysis. A Fourier analysis was performed to convert the epochs into the frequency domain. PLI was calculated using the following formula:
where F is the Fourier response, f is the frequency, c is the number of channels, k is the trial number and n is the number of trials. PLI was computed individually for each participant at each electrode and subsequently averaged across the forehead and fronto-central (FC3, FCz, and FC4) channel locations. Finally, the grand-average PLI was calculated by averaging the PLI values across participants.
2.6 Statistical analysis
We used the Friedman test for subjective ratings. The factor under consideration was the stimulus, which was characterized by six auditory stimuli. Subsequent post-hoc tests were conducted using the Wilcoxon signed-rank test. The Holm method was used to correct the p-values for multiple comparisons.
For the 40 Hz power and PLI data, we employed a linear mixed-effects model (LME) for statistical analysis. The stimuli (six auditory stimuli) and channel location (forehead or fronto-central) were defined as fixed effects. Participants were defined as having a random intercept. Our LMM model for Wilkinson notation was 40 Hz power or PLI values ∼ stimuli * channel location + (1 | Participant). The PLI values were subjected to a logit transformation before being applied to the LME. We performed LME analysis using the lme4 and lmerTest packages in the R. A type III ANOVA test with the Kenward–Roger method was used to determine the significance of the fixed effects. For post-hoc tests, multiple comparisons were performed based on the fitted LME using the Kenward–Roger method. Tukey’s method was used to correct the p-values for multiple comparisons. Post-hoc tests for the 40 Hz power and PLI were performed using emmeans package in the R.
Finally, we examined the potential impact of individual differences in musical sophistication and global sensitivity to music rewards on the magnitude of ASSR induced by sound stimuli. Specifically, we examined the correlation between individual GMSI and BMRQ scores and the power and PLI of the ASSR in the fronto-central region.
3 Results
3.1 Subjective ratings
The subjective ratings of the auditory stimuli are shown in Figure 2. The Friedman tests revealed significant differences in the stimuli for relaxation (χ2 = 24.8; p < 0.00015; η2 = 0.29), valence (χ2 = 20.1; p = 0.0012; η2 = 0.24), preference (χ2 = 12.9; p < 0.024; η2 = 0.15), pleasantness (χ2 = 21.0; p = 0.0008; η2 = 0.26), and strangeness (χ2 = 23.4; p < 0.0003; η2 = 0.29). However, no significant differences were found in the stimuli for absorption (χ2 = 2.45; p = 0.78; η2 = 0.029) and arousal (χ2 = 8.50; p < 0.13; η2 = 0.10).
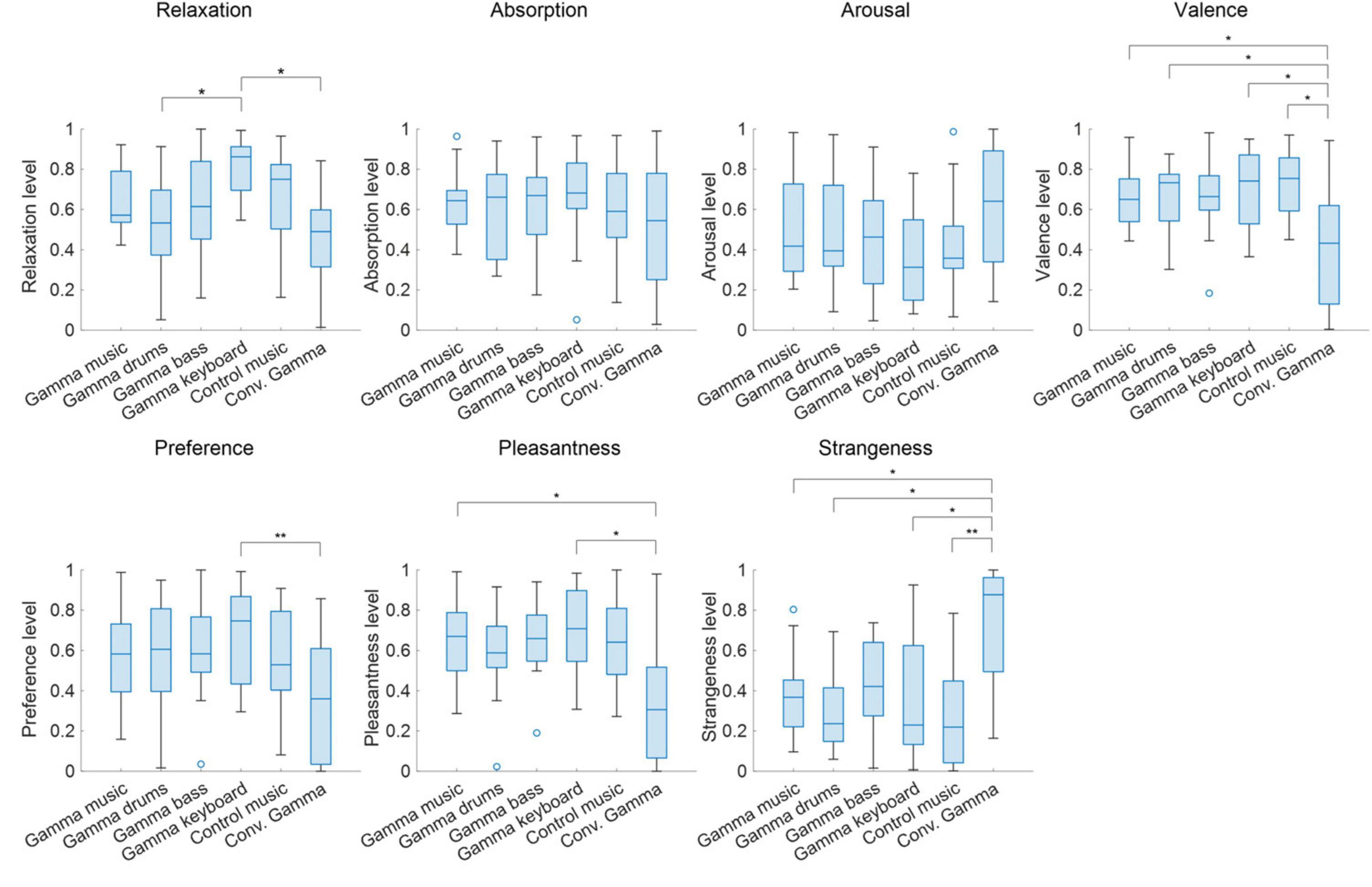
Figure 2. Box chart of subjective ratings for each of the sound stimuli. Values closer to 1 represent states of being relaxed, absorbed, awake, comfortable, preferred, pleasant, and strange, respectively, while values closer to 0 represent states of being excited, not absorbed, sleepy, uncomfortable, not preferred, unpleasant, and not strange. **p < 0.01, *p < 0.05.
Subsequent multiple comparison tests of relaxation revealed a significant difference, such that the gamma keyboard sound was associated with a relaxed rating compared to the gamma drums sound (Z = 3.01; p = 0.036; r = 0.73) and the Conv. Gamma (Z = 3.95; p = 0.011; r = 0.96). Subsequent multiple comparison tests of valence revealed a significant difference, such that the Conv. Gamma was associated with uncomfortable ratings compared to the gamma music (Z = 3.27; p = 0.016; r = 0.79), the gamma drums sound (Z = 3.01; p = 0.031; r = 0.73), the gamma keyboard sound (Z = 3.27; p = 0.016; r = 0.79), and the control music alone (Z = 3.14; p = 0.022; r = 0.76). Subsequent multiple comparison tests of preference revealed a significant difference, such that the Conv. Gamma was associated with an unpreferred rating compared to the gamma keyboard sound (Z = 3.48; p = 0.0076; r = 0.84). Subsequent multiple comparison tests of pleasantness revealed a significant difference, such that the Conv. Gamma was associated with unpleasant ratings compared to the gamma music (Z = 3.00; p = 0.038; r = 0.75) and the gamma keyboard sound (Z = 3.29; p = 0.015; r = 0.82). Subsequent multiple comparison tests of strangeness revealed a significant difference, such that the Conv. Gamma was associated with strangeness ratings compared to the gamma music (Z = 3.21; p = 0.019; r = 0.80), the gamma drums sound (Z = 3.21; p = 0.018; r = 0.80), the gamma keyboard sound (Z = 3.14; p = 0.020; r = 0.79), and the control music alone (Z = 3.91; p = 0.0014; r = 0.98). These findings suggest that the participants found the Conv. Gamma to be the least unpreferred option. On the other hand, the gamma keyboard sound was the most favored, evoking sensations of a more relaxed, comfortable, preferred, pleasant, and natural impression.
The total GMSI score was 86.12 ± 17.48 (Mean ± SD), and the total BMRQ score was 84.12 ± 9.96 (Mean ± SD).
3.2 EEG analysis
The grand-averaged power and PLI for each frequency in the forehead and fronto-central regions are shown in Figures 3A, 4A. Clear peaks were observed in both the 40 Hz power and PLI within both the forehead and fronto-central regions. This indicates that the 40 Hz component included in the auditory stimuli induced ASSR. Figures 3B, 4B show box charts of the 40 Hz power and PLI for each stimulus in the forehead and fronto-central regions.
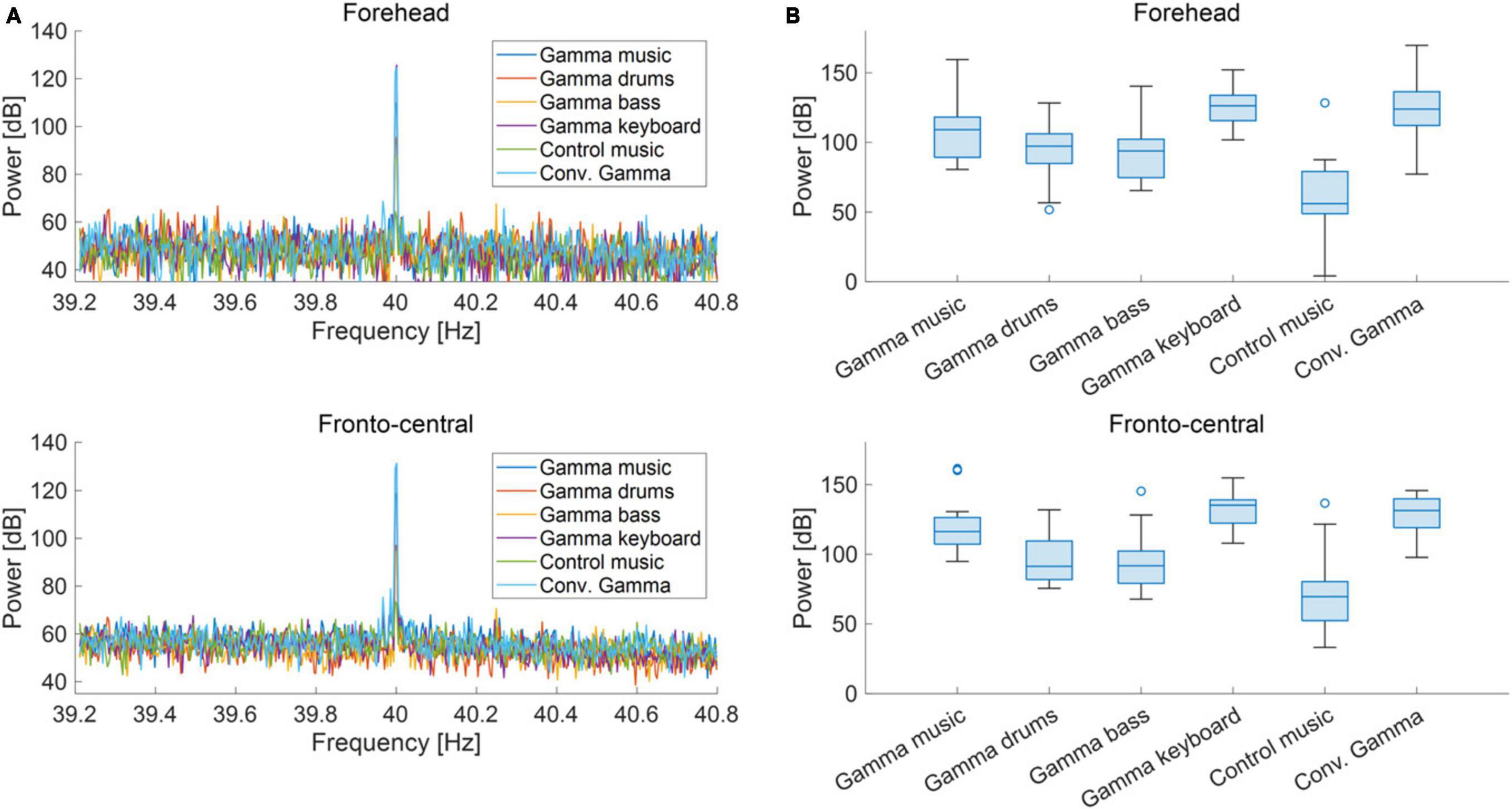
Figure 3. (A) Grand-averaged power at the forehead and fronto-central regions of each sound stimulus. (B) Box chart of 40 Hz power at the forehead and fronto-central regions of each sound stimuli.
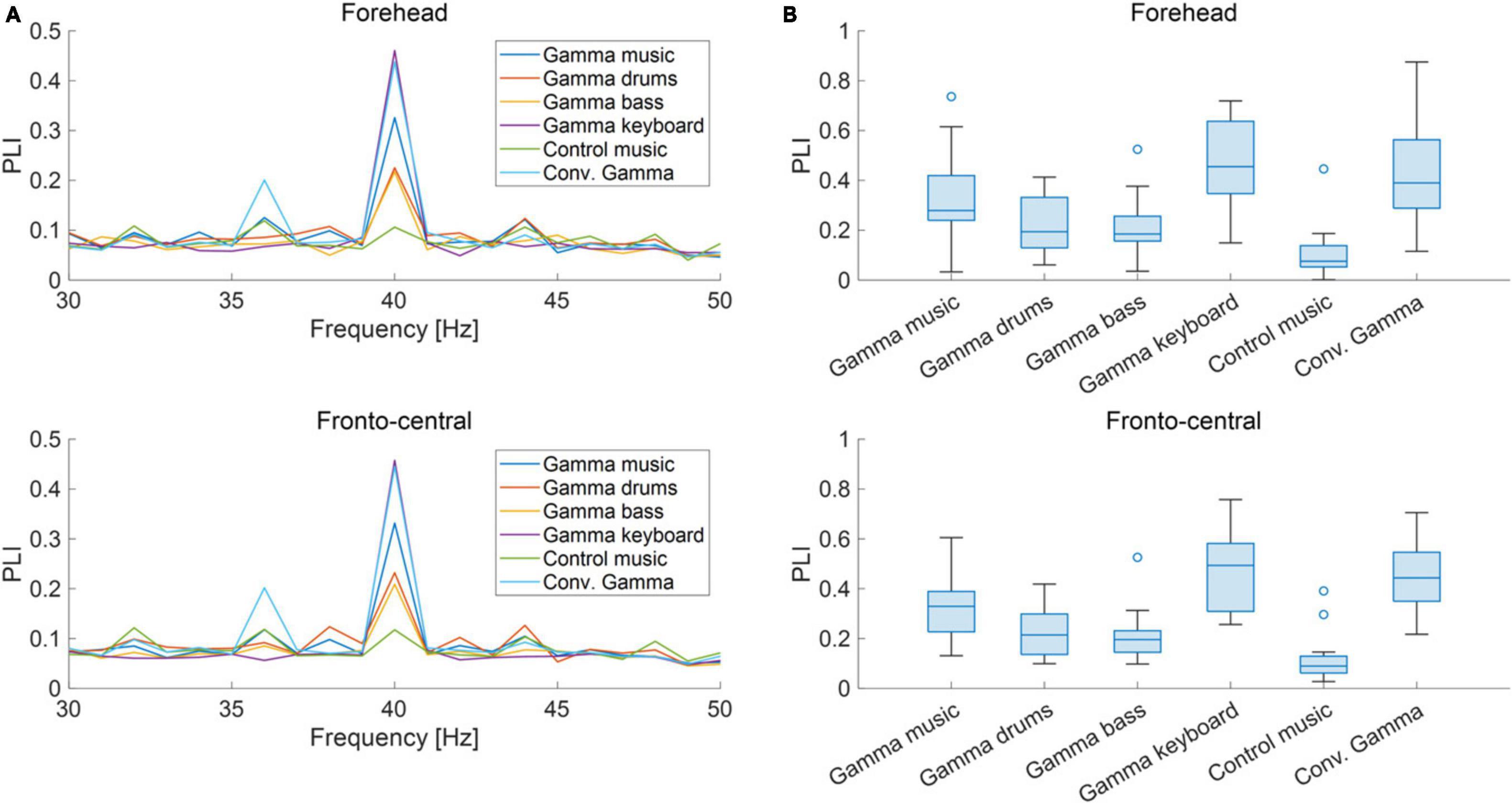
Figure 4. (A) Grand-averaged PLI at the forehead and fronto-central regions of each sound stimulus. (B) Box chart of 40 Hz PLI at the forehead and fronto-central regions of each sound stimuli.
The results of the statistical analyses for the 40 Hz power are summarized in Table 2. F-tests revealed the effects of both the stimuli [F(5, 176) = 35.3, p < 0.001] and channel location [F(5, 176) = 7.37, p = 0.0073]. The results of the statistical analyses of the 40 Hz PLI are summarized in Table 3. F-tests revealed the effects of the stimuli [F(5, 176) = 30.0, p < 0.0001]. Table 4 shows the results of the post-hoc analysis for a 40 Hz power. Table 5 shows the results of the post-hoc analysis for the 40 Hz PLI.
The correlations between individual GMSI and BMRQ scores and the power and PLI of the ASSR in the fronto-central region for each sound stimulus are shown in Figure 5. No significant correlations were observed for any of the sound stimuli or their combinations (p > 0.05).
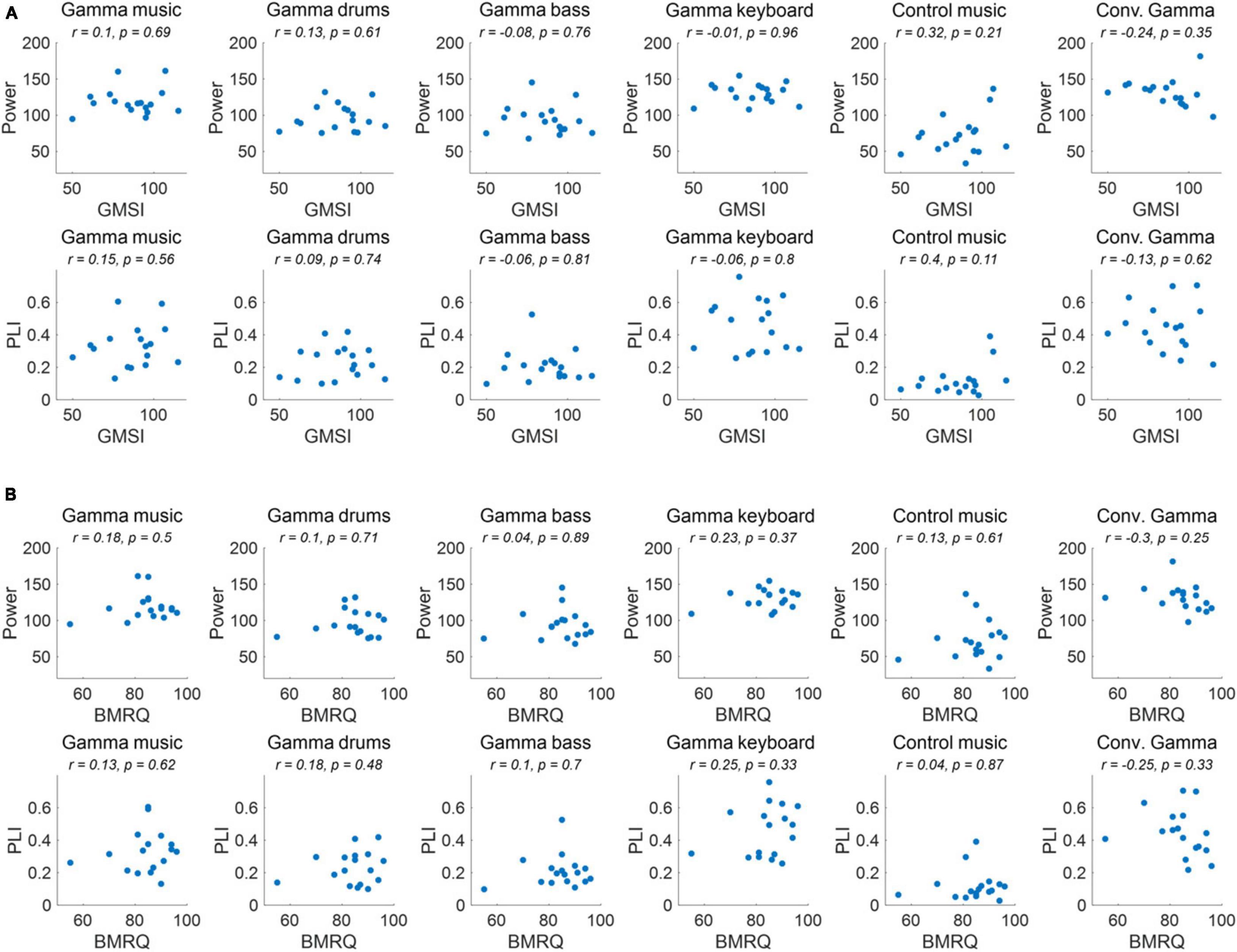
Figure 5. (A) Correlation of ASSR indicators and GMSI score for each of the sound stimuli. (B) Correlation of ASSR indicators and BMRQ score for each of the sound stimuli.
4 Discussion
We propose “gamma music” as an innovative form of gamma stimulation that combines 40 Hz auditory stimuli and music. This gamma music was composed of drums, bass, and keyboard instruments, each of which included 40 Hz frequency oscillations. We used six stimuli: gamma music, the three individual instruments composing it, control music that did not include a strong power at or around the 40 Hz frequency range, and control music with conventional typical 40 Hz tones. As 40 Hz steady stimuli induce ASSR, we used the 40 Hz ASSR power and PLI as indicators of neural activity while listing the six stimuli. We also collected subjective ratings for each music stimulus through VAS questionnaires.
As a result of the experiment, the main effect of the fixed effects for stimuli was confirmed for both 40 Hz power and PLI. Gamma music, gamma drums, gamma bass, gamma keyboard, and Conv. Gamma showed significantly higher values at both 40 Hz power and PLI than the control music alone. This indicates that gamma music and the three gamma instruments strongly induced ASSR. In particular, the gamma keyboard sound demonstrated the highest value at both 40 Hz power and PLI, indicating its potential to strongly induce ASSR. The Conv. Gamma had a simple sequence of tones repeating at 40 Hz (i.e., 1 ms long, 10 kHz tones played every 25 ms inter-tone interval), commonly used in previous research (Martorell et al., 2019), and the degree of ASSR was comparable to that of gamma music and each gamma instrument created in this study. Notably, the Conv. Gamma elicited feelings of being unrelaxed, uncomfortable, non-preferred, unpleasant, and strange feelings compared to the other stimuli. This finding aligns with the assertion of Tichko et al. (2022) that solely adding a 40 Hz auditory stimulus to existing music presents difficulties from the perspective of musical pleasure. Conversely, the subjective ratings of the gamma music sound developed in this study were comparable to those of the control music. Among the stimuli, the gamma keyboard sound garnered the highest ratings for feelings of relaxation, comfort, preference, pleasantness, and naturalness. These results indicate that gamma music, particularly gamma keyboard sound can induce a strong ASSR while preserving the comfortable and pleasant sensation of listening to music. To investigate the potential influence of individual differences in musical sophistication and global sensitivity of participants to music rewards might influence the power and PLI of the ASSRs, a correlational analysis was conducted. Despite this analysis, no statistically significant correlations emerged. Consequently, it remains inconclusive whether individual differences in musical sophistication and responsiveness to musical rewards play a substantial role in the observed variability of ASSR activity.
Gamma music has various potential applications as a new methodology for inducing ASSR. A 40 Hz auditory stimulus is often perceived as an unpleasant sound by listeners (Fastl, 1990; Tichko et al., 2022). Therefore, prolonged stimulus presentation can potentially increase the burden on listeners. This is an issue in basic research investigating ASSR because the prolonged stimulus presentation time required to induce ASSR is generally an issue. In recent years, studies have suggested that gamma stimulation in mice and humans can modulate brain cognitive function, making the importance of gamma stimuli even more pronounced (Iaccarino et al., 2016; Martorell et al., 2019; Chan et al., 2022). Our gamma musical composition has the potential to resolve the discomfort experienced by participants during ASSR and clinical gamma interventions.
Our gamma music renders gamma stimulation a pleasant and comfortable experience for the participants. This holds significant value, especially if the ASSR can be reliably extracted, even when simple measurement methods are used. In this study, we measured EEG signals in both the fronto-central region, where the ASSR is typically strongly measured, and the forehead, where measurement is possible even with disposable electrodes with little interference from hair. In terms of 40 Hz power, significant main effects were observed for the fixed effects of the channel location, revealing higher power in the fronto-central region compared to the forehead. However, no significant main effect of the fixed effect on channel location was confirmed in the PLI. Therefore, while it is preferable to measure the fronto-central region when using 40 Hz power as an indicator of ASSR, it is adequate to solely measure the PLI values in the forehead when measuring the magnitude of ASSR induced by gamma music. In a previous study, PLI was confirmed to be a robust indicator, even against motion noise (Yokota et al., 2017), effectively reflecting the magnitude of the ASSR. Although EEG measurement in the fronto-central region is the most reliable method for evaluating the magnitude of ASSR, the measurement of PLI at the forehead using gamma music would be beneficial as a more straightforward and more comfortable method of gamma stimulation.
Why did the gamma keyboard elicit the largest ASSR among the three stimuli: gamma drums, gamma bass, and gamma keyboard? In Figure 1, there seems to be no difference in the frequency responses or the auditory envelopes among the stimuli. For example, the magnitudes of the 40 Hz frequency responses in the auditory envelopes of both the gamma bass and gamma keyboard appear to be equivalent. These stimuli did not include strong power at or around 80 Hz, a harmonic of 40 Hz, suggesting that frequency responses other than 40 Hz were unlikely to have influenced the 40 Hz ASSR. However, when focusing on the musical characteristics, it was noticeable that the gamma keyboard had long, drawn-out sounds with little silence. In contrast, the gamma drums and gamma bass had intermittent silent sections, creating a sparser soundscape. These silent parts could have contributed to the reduction in the ASSR for the gamma drums and gamma bass.
Why do gamma keyboard sounds elicit more significant power and PLI than gamma music? Gamma music, a composite of gamma drums, bass, and keyboard sounds, exhibited the second greatest power and PLI, with larger values than gamma drums and gamma bass sounds. There are two plausible explanations for these outcomes. The first is the attenuation of the loudness of gamma keyboard sounds. Gamma music contains a gamma keyboard sound. However, its loudness is lower than that of gamma keyboard sound alone. This discrepancy arises from the perceptual loudness that was adjusted throughout the experimental stimuli. Consequently, the loudness of each musical instrument sound included in the gamma music was quieter than that of each sound alone. While the gamma keyboard sound induced the largest ASSR power and PLI, the gamma drums and bass sounds induced a larger ASSR than the control music; however, the degree of these responses was relatively small. In gamma music, the loudness of the gamma drums and bass sounds becomes even smaller, resulting in a smaller ASSR than the gamma keyboard sound alone. The second possibility is that the time responses to the 40 Hz components of each instrument may vary, leading to the cancellation of the phases of each 40 Hz component. ASSR is a phenomenon in which brain activity settles into a steady-state owing to the convolution of a specific stimulus frequency. Therefore, when different stimuli are presented simultaneously, the neural responses to each stimulus may influence the others, resulting in a weaker ASSR. Therefore, even with stimuli at the same frequency, when multiple sensory stimulus presentations are used, it may be necessary to present stimuli and analyze the signals while considering their mutual influence.
In this study, we demonstrated that gamma music serves as an effective acoustic source for inducing a robust 40 Hz ASSR. Our gamma music is considered to reduce the physical and psychological burden on participants in the measurement of ASSR compared to existing conventional 40 Hz stimuli, potentially accelerating fundamental research in ASSR. In the future, rather than focusing solely on 40 Hz, we intend to identify the individual gamma frequency (IGF) that elicits the most substantial ASSR response for each individual, develop personalized gamma music based on these frequencies, and verify its efficacy. Cortical phase-locked activity in the gamma range varies significantly across individuals (Zaehle et al., 2010; Gransier et al., 2021). The ASSR to IGF is reported to correlate more robustly with individual cognitive indices than with the 40 Hz ASSR (Griškova-Bulanova et al., 2022). Consequently, the response to IGF may more accurately reflect the state of neural networks associated with information processing. Stimulating the IGF with gamma music may pave the way for more precise measurements of an individual’s ASSR and foster its application as an effective biomarker for cognitive evaluation.
5 Conclusion
This study proposes a new form of gamma stimulation called gamma music, incorporating 40 Hz auditory stimuli into drums, bass, and keyboard instrument sounds. We showed that gamma music and each of the constituting gamma-sound instruments induced significantly higher values in 40 Hz ASSR power and PLI compared to control music that did not contain apparent 40 Hz frequency power. Notably, while the Conv. Gamma induced feelings of being unrelaxed, uncomfortable, non-preferred, unpleasant, and strange, gamma music, especially the gamma keyboard sound induced feelings of being relaxed, comfortable, preferred, pleasant, and natural in listeners. These results suggest that gamma music is a promising new method for inducing ASSR. In particular, the fact that our gamma sounds are pleasant to the human ear is beneficial for the long-term use of gamma stimulation. This music is expected to contribute to neuroscience research using ASSR and can be applied to gamma music-based interventions to enhance human cognitive functions.
Data availability statement
The datasets presented in this article are not readily available because part of this data cannot be shared without the participants’ consent. Requests to access the datasets should be directed to YY, yokota@vie.style.
Ethics statement
The studies involving humans were approved by the Shiba Palace Clinic Ethics Review Committee. The studies were conducted in accordance with the local legislation and institutional requirements. The participants provided their written informed consent to participate in this study.
Author contributions
YY: Data curation, Formal analysis, Investigation, Methodology, Resources, Software, Validation, Visualization, Writing – original draft, Writing – review and editing. KT: Resources, Software, Writing – review and editing. MC: Investigation, Software, Validation, Writing – review and editing. YN: Conceptualization, Methodology, Project administration, Supervision, Writing – review and editing. YI: Conceptualization, Funding acquisition, Methodology, Project administration, Supervision, Writing – review and editing. SF: Conceptualization, Investigation, Methodology, Project administration, Resources, Software, Supervision, Validation, Visualization, Writing – original draft, Writing – review and editing.
Funding
The author(s) declare financial support was received for the research, authorship, and/or publication of this article. This study was financially supported by Vie, Inc. (https://www.viestyle.co.jp/en/) in the form of salary received by YY, KT, MC, YN, YI, and SF. The specific roles of these authors are articulated in the “Author contributions” section.
Acknowledgments
We would like to extend their gratitude to Keigo Yoshida and Taishin Emura for managing the recruitment of participants. We would like to thank Editage (www.editage.jp) for English language editing. This study has been pre-published on bioRxiv as a pre-print (Yokota et al., 2023).
Conflict of interest
The authors have read the journal’s policy and have the following competing interests: YY, KT, MC, YN, YI, and SF associated with this research are employed by Vie, Inc. The authors would like to declare the following patents associated with this research: PCT/JP2023/028596. The authors would like to declare the following products in development associated with this research: VIE Tunes and VIE Tunes Pro. This does not alter our adherence to Frontiers policies on sharing data and materials.
Publisher’s note
All claims expressed in this article are solely those of the authors and do not necessarily represent those of their affiliated organizations, or those of the publisher, the editors and the reviewers. Any product that may be evaluated in this article, or claim that may be made by its manufacturer, is not guaranteed or endorsed by the publisher.
Supplementary material
The Supplementary Material for this article can be found online at: https://www.frontiersin.org/articles/10.3389/fnhum.2023.1287018/full#supplementary-material
References
Adaikkan, C., Middleton, S. J., Marco, A., Pao, P. C., Mathys, H., Kim, D. N. W., et al. (2019). Gamma entrainment binds higher-order brain regions and offers neuroprotection. Neuron 102, 929.e8–943.e8. doi: 10.1016/j.neuron.2019.04.011
Başar, E., Emek-Savaş, D. D., Güntekin, B., and Yener, G. G. (2016). Delay of cognitive gamma responses in Alzheimer’s disease. NeuroImage Clin. 11, 106–115. doi: 10.1016/j.nicl.2016.01.015
Chan, D., Suk, H. J., Jackson, B. L., Milman, N. P., Stark, D., Klerman, E. B., et al. (2022). Gamma frequency sensory stimulation in mild probable Alzheimer’s dementia patients: Results of feasibility and pilot studies. PLoS One 17:e0278412. doi: 10.1371/journal.pone.0278412
Fastl, H. (1990). The hearing sensation roughness and neuronal responses to AM-tones. Hear. Res. 46, 293–295. doi: 10.1016/0378-5955(90)90011-D
Fries, P., Nikolić, D., and Singer, W. (2007). The gamma cycle. Trends. Neurosci. 30, 309–316. doi: 10.1016/j.tins.2007.05.005
Galambos, R., Makeig, S., and Talmachoff, P. J. (1981). A 40-Hz auditory potential recorded from the human scalp. Proc. Natl. Acad. Sci. U.S.A. 78, 2643–2647. doi: 10.1073/pnas.78.4.2643
Gander, P. E., Bosnyak, D. J., and Roberts, L. E. (2010). Evidence for modality-specific but not frequency-specific modulation of human primary auditory cortex by attention. Hear. Res. 268, 213–226. doi: 10.1016/j.heares.2010.06.003
Górska, U., and Binder, M. (2019). Low and medium frequency auditory steady-state responses decrease during NREM sleep. Int. J. Psychophysiol. 135, 44–54. doi: 10.1016/j.ijpsycho.2018.11.003
Gransier, R., Hofmann, M., van Wieringen, A., and Wouters, J. (2021). Stimulus-evoked phase-locked activity along the human auditory pathway strongly varies across individuals. Sci. Rep. 11:143. doi: 10.1038/s41598-020-80229-w
Griffiths, B. J., Parish, G., Roux, F., Michelmann, S., van der Plas, M., Kolibius, L. D., et al. (2019). Directional coupling of slow and fast hippocampal gamma with neocortical alpha/beta oscillations in human episodic memory. Proc. Natl. Acad. Sci. U.S.A. 116, 21834–21842. doi: 10.1073/pnas.1914180116
Griskova, I., Morup, M., Parnas, J., Ruksenas, O., and Arnfred, S. M. (2007). The amplitude and phase precision of 40 Hz auditory steady-state response depend on the level of arousal. Exp. Brain. Res. 183, 133–138. doi: 10.1007/s00221-007-1111-0
Griškova-Bulanova, I., Živanović, M., Voicikas, A., Pipinis, E., Jurkuvënas, V., and Bjekić, J. (2022). Responses at individual gamma frequencies are related to the processing speed but not the inhibitory control. J. Pers. Med. 13:26. doi: 10.3390/jpm13010026
Gruber, T., Tsivilis, D., Montaldi, D., and Müller, M. M. (2004). Induced gamma band responses: An early marker of memory encoding and retrieval. NeuroReport 15, 1837–1841. doi: 10.1097/01.wnr.0000137077.26010.12
Herrmann, C. S., and Demiralp, T. (2005). Human EEG gamma oscillations in neuropsychiatric disorders. Clin. Neurophysiol. 116, 2719–2733. doi: 10.1016/j.clinph.2005.07.007
Herrmann, C. S., Munk, M. H. J., and Engel, A. K. (2004). Cognitive functions of gamma-band activity: Memory match and utilization. Trends. Cogn. Sci. 8, 347–355. doi: 10.1016/j.tics.2004.06.006
Howard, M. W., Rizzuto, D. S., Caplan, J. B., Madsen, J. R., Lisman, J., Aschenbrenner-Scheibe, R., et al. (2003). Gamma oscillations correlate with working memory load in humans. Cereb. Cortex 13, 1369–1374. doi: 10.1093/cercor/bhg084
Iaccarino, H. F., Singer, A. C., Martorell, A. J., Rudenko, A., Gao, F., Gillingham, T. Z., et al. (2016). Gamma frequency entrainment attenuates amyloid load and modifies microglia. Nature 540, 230–235. doi: 10.1038/nature20587
Jensen, O., and Lisman, J. E. (2005). Hippocampal sequence-encoding driven by a cortical multi-item working memory buffer. Trends. Neurosci. 28, 67–72. doi: 10.1016/j.tins.2004.12.001
Koelsch, S. (2014). Brain correlates of music-evoked emotions. Nat. Rev. Neurosci. 15, 170–180. doi: 10.1038/nrn3666
Koenig, T., Prichep, L., Dierks, T., Hubl, D., Wahlund, L. O., John, E. R., et al. (2005). Decreased EEG synchronization in Alzheimer’s disease and mild cognitive impairment. Neurobiol. Aging. 26, 165–171. doi: 10.1016/j.neurobiolaging.2004.03.008
Kucewicz, M. T., Berry, B. M., Kremen, V., Brinkmann, B. H., Sperling, M. R., Jobst, B. C., et al. (2017). Dissecting gamma frequency activity during human memory processing. Brain 140, 1337–1350. doi: 10.1093/brain/awx043
Kwon, J. S., O’donnell, B. F., Wallenstein, G. V., Greene, R. W., Hirayasu, Y., Nestor, P. G., et al. (1999). Gamma frequency-range abnormalities to auditory stimulation in schizophrenia. Arch. Gen. Psychiatry 56, 1001–1005.
Lisman, J. (2010). Working memory: The importance of theta and gamma oscillations. Curr. Biol. 20, R490–R492. doi: 10.1016/j.cub.2010.04.011
Martorell, A. J., Paulson, A. L., Suk, H. J., Abdurrob, F., Drummond, G. T., Guan, W., et al. (2019). Multi-sensory gamma stimulation ameliorates Alzheimer’s-associated pathology and improves cognition. Cell 177, 256.e22–271.e22. doi: 10.1016/j.cell.2019.02.014
Mas-Herrero, E., Marco-Pallares, J., Lorenzo-Seva, U., Zatorre, R. J., and Rodriguez-Fornells, A. (2013). Individual differences in music reward experiences. Music Percept. 31, 118–138. doi: 10.1525/mp.2013.31.2.118
Mathalon, D. H., and Sohal, V. S. (2015). Neural oscillations and synchrony in brain dysfunction and neuropsychiatric disorders it’s about time. JAMA Psychiatry 72, 840–844. doi: 10.1001/jamapsychiatry.2015.0483
Mormann, F., Fell, J., Axmacher, N., Weber, B., Lehnertz, K., Elger, C. E., et al. (2005). Phase/amplitude reset and theta-gamma interaction in the human medial temporal lobe during a continuous word recognition memory task. Hippocampus 15, 890–900. doi: 10.1002/hipo.20117
Müllensiefen, D., Gingras, B., Musil, J., and Stewart, L. (2014). The musicality of non-musicians: An index for assessing musical sophistication in the general population. PLoS One 9:e0089642. doi: 10.1371/journal.pone.0089642
O’Donnell, B. F., Vohs, J. L., Krishnan, G. P., Rass, O., Hetrick, W. P., and Morzorati, S. L. (2013). The auditory steady-state response (ASSR): A translational biomarker for schizophrenia. Suppl. Clin. Neurophysiol. 62, 101–112. doi: 10.1016/B978-0-7020-5307-8.00006-5
Orozco Perez, H. D., Dumas, G., and Lehmann, A. (2020). Binaural beats through the auditory pathway: From brainstem to connectivity patterns. eNeuro 7:ENEURO.0232-19.2020. doi: 10.1523/ENEURO.0232-19.2020
Osipova, D., Takashima, A., Oostenveld, R., Fernández, G., Maris, E., and Jensen, O. (2006). Theta and gamma oscillations predict encoding and retrieval of declarative memory. J. Neurosci. 26, 7523–7531. doi: 10.1523/JNEUROSCI.1948-06.2006
Parciauskaite, V., Bjekic, J., Griskova-Bulanova, I., and Rubio, M. E. (2021). Gamma-range auditory steady-state responses and cognitive performance: A systematic review. Brain Sci. 11:217. doi: 10.3390/brainsci
Peirce, J., Gray, J. R., Simpson, S., MacAskill, M., Höchenberger, R., Sogo, H., et al. (2019). PsychoPy2: Experiments in behavior made easy. Behav. Res. Methods 51, 195–203. doi: 10.3758/s13428-018-01193-y
Picton, T. W., John, M. S., Dimitrijevic, A., and Purcell, D. (2003). Human auditory steady-state responses. Int. J. Audiol. 42, 177–219. doi: 10.3109/14992020309101316
Rainville, P., Hofbauer, R. K., Bushnell, M. C., Duncan, G. H., and Price, D. D. (2002). Hypnosis modulates activity in brain structures involved in the regulation of consciousness. J. Cogn. Neurosci. 14, 887–901. doi: 10.1162/089892902760191117
Ross, B., Borgmann, C., Draganova, R., Roberts, L. E., and Pantev, C. (2000). A high-precision magnetoencephalographic study of human auditory steady-state responses to amplitude-modulated tones. J. Acoust. Soc. Am. 108, 679–691. doi: 10.1121/1.429600
Roux, F., Wibral, M., Mohr, H. M., Singer, W., and Uhlhaas, P. J. (2012). Gamma-band activity in human prefrontal cortex codes for the number of relevant items maintained in working memory. J. Neurosci. 32, 12411–12420. doi: 10.1523/JNEUROSCI.0421-12.2012
Russell, J. A. (1980). A circumplex model of affect. J. Pers. Soc. Psychol. 39, 1161–1178. doi: 10.1037/h0077714
Sadakata, M., Yamaguchi, Y., Ohsawa, C., Matsubara, M., Terasawa, H., von Schnehen, A., et al. (2022). The Japanese translation of the Gold-MSI: Adaptation and validation of the self-report questionnaire of musical sophistication. Mus. Sci. 27, 798–810. doi: 10.1177/10298649221110089
Shin, Y. W., O’Donnell, B. F., Youn, S., and Kwon, J. S. (2011). Gamma oscillation in schizophrenia. Psychiatry Investig. 8, 288–296. doi: 10.4306/pi.2011.8.4.288
Shirvalkar, P. R., Rapp, P. R., and Shapiro, M. L. (2010). Bidirectional changes to hippocampal theta-gamma comodulation predict memory for recent spatial episodes. Proc. Natl. Acad. Sci. U.S.A. 107, 7054–7059. doi: 10.1073/pnas.0911184107
Skosnik, P. D., Krishnan, G. P., and O’Donnell, B. F. (2007). The effect of selective attention on the gamma-band auditory steady-state response. Neurosci. Lett. 420, 223–228. doi: 10.1016/j.neulet.2007.04.072
Stam, C. J., van Cappellen van Walsum, A. M., Pijnenburg, Y. A. L., Berendse, H. W., de Munck, J. C., Scheltens, P., et al. (2002). Generalized synchronization of MEG recordings in Alzheimer’s disease: Evidence for involvement of the gamma band. J. Clin. Neurophysiol. 19, 562–574. doi: 10.1097/00004691-200212000-00010
Tallon-Baudry, C., Bertrand, O., Peronnet, F., and Pernier, J. (1998). Induced γ-band activity during the delay of a visual short-term memory task in humans. J. Neurosci. 18, 4244–4254. doi: 10.1523/JNEUROSCI.18-11-04244.1998
Thuné, H., Recasens, M., and Uhlhaas, P. J. (2016). The 40-Hz auditory steady-state response in patients with schizophrenia a meta-Analysis. JAMA Psychiatry 73, 1145–1153. doi: 10.1001/jamapsychiatry.2016.2619
Tichko, P., Kim, J. C., Large, E., and Loui, P. (2022). Integrating music-based interventions with Gamma-frequency stimulation: Implications for healthy ageing. Eur. J. Neurosci. 55, 3303–3323. doi: 10.1111/ejn.15059
Uhlhaas, P. J., Linden, D. E. J., Singer, W., Haenschel, C., Lindner, M., Maurer, K., et al. (2006). Dysfunctional long-range coordination of neural activity during gestalt perception in schizophrenia. J. Neurosci. 26, 8168–8175. doi: 10.1523/JNEUROSCI.2002-06.2006
van der Steen, J. T., van Soest-Poortvliet, M. C., van der Wouden, J. C., Bruinsma, M. S., Scholten, R. J., and Vink, A. C. (2018). Music-based therapeutic interventions for people with dementia. Cochrane Database of Syst. Rev. 5:CD003477. doi: 10.1002/14651858.CD003477.pub3
Van Vugt, M. K., Schulze-Bonhage, A., Litt, B., Brandt, A., and Kahana, M. J. (2010). Hippocampal gamma oscillations increase with memory load. J. Neurosci. 30, 2694–2699. doi: 10.1523/JNEUROSCI.0567-09.2010
Voicikas, A., Niciute, I., Ruksenas, O., and Griskova-Bulanova, I. (2016). Effect of attention on 40 Hz auditory steady-state response depends on the stimulation type: Flutter amplitude modulated tones versus clicks. Neurosci. Lett. 629, 215–220. doi: 10.1016/j.neulet.2016.07.019
Vuust, P., Heggli, O. A., Friston, K. J., and Kringelbach, M. L. (2022). Music in the brain. Nat. Rev. Neurosci. 23, 287–305. doi: 10.1038/s41583-022-00578-5
Yokota, Y., and Naruse, Y. (2015). Phase coherence of auditory steady-state response reflects the amount of cognitive workload in a modified N-back task. Neurosci. Res. 100, 39–45. doi: 10.1016/j.neures.2015.06.010
Yokota, Y., Tanaka, K., Chang, M., Naruse, Y., Imamura, Y., and Fujii, S. (2023). Gamma music: A new acoustic stimulus for gamma-frequency auditory steady-state response. bioRxiv [Preprint]. doi: 10.1101/2023.08.17.552385
Yokota, Y., Tanaka, S., Miyamoto, A., and Naruse, Y. (2017). Estimation of human workload from the auditory steady-state response recorded via a wearable electroencephalography system during walking. Front. Hum. Neurosci. 11:314. doi: 10.3389/fnhum.2017.00314
Zaehle, T., Lenz, D., Ohl, F. W., and Herrmann, C. S. (2010). Resonance phenomena in the human auditory cortex: Individual resonance frequencies of the cerebral cortex determine electrophysiological responses. Exp. Brain Res. 203, 629–635. doi: 10.1007/s00221-010-2265-8
Keywords: electroencephalogram, auditory steady-state response, gamma stimulation, music, gamma music
Citation: Yokota Y, Tanaka K, Chang M, Naruse Y, Imamura Y and Fujii S (2024) Gamma music: a new acoustic stimulus for gamma-frequency auditory steady-state response. Front. Hum. Neurosci. 17:1287018. doi: 10.3389/fnhum.2023.1287018
Received: 01 September 2023; Accepted: 18 December 2023;
Published: 11 January 2024.
Edited by:
Cunmei Jiang, Shanghai Normal University, ChinaReviewed by:
Theodore Zanto, University of California, San Francisco, United StatesFelipe Antunes, Federal Institute of Minas Gerais, Brazil
Jie Chen, Hunan Normal University, China
Copyright © 2024 Yokota, Tanaka, Chang, Naruse, Imamura and Fujii. This is an open-access article distributed under the terms of the Creative Commons Attribution License (CC BY). The use, distribution or reproduction in other forums is permitted, provided the original author(s) and the copyright owner(s) are credited and that the original publication in this journal is cited, in accordance with accepted academic practice. No use, distribution or reproduction is permitted which does not comply with these terms.
*Correspondence: Yusuke Yokota, yokota@vie.style